- 1Organisms and Environment, School of Biosciences, Cardiff University, Cardiff, United Kingdom
- 2Centre for Research in Anthropology (CRIA – NOVA FCSH/IN2PAST) , Lisbon, Portugal
- 3Department of Anthropology, School of Social Sciences and Humanities, Universidade Nova de Lisboa, Lisbon, Portugal
- 4Centro de Investigação de Montanha (CIMO), Instituto Politécnico de Bragança, Bragança, Portugal
- 5Centre for Ecology and Conservation (CEC), University of Exeter, Penryn, United Kingdom
- 6CIBIO, Centro de Investigação em Biodiversidade e Recursos Genéticos, InBio Laboratório Associado, Universidade do Porto, Vairão, Portugal
- 7BIOPOLIS Programs in Genomics, Biodiversity and Land Planning, CIBIO, Vairão, Portugal
- 8Instituto Gulbenkian de Ciência, Oeiras, Portugal
- 9Gola Rainforest National Park, Kenema, Sierra Leone
- 10Instituto da Biodiversidade e das Áreas Protegidas (IBAP), Bissau, Guinea-Bissau
- 11Indigo Consultants.org, Bissau, Guinea-Bissau
- 12RSPB/Gola Rainforest National Park, Kenema, Sierra Leone
- 13Nature Metrics, Guildford, United Kingdom
Food distribution and abundance can affect intra- and inter-dietary variation in non-human primates, influencing feeding ecology and altering behaviour. Natural and/or human-induced actions can influence the dynamics between primates and the environment, with associated impacts on socio-ecology and demography. This relationship in anthropogenic landscapes, however, is poorly understood. Here, we use DNA metabarcoding to obtain high resolution dietary diversity data, and multivariate generalised linear models to investigate variation in the diet of this threatened primate. We characterise the diet of the western red colobus (Piliocolobus badius) in both the better preserved Gola Rainforest National Park (GRNP, Sierra Leone), and in the fragmented forests of Cantanhez National Park (CNP, Guinea-Bissau), and evaluate biological, ecological and temporal differences. Dietary plant species richness was high in both protected areas, and the type of plants consumed varied significantly across seasons, space, and time. Although we identify dependence on a few key plants, red colobus in CNP consumed a higher average number of plant taxa than in GRNP, and 11% of the diet consisted of cultivated foods (e.g. mango). This is the first time a molecular approach has been used to investigate red colobus diet, and reveal dietary flexibility in degraded forests. Predicting the consequences of dietary change on long-term population persistence, however, remains a significant knowledge gap. Nevertheless, our results provide critical information to inform targeted regional conservation planning and implementation.
1 Introduction
The feeding ecology of non-human primates (hereafter primates) is driven by a dynamic relationship between the primate itself and its environment, with responses linked to morphology and physiology (Robbins and Hohmann, 2006). Most primate species live in tropical forests, where clear seasonal variation in rainfall, temperature, photoperiod, and phenology (van Schaik et al., 1993; van Schaik and Brockman, 2005; van Schaik and Pfannes, 2005) forces adaptation to spatial and temporal variation in food resources (Ma et al., 2017). Food distribution and abundance can strongly influence primate density, population dynamics, activity budgets, and relationships between individuals (Chapman et al., 2004; van Schaik and Brockman, 2005; Marshall and Leighton, 2006). Indeed, inter- and intra-species variation in dietary profiles is commonly observed, even among neighbouring groups of the same population occupying different home ranges. Time budgets can be adjusted for feeding, resting, travelling and in social behaviour, as in the case of a red colobus (Piliocolobus kirkii) group living in a habitat rich in herbaceous plants; this low-quality plant type was a large proportion of the diet, especially when tree foods were scarce, and individuals spent less time moving, and more time grooming and in aggressive interactions, than the forest group living in an area where tree foods were more available (Siex, 2003). Primate food selection and avoidance is linked to several causal factors, but it can only be a successful strategy if the nutritional requirements of the forager can be fulfilled with sufficient energy and other nutrients from the items of choice (Oftedal, 1991). In some cases, primates can shift diets to less preferred items, or complement it with cultivated items which are palatable, energy-rich and easily digestible (Naughton-Treves et al., 1998; Hockings and McLennan, 2012).
Natural variation in vegetation composition can negatively impact some primate populations, while others can thrive in subsequent forest regeneration events (Isabirye-Basuta and Lwanga, 2008). In the Anthropocene, however, humans (Homo sapiens) are a hyperkeystone species that can exert direct or indirect ecological impacts on other keystone species across different ecosystems (Worm and Paine, 2016). These changes may occur at a faster rate, more frequently and/or at a larger scale, and little is known about primate resilience to such alterations. Varying levels of habitat disturbance suggest differential flexibility among primate species and populations, and some may be unable to adapt to fast-changing environments (Onderdonk and Chapman, 2000; Isabirye-Basuta and Lwanga, 2008; Struhsaker, 2010; Dunham, 2017). A growing evidence base on primate behavioural flexibility and adaptation to forest fragmentation and anthropogenic habitats (Henle et al., 2004) shows that not all species are in decline, and some have higher resilience than expected. Primates exhibit behavioural and dietary flexibility to the environment in various forms, but dietary adjustments are the most frequently reported in the literature (McLennan et al., 2017).
Despite dietary and behavioural flexibility, some primates are highly vulnerable to tropical forest loss, degradation and ecosystem change, particularly arboreal or forest-dependent species (Isaac and Cowlishaw, 2004). A global meta-analysis showed negative impacts of anthropogenic habitat modification on primate communities and assemblages, potentially driving drastic population declines (Almeida-Rocha et al., 2017). Recently, populations of south American primates that appeared to sustain adaptive potential and resilience in reduced and fragmented habitats, were shown to experience limitations at the population level (see examples in Strier, 2021). Individual behavioural and dietary flexibility in howler monkeys (Allouata spp.) can mask limited post-disturbance recovery capacity at the population level, such as yellow fever outbreaks linked to local extinctions (Bicca-Marques et al., 2020). These and other factors operating at the ecological and biological levels (e.g. restricted gene flow among isolated populations in fragmented forests, reduced genetic diversity and increased vulnerability to stochastic events, such as natural disasters and disease) can determine the capacity for survival in altered environments. Understanding ecological and behavioural responses to human-induced changes in primate habitats is an urgent challenge given the growing number of primate populations living in closer contact with human populations (Estrada et al., 2020).
Analysis of plant and animal items in primate diet has traditionally required direct observations of feeding behaviour, and/or macro- or microscopic identification of digested food remains from stomach content or faecal samples. These methodologies require extensive observations, long distances to follow the groups, and preferentially habituated primates (Doran-Sheehy et al., 2006). Furthermore, data quality often depends on researcher expertise, and the type of habitat inhabited by the focal primate (e.g. it is harder to observe feeding events in higher and denser canopy) (Struhsaker, 2010). Moreover, taxonomic identification of food items is challenging, as highly digested plant remains in faeces can be indistinguishable. Hence, a molecular approach using DNA metabarcoding of items ingested by primates, has the potential to provide a more accurate and exhaustive analysis of diet composition (Pompanon et al., 2012; Taberlet et al., 2012). This technique allows for rapid identification of multiple taxa in a single experiment by combining DNA taxonomy and high-throughput sequencing (Coissac et al., 2012; Pompanon et al., 2012; Ji et al., 2013). By amplifying one or more targeted DNA sequences that exhibit consistent differences between taxa and are highly variable, it is possible to identify plants and animals against taxonomic reference databases of the organisms in question. This has been a successful alternative to more traditional approaches across many taxa, elucidating the dietary diversity of elusive species, and revealing rare prey items (Ait Baamrane et al., 2012; Lyke et al., 2019; Schmack et al., 2021; Querejeta et al., 2020).
Colobinae, a subfamily of the Old-World monkey family (species of Africa and Asia), are both arboreal and an easy target for commercial and subsistence hunting (Linder et al., 2021), rendering them particularly vulnerable to habitat alterations. Colobine monkeys have a complex evolutionary history, resulting in distinct anatomy, ecology and social dynamics (Ting, 2008; Linder et al., 2021). They differ from all other cercopithecines, and generally from other primates, with a large, multi-chambered stomach, a specialised gut microbiome, enlarged salivary glands and high-cusped molar teeth (Davies and Oates, 1994). These adaptations enable exploration and subsistence on difficult to digest, low-quality food items (Oates and Nash, 2011; Fleagle, 2013). Early studies identified colobines as leaf-eating monkeys (Davies and Oates, 1994), requiring the protein and fibre content of foods. However, later research showed that colobus monkeys, including red colobus (Piliocolobus spp.), feed mainly on leaf buds, flowers or immature seeds and fruits from a diverse range of plant species, using mature leaves when preferred food is scarce (Maisels et al., 1994; Oates, 1994; Koenig and Borries, 2001). Diversity is maintained throughout the year and appears not to correlate significantly with ripe fruit availability (Conklin-Brittain et al., 1998; Wrangham et al., 1998; Chapman et al., 1999). Like other primates, Piliocolobus species supplement its diet with nutritious and easily digestible food items (Mowry et al., 1996; Chapman and Chapman, 2002). In some cases, such foods are simply other plant parts or arthropods, but in more degraded habitats, cultivated species are also consumed (Galat-Luong and Galat, 2005; van Schaik and Brockman, 2005). The ashy red colobus (tephrosceles), for example, included cultivated bean seeds in the diet (Kibaja, 2014).
Research on red colobus feeding ecology has mainly focused on east rather than west African species. The western red colobus (Piliocolobus badius) occurs from Senegal to Côte d’Ivoire and is currently listed by IUCN Red List as Endangered (McGraw et al., 2020), due to habitat loss, degradation and fragmentation associated with global and local demand for natural resources, commercial and subsistence hunting (McGraw et al., 2020), and increased exposure to predators and infectious diseases transferred from humans and domesticated animals (Galat-Luong and Galat, 2005; Hillyer et al., 2015; Düx et al., 2017). This species occurs in two National Parks: Gola rainforest in Sierra Leone (GRNP), and Cantanhez in Guinea-Bissau (CNP); despite having small populations (5,000 individuals in GRNP, Linder et al., 2021; and < 500 individuals for an effective population size in CNP, Minhós et al., 2016), the red colobus conservation action plan for 2021–2026 identifies the two protected habitats as key areas for conservation (Linder et al., 2021). No dietary studies have been conducted for either population, but in a scan sampling of habituated badius of Tiwai Island Wildlife Sanctuary, southwest of GRNP (Figure 1), nearly half of the annual diet consisted of non-foliar items: seeds, young leaves, and mature leaf parts contributed to 73% of the species diet complemented with a high intake of whole fruits and flowers (Davies et al., 1999). Gathering evidence on dietary flexibility for populations exposed to different habitat threats is therefore crucial for the long-term conservation of this species.
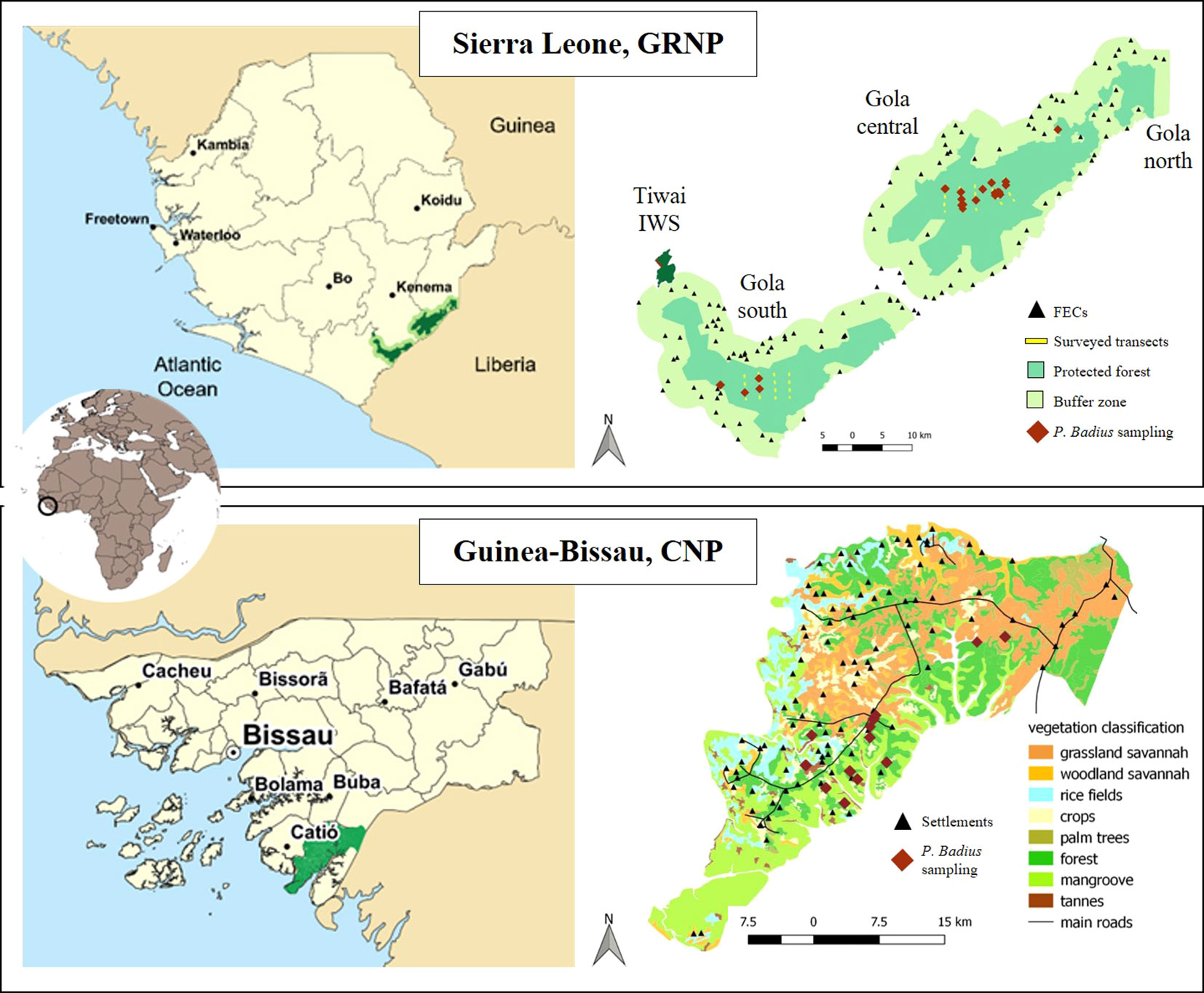
Figure 1 Maps indicating the locations of each PA in the countries, and where western red colobus non-invasive sampling took place (red diamonds). Gola Rainforest National Park (GRNP) in Sierra Leone is a protected forest composed of three forest blocks: Gola north, Gola central, and Gola south. The latter is where logging occurred more intensely across the PA. GRNP is surrounded by the community forest (buffer zone), where 122 villages (Forest Edge Communities – FECs) are located. In the southwest of the park, is the 12 km2 Tiwai Island Wildlife Sanctuary, a community conservation initiative established in 1987. Cantanhez National Park (CNP) in Guinea-Bissau has a higher degree of forest fragmentation and different vegetation types, characteristic of the CNP. The map depicts the location of all 110 villages within the national park and also the main road that crosses the PA from north to south.
In this study we aim to investigate the diet of western red colobus in two protected areas (PAs) in west Africa, with distinct anthropogenic and landscape characteristics. Using the UniPant specific primer (Moorhouse-Gann et al., 2018) to amplify the DNA of ingested plants, we can compare the diet of the red colobus population inhabiting the highly fragmented and anthropogenic landscape of CNP in Guinea-Bissau, with the population inhabiting one of the largest and well preserved west African forest, the GRNP in Sierra Leone. We investigate spatial and temporal dietary variations of western red colobus populations in each PA by i) characterising the diet and determining species richness; ii) describing local seasonal variation; iii) identifying putative sex, spatial and temporal differences; and iv) describing the species dietary flexibility in each landscape. Reflecting primate behaviour, we expect to observe intraspecific dietary variation across seasons in both PAs, but a lower plant richness, narrower niche breadth and higher presence of cultivated food items in the diet of the population inhabiting the more degraded habitat (CNP) than the more connected and protected forest of GRNP.
2 Materials and methods
2.1 Study areas
Our study focused on two PAs in west African countries, Guinea-Bissau and Sierra Leone (Figure 1), within the African wet tropical climate zone that supports some of the most biodiverse tropical forests in west Africa (White, 1983; Myers et al., 2000). Rainfall in both PAs is highest between May and November (rainy season), with a dry season from December to April. Most plant species in both PAs have one or two foliage peaks, with species-specific timings that allow young leaves to be present throughout most of the year (Oates, 1988; Bessa, 2014). Detailed information on forest types and common plants present in each PA can be found in Supplementary Tables S1, S2. Gola Rainforest National Park (GRNP), southeast Sierra Leone, comprises approximately 750 km2 of three largely intact rainforest blocks (Gola north, central and south). This PA is dominated by trees in the mature forest, with some areas of secondary forest and regrowth following historical logging exploitation and the use of the forest by militias and local communities during the civil war (1991–2002). There are no human settlements within the PA and anthropogenic activity is minimal, mainly due to heavy conservation patrol and the presence of researchers, although there are over one hundred villages with agricultural fields in the community forest (4 km wide area surrounding the protected forest). Cantanhez National Park (CNP) is a peninsula of roughly 1,000 km2 of a mosaic of sub-humid forest, secondary forest, savanna, mangrove, human settlements and agricultural fields in the southwest of Guinea-Bissau. The PA is densely populated by communities of diverse cultural groups who exploit naturally occurring trees (e.g. the palm Elaeis guineensis) and cultivated species (e.g. Anacaridum occidentale). These cash crop plantations have contributed to a small increase in vegetation cover in the park since the 1950s (Catarino and Palminha, 2018). Although flora and fauna are diverse, threats to biodiversity include deforestation from slash and burn practices, bushmeat hunting, invasive species, climate change, and reduced law enforcement and protection (Hockings and Sousa, 2013; Catarino and Palminha, 2018).
2.2 Sample collection
Between April–June and November–December 2018, we surveyed high primate density areas in GRNP (identified previously in Klop et al., 2008; Ganas, 2009; Brncic et al., 2010; Barca et al., 2018), among the most pristine forests in Gola central and south. We used research line transects established by GRNP, sampling five transects in Gola south, eight in Gola central and one site in the community forest where chimpanzees (Pan troglodytes verus) were observed. Each transect (<4 km) was surveyed twice due to returning to camp on the same path. To maximise sampling, we opportunistically collected primate faecal samples whenever we detected individuals visually or acoustically. A total of 382 putative red colobus faecal samples were collected in the field. In 2019, we conducted fieldwork in CNP, Guinea-Bissau between March–April, and October–November. We visited the best-preserved forest fragments in CNP known to harbour the target species (Minhós, 2012) and, in the absence of established transects, we travelled through paths used by local people. We surveyed 15 forest patches and the forest around the base camp in Jemberém (Figure 1). A total of 235 putative red colobus faecal samples were collected in CNP. Across both PAs, 617 primate faeces were sampled and preserved directly in HDPE plastic bottles (50 mL sterile screw top tubes) containing desiccating silica gel. This technique preserves samples unrefrigerated and allows DNA extraction from the host (primates) and all other organisms in its diet, including plants.
2.3 DNA extraction and metabarcoding amplification
Host and dietary DNA were extracted from ~200 mg of each faecal sample, using the QIAmp DNA stool kit (Qiagen, Manchester, UK), following manufacturer guidelines with slight modifications to reagent volumes, incubation and centrifugation periods. For each set of samples extracted, a positive and a negative control were added, with the latter used during DNA metabarcoding analysis. A total of 352 DNA samples barcoded taxonomically for western red colobus were selected for DNA metabarcoding. In addition, 60 DNA samples of badius previously collected in CNP during the 2009 dry season were added (Minhós, 2012).
Presence of plant DNA in 412 primate faecal extracts was confirmed with Polymerase Chain Reaction (PCR) amplification of the second internal transcriber spacer (ITS2) of nuclear ribosomal DNA using universal plant primers UniPlantF (5’-TGTGAATTGCARRATYCMG-3’) and UniPlantR (5’-CCCGHYTGAYYTGRGG-TCDC-3’), following Moorhouse-Gann et al., 2018. A unique combination of 10 bp molecular identifier (MID) tags was added to each sample for faeces identification in the post high-throughput-sequencing (HTS) step. PCRs of 25 μL reaction volumes containing final concentrations of 2.5× multiplex master mix and 2.5 μL of Q solution (Qiagen), 1.0 μM for each primer and ~ 60 ng of template DNA were performed. The PCR cycle initiated with a denaturation step of 95 °C for 10 minutes, followed by 40 cycles at 95 °C for 30 s, annealing temperature of 56 °C for 30 s, and 72 °C for 60 s, and a final extension of 10 min at 72 °C (Moorhouse-Gann et al., 2018). Each 96-well PCR plate included 80 primate samples, 12 negative controls, two blank controls and two positive controls. Negative controls were either extraction or initial PCR negatives treated as DNA samples and used to test primer contamination for each MID-tag. Blank samples (PCR mix and nuclease-free water) were placed in unused MID-tag combinations to identify tag-jumping. Positive controls were a mixture of plant DNA non-native to Africa used for amplification success and artefacts. Amplification of plant DNA was verified in 2% agarose gels and only samples that successfully amplified were selected to go through library preparation for 250-bp paired-end Illumina MiSeq HTS.
2.4 Library preparation and sequencing
All 374 (MID) tag PCR products were quantified (ng/μL) on a QIAxcel Advanced System (Qiagen) and negative controls were checked for PCR contamination. If contamination was detected, the PCR was repeated. Sample pooling for each 96-well PCR plate was based on equimolar concentrations among plant DNA samples, and the volume for negative and blank samples was the same as the average volume of plant DNA. Each pool of samples was cleaned using SPRIselect beads (Beckman Coulter, Brea, USA) in a ratio of 1:1.1 to retain fragments between 300 and 500 bp (ITS2 fragment size after (MID) tag PCR), and its concentration determined after clean-up using a Qubit dsDNA high-sensitivity assay kit (~61.7 ng/μL). Each sample pool was normalised to 100 ng of DNA for adaptor ligation under Illumina sequencing library preparation using a NEXTflex Rapid DNA-Seq Kit (Bio Scientific, Autin, USA) and AMPure XP Beads clean-up. A different Illumina index was assigned to each sample pool, and pools were sequenced for 250-bp paired-end reads using a Reagent Kit v2 on a Illumina MiSeq desktop sequencer (Illumina, San Diego, CA, USA). The sequencing and subsequent cleaning stages produced a dataset with 368 red colobus samples with good quality plant reads, five samples with zero plant reads, and one sample that showed some evidence of contamination. Six samples with no-results or contamination were excluded from the final dataset.
2.5 Bioinformatics and taxonomic identification
The bioinformatics pipeline followed Drake et al. (2022) with modifications following updated programs and improved methodology in the taxonomic assignment step. The pipeline was performed under LINUX and comprised programs and scripts written in BASH and PERL. Raw sequences were trimmed, aligned and checked for quality of reads using FastP v0.20 (Chen et al., 2018). Reads with q-score lower than 33 were discarded. Mothur v1.46.1 (Schloss et al., 2009) was used to assign reads to the respective sample IDs, according to the (MID) tag primer combinations, allowing for a minimum of one mismatch. Next, demultiplexing merged all reads from each sample into one fasta file. Usearch v11 was used with the Unoise3 command to denoise and remove chimeric sequences, and group identical sequences into zero radius operational taxonomic units (zOTUs). These were generated using a clustering identity threshold of 100% to avoid multiple species being grouped under the same OTU (conservative algorithm). At this stage, read abundance matrices (number of each zOTU reads per sample) were created along with a zOTU list, and further data clean up and filtering was performed with a combination of manual and Microsoft Excel processing. Reads originating from contamination, tag jumping and bleeding of over-represented taxa into other samples were removed using the combined maximum read count for blank, extraction negative and MID-tag PCR negative samples (Drake et al., 2022). A per zOTU threshold of 1% was further applied to eliminate low read counts. The value of the threshold was taken from a study that assessed contamination rates in complex field environments where samples are exposed to non-food DNA on the ground (Ando et al., 2018). After these cleaning steps, taxa were reduced by 24% but this more conservative approach was selected so plant diversity detected would not be inflated.
Taxon information for each taxonomic unit was obtained by comparison with sequences on the most up to date eukaryote nucleotide database from NCBI GenBank (2022). The BLASTn v2.12.0 (Altschul et al., 1990) was used in BLAST+ (Camacho et al., 2009) with a minimum percentage identity score of 96.02%, a value based on the mean value for the average genetic distances of sister species in angiosperms (3.98%, Qin et al., 2017). Additionally, the zOTUs retained were selected based on the plant species listed on both PAs botanical surveys (Klop et al., 2008; Catarino and Palminha, 2018) and presence in west Africa, or the African continent. In some cases, taxonomic identification could not be achieved for species, so genus or family identification was used. Fungal or bacterial sequences or other types of contamination (e.g. Homo sapiens, starch – Solanum spp. originating from the extraction kit spin columns) were not considered.
2.6 Dietary statistical analysis
A final dataset comprising 368 western red colobus samples (GRNP = 207; CNP: 2019 = 101, 2009 = 60) was transformed into presence/absence data, and frequency of occurrence (Fo%) was calculated for each zOTU. All statistical analyses were conducted in R Statistical software v4.1.3 (R Core Team, 2022).
Hill-diversity and sample coverage were estimated for each PA to assess dietary diversity and sampling efficiency of this study (Hill, 1973; Roswell et al., 2021). This method was preferred over sample size and effort-based standardisation, and asymptotic species-accumulation curves because they fail to account for the species-abundance distribution of the community sampled (Roswell et al., 2021; Tercel et al., 2022). Hill diversity is measured as the generalised mean species rarity and three exponents can be used to determine the sensitivity of the equation to rare species. All exponents were tested using the R package iNEXT (Hsieh et al., 2016): the most sensitive Hill-richness (l = 1), Hill-Shannon (l = 0) which responds to both high and low rare species, and the least sensitive Hill-Simpson (l = −1).
To evaluate seasonal variation in dietary composition, a model-based approach in the mvabund R package (Wang et al., 2012) using simultaneous GLMs (generalised linear models; function ‘ManyGLM’), a binomial error family and respective link function, and Monte Carlo resampling method were implemented. Data residuals were checked for normality for the modelled GLMs and Likelihood ratio tests (LRT), and resampled p-values are presented for the models selected. For visualisation purposes we used Vegan (Oksanen et al., 2019) and ggplot2 (Wickham et al., 2016). The same approach was used to identify the effect of sex, time and habitat in the diet of western red colobus. Dietary variation was compared between 112 females and 42 males in the GRNP population (smaller dataset due to unsuccessful amplification of the sex marker for some samples), between CNP populations sampled in the same four forest patches in 2009 (N = 53) and 2019 (N = 14), and between areas of each PA with distinct differences in vegetation. In GRNP, the south forest block was intensively logged, leading to the presence of more secondary forest. The dataset is smaller (91 samples from Gola central, 31 samples from Gola south) and it represents the rainy season only, as no samples were obtained for red colobus during the dry season in Gola South. In CNP, the north of the park is mainly covered by savanna, and less forest and mangrove. However, due to the small sample size in this region, a similar spatial comparison was not possible.
Mean plant richness per sample was calculated for the diet of badius populations in each PA and compared using non-parametric Kruskal-Wallis tests to determine significant differences between PAs, females and males in GRNP, red colobus in Gola central and south, and by individuals sampled in 2009 and 2019. Levin’s index of niche breadth (Levins, 1968) was estimated to compare the use of forest resources by the same groups of red colobus as above. We used the ‘niche.width’ function of the R package spaa (Zhang and Zhang, 2013) and standardised it with equation one from Razgour et al. (2011).
3 Results
3.1 Data filtering
The HTS generated approximately 12.39 million reads, reduced to 6,967,262 reads following filtering. An average of 7,147 DNA reads ( ± 3,593 SE) were obtained per sample and a total of 14,867 zOTUs compared with the NCBI GenBank reference database. Several zOTUs were discarded due to no or unsure taxa assignment (9.9%), taxa not native to or introduced in west Africa (1.1%), assigned to bacterial taxa (0.2%) and to fungi (3.4%). This last percentage is relatively high, possibly because the marker is also a universal barcode for fungi (Schoch et al., 2012) and some samples had developed fungi during the storage period. This study identified 1,699 dietary presence counts in 368 western red colobus samples, belonging to 97 plant taxa.
3.2 Piliocolobus badius diet
Western red colobus yielded 97 plant taxa (64 plant species and 33 genera) in the diet across the years and PAs, belonging to 40 families. An average of 4.48 plants ( ± SE 1.84) were detected in each sample with a minimum of one and a maximum of 11 taxa per sample. One fifth of plant taxa were detected in one single sample but it was still included in the analysis as this is a result obtained after cleaning the dataset for contamination. Overall, Hill-diversity estimates suggest that sampling conducted in both PAs was sufficient to cover a large majority of the dietary community (Figure 2, top graph). Less than 5% of the dietary taxa were not detected for each sample subset (dietary coverage ±95% CI: GRNP = 98.1 ± 0.9; CNP = 98.6 ± 0.9). The Hill-richness exponent (l = 1) provided higher diversity estimates than the other two exponents (Hill-Shannon, l = 0; Hill-Simpson, l = −1), which reach an asymptotic result at the same number of detections (read counts). This shows that the dietary community of western red colobus comprises many plant taxa consumed infrequently, as exponent l = 1 is more sensitive to rare species (Figure 2, bottom graph).
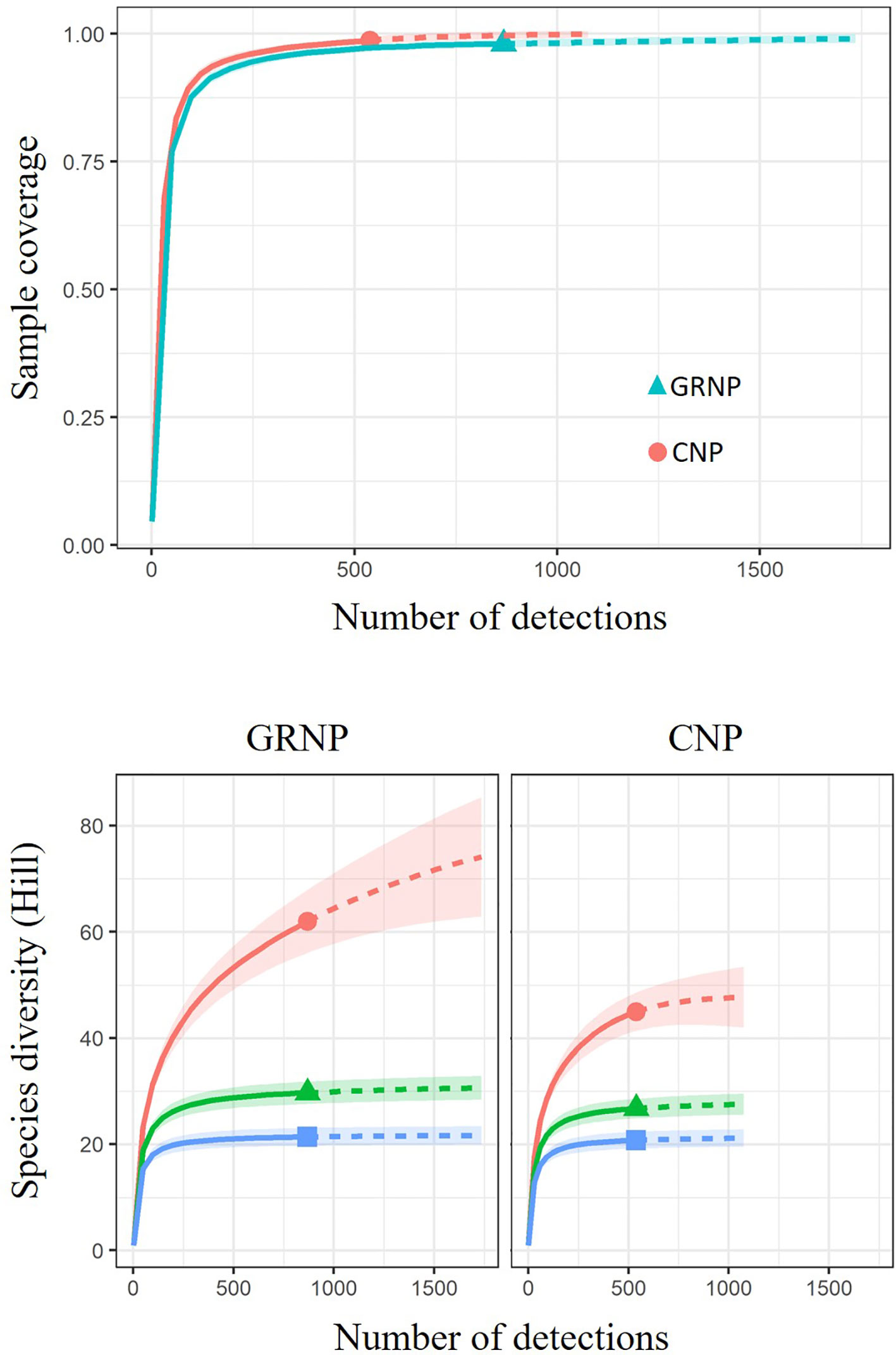
Figure 2 Plant species diversity and level of community coverage in the diet of western red colobus. The top graph demonstrates how much dietary community was possible to cover per number of detections for badius populations of GRNP (blue line and triangle) and CNP (red line and circle). These observations are extrapolated and shown by the dashed lines. On the bottom graphs, the percentage of plant taxa by number of dietary detections, generated with a DNA metabarcoding approach, is estimated in each protected area (GRNP and CNP). Results show three exponents with higher (1) to lower (−1) sensitivity to rarely consumed food items: Hill-richness (l = 1), red line with circle; Hill-Shannon (l = 0), green line with triangle; Hill-Simpson (l = −1), blue line with square. Solid lines are the observed diversity, which are further extrapolated (dashed lines). Shading around the line corresponds to 95% confidence intervals.
All plants detected in the diet of western red colobus were present in the botanical surveys conducted in each PA or have been recorded in the countries where sampling took place. More than half of these species were recorded as food items in previous red colobus research (Supplementary Tables S3–S5; Table 1) and represent plants with the highest frequency of occurrence in this study. The plants detected in the diet of the GRNP population belong mostly to the Fabaceae family (18.4% – one of the most common plant families in the park), Combretaceae (12.7%), Rubiaceae (9.6%) and Chrysobalanaceae (9.2%). These plants are mainly trees and shrubs, with vines and climbers being detected in fewer samples. A total of 62 plant taxa were identified in the diet of the GRNP population, and the top species consumed were Lophira alata, Anthonota macrophylla, Combretum spp. and Maranthes aubrevillei (Supplementary Table S3). We identified 45 plant taxa from 24 families in the diet of the CNP population sampled in 2019. Fabaceae, Moraceae and Rubiaceae contributed to nearly 50% of the primate diet. Treculia africana was detected in more than half of the samples, and four other species were present in more than 35–40% faecal samples (Supplementary Table S4). Trees and shrubs were identified as the most important in the diet of this population.
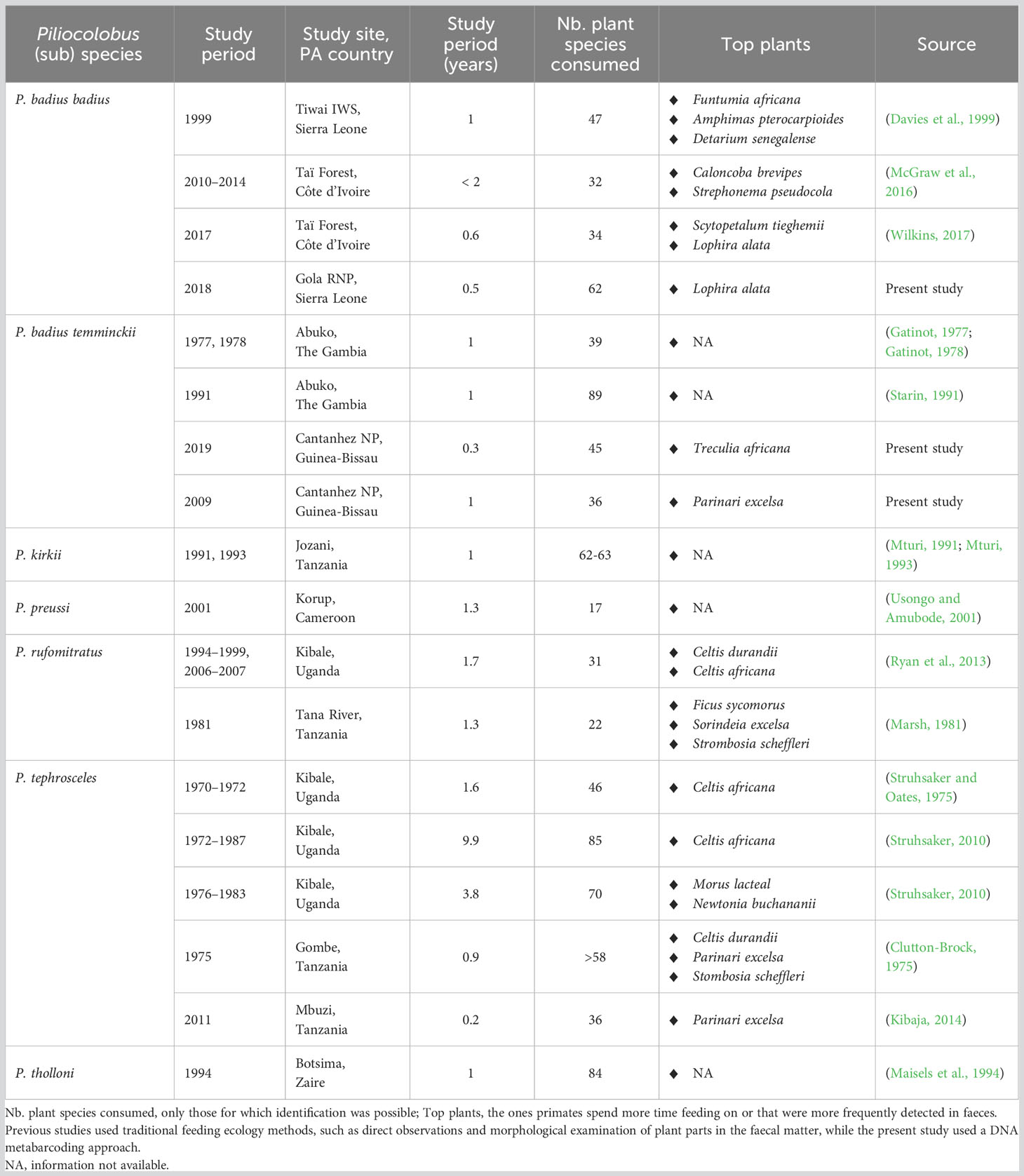
Table 1 Information on the present and previous dietary studies on red colobus (Pilicolobus spp.) across Africa.
3.3 Dietary variation
3.3.1 Seasonal variation
The top 20 plant taxa were all present in GRNP samples collected in the dry and rainy seasons, except Nauclea spp. which was only detected in samples collected during the rainy season. Fewer plant taxa were detected in the dry than in the rainy season (plant taxa: dry = 35, rainy = 54), but mean plant richness per sample was similar between seasons (dry = 4.23, rainy = 4.16; W = 5299.5, p-value > 0.05). However, seasonal dietary composition varied significantly (LRT = 328.1, p-value = 0.001; Figure 3). Seven plants contributed to this variation (p-value < 0.05) with the most significant being Cryptosepalum tetraphyllum only consumed in the dry season, and Nauclea spp. detected only in the rainy season.
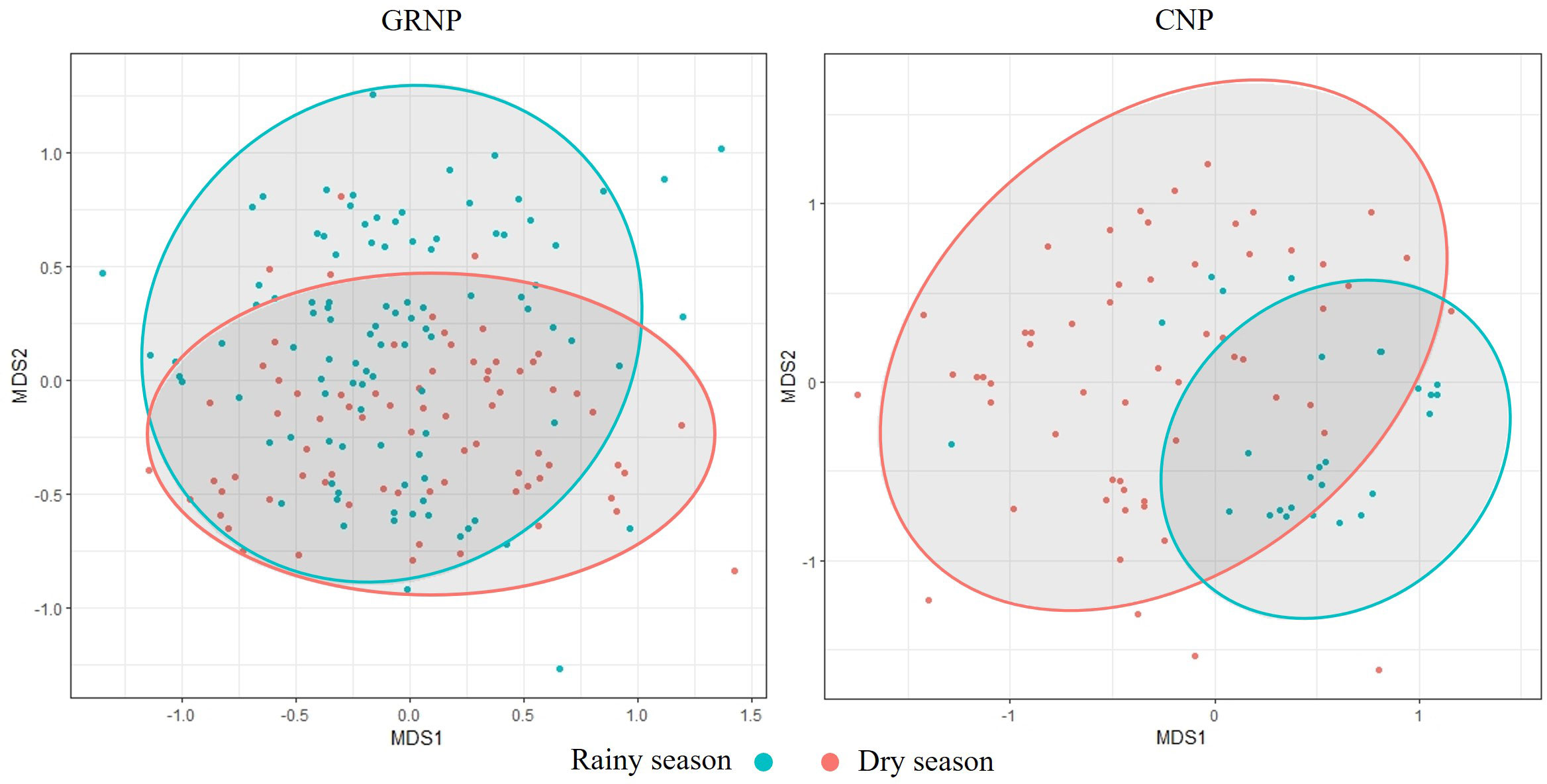
Figure 3 Seasonal dietary variation of the GRNP (N = 207) and CNP (N = 101) populations, represented by pairwise biplots from non-metric multidimensional scaling (NMDS) analysis. The diet of badius varied significantly between the dry season (red dots and ellipse) and the rainy season (blue dots and ellipse).
The most frequently detected plant taxa for the red colobus of CNP were all native to Guinea-Bissau, with Treculia africana being detected in more than 50% of samples. Most plants were detected in both seasons, with a few only present in the rainy (e.g. Uncaria africana) or dry season (e.g. Ceiba pentandra). Despite the total number of plants detected in samples from the 2019 dry season being higher (plant taxa: dry = 37, rainy = 28), the mean plant richness per sample was significantly lower than in the rainy season (dry = 4.69, rainy = 6.64; W = 480.5, p-value < 0.001). The MGLM model revealed that dietary composition in the dry and rainy seasons was significantly different (LRT = 398.6, p-value = 0.001, in Figure 3), and three of the twelve plant species that had a significant effect on seasonal variation (p-value < 0.02) were consumed in the dry season (Cryptosepalum tetraphyllum) or in the rainy season (Nauclea spp. and Daniellia ogea).
3.3.2 Biological variation
Differences in the number of plants (females = 4.06, males = 4.4; W = 1966, p-value > 0.05) or the type of plants ingested (LRT = 5.66, p-value = 0.134) by female and male red colobus of the GRNP population were not significant. Dietary niche for females and males of red colobus from GRNP was equal (Levin’s niche = 0.11). The overlap of the items detected in the diets of the two groups is clearly observed in the NMDS plot (Figure 4A).
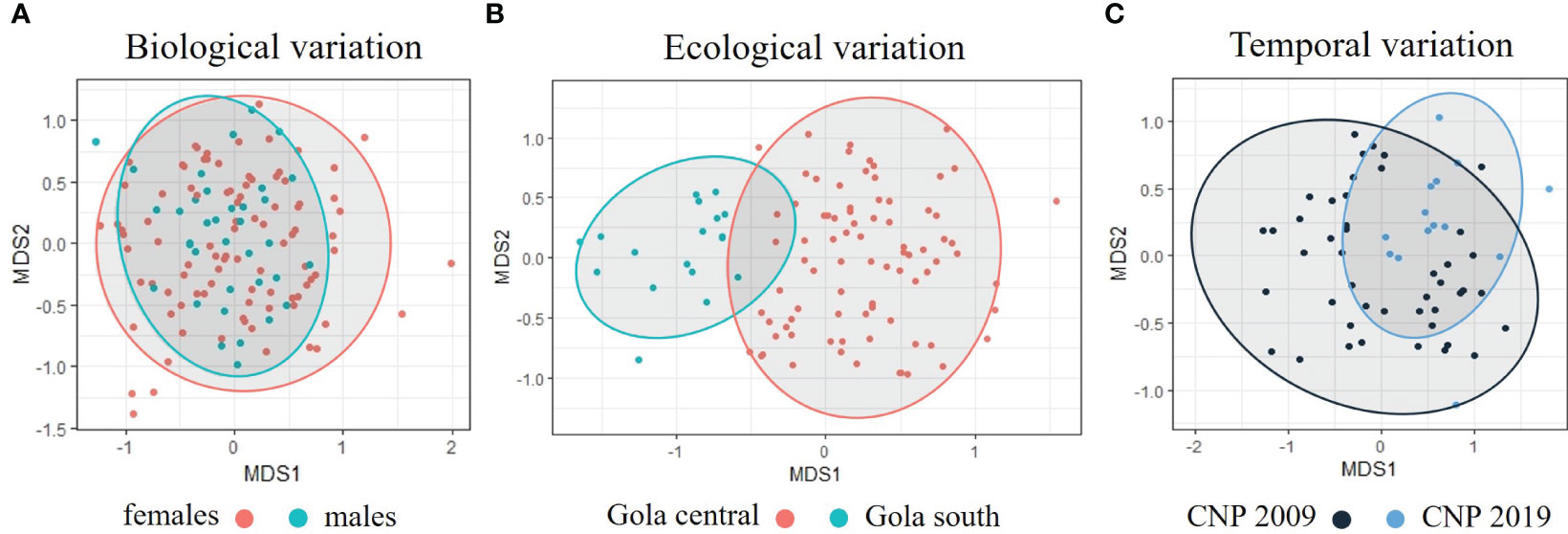
Figure 4 Biological, ecological, and temporal variation in the dietary composition of western red colobus is illustrated in three pairwise biplots from non-metric multidimensional scaling (NMDS) analysis. Panel (A) shows a non-significant variation in dietary composition of female (red circles and ellipses) and male red colobus (blue circles and ellipse). The NMDS plot (B) illustrates a significant variation in the food items consumed during the rainy season by badius individuals living in Gola central (red circles and ellipses) and Gola south (a more degraded forest in GRNP; blue circles and ellipse). The plot on the right (C) represents the significant variation in dietary composition of red colobus individuals sampled in 2009 (dark blue circles and ellipse) and in 2019 (light blue circles and ellipse).
3.3.3 Ecological variation
We detected significant differences in the mean number of plant taxa in red colobus from Gola central and Gola south blocks during the rainy season (Gola central = 4.5, Gola south = 3.0; W = 1849.5, p-value < 0.001), and in the variation of the dietary composition (LRT = 8.46, p-value = 0.001; Figure 4B). Nine plants contributed significantly to this variation (p-value < 0.05), with three of the most significant taxa being detected in Gola central samples only (Anthonotha spp., Maranthes aubrevillei and Pentadesma butyracea) and two mainly in Gola south (Terminalia spp. and Trichilia monadelpha). Niche width was wider for the red colobus groups in Gola central (0.10) than those in Gola south (0.04).
3.3.4 Temporal variation
The CNP red colobus population was sampled with a ten-year gap, in the dry season of 2009, and in the dry and rainy seasons of 2019. Additionally, only four of the forest patches were sampled at both time periods so, for comparison purposes, we only analysed samples from the dry season and from the following forests: Caghode, Cambeque, Jemberém and Madina. Plant species diversity was higher for the population sampled ten years ago but so was sample size (2009 = 54 samples, 32 taxa; 2019 = 14 samples, 22 taxa). Nonetheless, the mean number of plants in each sample was significantly different between years (2009 = 3.80, 2019 = 5.0; W = 240.5, p-value = 0.042) and Levin’s niche was wider in 2009 (2009 = 0.09, 2019 = 0.06). Moreover, even though dietary composition between time periods showed significant variation (LRT = 7.2, p-value = 0.005), the diet of individuals highly overlaps across time (Figure 4C), with only two plant species significantly contributing to the variation (Ceiba pentandra and Treculia africana) which were present in both time periods, but at a much lower frequency in 2009 (~14%; in 2019, 64%).
3.4 Dietary flexibility under different ecological conditions
The diet of the GRNP and CNP populations varied in the raw count of plant taxa and mean number of plants detected per sample (Table 2). Total plant richness was higher in GRNP than in CNP, despite botanical surveys providing similar numbers of recorded plants (899 species in GRNP, 863 species in CNP). However, mean plant richness per sample detected in red colobus diet from GRNP was significantly lower than in CNP (t = −3.37, df = 309.63, p-value < 0.001). Niche breadth was also narrower for the CNP (0.116) than for the GRNP population (0.120). As Gola south has been intensively used for logging and the vegetation is expected to contain a certain degree of secondary forest resembling a more degraded habitat, we tested the differences in the mean plant richness per sample against CNP, a degraded forest, and between CNP and Gola central only, as this most closely resembles a pristine forest within our dataset. Levin’s niche standardised with Razgour et al. (2011) equation was also calculated. We detected significantly fewer plants in the diet of red colobus sampled in Gola south during the rainy season, than in those from CNP during the same season (Gola south = 3.0, CNP = 6.64; W = 43, p-value < 0.001), and a narrower niche (Gola south = 0.04, CNP = 0.08). A similar pattern was detected between the continuous and well protected forest of Gola central and the altered landscape of CNP across both seasons (Gola central = 4.36, CNP = 5.33; W = 6547, p-value < 0.001), although niche width was similar (Levin’s niche for GRNP = 0.11 and CNP = 0.12).
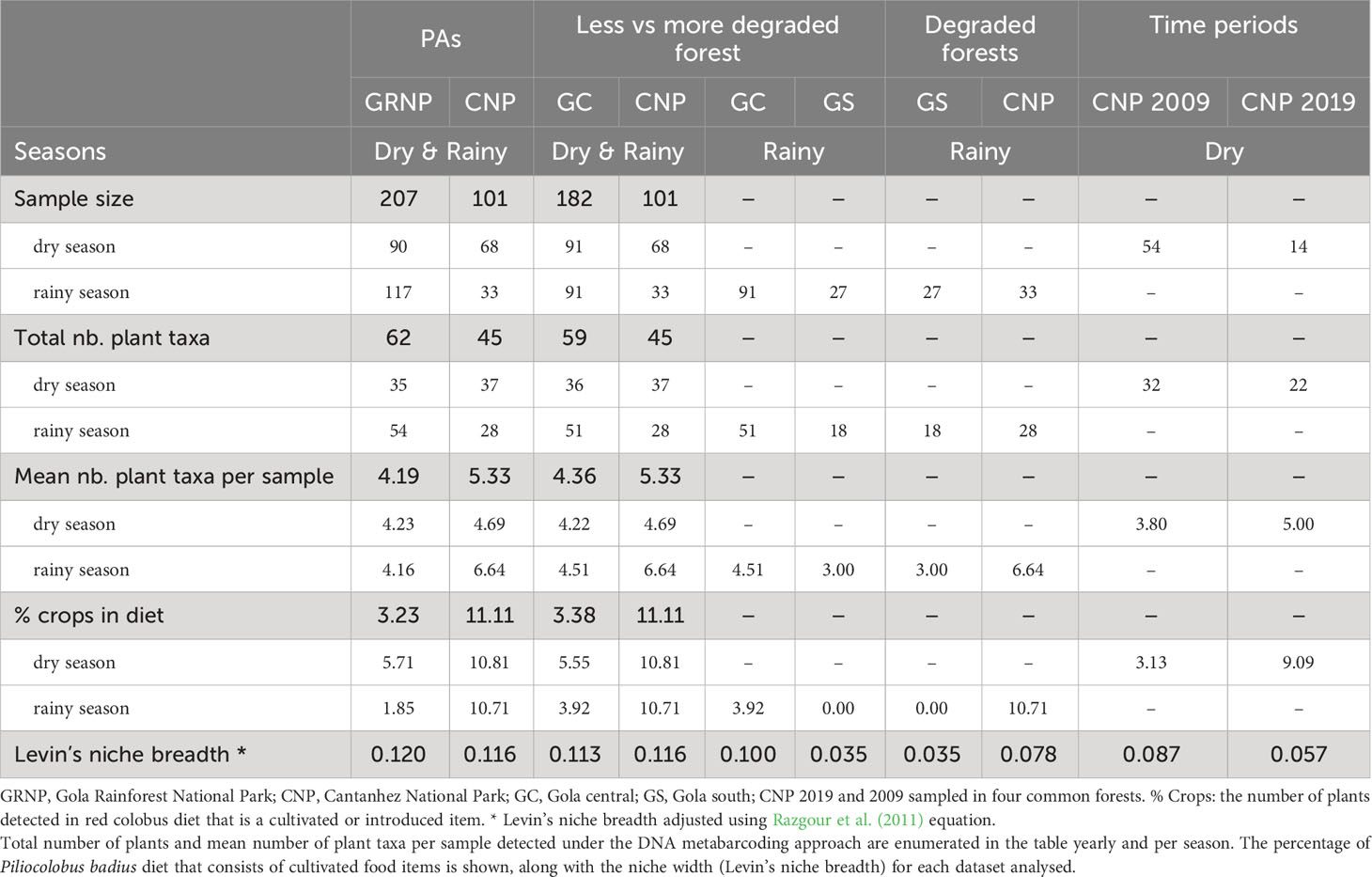
Table 2 Plant dietary richness of western red colobus populations of GRNP and CNP, compared across protected areas, habitat types, and time periods.
3.4.1 Consumption of cultivated plant species
Just over 7% of western red colobus diet, across study sites and years, comprises crops (defined here as native or introduced plant species cultivated in farming grounds outside or within the PAs, or naturally growing in the forest and exploited by local human communities). One introduced (Enterolobium spp.) and two native plants exploited by humans in Sierra Leone (Cola spp. and Coffea spp.) were detected for the GRNP population, but they were rarely present across samples (Fo < 2.5%; Supplementary Table S3). Despite being native, Kola trees are sometimes grown as crops by local villagers. However, as in this PA most red colobus groups occur within the protected forest and so did the sampling, Cola spp. will not be considered a crop. Hence, crops Enterolobium spp. and Coffea spp. accounted for 3.2% of badius diet in GRNP. Both items were eaten during the dry and rainy season, but Coffea spp. had a higher frequency of consumption in the dry season, when the unripe fruit is available. The percentage of crops identified for samples in Gola central during the rainy season was 3.9, while absent in samples from Gola south. A higher number of crops was identified in the dietary composition of the red colobus population of CNP (11%). However, all items had low frequencies of occurrence compared to the wild plant taxa consumed: mango (8.9%), coffee (4%), Solanum spp. (2%), cashew (1%) and Cucumis spp. (1%) (Supplementary Table S4). In the 2009 dataset, we detected one single crop (Mangifera indica) with a Fo of 33.96% in the diet of the red colobus group from Jemberém forest, the closest group to a human settlement (Supplementary Table S5). In 2019, crops were present three times more in the diet of badius than in 2009, and with higher frequencies of occurrence (M. indica = 64.29%, Solanum spp. = 7.14%). Again, the group which consumed more crops was the one sampled in the neighbouring forest of Jemberém. However, in the more recent sampling period, another badius group occupying a forest close to the village of Madina also consumed cultivated items.
4 Discussion
4.1 Western red colobus diet
This study is the first application of DNA metabarcoding sequencing to investigate the diet of western red colobus species in west Africa. The method proved successful when using non-invasive samples, yielding 97 plant taxa (62 for the GRNP population and 45 in CNP) in the diet of Piliocolobus badius. Four to five plant taxa were most frequently detected in the diet of each badius population, suggesting a degree of dietary specialism and the importance of those plants for the survival of these threatened primates. Red colobus preferentially feed on the largest and most common plant species in their habitat (Oates, 1994), here observed in the GRNP population (e.g. Lophira alata, Maranthes aubrevillei, Supplementary Table S3) over other taxa. Lophira alata was also identified as an important food item for the red colobus population of Taï, Côte d’Ivoire (Wilkins, 2017). It was not possible to infer a similar correlation for the CNP population as abundance data for plant species is not yet available for this PA. However, should our results support the theory, we could suggest that Treculia africana, Parinari excelsa and Malacantha alnifolia could be some of the largest and more common trees in CNP, as they had the highest frequencies in badius diet (Supplementary Tables S4, S5). As for badius of Taï and in Tiwai and for rufomitratus in Kibale, Uganda (Davies et al., 1999; Ryan et al., 2013; Wilkins, 2017), figs from Ficus spp. and fruits of Parinari excelsa trees were important food items for the CNP population (Fo >30%). These two plant taxa are present in most habitats of the CNP, suggesting that red colobus inhabiting more degraded forest patches still have access to important foods.
Although DNA metabarcoding does not provide quantitative data for the items consumed, the frequency of occurrence demonstrates the most commonly present plant taxa in the diet of red colobus. In this study, trees and shrubs were the most detected plant types, reflecting arboreal behaviour of Piliocolobus. The present study detected a higher dietary richness for badius than previous studies employing traditional methods to study the feeding ecology of red colobus species across Africa for longer periods of time (min. = 17, max. = 89 plant species; Table 1). This was expected, as DNA metabarcoding is a more robust technique featuring higher taxonomic resolution to detect rarer food items consumed or more easily digestible foods (Pompanon et al., 2012; Ando et al., 2013; Sousa et al., 2019). The efficiency of the method is further supported by the high proportion of plant taxa identified that did not occur frequently in the diet of the sampled individuals, and that have not been reported in previous red colobus dietary studies.
4.2 Variation in diet
Females and males did not have significantly different diets (Figure 4A), despite assumptions that overall energy costs are greater for females than males associated with gestation and lactation (Key and Ross, 1999). Dietary variation between seasons, habitats and time periods was significant. Annual fluctuation in the diet of western red colobus was observed in both PAs (Figure 3), but results do not follow a specific pattern. Although the lack of phenology data during the study period does not permit a direct correlation with young leaves, seed and fruit availability, previous regional studies indicate that these plant parts are more commonly produced in the dry season (Munro et al., 2013; Bessa, 2014; Hockings et al., 2020). Overall, the GRNP population demonstrated a much higher consumption of different plant taxa during the rainy season (when preferred food is less available), while an opposite trend was observed for the CNP population (Table 2). These differences may reflect food availability in each habitat and food preferences by the primate groups within each population. Several years of data on the b. tephrosceles of Kibale forests (Uganda) suggest that significant inter-annual variation in dietary composition reflects the complex diversity of the habitat (Struhsaker, 2010). At the time of the study, Kibale was a relatively large old-growth rainforest, a type of habitat preferred by red colobus. However, only a few plant species accounted for the majority of the diet, reinforcing this species as a specialist consumer (Struhsaker, 2010). One limitation of DNA metabarcoding is the fact that food items cannot be quantified. Hence, the lower mean plant richness per sample observed in GRNP during the rainy season (Table 2) may indicate that red colobus consume higher quantities of few preferred foods to meet the daily nutritional requirements, but because individuals may have to cover a wider range of the PA during food scarcity and as a response to intra-group competition, it contributes to an increase in the total number of plant taxa consumed by the population overall. We cannot exclude the fact that competition for food with other sympatric primates with similar dietary requirements (e.g. Colobus polykomos, Chlorocebus aethiops sabaeus) may also influence the patterns we found. In this study, the top four trees and shrubs in the GRNP red colobus diet (Supplementary Table S3) can produce leaves mostly year-round, with simultaneous periods of flower and fruit production (Arbonnier, 2002), providing food even during low preferred food availability.
In CNP, more overall plant taxa were detected in the dry season but the mean plant richness per sample was significantly higher in the rainy season (Table 2). This contrasts with our inference for GRNP and may represent restricted food access in a more degraded habitat. As preferred food is less abundant in the rainy season, the CNP population, with a smaller area of forest to explore and likely less diverse plant species, reduced its plant taxa intake overall but each individual had to feed on more plants to meet its dietary requisites. Protein-to-fibre ratio of food items may determine leaf choice in herbivores, and hence limit colobine population size (Milton, 1979), while large groups have higher group feeding competition and require more energy for travelling in search of clumped food (Wrangham et al., 1993; Chapman, 2000). Therefore, as a response to low preferred food availability (with lower protein-to-fibre ratio), red colobus in CNP may have to cover different parts of the forests in their daily travel distance, increasing the number of plant taxa in the overall diet of the population. The most heavily consumed plant by the CNP population, particularly in the rainy season, was Treculia africana (Supplementary Table S4), which appears to provide food annually as the flowering period is from October until February, and its large fruits, containing many seeds, can be present all year-round depending on region and environmental conditions (USDA, 2015).
Spatial differences detected in the diet of GRNP red colobus during the rainy season showed each individual from Gola central feeding, on average, on more plant taxa than those from Gola south forest block, having a wider niche, and varying significantly in the plants consumed (Figure 4B). Variation in the vegetation cover, where Gola south is more likely covered by a higher area of secondary forest due to intense forest logging, could influence the type of preferred food availability, impacting directly on the dietary choices of red colobus. Indeed, two of the plants that contributed most to the dietary variation and were mostly consumed by the group in Gola south, can be used as timber (Terminalia spp.) or are a type of understorey plant of rainforest common in secondary growth areas (Trichillia monodelpha).
In a temporal analysis performed on the diet of badius in Taï forest (Côte d’Ivoire), where 20 years of vegetation transformation resulting from changes in the rainfall, fragmentation, and increased logging have taken place, there were clear reductions in dietary diversity and number of species consumed over time (Wilkins, 2017). This was also observed in the CNP population, but could be due to the small sample size in 2019 as the mean plant richness per sample was higher than in 2009. However, extant individuals have a narrower dietary niche and more frequently consume items from some of the largest trees in the park. The variation observed in the diet of red colobus from CNP across time requires further investigation, including temporal phenological surveys to elucidate whether changes are related to actual alterations in the vegetation or simply intra-specific food choices.
4.3 Dietary flexibility
Despite niche width being similar in both PAs, the population of GRNP fed on almost 20 more plant taxa than the CNP population. Hill-diversity indices indicate that this is a real difference and not an artefact of the different sample size (Figure 2). Nonetheless, the mean plant richness per sample was considerably higher for the CNP population. These results were similar to trends inferred in the comparison between Gola central (the most continuous and pristine forest) and CNP (degraded forest) populations, as well as for red colobus groups sampled at two time periods in CNP. It may be that in the degraded forests of CNP, particularly when preferred food is scarce, red colobus must feed on more plant species to meet dietary requirements, probably relying on many poorer quality foods. Contrastingly, badius inhabiting the well preserved, larger and more connected forest of GRNP can likely meet daily dietary requirements relying only on preferred plant species, which are available even during the most challenging season. However, spatial analysis in GRNP between less and more degraded forests (Gola central and Gola south, respectively), and the comparison between two more degraded habitats (Gola south and CNP), reflect dietary differences that cannot be directly associated with red colobus habitat (Table 2). The variation in the dietary patterns illustrated by this study pose a challenge for predicting western red colobus adaptation to rapid anthropogenic changes in vegetation, with unpredictable consequences to this endangered primate socio-ecology, namely group size and social structure, spatial distribution, daily travel or dispersal dynamics, and consequently long-term persistence. Another study of the impact of modified landscapes on the dietary flexibility of female red colobus in Kibale, Uganda, identified behavioural flexibility in the group living in the logged forest in comparison to the old-growth areas (Milich et al., 2014). Females from the logged areas fed on fewer resources from a greater number of plant species, enabling similar population densities to female groups in the old-growth areas.
Cultivated food items were more prevalent in the diet at both time points in CNP than in the GRNP population (Supplementary Tables S3–S5; Table 2). The inclusion of cultivated items in the diet of a highly forest-dependent primate is probably a response to living in a more degraded habitat and nearer cultivated fields, which provide access to more energetic food items. This behaviour has been observed in other populations of red colobus that could not meet the dietary requirements from preferred wild food due to forest degradation (Galat-Luong and Galat, 2005; Kibaja, 2014). The crops detected in the diet of red colobus in CNP are present near settlements, in gardens or in monoculture orchards. There are mango trees in the dense forest, but in general we demonstrate that badius is foraging on grounds that are commonly used by humans. Coffea spp. was consumed by red colobus in GRNP in both seasons, as was Enterolobium spp. Coffee trees are cultivated in orchards that are managed under an agroforestry system. As most coffee species are intolerant to direct sunlight and benefit from a soil enriched by the fallen leaves of high canopy trees (Alemu, 2015), farmers in Gola region plant coffee trees under tall trees (information provided by conversations with farmers during fieldwork and direct observations). In South America, farmers use Enterolobium spp. as a shading tree in coffee plantations, and some species of this taxa have been introduced in Sierra Leone, likely explaining our results. As we expected, the diet of red colobus from CNP included more human-cultivated foods, probably due to lower availability of forest, wild preferred foods, and the closer proximity with humans and therefore access to crops. This pattern is reinforced by the spatial distribution of faecal samples. In CNP samples were collected relatively close to human settlements, whereas in GRNP most samples were collected deep in the forest, distant from the villages and crops. Our results show that the different PA landscape and management strategies have a direct impact on the spatial distribution and habitat use by western red colobus, and consequently on the diet composition of this specialist primate.
4.4 Contribution to primate conservation
Habitat degradation due to vegetation loss, fragmentation and transformation to farmland is a major threat to red colobus population sustainability (Struhsaker, 2010; Linder et al., 2021). One solution to minimise biodiversity loss is to implement new and/or improve management of existing PAs. However, not all areas are suitable habitat for primates (e.g. sand dune vegetation, high altitude forests) (Estrada, 2006) and, although important for wildlife and ecosystem conservation, many do not offer the best conditions for primate populations to thrive (Chape et al., 2005). Many are poorly managed, understaffed or simply exist on paper, and are commonly small vegetation islands surrounded by altered landscape (DeFries et al., 2005; UNEP-WCMC, & IUCN, 2022). We suggest that the large and well preserved forest of GRNP may provide sufficient natural resources for western red colobus to maintain dietary requirements, but some evidence of dietary flexibility to habitats where humans are present is emerging. The fact that in the more disturbed forests of CNP, red colobus include more crops in its diet and, during the seasons of lower food availability, have to rely on more plant species to meet the dietary requirements show some level of dietary flexibility. This is also the case with other primates (e.g. Pan troglodytes, Macaca spp., Cercopithecus spp.; Hill, 2018). It is therefore essential to better understand how primates use agroforest areas, and plan, along with local communities, the most sustainable way to manage the landscape in order to improve non-human primate persistence in anthropogenic protected areas and local livelihoods. Work is already underway in GRNP, where cocoa beans from plantations with increased biodiversity are preferred to those that exclude animals that feed on this crop. This strategy could serve as a precursor to exploring the growth of other crops along with endemic species. In CNP, the complex and dynamic use of the landscape may have a significant impact on red colobus ecology as its flexibility to a disturbed environment is clearly visible from our results. Despite some dietary flexibility which currently allows the species to cope with a decrease in the forest habitat that is being converted into monocultures, reduced wild food resources may push red colobus individuals to live in smaller forest fragments with scarcer natural foods, and consequently rely more on human cultivated foods. Moreover, red colobus are a highly vulnerable and targeted primate for bushmeat hunting (Minhós et al., 2013), implying that a closer proximity to humans in search of food sources increases exposure to hunters and threatens survival. Overall, our results show that large and preserved forests are not only important to provide good quality food to maintain sustainable red colobus populations, but also ensure that these primates maintain a safer distance from neighbouring human communities.
5 Conclusion
Anthropogenic pressure will continue to affect natural habitats and weakly protected areas, and thus, well preserved forests like GRNP may play a crucial role in the socio-ecological stability of threatened non-human primate populations. Differences in red colobus diet, such as those detected within and between PAs in this study, could be explained by the nutritious value of each food item, plant species distribution and abundance across the landscapes, and consequently, seasonal food availability; but it could also reflect the dietary flexibility of this specialist primate species to the habitat. Identifying the cause of dietary diversity and variation proved difficult in this short-term research mostly due to the complex species socio-ecology and variations in natural resource availability. Therefore, it is important to use integrative approaches to understand how primates respond to habitat transformations in space and time, to provide and implement sustainable and achievable conservation strategies within and outside PAs. The identification of important plants in species diets is a key factor to consider for conservation management, including future reforestation campaigns. Strategic plant species and locations must be well defined for the sustainability of projects, including plant growth success and feasibility of habitat use by red colobus and other species, and its interplay with local livelihoods.
Data availability statement
The original contributions presented in the study are included in the article/Supplementary Files. Further inquiries can be directed to the corresponding author.
Author contributions
IAP: Conceptualization, Data curation, Formal analysis, Funding acquisition, Investigation, Methodology, Project administration, Resources, Visualization, Writing – original draft, Writing – review & editing. FB: Data curation, Methodology, Writing – review & editing, Investigation, Project administration. NS: Investigation, Writing – review & editing. MSo: Investigation, Writing – review & editing. MC: Investigation, Writing – review & editing. ITC: Investigation, Writing – review & editing. CR: Investigation, Writing – review & editing. BB: Project administration, Resources, Writing – review & editing. BST: Project administration, Resources, Writing – review & editing. MSw: Resources, Writing – review & editing. ARB: Project administration, Resources, Writing – review & editing. QQ: Project administration, Writing – review & editing. MJFDS:Investigation, Writing – review & editing, Funding acquisition. AFM: Resources, Writing – review & editing, Supervision. MWB: Project administration, Resources, Supervision, Conceptualization, Methodology, Writing – original draft. TM: Funding acquisition, Investigation, Writing – review & editing, Conceptualization, Methodology, Project administration, Resources, Supervision, Writing – original draft, Formal analysis, Visualization.
Funding
The author(s) declare financial support was received for the research, authorship, and/or publication of this article. IAP was supported by a doctoral fellowship from Foundation for Science and Technology (FCT), Portugal (SFRH/BD/118444/2016), as well as FB (2020.05839.BD). Field and laboratory work was financed by the FCT under the project PRIMATOMICS (PTDC/IVC‐ANT/3058/2014). Publication fees were supported by Cardiff University.
Acknowledgments
Fieldwork for sample collection would not have been possible without permission from the Sierra Leonean and Guinea-Bissau authorities, especially the Gola Rainforest National Park and the National Protected Area Authority, Cantanhez National Park and the Instituto da Biodiversidade e das Áreas Protegidas. The authors would like to thank the community researchers and park rangers who assisted with sampling, Jordan Cuff and Maximillian T. G. Tercel for their support in the laboratory and bioinformatic analysis, and Neil D. Cook for a final formatting revision. We are thankful to Angela Marchbank, Catherine Bresner and Trudy Workman at the Genomic Hub, Cardiff University, Biosciences, for their valuable input and knowledge on the DNA metabarcoding project designing and library preparation and high-throughput sequencing. The authors would like to thank the two reviewers for their constructive criticism of the manuscript.
Conflict of interest
The authors declare that the research was conducted in the absence of any commercial or financial relationships that could be construed as a potential conflict of interest.
Publisher’s note
All claims expressed in this article are solely those of the authors and do not necessarily represent those of their affiliated organizations, or those of the publisher, the editors and the reviewers. Any product that may be evaluated in this article, or claim that may be made by its manufacturer, is not guaranteed or endorsed by the publisher.
Supplementary material
The Supplementary Material for this article can be found online at: https://www.frontiersin.org/articles/10.3389/fevo.2023.1280277/full#supplementary-material
References
Ait Baamrane M. A., Shehzad W., Ouhammou A., Abbad A., Naimi M., Coissac E., et al. (2012). Assessment of the food habits of the moroccan dorcas gazelle in M’Sabih Talaa, west central Morocco, using the trnL approach. PloS One 7 (4), e35643. doi: 10.1371/journal.pone.0035643
Alemu M. M. (2015). Effect of tree shade on coffee crop production. J. Sustain. Dev. 8 (9). doi: 10.5539/jsd.v8n9p66
Almeida-Rocha J. M., Peres C. A., Oliveira L. C. (2017). Primate responses to anthropogenic habitat disturbance: A pantropical meta-analysis. Biol. Conserv. 215, 30–38. doi: 10.1016/j.biocon.2017.08.018
Altschul S. F., Gish W., Miller W., Myers E. W., Lipman D. J. (1990). Basic local alignment search tool. J. Mol. Biol. 215 (3). doi: 10.1016/S0022-2836(05)80360-2
Ando H., Fujii C., Kawanabe M., Ao Y., Inoue T., Takenaka A. (2018). Evaluation of plant contamination in metabarcoding diet analysis of a herbivore. Sci. Rep. 8 (1). doi: 10.1038/s41598-018-32845-w
Ando H., Setsuko S., Horikoshi K., Suzuki H., Umehara S., Inoue-Murayama M., et al. (2013). Diet analysis by next-generation sequencing indicates the frequent consumption of introduced plants by the critically endangered red-headed wood pigeon (Columba janthina niten) in oceanic island habitats. Ecol. Evol. 3 (12), 4057–4069. doi: 10.1002/ece3.773
Arbonnier M. (2002). Arbres, arbustes et lianes des zones sèches d’Afrique de l’Ouest. 2. éd (Montpellier : Paris: CIRAD ; MNHN).
Barca B., Turay B. S., Kanneh B. A., Tayleur C. (2018). Nest Ecology and Conservation of Western Chimpanzees (Pan troglodytes verus) in Gola Rainforest National Park, Sierra Leone. Primate Conserv. 32.
Bessa J. (2014). Chimpanzee feeding behaviour at Caiquene-Cadique in Cantanhez National Park, Guinea-Bissau (Coimbra, Portugal: Universidade de Coimbra).
Bicca-Marques J. C., Chaves Ó.M., Hass G. (2020). Howler monkey tolerance to habitat shrinking: Lifetime warranty or death sentence? Am. J. Primatology 82 (4). doi: 10.1002/ajp.23089
Brncic T. M., Amarasekaran B., McKenna A. (2010). Sierra leone national chimpanzee census (Freetown, Sierra Leone: Tacugama chimpanzee sanctuary).
Camacho C., Coulouris G., Avagyan V., Ma N., Papadopoulos J., Bealer K., et al. (2009). BLAST+: architecture and applications. BMC Bioinf. 10 (1), 421. doi: 10.1186/1471-2105-10-421
Catarino L., Palminha A. (2018). Plano de Gestão das Florestas do Parque Nacional de Cantanhez. Inventário Florestal do Parque Nacional de Cantanhez (Bissau: Instituto da Biodiversidade e das Áreas Protegidas).
Chape S., Harrison J., Spalding M., Lysenko I. (2005). Measuring the extent and effectiveness of protected areas as an indicator for meeting global biodiversity targets. Philos. Trans. R. Soc. B: Biol. Sci. 360 (1454), 443–455. doi: 10.1098/rstb.2004.1592
Chapman C. A. (2000). “Determinants of group size in primates: the importance of travel costs,” in On the move: How and why animals travel in group. (Chicago, Illinois, USA: University of Chicago Press)
Chapman C. A., Chapman L. J. (2002). Foraging challenges of red colobus monkeys: influence of nutrients and secondary compounds. Comp. Biochem. Physiol. Part A: Mol. Integr. Physiol. 133 (3), 861–875. doi: 10.1016/S1095-6433(02)00209-X
Chapman C. A., Chapman L. J., Naughton-Treves L., Lawes M. J., Mcdowell L. R. (2004). Predicting folivorous primate abundance: validation of a nutritional model. Am. J. Primatology 62 (2), 55–69. doi: 10.1002/ajp.20006
Chapman C. A., Wrangham R. W., Chapman L. J., Kennard D. K., Zanne A. E. (1999). Fruit and flower phenology at two sites in Kibale National Park, Uganda. J. Trop. Ecol. 15, 189–211. doi: 10.1017/S0266467499000759
Chen S., Zhou Y., Chen Y., Gu J. (2018). fastp: an ultra-fast all-in-one FASTQ preprocessor. Bioinformatics 34 (17), i884–i890. doi: 10.1093/bioinformatics/bty560
Clutton-Brock T. H. (1975). Feeding behaviour of red colobus and black and white colobus in East Africa. Folia Primatologica 23 (3), 165–207. doi: 10.1159/000155671
Coissac E., Riaz T., Puillandre N. (2012). Bioinformatic challenges for DNA metabarcoding of plants and animals: BIOINFORMATIC FOR DNA METABARCODING. Mol. Ecol. 21 (8), 1834–1847. doi: 10.1111/j.1365-294X.2012.05550.x
Conklin-Brittain N. L., Wrangham R. W., Hunt K. D. (1998). Dietary response of chimpanzees and cercopithecines to seasonal variation in fruit abundance. II. Macronutrients. Int. J. primatology 19 (6), 971–998. doi: 10.1023/A:1020370119096
Davies A. G., Oates J. F. (1994). Colobine monkeys: their ecology, behaviour, and evolution (New York, N.Y: Cambridge University Press).
Davies A. G., Oates J. F., Dasilva G. L. (1999). Patterns of frugivory in three west African colobine monkeys. Int. Jounal Primatology 20 (3).
DeFries R., Hansen A., Newton A. C., Hansen M. C. (2005). Increasing isolation of protected areas in tropical forests over the past twenty years. Ecol. Appl. 15 (1), 19–26. doi: 10.1890/03-5258
Doran-Sheehy D. M., Shah N. F., Heimbauer L. A. (2006). Sympatric western gorilla and mangabey diet: re-examination of ape and monkey foraging strategies. Feeding Ecol. apes other primates, 49–72.
Drake L. E., Cuff J. P., Young R. E., Marchbank A., Chadwick E. A., Symondson W. O. C. (2022). An assessment of minimum sequence copy thresholds for identifying and reducing the prevalence of artefacts in dietary metabarcoding data. Methods Ecol. Evol. 13 (3), 694–710. doi: 10.1111/2041-210X.13780
Dunham N. T. (2017). Feeding Ecology and Dietary Flexibility of Colobus angolensis palliatus in Relation to Habitat Disturbance. Int. J. Primatology 38 (3), 553–571. doi: 10.1007/s10764-017-9965-x
Düx A., Schuenemann V. J., Gogarten J. F., De Nys H. M., Nieselt K., Mayhew M. A., et al. (2017). A15 Rapid radiation of Treponema pallidum pertenue in wild non-human primates. Virus Evol. 3 (suppl_1). doi: 10.1093/ve/vew036.014
Estrada A. (2006). “Human and non-human primate co-existence in the Neotropics: a preliminary view of some agricultural practices as a complement for primate conservation,” in Ecological and environmental anthropology (Athens, Georgia, United States: University of Georgia), 3.
Estrada A., Garber P. A., Chaudhary A. (2020). Current and future trends in socio-economic, demographic and governance factors affecting global primate conservation. PeerJ 8, e9816. doi: 10.7717/peerj.9816
Fleagle J. G. (2013). Primate adaptation and evolution. 3rd ed (Amsterdam ; Boston: Elsevier/Academic Press).
Galat-Luong A., Galat G. (2005). Conservation and survival adaptations of Temminck’s red colobus (Procolobus badius temmincki), in Senegal. Int. J. Primatology 26 (3), 585–603. doi: 10.1007/s10764-005-4367-x
Ganas J. (2009). Population status survey and monitoring of western chimpanzees (Pan troglodytes verus) in the Gola Forest Reserve, Sierra Leone (Kenema, Sierra Leone: U.S. Fish and Wildlife Service).
Gatinot B. L. (1977). Le regimé alimentaire du colobe bai au Sénégal. Mammalia 41, 373–402. doi: 10.1515/mamm.1977.41.4.373
Gatinot B. L. (1978). “Characteristics of the diet of West African red colobus,” in Recent advances in primatology (London, New York, and San Francisco: Academic Press), 253–255.
Henle K., Lindenmayer D. B., Margules C. R., Saunders D. A., Wissel C. (2004). Species survival in fragmented landscapes: where are we now? Biodiversity Conserv. 13 (1), 1–8.
Hill C. M. (2018). Crop foraging, crop losses, and crop raiding. Annu. Rev. Anthropology 47 (1), 377–394. doi: 10.1146/annurev-anthro-102317-050022
Hill M. O. (1973). Diversity and evenness: a unifying notation and its consequences. Ecology 54 (2), 427–432. doi: 10.2307/1934352
Hillyer A. P., Armstrong R., Korstjens A. H. (2015). Dry season drinking from terrestrial man-made watering holes in arboreal wild Temminck’s red colobus, The Gambia. Primate Biol. 2 (1), 21–24. doi: 10.5194/pb-2-21-2015
Hockings K. J., McLennan M. R. (2012). From forest to farm: systematic review of cultivar feeding by chimpanzees – management implications for wildlife in anthropogenic landscapes. PloS One 7 (4), e33391. doi: 10.1371/journal.pone.0033391
Hockings K. J., Parathian H., Bessa J., Frazão-Moreira A. (2020). Extensive overlap in the selection of wild fruits by chimpanzees and humans: implications for the management of complex social-ecological systems. Front. Ecol. Evol. 8. doi: 10.3389/fevo.2020.00123
Hockings K. J., Sousa C. (2013). Human-chimpanzee sympatry and interactions in cantanhez national park, Guinea-bissau: current research and future directions. Primate Conserv. 26 (1), 57–65. doi: 10.1896/052.026.0104
Hsieh TC., Ma KH., Chao A. (2016). iNEXT: an R package for rarefaction and extrapolation of species diversity (Hill numbers). Methods Ecol. Evol. 7 (12), 1451–6.
Isaac N. J., Cowlishaw G. (2004). How species respond to multiple extinction threats. Proc. R. Soc. London. Ser. B: Biol. Sci. 271 (1544), 1135–1141.
Isabirye-Basuta G. M., Lwanga J. S. (2008). Primate populations and their interactions with changing habitats. Int. J. Primatology 29 (1). doi: 10.1007/s10764-008-9239-8
Ji Y., Ashton L., Pedley S. M., Edwards D. P., Tang Y., Nakamura A., et al. (2013). Reliable, verifiable and efficient monitoring of biodiversity via metabarcoding. Ecol. Lett. 16 (10), 1245–1257. doi: 10.1111/ele.12162
Key C., Ross C. (1999). Sex differences in energy expenditure in non–human primates. Proc. R. Soc. London. Ser. B: Biol. Sci. 266 (1437), 2479–2485. doi: 10.1098/rspb.1999.0949
Kibaja M. (2014). Diet of the ashy red colobus (Piliocolobus tephrosceles) and crop-raiding in a forest-farm mosaic, mbuzi, rukwa region, Tanzania. Primate Conserv. 28 (1), 109–116. doi: 10.1896/052.028.0108
Koenig A., Borries C. (2001). Socioecology of Hanuman langurs: the story of their success. Evolutionary Anthropology: Issues News Reviews: Issues News Rev. 10 (4), 122–137.
Levins R. (1968). Evolution in changing environments: some theoretical explorations. (MPB-2) (Princeton, New Jersey, United States: Princeton University Press). doi: 10.1515/9780691209418/html
Linder J. M., Cronin D. T., Ting N., Abwe E. E., Davenport T. R., Detwiler K., et al. (2021). Red colobus (Piliocolobus) conservation action plan 2021-2026 (Gland, Switzerland: IUCN, International Union for Conservation of Nature). Available at: https://portals.iucn.org/library/node/49478.
Lyke M. M., Di Fiore A., Fierer N., Madden A. A., Lambert J. E. (2019). Metagenomic analyses reveal previously unrecognized variation in the diets of sympatric Old World monkey species. PloS One 14, ), e0218245. doi: 10.1371/journal.pone.0218245
Ma C., Fan P.-F., Zhang Z.-Y., Li J.-H., Shi X.-C., Xiao W. (2017). Diet and feeding behavior of a group of 42 Phayre’s langurs in a seasonal habitat in Mt. Gaoligong Yunnan China. Am. J. Primatology 79 (10), e22695. doi: 10.1002/ajp.22695
Maisels F., Gautier-Hion A., Gautier J. (1994). Diets of two sympatric colobines in zaire: more evidence on seed-eating in forests on poor soils. Int. Jounal Primatology 15 (5). doi: 10.1007/BF02737427
Marsh C. (1981). Diet choice among red colobus (Colobus badius rufomitratus) on the Tana River, Kenya. Folia Primatologica 35 (2–3), 147–178. doi: 10.1159/000155971
Marshall A. J., Leighton M. (2006). “How does food availability limit the population density of white-bearded gibbons?,” in Feeding ecology in apes and other primates. Ecological, physiological and behavioural aspects. Eds. Hohmann ,. G., Robbins M. M., Boesch C. (Cambridge, United Kingdom: Cambridge University Press), 313–336.
McGraw S., Gonedelé Bi S., Oates J. F. (2020). “Piliocolobus badius ssbadius,” in The IUCN red list of threatened species 2020 (Gland, Switzerland: IUCN), e.T40009A92646161.
McGraw W. S., van Casteren A., Kane E., Geissler E., Burrows B., Daegling D. J. (2016). Feeding and oral processing behaviors of two colobine monkeys in Tai Forest, Ivory Coast. J. Hum. Evol. 98, 90–102. doi: 10.1016/j.jhevol.2015.06.001
McLennan M. R., Spagnoletti N., Hockings K. J. (2017). The implications of primate behavioral flexibility for sustainable human–primate coexistence in anthropogenic habitats. Int. J. Primatology 38 (2), 105–121. doi: 10.1007/s10764-017-9962-0
Milich K. M., Stumpf R. M., Chambers J. M., Chapman C. A. (2014). Female red colobus monkeys maintain their densities through flexible feeding strategies in logged forests in Kibale National Park, Uganda: Ideal Distribution in Primates. Am. J. Phys. Anthropology 154 (1), 52–60. doi: 10.1002/ajpa.22471
Milton K. (1979). Factors influencing leaf choice by howler monkeys: a test of some hypotheses of food selection by generalist herbivores. Am. Nat. 114 (3), 362–378. doi: 10.1086/283485
Minhós T. (2012). Socio-genetics and population structure of two African colobus monkeys in Cantanhez National Park, Guinea Bissau (PhD, Cardiff, U.K: Cardiff University).
Minhós T., Chikhi L., Sousa C., Vicente L. M., da Silva M. F., Heller R., et al. (2016). Genetic consequences of human forest exploitation in two colobus monkeys in Guinea Bissau. Biol. Conserv. 194, 194–208. doi: 10.1016/j.biocon.2015.12.019
Minhós T., Nixon E., Sousa C., Vicente L. M., da Silva M. F., Sá R., et al. (2013). Genetic evidence for spatio-temporal changes in the dispersal patterns of two sympatric African colobine monkeys. Am. J. Phys. Anthropology 150 (3), 464–474. doi: 10.1002/ajpa.22223
Moorhouse-Gann R. J., Dunn J. C., de Vere N., Goder M., Cole N., Hipperson H., et al. (2018). New universal ITS2 primers for high-resolution herbivory analyses using DNA metabarcoding in both tropical and temperate zones. Sci. Rep. 8 (1). doi: 10.1038/s41598-018-26648-2
Mowry C. B., Decker B. S., Shure D. J. (1996). The role of phytochemistry in dietary choices of Tana River red colobus monkeys (Procolobus badius rufomitratus). Int. J. Primatology 17 (1), 63–84. doi: 10.1007/BF02696159
Mturi F. A. (1991). The feeding ecology and behaviour of the Red Colobus monkey. Doctoral dissertation, Ph. D. dissertation (Dar es Salaam, Tanzania: University of Dar Es Salaam)
Mturi F. A. (1993). Ecology of the Zanzibar red colobus monkey, Colobus badius kirkii (Gray, 1968), in comparison with other red colobines. (Cambridge University Press, Cambridge, United Kingdom)
Munro P., van der Horst G., Erskine G., Kebbay M., Massaquoi S. (2013). The Role of Non-Timber Forest Products (NTFPs) in Community Livelihoods around the Gola Forest National Park and Tiwai Island Wildlife Sanctuary: Current uses and future possibilities (Sierra Leone: Welthungerhilfe and the Environmental Foundation for Africa (EFA).
Myers N., Mittermeier R. A., Mittermeier C. G., Da Fonseca G. A., Kent J. (2000). Biodiversity hotspots for conservation priorities. Nature 403 (6772). doi: 10.1038/35002501
Naughton-Treves L., Treves A., Chapman C., Wrangham R. (1998). Temporal patterns of crop-raiding by primates: linking food availability in croplands and adjacent forest. J. Appl. Ecol. 35 (4), 596–606. doi: 10.1046/j.1365-2664.1998.3540596.x
Oates J. F. (1988). The diet of the olive colobus monkey, Procolobus verus, in Sierra Leone. Int. J. primatology 9 (5). doi: 10.1007/BF02736220
Oates J. F. (1994). “The natural history of African colobines,” in Colobine monkeys: their ecology behaviour and evolution (Cambridge, U.K: Cambridge University Press), 75–128.
Oates J. F., Nash S. D. (2011). Primates of West Africa: a field guide and natural history (Arlington, VA: Conservation International).
Oftedal O. T. (1991). The nutriotional consequences of foraging in primates: the relationship of nutrient intakes to nutrient requirements. Philos. Trans. R. Soc. B: Biol. Sci. 334 (1270), 161–170.
Oksanen J., Blanchet F. G., Friendly M., Kindt R., Legendre P., McGlinn D., et al. (2019). “Vegan: community ecology package (version 2.5-6),” in The comprehensive R archive network.
Onderdonk D. A., Chapman C. A. (2000). Coping with forest fragmentation: the primates of kibale national park, Uganda. Int. Jounal Primatology 21 (4), 587–611. doi: 10.1023/A:1005509119693
Pompanon F., Deagle B. E., Symondson W. O. C., Brown D. S., Jarman S. N., Taberlet (2012). Who is eating what: diet assessment using next generation sequencing: NGS DIET ANALYSIS. Mol. Ecol. 21 (8), 1931–1950. doi: 10.1111/j.1365-294X.2011.05403.x
Qin Y., Li M., Cao Y., Gao Y., Zhang W. (2017). Molecular thresholds of ITS2 and their implications for molecular evolution and species identification in seed plants. Sci. Rep. 7 (1), 17316. doi: 10.1038/s41598-017-17695-2
Querejeta M., Lefort M.-C., Bretagnolle V., Boyer S. (2020). Metabarcoding faecal samples to investigate spatiotemporal variation in the diet of the endangered Westland petrel (Procellaria westlandica). bioRxiv, 2020-10.
R Core Team (2022). R: A language and environment for statistical computing (Vienna, Austria: R Foundation for Statistical Computing).
Razgour O., Clare E. L., Zeale M. R., Hanmer J., Schnell I. B., Rasmussen M., et al. (2011). High-throughput sequencing offers insight into mechanisms of resource partitioning in cryptic bat species: Molecular Diet Analysis of Cryptic Species. Ecol. Evol. 1 (4), 556–570. doi: 10.1002/ece3.49
Robbins M. M., Hohmann G. (2006). “Primate feeding ecology: an integrative approach,” in Feeding ecology in apes and other primates: ecological, physical, and behavioral aspects (Cambridge, United Kingdom: Cambridge University Press), 1–10.
Roswell M., Dushoff J., Winfree R. (2021). A conceptual guide to measuring species diversity. Oikos 130 (3), 321–338. doi: 10.1111/oik.07202
Ryan A. M., Chapman C. A., Rothman J. M. (2013). How do differences in species and part consumption affect diet nutrient concentrations? A test with red colobus monkeys in Kibale National Park, Uganda. Afr. J. Ecol. 51 (1), 1–10. doi: 10.1111/j.1365-2028.2012.01346.x
Schloss P. D., Westcott S. L., Ryabin T., Hall J. R., Hartmann M., Hollister E. B., et al. (2009). Introducing mothur: open-source, platform-independent, community-supported software for describing and comparing microbial communities. Appl. Environ. Microbiol. 75 (23), 7537–7541. doi: 10.1128/AEM.01541-09
Schmack J. M., Lear G., Astudillo-Garcia C., Boyer S., Ward D. F., Beggs J. R. (2021). DNA metabarcoding of prey reveals spatial, temporal and diet partitioning of an island ecosystem by four invasive wasps. J. Appl. Ecol. 58 (6), 1199–1211. doi: 10.1111/1365-2664.13856
Schoch C. L., Seifert K. A., Huhndorf S., Robert V., Spouge J. L., Levesque C. A., et al. (2012). Nuclear ribosomal internal transcribed spacer (ITS) region as a universal DNA barcode marker for Fungi. Proc. Natl. Acad. Sci. 109 (16), 6241–6246. doi: 10.1073/pnas.1117018109
Siex K. S. (2003). Effects of population compression on the demography, ecology, and* behavior of the Zanzibar red colobus monkey (Procolobus kirkii) (Durham, North Carolina, United States: Duke University).
Sousa L. L., Silva S. M., Xavier R. (2019). DNA metabarcoding in diet studies: Unveiling ecological aspects in aquatic and terrestrial ecosystems. Environ. DNA 1 (3), 199–214. doi: 10.1002/edn3.27
Starin E. D. (1991). Socioecology of the red colobus monkey in the Gambia with particular reference to female-male differences and transferPattern. PhD thesis (New York: City University of New York).
Strier K. B. (2021). The limits of resilience. Primates 62 (6), 861–868. doi: 10.1007/s10329-021-00953-3
Struhsaker T. T. (2010). The red colobus monkeys: variation in demography, behavior, and ecology of endangered species (Oxford ; New York: Oxford University Press).
Struhsaker T. T., Oates J. F. (1975). Comparison of the behavior and ecology of red colobus and black-and-white colobus monkeys in Uganda: a summary. Socio-ecology Psychol. primates. Mouton Hague, 103–123. doi: 10.1515/9783110803839.103
Taberlet P., Coissac E., Hajibabaei M., Rieseberg L. H. (2012). Environmental DNA: ENVIRONMENTAL DNA. Mol. Ecol. 21 (8), 1789–1793. doi: 10.1111/j.1365-294X.2012.05542.x
Tercel M. P. T. G., Moorhouse‐Gann R. J., Cuff J. P., Drake L. E., Cole N. C., Goder M., et al. (2022). DNA metabarcoding reveals introduced species predominate in the diet of a threatened endemic omnivore, Telfair’s skink (Leiolopisma telfairii). Ecol. Evol. 12 (1). doi: 10.1002/ece3.8484
Ting N. (2008). Mitochondrial relationships and divergence dates of the African colobines: evidence of Miocene origins for the living colobus monkeys. J. Hum. Evol. 55 (2), 312–325. doi: 10.1016/j.jhevol.2008.02.011
UNEP-WCMC, & IUCN (2022) Protected planet: The world database on protected areas (WDPA). Available at: www.protectedplanet.net.
USDA N. (2015). The plants database (Greensboro: National Plant Data Team). Available at: http://plants.usda.gov.
Usongo L. I., Amubode F. O. (2001). Nutritional ecology of Preuss’s red colobus monkey (Colobus badius preussi Rahm1970) in Korup National Park, Cameroon. Afr. J. Ecol. 39, 121–125. doi: 10.1046/j.1365-2028.2000.00279.x
van Schaik C., Brockman D. K. (2005). “Seasonality in primate ecology, reproduction, and life history: An overview,” in Seasonality in primates. Eds. Brockman D. K., van Schaik C. (Cambridge: Cambridge University Press), 3–20.
van Schaik C., Pfannes K. R. (2005). “Tropical climates and phenology: A primate perspective,” in Seasonality in primates. Eds. Brockman D. K., van Schaik C. (Cambridge: Cambridge University Press), 23–54.
van Schaik C. P., Terborgh J. W., Wright S. J. (1993). The phenology of tropical forests: adaptive significance and consequences for primary consumers. Annu Rev Ecol Evol Syst. 24, 353–377. doi: 10.1146/annurev.es.24.110193.002033
Wang Y., Naumann U., Wright S. T., Warton D. I. (2012). mvabund–an R package for model-based analysis of multivariate abundance data. Methods Ecol. Evol. 3 (3). doi: 10.1111/j.2041-210X.2012.00190.x
White F. (1983). The vegetation of Africa: a descriptive memoir to accompany the Unesco/AETFAT/UNSO vegetation map of Africa (Paris: Unesco).
Wickham H., Chang W., Wickham M. H. (2016). ggplot2: create elegant data visualisations using the grammar of graphics. R package version 3.2. 1. (College Station, TX, United States: Stata Software Package).
Wilkins M. A. (2017). Interannual variation in the diets of Piliocolobus badius badius from the Taï Forest of Cote d’Ivoire. (Colombus, Ohio, United States: The Ohio State University)
Worm B., Paine R. T. (2016). Humans as a hyperkeystone species. Trends Ecol. Evol. 31 (8), 600–607. doi: 10.1016/j.tree.2016.05.008
Wrangham R. W., Conklin-Brittain N. L., Hunt K. D. (1998). Dietary response of chimpanzees and cercopithecines to seasonal variation in fruit abundance. I. Antifeedants. Int. J. Primatology 19 (6), 949–970. doi: 10.1023/A:1020318102257
Wrangham R. W., Gittleman J. L., Chapman C. (1993). Constraints on group size in primates and carnivores: population density and day-range as assays of exploitation competition. Behav. Ecol. Sociobiology 32 (3), 199–209. doi: 10.1007/BF00173778
Keywords: non-human primates, feeding ecology, DNA metabarcoding, non-invasive sampling, west Africa, Piliocolobus badius, altered habitats
Citation: Aleixo-Pais I, Borges F, Sesay N, Songe M, Cassama M, Camara IT, Ramos C, Barca B, Turay BS, Swaray M, de Barros AR, Quecuta Q, Ferreira da Silva MJ, Frazão-Moreira A, Bruford MW and Minhós T (2023) Dietary flexibility of western red colobus in two protected areas with contrasting anthropogenic pressure. Front. Ecol. Evol. 11:1280277. doi: 10.3389/fevo.2023.1280277
Received: 19 August 2023; Accepted: 23 October 2023;
Published: 14 November 2023.
Edited by:
Wei Wang, Chinese Research Academy of Environmental Sciences, ChinaReviewed by:
Valeria Torti, University of Turin, ItalyThomas Göttert, Eberswalde University for Sustainable Development, Germany
Copyright © 2023 Aleixo-Pais, Borges, Sesay, Songe, Cassama, Camara, Ramos, Barca, Turay, Swaray, de Barros, Quecuta, Ferreira da Silva, Frazão-Moreira, Bruford and Minhós. This is an open-access article distributed under the terms of the Creative Commons Attribution License (CC BY). The use, distribution or reproduction in other forums is permitted, provided the original author(s) and the copyright owner(s) are credited and that the original publication in this journal is cited, in accordance with accepted academic practice. No use, distribution or reproduction is permitted which does not comply with these terms.
*Correspondence: Isa Aleixo-Pais, isa.aleixopais@gmail.com