- 1Jilin Provincial Key Laboratory of Animal Resource Conservation and Utilization, Northeast Normal University, Changchun, China
- 2Key Laboratory of Vegetation Ecology of Education Ministry, Institute of Grassland Science, Northeast Normal University, Changchun, China
- 3Key Laboratory of Animal Physiology, Biochemistry and Molecular Biology of Hebei Province, College of Life Sciences, Hebei Normal University, Shijiazhuang, China
- 4College of Life Science, Jilin Agricultural University, Changchun, China
- 5Key Laboratory of Southwest China Wildlife Resources Conservation of Ministry of Education, China West Normal University, Nanchong, China
Animals living in a complex social environment emit diverse acoustic signals and thus serve as excellent subjects for understanding the evolution of acoustic communication. Previous studies have focused on the complexity of social calls in some group-living animals, yet the determinants of vocal complexity at the intraspecific level remain unclear. Here, we aimed to assess the influence of group composition on social call complexity in Himalayan leaf-nosed bats (Hipposideros armiger) in the non-breeding season. The bats divided into three groups with the same number of individuals but with different sex ratio compositions. We monitored social vocalizations for the all-male group, the all-female group, the mixed group, and also quantified vocal complexity for each group based on multiple acoustic metrics, including vocal repertoire, call sequences, the diversity index, and information capacity. The results showed that there were significant differences in the composition of call sequences among the three bat groups. The number of vocalizations was the highest in the mixed group, while the social call complexity was the highest in the all-male group, followed by the all-female group, and was the lowest in the mixed group. The results suggest that sex ratio potentially influence the vocal repertoire in Himalayan leaf-nosed bats. Our findings might provide a cue for vocalization research to investigate sex ratio in social groups as a potential driver for vocal complexity.
Introduction
Acoustic signals play a vital role in transmitting social information and mediating a series of life history events such as foraging and reproduction (Bradbury and Vehrencamp, 2011; Laiolo, 2012; Charlton et al., 2013; Jin et al., 2015; Kalan and Boesch, 2015). Many animals emit complex vocalizations to engage in social interactions, especially in varying ecological and social environments (Freeberg, 2006; Freeberg et al., 2012b; Bhat et al., 2022; Eleuteri et al., 2022; Naguib et al., 2022). For example, territorial meerkats (Suricata suricatta) also use contact calls, potentially to maintain social organization during foraging (Townsend et al., 2010). The duet is widely used by Barbets (Capitonidae) in mate guarding and dominance against subordinate group members (Soma and Brumm, 2020). Despite the long-term attention within the scientific community, how animals have evolved complex and diverse acoustic signals remains an open question.
Vocal complexity in animals often refers to vocalizations containing a large number of structurally and functionally distinct sound elements or possessing a high amount of information (Freeberg et al., 2012a). Vocal complexity can be measured using three indicators, i.e., repertoire size, gradation variation, and information entropy (Oller and Griebel, 2008; Freeberg et al., 2012a; Kershenbaum, 2014). Repertoire size is usually used to characterize the vocal complexity of birdsongs, as it indicates the number of discretely different vocal sequences used by an individual (Catchpole and Slater, 2003; Kershenbaum, 2014). Gradation variation refers to the variety of repertoires that convey information (Marler, 1976). Variability in call structure is another potential way by which the amount of transmitted information can be increased (Davidson and Wilkinson, 2004; Bouchet et al., 2013; Peckre et al., 2019). Moreover, information entropy or uncertainty is an important indicator used to measure the amount of information in information theory (Freeberg and Lucas, 2012). Typically, the greater the diversity of elements within a particular signaling system, the more potential information or complexity in the signaling system (Freeberg and Lucas, 2012).
Acoustic signaling has been predicted to be the result of a combination of several selective pressures, including the social environment (e.g., the number of group members, group composition, and diversity) (McComb and Semple, 2005; Freeberg et al., 2012b; Pougnault et al., 2022), ecology (e.g., predation, habitat environment) (Ouattara et al., 2009; Ord and Garcia-Porta, 2012), morphology (e.g., body size) (Podos, 2001; Charlton and Reby, 2016), and phylogeny (e.g., phylogenetic constraints) (Thinh et al., 2011; Luo et al., 2017). Current research mainly focuses on the driving forces of complex social calls in amphibians, birds, rodents, and non-human primates (Ord and Garcia-Porta, 2012; Peckre et al., 2019). Several hypotheses have been proposed, such as the social complexity hypothesis (Freeberg et al., 2012a), the ecological complexity hypothesis (Ord and Garcia-Porta, 2012), and the neutral hypothesis (Grant and Grant, 2009). Among these, the social complexity hypothesis has received widespread attention from researchers. The social complexity hypothesis suggests that complex animal communication is usually associated with the evolution of a complex society (e.g., as individuals interact more frequently in different contexts, the social signals that regulate these interactions tend to be more diverse) (Freeberg et al., 2012a). Blumstein and Armitage (1997) found that the social complexity index of 22 ground-dwelling squirrel species significantly positively correlated with alarm call repertoire size (Blumstein and Armitage, 1997). Similarly, the species experiencing more uncertain social interactions displayed greater vocal diversity and flexibility in macaques (Rebout et al., 2020).
Sex ratio, an important metric of demographic data, is the ratio of the number of male and female individuals in a population and is one of the most important characteristics that significantly affects the spousal relationship and reproductive potential ability (Kokko and Jennions, 2008; Booksmythe et al., 2017). Polygamous males in nine species of wrens (Troglodytidae) have larger song repertoires compared to monogamous counterparts (Kroodsma, 1977). However, the sex ratio did not alter the vocal complexity in Carolina chickadees (Poecile carolinensis) (Freeberg, 2006). Whether sex ratio can be used as an indicator of social complexity that affects bird vocal complexity in different species invites further study. In mammals, some studies also suggested the potential influence of sex ratio on vocal complexity. For example, the low number of females in spring increases competition between males and significantly increases the amount and diversity of vocal activity in brown long-eared bats (Plecotus auritus) (Furmankiewicz et al., 2013). However, the influence of sex ratio on vocal complexity in mammals also remains unclear.
Bats comprise the second most diverse mammalian order, which are generally gregarious and long-lived. Accordingly, bats have an increased likelihood of social interactions and need to effectively convey more diverse messages (Chaverri et al., 2018). They emit echolocation calls for navigation and localization, as well as highly complex social calls for maintaining social stability (Kanwal et al., 1994; Ma et al., 2006; Kobayasi et al., 2012; Eckenweber and Knornschild, 2013). Many bat species live in highly clustered populations, and their populations have a complex social structure with dozens to tens of thousands of individuals of different ages, sex, reproductive status, and kinship (Kerth et al., 2011). Therefore, bats serve as an ideal model for testing the social complexity hypotheses, which will ultimately improve our understanding of animal communication (Chaverri et al., 2018). A previous study found a positive relationship between the information content of vocalizations and social group size in 61 Chiroptera species (Knörnschild et al., 2020). However, there is little evidence on whether other aspects of social organization affect social calls that would suggest a relationship between social and vocal complexity in bats.
Several bat species live in fission–fusion societies (Kerth, 2008) in which group dynamics are highly seasonal, and social members interact frequently among different subgroups. In fission–fusion societies, females form summer groups where they give birth and raise their offspring, while males typically live in all-male groups (Ortega, 2016). As a result, a colony consists of multiple social groups that change in sex ratio, group size, and composition (Patriquin et al., 2010; Kerth and Van Schaik, 2012), thereby leading to different social interactions that result in differences in vocal complexity. Because of the unique intraspecies interactions, testing the social complexity hypothesis using bats as a model would likely provide insights into improving our understanding of animal communication.
Populations of the Great Himalayan leaf-nosed bat (Hipposideros armiger) have been observed in India and Nepal, central and southeastern China, and in much of peninsular Southeast Asia (Bates et al., 2020). This species typically roosts in caves where hundreds of individuals share day and night roosts (Cheng and Lee, 2004). A previous study found that H. armiger has a harem mating system and forms groups that include one male and several females (Yang, 2011). Pregnant females usually give birth to one infant each year between May and early June. The female and infant bats form separate breeding colonies, while the male bats form non-breeding colonies (Chen, 1995). Based on the composition of their colonies, the Himalayan leaf-nosed bats often live in fission–fusion societies in summer (Xiong, 1975; Chen, 1995). Juvenile female bats show higher fidelity to their natal roost than males and bats of other ages (Cheng and Lee, 2004). Moreover, this species is highly gregarious, possessing active and diverse social vocalizations with 35 distinct syllable types, including 18 simple syllable types and 17 composite types (Lin et al., 2016). They engage in antagonistic interactions daily to defend their day-roost territory through aggressive calls and visually pronounced aggressive displays (Sun et al., 2018). This species clearly exhibits extreme variation in social structure, which makes them excellent subjects for investigating the evolutionary connections between social and vocal complexity.
The goal of this study was to test whether sex ratio affects the complexity of social calls in the Himalayan leaf-nosed bats. We recorded the social calls of three different groups with the same group size but different sex ratios: all-male, all-female, and a mixture of the sexes. The complexity of social calls was measured on multiple levels, including syllables, call sequences, the diversity index (DI), and information capacity (ΣHs). Then, these variables were compared to test whether they significantly differed between groups. We predicted that the mixed group would have the highest vocal complexity, as its members may potentially play more distinct social roles. Additionally, because the complexity of male vocal signals is influenced by sexual selection in many taxa, we also predicted that the all-male group would have higher vocal complexity than the all-female group.
Materials and methods
Study subjects
From July to August 2016, 18 adult H. armiger (9 ♀, 9 ♂) were captured from a cave-dwelling population in Shanxi Province, China. The population contained more than 500 individuals by using thermal infrared cameras. Males were considered adult if they had epididymides, enlarged testes, or both; a sealed epiphyseal gap; brown fur; and worn canine cusps (Cheng and Lee, 2002). Females were considered mature if they were pregnant or showed signs of parturition or nursing (i.e., swollen, and elongated nipples, public nipples, or both) (Racey, 1988). Previous studies found that females gave birth from May to early June (Chen, 1995; Cheng and Lee, 2002). Therefore, the captured females had not given birth or completed lactation after giving birth during the experiment. The bats were captured by using mist nets at the entrance of the cave when the bats flew out of the cave to forage. Captured bats were put in cloth bags and transported to the temporary laboratory near the roosting cave of the bats (a rectangular tent, 2 m × 1.5 m × 1.8 m). The humidity was maintained at ∼60%, and the temperature was controlled at ∼23°C. To identify different individuals, we used a 4.2 mm-diameter marker ring (Porzana Ltd, Icklesham, UK) to mark the forearm of each bat. Previous studies had shown that marker rings did not alter the behavior of the bats (Kunz and Weise, 2009; Jiang et al., 2017). During the experiment, bats were fed fresh mealworms (Tenebrio molitor) with added mineral elements and vitamins and provided with water ad libitum. The bats were released in the temporary laboratory and flew freely until they could actively prey on the mealworms and drink water from the dishes on the walls. The acclimatizing process took 3 days.
Data collection
Before the recording experiments, these bats were divided into three groups including the all-male group, the all-female group, and the mixed group, and were placed in three cages (the size of each cage: 80 cm × 60 cm × 70 cm) with a mesh size of 1 cm × 1 cm, respectively. The top of the cage was covered by a cotton cloth to create a dark environment. Each group consisted of six individuals, of which the mixed group consisted of three males and three females. Infrared cameras (HDR-CX 760E; Sony Corp., Tokyo, Japan) and ultrasonic recorders (UltraSoundGate 116; Avisoft Bioacoustics, Berlin, Germany) were arranged in parallel and pointed at the bats to record their behaviors and vocalizations (Figure 1). The distance between the microphone and bats was 1 m in each recording trial to avoid variations in the sound pressure of the recorded calls. Sound recordings were set at a sampling rate of 375 kHz and 16 bits resolution. Each recording file was 60 s long. Previous studies have found that this species vocalizes most actively approximately 2 h before they leave the cave to nightly forage and during the early morning hours after they return to the cave from foraging (Lin et al., 2016; Sun et al., 2020). Therefore, recording sessions were scheduled from 19:30 in the evening to 8:30 on the next morning to include the active vocal period of the bats. Each group was recorded for the same amount of time for 10 days until no new syllables were found. After the experiment, the bats were released in their original cave.
Social calls were analyzed using the software Avisoft SASLab Pro (version 5.1; R. Specht, Avisoft Bioacoustics, Berlin, Germany). Behavioral videos were analyzed using Qvod-Player with a resolution of 25 frames/s (version 5.0.80, Shenzhen Qvod Technology Co., Ltd, Guangdong, China). We looped each individual bat, and then recorded the social calls and behaviors of the bats simultaneously. According to our observations, social calls of bats are often accompanied by body vibrations and mouth movements, and the calls are generally audible. Therefore, the individual vocalizations were determined by matching the time of the sound wave and the behavioral video, as the pattern of body or mouth movements on the video corresponded to the pattern of sounds appearing on the real-time spectrogram of the sonograph (Sun et al., 2018; Zhang et al., 2019).
Classification of syllable types
The syllable is the basic unit of social calls. A simple syllable typically consists of a single element, while a composite syllable consists of different elements with no interval between any two elements. In a previous study, Lin et al. (2016) demonstrated the structural diversity of communicative vocalizations in H. armiger and classified sounds into 35 distinct syllable types. We classified the types of syllables following the methods proposed by Kanwal et al. (1994) and Lin et al. (2016). In general, composites were named according to the combination of simple syllables without any intervening silence interval (separated by a dash) and abbreviated accordingly. We also defined some new composites that had not been previously described (Supplementary Figures 1–3). Most of the individuals emitted aggressive social calls when they were disturbed by others, either one bat approached another, or potential opponents faced each other, but did not approach (Sun et al., 2018). In addition, the calls in mild behavioral contexts were often emitted individually. Therefore, although temporal overlap occurred frequently in the recordings, there were a considerable number of calls without temporal overlap (approximately 75.34% of the call sequences had no temporal overlap). In our study, only calls with no overlap and a high signal-to-noise ratio (>40 dB) were retained for further analyses. Syllables were classified by visual inspection of the spectrograms using Avisoft-SASLab Pro (version 5.2, R. Specht, Avisoft Bioacoustics, Berlin, Germany) with a fast Fourier transform (FFT: 1024; Hamming window: 75%; frame size: 75% overlap; temporal resolution: 1.024 ms; frequency resolution: 0.244 kHz). We identified sounds based on the maximum frequency, minimum frequency, duration, number of harmonics, noisiness, spectrographic patterns, and temporal composition of constant frequency (CF), frequency modulation (FM), and noise bursts (NBs). Finally, the syllable types of each individual bat were counted. We calculated the Sorensen index of the same syllables in three groups. The formula is as follows:
Where, SI: the Sorensen index; a, b: the number of syllables of the two groups; c: The number of syllables shared by the two groups.
Analysis of call sequences
A call sequence usually consists of several syllables (the number of syllables ≥ 2). The interval between syllables is defined as the time from the end of one syllable to the start of the next. We measured the inter-syllable intervals of 5,667 syllables and obtained a distribution graph (Figure 2), which was used as the basis for identifying call sequences. The trough between peaks at an inter-syllable interval of 80 ms was used to divide the call sequences (Figure 2). If the inter-syllable interval between two adjacent syllables was more than 80 ms, the separated call sequences were identified (Figure 3). Call sequences were divided into “Single” (consisting of one syllable, inter-syllable interval > 80 ms), “Repeated” (consisting of single syllable repetition, the number of syllables ≥ 2), and “Combined” sequences (consisting of a variety of different syllable types). We counted the number and proportion of different types of call sequences in the three groups. For the three types of call sequences in each group, we analyzed the proportion of intra-individual variation (the mean of the individual standard deviations of the proportions of the three types of call sequences) and presented the proportion of each type of call sequence versus all call sequences, which includes call sequences more than 1% (Some call sequences have low emitted rates, but the number of types of sequences is shown).
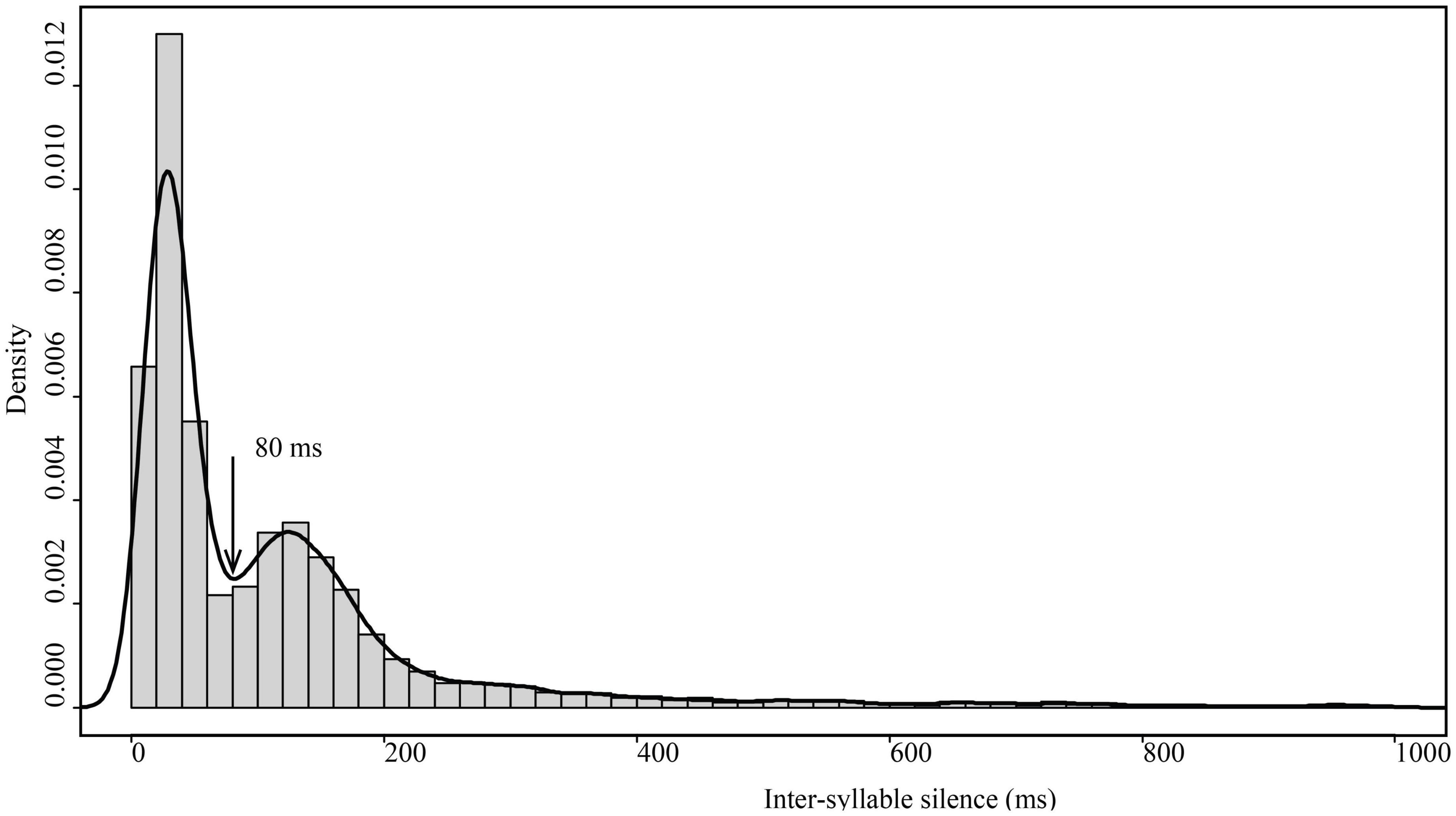
Figure 2. The distribution of inter-syllable intervals of H. armiger social calls. The solid line represents the smooth curve of the histogram. The arrow indicates the inter-syllable intervals at 80 ms, which was the threshold used to identify call sequences.
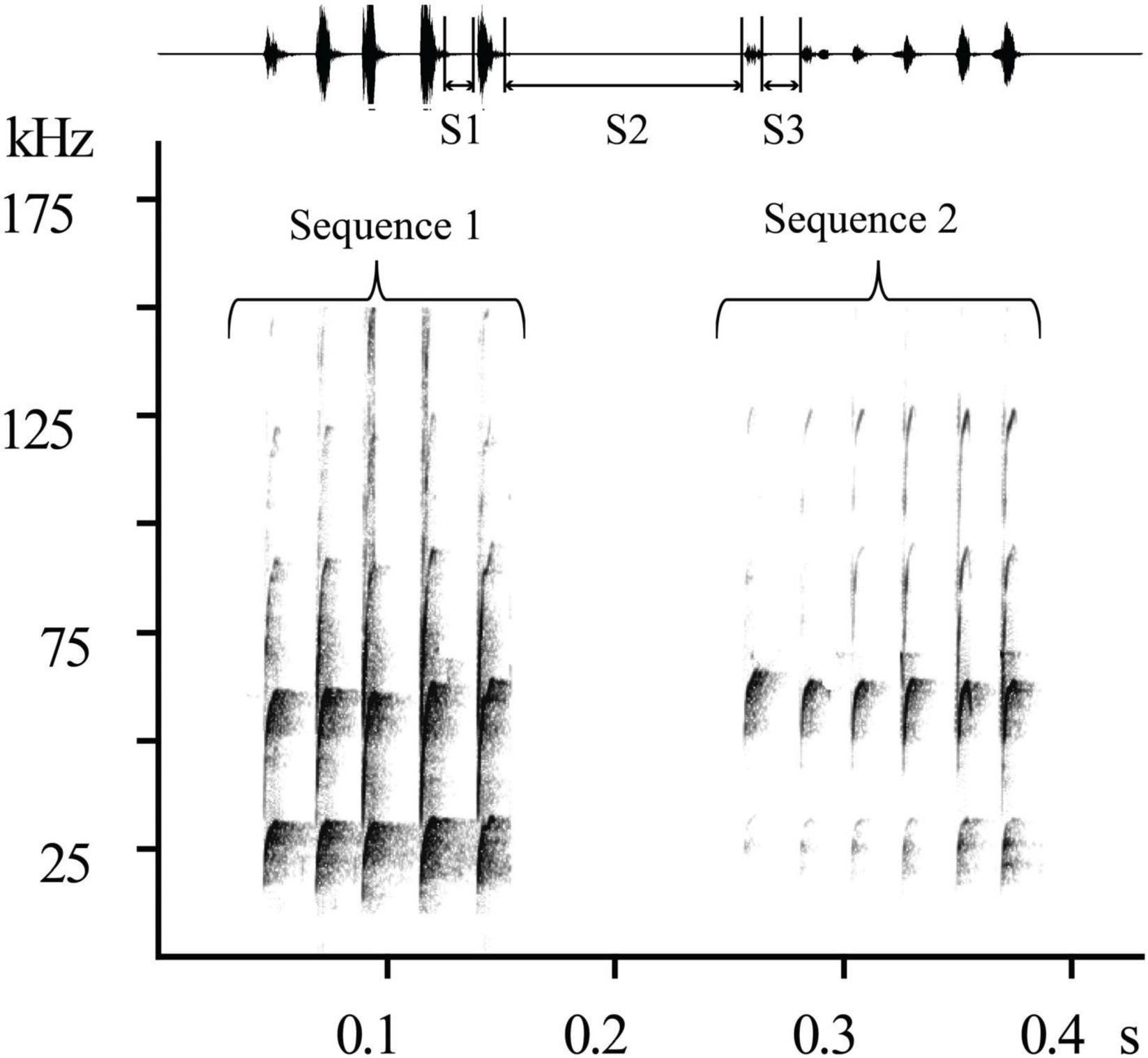
Figure 3. Schematic diagram of call sequences division. S1, S2, and S3 are the intervals between different syllables. If S2 > 80 ms > S1, S3, Sequence 1 and Sequence 2 were divided into two independent call sequences.
Analysis of vocalization diversity
To estimate the diversity of social vocalizations in bats, we calculated the diversity index following the method of Shannon and Weaver (1949). For each individual, we classified the call sequences as “Single,” “Repeated,” or “Combined” and then computed their occurrence frequency following previously described methods (Bouchet et al., 2013). We calculated the diversity index (DI) values at the group and inter-individual levels as follows:
where Hi max represents the value of all signal types uttered at the same frequency, Hi represents the actual observed values (Bouchet et al., 2013), Pi represents the occurrence frequency of each call sequence, and n represents the number of call sequence types.
Measurement of information capacity
We quantified the potential ability of coding information by measuring information capacity based on the information theory method (Shannon and Weaver, 1949; Beecher, 1989). The information content of one parameter, Hs, is a standard measure used to quantify individuality (Medvin et al., 1993; Wilkinson, 2003; Blumstein and Munos, 2005; Pollard et al., 2010). This parameter expresses a signal’s ability to reduce a receiver’s initial uncertainty about the identity of the signaler down to a minimum level (Pollard and Blumstein, 2011). Bent upward frequency modulation (bUFM) is the syllable most frequently emitted in an antagonistic context and plays an important role in H. armiger territory defense (Sun et al., 2018; Sun et al., 2020). Significant differences of the acoustic parameters of bUFM have been observed between individuals, suggesting that bUFM may encode discriminable signatures (Sun et al., 2018). Therefore, we selected bUFM as the representative syllable type for measuring information capacity. Ten bUFM syllables were randomly selected from different call sequences and measured with a standardization of 75% for each individual. Twenty-seven acoustic parameters, including the maximum frequency, minimum frequency, and bandwidth, of the second harmonic bUFM syllables were measured (Supplementary Table 1). Then, a principal components analysis (PCA) was conducted to calculate the contribution of these 27 acoustic parameters. We obtained six principal components (PCs) for the all-female and mixed groups and five PCs for the all-male group (Supplementary Table 2). Prior to statistical analysis, we tested the normal distribution of the data. If the data were not normal, we added 10 to the factor scores and then performed a log10 transformation until the data were normally distributed. A one-way analysis of variance (ANOVA) was conducted using the factor scores to obtain the F values (P < 0.05). Then, the Hs information was calculated using the following formula (Beecher, 1989; Sebe et al., 2010):
where F is the ANOVA result, n is the number of syllables, and k is the number of bats. The higher the Hs value, the greater the coding potential of the individual.
Statistical analysis
Kolmogorov-Smirnov tests were used to confirm whether all variables were normally distributed (P > 0.05). A one-way ANOVA was used to test whether the total number of call sequences and the number of each call sequences type of “Single,” “Repeated,” and “Combined differed among the three groups. Chi-square tests were conducted to compare the proportion of each type of “Single,” “Repeated,” and “Combined” sequences in the three groups. A one-way ANOVA was used to test significant differences between the diversity index (DI) values across the three groups. The basic sample units of all the ANOVA analysis were individual bats. Fisher’s least significant difference (LSD) post-hoc multiple comparison tests were employed to examine whether the diversity index (DI) values significantly differed between any two groups. All statistical analyses were conducted using SPSS version 20.0 (SPSS Inc., Chicago, IL, USA).
Results
A total of 1718 min of sound wave files were obtained from three groups (nine females and nine males). The sounds were divided into syllables, and call sequences were based on the distribution of inter-syllable intervals (Figure 2). We obtained a total of 1,552 call sequences from the all-male group (mean ± SD: 258.7 ± 136.6), 1,453 from the all-female group (242.2 ± 129.2), and 4,419 from the mixed group (736.5 ± 290.9). Then, the syllable types, call sequences, diversity index, and information capacity were compared between the three groups.
Types of syllables
We classified a total of 62 syllable types in the social vocalizations of the three groups (Figure 4 and Supplementary Figures 1–3, Supplementary Table 3). There were 31 types in the all-male group, including 15 single syllables and 16 composites, 38 in the all-female group, including 17 single syllables and 21 composites, and 40 in the mixed group, including 17 single syllables and 23 composites. A total of 18 types were shared among the three groups, including 13 single syllables and five composites (Figure 4). The syllable types in the all-male group and the all-female group were the most similar as revealed by the Sorensen Index (0.637). Both groups were less similar to the mixed group: 0.589 similarity between the mixed group and the all-female group and 0.563 between the mixed group and the all-male group. Since most of the single syllable types were shared among the three groups, the vocalization differences between the three groups are likely due to variations in the composites (Supplementary Table 4).
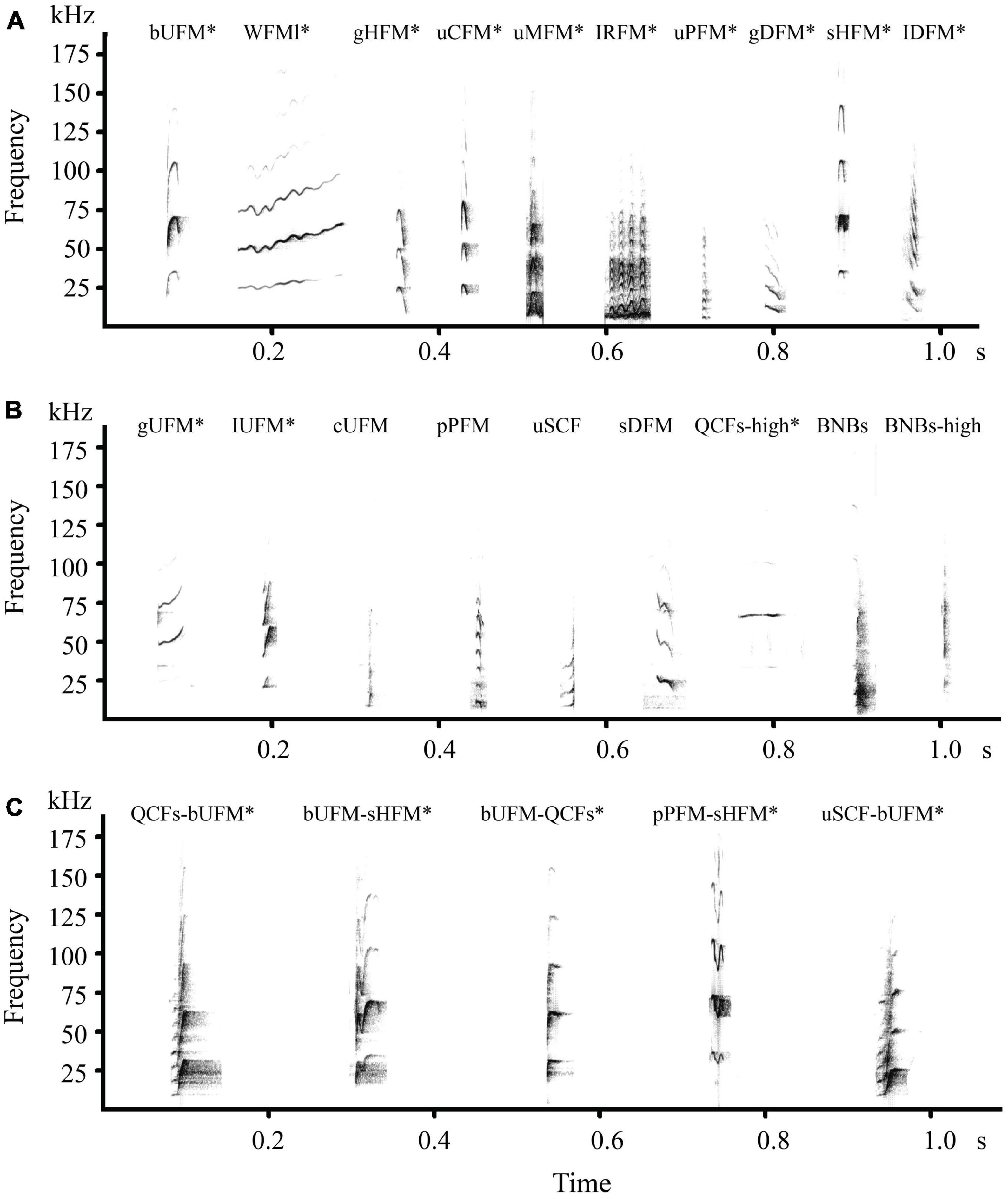
Figure 4. Sound spectrograms of syllable types of Hipposideros armiger. (A,B) All the single syllables in the vocal repertoire of the three groups; (C) composites shared in the three groups; *Syllable types shared by the three groups. Other composites are shown in the Supplementary Figures 1–3, Supplementary Table 3. bUFM, bent upward FM; WFMl, long duration, wrinkled FM; gHFM, gliding humped FM; uCFM, upward chevron FM; uMFM, upward mexican-hat FM; IRFM, linear rippled FM; uPFM, upward paraboloid FM; gDFM, gliding downward FM; sHFM: single humped FM; IDFM, linear downward FM; gUFM, gliding upward FM; IUFM, linear upward FM; cUFM, checked upward FM; pPFM, plateaued paraboloid FM; uSCF, upward short CF; sDFM, stepped downward FM; QCFs-high, short duration, high-frequency quasi-CF; BNBs, short broadband noise burst; BNBs-high, short broadband, high-frequency noise burst; QCFs-bUFM, quasi CF-bent upward FM; bUFM-sHFM, bent upward FM-single humped FM; bUFM-QCFs, bent upward FM-quasi-CF; pPFM-sHFM, plateaued paraboloid FM-single humped FM; uSCF-bUFM, upward short CF-bent upward FM.
Call sequences
After distinguishing different syllable types and measuring inter-syllable intervals, we categorized the social calls into three types of call sequences: “Single,” “Repeated,” and “Combined.” The intra-individual variation in the proportions of the three groups was 0.3489 in the mixed group, 0.2759 in the all-female group, and 0.2515 in the all-male group. There were no significant differences detected in the total number of call sequence types between the three groups (df = 2, F = 0.268, P = 0.769). For the number of each type of call sequences among the three groups, no significant differences were detected for the “Single (df = 2, F = 1.622, P = 0.230),” “Repeated (df = 2, F = 0.690, P = 0.517),” or “Combined (df = 2, F = 0.132, P = 0.877)” sequences. There were fewer “Single” and “Repeated” sequences than “Combined” sequences, which comprised the greatest proportion of sequence types among the three groups (Table 1).
Chi-square tests were performed to compare the proportion of each type of call sequence between the three groups (Figure 5). The proportion of “Single” sequences significantly differed between the three groups (χ2 = 69.278, df = 2, P < 0.001) and was the highest in the all-female group (0.185). The proportion of “Repeated” sequences also significantly differed between the three groups (χ2 = 1.634, df = 2, P < 0.001) and was the highest in the mixed group (0.702). The proportion of “Combined” sequences significantly differed between the three groups as well (χ2 = 13.816, df = 2, P < 0.001) and was the highest in the all-male group (0.209).
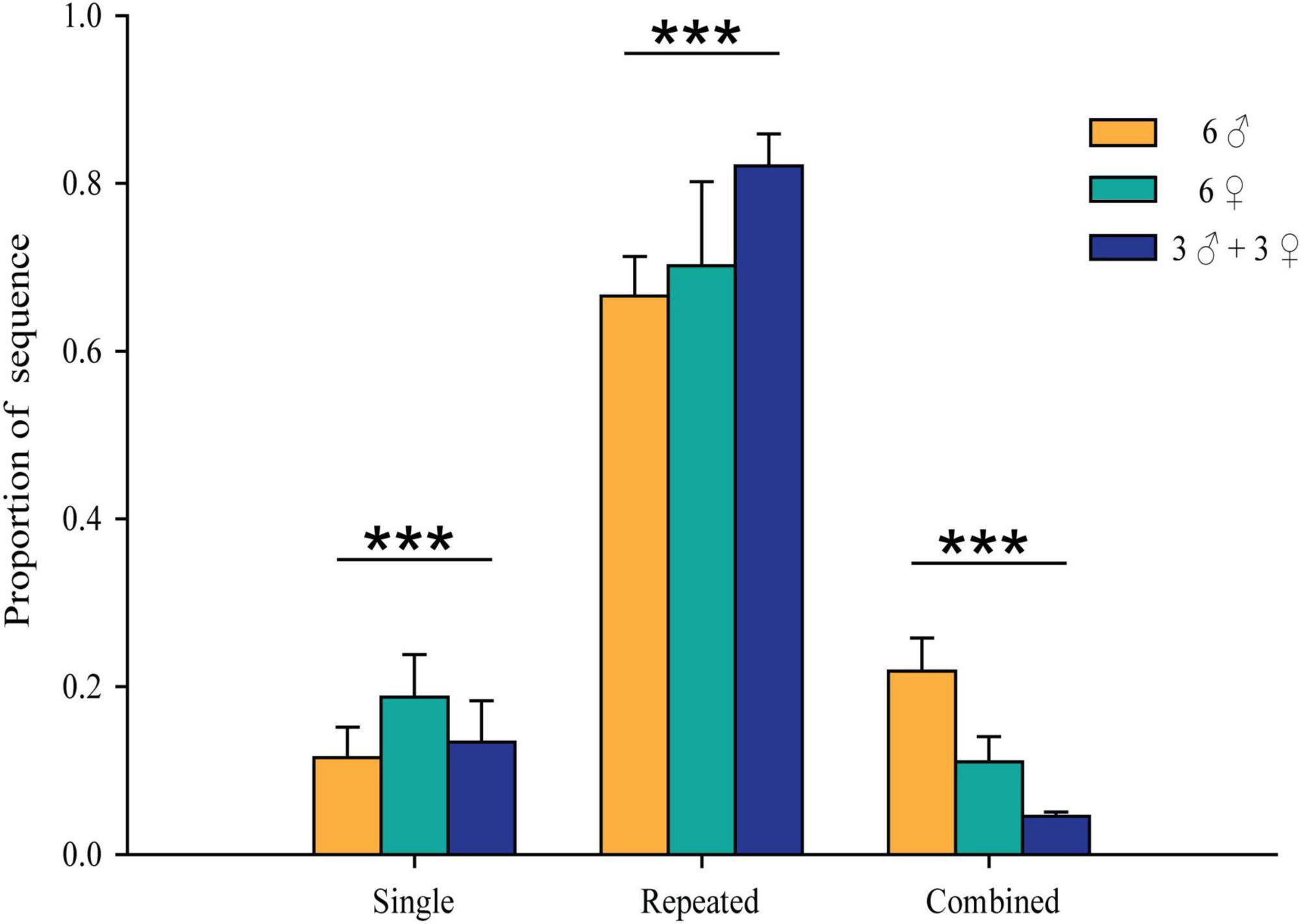
Figure 5. Proportions of the three types of social call sequences among the three Hipposideros armiger groups composed of different sex ratios. ***P < 0.001.
We also compared the proportion of call sequences with the same composition for each type of call sequence among the three groups. The proportion of call sequences composed of WFMl was the highest in the “Single” sequences, bUFMbUFM… was the highest in the “Repeated” sequences, and sHFMsHFM… bUFMbUFM… were the highest in the “Combined” sequences (Figure 6). Moreover, bUFMbUFM… occupied the greatest proportion of sequence types at 67.72, 69.79, and 83.14% in the all-male, all-female, and mixed groups, respectively (Figure 6).
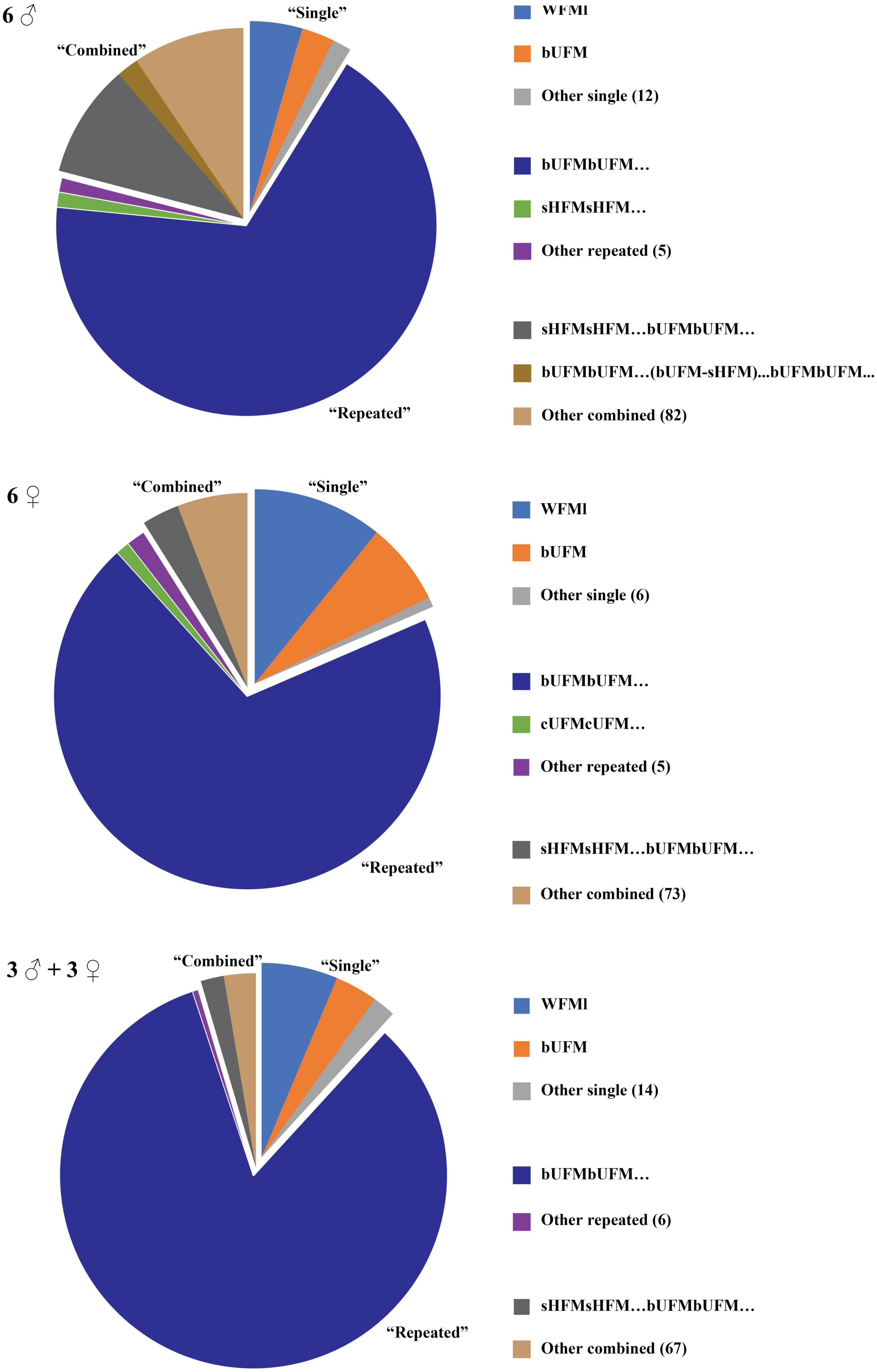
Figure 6. Proportion of call sequence types among the three groups. Sequences with occurrence frequencies >1% are represented in the figure. “Other” indicates sequences with frequencies <1%. Numbers in parentheses indicate the number of sequence types.
The diversity index of social vocalization
We calculated the diversity index (DI) values of social vocalizations based on the three types of call sequences. Interestingly, the diversity index (DI) value of the all-male group was the highest (0.718), followed by the all-female group (0.692) and the mixed group (0.493). Significant differences were detected among the diversity index (DI) values between the three groups at the individual level (F = 5.379, df = 2, P = 0.017). Fisher’s LSD also detected significant differences (P = 0.007) between the all-male (DI = 0.713 ± 0.052) and mixed groups (DI = 0.495 ± 0.060) and significant differences (P = 0.025) between the all-female (DI = 0.671 ± 0.034) and mixed groups (Figure 7).
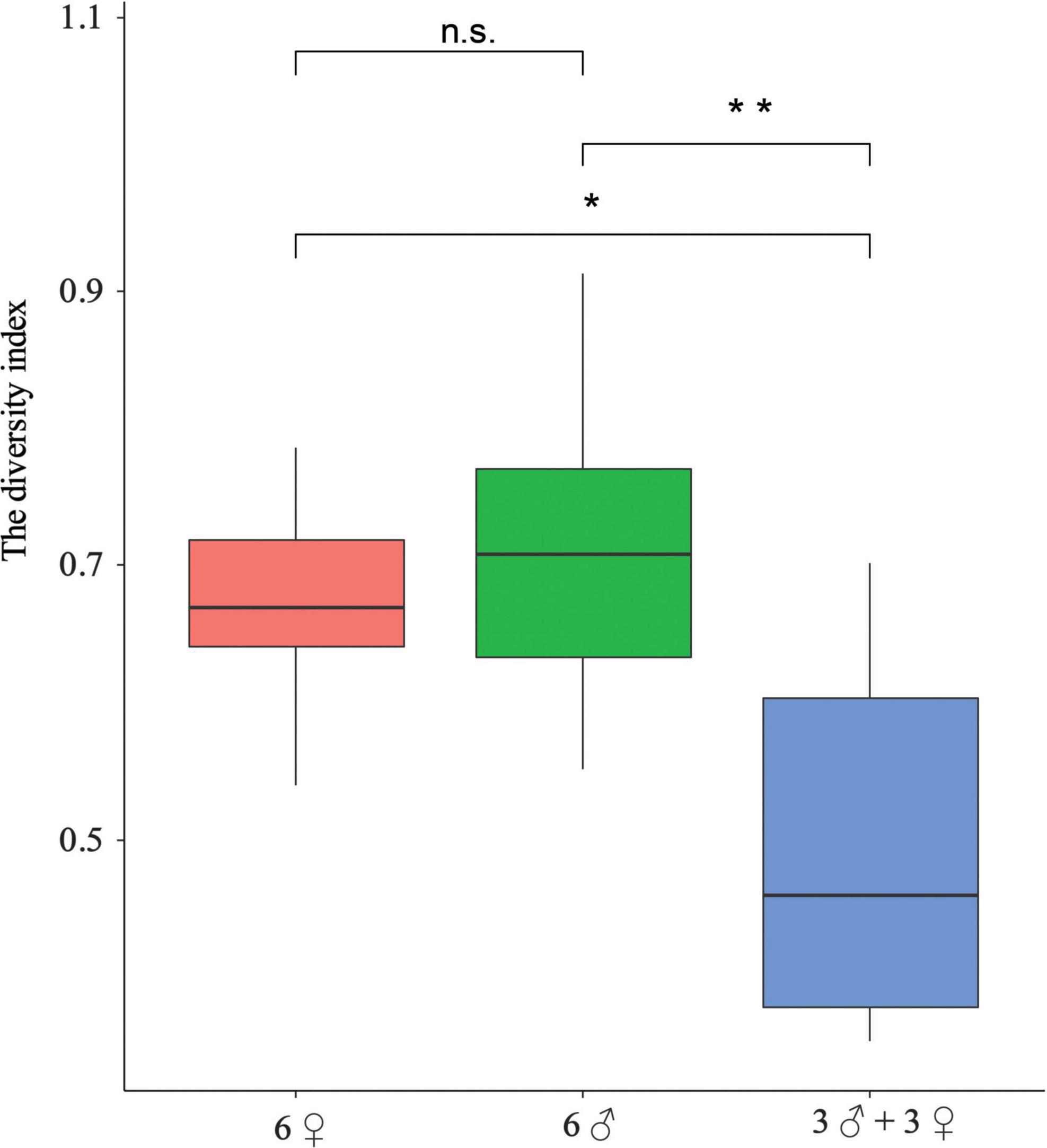
Figure 7. Comparison of the diversity index (DI) of social calls between the three groups. ***P < 0.001; **P < 0.01; *P < 0.05; n.s., non-significant.
Measurement of information capacity
We measured the acoustic parameters of the second harmonic bUFM syllable to calculate information capacity. The mixed group had the lowest value at 0.958415, the all-female group was 2.700728, and the all-male group had the highest value at 3.365663. These results were consistent with the diversity index values, suggesting that the all-male group had the greatest potential for coding information in social vocalizations, followed by the all-female group and the mixed group, which had the lowest coding potential.
Discussion
The present study investigated on the effect of sex ratio on the vocal complexity of Hipposideros armiger. By comparing multiple levels of acoustic metrics, the results showed the variations and differences of the acoustic metrics at multiple levels including syllables, call sequences, diversity index and information capacity among the three groups with different sex ratios. The results suggest that the sex ratio potentially affects the vocal complexity of H. armiger. Most previous studies that investigated the correlation between the vocal complexity and social structure have focused on different social organizations at the interspecies level (Kroodsma, 1977; Baker, 1984; Bouchet et al., 2013; Rebout et al., 2020). Our study verifies that sex ratio, as a representative metric of social organization, plays an important role in animal communication at the intraspecies level. The study invites future studies on the driving forces of vocal complexity in mammals to consider both common key metrics (e.g., social size and density) and social organization.
Interestingly, our results demonstrated that the all-male group had the highest diversity index values and information capacity of the vocal repertoires, while the mixed group had the lowest for both variables. This supported that the all-male group emits the most complex social calls. The diversity index (DI) represents the uniformity and variation of social vocalization. Our results confirmed that the proportions of the three types of call sequences in the all-male group were more uniform than those of the other groups, where the intra-individual variation values of the proportions of the three types of call sequences were 0.3489 in the mixed group, 0.2759 in the all-female group, and 0.2515 in the all-male group. Along with complex vocalizations, the male bats showed many territorial and aggressive behaviors. Previous studies indicated that bats in a male-male combination exhibited more aggressive and territorial behaviors for territorial competition or to establish a dominance hierarchy, along with a display of diverse and complex vocalizations. For example, H. armiger males were observed to use various and multiple vocalizations in antagonistic interactions (Sun et al., 2018). Similarly, Seba’s short-tailed fruit bat (Carollia perspicillata) males used different vocalization types with sufficient variation to encode individual signatures during aggressive displays (Fernandez et al., 2014). These phenomena were also detected in two Oreobates species (Akmentins, 2011). In addition, individual signatures of acoustic signals as a type of informational capacity have been studied in several bat species, such as the territorial trills and courtship calls of Seba’s short-tailed bat (C. perspicillata) (Fernandez et al., 2014; Knörnschild et al., 2014), the territorial songs of S. bilineata (Davidson and Wilkinson, 2002), and the inquiry calls and response calls of Spix’s disk-winged bat (Thyroptera tricolor) (Chaverri and Gillam, 2013). In general, the information encoded by acoustic parameters is related with the individual characteristics and emotional state of the sender (Morton, 1977; Morton, 1982; Elie et al., 2011). It was discovered that bUFM encoded different information potentials in aggressive circumstances, i.e., H. armiger increased the frequency bandwidth but reduced the minimum frequency within the syllable as aggression intensity increased (Sun et al., 2018). Moreover, there is experimental evidence that bUFM calls may serve the function of territorial defense (Zhang et al., 2021). In this study, we found that the all-male group had the highest information capacity, and it has been speculated that the emotional state of the vocalizers may play an important role, as the all-male group showed more frequent aggressive behaviors such as boxing and pushing than the other two groups. This is consistent with group membership of different conspecific social groups possibly being encoded in the acoustic structure of vocalizations in primates (Toshiaki et al., 2006; Crockford et al., 2010).
Social competition is one of the driving forces of the evolution of vocal complexity. It has been found in animal groups such as mammals (Fernández-Vargas, 2018), birds (Dalziell and Welbergen, 2016), and lissamphibians (Zhu et al., 2017). In natural groups, competition between males and competition between females can lead to aggressive behavior and may promote complex calls. In this study, the all-female group showed many territorial and aggressive behaviors along with abundant vocalizations as well. In highly clustered bat colonies, females encounter many competitive pressures, including limited habitats and food resources. For example, female Asian particolored bats (Vespertilio sinensis) frequently compete for central roost spots in nursery colonies and emit aggressive vocalizations (Zhao et al., 2019). Our results suggested that intense social interactions with complex vocalizations also exist in female Himalayan leaf-nosed bats. The correlation between higher vocal complexity and aggressive levels in the all-male and all-female groups suggest that aggression may facilitate complex social vocalizations, which is consistent with previous studies.
The proportion and number of “Combined” sequences in the all-male group in this study were significantly higher than those in the other groups (Figure 4), suggesting that male bats may have a stronger ability to switch between different syllables and combine more complex social call sequences. This result corresponds with the findings in other taxa, including primates (Arnold and Zuberbühler, 2006; Ouattara et al., 2009; Cäsar et al., 2013), birds (Engesser et al., 2016; Suzuki et al., 2016), and dwarf mongooses (Helogale parvula) (Collier et al., 2020), as well as with a previous study that observed “Combined” sequences in greater horseshoe bats (Rhinolophus ferrumequinum) that encountered aggressive or distressing circumstances (Zhang et al., 2019). In H. armiger, a previous study also found that vocalizations of “Combined” sequences were frequently emitted during antagonistic interactions, such as when a bat was bit or attacked suddenly by another bat (Sun et al., 2018). The functions of “Combined” vocalizations have been documented in other animal taxa. For example, serrate-legged small treefrogs (Kurixalus odontotarsus) produce more compound calls to attract females and suppress rivals (Zhu et al., 2017). Bocon toadfish (Amphichthys cryptocentrus) emit compound calls with broadband and tonal components to transmit individual selection-linked information to females (Staaterman et al., 2018). Mountain (Gorilla beringei beringei) and western gorillas (Gorilla gorilla gorilla) combine acoustic units into compound vocalizations to constitute a form of phonological syntax to transmit more messages (Hedwig et al., 2015). Our study provides an extra potential function of the combinatoriality in animal communication (i.e., “Combined” sequences play important roles in intense social interactions among H. armiger males). From the results, we speculate that the syntax may exist in H. armiger social vocalizations, but this requires further investigation.
Nevertheless, “Repeated” sequences occurred more frequently in the vocal repertoires of H. armiger, where the occurrence frequencies of “Repeated” sequences were 66.58, 70.19, and 82.05% in the all-male, all-female, and mixed groups, respectively. Similar findings have been observed in a broad range of animal taxa, but the function of repeated calls varies across species and contexts, such as the interactive calling of male American green tree frogs (Hyla cinerea) and family-specific calls of stripe-backed wrens (Campylorhynchus nuchalis) (Klump and Gerhardt, 1992; Price, 1999). Additionally, repeated call sequences of long-finned pilot whales (Globicephala melas) were suggested to maintain contact and cohesion, as well as possibly play a role in individual or group identification (Zwamborn and Whitehead, 2017). Single syllable repetitive calls are the most probable sequence form of greater horseshoe bats (R. ferrumequinum) under aggressive background (Zhang et al., 2019). Call repetition may increase the possibility of being detected or suggest higher behavioral intensity (Ficken et al., 1994; Moors and Terhune, 2004). In our study, H. armiger tended to use “Repeated” sequences in antagonistic interactions. In a previous study, it was discovered that bUFM sequences encoded different information potentials in aggressive circumstances (Sun et al., 2018). In aggressive context, repeated calls can increase the intensity of a signal display and may convey stronger emotional states (Payne and Pagel, 1997). Call repetition is a type of redundancy used to reduce the masking of signals from background noise or calls of other individuals (Brumm and Slater, 2006). Therefore, the “Repeated” sequences observed in this study may indicate better co-ordination in radical conflicts to obtain more roosting space during competition, as well as possibly serve as a social signature.
We did not classify specific behavioral context, but we do have some interesting behavioral observations (unpublished observations). For example, under a mild behavioral context, such as sleep awakening and grooming behaviors, pure-tone WFML occurred most in the three groups, while bUFMbUFM… sequences occurred most in the low-level aggressive behaviors, and multi-syllable combination sequences occurred most in the high-level aggressive behaviors. We assume that the phenomena may follow the motivation-structure hypothesis, although behavioral studies are needed to confirm the social significance of syllables. WFML is a pure tone with small amplitude and usually does not elicit activity from other bats, presumably being a soft appeasement call. bUFM is the most frequently emitted call, and a previous study suggested that bUFM calls may serve the function of territorial defense (Zhang et al., 2021). Additionally, multiple syllable calls are emitted by bats that have been bitten by another bat in aggressive contexts in H. armiger (Lin et al., 2016; Sun et al., 2018), and we speculate that they may indicate intense conflict between individuals.
However, some limitations still exist in the present study. First, there are individual differences in vocal output. Especially in all-male group and mixture group, two male individuals produced more vocalizations than others (Table 1). Some studies have shown that vocal output in animals depends on (1) population density (more individuals not only calling at higher densities but also more frequently) (Martínez and Zuberogoitia, 2003; Nijman, 2007; Laiolo and Tella, 2008); (2) annual cycle stage (with animals calling more frequently during the mating and/or the breeding season) (Zuberogoitia, 2011; Mori et al., 2014); and (3) environmental conditions (e.g., rain and wind (Francis et al., 2011a,b). In addition, individual biological attributes, such as sex and age, also have important effects on vocalizations, which have been found in birds (Zuberogoitia et al., 2019), bats (Habersetzer and Marimuthu, 1986) and primates (De Gregorio et al., 2021). Furthermore, social attributes could influence the vocal output. The males produce more courtship sounds than losers and show significantly shorter courtship latencies and longer courtship durations in the cichlid fish Oreochromis mossambicus (Amorim and Almada, 2005). Male Saccopteryx bilineata with a higher reproductive success trend to utter more territorial songs per day (Behr et al., 2006). In this study, the two male individuals with more vocalizations might be the dominant individuals in the groups, but it needs further study. Future research could be conducted to investigate the interactions between males of different social status during the mating season, and more samples are needed to improve the generalizability of the results in future studies.
In addition, the experiments were conducted with artificial experimental design because it is difficult to classify individual calls in a natural population with hundreds of individuals. Social vocalizations were recorded from Himalayan leaf-nosed bats maintained in captive research colonies. Furthermore, we observed two types of aggressive behavior, consistent with previous research (Sun et al., 2018). One is the non-physical contact behavior background, or ritualized radical behavior, which emits many bUFM repeated sequences. The second is the aggressive context of physical contact, which produces a lot of multi-syllable combination sequences. With the development of technological equipment, especially some small portable sound recorders, future research could record more natural social calls in the field habitat, which could help us understand the evolution of animal acoustic communication.
Overall, we assessed the vocal complexity of different social organizations of H. armiger at the intraspecific level and found that bat groups composed of the same sex produced more complex vocalizations than the mixed sex group. The results suggested that sex ratio as a potential driver of the vocal complexity of bats. Therefore, we suggest that the effects of multiple social factors should be comprehensively considered in social complexity research. Bats are a highly social taxa with many complex and unique social behaviors, the present study provides cues for vocalization research to consider social organization, social structure, and mating and breeding systems to explore and interpret the evolutionary processes of vocal complexity.
Data availability statement
The original contributions presented in this study are included in the article/Supplementary material, further inquiries can be directed to the corresponding authors.
Ethics statement
This animal study was reviewed and approved by Northeast Animal Research Authority of Northeast Normal University.
Author contributions
HG, TJ, JF, BL, and YL participated in the study design and data analysis. HG, CS, XZ, and LG collected the data in the field and laboratory. HG drafted the manuscript. YL revised the manuscript. All authors read and approved the final version.
Funding
This research was supported by the National Natural Science Foundation of China (Grant Nos.: 32171484 and 32192424).
Acknowledgments
We thank Biye Shi and Yanping Yu for their assistance in obtaining the data and collecting bats in the field. We also thank LetPub (http://www.letpub.com) for providing linguistic assistance during the preparation of this manuscript.
Conflict of interest
The authors declare that the research was conducted in the absence of any commercial or financial relationships that could be construed as a potential conflict of interest.
Publisher’s note
All claims expressed in this article are solely those of the authors and do not necessarily represent those of their affiliated organizations, or those of the publisher, the editors and the reviewers. Any product that may be evaluated in this article, or claim that may be made by its manufacturer, is not guaranteed or endorsed by the publisher.
Supplementary material
The Supplementary Material for this article can be found online at: https://www.frontiersin.org/articles/10.3389/fevo.2023.955540/full#supplementary-material
References
Akmentins, M. S. (2011). Vocal repertoire of two species of Oreobates Jimenez de la Espada, 1872 (Anura: Strabomantidae) of the Yungas Andean Forest, NW Argentina. J. Nat. Hist. 45, 1789–1799. doi: 10.1080/00222933.2011.560967
Amorim, M. C. P., and Almada, V. C. (2005). The outcome of male-male encounters affects subsequent sound production during courtship in the cichlid fish Oreochromis mossambicus. Anim. Behav. 69, 595–601. doi: 10.1016/j.anbehav.2004.06.016
Arnold, K., and Zuberbühler, K. (2006). Language evolution: semantic combinations in primate calls. Nature 441:303. doi: 10.1038/441303a
Baker, C. (1984). Social care behaviour of captive slender mongooses (Herpestes sanguineus). Mammalia 48, 43–52. doi: 10.1515/mamm.1984.48.1.43
Bates, P. J. J., Bumrungsri, S., Francis, C., Csorba, G., and Oo, S. S. L. (2020). Hipposideros armiger. The IUCN Red List of Threatened Species version 2020. 2(2307-8235 (online)). doi: 10.2305/IUCN.UK.2020-2.RLTS.T10110A22097743.e
Beecher, M. D. (1989). Signalling systems for individual recognition: an information theory approach. Anim. Behav. 38, 248–261. doi: 10.1016/S0003-3472(89)80087-9
Behr, O., Von Helversen, O., Heckel, G., Nagy, M., Voigt, C. C., and Mayer, F. (2006). Territorial songs indicate male quality in the sac-winged bat Saccopteryx bilineata (Chiroptera, Emballonuridae). Behav. Ecol. 17, 810–817. doi: 10.1093/beheco/arl013
Bhat, A. S., Sane, V. A., Seshadri, K. S., and Krishnan, A. (2022). Behavioural context shapes vocal sequences in two anuran species with different repertoire sizes. Anim. Behav. 184, 111–129. doi: 10.1016/j.anbehav.2021.12.004
Blumstein, D. T., and Armitage, K. B. (1997). Does sociality drive the evolution of communicative complexity? A comparative test with ground-dwelling sciurid alarm calls. Am. Nat. 150:179. doi: 10.1086/286062
Blumstein, D. T., and Munos, O. (2005). Individual, age and sex-specific information is contained in yellow-bellied marmot alarm calls. Anim. Behav. 69, 353–361. doi: 10.1016/j.anbehav.2004.10.001
Booksmythe, I., Mautz, B., Davis, J., Nakagawa, S., and Jennions, M. D. (2017). Facultative adjustment of the offspring sex ratio and male attractiveness: a systematic review and meta-analysis. Biol. Rev. 92, 108–134. doi: 10.1111/brv.12220
Bouchet, H., Blois-Heulin, C., and Lemasson, A. (2013). Social complexity parallels vocal complexity: a comparison of three non-human primate species. Front. Psychol. 4:390. doi: 10.3389/fpsyg.2013.00390
Bradbury, J. W., and Vehrencamp, S. L. (2011). Principles of animal communication, Second Edn. Sunderland: Sinauer Associates.
Brumm, H., and Slater, P. J. B. (2006). Ambient noise, motor fatigue, and serial redundancy in chaffinch song. Behav. Ecol. Sociobiol. 60, 475–481. doi: 10.1007/s00265-006-0188-y
Cäsar, C., Zuberbühler, K., Young, R. J., and Byrne, R. W. (2013). Titi monkey call sequences vary with predator location and type. Biol. Lett. 9:20130535. doi: 10.1098/rsbl.2013.0535
Catchpole, C. K., and Slater, P. J. B. (2003). Bird song: biological themes and variations. Cambridge: Cambridge University Press.
Charlton, B. D., and Reby, D. (2016). The evolution of acoustic size exaggeration in terrestrial mammals. Nat. Commun. 7:12739. doi: 10.1038/ncomms12739
Charlton, B. D., Frey, R., McKinnon, A. J., Fritsch, G., Fitch, W. T., and Reby, D. (2013). Koalas use a novel vocal organ to produce unusually low-pitched mating calls. Curr. Biol. 23, R1035–R1036. doi: 10.1016/j.cub.2013.10.069
Chaverri, G., Ancillotto, L., and Russo, D. (2018). Social communication in bats. Biol. Rev. 93, 1938–1954.
Chaverri, G., and Gillam, E. H. (2013). Sound amplification by means of a horn-like roosting structure in Spix’s disc-winged bat. Proc. R. Soc. B Biol. Sci. 280:20132362. doi: 10.1098/rspb.2013.2362
Chen, S. (1995). Activity pattern and food habit of sympatric Formosan leaf-nosed bat (Hipposideros armiger) and Formosan horseshoe bat (Rhinolophus monoceros) in Yangmingshan area. Master’s thesis. Taipei: National Taiwan University.
Cheng, H. C., and Lee, L. L. (2002). Postnatal growth, age estimation, and sexual maturity in the Formosan leaf-nosed bat (Hipposideros terasensis). J. Mammal. 83, 785–793. doi: 10.1644/1545-15422002083<0785:PGAEAS<2.0.CO;2
Cheng, H. C., and Lee, L. L. (2004). Temporal variations in the size and composition of Formosan leaf-nosed bat (Hipposideros terasensis) colonies in Central Taiwan. Zool. Stud. 43, 787–794.
Collier, K., Radford, A. N., Stoll, S., Watson, S. K., Manser, M. B., Bickel, B., et al. (2020). Dwarf mongoose alarm calls: investigating a complex non-human animal call: dwarf mongoose complex alarm calls. Proc. R. Soc. B Biol. Sci. 287:20192514. doi: 10.1098/rspb.2019.2514
Crockford, C., Herbinger, I., Vigilant, L., and Boesch, C. (2010). Wild chimpanzees produce group-specific calls: a case for vocal learning? Ethology 110, 221–243.
Dalziell, A. H., and Welbergen, J. A. (2016). Elaborate mimetic vocal displays by female superb lyrebirds. Front. Ecol. Evol. 4:34. doi: 10.3389/fevo.2016.00034
Davidson, S. M., and Wilkinson, G. S. (2002). Geographic and individual variation in vocalizations by male Saccopteryx bilineata (Chiroptera: Emballonuridae). J. Mammal. 83, 526–535. doi: 10.1644/1545-15422002083<0526:GAIVIV<2.0.CO;2
Davidson, S. M., and Wilkinson, G. S. (2004). Function of male song in the greater white-lined bat, Saccopteryx bilineata. Anim. Behav. 67, 883–891.
De Gregorio, C., Carugati, F., Estienne, V., Valente, D., Raimondi, T., Torti, V., et al. (2021). Born to sing! Song development in a singing primate. Curr. Zool. 67, 597–608. doi: 10.1093/cz/zoab018
Eckenweber, M., and Knornschild, M. (2013). Social influences on territorial signaling in male greater sac-winged bats. Behav. Ecol. Sociobiol. 67, 639–648. doi: 10.1007/s00265-013-1483-z
Eleuteri, V., Henderson, M., Soldati, A., Badihi, G., Zuberbühler, K., and Hobaiter, C. (2022). The form and function of chimpanzee buttress drumming. Anim. Behav. 192, 189–205. doi: 10.1016/j.anbehav.2022.07.013
Elie, J. E., Soula, H. A., Mathevon, N., and Vignal, C. (2011). Dynamics of communal vocalizations in a social songbird, the zebra finch (Taeniopygia guttata). J. Acoust. Soc. Am. 129:4037. doi: 10.1121/1.3570959
Engesser, S., Ridley, A. R., and Townsend, S. W. (2016). Meaningful call combinations and compositional processing in the southern pied babbler. Proc. Natl. Acad. Sci. U.S.A. 113, 5976–5981. doi: 10.1073/pnas.1600970113
Fernandez, A. A., Fasel, N., Knörnschild, M., and Richner, H. (2014). When bats are boxing: aggressive behaviour and communication in male Seba’s short-tailed fruit bat. Anim. Behav. 98, 149–156. doi: 10.1016/j.anbehav.2014.10.011
Fernández-Vargas, M. (2018). Presence of a potential competitor and its individual identity modulate ultrasonic vocalizations in male hamsters. Anim. Behav. 145, 11–27. doi: 10.1016/j.anbehav.2018.08.014
Ficken, M. S., Hailman, E. D., and Hailman, J. P. (1994). The chick-a-dee call system of the Mexican chickadee. Condor 96, 70–82. doi: 10.2307/1369065
Francis, C. D., Ortega, C. P., and Cruz, A. (2011a). Different behavioural responses to anthropogenic noise by two closely related passerine birds. Biol. Lett. 7, 850–852. doi: 10.1098/rsbl.2011.0359
Francis, C. D., Ortega, C. P., and Cruz, A. (2011b). Vocal frequency change reflects different responses to anthropogenic noise in two suboscine tyrant flycatchers. Proc. R. Soc. B Biol. Sci. 278, 2025–2031. doi: 10.1098/rspb.2010.1847
Freeberg, T. M., and Lucas, J. R. (2012). Information theoretical approaches to chick-a-dee calls of carolina chickadees (Poecile carolinensis). J. Comp. Psychol. 126, 68–81. doi: 10.1037/a0024906
Freeberg, T. M., Dunbar, R. I. M., and Ord, T. J. (2012a). Social complexity as a proximate and ultimate factor in communicative complexity. Philos. Trans. R. Soc. B Biol. Sci. 367, 1785–1801. doi: 10.1098/rstb.2011.0213
Freeberg, T. M., Ord, T. J., and Dunbar, R. I. (2012b). The social network and communicative complexity: preface to theme issue. Philos. Trans. R. Soc. Lond. B. Biol. Sci. 367, 1782–1784. doi: 10.1098/rstb.2011.0294
Furmankiewicz, J., Duma, K., Manias, K., and Borowiec, M. (2013). Reproductive status and vocalisation in swarming bats indicate a mating function of swarming and an extended mating period in Plecotus auritus. Acta Chiropterol. 15, 371–385. doi: 10.3161/150811013X678991
Grant, P. R., and Grant, B. R. (2009). The secondary contact phase of allopatric speciation in Darwin’s finches. Proc. Natl. Acad. Sci. U.S.A. 106, 20141–20148. doi: 10.1073/pnas.0911761106
Habersetzer, J., and Marimuthu, G. (1986). Ontogeny of sounds in the echolocating bat Hipposideros speoris. J. Comp. Physiol. 158, 247–257.
Hedwig, D., Mundry, R., Robbins, M. M., and Boesch, C. (2015). Contextual correlates of syntactic variation in mountain and western gorilla close-distance vocalizations: indications for lexical or phonological syntax? Anim. Cogn. 18, 423–435. doi: 10.1007/s10071-014-0812-6
Jiang, T., Huang, X., Wu, H., and Feng, J. (2017). Size and quality information in acoustic signals of Rhinolophus ferrumequinum in distress situations. Physiol. Behav. 173, 252–257. doi: 10.1016/j.physbeh.2017.02.025
Jin, L., Yang, S., Kimball, R. T., Xie, L., Yue, X., Luo, B., et al. (2015). Do pups recognize maternal calls in pomona leaf-nosed bats, Hipposideros pomona? Anim. Behav. 100, 200–207. doi: 10.1016/j.anbehav.2014.12.006
Kalan, A. K., and Boesch, C. (2015). Audience effects in chimpanzee food calls and their potential for recruiting others. Behav. Ecol. Sociobiol. 69, 1701–1712. doi: 10.1007/s00265-015-1982-1
Kanwal, J. S., Matsumura, S., Ohlemiller, K., and Suga, N. (1994). Analysis of acoustic elements and syntax in communication sounds emitted by mustached bats. J. Acoust. Soc. Am. 96, 1229–1254. doi: 10.1121/1.410273
Kershenbaum, A. (2014). Entropy rate as a measure of animal vocal complexity. Bioacoustics 23, 195–208. doi: 10.1080/09524622.2013.850040
Kerth, G. (2008). Causes and consequences of sociality in bats. BioScience 58, 737–746. doi: 10.1641/B580810
Kerth, G., and Van Schaik, J. (2012). Causes and consequences of living in closed societies: lessons from a long-term socio-genetic study on Bechstein’s bats. Mol. Ecol. 21, 633–646. doi: 10.1111/j.1365-294X.2011.05233.x
Kerth, G., Perony, N., and Schweitzer, F. (2011). Bats are able to maintain long-term social relationships despite the high fission-fusion dynamics of their groups. Proc. R. Soc. B Biol. Sci. 278, 2761–2767. doi: 10.1098/rspb.2010.2718
Klump, G. M., and Gerhardt, H. C. (1992). “Mechanisms and function of call-timing in male-male interactions in frogs,” in Playback and studies of animal communication, ed. P. K. McGregor (Boston, MA: Springer), 153–174.
Knörnschild, M., Feifel, M., and Kalko, E. K. V. (2014). Male courtship displays and vocal communication in the polygynous bat Carollia perspicillata. Behaviour 151, 781–798. doi: 10.1163/1568539X-00003171
Knörnschild, M., Fernandez, A. A., and Nagy, M. (2020). Vocal information and the navigation of social decisions in bats: is social complexity linked to vocal complexity? Funct. Ecol. 34, 322–331. doi: 10.1111/1365-2435.13407
Kobayasi, K. I., Hage, S. R., Berquist, S., Feng, J., Zhang, S., and Metzner, W. (2012). Behavioural and neurobiological implications of linear and non-linear features in larynx phonations of horseshoe bats. Nat. Commun. 3, 1184–1184. doi: 10.1038/ncomms2165
Kokko, H., and Jennions, M. D. (2008). Parental investment, sexual selection and sex ratios. J. Evolut. Biol. 21, 919–948. doi: 10.1111/j.1420-9101.2008.01540.x
Kroodsma, D. E. (1977). Correlates of song organization among North American Wrens. Am. Nat. 111, 995–1008.
Kunz, T., and Weise, C. (2009). “Methods and devices for marking bats,” in Ecological and behavioral methods for the study of bats, 2nd Edn, eds T. Kunz and S. Parsons (Baltimore, MD: Johns Hopkins University Press). doi: 10.1016/0165-0270(79)90017-7
Laiolo, P. (2012). Interspecific interactions drive cultural co-evolution and acoustic convergence in syntopic species. J. Anim. Ecol. 81, 594–604. doi: 10.1111/j.1365-2656.2011.01946.x
Laiolo, P., and Tella, J. L. (2008). Social determinants of songbird vocal activity and implications for the persistence of small populations. Anim. Conserv. 11, 433–441.
Lin, A., Jiang, T., Feng, J., and Kanwal, J. S. (2016). Acoustically diverse vocalization repertoire in the Himalayan leaf-nosed bat, a widely distributed Hipposideros species. J. Acoust. Soc. Am. 140:3765. doi: 10.1121/1.4966286
Luo, B., Huang, X., Li, Y., Lu, G., Zhao, J., Zhang, K., et al. (2017). Social call divergence in bats: a comparative analysis. Behav. Ecol. 28, 533–540. doi: 10.1093/beheco/arw184
Ma, J., Kobayasi, K., Zhang, S., and Metzner, W. (2006). Vocal communication in adult greater horseshoe bats, Rhinolophus ferrumequinum. J. Comp. Physiol. A 192, 535–550.
Marler, P. (1976). “Social organization, communication and graded signals: the chimpanzee and the gorilla,” in Growing points in ethology, eds P. P. G. Bateson and R. A. Hinde (Cambridge: Cambridge University Press). doi: 10.1159/000121880
Martínez, J., and Zuberogoitia, I. (2003). vocal behavior of Eagle Owls Bubo bubo: effects of season, density and territory quality. Ardeola Rev. Ibérica Ornitol. 50, 1–9.
McComb, K., and Semple, S. (2005). Coevolution of vocal communication and sociality in primates. Biol. Lett. 1, 381–385. doi: 10.1098/rsbl.2005.0366
Medvin, M. B., Stoddard, P. K., and Beecher, M. D. (1993). Signals for parent-offspring recognition: a comparative analysis of the begging calls of cliff swallows and barn swallows. Anim. Behav. 45, 841–850. doi: 10.1006/anbe.1993.1105
Moors, H. B., and Terhune, J. M. (2004). Repetition patterns in Weddell seal (Leptonychotes weddellii) underwater multiple element calls. J. Acoust. Soc. Am. 116, 1261–1270. doi: 10.1121/1.1763956
Mori, E., Menchetti, M., and Ferretti, F. (2014). Seasonal and environmental influences on the calling behaviour of Eurasian Scops Owls. Bird Study 61, 277–281. doi: 10.1080/00063657.2014.897303
Morton, E. S. (1977). On the occurrence and significance of motivation-structural rules in some bird and mammal sounds. Am. Nat. 111, 855–869. doi: 10.1016/j.beproc.2009.04.008
Morton, E. S. (1982). “Grading, discreteness, redundancy, and motivation-structural rules,” in Acoustic communication in birds, eds D. E. Kroodsma, E. H. Miller, and H. Oullett (New York: Academic Press).
Naguib, M., Titulaer, M., Waas, J. R., van Oers, K., Sprau, P., and Snijders, L. (2022). Prior territorial responses and home range size predict territory defense in radio-tagged great tits. Behav. Ecol. Sociobiol. 76:35. doi: 10.1007/s00265-022-03143-3
Nijman, V. (2007). Effects of vocal behaviour on abundance estimates of rainforest galliforms. Acta Ornithol. 42, 186–190.
Oller, D. K., and Griebel, U. (2008). Evolution of communicative flexibility: complexity, creativity, and adaptability in human and animal communication. Cambridge, MA: MIT Press.
Ord, T. J., and Garcia-Porta, J. (2012). Is sociality required for the evolution of communicative complexity? Evidence weighed against alternative hypotheses in diverse taxonomic groups. Philos. Trans. R. Soc. Lond. B. Biol. Sci. 367, 1811–1828. doi: 10.1098/rstb.2011.0215
Ouattara, K., Lemasson, A., and Zuberbuhler, K. (2009). Campbell’s monkeys concatenate vocalizations into context-specific call sequences. Proc. Natl. Acad. Sci. U.S.A. 106, 22026–22031. doi: 10.1073/pnas.0908118106
Patriquin, K. J., Leonard, M. L., Broders, H. G., and Garroway, C. J. (2010). Do social networks of female northern long-eared bats vary with reproductive period and age? Behav. Ecol. Sociobiol. 64, 899–913. doi: 10.1007/s00265-010-0905-4
Payne, R. J. H., and Pagel, M. (1997). Why do animals repeat displays? Anim. Behav. 54, 109–119. doi: 10.1006/anbe.1996.0391
Peckre, L., Kappeler, P. M., and Fichtel, C. (2019). Clarifying and expanding the social complexity hypothesis for communicative complexity. Behav. Ecol. Sociobiol. 73:11. doi: 10.1007/s00265-018-2605-4
Podos, J. (2001). Correlated evolution of morphology and vocal signal structure in Darwin’s finches. Nature 409, 185–188. doi: 10.1038/35051570
Pollard, K. A., and Blumstein, D. T. (2011). Social group size predicts the evolution of individuality. Curr. Biol. 21, 413–417. doi: 10.1016/j.cub.2011.01.051
Pollard, K. A., Blumstein, D. T., and Griffin, S. C. (2010). Pre-screening acoustic and other natural signatures for use in noninvasive individual identification. J. Appl. Ecol. 47, 1103–1109. doi: 10.1111/j.1365-2664.2010.01851.x
Pougnault, L., Levrero, F., Leroux, M., Paulet, J., Bombani, P., Dentressangle, F., et al. (2022). Social pressure drives “conversational rules” in great apes. Biol. Rev. 97, 749–765. doi: 10.1111/brv.12821
Price, J. J. (1999). Recognition of family-specific calls in stripe-backed wrens. Anim. Behav. 57, 483–492. doi: 10.1006/anbe.1998.1018
Racey, P. A. (1988). “Reproductive assessment of bats,” in Ecological and behavioral methods for the study of bats, ed. T. Kunz (Washington, D.C: Smithsonian Institution Press).
Rebout, N., De Marco, A., Lone, J. C., Sanna, A., Cozzolino, R., Micheletta, J., et al. (2020). Tolerant and intolerant macaques show different levels of structural complexity in their vocal communication: vocal complexity in macaques. Proc. R. Soc. B Biol. Sci. 287:20200439. doi: 10.1098/rspb.2020.0439rspb20200439
Sebe, F., Duboscq, R., Aubin, R., Ligout, R., and Poindron, R. (2010). Early vocal recognition of mother by lambs: contribution of low- and high-frequency vocalizations. Anim. Behav. 79, 1055–1066.
Shannon, C. E., and Weaver, W. (1949). The mathematical theory of communication. Chicago, IL: University of Illinois Press.
Soma, M., and Brumm, H. (2020). Group living facilitates the evolution of duets in barbets: group living and the evolution of duets. Biol. Lett. 16:20200399. doi: 10.1098/rsbl.2020.0399rsbl20200399
Staaterman, E., Brandl, S. J., Hauer, M., Casey, J. M., Gallagher, A. J., and Rice, A. N. (2018). Individual voices in a cluttered soundscape: acoustic ecology of the Bocon toadfish, Amphichthys cryptocentrus. Environ. Biol. Fish. 101, 979–995. doi: 10.1007/s10641-018-0752-0
Sun, C., Jiang, T., Gu, H., Guo, X., Zhang, C., Gong, L., et al. (2020). Geographical variation of social calls and vocal discrimination in male Himalayan leaf-nosed bats. Anim. Behav. 170, 15–26. doi: 10.1016/j.anbehav.2020.10.003
Sun, C., Jiang, T., Kanwal, J. S., Guo, X., Luo, B., Lin, A., et al. (2018). Great Himalayan leaf-nosed bats modify vocalizations to communicate threat escalation during agonistic interactions. Behav. Process. 157, 180–187. doi: 10.1016/j.beproc.2018.09.013
Suzuki, T. N., Wheatcroft, D., and Griesser, M. (2016). Experimental evidence for compositional syntax in bird calls. Nat. Commun. 7:10986. doi: 10.1038/ncomms10986
Thinh, V. N., Hallam, C., Roos, C., and Hammerschmidt, K. (2011). Concordance between vocal and genetic diversity in crested gibbons. BMC Evolut. Biol. 11:36. doi: 10.1186/1471-2148-11-36
Toshiaki, T., Sugiura, H., and Masataka, N. (2006). Cross-sectional and longitudinal studies of the development of group differences in acoustic features of coo calls in two groups of Japanese macaques. Ethology 112, 7–21.
Townsend, S. W., Hollén, L. I., and Manser, M. B. (2010). Meerkat close calls encode group-specific signatures, but receivers fail to discriminate. Anim. Behav. 80, 133–138. doi: 10.1016/j.anbehav.2010.04.010
Xiong, Y. (1975). Ecological observations on some species of bats at Huahong Dong cave in Kunming, Yunnan. Acta Zool. Sin. 21, 336–343.
Yang, Y. (2011). Mating system and kinship of the Formasan leaf-nosed bat, Hipposideros armiger erasensis (Chiroptera, Hippsideridae). Taichung: National Chung Hsing University.
Zhang, C., Sun, C., Wang, Z., Lin, P., Xiao, Y., Metzner, W., et al. (2021). Minor modification of frequency modulated call parameters underlies a shift in behavioral response in the Great Himalayan leaf-nosed bats, Hipposideros armiger. J. Mammal. 102, 457–467. doi: 10.1093/jmammal/gyab019
Zhang, K., Liu, T., Liu, M., Li, A., Xiao, Y., Metzner, W., et al. (2019). Comparing context-dependent call sequences employing machine learning methods: an indication of syntactic structure of greater horseshoe bats. J. Exp. Biol. 222, jeb214072. doi: 10.1242/jeb.214072
Zhao, X., Jiang, T., Liu, H., Wang, Y., Liu, Y., and Feng, J. (2019). Acoustic signalling of aggressive intent in the agonistic encounters of female Asian particoloured bats. Anim. Behav. 149, 65–75. doi: 10.1016/j.anbehav.2019.01.012
Zhu, B., Wang, J., Sun, Z., Yang, Y., Wang, T., Brauth, S. E., et al. (2017). Competitive pressures affect sexual signal complexity in Kurixalus odontotarsus: insights into the evolution of compound calls. Biol. Open 6, 1913–1918. doi: 10.1242/bio.028928
Zuberogoitia, I. (2011). “Weather influence on breeding success of the tawny owl on the southwest limit of Eurosiberian Region,” in Ecology and conservation of european forest-dwelling raptors, eds I. Zuberogoitia and J. E. Martínez (Bilbao: Diputación Foral de Bizkaia).
Zuberogoitia, I., Burgos, G., González-Oreja, J. A., Etxebarria, J. M., and Zabala-Albizua, J. (2019). Factors affecting spontaneous vocal activity of Tawny Owls Strix aluco and implications for surveying large areas. IBIS 161, 495–503.
Keywords: sex ratio, vocal complexity, social organization, aggression, bat
Citation: Gu H, Sun C, Gong L, Zhao X, Feng J, Luo B, Jiang T and Liu Y (2023) Sex ratio potentially influence the complexity of social calls in Himalayan leaf-nosed bat groups. Front. Ecol. Evol. 11:955540. doi: 10.3389/fevo.2023.955540
Received: 28 May 2022; Accepted: 17 April 2023;
Published: 03 May 2023.
Edited by:
Han Li, University of Nebraska Omaha, United StatesReviewed by:
Shizuko Hiryu, Doshisha University, JapanThomas Göttert, Eberswalde University for Sustainable Development, Germany
Copyright © 2023 Gu, Sun, Gong, Zhao, Feng, Luo, Jiang and Liu. This is an open-access article distributed under the terms of the Creative Commons Attribution License (CC BY). The use, distribution or reproduction in other forums is permitted, provided the original author(s) and the copyright owner(s) are credited and that the original publication in this journal is cited, in accordance with accepted academic practice. No use, distribution or reproduction is permitted which does not comply with these terms.
*Correspondence: Ying Liu, bGl1eTI1MkBuZW51LmVkdS5jbg==; Tinglei Jiang, amlhbmd0bDczMUBuZW51LmVkdS5jbg==