- 1Department of Biology, University of Turku, Turku, Finland
- 2Anthropogenic Effects Research Group, Swiss Ornithological Institute, Sempach, Switzerland
Senescence is defined as the general deterioration of the organism (i.e. physiology, morphology, reproduction), and is associated with increasing mortality and decreasing fertility with age. Although senescence has now been widely reported in wild animals, little is known on whether senescence affects all traits, whether this process is synchronized across traits, and whether males and females are affected in the same way. Using an individual-based monitoring of 20+ years in free-living population of Alpine swifts (Tachymarptis melba), we investigated age-dependent variation between sexes and between six biometric traits, 4 reproductive traits, and 1 measure of parasite burden. We accounted for selective disappearance and terminal effects in our analyses. Our results provide general support for age-dependent variation at adulthood in 8 out of the 11 traits investigated. Most traits showed a variation with 2 thresholds, with first a strong improvement until 4 to 12 years of age (e.g., increased fork length, decreased parasite load, or earlier laying date) followed by a plateau and a decline at older ages. The age of the second threshold showed sex specific asynchrony, with an earlier threshold in males than in females for tail length, parasite burden and laying date, as well as moderate asynchrony across traits. Rates of senescence differed between sexes, with stronger senescence of the tail in females than in males and with evidence of reproductive senescence in females but not in males. We also found evidence of terminal investment in males with respect to brood size at hatching and terminal decline with increased asymmetry of the fork and decreased body mass. We found evidence of selective appearance with males with longer fork and little fork asymmetry starting to reproduce earlier in life, and females that start to reproduce earlier tending to higher reproductive success. Finally, we found selective disappearance of males with longer tails and marginal effect of selective disappearance of females with lower body mass. We discuss how natural or sexual selection may have led to these trait- and sex-specific patterns of aging in this long-lived bird.
Introduction
Senescence is the age-related decline of phenotypic traits, that can be measured at the whole organism from a decline in morphological traits and physical performances, such as grip strength in humans (Sayer and Kirkwood, 2015) or number days spent rutting in red deer (Cervus elaphus; Nussey et al., 2009), to a decline in survival and reproduction (Bouwhuis et al., 2012; Nussey et al., 2013; Hayward et al., 2015; Bouwhuis and Vedder, 2017; Cooper et al., 2021). Although signs of senescence were once thought to be restricted to humans and laboratory models (Kirkwood, 2002; Masoro and Austad, 2005), senescence has now been widely reported in natural populations of animals (Nussey et al., 2008; Nussey et al., 2013; Fletcher and Selman, 2015). Studying senescence in the wild, however, is difficult because it requires long-term longitudinal data collected from large numbers of individuals of known age that live long enough to observe signs of senescence (Nussey et al., 2008). Despite an increasing number of longitudinal data on wild populations of vertebrates (Clutton-Brock and Sheldon, 2010), most studies on senescence to date are primarily limited to survival and reproductive traits (Nussey et al., 2013; Bouwhuis and Vedder, 2017; Lemaître and Gaillard, 2017; Lemaître et al., 2020). Many studies are also limited to female reproductive aging (Nussey et al., 2013), with male reproductive success often more difficult to measure due to uncertainties about paternity (Bouwhuis et al., 2009; Bouwhuis et al., 2010). This bias in single-trait and single-sex studies may limit our understanding of senescence for a given species as well as across species (Lemaître and Gaillard, 2017).
As opposed to the first hypotheses of evolutionary biologists, presuming that natural selection should synchronize senescence between different traits (Williams, 1957; Maynard Smith, 1962; Williams, 1999), studies in humans and laboratory animals have shown that senescence of different traits can often be uncoupled (Burger and Promislow, 2006; Martin et al., 2007; Nussey et al., 2009; Bansal et al., 2015; Moorad and Ravindran, 2022). A growing number of studies provide similar evidence of asynchronous senescence between traits in wild animals (Bouwhuis et al., 2012; Nussey et al., 2013; Hayward et al., 2015; Bouwhuis and Vedder, 2017; Cooper et al., 2021; Fay et al., 2021). However, much remains to be done to understand which traits are senescing first and why (Moorad and Ravindran, 2022). This research question is important to investigate whether, for example, reproductive senescence is induced by a decline in foraging ability, which in turn is induced by a decline in muscle mass and physical activity. This would predict an earlier onset of senescence in mass versus foraging ability versus reproduction.
Although there are fewer studies on male than female senescence in the wild, evidence of senescence was found in both sexes and, more interestingly, males are often found to senesce faster than females in mammals (Nussey et al., 2013). Several evolutionary hypotheses have been proposed to explain differences between the sexes in their rate of senescence. One of the best-known factors leading to sex-specific rate of senescence is a difference in their exposure to environmentally induced mortality, with the sex with greater mortality expected to senesce at the fastest rate (Williams, 1957; Bonduriansky et al., 2008). Accordingly, males in polygynous species often suffer higher mortality, as a result of male-male competition, and display faster rates of senescence (Lemaître et al., 2020; Bronikowski et al., 2022). Less is known however on sex-specific rates of aging in socially monogamous species (Nussey et al., 2013). As these species often show lower sexual size dimorphism and more balanced reproductive allocation between the sexes, sex-specific senescence is less expected in monogamous species than in polygynous ones (Promislow, 2003; Clutton-Brock and Isvaran, 2007). Studies in monogamous species are however showing a great diversity in the rates of senescence between the sexes, from no differences between the sexes in socially monogamous and cooperative breeding meerkats (Suricata suricatta; Thorley et al., 2020), to faster rates of senescence in male wandering albatrosses (Diomedaexulans; Pardo et al., 2013), male white-tailed eagles (Haliaeetus albicilla; Murgatroyd et al., 2018), and male red wolves (Canis rufus; Sparkman et al., 2017), and finally faster senescence in female common guillemots (Uria aalge; Reed et al., 2008). Sex-specific rates of senescence in monogamous species may be influenced by the life-history strategies adopted by each sex, and thus by the trade-offs involved in these strategies (Nussey et al., 2008; Lemaître and Gaillard, 2017). A greater allocation of one sex to parental care, or greater allocation in physiologically demanding activities (e.g. growth, foraging, territorial defense, secondary sexual characteristics) would thus increase its senescence rate (Reid et al., 2003; Reed et al., 2008; Nussey et al., 2009; Murgatroyd et al., 2018). To reach a better understanding of the variation of senescence in the wild, more studies investigating sex-specific senescence patterns are needed, especially in monogamous species, and ideally on phenotypic traits other than just reproduction (Nussey et al., 2009; Nussey et al., 2013).
Finally, when studying age-related variation among traits and sexes in free-living populations, it is also essential to account for heterogeneity between individuals in their rates of aging (Vaupel et al., 1979). In other words, it is essential to separate within- and between-individual effects on age-related changes (van de Pol and Verhulst, 2006). Indeed, age-related changes observed at the population level can be induced by within-individual changes (improvement early in reproductive life and senescence later in life) as well as by between-individual changes (selective appearance and disappearance of certain phenotypes; van de Pol and Verhulst, 2006). Between-individual effects, such as, for example, the early disappearance from the population of individuals with a low reproductive success (i.e. selective disappearance of poor breeders), were highlighted in most avian studies on senescence (Vedder and Bouwhuis, 2018) and can mask the decline of reproductive traits due to actual senescence (van de Pol and Verhulst, 2006; Nussey et al., 2008). Researchers thus need to control for demographic effects when aiming to measure actual senescence. Similarly, before the death of individuals, the age-related variation of fitness traits can change direction due to terminal investment (Clutton-Brock, 1984; Froy et al., 2013), with an expected increase of performance the last year of life, or due to terminal illness (Coulson and Fairweather, 2001; Rattiste, 2004), with an expected decrease of performance the last year of life. These terminal effects can also mask or be mistaken for a gradual age-related decline due to senescence, especially in short-lived species. In recent years, authors investigating senescence in the wild increasingly account for between-individual variations by assessing selective disappearance, as well as terminal effects (Bouwhuis et al., 2009; Martin and Festa-Bianchet, 2011; Froy et al., 2013; Hayward et al., 2015; Zhang et al., 2015; Dingemanse et al., 2020; Cooper et al., 2021) and emphasize the need to control for these effects in studies on senescence.
In this study, we investigated sex and trait differences in aging trajectories in the socially monogamous and long-lived Alpine swift (Tachymarptis melba). Data were collected for 11 traits in both sexes throughout their reproductive lives. This longitudinal approach allowed testing for age-dependent variations while accounting for selective disappearance and terminal effects. Alpine swifts start breeding at 2 to 4 years of age (median age at first reproduction is 3 years of age; Tettamanti et al., 2012) and may live up to 26 years (median lifespan of breeders is 7 years of age; Arn, 1960; Bize et al., 2009). This migratory bird is socially monogamous and reproduces in colonies of up to several hundred pairs. Males and females provide equal parental care during the breeding period (Bize et al., 2004b), and previous studies found no difference in survival between the sexes (Bize et al., 2008a; Bize et al., 2014). Females lay a modal clutch of 3 eggs, and both sexes then incubate the eggs for 20 days and feed their nestlings up to fledging, which occurs 50 to 70 days after hatching (Bize et al., 2004b). Although males have a slightly larger body size than females (1% for wing length to 7.5% for fork length) (Bize et al., 2006a; Table A1 in appendix), this bird is sexually monomorphic to human eye, and molecular tools are required to reliably sex individuals.
The Alpine swift is an interesting model to explore differences in senescence between the sexes and asynchrony across traits for two reasons. First, as this bird species appears to be socially monogamous and sexually monomorphic, with both sexes equally involved in reproductive tasks, it can be expected that there will be few sex differences in senescence rates compared to polygynous species and species with high sexual size dimorphism, if differences in reproductive allocation are the main driver of sex-specific senescence rates. Second, as the Alpine swift is an extreme case of aerial bird species where adults spend most of their lives flying (they can fly up to 200 days without landing; Liechti et al., 2013), we can expect differences across traits in selection pressures and their rates of senescence (Moorad and Ravindran, 2022). This might be particularly true for traits, such as tail and wing length and symmetry, which have strong consequences on aerodynamics and energy expenditure during flight (Thomas, 1993a; Thomas, 1993b; Norberg, 1995a). Differences in the onset and rate of senescence between morphometric traits (body mass, wing length, tail length, tail asymmetry) and reproductive traits (laying date, clutch size, brood size at fledging) remain however rarely investigated.
Material and methods
Study population and general methods
The data were collected between 1999 and 2022 in two Alpine swift colonies located under the roof of two old buildings in Biel (Stadtkirche) and Solothurn (Bieltor), Switzerland. During this study period, the number of breeding pairs remained constant in Solothurn (about 50 pairs), whereas in Biel it declined from about 100 pairs to 60 pairs. Since 1999 there has been an intensive individual-based monitoring of adults and their reproductive success in both colonies. Fledglings are primarily recruited locally (i.e. 60% of the recruits), and recruits do not disperse after starting to breed in a colony (no “breeding dispersal”; Bize et al., 2017). Furthermore, as the annual capture probability of birds after they start breeding is virtually 1 (SE = 0.003) (Bize et al., 2006a), there is exceptional repeated information throughout the breeding life of adults. In this population, 68.8% of the breeders have been ringed as nestlings and are thus of known age. If a breeder is not captured for 2 consecutive years, it is considered dead (Bize et al., 2009).
Each year, nests were regularly visited to record laying dates, clutch sizes, and brood sizes at hatching and fledging, and to ring the nestlings. Adults were captured by hand while sitting on their eggs or brooding nestlings. As in the Alpine swift both parents must incubate the clutch and provision the brood, the brood size at hatching and fledging depends on both parents. Although we have little information in this species regarding the contribution of each sex to pre-hatching traits (i.e. laying date and clutch size), males may have thus an indirect impact on pre-hatching traits if females allocate differently in reproduction according to the quality (i.e. age) of their mate, as seen in other bird species (Komers and Dhindsa, 1989; Johnsen et al., 2005; Michl et al., 2005; Segami et al., 2021).
Adults were individually identified with a ring they received as nestlings or when they were first captured as adults and had not been ringed before. Each year when an adult was captured, we measured with a ruler to the nearest mm: wing length on the flattened and straightened closed wing, tail length from the base to the tip of the outermost tail feather, and fork length as the difference in length between the tip of the innermost and outermost tail feathers. Both the right and left lengths of the fork were measured, and we computed the absolute difference in length as an estimate of fork fluctuating asymmetry. Swifts have very short tarsi and thus, as an alternative measure of skeletal size, we measured their sternum length with a caliper to the nearest 0.1mm. With each capture of an adult, the individuals are weighed with a scale at the nearest 0.1 g, and we counted the number of ectoparasitic louse-flies in their plumage. Alpine swifts are heavily infested by the hematophagous louse-fly Crataerina melbae (Diptera, Hippoboscidae) that has been previously demonstrated to affect nestling growth (Bize et al., 2003; Bize et al., 2004a) and adult reproductive success (Bize et al., 2004b). When infested by blood‐sucking insects, hosts can defend themselves with cutaneous immune responses that make the biting site unfavorable for feeding by hematophagous insects and can cause damage to the parasite tissue (Wikel, 1996; Wikel, 1999; Owen et al., 2010). In agreement with this, the cutaneous immune response of Alpine swifts was found to affect the survival and blood meal size of the louse-flies (Bize et al., 2008b). To assess the parasite burden of the swifts, we counted the number of louse-flies in adult plumage.
Hence, the 11 phenotypic traits collected in this study can be grouped into three different broad categories, namely, biometric traits (wing, tail, fork, fork asymmetry, sternum, body mass), parasite load which could reflect the cutaneous immunity of the bird, and reproductive traits (laying date, clutch size, brood size at hatching, brood size at fledging).
Statistical analyses
All the analyses were performed using R version 4.1.2 (R Core Team, 2022). The birds used in this study ranged between 1 to 22 years of age. The data include repeated measurements for each individual throughout its breeding life (mean ± SE breeding observations per individual: 3.8 ± 0.1; 1st and 3rd quantiles: 1 and 5 observations per individual). At both ends of our age spectrum, we only had 2 observations of breeding birds at 1 year and 9 individuals with 14 observations at 19 years of age and older. To avoid biases caused by extreme values and thus improve the robustness of our results, we pooled observations at 1 year of age with those at 2 years of age and we pooled together in the same age category all the observations at 19 years of age and over (following Froy et al., 2013; Fay et al., 2021).
First, to tease apart changes in the phenotype driven by age-related effects, selective disappearance, and terminal illness or investment (van de Pol and Verhulst, 2006; Froy et al., 2013), we restricted our analyses to breeding adults (N = 244 males and 307 females) whose age was known (i.e. ringed at nestling or at one year of age, when still having different plumage characteristics than adults) and for which we had complete life history (i.e. followed from their first reproduction to death). We assigned a lifespan to individuals found dead or not seen since at least 2020 (i.e. not captured for at least 2 consecutive years). We used a mixed model approach that follows equation (1) in van de Pol and Verhulst (2006) which allows teasing apart within-individual age effects (experience, senescence) from demographic effects (selective appearance and disappearance):
which is a two‐level random intercept model with individual i, measurement j, random intercept term , residual error term , within‐individual change (), selective appearance effect () and selective disappearance (. By modeling the age of individuals as a continuous variable that is not centered on a mean individual age (i.e. ), our models test for an absolute effect of age (i.e. chronological effect associated with a general pattern of senescence) rather than a relative effect of age (i.e. biological effect with individual deviation from the general pattern of senescence) (see Fay et al., 2021).
In all our mixed models, we also included a two-level fixed factor ‘last observation’ (last breeding observation = 1, previous breeding observation = 0) to test for terminal effects (terminal investment vs. terminal illness) that can mask the effect of senescence (Bouwhuis et al., 2009; Froy et al., 2013), and a two-level fixed factor ‘colony’ (Biel, Solothurn) to control for possible differences between breeding sites. We included individual ring and year of measurement as random factors to control for pseudo-replication and temporal heterogeneity. Parasite load varied with the date of capture following a quadratic curve (Bize et al., 2003), so we controlled for this variation by including linear and quadratic terms for the day of capture in the model testing the age-related variation of parasite load. Similarly, when analyzing body mass, we also controlled in our models for quadratic variations in mass in relation to the hour of the day and to the day of capture during the breeding season1. As we considered individuals from their first reproduction, individuals included in the analyses were already of adult size. Although sternum length is fixed in adulthood (no within-individual variation), we nevertheless kept this trait as dependent variable in our analyses as it may still show between-individual variation (e.g. selective disappearance of smaller individuals).
To identify first the variation of the 11 phenotypic traits with age in our population, we compared a series of mixed effects models separately for each trait and for male and female swifts. We tested models without any effect of age, with age as a linear effect, age as a quadratic effect, and age with different breakpoints known as threshold models (following Berman et al., 2009). We tested a single threshold varying between 4 to 12 years, and double thresholds one varying between 4 to 12 years and a second between 8 and 16 with at least 3 years intervals between the two. We compared these models using maximum likelihood (ML) estimation and identified the models that best described the variation with age of the phenotypic traits according to the Akaike Information Criterion (AIC). For each trait and each sex, we selected the models with ΔAIC<2 (Burnham and Anderson, 2002). If several models were selected, we used a model averaging approach (following Froy et al., 2013) using the R package MuMIn (Bartoń, 2011) to obtain the coefficient estimates of the co-variables in the models and assess the between individual effects (selective appearance and disappearance) and terminal effects for each trait and sex.
For the traits where we detected variation with age late in life, we then carried a second set of analyses to formally test for decline in late life (i.e. senescence), and whether this decline differed between sexes. From the best models (with ΔAIC<2), we calculated for each trait and sex the mean age of each threshold. We then built trait-specific ‘late’ dataset by pooling observations from both sexes made after the onset of senescence (i.e. using sex and trait specific thresholds). We considered the age at the second threshold as the potential onset of senescence for traits with two thresholds. We investigated sex-specific senescence using linear mixed models with age as linear effect to estimate the slope after the threshold and testing the interaction between age and sex. In these models, we also included individual ring and year of measurement as random factors, and we kept as fixed effect the variables selected from our previous approach using model averaging. In this second set of analyses, we increased our sample sizes at late age by taking into account data from individuals (N = 319 males and 391 females) whose age and lifespan were known, but for which we did not know their age at first reproduction, as they started reproducing before the start of our individual monitoring (before 2000). Nestling Alpine swifts in the colonies Biel and Solothurn have been ringed since at least 1968, and thus most individuals were of known age at the start of this individual monitoring. To compare rates of senescence across phenotypic traits, we scaled (i.e. standardized to zero mean and unit variance) the traits prior to the analyses.
As recommended by Schielzeth et al. (2020), we fitted lmer models (Gaussian distribution) to all studied traits to allow biologically meaningful comparisons of estimates across models even if not all the traits followed a Gaussian distribution. These authors showed that mixed models provide robust estimates of fixed effects even when the distributional assumption is strongly violated. Sample sizes may differ between models and traits due to missing values for some traits or some individuals.
Results
Age-related variation of trait
Our comparison of models showed that age-related variations in our phenotypic traits were usually best modelled with threshold models rather than quadratic models (See AIC table presented in appendix, Table A2). Results of the best models presented in Figure 1 show an age-related variation in all traits studied, at the exception of fork fluctuating asymmetry (Figure 1D), sternum length (Figure 1E), and body mass (Figure 1F). Models with 2 thresholds best described the predominant pattern of aging, as observed in both sexes for wing length (Figure 1A), tail length (Figure 1B), fork length (Figure 1C), parasite load (Figure 1G), laying date (Figure 1H), clutch size (Figure 1I), brood size at hatching (Figure 1J) and fledging (Figure 1K). Among these traits, the length of the wing, tail, fork, the clutch size, brood size at hatching and fledging showed an increase in both males and females early in life, until the first or second threshold, followed by a decline in at least one of the two sexes. Parasite burden and laying date show the opposite pattern with a decrease early in life, corresponding to a decrease in parasite load and an advancement of the laying date in the early reproductive years (Figures 1G, H).
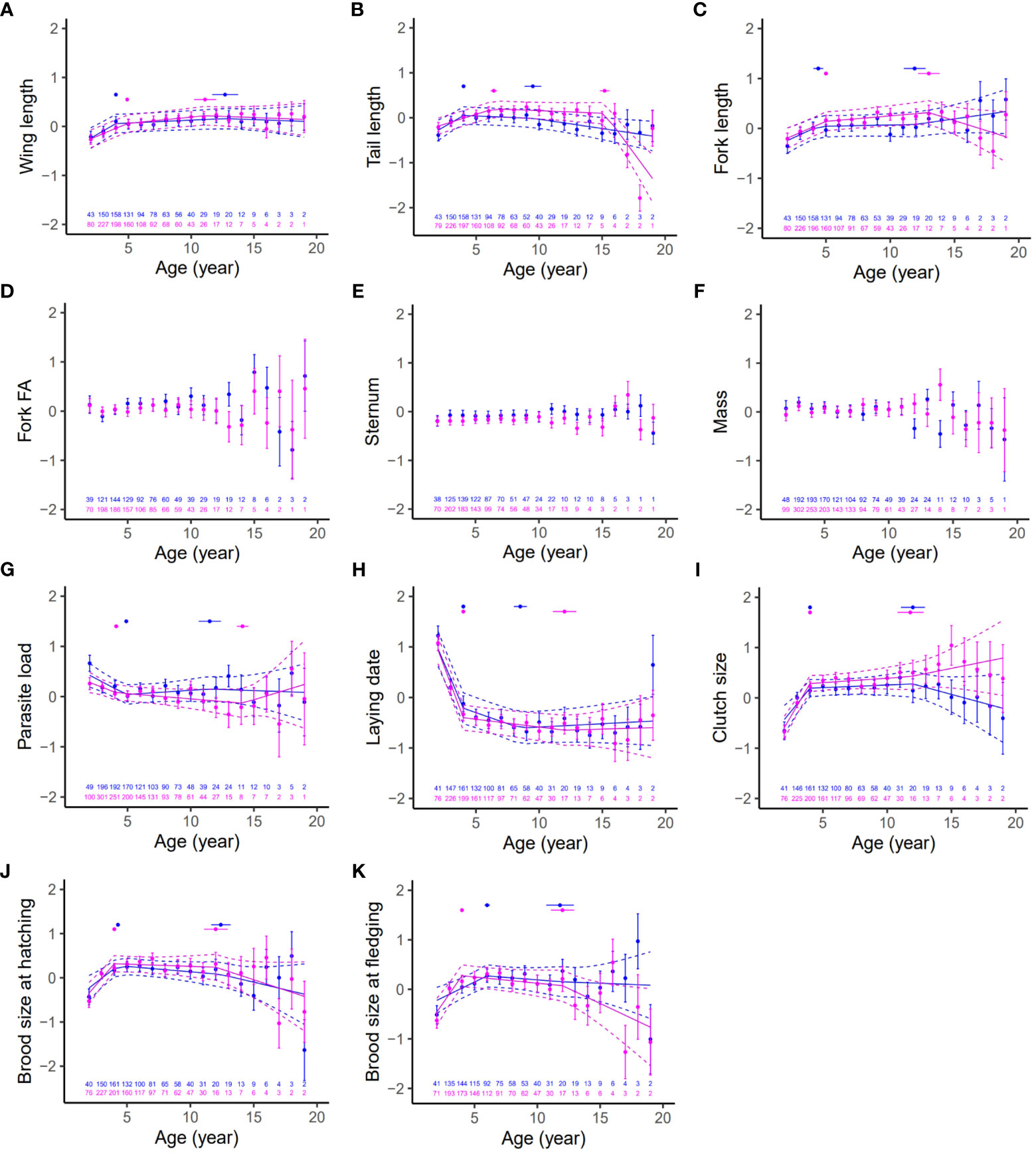
Figure 1 Age-related variation in biometric traits, parasite burden, and reproductive traits in male (blue) and female (pink) Alpine swifts. Traits (Y-axis) are scaled and centered and age (X-axis) is expressed in years, allowing for an immediate comparison across traits and between sexes of rates of change with age. The graphs show the segments obtained from the predictions of the threshold models with 95% CI (dashed lines) and the mean value of the trait at each age (mean ± SE in blue for the males and pink for the females). The horizontal lines above the segments show the range of variation of the thresholds for each sex calculated from the models with ΔAIC<2 (mean thresholds ± SE). If the “null” model with no age-related variation was retained by our model selection, we did not plot the segments from the prediction of the thresholds model (i.e. fork FA, sternum, mass). For each of the traits, sample sizes at a given age are indicated at the bottom of each panel in blue for the males and pink for the females.
Selective appearance, selective disappearance and terminal effects
Estimates of selective appearance, selective disappearance and terminal effects derived from model averaging of the best models are presented in the Figures 2A–C. We found evidence of selective appearance (i.e. measured by including AFR) for male fork length and fluctuating asymmetry and in female brood size at hatching and fledging (Figure 2A). Males with longer and more symmetrical fork start to reproduce earlier. Females that tend to initiate their reproduction late in life tend to have lower brood size at hatching and fledging.
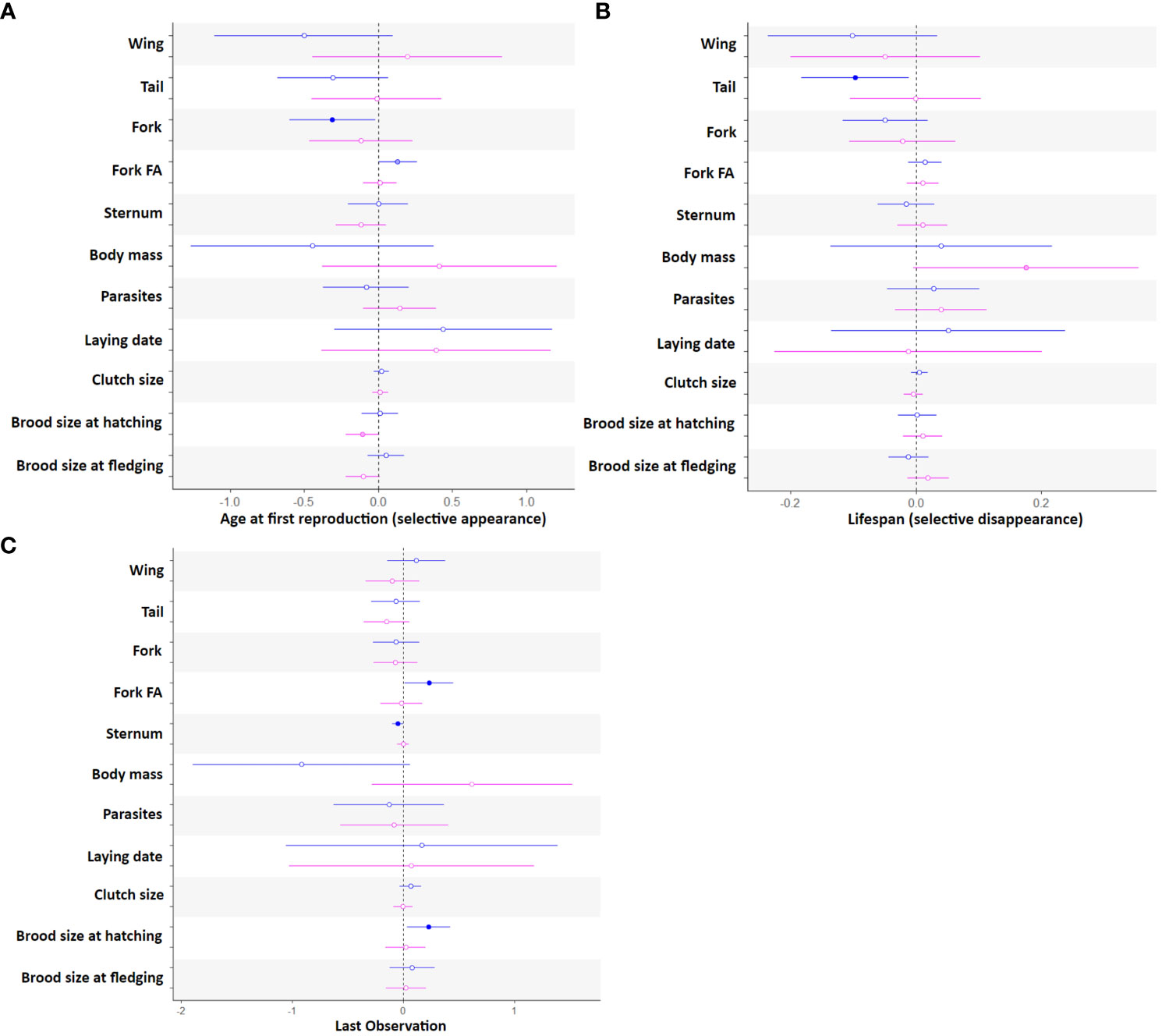
Figure 2 (A–C) Estimates with 95% confidence for selective appearance (A), selective disappearance (B) and terminal effects (C) in female (pink) and male (blue) Alpine swifts. The significance of each estimate is indicated by filled circle when significant (P< 0.05), by lightly colored circles when marginally significant (0.05 ≤ P ≤ 0.06), and by open circles when non-significant (P<0.06).
We found evidence of selective disappearance (i.e. effect of lifespan) for male tail length (Figure 2B), with males with longer tails being shorter lived and gradually disappearing from older age categories. We also observe a trend for selective disappearance of females of lower body mass (Figure 2B).
Finally, we found support for terminal effects (i.e. last year of observation) on male fork fluctuating asymmetry, sternum length, and brood size at hatching (Figure 2C). In their last year of life, males produced more offspring at hatching, and they also show an increase in fork asymmetry and a decrease in sternum length. Males also tend to show a decrease in body mass the last year before death (Figure 2C; Table A3 in appendix).
Onset of senescence and late life rates of aging
For all the traits showing a variation with age, we then looked at age-related variation late in life (i.e. after the last threshold) to formally test for the presence of individual senescence. We found evidence of a decline with age late in life for 4 of the 11 traits (i.e. wing, tail, brood size at hatching, brood size at fledging; Figure 3). This decline was different between males and females for tail length, brood size at hatching and at fledging (Figure 3). Senescence rates for tail length were higher in females than in males, and senescence on the number of offspring at hatching and fledging were only visible in females.
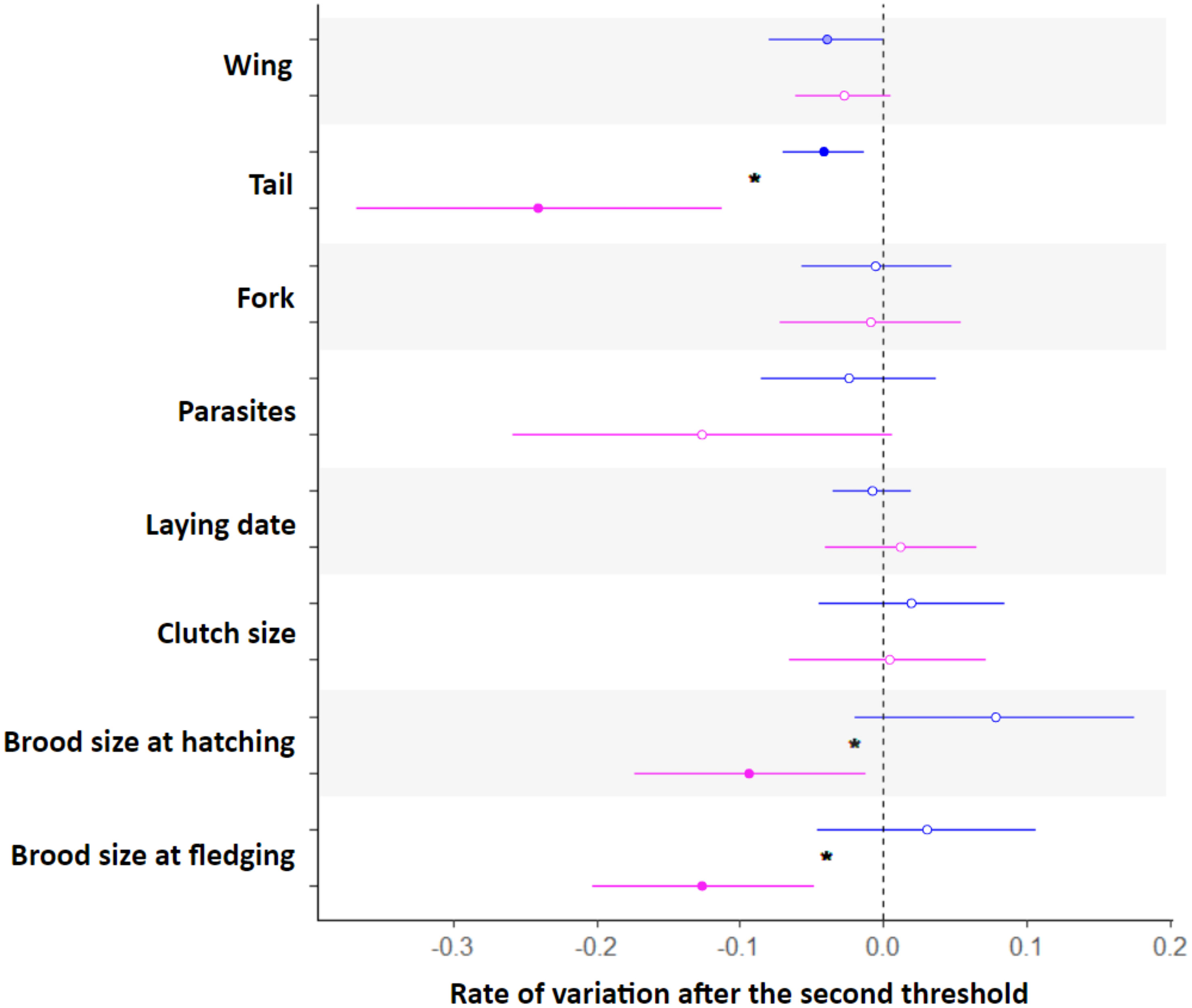
Figure 3 Estimates of rates of ageing with 95% confidence in female (pink) and male (blue) Alpine swifts. The significance of each estimate is indicated by a filled circle when significant (P< 0.05), by lightly colored circles when marginally significant (0.05 ≤ P ≤ 0.06), and by open circles when non-significant (P< 0.06). Significant differences in the rate of ageing between males and females for a given trait are indicated by a star symbol. The traits with no evidence of age-related variation (i.e. sternum, fork fluctuating asymmetry, body mass) were removed from these analyses.
Finally, threshold estimates presented in Figure 1 show asynchrony in the age at onset of senescence (i.e. second threshold) between sexes, with this onset being earlier in males for tail length and parasite load (Figures 1G, H).
Discussion
Our analysis of age-related variation in eleven phenotypic and fitness-related traits in the long-lived Alpine swift shows evidence for variation in adulthood for all traits studied except sternum length, body mass and fork fluctuating asymmetry. Sternum length is a skeletal feature that is not expected to change once birds reach their final adult size (i.e., just prior to fledging in the Alpine swift; Bize et al., 2006b), and thus the lack of within-individual variation in sternum length in adulthood was expected. Although the absence of variation with age for the body mass and fluctuating asymmetry of the fork suggests a relatively stable body condition in this bird, we observe a tendency of decrease of body mass and detected an increased asymmetry of the fork in males the last year before death. In females, a senescence in body mass might be masked in our study at the population level by the selective disappearance of individuals with lower mass. The variation with age of the other traits was best described by segment models with 2 thresholds, such that traits show an increase early in life followed by a plateau and/or decline later in life. This ‘bell-shape’ pattern is consistent with what has been observed in numerous species (Catry et al., 2006; Lecomte et al., 2010; Frankish et al., 2020; Saraux and Chiaradia, 2022). In the Alpine swifts, most traits showed increase or improvement until 4 to 12 years of age (e.g., increased fork length, decreased parasite load, or earlier laying date) followed by a decline at older ages. Such widespread evidence of trait improvement over the first year of breeding is striking, and the mechanisms explaining improvement with age (e.g. appearance or disappearance of certain phenotypes;. constraint vs restraint hypotheses; Forslund and Pärt, 1995; Saraux and Chiaradia, 2022), remain to be investigated in detail in this species.
Selective appearance, selective disappearance and terminal effects
We used a statistical approach to separate within-individual effects (aging per se) from demographic effects (van de Pol and Verhulst, 2006; Fay et al., 2021). This approach is important as we found evidence of demographic effects on age-dependent trait variation. Interestingly, we found selective appearance of males with shorter fork and a trend for males with higher asymmetry of their fork. Hence, males with longer and symmetrical fork start reproducing earlier. The length and symmetry of the fork could thus reflect the quality of a male and be involved in its mating success. This result brings support to a previous phylogenetic comparative analyses suggesting that fork depth is under sexual selection in swifts (Hasegawa and Arai, 2020). In females, we observed a trend for selective appearance of females with lower reproductive performance. Females that start reproducing earlier show higher brood size at hatching and fledging. Therefore, the selective appearance of males with shorter fork and females with lower reproductive performance further supports the strong increase of these traits observed early in life. At older ages, we observed the selective disappearance of males with longer tails and a tendency for females with lower body mass. The latter might be related to the general quality of females suggesting that higher quality females might have a higher longevity (Weladji et al., 2006; Tettamanti et al., 2015). This result could mask a potential decline of body mass in females at older ages in our study.
We also found terminal effects, as an increased brood size at hatching the last year before death in males. However, we cannot know if the death of the males after this breeding season is the consequence of the increased reproduction or the cause. The increased brood size the last year of life supports a terminal investment of males during incubation. Another possible explanation could be that males, before their death, mate with younger females increasing the performance of their last reproduction. However, this increased reproduction can also involve high costs and therefore be the cause of death, via a trade-off between allocation of energy for reproduction at the expense of survival (Stearns, 1992). This is also consistent with the trend observed in males for a terminal illness with a decrease of body mass and the increase of fork asymmetry the last year before death.
Age-related changes in flight-related biometric traits
The Alpine swift is a very aerial bird that can remain in flight without interruption for over 200 days (Liechti et al., 2013). Our results show that biometric traits associated with flight, namely wing and tail, vary throughout adult life, with an increase in size until 4 to 6 years of age followed by a decline after 9 to 15 years of age. In particular, tail length showed strong senescence in both sexes, although the decrease in males might be partly driven by selective disappearance of individuals with longer tails. The decline was moderate for wing length in both sexes. These results are qualitatively comparable to the patterns of variation with age of morphological traits observed in the barn swallow (Hirundo rustica, Møller and de Lope, 1999). Feather abrasion over time (days to years) may explain the reduction in wing and tail length with age. However, since Alpine swifts molt every year their wing and tail feathers, the decrease of length observed with age might rather be explained by a decrease of feather growth rate with age, as recently evidenced in the central tail feathers of barn swallows (Adamkova et al., 2022). The decline in biometric traits late in life could have implications for flight ability in Alpine swifts since body mass and the length and shape of the wings, tail, and fork influence flight maneuverability and energy expenditure during flight in birds (Evans and Thomas, 1992; Thomas, 1993c; Norberg, 1995a). Swifts have long narrow wings and low wing load, which are biometric features that allow them to make sharp turns to capture insects (Norberg, 1995b). Hence, the shortening of tail and wing length with age in the Alpine swift could increase the cost of flight and decrease flight performance and foraging success at older ages, as observed in the grey-headed albatross (Catry et al., 2006). These decreases in flight performance with age could, in turn, have an impact on reproductive success in later life. However, in our study system, this hypothesis is only partially supported, because, although we found strong evidence of senescence for flight-related traits in both sexes, we only found evidence for reproductive senescence in females. Therefore, the senescence of reproductive traits in this species is probably not simply explained by changes in size and body condition alone, as in this case we would also expect to see a decline in reproductive parameters in males as well. The decline of biometric traits later in the life we observe in Alpine swift might also have an impact on their migration. This is even more likely since the ratio between the size of the bird, the length and shape of the wings and tail are associated with specific tactics of migration, which in this species for instance would favor energy-minimization (Norberg, 1995b). To confirm this hypothesis, it would be necessary to study the characteristics of migrations (Meier et al., 2020) after the onset of the senescence of biometric traits to test whether senescence impacts the distances traveled and/or the travel time to reach the breeding location. A study on barn swallows showed that tail and wing length decreased in older individuals, as did their migratory performance, resulting in a later arrival on the breeding site (Møller and de Lope, 1999).
Age-related changes in parasite load and immunosenescence
Parasites derive their resources from their hosts, and hosts exposed to parasites may therefore incur fitness costs (Møller et al., 2009a). Hosts can, however, defend themselves against parasites by mounting immune responses (Wikel, 1996; Wikel, 1999; Owen et al., 2010), which can limit the amount of resources extracted by hosts and their infestation load. Hence, one hypothesis is that the decline of the immune responses in old age leads to greater exposure to parasites (Møller and de Lope, 1999; Hayward et al., 2015; Peters et al., 2019) and, in turn, a decline in host performance in old age. Alpine swifts are heavily infested by blood-sucking louse-flies that are known to negatively impact the reproduction of this bird species (Bize et al., 2003; Bize et al., 2004a; Bize et al., 2004b; Bize et al., 2005), and a previous study showed that the cutaneous immune response of swifts plays an important role to defend themselves against louse-flies (Bize et al., 2008b). We found no evidence that parasite load differed between the sexes or increased with increasing age. The lack of sex difference in parasite load in adult swifts is similar to what has been found in nestlings where males and females show similar levels of parasite infestation and cell-mediated immunity (Bize et al., 2005). Overall, our results are similar to those of a recent study on the common tern (Sterna hirundo), a long-lived seabird, which found no difference between males and females in innate immune parameters and no clear senescence of these parameters (Bichet et al., 2022). In Seychelles warblers (Acrocephalus sechellensis) as well, the infection of a blood parasite does not increase in older birds, providing no evidence for immunosenescence (Hammers et al., 2016). Also, recent meta-analyses did not find evidence of differences between the sexes in immunity and immunosenescence in wild populations (Kelly et al., 2018; Peters et al., 2019). Hence, our results provide little support for immunosenescence as an important driver of reproductive or biometric trait senescence in the Alpine swift. However, studies with direct measures of the immune system and greater diversity of measures of exposure to parasites (such as blood meal size) (Bize et al., 2008b) are needed to gain greater insights on immunosenescence in this host-parasite system.
Age-related changes in reproductive traits
Our results show that, as observed in other vertebrates in the wild (Lemaître and Gaillard, 2017; Cooper et al., 2021), the reproductive performances of Alpine swifts first increased with age over the first reproductive attempts for both males and females, a finding that can is often attributed to birds gaining breeding experience early in life (Forslund and Pärt, 1995; Saraux and Chiaradia, 2022). However, there were marked differences between the sexes later in life as we detected evidence of reproductive senescence in females that was not observed in males. That is, after reaching a peak, females showed a decline in reproductive performance later in life, with strong evidence for senescence in brood size at hatching and fledging from around 12 years. In males, egg-laying date advances in the early reproductive years, and clutch size, brood size at hatching and fledging increase before reaching a plateau at older ages. Note that since males do not lay eggs, evidence of male age-related variation in laying date and clutch size is likely to reflect selection or differential allocation by females based on male age and reproductive experience (Komers and Dhindsa, 1989; Michl et al., 2005; Segami et al., 2021). That is, females may lessen their allocation into reproduction (delay breeding and produce fewer eggs) when mating with young and inexperienced males compared to old and experienced males. Altogether, these results indicate stronger signs of reproductive senescence in females compared to males in the long-lived Alpine swift. Our results are consistent with observations in wild Soay sheep, with a decline in reproductive performance in females contrary to an increase of reproductive performance in males which then plateaus (Hayward et al., 2015). However, this contrasts with findings in the wandering albatross which is a long-lived and monogamous bird like the Alpine swift, with similar parental roles between males and females (Pardo et al., 2013). The breeding probabilities of males albatross decrease at a faster rate than in females (Pardo et al., 2013).
Contrary to polygynous and highly dimorphic species, with sex-specific allocation to sexual signaling and mating, it is less evident to identify potential causes of these sex-specific patterns of reproductive senescence in monogamous and monomorphic species. In the great tit (Parus major), it was found that half of the decline in reproductive success is related to reduction in brood size at hatching and fledging, and thus to egg failure and nestling mortality; clutch size did not contribute to senescence of recruit production (Bouwhuis et al., 2009). The authors of this study suggested potential proximate mechanisms underlying the senescence in reproductive success, such as incubation behavior, the composition and quality of eggs, or a decline of male fertilization efficiency and sperm quality as reviewed also in Lemaître and Gaillard (2017). In our study population, however, results show no senescence of male reproductive traits and even an improvement in the last breeding attempt before death. It is thus unlikely that male fertility and sperm quality decrease with age in the Alpine swift, as opposed to other wild birds (e.g. Møller et al., 2009b). Another explanation could be differences in energy expenditure between males and females during reproduction. Although males, as well as females, do incubate the clutch and provision the brood, the production of eggs by females is energetically demanding and can lead to costs as reviewed by Williams (2005). For example, in a wild population of lesser black-backed gulls (Larus fuscus), experiments where females were forced to lay extra eggs show consequences not only on the quality of the extra eggs (Nager et al., 2000), but also females’ rearing capacity (Monaghan et al., 1998) and their local return rates (Nager et al., 2001). Hence, Alpine swift females may have greater reproductive costs than males due to egg production, which in the long run might explain the decline in reproductive traits observed in females as opposed to males in our population. Furthermore, although Martins and Wright (1993) reported in the closely related common swift that males and females show similar levels of self-feeding (Martins and Wright, 1993), and by extension self-maintenance, it cannot be excluded that males and females’ life history strategies can evolve with age, as seen in the wandering albatross (Lecomte et al., 2010). In this species, the decline in reproductive performance suggests that older males allocate more in self-maintenance as opposed to reproduction compared to young males (Lecomte et al., 2010). Insights on sex and age-related variation on the energetics and foraging behaviors in the Alpine swifts will be required to answer these questions. Furthermore, to better understand the mechanisms behind variation in reproductive senescence between males and females, it would be interesting to investigate other physiological traits, especially related to reproduction in females. For example, it has been suggested that the deregulation with age of the pituitary-hypothalamic-ovarian axis, as a consequence of a somatic deterioration, could be an important driver of reproductive senescence in females (Lemaître and Gaillard, 2017). How males and females differ in the regulation of their oxidative balance could also be an interesting lead in the Alpine swift, as previous research in this species showed senescence in cell resistance to oxidative stress (Bize et al., 2014) as well as sex-specific covariation between resistance to oxidative stress and fecundity and survival (Bize et al., 2008a). Males who survived to the next breeding season tended to be more resistant to oxidative stress, and females with higher resistance to oxidative stress laid larger clutches (Bize et al., 2008a). In our population, reproductive senescence might thus be due to global physiological decline with age, associated with costs of reproduction, and/or to an allocation later in life in somatic functions responsible for longer lifespan as hypothesized above.
Finally, sexual selection by females for males that are more resistant to aging, especially at older ages, or by males for females that are more prone to allocate in reproduction, could also partially account for the difference in senescence patterns between males and females in our population (Promislow, 2003). Although there is currently little information on sexual selection in the Alpine swift2 phylogenetic comparative analyses are suggesting that fork depth is under sexual selection in swifts (Hasegawa and Arai, 2020).
Asynchrony in senescence across traits
Our results support the growing evidence that asynchrony in senescence between traits is a common pattern in wild animals (Bouwhuis et al., 2012; Nussey et al., 2013; Hayward et al., 2015; Bouwhuis and Vedder, 2017; Cooper et al., 2021; Fay et al., 2021). In the Alpine swifts, tail length started to senescence earlier in males (~9 years) than in females (~15 years) and presented faster rate of senescence than other biometric traits. Among the different tail shapes of birds, forked tails, as for example in swifts and swallows, are more vulnerable to damage (Thomas, 1997). Moreover, aerodynamic performances of birds are more sensitive to a variation of the shape of the wings than the tail (Thomas, 1997). Thus, a decrease in tail length would have a lower impact on flight performances compared to a decrease in wing length. From these observations, and because the tail of birds also shows higher fluctuating asymmetry than the wings, Thomas (1997) suggested that natural selection might act differently on the tail and wings. This assumption could also partly explain the higher senescence of the tail observed in the Alpine swift, compared to the wings. The length of the tail might not be subjected to strong selection for better resistance, and neither would benefit for a selection in greater allocation in self-maintenance to preserve its length.
Regarding reproductive traits that show senescence in females, it is interesting to note that the effects of senescence are becoming increasingly evident as allocation to reproduction progresses, from no detectable effects on egg laying and clutch size to detectable effects on brood size at hatching and fledging. It suggests that reproductive senescence in females might be linked to an accumulation of costs of reproduction, from egg laying to incubation and food provisioning. Our results are in line with the study of Bouwhuis et al. (2009) on female great tits, which found that the age at peak of performance occurs earlier for the number of fledglings than the clutch size, and that the number of fledglings declines post-peak contrary to the clutch size. However, the opposite pattern was observed in the White-tailed ptarmigan (Lagopus leucurus) (Wiebe and Martin, 1998). Individuals from the oldest age classes showed senescence of early breeding stage traits (lay date, clutch size) but showed the highest renesting rate and fledging success. The identification of traits that senesce at faster rates within and across species may provide a valuable starting point to gain insights on the main mechanisms of aging regulating changes in morphology, physical performance, and reproductive success.
Data availability statement
The original contributions presented in the study are publicly available. This data can be found here: Zenodo, Available at: https://doi.org/10.5281/zenodo.8329067.
Ethics statement
The animal study was reviewed and approved by Swiss Federal Agency of Environment, Forests and Landscapes (project number BE[72] and SO[72]).
Author contributions
PB and SR designed the study. PB carried out the field work. HM and PB carried out the statistical analyses. HM drafted the manuscript and all authors revised it. All authors contributed to the article and approved the submitted version.
Funding
Fieldwork was funded over the years by grants from the Swiss National Science Foundation (PA00A-109009, 31003A_124988), Carnegie Trust (RIG007773) and University of Aberdeen Research Board to PB. SR was supported by grants from the Turku Collegium for Science and Medicine, and the Academy of Finland (324257), and HM by grants from the Finnish National Agency for Education (EDUFI Fellowship), and the Biology, Geography, and Geology doctoral program of the University of Turku to HM.
Acknowledgments
We thank the many field workers, and especially Charlotte Karsegard, who contributed to data collection over the 20 years in Biel and Solothurn.
Conflict of interest
The authors declare that the research was conducted in the absence of any commercial or financial relationships that could be construed as a potential conflict of interest.
Publisher’s note
All claims expressed in this article are solely those of the authors and do not necessarily represent those of their affiliated organizations, or those of the publisher, the editors and the reviewers. Any product that may be evaluated in this article, or claim that may be made by its manufacturer, is not guaranteed or endorsed by the publisher.
Supplementary material
The Supplementary Material for this article can be found online at: https://www.frontiersin.org/articles/10.3389/fevo.2023.983266/full#supplementary-material
Footnotes
- ^ Dumas, M. N., St Lawrence, S., Masoero, G., Bize, P., and Martin, J. G. A. Variation in adult body mass is heritable, positively genetically correlated and under antagonistic selection between the sexes in a bird with little apparent sexual dimorphism. J. Anim. Ecol.
- ^ Dumas, M. N., St Lawrence, S., Masoero, G., Bize, P., and Martin, J. G. A. Variation in adult body mass is heritable, positively genetically correlated and under antagonistic selection between the sexes in a bird with little apparent sexual dimorphism. J. Anim. Ecol.
References
Adamkova M., Tomasek O., Albrecht T. (2022). An unexpected age-related pattern in feather growth contributes to age-dependent ornament expression in a passerine bird. J. Ornithol. 163, 987–996 doi: 10.1007/s10336-022-01990-5
Bansal A., Zhu L. J., Yen K., Tissenbaum H. A. (2015). Uncoupling lifespan and healthspan in Caenorhabditis elegans longevity mutants. Proc. Natl. Acad. Sci. U. S. A. 112, 277–286. doi: 10.1073/pnas.1412192112
Bartoń K. (2011) MuMIn: multi-model inference. Available at: https://CRAN.R-project.org/package=MuMIn (Accessed April 19, 2023).
Berman M., Gaillard J.-M., Weimerskirch H. (2009). Contrasted patterns of age-specific reproduction in long-lived seabirds. Proc. R. Soc B Biol. Sci. 276, 375–382. doi: 10.1098/rspb.2008.0925
Bichet C., Moiron M., Matson K. D., Vedder O., Bouwhuis S. (2022). Immunosenescence in the wild? A longitudinal study in a long-lived seabird. J. Anim. Ecol. 91, 458–469. doi: 10.1111/1365-2656.13642
Bize P., Cotting S., Devevey G., van Rooyen J., Lalubin F., Glaizot O., et al. (2014). Senescence in cell oxidative status in two bird species with contrasting life expectancy. Oecologia 174, 1097–1105. doi: 10.1007/s00442-013-2840-3
Bize P., Criscuolo F., Metcalfe N. B., Nasir L., Monaghan P. (2009). Telomere dynamics rather than age predict life expectancy in the wild. Proc. R. Soc B Biol. Sci. 276, 1679–1683. doi: 10.1098/rspb.2008.1817
Bize P., Daniel G., Viblanc V. A., Martin J. G. A., Doligez B. (2017). Negative phenotypic and genetic correlation between natal dispersal propensity and nest-defence behaviour in a wild bird. Biol. Lett. 13, 20170236. doi: 10.1098/rsbl.2017.0236
Bize P., Devevey G., Monaghan P., Doligez B., Christe P. (2008a). Fecundity and survival in relation to resistance to oxidative stress in a free-living bird. Ecology 89, 2584–2593. doi: 10.1890/07-1135.1
Bize P., Gasparini J., Klopfenstein A., Altwegg R., Roulin A. (2006a). Melanin-based coloration is a nondirectionally selected sex-specific signal of offspring development in the alpine swift. Evol. Int. J. Org. Evol. 60, 2370–2380. doi: 10.1111/j.0014-3820.2006.tb01871.x
Bize P., Jeanneret C., Klopfenstein A., Roulin A. (2008b). What makes a host profitable? Parasites balance host nutritive resources against immunity. Am. Nat. 171, 107–118. doi: 10.1086/523943
Bize P., Metcalfe N. B., Roulin A. (2006b). Catch-up growth strategies differ between body structures: interactions between age and structure-specific growth in wild nestling Alpine Swifts. Funct. Ecol. 20, 857–864. doi: 10.1111/j.1365-2435.2006.01157.x
Bize P., Roulin A., Tella J. L., Richner H. (2005). Female-biased mortality in experimentally parasitized Alpine Swift Apus melba nestlings. Funct. Ecol. 19, 405–413. doi: 10.1111/j.1365-2435.2005.00995.x
Bize P., Roulin A., Bersier L.-F., Pfluger D., Richner H. (2003). Parasitism and developmental plasticity in Alpine swift nestlings. J. Anim. Ecol. 72, 633–639. doi: 10.1046/j.1365-2656.2003.00734.x
Bize P., Roulin A., Richner H. (2004a). Parasitism, developmental plasticity and bilateral asymmetry of wing feathers in alpine swift, apus melba, nestlings. Oikos 106, 317–323. doi: 10.1111/j.0030-1299.2004.13019.x
Bize P., Roulin A., Tella J. L., Bersier L.-F., Richner H. (2004b). Additive effects of ectoparasites over reproductive attempts in the long-lived alpine swift. J. Anim. Ecol. 73, 1080–1088. doi: 10.1111/j.0021-8790.2004.00880.x
Bonduriansky R., Maklakov A., Zajitschek F., Brooks R. (2008). Sexual selection, sexual conflict and the evolution of ageing and life span. Funct. Ecol. 22, 443–453. doi: 10.1111/j.1365-2435.2008.01417.x
Bouwhuis S., Charmantier A., Verhulst S., Sheldon B. C. (2010). Trans-generational effects on ageing in a wild bird population. J. Evol. Biol. 23, 636–642. doi: 10.1111/j.1420-9101.2009.01929.x
Bouwhuis S., Choquet R., Sheldon B. C., Verhulst S. (2012). The forms and fitness cost of senescence: age-specific recapture, survival, reproduction, and reproductive value in a wild bird population. Am. Nat. 179, 15–27. doi: 10.1086/663194
Bouwhuis S., Sheldon B. C., Verhulst S., Charmantier A. (2009). Great tits growing old: selective disappearance and the partitioning of senescence to stages within the breeding cycle. Proc. R. Soc B Biol. Sci. 276, 2769–2777. doi: 10.1098/rspb.2009.0457
Bouwhuis S., Vedder O. (2017). “Avian escape artists?: Patterns, processes and costs of senescence in wild birds,” in The evolution of senescence in the tree of life. Eds. Shefferson R. P., Jones O. R., Salguero-Gómez R. (Cambridge, UK: Cambridge University Press), 156–174. doi: 10.1017/9781139939867.008
Bronikowski A. M., Meisel R. P., Biga P. R., Walters J. R., Mank J. E., Larschan E., et al. (2022). Sex-specific aging in animals: Perspective and future directions. Aging Cell 21, e13542. doi: 10.1111/acel.13542
Burger J. M. S., Promislow D. E. L. (2006). Are functional and demographic senescence genetically independent? Exp. Gerontol. 41, 1108–1116. doi: 10.1016/j.exger.2006.08.008
Burnham K. P., Anderson D. R. (2002). Model selection and multimodel inference. (New York, USA: Springer). doi: 10.1007/b97636
Catry P., Phillips R. A., Phalan B., Croxall J. P. (2006). Senescence effects in an extremely long-lived bird: the grey-headed albatross Thalassarche chrysostoma. Proc. Biol. Sci. 273, 1625–1630. doi: 10.1098/rspb.2006.3482
Clutton-Brock T. H. (1984). Reproductive effort and terminal investment in iteroparous animals. Am. Nat. 123, 212–229. doi: 10.1086/284198
Clutton-Brock T. H., Isvaran K. (2007). Sex differences in ageing in natural populations of vertebrates. Proc. Biol. Sci. 274, 3097–3104. doi: 10.1098/rspb.2007.1138
Clutton-Brock T., Sheldon B. C. (2010). Individuals and populations: the role of long-term, individual-based studies of animals in ecology and evolutionary biology. Trends Ecol. Evol. 25, 562–573. doi: 10.1016/j.tree.2010.08.002
Cooper E. B., Bonnet T., Osmond H. L., Cockburn A., Kruuk L. E. B. (2021). Aging and Senescence across Reproductive Traits and Survival in Superb Fairy-Wrens (Malurus cyaneus). Am. Nat. 197, 111–127. doi: 10.1086/711755
Coulson J. C., Fairweather J. A. (2001). Reduced reproductive performance prior to death in the Black-legged Kittiwake: senescence or terminal illness? J. Avian Biol. 32, 146–152. doi: 10.1034/j.1600-048X.2001.320207.x
Dingemanse N. J., Moiron M., Araya-Ajoy Y. G., Mouchet A., Abbey-Lee R. N. (2020). Individual variation in age-dependent reproduction: Fast explorers live fast but senesce young? J. Anim. Ecol. 89, 601–613. doi: 10.1111/1365-2656.13122
Evans M. R., Thomas A. L. R. (1992). The aerodynamic and mechanical effects of elongated tails in the scarlet-tufted malachite sunbird: measuring the cost of a handicap. Anim. Behav. 43, 337–347. doi: 10.1016/S0003-3472(05)80229-5
Fay R., Ravussin P.-A., Arrigo D., Rönn J., Schaub M. (2021). Age-specific reproduction in female pied flycatchers: evidence for asynchronous aging. Oecologia 196, 723–734. doi: 10.1007/s00442-021-04963-2
Fletcher Q. E., Selman C. (2015). Aging in the wild: Insights from free-living and non-model organisms. Exp. Gerontol. 71, 1–3. doi: 10.1016/j.exger.2015.09.015
Forslund P., Pärt T. (1995). Age and reproduction in birds — hypotheses and tests. Trends Ecol. Evol. 10, 374–378. doi: 10.1016/S0169-5347(00)89141-7
Frankish C. K., Manica A., Phillips R. A. (2020). Effects of age on foraging behavior in two closely related albatross species. Mov. Ecol. 8, 7. doi: 10.1186/s40462-020-0194-0
Froy H., Phillips R. A., Wood A. G., Nussey D. H., Lewis S. (2013). Age-related variation in reproductive traits in the wandering albatross: evidence for terminal improvement following senescence. Ecol. Lett. 16, 642–649. doi: 10.1111/ele.12092
Hammers M., Komdeur J., Kingma S. A., Hutchings K., Fairfield E. A., Gilroy D. L., et al. (2016). Age-specific haemosporidian infection dynamics and survival in Seychelles warblers. Sci. Rep. 6, 29720. doi: 10.1038/srep29720
Hasegawa M., Arai E. (2020). Fork tails evolved differently in swallows and swifts. J. Evol. Biol. 33, 911–919. doi: 10.1111/jeb.13622
Hayward A. D., Moorad J., Regan C. E., Berenos C., Pilkington J. G., Pemberton J. M., et al. (2015). Asynchrony of senescence among phenotypic traits in a wild mammal population. Exp. Gerontol. 71, 56–68. doi: 10.1016/j.exger.2015.08.003
Johnsen A., Delhey K., Schlicht E., Peters A., Kempenaers B. (2005). Male sexual attractiveness and parental effort in blue tits: a test of the differential allocation hypothesis. Anim. Behav. 70, 877–888. doi: 10.1016/j.anbehav.2005.01.005
Kelly C., Stoehr A., Nunn C., Smyth K., Prokop Z. (2018). Sexual dimorphism in immunity across animals: a meta-analysis. Ecol. Lett. 21, 1885–1894. doi: 10.1111/ele.13164
Kirkwood T. B. L. (2002). Evolution of ageing. Mech. Ageing Dev. 123, 737–745. doi: 10.1016/s0047-6374(01)00419-5
Komers P. E., Dhindsa M. S. (1989). Influence of dominance and age on mate choice in black-billed magpies: an experimental study. Anim. Behav. 37, 645–655. doi: 10.1016/0003-3472(89)90043-2
Lecomte V. J., Sorci G., Cornet S., Jaeger A., Faivre B., Arnoux E., et al. (2010). Patterns of aging in the long-lived wandering albatross. Proc. Natl. Acad. Sci. 107, 6370–6375. doi: 10.1073/pnas.0911181107
Lemaître J.-F., Gaillard J.-M. (2017). Reproductive senescence: new perspectives in the wild. Biol. Rev. 92, 2182–2199. doi: 10.1111/brv.12328
Lemaître J.-F., Ronget V., Tidière M., Allainé D., Berger V., Cohas A., et al. (2020). Sex differences in adult lifespan and aging rates of mortality across wild mammals. Proc. Natl. Acad. Sci. U. S. A. 117, 8546–8553. doi: 10.1073/pnas.1911999117
Liechti F., Witvliet W., Weber R., Bächler E. (2013). First evidence of a 200-day non-stop flight in a bird. Nat. Commun. 4, 2554. doi: 10.1038/ncomms3554
Martin G. M., Bergman A., Barzilai N. (2007). Genetic determinants of human health span and life span: progress and new opportunities. PloS Genet. 3, 1121–1130. doi: 10.1371/journal.pgen.0030125
Martin J. G. A., Festa-Bianchet M. (2011). Age-independent and age-dependent decreases in reproduction of females. Ecol. Lett. 14, 576–581. doi: 10.1111/j.1461-0248.2011.01621.x
Martins T. L. F., Wright J. (1993). Cost of reproduction and allocation of food between parent and young in the swift (Apus apus). Behav. Ecol. 4, 213–223. doi: 10.1093/beheco/4.3.213
Masoro E. J., Austad S. N. (2005). Handbook of the biology of aging (Amsterdam: Elsevier Academic Press).
Maynard Smith J. (1962). Review lectures on senescence - I. the causes of ageing. Proc. R. Soc. B. Biol. Sci. 115–127 doi: 10.1098/rspb.1962.0065
Meier C. M., Karaardıç H., Aymí R., Peev S. G., Witvliet W., Liechti F. (2020). Population-specific adjustment of the annual cycle in a super-swift trans-Saharan migrant. J. Avian Biol. 51, e02515. doi: 10.1111/jav.02515
Michl G., Török J., Péczely P., Garamszegi L. Z., Schwabl H. (2005). Female collared flycatchers adjust yolk testosterone to male age, but not to attractiveness. Behav. Ecol. 16, 383–388. doi: 10.1093/beheco/ari002
Møller A. P., Arriero E., Lobato E., Merino S. (2009a). A meta-analysis of parasite virulence in nestling birds. Biol. Rev. 84, 567–588. doi: 10.1111/j.1469-185X.2009.00087.x
Møller A. P., De Lope F. (1999). Senescence in a short-lived migratory bird: age-dependent morphology, migration, reproduction and parasitism. J. Anim. Ecol. 68, 163–171. doi: 10.1046/j.1365-2656.1999.00274.x
Møller A. P., Mousseau T. A., Rudolfsen G., Balbotin J., Marzal A., Hermosell I., et al. (2009b). Senescent sperm performance in old male birds. J. Evol. Biol. 22, 334–344. doi: 10.1111/j.1420-9101.2008.01650.x
Monaghan P., Nager R. G., Houston D. C. (1998). The price of eggs: increased investment in egg production reduces the offspring rearing capacity of parents. Proc. Biol. Sci. 265, 1731–1735. doi: 10.1098/rspb.1998.0495
Moorad J. A., Ravindran S. (2022). Natural selection and the evolution of asynchronous aging. Am. Nat. 199, 551–563. doi: 10.1086/718589
Murgatroyd M., Roos S., Evans R., Sansom A., Whitfield D. P., Sexton D., et al. (2018). Sex-specific patterns of reproductive senescence in a long-lived reintroduced raptor. J. Anim. Ecol. 87, 1587–1599. doi: 10.1111/1365-2656.12880
Nager R. G., Monaghan P., Houston D. C. (2000). Within-clutch trade-offs between the number and quality of eggs: experimental manipulations in gulls. Ecology 81, 1339–1350. doi: 10.2307/177212
Nager R. G., Monaghan P., Houston D. C. (2001). The cost of egg production: increased egg production reduces future fitness in gulls. J. Avian Biol. 32, 159–166. doi: 10.1034/j.1600-048X.2001.320209.x
Norberg U. M. (1995a). How a long tail and changes in mass and wing shape affect the cost for flight in animals. Funct. Ecol. 9, 48–54. doi: 10.2307/2390089
Norberg U. M. (1995b). Wing design and migratory flight. Isr. J. Zool. 41, 297–305 doi: 10.1080/00212210.1995.10688801
Nussey D. H., Coulson T., Festa-Bianchet M., Gaillard J.-M. (2008). Measuring senescence in wild animal populations: towards a longitudinal approach. Funct. Ecol. 22, 393–406. doi: 10.1111/j.1365-2435.2008.01408.x
Nussey D. H., Froy H., Lemaitre J.-F., Gaillard J.-M., Austad S. N. (2013). Senescence in natural populations of animals: widespread evidence and its implications for bio-gerontology. Ageing Res. Rev. 12, 214–225. doi: 10.1016/j.arr.2012.07.004
Nussey D. H., Kruuk L. E. B., Morris A., Clements M. N., Pemberton J. M., Clutton-Brock T. H. (2009). Inter- and intrasexual variation in aging patterns across reproductive traits in a wild red deer population. Am. Nat. 174, 342–357. doi: 10.1086/603615
Owen J. P., Nelson A. C., Clayton D. H. (2010). Ecological immunology of bird-ectoparasite systems. Trends Parasitol. 26, 530–539. doi: 10.1016/j.pt.2010.06.005
Pardo D., Barbraud C., Weimerskirch H. (2013). Females better face senescence in the wandering albatross. Oecologia 173, 1283–1294. doi: 10.1007/s00442-013-2704-x
Peters A., Delhey K., Nakagawa S., Aulsebrook A., Verhulst S. (2019). Immunosenescence in wild animals: meta-analysis and outlook. Ecol. Lett. 22, 1709–1722. doi: 10.1111/ele.13343
Promislow D. (2003). Mate choice, sexual conflict, and evolution of senescence. Behav. Genet. 33, 191–201. doi: 10.1023/a:1022562103669
Rattiste K. (2004). Reproductive success in presenescent common gulls (Larus canus): the importance of the last year of life. Proc. R. Soc Lond. B Biol. Sci. 271, 2059–2064. doi: 10.1098/rspb.2004.2832
R Core Team (2022) R: A language and environment for statistical computing (Vienna Austria: R Found. Stat. Comput). Available at: https://www.r-project.org/ (Accessed September 27, 2022).
Reed T. E., Kruuk L. E. B., Wanless S., Frederiksen M., Cunningham E. J. A., Harris M. P. (2008). Reproductive senescence in a long-lived seabird: rates of decline in late-life performance are associated with varying costs of early reproduction. Am. Nat. 171, 89–101. doi: 10.1086/524957
Reid J. M., Bignal E. M., Bignal S., McCracken D. I., Monaghan P. (2003). Age-specific reproductive performance in red-billed choughs pyrrhocorax pyrrhocorax: patterns and processes in a natural population. J. Anim. Ecol. 72, 765–776. doi: 10.1046/j.1365-2656.2003.00750.x
Saraux C., Chiaradia A. (2022). Age-related breeding success in little penguins: a result of selection and ontogenetic changes in foraging and phenology. Ecol. Monogr. 92, e01495. doi: 10.1002/ecm.1495
Sayer A. A., Kirkwood T. B. L. (2015). Grip strength and mortality: a biomarker of ageing? Lancet 386, 226–227. doi: 10.1016/S0140-6736(14)62349-7
Schielzeth H., Dingemanse N. J., Nakagawa S., Westneat D. F., Allegue H., Teplitsky C., et al. (2020). Robustness of linear mixed-effects models to violations of distributional assumptions. Methods Ecol. Evol. 11, 1141–1152. doi: 10.1111/2041-210X.13434
Segami J. C., Lind M. I., Qvarnström A. (2021). Should females prefer old males? Evol. Lett. 5, 507–520. doi: 10.1002/evl3.250
Sparkman A. M., Blois M., Adams J., Waits L., Miller D. A. W., Murray D. L. (2017). Evidence for sex-specific reproductive senescence in monogamous cooperatively breeding red wolves. Behav. Ecol. Sociobiol. 71, 1–11. doi: 10.1007/s00265-016-2241-9
Tettamanti F., Grignolio S., Filli F., Apollonio M., Bize P. (2015). Senescence in breeding success of female Alpine chamois (Rupicapra rupicapra): the role of female quality and age. Oecologia 178, 187–195. doi: 10.1007/s00442-014-3197-y
Tettamanti F., Witvliet W., Bize P. (2012). Selection on age at first and at last reproduction in the long-lived Alpine Swift Apus melba. Ibis 154, 338–344. doi: 10.1111/j.1474-919X.2012.01215.x
Thomas A. L. R. (1993a). On the aerodynamics of birds’ tails. Philos. Trans. R. Soc Lond. B. Biol. Sci. 340, 361–380. doi: 10.1098/rstb.1993.0079
Thomas A. L. R. (1993b). The aerodynamic costs of asymmetry in the wings and tail of birds: asymmetric birds can’t fly round tight corners. Proc. R. Soc Lond. B Biol. Sci. 254, 181–189. doi: 10.1098/rspb.1993.0144
Thorley J., Duncan C., Sharp S. P., Gaynor D., Manser M. B., Clutton-Brock T. (2020). Sex-independent senescence in a cooperatively breeding mammal. J. Anim. Ecol. 89, 1080–1093. doi: 10.1111/1365-2656.13173
van de Pol M., Verhulst S. (2006). Age-dependent traits: a new statistical model to separate within- and between-individual effects. Am. Nat. 167, 766–773. doi: 10.1086/503331
Vaupel J. W., Manton K. G., Stallard E. (1979). The impact of heterogeneity in individual frailty on the dynamics of mortality. Demography 16, 439–454. doi: 10.2307/2061224
Vedder O., Bouwhuis S. (2018). Heterogeneity in individual quality in birds: overall patterns and insights from a study on common terns. Oikos 127, 719–727. doi: 10.1111/oik.04273
Weladji R. B., Gaillard J.-M., Yoccoz N. G., Holand Ø., Mysterud A., Loison A., et al. (2006). Good reindeer mothers live longer and become better in raising offspring. Proc. R. Soc B Biol. Sci. 273, 1239–1244. doi: 10.1098/rspb.2005.3393
Wiebe K. L., Martin K. (1998). Age-specific patterns of reproduction in White-tailed and Willow Ptarmigan Lagopus leucurus and L. lagopus. Ibis 140, 14–24. doi: 10.1111/j.1474-919X.1998.tb04536.x
Wikel S. K. (1996) The immunology of host-ectoparasitic arthropod relationships (CAB International). Available at: https://www.cabi.org/isc/abstract/19970500344 (Accessed October 13, 2022).
Wikel S. K. (1999). Modulation of the Host Immune System by Ectoparasitic Arthropods: Blood-feeding and tissue-dwelling arthropods manipulate host defenses to their advantage. BioScience 49, 311–320. doi: 10.2307/1313614
Williams G. C. (1957). Pleiotropy, natural selection, and the evolution of senescence. Evolution 11, 398–411. doi: 10.2307/2406060
Williams G. C. (1999). The tithonus error in modern gerontology. Q. Rev. Biol. 74, 405–415. doi: 10.1086/394111
Williams T. D. (2005). Mechanisms underlying the costs of egg production. BioScience 55, 39–48. doi: 10.1641/0006-3568(2005)055[0039:MUTCOE]2.0.CO;2
Keywords: life-history theory, senescence, long-lived species, natural selection, selective disappearance, terminal effect, Tachymarptis (Apus) melba
Citation: Moullec H, Reichert S and Bize P (2023) Aging trajectories are trait- and sex-specific in the long-lived Alpine swift. Front. Ecol. Evol. 11:983266. doi: 10.3389/fevo.2023.983266
Received: 30 June 2022; Accepted: 28 August 2023;
Published: 19 September 2023.
Edited by:
Jean-Michel Gaillard, Université Claude Bernard Lyon 1, FranceReviewed by:
Roi Dor, Open University of Israel, IsraelSandra Bouwhuis, Heligoland Bird Observatory, Germany
Karen Wiebe, University of Saskatchewan, Canada
Copyright © 2023 Moullec, Reichert and Bize. This is an open-access article distributed under the terms of the Creative Commons Attribution License (CC BY). The use, distribution or reproduction in other forums is permitted, provided the original author(s) and the copyright owner(s) are credited and that the original publication in this journal is cited, in accordance with accepted academic practice. No use, distribution or reproduction is permitted which does not comply with these terms.
*Correspondence: Héloïse Moullec, heloise.moullec@gmail.com; Pierre Bize, pierre.bize@vogelwarte.ch