- Department of Geography, McGill University, Montreal, QC, Canada
Disturbed salt marshes may recover with little additional management once tidal inundation is restored. We assessed the success of such recovery by comparing the invertebrate biota of Bay of Fundy salt marsh pools in a reference site at Dipper Harbour to that of Saints Rest marsh that had been drained for over a century and to which tidal flooding had been returned ~50 years prior to our study. The sediments and vegetation of salt marsh pools were sampled seasonally throughout one year. Average biomass of pool invertebrates ranged from 1.8 to 4.0 g dry wt m−2, depending on the amount of vegetation cover in the pools. The most abundant organisms of the pools were the gastropod Ecrobia truncata (=Hydrobia tottentei), Tubificidae (=Naididae) oligochaetes, and Chironomidae (=Chironomini). We compared overall abundance and biomass of the invertebrates in the pool communities, assessing the month of sampling, pool elevation, and source marsh as explanatory variables. Our analyses revealed that marsh origin of pools seldom explained a significant amount of variance, and when it did, the proportion of variance explained was usually lower than elevation of pools and month of sampling. Diversity of invertebrates found in all pools was higher at the recovering site with species richness >40% higher than in the reference site. We conclude that after an estimated 50 years since dyke failure and return of tidal flooding to Saints Rest marsh, that the ecosystem function represented by pools and their fauna has recovered.
1 Introduction
Tidal salt marshes are generally flat wetlands considered to be highly productive and dominated by a small number of extremely salt tolerant plant species (Mitsch and Gosselink, 2015). Many tidal salt marshes contain permanently flooded pools that add diversity and value to the habitat provided by the marsh. Pools provide habitat for fish (Smith and Able, 1994; Adamowicz, 2002) and invertebrates (Nicols, 1935, Bromley and Bleakney, 1979, Clarke et al., 1984), and, in turn, valuable feeding sites for birds (Burger et al., 1982; Clarke et al., 1984; Erwin, 1996). Clarke et al. (1984) found that bird use was strongly correlated with pool density in Massachusetts salt marshes, particularly for shorebirds, herons, and terns. These pools may be particularly important on macrotidal coasts such as the Bay of Fundy, where some surfaces of the salt marshes are flooded only intermittently, at extreme (spring) tides (Byers and Chmura, 2007). Standing pools of water on the surface of the marsh provide a refuge for organisms, such as fish and aquatic invertebrates, which would not be able to survive on the marsh surface between flood tide events.
Despite their apparent ecological importance in salt marshes, relatively few studies have focused specifically on the invertebrate biota of pools in natural or restored salt marshes. Early studies found few species in pools (Nixon and Oviatt, 1973; Campbell and Denno, 1978). The challenge of isolation and identification of invertebrate fauna also may discourage investigators from using them as an indicator of restoration success (Laymen and Rypel, 2020). Most salt marshes in the Bay of Fundy (85%) have been altered or lost, with dyking and drainage of marshes beginning in the 17th century by early French Acadian settlers (Butzer, 2002). Beginning in 2010 managed realignment, the construction of a new dyke inland followed by strategic breaching of the original dyke, has been employed to restore some of these drained marshes. Over the first 8 years of monitoring at the first managed realignment site, vegetation at the restored and reference sites showed increasing similarity but recovery of invertebrate populations lagged (Virgin et al., 2020). Indeed, studies of other restored marshes indicate that some components of the ecosystem recover more quickly than others. For instance, creek use by nekton can return to the condition of an undisturbed marsh in as little as one year (Roman et al., 2002), but Allen et al. (1994) found that the forage value for Fundulus heteroclitus of a restored marsh had not recovered after 15 years. In Connecticut, Warren et al. (2002) reported that invertebrate populations may take over 20 years to recover.
On the Bay of Fundy some historic dykes have been breached by storms and tidal marshes subsequently left to recover without human intervention or management. These sites have served as natural laboratories to examine the long-term outcome of salt marsh restoration with respect to surface hydrology (MacDonald et al., 2010) and vegetation (Byers and Chmura, 2007). In this study we compare a previously dyked and drained marsh at Saint John, New Brunswick (Saints Rest marsh, now a protected site) to that of an undisturbed marsh in Dipper Harbour, New Brunswick to determine the extent of recovery of the pool invertebrate fauna. Saints Rest marsh had been subject to an unmanaged recovery following the breaching of the dyke that occurred 50 years prior to this study. As environmental conditions vary with pool elevation (Noël and Chmura 2011), we hypothesized that invertebrate communities would vary with pool elevation, thus include this as a variable in our analyses.
2 Methods
2.1 Study sites
The Bay of Fundy has a steep gradient in tidal range, varying from 4 m near the mouth of the Bay to >16 m at its upper reaches (Canadian Hydrographic Survey, 2005). On the New Brunswick coast of the Bay of Fundy as the tidal range increases, so to do the sediment supply and rates of sediment deposition in the salt marshes (Chmura et al., 2001). The greater the distance between marshes, the greater is the difference in these conditions, and the shift in tidal range also causes a shift in the elevation of mean high water (Gordon et al., 1985). Thus, proximity along the coastline is an essential consideration in selecting reference sites to compare to restored marshes. The Dipper Harbour marsh was the only undisturbed marsh with an appropriate geomorphic context (a single main channel) and accessibility for our year-round study. (See Figure 1 in Noël and Chmura (2011) for the location of each marsh on the Bay of Fundy coast.)
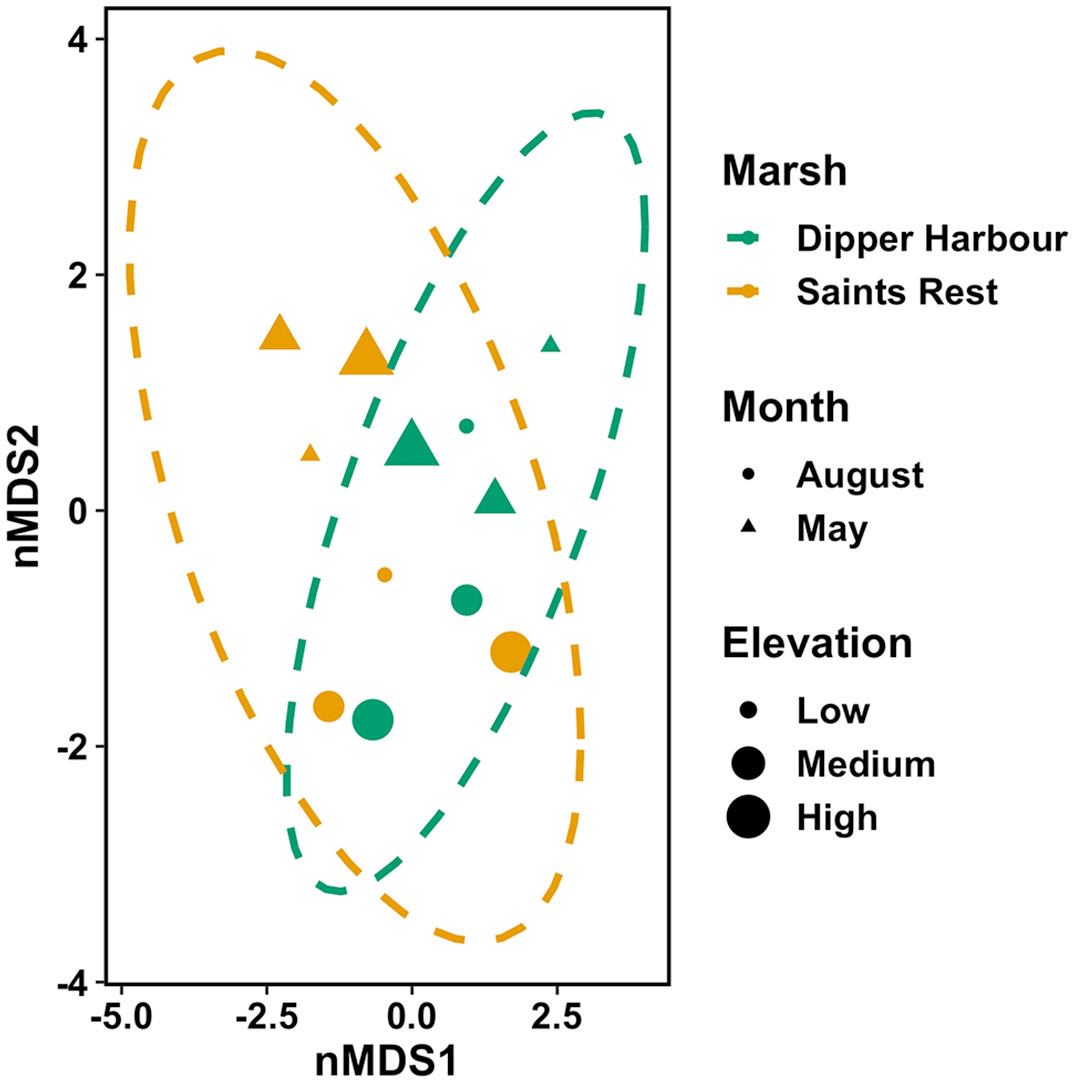
Figure 1 nMDS ordination plot for invertebrate fourth-root of invertebrate dry mass in vegetation of pools from July 2004 to July 2005 at Dipper Harbour and Saints Rest salt marshes using Euclidean distance. Each symbol represents the assemblage of invertebrates in a sample. Plot ellipses represent the 95% confidence regions for group clusters. Stress=0.12.
Saints Rest marsh, located on the lower Bay of Fundy in Saint John, New Brunswick, has a long history of human use and alteration including dyking and ditching dating back at least 140 years, construction of roads, use as a rifle range, and currently a sewage treatment plant discharges into the head of the creek. (See Figure 2 in Noël and Chmura (2011) for a map of Saints Rest marsh and location of pools.) The relatively undisturbed reference site, Dipper Harbour marsh, is 28 km southwest of Saints Rest marsh. (See Figure 3 in Noël and Chmura (2011) for a map of Dipper Harbour marsh and location of pools.) Additional descriptions of the marshes have been provided by Thomas (1983); Chmura et al. (1997); MacDonald et al. (2010), and Byers and Chmura (2007).
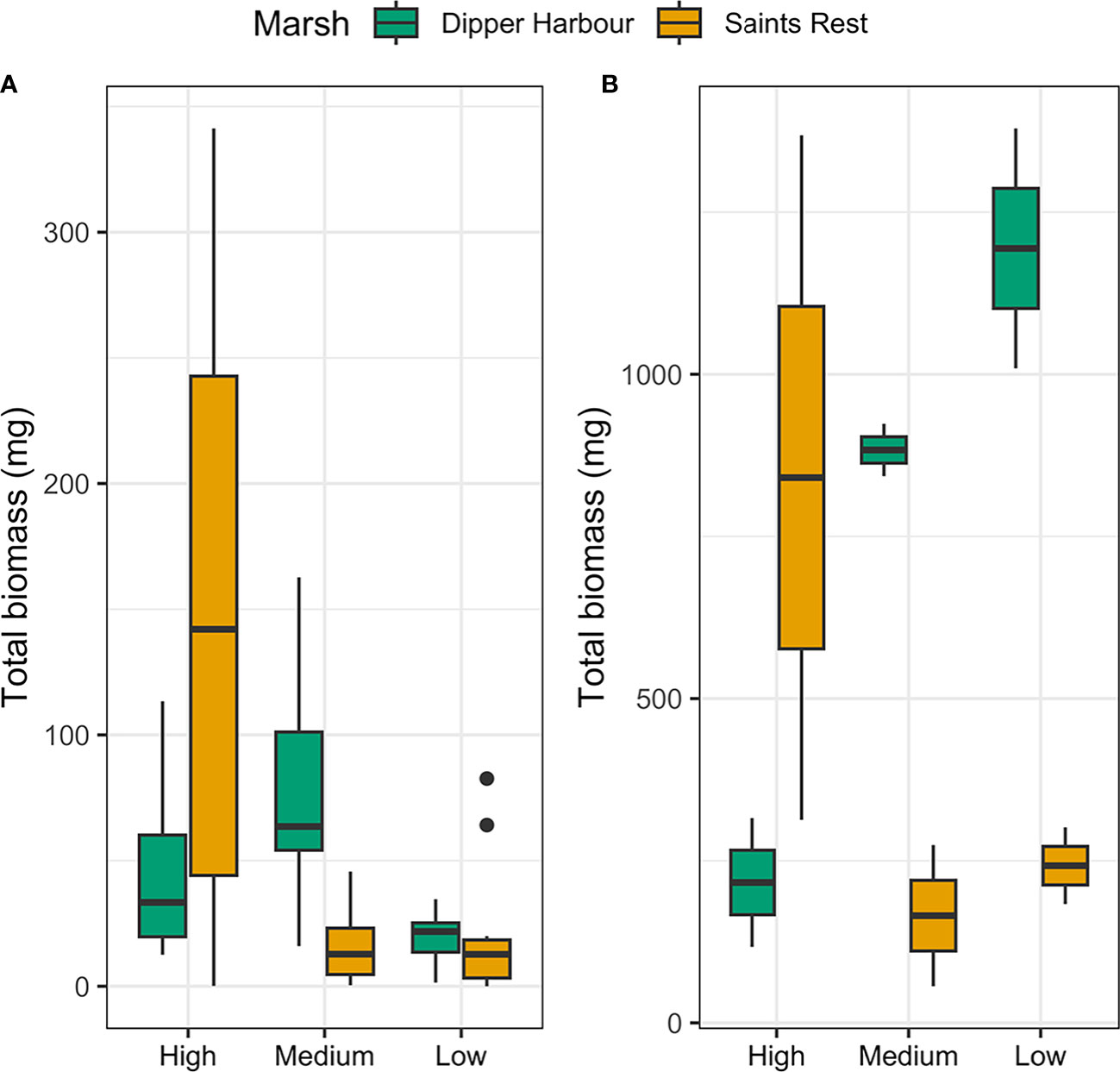
Figure 2 Box plots showing total biomass (mg) of (A) all macrofauna found in 300 cm−3 sediment samples and (B) all invertebrates (mg) in 0.25 m2 samples of vegetation in pools from July 2004 to July 2005 at Dipper Harbour and Saints Rest salt marshes. The horizontal bar represents the median; lower and upper box edges are 25th and 75th percentiles, respectively; whiskers are 1.5 × 25th and 75th percentiles, and dots are outliers.
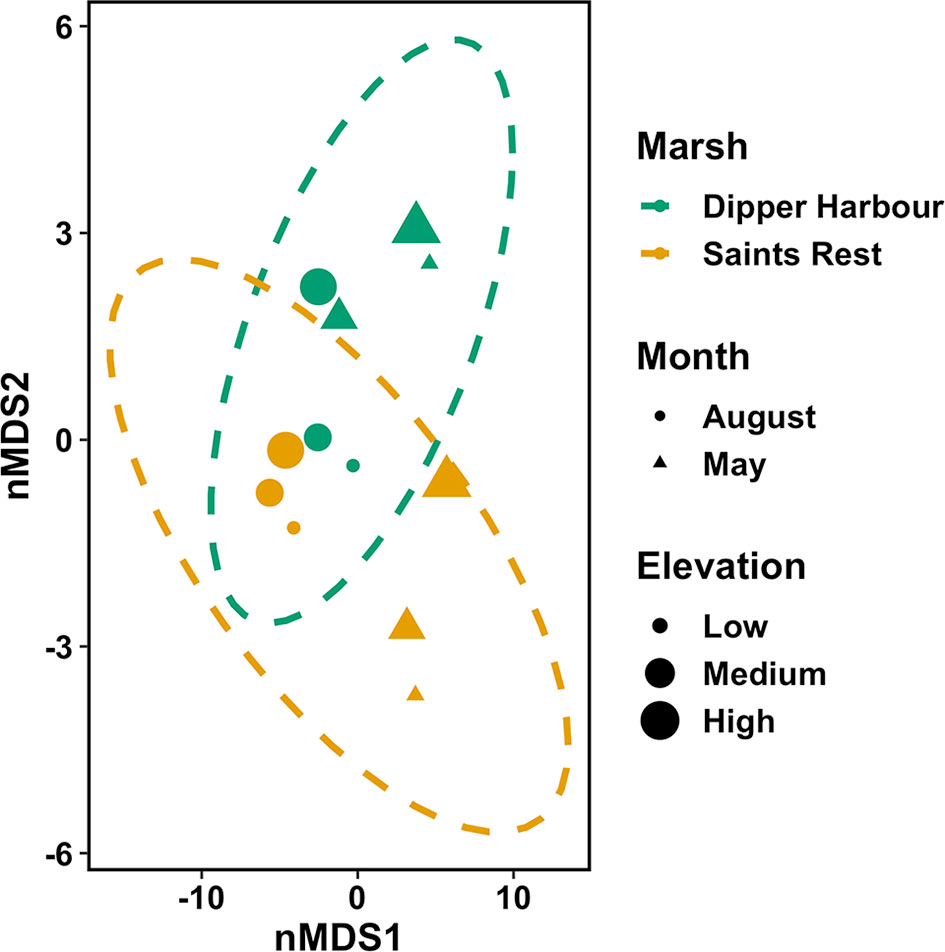
Figure 3 nMDS ordination plot for invertebrate fourth-root abundance of invertebrates in vegetation of salt marsh pools from July 2004 to July 2005 at Dipper Harbour and Saints Rest salt marshes using Euclidean distance. Each symbol represents the assemblage of invertebrates in a sample. Plot ellipses represent the 95% confidence regions for group clusters. Stress=0.04.
Based on surrounding vegetation we selected pools to sample that represented three marsh elevations: “high”, “mid” and “low”. At each marsh one pool from each elevation was selected for environmental analyses as described by Noël and Chmura (2011) – these were the first to be sampled and referred to as our “main” pools.
2.2 Invertebrate sampling
A Wildco™ hand corer with a diameter of 5 cm was used to collect sediment samples to a depth of 15 cm. The sample size was determined to be adequate after constructing a species area curve from repeated sediment samples (5) collected in one pool at Dipper Harbour, although large differences in abundance from sample to sample were noted.
Sediment samples were collected from a pool at each of the three elevations in each marsh during July 28–30, 2004. Between August 25 and September 2 these pools were sampled again, along with 5 additional pools on each marsh, for a total of 8 pools per marsh. The 3 pools first sampled on each marsh were sampled again in May 2005. In each pool, sediment samples were taken ~0.5 m and ~2.5 m in from the edge of the pool and combined for further analyses. The sediment samples were sieved through a 0.5 mm screen and the portion retained was placed in a 5% buffered formalin solution for a few days, then transferred to a 50% isopropyl alcohol solution for preservation until sorting and identification. The samples were transferred to a rose bengal staining solution for 1.5 h to stain the invertebrates so they could be more easily sorted from roots and other debris in the sample using a dissecting microscope. Meiofauna (defined as those passing through a 0.5 mm screen), such as ostracods, foraminifera and copepods, were quantified when encountered in the samples. However, due to the mesh size used these counts cannot be considered complete and so only macroinvertebrates were included in the final analyses of invertebrate communities in the sediments.
On 5 dates from July 2004 to July 2005 the percent cover of aquatic vegetation was visually estimated in the main pools at Dipper Harbour and in the main pools on 4 dates over the same period at Saints Rest (Table 1). In mid-August 2004 and again in May 2005, a single 0.25 m2 sample of aquatic vegetation (widgeon grass, Ruppia maritima, and filamentous algae) was cut from each of the 6 main study pools at a randomly selected location within the pool, and removed carefully so as to not dislodge any invertebrates. Samples taken in August were rinsed multiple times over a 0.5 mm mesh screen. The May samples were not rinsed, which allowed for accurate meiofauna counts as well as macrofauna. The algae and R. maritima were separated and the wet weight of each was determined for all samples.
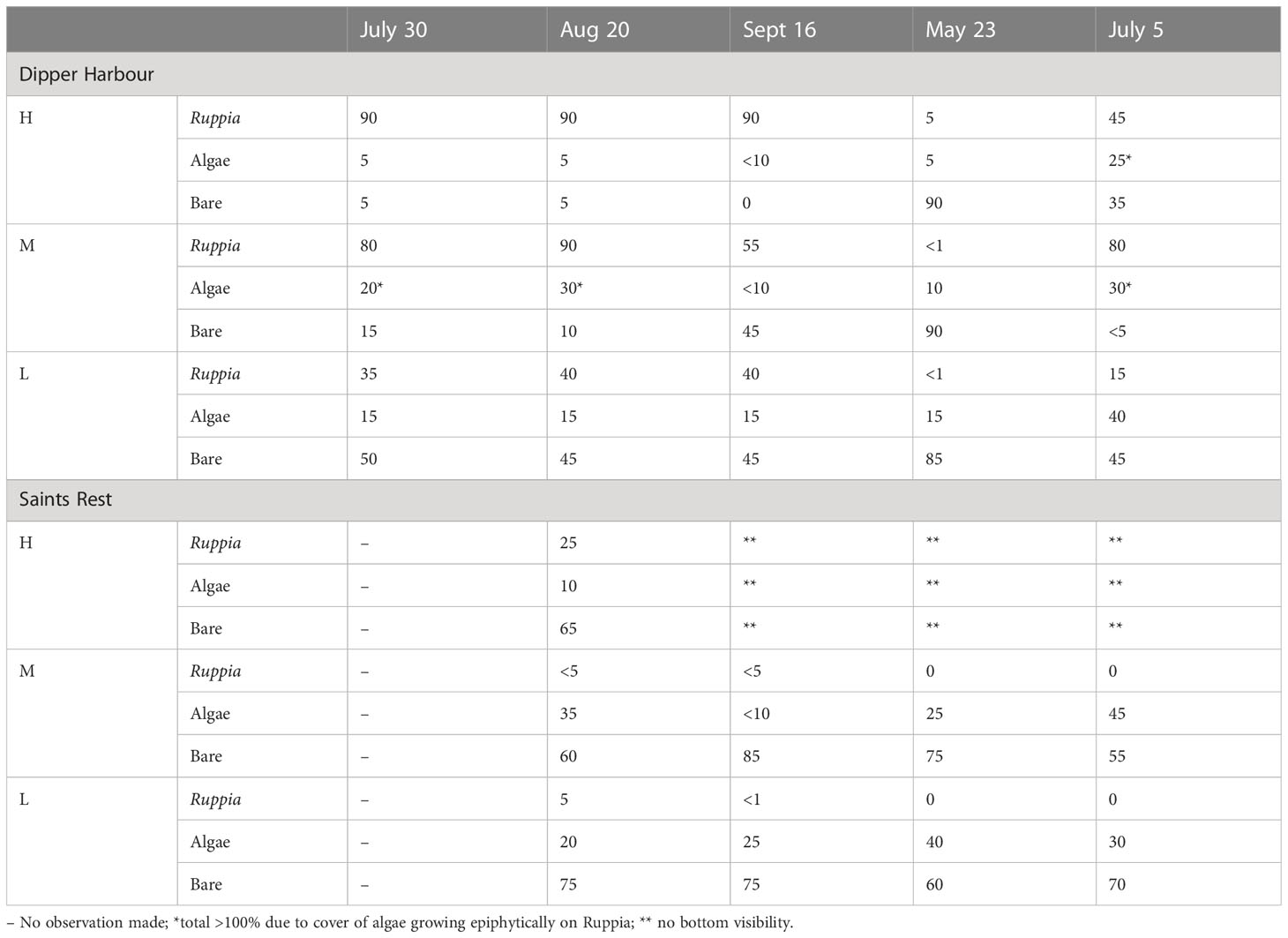
Table 1 Percent cover of aquatic vegetation in high (H), mid (M), and low (L) marsh pools from July 2004 to July 2005.
The filamentous algae from the August samples were identified to genera. Invertebrates in sediment and vegetation samples were hand sorted under a dissecting microscope. Small species and diagnostic features (such as chironomid heads) were mounted on slides and examined under a compound microscope. Invertebrates were identified to the lowest practical taxonomic level using the resources listed in Supplementary Table 1. Voucher specimens were placed in the permanent collection of the Atlantic Reference Centre in St. Andrews, New Brunswick.
Dry weight was determined for species of macrofauna that comprised more than 10% of the total invertebrate abundance of 3 or more samples. To determine dry weight, specimens were randomly selected from samples which contained a large number of individuals of the species to be weighed. With the exception of Ecrobia truncata and Gammarus mucronatus there was little variation in the size and wet weight of most species among samples. For this reason, a subset from the summer and spring sampling periods were weighed separately for only these two species. One to 100 individuals of each species representing more than 5% of total species abundance of samples from the 6 main study pools were dried and weighed. The number of individuals weighed was higher for smaller species. Specimens were soaked in distilled water overnight to remove alcohol, then oven-dried at 120°C for 24 h. To calculate g m−2 we normalized the area of sampled and assumed a 15-cm sediment depth, as this was the depth cored.
2.3 Analyses
Our analyses were designed to compare pool vegetation and invertebrates of the reference marsh, Dipper Harbour to that of the recovering marsh, Saints Rest. Pool elevation (high, mid, and low) and sample month were tested as explanatory variables. All statistical analyses were performed using R.
Species Richness, the Shannon-Wiener index of diversity (H=−∑ pi ln pi, where p=the proportion of taxa i to total taxa count of sample) and equitability (J=H/lnS, where S=species richness) were calculated for all samples and for combined data for each pool (Begon et al., 1990). Species Richness was based on, for example, the total number of species found in sediments of all low pools, and the total found in sediments of all pools in the marsh. The Shannon Wiener index calculation was based on average abundance of species in each pool elevation across all sample events.
To examine the variation in species composition and invertebrate biomass, we used non-metric multidimensional scaling (nMDS) of the two marshes using the fourth-root taxa abundance and biomass data. We used Permutational multivariate analysis of variance (PERMANOVA), with Euclidean distances, to test significance of independent variables on the abundance and biomass of invertebrate taxa (Anderson, 2001) using the Vegan package in R (Oksanen et al., 2008). Note that we only evaluated main effects and not any possible interactions. All the PERMANOVA analysis were done in fourth-root transformed data. Bar plots of taxa abundance data are made with fourth-root transformed data. Bar and boxplots for taxa and total dry biomass are shown in absolute values without transformations. To determine the importance of species in their contribution to dissimilarities between grouping variables we performed SIMPER analysis (Clarke, 1993). As the difference between the marshes was the primary question of this study, our analyses and visualizations primarily focus on contrasting the two marshes. Within-group dispersion was assessed with the vegan package betadisper (Oksanen et al., 2008). If dispersion between groups is unequal, the statistical significance identified using PERMANOVA may result from dispersion patterns rather than the species composition differences. We used ANOVA to test if the dispersions of one or more groups were different. To compare the total biomass in vegetation and sediment samples in the marshes we used a t-test. As the variance in total biomass differed, we used unequal variance t-test. Similarly, to test the differences in total biomass along elevation gradient and across sampling months we used Kruskal Wallis test because of differing variance in the groups.
3 Results
3.1 Pool vegetation
The only vascular plant that grew in most pools was R. maritima (widgeon grass), though isolated stems of Sporobolus alterniflorus (=Spartina alterniflora) frequently encroached into the pools with sloping sides and pools with sloping sides near the upland sometimes graded into stands of Bolboschoenus maritimus (=Schoenoplectus robustus). Zostera marina occurred in a few of the lowest elevation pools. Thick mats of filamentous algae grew in virtually all pools from May to late fall (Table 1). No attempt was made to quantify the abundance of each species of filamentous algae, though it was noted that Cladophora sp. was the dominant species of the algal mats and Ulva (=Enteromorpha) sp. tended to occur in some areas of the pools. Less common algae identified from the pools included Rhizoclonium sp., Cylindrocapsa sp. and Ectocarpus sp. Cyanobacteria (Cyanophyceae) were epiphytic on species of algae, on R. maritima, and also on bits of wood and rhizomes mixed in with the samples.
3.2 Invertebrates
3.2.1 Invertebrates in pool vegetation
Fauna in pool vegetation averaged 0.86 g dry weight per 100 g of wet vegetation. The average weight of vegetation in a 0.25 m−2 sample was 65 g, giving an average macroinvertebrate biomass of 2.24 g m−2. We considered counts of 21 invertebrate taxa found in our samples from pool vegetation for our statistical analyses (Supplementary Table 2). Eight taxa were retained for analysis by biomass (Supplementary Table 3). There was no significant difference in biomass within vegetation samples across sampling months or as a function of elevation. Marsh explains 20% of the variability (Table 2), but there is no clear separation of marshes visible in the corresponding nMDS ordination plot (Figure 1). Although total invertebrate biomass within pool vegetation showed some difference between the marshes (including a differing elevational pattern; Figure 2B), the effect of marsh site was not significant (t=1.29, df=10, p=0.22).
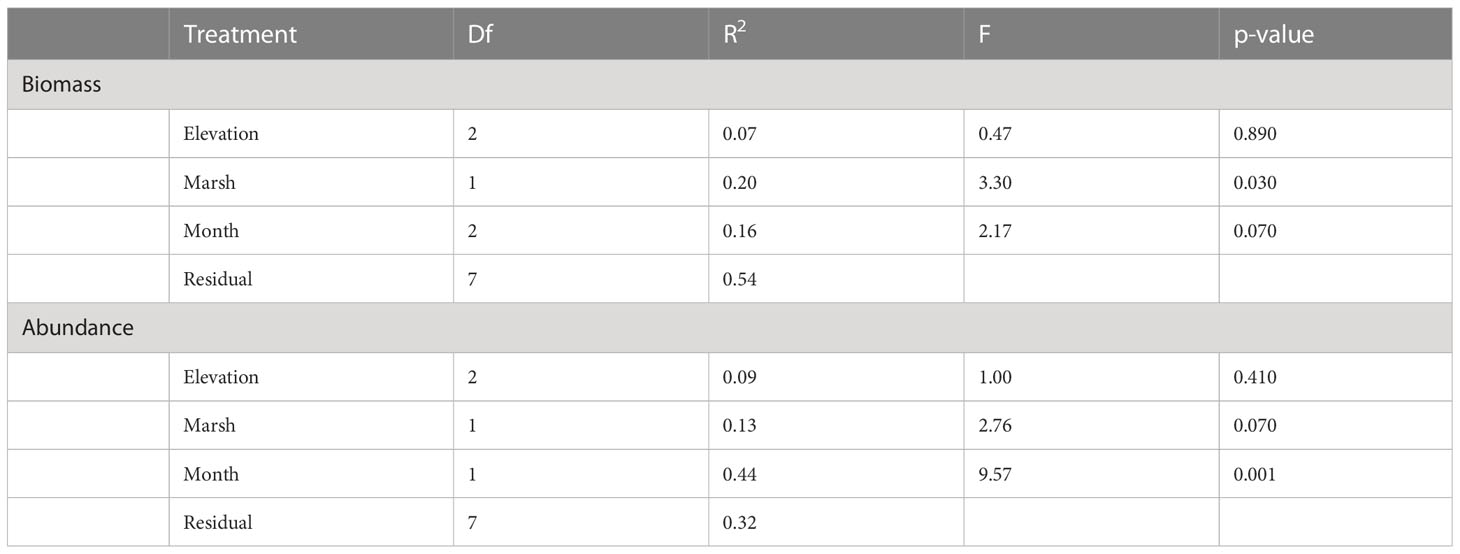
Table 2 Results of PERMANOVA with Euclidean distance with 10,000 permutations for biomass and abundances of invertebrates in vegetation of pools at Dipper Harbour and Saints Rest salt marshes from July 2004 to July 2005.
The nMDS ordination plot (Figure 3) of invertebrate community composition based upon abundance in the 6 main pools showed that communities of the two marshes are generally distinct in May, but not in August. There was no significant difference in species composition recovered from vegetation samples based on elevation or marsh, while there was a significant difference by sampling month (Table 2). Sampling month explained 44% of the variability. Comparing dispersion between marshes using ANOVA revealed no significant difference between the two marshes (F=4.56, df=1 & 10, p=0.06). SIMPER analysis identified the cumulative combinations of the most influential taxa as, in descending order, copepods, ostracods, Tubificidae (=Naididae) and Ecrobia truncata in distinguishing the difference in communities in August 2004 and May 2005. Whereas ostracods, copepods, Tubificidae, Ceratopogonidae and Chrinomidae (=Chironomini), in descending order, explained 54% of the differences observed between marshes in vegetation samples (Figure 4).
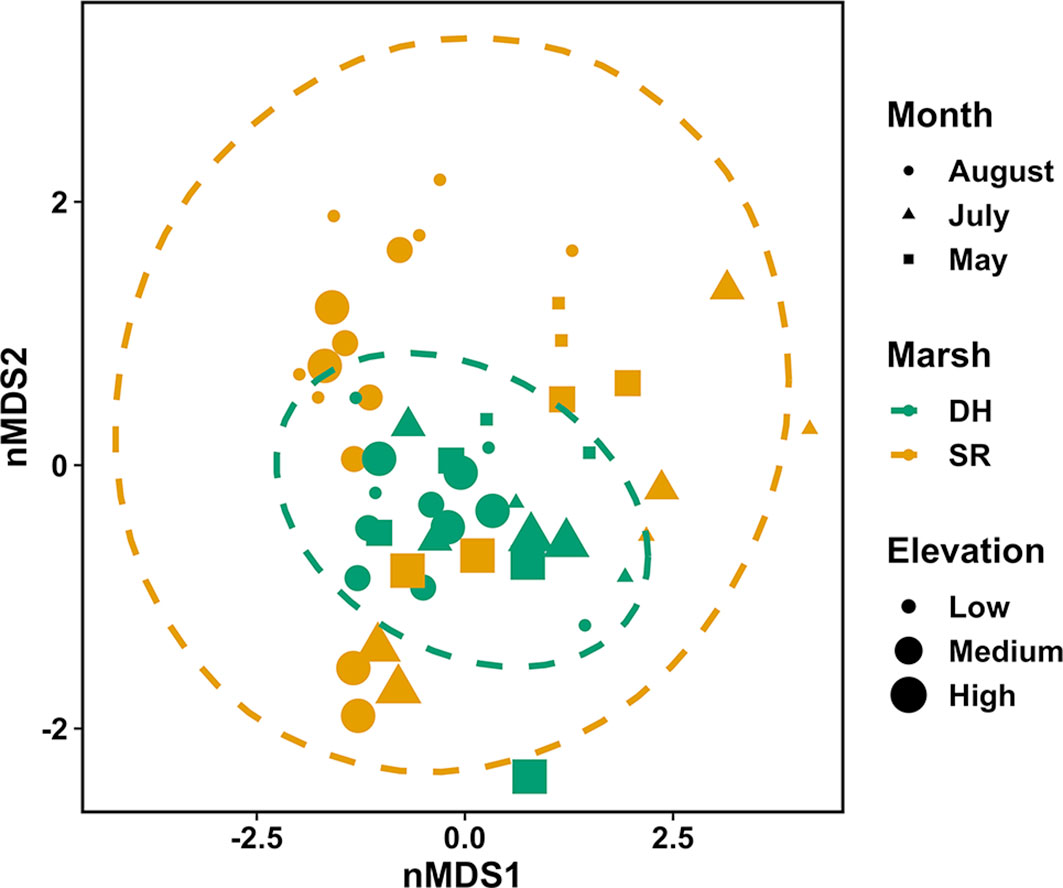
Figure 4 nMDS ordination plot for fourth-root abundance of macroinvertebrates in sediments of salt marsh pools from July 2004 to July 2005 at Dipper Harbour and Saints Rest salt marshes using Euclidean distance. Each symbol represents the assemblage of invertebrates in a sample. Plot ellipses represent the 95% confidence regions for group clusters. Stress=0.17.
The overall species richness (the total in all pools) of invertebrates in vegetation of Dipper Harbour pools was 16 while that at Saints Rest was slightly higher, 19, and this pattern was consistent when considering pools at individual elevations (Table 3). Diversity, as expressed by the Shannon Weiner Index (based upon abundances) was also higher at Saints Rest, when considering the total in all pools, yet its value was lower in for the low and high elevation pools at Saints Rest.
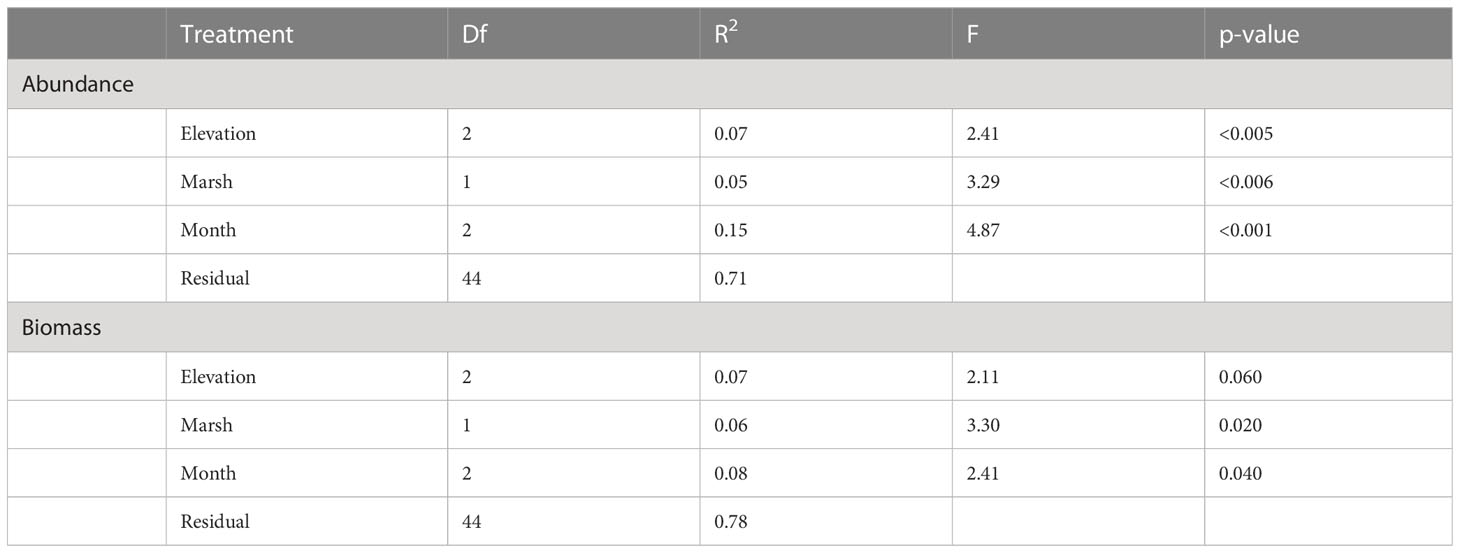
Table 3 Results of PERMANOVA with Euclidean distance with 10,000 permutations for abundance and biomass of invertebrates in sediments of pools at Dipper Harbour and Saints Rest salt marshes from July 2004 to July 2005.
3.2.2 Invertebrates in pool sediments
We considered counts of 23 invertebrate taxa found in our samples from pool sediments for our statistical analyses (Supplementary Table 4). Marsh, sampling month and elevation significantly contribute to difference in abundance of the invertebrate communities (Table 3). The largest variation was explained by sampling month (15%), followed by elevation (7%), with marsh contributing the least (5%). A comparison of variance between Saints Rest and Dipper Harbour marshes showed a significant difference in beta dispersion (F=18.79, df=1 & 48, p<0.001), with Saints Rest having higher variance (Figure 4). The SIMPER analysis showed that most influential taxa contributing a cumulative 70% of the dissimilarity between the two marshes are, in descending order, Ecrobia truncata, Tubificidae (=Naididae), Chironomidae (=Chironomini), Coroxidae, Ephydridae, Gammarus mucronatus and Manayunkia aestuaria (Figure 5).
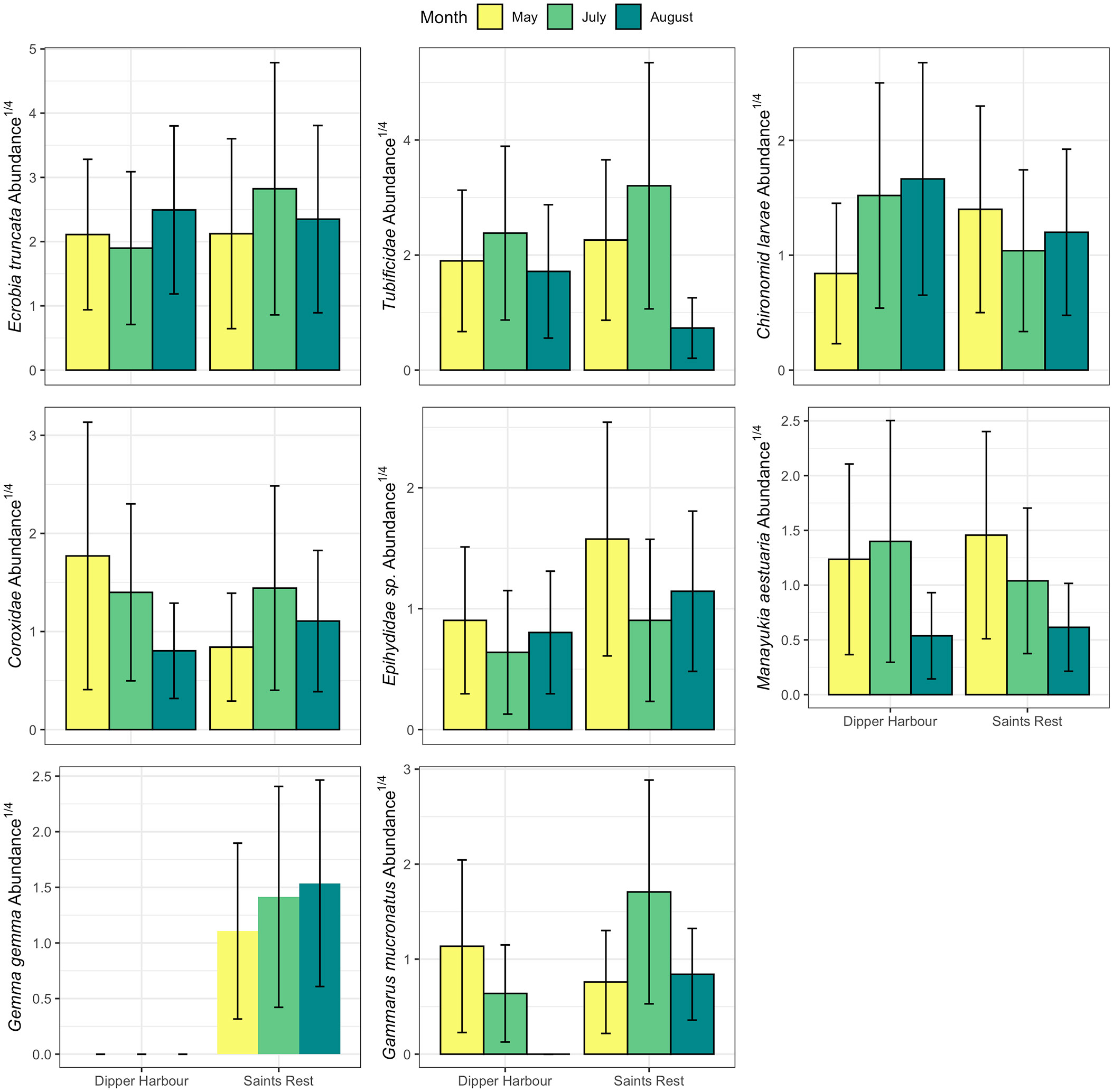
Figure 5 Mean (and se) of fourth-root abundance of the most influential macroinvertebrates taxa contributing a cumulative 70% of the dissimilarity between the two marshes in 300 cm−3-samples of sediments from salt marsh pools sampled in May, July and August in 2004 and 2005 and averaged for pool elevation. For each marsh n=6 for May and July and in August, n=12 and 14 for Saints Rest and Dipper Harbour, respectively.
The average total biomass of invertebrates in the pool sediment was 1.79 g dry weight m−2. When combined with the biomass of invertebrates in the vegetation, the potential total pool biomass was 4.03 g m−2 if there was 100% vegetation coverage. Seven taxa were retained for analysis by biomass (Supplementary Table 5). Although invertebrate total biomass in sediment samples showed some difference between the marshes (Figure 2A), an unequal variance t-test showed that the pattern was not statistically significant (t=0.56, df=35.03, p=0.57). Similarly, using the Kruskal-Wallis test there was no significant difference in biomass in sediment samples across sampling months (Chi-sq=1.53, df=2, p= 0.46). However, a Kruskal Wallis test on sediment samples revealed a statistical difference in total biomass as a function of elevation and higher elevation tended to have higher biomass values (Chi-sq=7.96, df=2, p=0.02).
Using fourth root data of the dry mass for the invertebrate community revealed that both marsh and month show significant influence, albeit marsh explains slightly less of the variability (Table 3). A comparison of variance between Saints Rest and Dipper Harbour marshes showed a significant difference in beta dispersion (F=15.20, df=1 & 48, p<0.001), with Saints Rest having higher variance (Figure 6). SIMPER analysis of dry biomass in fourth root data showed that E. tuncata, Chrinomidae (=Chironomini), and G. mucronatus explain 70% of the dissimilarity between two marsh types (Figure 7).
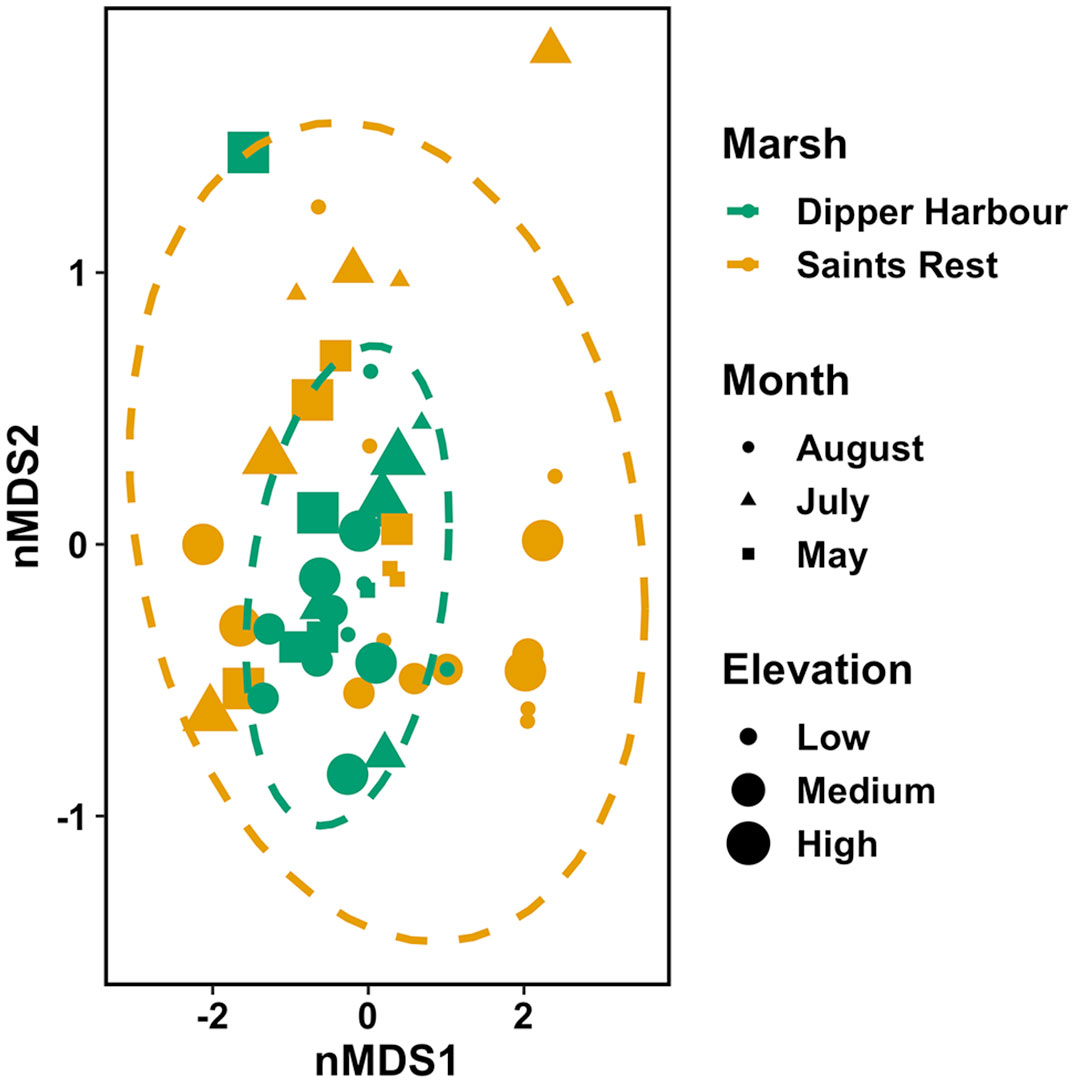
Figure 6 nMDS ordination plot for fourth-root of macroinvertebrate dry biomass (mg) in 300 cm−3-samples of sediment collected from pools from July 2004 to July 2005 at Dipper Harbour and Saints Rest salt marshes using Euclidean distance. Each symbol represents the assemblage of invertebrates in a sample. Plot ellipses represent the 95% confidence regions for group clusters. Stress=0.11.
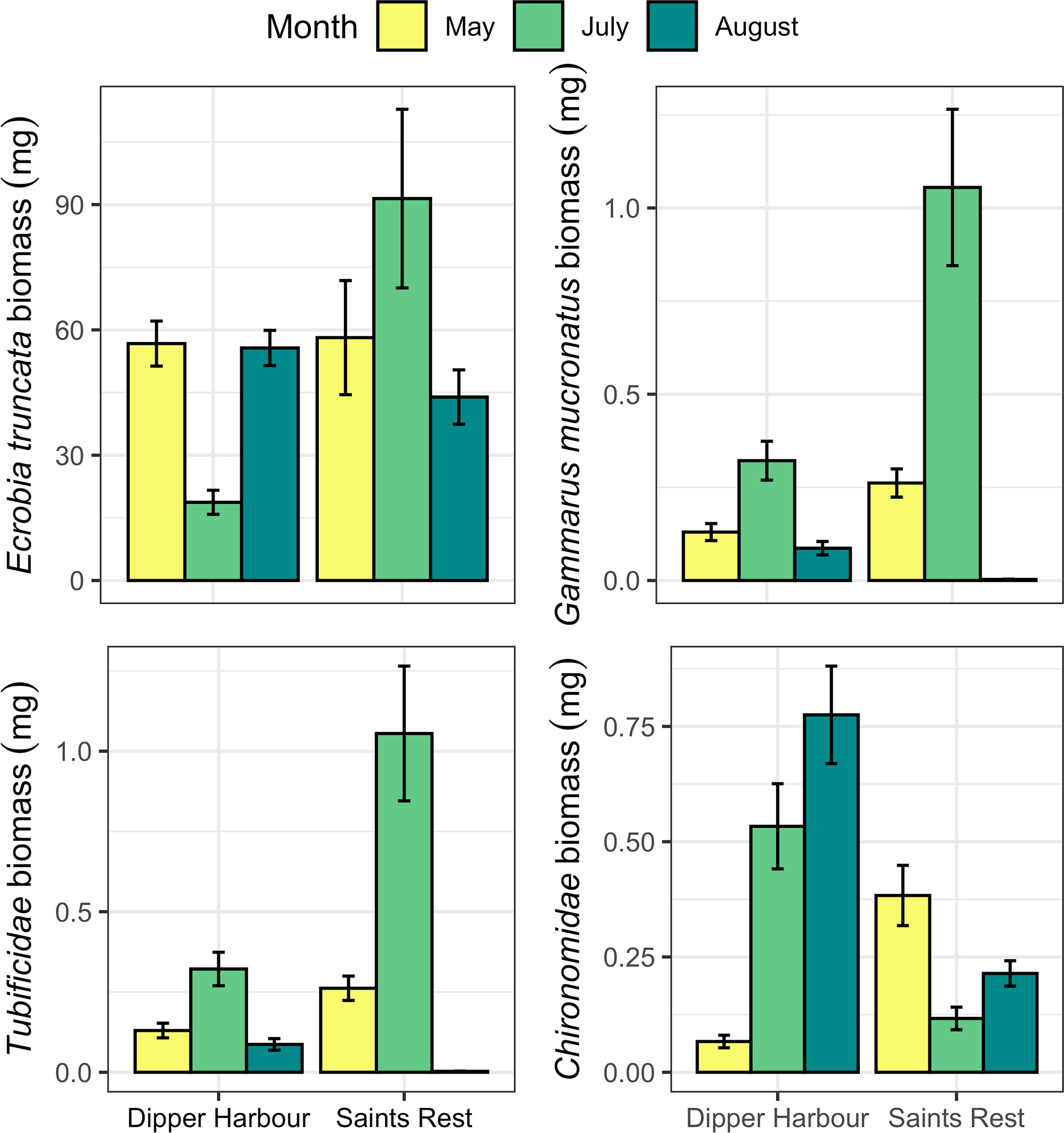
Figure 7 Mean (and se) of dry biomass (mg/300 cm−3-sample) of the four most abundant macroinvertebrates in 300 cm−3-samples of sediments from pools at Dipper Harbour and Saints Rests salt marshes, averaged over pool elevation for July 2004 to August 2005. For July and May, n=6 for DH and SR. For August samples n=14 for SR and n=12 for DH.
The cumulative species richness (the total in all pools) of invertebrates in sediments of Dipper Harbour pools was 17 while that at Saints Rest was 40% higher, i.e., 24 (Table 4). Diversity, as expressed by the Shannon Weiner Index (based upon abundances) was also higher for the total in all pools at Saints Rest, as well as for the mid and low elevation pools there.
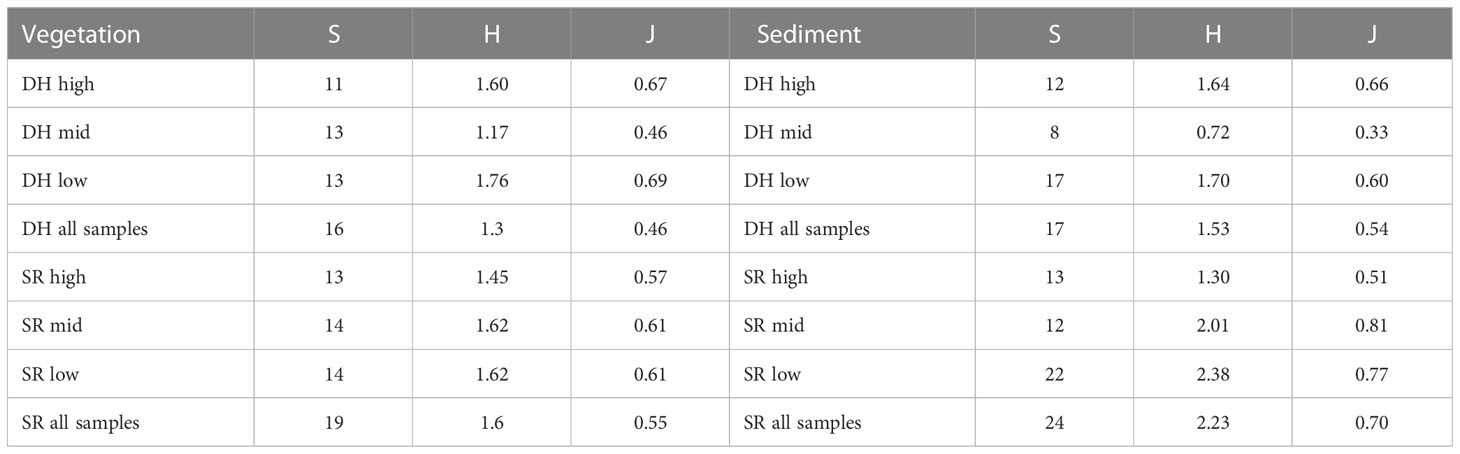
Table 4 Cumulative measures of diversity (e.g., total number of species found in low pools) calculated as species richness (S), Shannon Weiner Index (H) and eveness (J) of invertebrates in pools at high, mid and low elevations at Saints Rest (SR) and Dipper Harbour (DH) marshes from July 2004 to July 2005. H calculations are based upon average abundances.
4 Discussion
4.1 Vegetation
Vegetation in the pools of both salt marshes was highly dominated by the one vascular plant, R. maritima, and Cladophora sp., a filamentous green alga. The higher percent cover of this aquatic vegetation in Dipper Harbour than in Saints Rest marsh overall (Table 1) was likely due to much greater bird use (grazing) of the pools in Saints Rest marsh that we observed. Large flocks of Canada geese and black ducks were frequently observed in the pools at Saints Rest while birds observed at Dipper Harbour were generally in small groups or solitary. Flooding lifts algal mats and allows them to float out of pools, and we assume that the more frequent flooding of low marsh pools was responsible for the low amount of vegetation observed in them. As compared to 2004, the decreased cover of R. maritima in 2005 may be due to the much drier summer weather causing extensive drying of most of the pools.
4.2 Invertebrates
Our analyses showed that pool elevation and month of sampling often explained more of the variability in invertebrate samples than whether they came from the reference marsh at Dipper Harbour or “recovering” Saints Rest marsh. The marsh origin had no significant influence in abundance of invertebrates in communities within pool vegetation. Although it was a significant influence on abundance of invertebrates in pool sediments, marsh origin explained the least amount of the variability (5%). Lower abundances of some species in Saints Rest pool sediments were likely due to more intensive grazing at this site, as noted above.
The observation of more intensive grazing at Saints Rest and our diversity indices suggest that the invertebrate communities in its pools have as much value as those in the reference marsh at Dipper Harbour. Overall diversity, as revealed by the Shannon Weiner Index or Species Richness, is similar or higher in Saints Rest, the recovering marsh. Although it may take greater than 20 years for invertebrate populations to recover (Warren et al., 2002) the status of invertebrate populations in pools at Saints Rest indicated that populations will take less than 50 years for this component of the marsh ecosystem to recover.
4.2.1 Importance of pools to wildlife
Our data and observations indicate that Saints Rest marsh is supporting the critical function played by invertebrate populations, as a food source for higher trophic levels, adding to that provided by mudflats. Over the course of our study, migrating shorebirds were frequently observed feeding in the marsh pools at high tide at Saints Rest marsh. Mudflats in the upper Bay of Fundy are highly valued as a feeding ground for migrating shorebirds. For instance, they are a critical stopover site for Semipalmated Sandpipers (Calidris pusilla) which feed on C. volutator, polychaetes and likely ostracods (MacDonald et al., 2012; Quinn and Hamilton, 2012; Gerwing et al., 2016). As compared to mudflats, some species of shorebirds feed primarily in the marsh on the return migration in spring (Hicklin and Smith, 1979) and our marsh pools provided a number of the same prey taxa as mudflats. In the lower Bay of Fundy Gratto and Thomas (1984) reported prey species of sandpipers included species characteristic of marsh pools such as E. truncata, Chironomid larvae and pupae, all found at Saints Rest. In addition to direct foraging in pools, insects emerging from pools are a food source for passerines, such as the salt marsh sharp-tailed sparrow, Ammodramus caudacutus (Greenlaw and Rising, 1994). Insect remains from at least four different taxa were observed at Saints Rest (Supplementary Table 2).
The importance of marsh pools and other shallow littoral habitats to fish as nursery, foraging and refuge sites is well recognized (e.g., Raposa and Roman, 2001; Adamowicz, 2002; Raposa, 2003; Able et al., 2005). Though not quantified, we observed mummichogs (Fundulus heteroclitus) in all sampled pools in all seasons and frequently in high abundance. Important prey items of mummichogs, such as amphipods, tanids, copepods and polychaetes (Kneib and Stiven, 1978), were collected in all of the pools sampled (Supplementary Table 2).
The abundance of several species of meiofauna observed, particularly in the un-sieved vegetation samples demonstrated that much of the diversity and production of these pools may be missed when meiofauna are not captured (Supplementary Table 2). For instance, marsh pools have been shown to have very diverse copepod communities, relative to macroinvertebrate diversity (Ruber et al., 1994). Some species of meiofauna are important prey items to fish (Kneib and Stiven, 1978; Ward and Fitzgerald, 1983), some species of birds (Gratto and Thomas, 1984; Gaston, 1992), and are important in transferring energy to higher trophic levels as prey for macroinvertebrates.
5 Conclusions
Approximately 50 years after dyke failure and return of tidal flooding, the invertebrate fauna of pools in the originally drained marsh was nearly indistinguishable from that of the reference marsh. We consider that the marsh has “recovered” with respect to pool fauna. The high invertebrate diversity and presumably secondary production of Bay of Fundy salt marsh pools is thus another ecosystem service to be considered when determining the value of restoration of its salt marshes.
When comparing restoration to reference sites to assess the progress of salt marsh restoration it is important to compare pools at similar elevations and time of year as these factors influence the invertebrate fauna found in pools. Including pools over a gradient of elevations will help to reflect the environmental variability that affects invertebrate species composition. In fact, a consideration in planning for salt marsh restoration should be to create pools with varied elevations. Future studies of pool invertebrates also should not be restricted to populations in the vegetation as the sediment samples have greater potential to reveal differences.
Data availability statement
The raw data supporting the conclusions of this article will be made available by the authors, without undue reservation.
Author contributions
PN and GC conceived the study, PN designed and executed the field and laboratory research. PN and BS conducted the analyses. All authors contributed to the article and approved the submitted version.
Funding
Financial support to PN was provided through a joint Natural Sciences and Engineering Research Council – J.D. Irving Limited industrial postgraduate scholarship. A Huntsman Marine Science Centre R.C. Frazee McGill Scholarship to PN funded use of lab facilities at the Atlantic Reference Centre in St. Andrews, New Brunswick. Partial support for field costs was provided by a grant from the New Brunswick Wildlife Council’s Wildlife Trust Fund to the Atlantic Coastal Action Program (ACAP) in Saint John. GC provided support from her Natural Sciences and Engineering Research Council Discovery Grant.
Acknowledgments
We are grateful to staff of the Atlantic Reference Centre for assistance in processing and identification of invertebrates, particularly Dr. Gerhard Pohle and Mary Greenlaw. Alison McAslan (University of New Brunswick Saint John) provided lab space and assistance in algae identifications. Anne Sabourin assisted in the invertebrate processing. Special thanks go to Mr. Kelly Honeyman who helped to make this funding possible. Kelly and the staff at the Irving Nature Park, Joanne Sheils and Samantha Perrin, assisted with field research, Sabrina St. Georges helped to manage spreadsheets and calculations within them, and Tracey Lavigne assisted with figures. We appreciate comments from two reviewers and Myriam Barbeau that helped to improve the manuscript.
Conflict of interest
Author PN was employed by the company J.D. Irving Limited.
The remaining authors declare that the research was conducted in the absence of any commercial or financial relationships that could be construed as a potential conflict of interest.
Publisher’s note
All claims expressed in this article are solely those of the authors and do not necessarily represent those of their affiliated organizations, or those of the publisher, the editors and the reviewers. Any product that may be evaluated in this article, or claim that may be made by its manufacturer, is not guaranteed or endorsed by the publisher.
Supplementary material
The Supplementary Material for this article can be found online at: https://www.frontiersin.org/articles/10.3389/fevo.2023.994533/full#supplementary-material
References
Able K. W., Smith K. J., Hagan S. M. (2005). Fish composition and abundance in New Jersey salt marsh pools: sampling technique effects. NE Natural. 12, 485–502. doi: 10.1656/1092-6194(2005)012[0485:FCAAIN]2.0.CO;2
Adamowicz S. C. (2002). New England salt marsh pools: Analysis of geomorphic and geographic parameters, macrophyte distribution and nekton use (University of Rhode Island, RI, USA: Ph.D. Dissertation).
Allen E. A., Fell P. E., Peck M. A., Gieg J. A., Guthke C. R., Newkirk M. D. (1994). Gut contents of common mummichogs, Fundulus heteroclitus L., in a restored impounded marsh and in natural reference marshes. Estuar 17, 462–471. doi: 10.2307/1352676
Anderson M. J. (2001). A new method for non-parametric multivariate analysis of variance. Austral Ecol. 26, 32–46.
Begon M., Harper J. L., Townsend C. R. (1990). Ecology: Individuals, Populations, and Communities (Cambridge, MA, USA: Blackwell Scientific Publications, Inc).
Bromley J. E. C., Bleakney S. (1979). Taxonomic survey of benthic fauna in estuarine saltmarsh pools, Minas Basin, Bay of Fundy. Proc. N. S. Inst. Sci. 29, 411–446.
Burger J., Shisler J., Lesser F. (1982). Avian utilization on six salt marshes in New Jersey. Biol. Cons. 23, 187–212. doi: 10.1016/0006-3207(82)90075-1
Butzer K. W. (2002). French wetland agriculture in Atlantic Canada and its European roots: different avenues to historical diffusion. Ann. Assoc. Amer. Geog. 92 (3), 451–470. doi: 10.1111/1467-8306.00299
Byers S. E., Chmura G. L. (2007). Salt marsh vegetation recovery on the Bay of Fundy. Estuar. Coasts 30 (5), 869–877.
Campbell B. C., Denno R. F. (1978). The structure of the aquatic insect community associated with intertidal pools in New Jersey. Ecol. Entomol. 3, 181–187. doi: 10.1111/j.1365-2311.1978.tb00917.x
Canadian Hydrographic Survey (2005). “Canadian Tide and Current Tables. Volume 1,” in Atlantic Coast and Bay of Fundy (Sidney, British Columbia: Fisheries and Oceans Canada).
Chmura G. L., Chase P., Bercovitch J. (1997). Climatic controls in the middle marsh zone in the Bay of Fundy. Estuar 20, 689–699. doi: 10.2307/1352244
Chmura G. L., Coffey A., Crago R. (2001). Variation in surface sediment deposition on salt marshes on the Bay of Fundy. J. Coast. Res. 17 (1), 221–227.
Clarke K. R. (1993). Non-parametric multivariate analyses of changes in community structure. Austral. J. Ecol. 18, 117–143. doi: 10.1111/j.1442-9993.1993.tb00438.x
Clarke J. A., Harrington B. A., Hruby T., Wasserman F. E. (1984). The effect of ditching for mosquito control on salt marsh use by birds in Rowley, Massachusetts. J. Field Ornith 55, 160–180.
Erwin R. M. (1996). Dependence of waterbirds and shorebirds on shallow-water habitats in the mid-Atlantic coastal region: an ecological profile and management recommendations. Estuar 19, 213–219. doi: 10.2307/1352226
Gaston G. R. (1992). Green-winged teal ingest epibenthic meiofauna. Estuar 15, 227–229. doi: 10.2307/1352696
Gerwing T. G., Kim J. H., Hamilton D. J., Barbeau M. A., Addison J. A. (2016). Diet reconstruction using next-generation sequencing increases the known ecosystem usage by a shorebird. Auk: Ornithol. Adv. 133 (2), 168–177. doi: 10.1642/AUK-15-176.1
Gordon D. C., Crawford D. J., Desplanque C. (1985). Observations on the ecological importance of salt marshes in the Cumberland Basin, a macrotidal estuary in the Bay of Fundy. Estuarine, Coastal and Shelf Science, 20, 205–227.
Gratto G. W., Thomas M. L. H. (1984). Some aspects of the foraging ecology of migrant juvenile sandpipers in the outer Bay of Fundy. Can. J. Zool. 62, 1889–1892. doi: 10.1139/z84-276
Greenlaw J. S., Rising J. D. (1994). “Sharp-tailed sparrows (Ammodramus caudacutus),” in The Birds of North America, no. 112. Eds. Poole A., Gill F. (Philadelphia, Pennsylvania: The Academy of Natural Sciences).
Hicklin P. W., Smith P. C. (1979). The diets of five species of migrant shorebirds in the Bay of Fundy. Proc. N. S. Inst. Sci. 29, 483–488.
Kneib R. T., Stiven A. E. (1978). Growth, reproduction and feeding of Fundulus heteroclitus (L.) on a North Carolina saltmarsh. J. Exp. Mar. Biol. Ecol. 31, 121–140. doi: 10.1016/0022-0981(78)90125-9
Laymen C. A., Rypel A. L. (2020). Secondary production is an underutilized metric to assess restoration initiatives. Food Webs 25, e00174. doi: 10.1016/j.fooweb.2020.e00174
MacDonald E. C., Ginn M. G., Hamilton D. J. (2012). Variability in foraging behavior and implications for diet breadth among Semipalmated Sandpipers staging in the upper Bay of Fundy. Condor 114, 135–144. doi: 10.1525/cond.2012.100246
MacDonald G. K., Noel P. E., van Proosdij D., Chmura G. L. (2010). The legacy of agricultural reclamation on surface hydrology of two recovering salt marshes of the Bay of Fundy, Canada. Est. Coasts 33, 151–160. doi: 10.1007/s12237-009-9222-4
Nicols E. A. T. (1935). The ecology of a salt marsh. J. Mar. Biol. Assoc. UK 20, 203–261. doi: 10.1017/S0025315400045203
Nixon S. W., Oviatt C. A. (1973). Ecology of a New England salt marsh. Ecol. Monog. 43, 463–498. doi: 10.2307/1942303
Noël P. E., Chmura G. L. (2011). Spatial and environmental variability of pools on a natural and a recovering salt marsh in the Bay of Fundy. J. Coast. Res. 27 (5), 847–856. doi: 10.2112/JCOASTRES-D-10-00084.1
Oksanen J., Kindt R., Legendre P., O'Hara B., Simpson G. L., Solymos P., et al. (2008). Vegan: Community Ecology Package (R package version 1.15-1).
Quinn J. T., Hamilton D. J. (2012). Variation in diet of Semipalmated Sandpipers (Calidris pusilla) during stopover in the upper Bay of Fundy, Canada. Can. J. Zool. 90, 1181–1190. doi: 10.1139/z2012-086
Raposa K. B. (2003). Overwintering habitat selection by the mummichog, Fundulus heteroclitus, in a Cape Cod (USA) salt marsh. Wetl. Ecol. Manage. 11, 175–182. doi: 10.1023/A:1024244317173
Raposa K. B., Roman C. T. (2001). Seasonal habitat use patterns of nekton in a tide restricted and unrestricted New England salt marsh. Wetlands 21, 451–461. doi: 10.1672/0277-5212(2001)021[0451:SHUPON]2.0.CO;2
Roman C. T., Raposa K. B., Adamowicz S. C., James-Pirri M.-J., Catena J. G. (2002). Quantifying vegetation and nekton response to tidal restoration of a New England salt marsh. Rest. Ecol. 10, 450–460. doi: 10.1046/j.1526-100X.2002.01036.x
Ruber E., Gilbert A., Montagna P. A., Gillis G., Cummings E. (1994). Effects of impounding coastal salt marsh for mosquito control on microcrustacean populations. Hydrobiol 292/293, 497–503. doi: 10.1007/BF00229977
Smith K. J., Able K. W. (1994). Salt marsh tide pools as winter refuges for the mummichog, Fundulus heteroclitus, in New Jersey. Est 17, 226–234. doi: 10.2307/1352572
Thomas M. L. H. (1983). “Salt marsh systems,” in Marine and Coastal Systems of the Quoddy Region, New Brunswick, vol. 64 . Ed. Thomas M. L. H. (Can. Spec. Publ. Fisheries and Aquatic Sciences), 107–118, 306 p.
Virgin D. S., Beck A. D., Boone L. K., Dykstra A. K., Ollerhead J., Barbeau M. A., et al. (2020). A managed realignment in the upper Bay of Fundy: Community dynamics during salt marsh restoration over 8 years in a megatidal, ice-influenced environment. Ecol. Eng. 149, 105713. doi: 10.1016/j.ecoleng.2020.105713
Ward G., Fitzgerald G. J. (1983). Fish predation on the macrobenthos of tidal salt marsh pools. Can. J. Zoo. 61, 358–1361. doi: 10.1139/z83-182
Keywords: invertebrate diversity, invertebrate biomass, salt marsh restoration, salt marsh pools, elevation gradient
Citation: Noel PE, Sharma B and Chmura GL (2023) Invertebrate communities of Bay of Fundy salt marsh pools: comparison of a natural and recovering marsh. Front. Ecol. Evol. 11:994533. doi: 10.3389/fevo.2023.994533
Received: 14 July 2022; Accepted: 24 July 2023;
Published: 21 August 2023.
Edited by:
Myriam A. Barbeau, University of New Brunswick Fredericton, CanadaReviewed by:
Spencer Virgin, University of Canterbury, New ZealandJohn Durand, University of California, Davis, United States
Copyright © 2023 Noel, Sharma and Chmura. This is an open-access article distributed under the terms of the Creative Commons Attribution License (CC BY). The use, distribution or reproduction in other forums is permitted, provided the original author(s) and the copyright owner(s) are credited and that the original publication in this journal is cited, in accordance with accepted academic practice. No use, distribution or reproduction is permitted which does not comply with these terms.
*Correspondence: Gail L. Chmura, Z2FpbC5jaG11cmFAbWNnaWxsLmNh
†Present address: Paula E. Noel, Nature Conservancy of Canada, Fredericton, NB, Canada