Different wildfire types promoted two-step terrestrial plant community change across the Triassic-Jurassic transition
- 1Henan International Joint Laboratory of Green Low Carbon Water Treatment Technology and Water Resources Utilization, Henan Key Laboratory of Water Pollution Control and Rehabilitation Technology, School of Municipal and Environmental Engineering, Henan University of Urban Construction, Pingdingshan, Henan, China
- 2State Key Laboratory of Coal Resources and Safe Mining, College of Geoscience and Surveying Engineering, China University of Mining and Technology, Beijing, China
- 3Research Institute of Petroleum Exploration and Development, Petro China, Beijing, China
- 4State Key Laboratory of Hydroscience and Engineering, Department of Hydraulic Engineering, Tsinghua University, Beijing, China
- 5General Prospecting Institute of China National Administration of Coal Geology, Beijing, China
- 6PetroChina Coalbed Methane Company Limited, Beijing, China
- 7School of Geography, Earth and Environmental Sciences, The University of Birmingham, Edgbaston, Birmingham, United Kingdom
- 8Birmingham Institute of Forest Research, The University of Birmingham, Birmingham, United Kingdom
Frequent wildfires associated with emplacement of the Central Atlantic Magmatic Province (CAMP) are thought to have been important drivers of two significant changes in terrestrial plant communities and diversity during the Triassic-Jurassic Mass Extinction (TJME, ca. 201.51 Ma). However, it remains to be investigated whether these two changes are potentially related to different wildfire types. To better understand this relationship, we used a new method to reanalyze fossil pollen and spores across the Triassic-Jurassic transition in the Jiyuan Basin from the North China Plate. Results show that two peaks in wildfire frequency experienced different types of wildfires, with each linked to significant changes in plant communities and diversity losses. In the first wildfire peak, canopy fires dominated and are accompanied by significant losses of canopy forming plants, while in the second wildfire peak, ground cover fires dominated accompanied by significant losses of ground cover plants. Changes in atmospheric humidity conditions were an important control on the two different wildfire peaks. Relatively humid climatic conditions corresponded to the prevalence of canopy fires and hindered the spread and development of ground cover fires in wet surface conditions. Conversely, relatively arid climatic conditions corresponded with the prevalence of ground cover fires in dry surface environments. Our results provide a potential relationship between terrestrial plant communities and wildfire types, which is important to further understanding of terrestrial environmental and floral changes driven by Large Igneous Provinces.
1 Introduction
The Triassic-Jurassic mass extinction (TJME, ca. 201.51Ma) represented one of the most severe biological crises of the Phanerozoic that resulted in the loss of approximately 50% of marine genera and a two-phase decline in plant diversity on land (McElwain et al., 1999; Hallam, 2002; McGhee et al., 2004; Wignall and Bond, 2008; Callegaro et al., 2012; McGhee et al., 2013; Wotzlaw et al., 2014; Wignall and Atkinson, 2020; Lindström, 2021; Zhang et al., 2022). Currently available evidence suggests that the TJME can be attributed to the environmental consequences of pulsed eruptions in the Central Atlantic Magmatic Province (CAMP) (Hesselbo et al., 2002; Ruhl and Kürschner, 2011; Callegaro et al., 2012; Greene et al., 2012; Callegaro et al., 2013; Dal Corso et al., 2014; Percival et al., 2017; Lindström et al., 2019; Panfili et al., 2019; Ruhl et al., 2020; Wignall and Atkinson, 2020; Lindström et al., 2021; Shen et al., 2022; Zhang et al., 2022). It is hypothesized that CAMP volcanism released large amounts of isotopically light CO2 into the atmosphere across Triassic-Jurassic (T-J) transition, through both direct release and indirect action from magmatic intrusion into organic layers, causing globally significant negative carbon isotope excursions (CIEs) in marine and terrestrial strata (e.g., Hesselbo et al., 2002; Dal Corso et al., 2014; Lindström et al., 2019; Ruhl et al., 2020; Wignall and Atkinson, 2020; Lindström et al., 2021; Shen et al., 2022; Zhang et al., 2022), global warming (Shen et al., 2022), ocean anoxia (Jost et al., 2017), and ocean acidification (van de Schootbrugge et al., 2007; Greene et al., 2012), increased chemical weathering (Shen et al., 2022), and frequent wildfires (Song et al., 2020; Zhang et al., 2022).
Frequent wildfires across the T-J transition are thought to be the main cause of changes in terrestrial plant communities and diversity at that time (e.g., Song et al., 2020; Zhang et al., 2022). Studies from the western Tethys (including North America, East Greenland, Poland, Denmark, and Sweden) (Jones et al., 2002; Marynowski and Simoneit, 2009; Belcher et al., 2010; Pole et al., 2018; Lindström et al., 2019; Lindström et al., 2021) and eastern Tethys (including South and North China) (Song et al., 2020; Zhang et al., 2022) have demonstrated that the influence of global warming on wildfires has had a significant impact on altering land vegetation and ecosystems, ultimately resulting in soil erosion after the loss of vegetation (van de Schootbrugge et al., 2020; Lindström et al., 2021). Furthermore, previous investigations on inertinite (charcoal) content and palynology in Sweden and Denmark in the western Tethys have shown that changes in plant communities are closely related to wildfire types, with different types of wildfires including ground and canopy fires causing plant communities changes (Petersen and Lindström, 2012). This relationship may also exist in the eastern Tethys region, but further study is required to evaluate this.
In this study, we investigate the ZJ-1 borehole in the Jiyuan Basin on the southern North China Plate (NCP) in the eastern Tethys. We use a new method to reanalyze previously published palynological data from Zhang et al. (2022), and combine them with published fossil charcoal and geochemical evidence (also from Zhang et al., 2022), to reveal the potential relationship between terrestrial floras and wildfire types through the T-J transition.
2 Geological setting
During the Late Triassic, the NCP was situated around 30–40°N in the eastern Tethys (Greene et al., 2012; Zhang et al., 2022) (Figures 1A, B). Prototype basin reconstructions indicate that in the Late Triassic the Jiyuan Basin was located on the southeast part of the Ordos Basin (Liu et al., 2013) (Figure 1B). Provenance of sediment in the Jiyuan Basin was mainly from the northern Qinling-Dabie Orogenic Belt and the southern NCP located to the south (Yang et al., 2012) (Figures 1A, B).
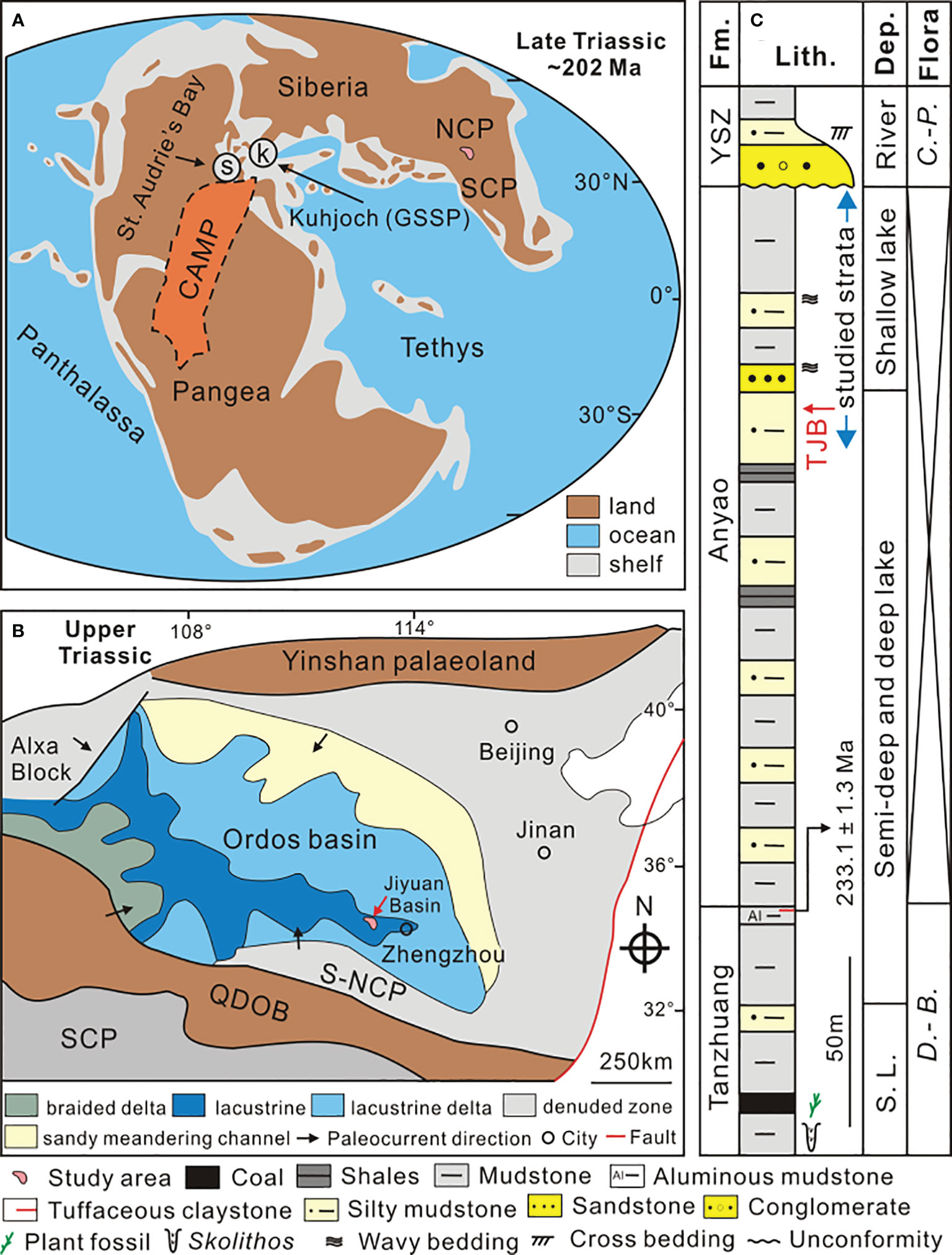
Figure 1 Location and geological context for the study area. (A), Palaeogeographic reconstruction for the Late Triassic (~202 Ma) showing location of the NCP and approximate extent of CAMP (revised after Greene et al., 2012); (B) Simplified tectonic map of the southern NCP during the Late Triassic showing the location of study area (modified from Liu et al., 2013); (C) Stratigraphic framework of the Tanzhuang Formation to the Lower Yangshuzhuang (YSZ) Formation from the Jiyuan Basin (modified from Yang et al., 2012). CAMP, Central Atlantic Magmatic Province; NCP, North China Plate; SCP, South China Plate; QDOB, Qinling-Dabie Orogenic Belt; S-NCP, southern North China Plate; SCP, South China Plate; Fm., Formation; m & s, coal, mudstone and silty mudstone; s., sandstone; c, conglomerate; Dep., Depositional environment; C.-P., Coniopteris-Phoenicopsis.
Figure 1C displays the stratigraphic succession of lithological changes and the position of fossil plants assemblages from the Late Triassic to the Middle Jurassic in the Jiyuan Basin. Previous studies have constrained the geological ages of the Tanzhuang and Anyao formations from the Late Triassic to Early Jurassic based on zircon U-Pb dating, δ13Corg chemostratigraphic patterns, and palynology biostratigraphy (Lu et al., 2021; Zhang et al., 2022) (Figure 1C).
3 Materials and methods
Palynological and charcoal data (Zhang et al., 2022; sampling locations are shown in Figure 2) were re-analyzed using new method (see below) from the Anyao Formation of the ZJ-1 borehole (35.07001°N, 112.47338°E) in the Jiyuan Basin (Figures 1A, B). Variations in spore and pollen compositions through the studied strata were used to reconstruct climatic conditions based on the climatic preferences of the parent plants (e.g., Li et al., 2020; Zhang et al., 2022; Zhang et al., 2023a; Zhang et al., 2023b; Zhang et al., 2023c). To reveal detailed changes in climatic conditions and plant diversity in the study area, we undertook non-metric multidimensional scaling ordination analysis (nMDS) and Simpson (1–D) Diversity Index analysis based on palynological fossils (e.g., Slater et al., 2019; Zhang et al., 2023b). Spore and pollen data were ordinated using nMDS analysis (at two dimensions) with the Bray–Curtis similarity matrix, a useful ordination method for detecting patterns of co-occurrence among taxa as well as ecological gradients (e.g., Zhang et al., 2023b). Simpson (1–D) values were calculated as 1–Σ (pi)2, where p is the proportional abundance of species and i is an unbiased measure of evenness, which ranges from zero (one taxon dominates the community completely) to one (all taxa have equal abundance) (e.g., Slater et al., 2019). According to the preferred ecological habits of plants, we reconstructed the vegetation types reflected by the palynological fossils, and identified ground cover, understory, and upper canopy plants (e.g., Petersen and Lindström, 2012). Charcoal abundance was used as a proxy for wildfire (e.g., Scott, 2000; Glasspool and Scott, 2010; Glasspool et al., 2015; Lu et al., 2020; Zhang et al., 2023a). To reveal burning temperatures and type of wildfires, we measured inertinite (charcoal) reflectance values on polished blocks using a Leica DM4500P LED reflected light microscope with a × 50 oil immersion objective. Reflectance values were identified for over 100 points in each sample.
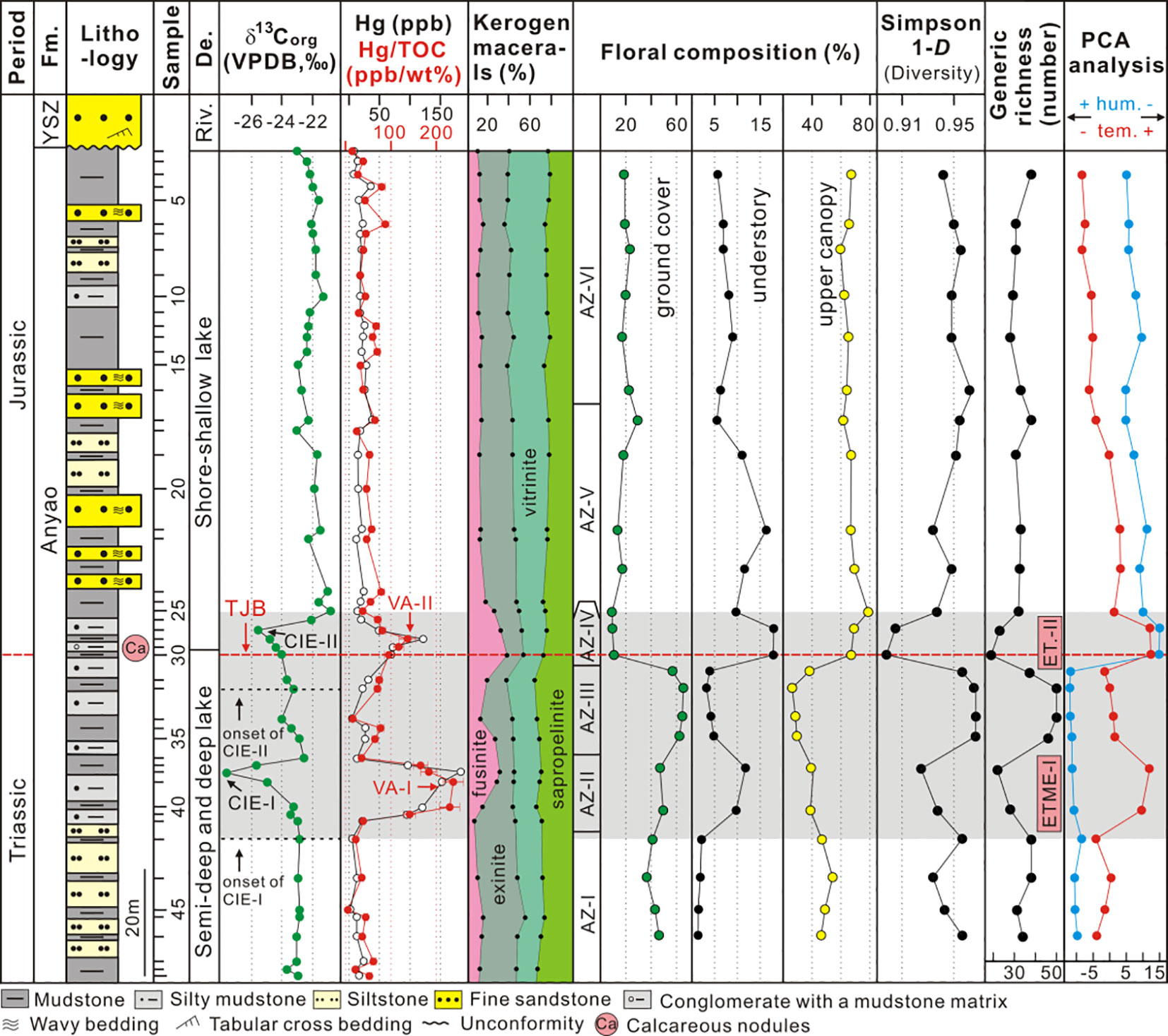
Figure 2 Results of δ13Corg values, Hg concentrations, Hg/TOC ratios, kerogen macerals, floral composition, Simpson (1-D) diversity values, generic richness (number of different genera), and principal components analysis (PCA) from the studied borehole in the Jiyuan Basin. δ13Corg values, Hg concentrations, Hg/TOC ratios, kerogen macerals, generic richness, and PCA data are from Zhang et al. (2022).
4 Results and analysis
4.1 Palynological assemblages and plant diversity
Thirty-four spore, thirty-two pollen, and eight algae genera have been identified in the study area (Zhang et al., 2022) (Supplementary Table 1). According to the palynomorph composition, palynomorph abundance variations, and straigraphically constrained cluster analysis (CONISS) of spore-pollen data, it can be divided into the six palynological assemblage zones (Zhang et al., 2022) (Figure 2). In these palynological assemblage zones, two significant plant diversity declines occurred (Figure 2). In AZ-II, there was a decrease of around 45% in the proportion of spores and pollen, with the number of genera decreasing from 38 to 21 (Zhang et al., 2022) (Figure 2). Similarly, in AZ-IV, there was a decrease of approximately 44%, with a reduction from 50 to 28 genera (Zhang et al., 2022) (Figure 2). Moreover, AZ-IV experienced a significant rise in the abundance of Cythidites spores and Classopollis pollen, and it marked the first appearance of the Cerbropollenites pollen type (Zhang et al., 2022).
4.2 nMDS and Simpson (1-D) values
In this study, re-analyzing the palynological fossils using nMDS show that AZ-II and AZ-IV were intervals with relatively warm climatic conditions (Figure 3). This is consistent with previous results from principal component analysis (PCA) in the Jiyuan Basin (Zhang et al., 2022) (Figure 2). Results from the Simpson (1-D) analysis also identified two obvious declines in plant diversity during AZ-II and AZ-IV (Figure 2). This is consistent with the vegetation reconstruction results of palynological fossils in the studied Jiyuan Basin (Zhang et al., 2022).
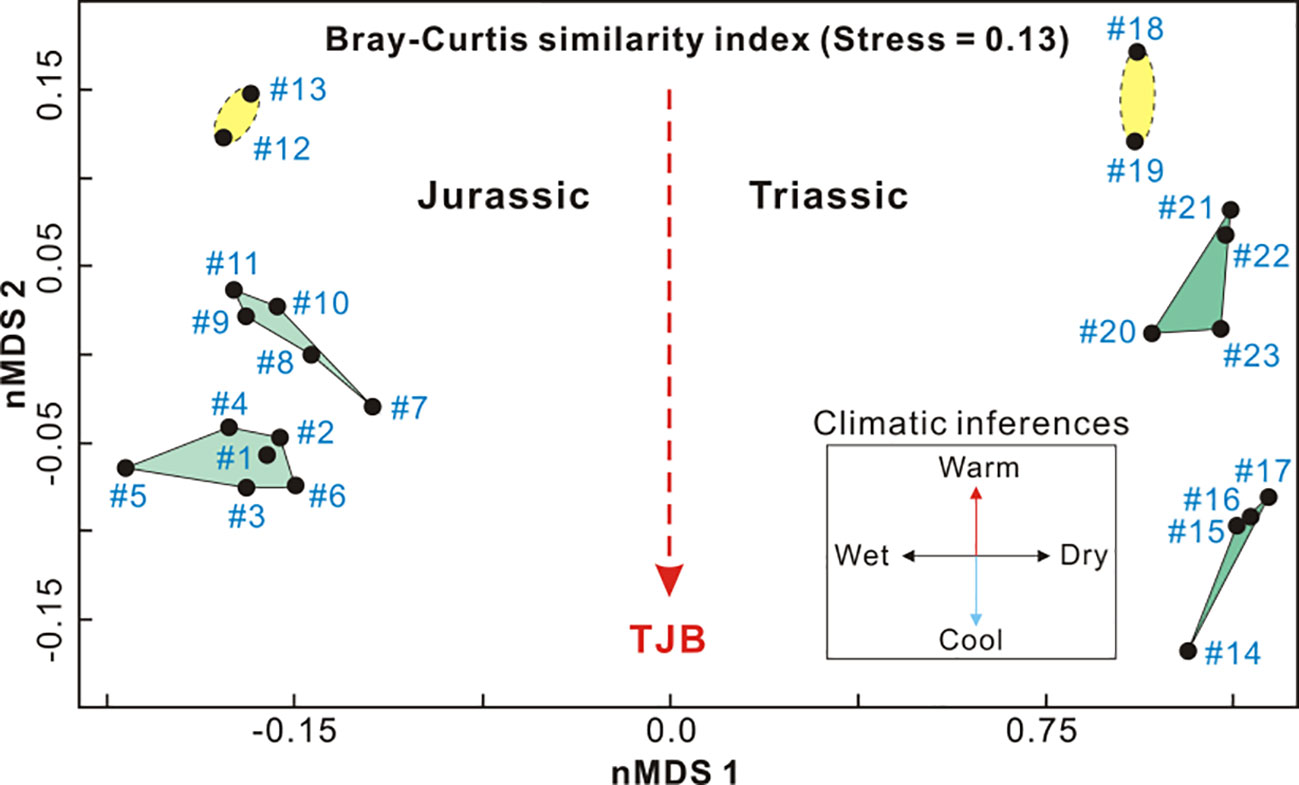
Figure 3 Non-metric multidimensional scaling (nMDS) plot of spore-pollen data from the studied borehole in the Jiyuan Basin. Insert box indicates inferred climatic changes through this interval based on spore-pollen fossils and sedimentary features (Zhang et al., 2022).
4.3 Changes in terrestrial plant community
In this study, the ecological habits of palynological fossil genera were divided according previous classifications (see Petersen and Lindström, 2012) to include ground cover, understory, and upper canopy plants (Supplementary Table 2). Changes in the plant community structure in the study area are significant and can be divided into six stages that correspond to the changes in the palynological assemblages (Figure 2).
In AZ-I, the relative abundance of upper canopy plants (46.26–54.17%) are slightly more dominant than ground cover plants (36.57–46.26%), with understory plants relatively rare (1.46–2.12%) (Figure 2). In AZ-II, the relative abundance of ground cover plants increases and dominates (47.06–49.51%), with upper canopy plants less abundant (38.84–39.21%) but with an increase in understory plants (9.71–11.76%) (Figure 2). In AZ-III, the relative abundance of ground cover plants develops rapidly and become dominant (56.86–65.38%), followed by a slight reduction in upper canopy plants (26.28–38.24%), while understory plants are significantly less abundant and relatively rare (3.21–4.79%) (Figure 2). AZ-IV sees the relative abundance of canopy plants becoming dominant (66.96–68.86%), followed by a rapid increase in understory plants (17.85–17.92%), while ground cover plants show a rapid decrease and are relatively sparce (9.43–10.71%) (Figure 2). In AZ-V and AZ-VI, canopy plants are dominant (61.47–78.71%; 59.48–67.01%), followed by ground cover plants (9.03–29.36%; 17.07–23.28%), with understory plants relatively rare (5.50–16.31%; 5.67–8.94%) (Figure 2).
4.4 Inertinite (charcoal) reflectance
In this study, we tested the inertinite reflectance during two peaks in wildfire frequency across the T-J transition in which there are elevated levels of inertinite (Figure 2); results are presented in Supplementary Table 3. During the first wildfire peak, inertinite reflectance values varied between 1.7 and 4.7%Ro, and most (65%) values were between 3.8 and 4.3%Ro. During the second wildfire peak, inertinite reflectance values varied between 0.7 and 3.1%Ro, and most (62%) were between 1.1 and 1.7%Ro.
5 Discussion
5.1 Changes in terrestrial plant communities and wildfire styles
Recent studies based on chemostratigraphy (δ13Corg patterns) and palynological biostratigraphy have constrained the geological age of the target strata in the studied Jiyuan Basin and indicate that the T-J boundary is located in the upper part of Anyao Formation; this position roughly corresponds to palynological sample #JY-6 in the JZ-1 core (Zhang et al., 2022) and is consistent with our nMDS analysis (Figure 3). In this study, nMDS analysis showed that there were significant differences between Late Triassic and Early Jurassic plant types accompanied by a significant decline in plant diversity inferred from the Simpson (1-D) value (Figure 2). Similar phenomena have been observed in the United Kingdom (Bonis et al., 2010), Germany (van de Schootbrugge et al., 2009; Lindström et al., 2019; Lindström et al., 2021), Denmark (Lindström et al., 2019; Lindström et al., 2021), Austria (Bonis et al., 2009), North America (Olsen et al., 2002), Australia (De Jersey and McKellar, 2013), and New Zealand (De Jersey and McKellar, 2013) and appears to represent a global signal (Wignall and Atkinson, 2020).
Changes in terrestrial plant types and diversity across the T-J transition were greatly influenced by the occurrence of frequent wildfires (Song et al., 2020; Zhang et al., 2022). In the study area, there were two significant peaks in the abundance of fusinite content and charcoal fossils across the T-J transition, indicating that there were two intervals with more frequent wildfires (Zhang et al., 2022). Frequent wildfires seem to be a global phenomenon across the T-J transition, with similar records recorded in North America, East Greenland, Poland, Denmark, Sweden, and South and North China (Jones et al., 2002; Belcher and McElwain, 2008; Marynowski and Simoneit, 2009; Pole et al., 2018; Lindström et al., 2019; Song et al., 2020; Lindström et al., 2021; Zhang et al., 2022). The two wildfire peaks in the study area were accompanied by significant changes in plant types and diversity, suggesting that frequent wildfires are the primary cause of significant changes in terrestrial plant ecosystems (Zhang et al., 2022). In this study, ground cover plants flourished during the first wildfire peak across AZ-II and AZ-III, while the abundance of understory and upper canopy plants decreased (Figure 2). During the second wildfire peak across AZ-III and AZ-IV, upper canopy plants flourished and ground cover plant significantly decreased (Figure 2).
Different types of wildfires may be responsible for changes in different plant types across the T-J transition. Previous studies have shown that ground, surface, and canopy wildfires are characterized by significant differences in burning temperature (Jones, 1997; Scott, 2000). Ground fires have a maximum burning temperature of 400°C during ground litter burns (Scott and Jones, 1994; Scott, 2000), while surface fires burn litter, herbs, and shrubs and attain a maximum temperature of 600°C (Scott and Jones, 1994; Scott, 2000). However, canopy fires burn larger trees and shrubs and can reach higher maximum temperatures of 800°C (Scott and Jones, 1994; Scott, 2000). Extensive experimental data in previous studies have shown that changes in inertinite reflectance values can reflect burning temperatures and type of wildfires (e.g., Jones et al., 1991; Scott, 2000; Scott and Glasspool, 2007; Petersen and Lindström, 2012; Xu et al., 2022). The burning temperature, denoted as T, can be represented by the linear regression equation T = 184.10 + 117.76%Ro (r2 = 0.91) (Jones, 1997), where %Ro corresponds to the measurable inertinite reflectance. In this study, changes in inertinite reflectance values provide evidence for wildfire types across the T-J transition. According to the above equation, burning temperature of the two wildfire peaks in the study area vary from 631.59–690.47°C and 313.64–384.29° C respectively. The first wildfire peak had higher burn temperatures and was likely dominated by canopy type wildfire, while the second wildfire peak had lower temperatures and was dominated by ground cover type wildfire (Figure 2). We consider that in the first wildfire peak canopy fires promoted significant change in plant community composition marked by the disappearance of canopy plants, whereas in the second peak ground cover fires promoted significant changes in plant community composition marked by the disappearance of surface plants. Similar changes in terrestrial wildfire patterns have been observed in Sweden and Denmark (Petersen and Lindström, 2012).
5.2 CAMP as a potential driver of the Triassic-Jurassic transition changes
Recent studies have shown that CAMP emplacement was an important driver of global environment, climate, and floral change across the T-J transition (e.g., Hesselbo et al., 2002; Ruhl and Kürschner, 2011; Greene et al., 2012; Percival et al., 2017; Lindström et al., 2019; Ruhl et al., 2020; Shen et al., 2022; Zhang et al., 2022). CAMP released massive amounts of isotopically light CO2 into the reservoir of the exogenic carbon cycle and drove major global warming (Ruhl and Kürschner, 2011; Ruhl et al., 2020; Zhang et al., 2022). Warming climatic conditions at this time are supported by oxygen isotopes from Lavernock Point (United Kingdom) in the northwest Tethys (Korte et al., 2009), pCO2 increases in pedogenic carbonates from the Newark Basin and the stomatal index (plant fossil cuticles) from Greenland, Sweden, and Northern Ireland (McElwain et al., 1999; Bonis et al., 2010; Schaller et al., 2011; Steinthorsdottir et al., 2011; Schaller et al., 2012; Lindström et al., 2021), and PCA analysis and sedimentological evidence (increased calcareous nodules) in North China (Zhang et al., 2022).
In the context of global warming climatic conditions, in higher temperatures lightning becomes more frequent and wildfires can be more destructive (Glasspool et al., 2015; Miller and Baranyi, 2021). As lightning is the main cause of wildfire, higher temperatures associated with CAMP volcanism would be expected to have increased the frequency and destructiveness of wildfire events (e.g., van de Schootbrugge et al., 2008; Belcher et al., 2010; Glasspool et al., 2015; Lu et al., 2020; Lu et al., 2022; Zhang et al., 2022; Zhang et al., 2023a; Zhang et al., 2023c). Wildfires are influenced by a variety of factors, including the supply of combustible material, oxygen concentration required for combustion, a conducive climate with low moisture, and an ignition mechanism (Scott, 2000; Glasspool et al., 2015; Lu et al., 2020; Zhang et al., 2022). During the T-J transition, the abundant terrestrial vegetation in the Jiyuan Basin provided ample fuel for wildfires (Zhang et al., 2022). Widespread wildfires across the globe provide evidence for the presence of atmospheric oxygen concentrations suitable for wildfires during this time (see Section 5.1). Global warming climatic conditions during the T-J transition also promoted frequent lightning activity (the primary ignition mechanism) (Scott, 2000; Glasspool et al., 2015; Miller et al., 2017; Lu et al., 2020; Zhang et al., 2022; Zhang et al., 2023a). However, the two periods of warming climatic conditions across the T-J transition that saw significant changes in plant communities and diversity in the study area were accompanied by different types of wildfires (see Section 5.1) (Figure 2). Different atmospheric humidity conditions in the study area under global warming may be the main reason for the different types of wildfires.
In the study area, changes in vegetation types, PCA analysis results, and the widespread occurrence of calcareous nodules indicate that there are differences in atmospheric humidity conditions during the two pulses of plant diversity collapse across the T-J transition (Zhang et al., 2022) (Figure 2). During the first pulse of plant diversity collapse and its subsequent recovery phase, relatively humid climatic conditions prevailed in the study area indicated by an increase in hygrophytes (Zhang et al., 2022). Similar phenomena are also seen in the western Tethys (including Hungary, Great Britain, Denmark, Greenland, and Canada) and the southern Pangaea supercontinent (New Zealand and Australia) (van de Schootbrugge et al., 2009; Lindström, 2016). During the second pulse of plant diversity collapse, relatively arid climatic conditions prevailed in the study area inferred from increases in xerophytes and calcareous nodules (Zhang et al., 2022). Similar climatic conditions have been observed in South China (Huang, 2001; Zhou et al., 2021). In this study, during the first decline in plant diversity (TJME-I) that accompanied by the prevalence of canopy fires, relatively humid conditions caused an increase in the humidity of near-surface plants (including herbaceous plants and shrubs) and plant litter (fuel), which was detrimental to wildfires burning near-surface plants and development of ground cover fires (Figure 4). During the second plant diversity decline (TJME-II), the relatively arid conditions during TJME-II also promoted the decrease in humidity of near-surface plants and plant litter, which was conducive to their burning by ground cover fire, leading to the rapid spread and development of ground cover fires (Figure 4). Therefore, we consider that the different atmospheric humidity conditions in the context of global warming are the main cause of the different types of wildfires in the study area across the T-J transition. Furthermore, we also consider that the combined effect of the variable eruption intensity of the bimodal CAMP, and the southward migration of the tropical pressure convergence zone, may have played a role in promoting different wildfires types through driving climate change (Song et al., 2020; Zhang et al., 2022).
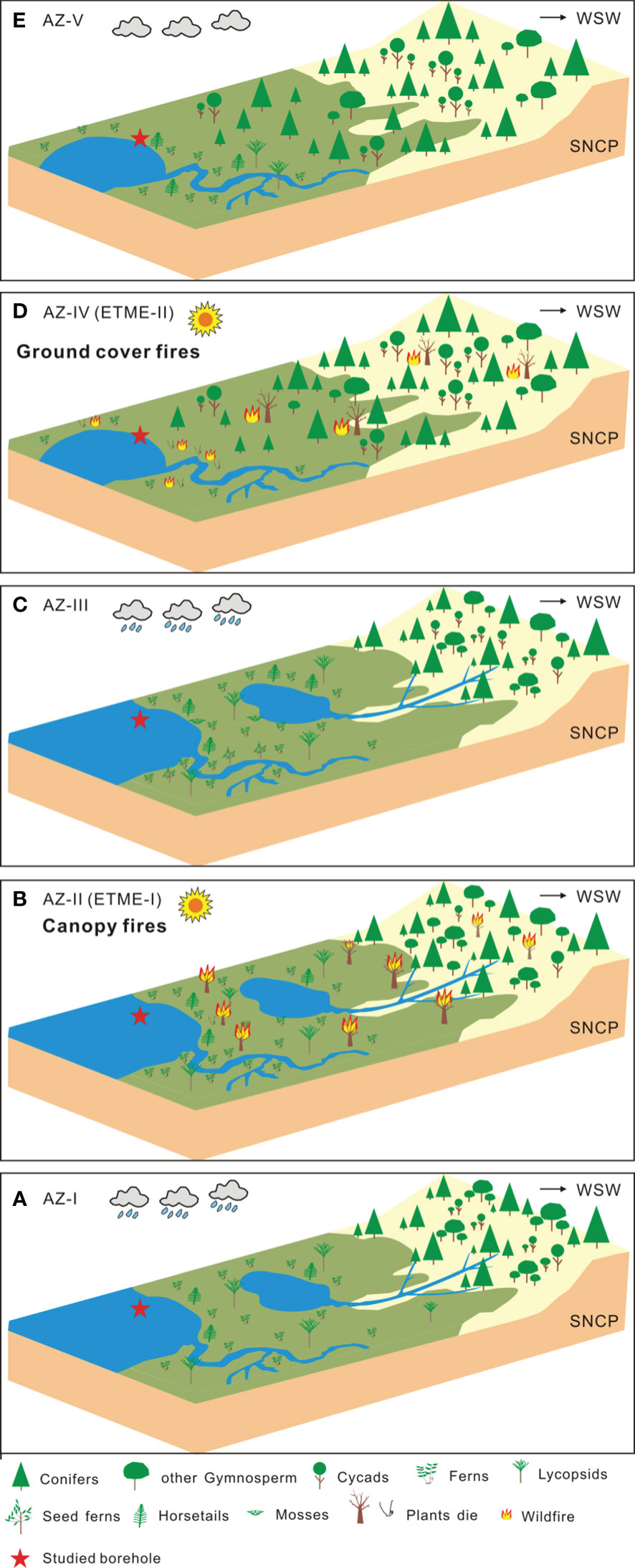
Figure 4 Paleoenvironmental reconstruction of the Jiyuan Basin (A–E) illustrating two distinct wildfire types across the Triassic-Jurassic transition. AZ-I to AZ-V, palynology assemblage zone (AZ) I to the V; ETME-I, first stage of the end Triassic mass extinction; ETME-II, second stage of the end Triassic mass extinction; WSW, west-southwest; SNCP, southern North China Plate.
6 Conclusions
1. Through the Triassic-Jurassic transition in the Jiyuan Basin, two intervals with peaks in wildfire frequency are identified. Inertinite reflectance values, serving as a proxy for burn temperature, records different types of wildfires for the two peaks. In the first peak, wildfires had high burn temperatures indicative of canopy fires and were accompanied by the loss of canopy plants, while in the second peak, wildfires had lower burn temperatures characteristic of ground cover fires and were accompanied by the loss of ground cover plants. The different types of wildfire resulted in significant but different changes in plant community composition, and each included a significant loss in plant diversity. Wildfire was important through the T-J transition in the Jiyuan Basin in shaping plant community composition and was a major factor contribuing to the Triassic-Jurassic boundary terrestrial diversity loss and mass extinction.
2. Changes in atmospheric humidity conditions were a significant driver of the two different wildfire peaks. In the study area, atmospheric humidity was significantly different during the two intervals of plant community change. Relatively humidity climatic conditions corresponded first to the prevelance of the canopy fires with very humid conditions and surface environments hindering the development and spread of ground cover fires. Relatively arid climatic conditions corresponded with the second peak in wildfire with the prevalence of ground cover fires, with arid conditions promoting the development and spread of ground cover fires.
Data availability statement
The original contributions presented in the study are included in the article/Supplementary Material, further inquiries can be directed to the corresponding authors.
Author contributions
PZ: Writing – original draft, Writing – review & editing. MY: Writing – review & editing. JL: Writing – original draft, Writing – review & editing. ZJ: Writing – review & editing. KZ: Writing – review & editing. XX: Writing – review & editing. LeW: Writing – review & editing, Validation. LW: Writing – review & editing. YZ: Writing – review & editing. HC: Writing – review & editing. XZ: Writing – review & editing. YG: Writing – review & editing. HY: Writing – review & editing. LS: Writing – review & editing. JH: Writing – original draft, Writing – review & editing.
Funding
The author(s) declare financial support was received for the research, authorship, and/or publication of this article. Financial support was provided from the Science Fund for Creative Research Groups of the National Natural Science Foundation of China (No. 42321002), the National Natural Science Foundation of China (Grant no. 41772161 and 41472131), the Fund of Henan University of Urban Construction (KQ2023019, KH2023207, and KX2023217), and the National Science and Technology Major Project (Award no. 2017ZX05009-002).
Conflict of interest
MY is employed by PetroChina, XX is employed by China National Administration of Coal Geology, and LW is employed by PetroChina Coalbed Methane Company Limited.
The remaining authors declare that the research was conducted in the absence of any commercial or financial relationships that could be constructed as a potential conflict of interest.
Publisher’s note
All claims expressed in this article are solely those of the authors and do not necessarily represent those of their affiliated organizations, or those of the publisher, the editors and the reviewers. Any product that may be evaluated in this article, or claim that may be made by its manufacturer, is not guaranteed or endorsed by the publisher.
Supplementary material
The Supplementary Material for this article can be found online at: https://www.frontiersin.org/articles/10.3389/fevo.2024.1329533/full#supplementary-material
References
Belcher C. M., Mander L., Rein G., Jervis F. X., Haworth M., Hesselbo S. P., et al. (2010). Increased fire activity at the Triassic/Jurassic boundary in Greenland due to climate-driven floral change. Nat. Geosci. 3, 426–429. doi: 10.1038/ngeo871
Belcher C. M., McElwain J. C. (2008). Limits for combustion in low O2 redefine paleoatmospheric predictions for the Mesozoic. Science 321, 1197–1200. doi: 10.1126/science.1160978
Bonis N. R., Kürschner W. M., Krystyn L. (2009). A detailed palynological study of the Triassic-Jurassic transition in key sections of the Eiberg Basin (Northern Calcareous Alps, Austria). Rev. Palaeobot. Palynol. 156, 376–400. doi: 10.1016/j.revpalbo.2009.04.003
Bonis N. R., Ruhl M., Kürschner W. M. (2010). Milankovitch-scale palynological turnover across the Triassic-Jurassic transition at St. Audrie’s Bay, SW UK. J. Geol. Soc London. 167, 877–888. doi: 10.1144/0016-76492009-141
Callegaro S., Marzoli A., Bertrand H., Chiaradia M., Reisberg L., Meyzen C., et al. (2013). Upper and lower crust recycling in the source of CAMP basaltic dykes from southeastern North America. Earth Planet. Sci. Lett. 376, 186–199. doi: 10.1016/j.epsl.2013.06.023
Callegaro S., Rigo M., Chiaradia M., Marzoli A. (2012). Latest Triassic marine Sr isotopic variations, possible causes and implications. Terra Nov. 24, 130–135. doi: 10.1111/j.1365-3121.2011.01046.x
Dal Corso J., Marzoli A., Tateo F., Jenkyns H. C., Bertrand H., Youbi N., et al. (2014). The dawn of CAMP volcanism and its bearing on the end-Triassic carbon cycle disruption. J. Geol. Soc London. 171, 153–164. doi: 10.1144/jgs2013-063
De Jersey N. J., McKellar J. L. (2013). The palynology of the Triassic-Jurassic transition in southeastern Queensland, Australia, and correlation with New Zealand. Palynology 37, 77–114. doi: 10.1080/01916122.2012.718609
Glasspool I. J., Scott A. C. (2010). Phanerozoic concentrations of atmospheric oxygen reconstructed from sedimentary charcoal. Nat. Geosci. 3, 627–630. doi: 10.1038/ngeo923
Glasspool I. J., Scott A. C., Waltham D., Pronina N., Shao L. (2015). The impact of fire on the late Paleozoic Earth System. Front. Plant Sci. 6. doi: 10.3389/fpls.2015.00756
Greene S. E., Martindale R. C., Ritterbush K. A., Bottjer D. J., Corsetti F. A., Berelson W. M. (2012). Recognising ocean acidification in deep time: An evaluation of the evidence for acidification across the Triassic-Jurassic boundary. Earth-Science Rev. 113, 72–93. doi: 10.1016/j.earscirev.2012.03.009
Hallam A. (2002). How catastrophic was the end-Triassic mass extinction? Lethaia 35, 147–157. doi: 10.1080/002411602320184006
Hesselbo S. P., Robinson S. A., Surlyk F., Piasecki S. (2002). Terrestrial and marine extinction at the Triassic-Jurassic boundary synchronized with major carbon-cycle perturbation: A link to initiation of massive volcanism? Geology 30, 251–254. doi: 10.1130/0091-7613(2002)030<0251:TAMEAT>2.0.CO;2
Huang Q. (2001). Early Jurassic flora and paleoenvironment in Daxian and Kaixian counties, northern border of Sichuan basin. Earth Sci. China Univ. Geosci. 26, 221–227.
Jones T. P. (1997). Fusain in late Jurassic sediments from Witch Ground Graben, North Sea, U.K. Mededelingen Nederlands Instituut voor Toegepaste Geowetenschappen TNO 58, 93–103.
Jones T. P., Ash S., Figueiral I. (2002). Late triassic charcoal from petrified forest national park, arizona, USA. Palaeogeogr. Palaeoclimatol. Palaeoecol. 188, 127–139. doi: 10.1016/S0031-0182(02)00549-7
Jones T. P., Scott A. C., Cope M. (1991). Reflectance measurements and the temperature of formation of modern charcoals and implications for studies of fusain. Bull. Soc Geol. Fr. 162, 193–200.
Jost A. B., Bachan A., van de Schootbrugge B., Lau K. V., Weaver K. L., Maher K., et al. (2017). Uranium isotope evidence for an expansion of marine anoxia during the end-Triassic extinction. Geochemistry Geophys. Geosystems 18, 3093–3108. doi: 10.1002/2017GC006941
Korte C., Hesselbo S. P., Jenkyns H. C., Rickaby R. E. M., Spötl C. (2009). Palaeoenvironmental significance of carbon- and oxygen-isotope stratigraphy of marine Triassic-Jurassic boundary sections in SW Britain. J. Geol. Soc London. 166, 431–445. doi: 10.1144/0016-76492007-177
Li L., Wang Y., Kürschner W. M., Ruhl M., Vajda V. (2020). Palaeovegetation and palaeoclimate changes across the Triassic – Jurassic transition in the Sichuan Basin, China. Palaeogeogr. Palaeoclimatol. Palaeoecol. 556, 109891. doi: 10.1016/j.palaeo.2020.109891
Lindström S. (2016). Palynofloral patterns of terrestrial ecosystem change during the end-Triassic event – a review. Geol. Mag. 153, 223–251. doi: 10.1017/S0016756815000552
Lindström S. (2021). Two-phased mass rarity and extinction in land plants during the end-Triassic climate crisis. Front. Earth Sci. 9. doi: 10.3389/feart.2021.780343
Lindström S., Callegaro S., Davies J., Tegner C., van de Schootbrugge B., Pedersen G. K., et al. (2021). Tracing volcanic emissions from the Central Atlantic Magmatic Province in the sedimentary record. Earth-Science Rev. 212, 103444. doi: 10.1016/j.earscirev.2020.103444
Lindström S., Sanei H., van de Schootbrugge B., Pedersen G. K., Lesher C. E., Tegner C., et al. (2019). Volcanic mercury and mutagenesis in land plants during the end-Triassic mass extinction. Sci. Adv. 5, eaaw4018. doi: 10.1126/sciadv.aaw4018
Liu S., Su S., Zhang G. (2013). Early Mesozoic basin development in North China: Indications of cratonic deformation. J. Asian Earth Sci. 62, 221–236. doi: 10.1016/j.jseaes.2012.09.011
Lu J., Wang Y., Yang M., Zhang P., Bond D. P. G., Shao L., et al. (2022). Diachronous end-Permian terrestrial ecosystem collapse with its origin in wildfires. Palaeogeogr. Palaeoclimatol. Palaeoecol. 594, 110960. doi: 10.1016/j.palaeo.2022.110960
Lu J., Zhang P., Dal Corso J., Yang M., Wignall P. B., Greene S. E., et al. (2021). Volcanically driven lacustrine ecosystem changes during the Carnian Pluvial Episode (Late Triassic). Proc. Natl. Acad. Sci. U. S. A. 118, e2109895118. doi: 10.1073/pnas.2109895118
Lu J., Zhang P., Yang M., Shao L., Hilton J. (2020). Continental records of organic carbon isotopic composition (δ13Corg), weathering, paleoclimate and wildfire linked to the End-Permian Mass Extinction. Chem. Geol. 558, 119764. doi: 10.1016/j.chemgeo.2020.119764
Marynowski L., Simoneit B. R. T. (2009). Widespread upper Triassic to lower Jurassic wildfire records from Poland: Evidence from charcoal and pyrolytic polycyclic aromatic hydrocarbons. Palaios 24, 785–798. doi: 10.2110/palo.2009.p09-044r
McElwain J. C., Beerling D. J., Woodward F. I. (1999). Fossil plants and global warming at the Triassic-Jurassic boundary. Science 285, 1386–1390. doi: 10.1126/science.285.5432.1386
McGhee G. R., Clapham M. E., Sheehan P. M., Bottjer D. J., Droser M. L. (2013). A new ecological-severity ranking of major Phanerozoic biodiversity crises. Palaeogeogr. Palaeoclimatol. Palaeoecol. 370, 260–270. doi: 10.1016/j.palaeo.2012.12.019
McGhee G. R., Sheehan P. M., Bottjer D. J., Droser M. L. (2004). Ecological ranking of Phanerozoic biodiversity crises: Ecological and taxonomic severities are decoupled. Palaeogeogr. Palaeoclimatol. Palaeoecol. 211, 289–297. doi: 10.1016/j.palaeo.2004.05.010
Miller C. S., Baranyi V. (2021). “Triassic climates,” in Encyclopedia of geology. Eds. Alderton D., Elias S. A. B. T. (Oxford: Elsevier), 514–524. doi: 10.1016/B978-0-12-409548-9.12070-6
Miller C. S., Peterse F., da Silva A. C., Baranyi V., Reichart G. J., Kürschner W. M. (2017). Astronomical age constraints and extinction mechanisms of the Late Triassic Carnian crisis. Sci. Rep. 7, 2557. doi: 10.1038/s41598-017-02817-7
Olsen P. E., Kent D. V., Sues H. D., Koeberl C., Huber H., Montanari A., et al. (2002). Ascent of dinosaurs linked to an iridium anomaly at the Triassic-Jurassic boundary. Science 296, 1305–1307. doi: 10.1126/science.1065522
Panfili G., Cirilli S., Corso J. D., Bertrand H., Medina F., Youbi N., et al. (2019). New biostratigraphic constraints show rapid emplacement of the Central Atlantic Magmatic Province (CAMP) during the end-Triassic mass extinction interval. Glob. Planet. Change 172, 60–68. doi: 10.1016/j.gloplacha.2018.09.009
Percival L. M. E., Ruhl M., Hesselbo S. P., Jenkyns H. C., Mather T. A., Whiteside J. H. (2017). Mercury evidence for pulsed volcanism during the end-Triassic mass extinction. Proc. Natl. Acad. Sci. U. S. A. 114, 7929–7934. doi: 10.1073/pnas.1705378114
Petersen H. I., Lindström S. (2012). Synchronous wildfire activity rise and mire deforestation at the Triassic–Jurassic Boundary. PloS One 7, e47236. doi: 10.1371/journal.pone.0047236
Pole M., Wang Y., Dong C., Xie X., Tian N., Li L., et al. (2018). Fires and storms—a Triassic–Jurassic transition section in the Sichuan Basin, China. Palaeobiodiversity Palaeoenvironments 98, 29–47. doi: 10.1007/s12549-017-0315-y
Ruhl M., Hesselbo S. P., Al-Suwaidi A., Jenkyns H. C., Damborenea S. E., Manceñido M. O., et al. (2020). On the onset of Central Atlantic Magmatic Province (CAMP) volcanism and environmental and carbon-cycle change at the Triassic–Jurassic transition (Neuquén Basin, Argentina). Earth-Science Rev. 208, 103229. doi: 10.1016/j.earscirev.2020.103229
Ruhl M., Kürschner W. M. (2011). Multiple phases of carbon cycle disturbance from large igneous province formation at the Triassic-Jurassic transition. Geology 39, 431–434. doi: 10.1130/G31680.1
Schaller M. F., Wright J. D., Kent D. V. (2011). Atmospheric PCO2 perturbations associated with the central atlantic magmatic province. Science 331, 1404–1409. doi: 10.1126/science.1199011
Schaller M. F., Wright J. D., Kent D. V., Olsen P. E. (2012). Rapid emplacement of the Central Atlantic Magmatic Province as a net sink for CO2. Earth Planet. Sci. Lett. 323–324, 27–39. doi: 10.1016/j.epsl.2011.12.028
Scott A. C. (2000). The Pre-Quaternary history of fire. Palaeogeogr. Palaeoclimatol. Palaeoecol. 164, 281–329. doi: 10.1016/S0031-0182(00)00192-9
Scott A. C., Glasspool I. J. (2007). Observations and experiments on the origin and formation of inertinite group macerals. Int. J. Coal Geol. 70, 53–66. doi: 10.1016/j.coal.2006.02.009
Scott A. C., Jones T. P. (1994). The nature and influence of fire in Carboniferous ecosystems. Palaeogeogr. Palaeoclimatol. Palaeoecol. 106, 91–112. doi: 10.1016/0031-0182(94)90005-1
Shen J., Yin R., Zhang S., Algeo T. J., Bottjer D. J., Yu J., et al. (2022). Intensified continental chemical weathering and carbon-cycle perturbations linked to volcanism during the Triassic–Jurassic transition. Nat. Commun. 13, 299. doi: 10.1038/s41467-022-27965-x
Slater S. M., Twitchett R. J., Danise S., Vajda V. (2019). Substantial vegetation response to Early Jurassic global warming with impacts on oceanic anoxia. Nat. Geosci. 12, 462–467. doi: 10.1038/s41561-019-0349-z
Song Y., Algeo T. J., Wu W., Luo G., Li L., Wang Y., et al. (2020). Distribution of pyrolytic PAHs across the Triassic-Jurassic boundary in the Sichuan Basin, southwestern China: Evidence of wildfire outside the Central Atlantic Magmatic Province. Earth-Science Rev. 201, 102970. doi: 10.1016/j.earscirev.2019.102970
Steinthorsdottir M., Jeram A. J., McElwain J. C. (2011). Extremely elevated CO2 concentrations at the Triassic/Jurassic boundary. Palaeogeogr. Palaeoclimatol. Palaeoecol. 308, 418–432. doi: 10.1016/j.palaeo.2011.05.050
van de Schootbrugge B., Payne J. L., Tomasovych A., Pross J., Fiebig J., Benbrahim M., et al. (2008). Carbon cycle perturbation and stabilization in the wake of the Triassic-Jurassic boundary mass-extinction event. Geochemistry Geophys. Geosystems 9, Q04028. doi: 10.1029/2007GC001914
van de Schootbrugge B., Quan T. M., Lindström S., Püttmann W., Heunisch C., Pross J., et al. (2009). Floral changes across the Triassic/Jurassic boundary linked to flood basalt volcanism. Nat. Geosci. 2, 589–594. doi: 10.1038/ngeo577
van de Schootbrugge B., Tremolada F., Rosenthal Y., Bailey T. R., Feist-Burkhardt S., Brinkhuis H., et al. (2007). End-Triassic calcification crisis and blooms of organic-walled ‘disaster species.’ Palaeogeogr. Palaeoclimatol. Palaeoecol. 244, 126–141. doi: 10.1016/j.palaeo.2006.06.026
van de Schootbrugge B., van der Weijst C. M. H., Hollaar T. P., Vecoli M., Strother P. K., Kuhlmann N., et al. (2020). Catastrophic soil loss associated with end-Triassic deforestation. Earth-Science Rev. 210, 103332. doi: 10.1016/j.earscirev.2020.103332
Wignall P. B., Atkinson J. W. (2020). A two-phase end-Triassic mass extinction. Earth-Science Rev. 208, 103282. doi: 10.1016/j.earscirev.2020.103282
Wignall P. B., Bond D. P. G. (2008). The end-Triassic and Early Jurassic mass extinction records in the British Isles. Proc. Geol. Assoc. 119, 73–84. doi: 10.1016/S0016-7878(08)80259-3
Wotzlaw J. F., Guex J., Bartolini A., Gallet Y., Krystyn L., McRoberts C. A., et al. (2014). Towards accurate numerical calibration of the Late Triassic: High-precision U-Pb geochronology constraints on the duration of the Rhaetian. Geology 42, 571–574. doi: 10.1130/G35612.1
Xu X., Shao L., Eriksson K. A., Zhou J., Wang D., Hou H., et al. (2022). Widespread wildfires linked to early Albian Ocean Anoxic Event 1b: Evidence from the Fuxin lacustrine basin, NE China. Glob. Planet. Change 215, 103858. doi: 10.1016/j.gloplacha.2022.103858
Yang W., Yang J., Wang X., Du Y. (2012). Geochronology from Middle Triassic to Middle Jurassic detrital zircons in Jiyuan basin and its implications for the Qinling Orogen. Earth Sci. China Univ. Geosci. 37, 489–500. doi: 10.3799/dqkx.2012.055
Zhang P., Lu J., Yang M., Bond D. P. G., Greene S. E., Liu L., et al. (2022). Volcanically-induced environmental and floral changes across the Triassic-Jurassic (T-J) transition. Front. Ecol. Evol. 10. doi: 10.3389/fevo.2022.853404
Zhang P., Yang M., Jiang Z., Zhou K., Xu X., Chen H., et al. (2023c). Significant floral changes across the Permian-Triassic and Triassic-Jurassic transitions induced by widespread wildfires. Front. Ecol. Evol. 11. doi: 10.3389/fevo.2023.1284482
Zhang P., Yang M., Lu J., Bond D. P. G., Zhou K., Xu X., et al. (2023a). End-Permian terrestrial ecosystem collapse in North China: Evidence from palynology and geochemistry. Glob. Planet. Change 222, 104070. doi: 10.1016/j.gloplacha.2023.104070
Zhang P., Yang M., Lu J., Jiang Z., Zhou K., Liu H., et al. (2023b). Middle Jurassic terrestrial environmental and floral changes linked to volcanism: Evidence from the Qinghai-Tibet Plateau, China. Glob. Planet. Change 223, 104094. doi: 10.1016/j.gloplacha.2023.104094
Keywords: central Atlantic magmatic province, Triassic-Jurassic transition, terrestrial plant community, wildfire types, charcoal
Citation: Zhang P, Yang M, Lu J, Jiang Z, Zhou K, Xu X, Wang L, Wu L, Zhang Y, Chen H, Zhu X, Guo Y, Ye H, Shao L and Hilton J (2024) Different wildfire types promoted two-step terrestrial plant community change across the Triassic-Jurassic transition. Front. Ecol. Evol. 12:1329533. doi: 10.3389/fevo.2024.1329533
Received: 29 October 2023; Accepted: 10 January 2024;
Published: 26 January 2024.
Edited by:
Dangpeng Xi, China University of Geosciences, ChinaReviewed by:
Dieter Uhl, Senckenberg Research Institute and Natural History Museum Frankfurt, GermanyCopyright © 2024 Zhang, Yang, Lu, Jiang, Zhou, Xu, Wang, Wu, Zhang, Chen, Zhu, Guo, Ye, Shao and Hilton. This is an open-access article distributed under the terms of the Creative Commons Attribution License (CC BY). The use, distribution or reproduction in other forums is permitted, provided the original author(s) and the copyright owner(s) are credited and that the original publication in this journal is cited, in accordance with accepted academic practice. No use, distribution or reproduction is permitted which does not comply with these terms.
*Correspondence: Jing Lu, lujing@cumtb.edu.cn; Zhongfeng Jiang, jiangzf@huuc.edu.cn