- 1Institute of Global Innovation Research, Tokyo University of Agriculture and Technology, Fuchu, Tokyo, Japan
- 2Illinois Natural History Survey, Prairie Research Institute, University of Illinois, Champaign, IL, United States
Vertebrate scavenging contributes to key ecosystem functions through the cycling of nutrients from carrion. However, the dynamics of scavenging are complex with many unknowns, necessitating the use of multiple metrics to measure the dynamics within vertebrate scavenger assemblages and the impact of scavenging on ecological systems. We propose a conceptual model representing a series of the scavenging processes that are critical to ecosystem functioning. The model includes 13 key metrics based on 4 primary questions to consider in studies of vertebrate scavenging to explain how scavenging dynamics are related to ecosystem functions. Our model enhances understanding of how scavenging dynamics impact ecosystem functions at different spatial and temporal scales and offers a framework for future studies to refine our understanding of scavenging across different ecosystems.
1 Introduction
Consumers of dead animal tissues (i.e., carrion) can be classified into two main groups: scavengers (which consist of vertebrates and invertebrates) and decomposers (which consist of microorganisms; Olea et al., 2019). Vertebrate scavengers often act as the primary consumers of carrion, and by consuming the carcasses they keep the energy from carrion flowing at higher levels in food webs (DeVault et al., 2003). This scavenging process contributes to ecosystem maintenance through various ecosystem functions [e.g., food web dynamics (Steinbeiser et al., 2018; Wilson and Wolkovich, 2011), nutrient cycling (DeVault et al., 2003; Wenting et al., 2024), nutrient movement (Payne and Moore, 2006; Subalusky et al., 2017; Quaggiotto et al., 2018), disease control (Vicente and VerCauteren, 2019; Moleón and Sánchez-Zapata, 2021)]. Therefore, understanding vertebrate scavenging and the factors that drive its various dynamics are important to clarify ecological maintenance mechanisms involved in the carrion decomposition. Here, we define scavenging dynamics as the carrion–scavenger interactions that directly and indirectly provide ecosystem functions through carrion consumption, and the mechanisms that maintain these interactions.
The scavenging process is complex, and there are multiple metrics that can be used to measure scavenging dynamics within vertebrate scavenger assemblages and the impact of scavenging dynamics on ecological systems. Nevertheless, there is little consensus to date on the best variables and metrics to explain scavenger dynamics. This knowledge gap is an important topic of discussion, as the specific metrics and variables chosen can affect the interpretation of data and conclusions, as well as comparisons across different geographical areas. To begin the discussion of filling this knowledge gap, we outline 4 primary questions (Figure 1) and 13 key metrics (Table 1) to consider in studies of vertebrate scavenging. By tracking these metrics across different systems, researchers can gain a comprehensive understanding of vertebrate scavenger communities and their ecological roles. This is especially important for vertebrate scavenger communities, which are increasingly threatened by anthropogenic activity and development around the world (Newsome et al., 2024; Sebastián-González et al., 2019, Sebastián-González et al., 2020). We briefly explain what can be derived from these questions and metrics, along with their challenges, and provide our perspectives on future prospects based on the conceptual model we propose.
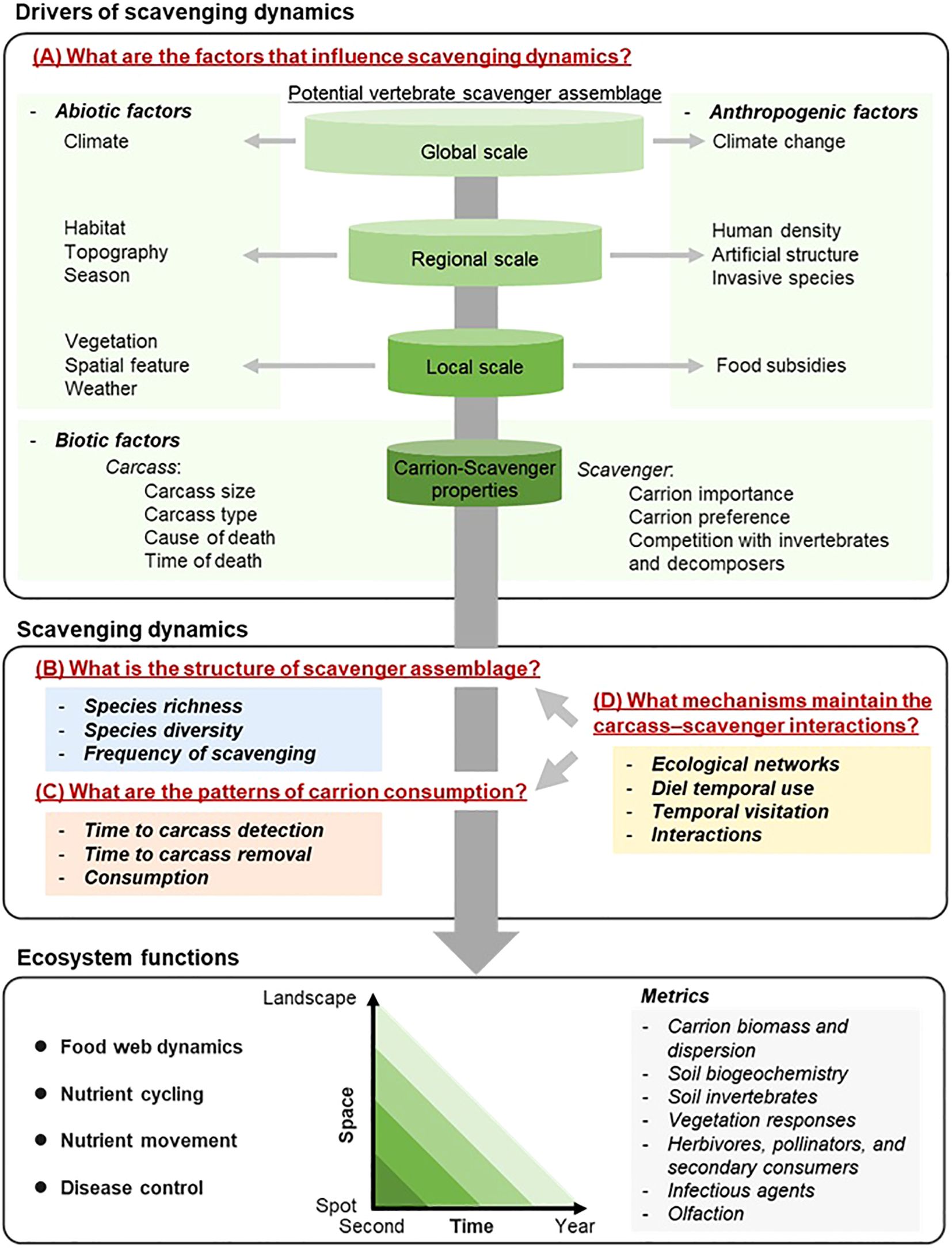
Figure 1. The conceptual model represents a series of scavenging dynamics and ecosystem functioning. The model explains 13 metrics (bold italics; Table 1) by four primary questions (red text; groups in Table 1) to consider in scavenging research. The observed scavenging dynamics will be filtered out from the potential scavenger assemblage. These filters encompass three factors (abiotic factors, biotic factors, anthropogenic factors) operating across varying spatial and temporal scales (global scale, regional scale, local scale, carrion–scavenger properties properties). The observed scavenging dynamics provide four ecosystem functions (food web dynamics, nutrient cycling, nutrient movement, and disease control). The metrics of the four ecosystem functions (gray box) integrate the ecological indicators proposed by Newsome et al. (2021). Each ecosystem function operates at different spatial and temporal scales because scavenging dynamics span across different spatial and temporal scales.
2 How do we understand scavenging dynamics?
The form of scavenging dynamics can be evaluated from three perspectives: (1) the scavengers—“what is the structure of scavenger assemblage?”; (2) the carcasses—“what are the patterns of carrion consumption?”; and (3) the mechanisms—“what mechanisms maintain the carcass–scavenger interactions?” Clarifying these three perspectives forms the foundation for comparing scavenger communities across ecosystems and lead to the development of applied research including meta-analyses (Sebastián-González et al., 2019, Sebastián-González et al., 2020; Montenegro et al., 2025).
Identifying scavenger assemblages promotes understanding of the ecological community and the diversity of scavengers. This provides foundational information for the conservation of scavenger species (including functional traits or species vulnerability), and metrics of richness and diversity are useful at local and global scales. The development of camera trap technology has allowed the identification of vertebrate scavenger species and assemblages to progress rapidly (Newsome et al., 2021). However, there is still a geographical bias in the regions studied, and there is a need to accumulate more knowledge in South America, Africa, and Asia. In addition, small animals such as rodents and ectotherms such as reptiles are often missed by camera traps and are thus often not recognized as scavengers (e.g., Gerke et al., 2022; Smith et al., 2017). In terrestrial ecosystems, knowledge of underground and arboreal spaces in three-dimensional environments is also limited compared to ground-level spaces (Smith et al., 2017). Moreover, the knowledge of marine and freshwater ecosystems is also limited due to the lack of development of low-cost observation techniques. Therefore, further research on these species, spaces, and ecosystems is needed.
The perspective of the carrion is directly linked to the evaluation of the ecosystem functions provided by scavenging. This is because the dynamics for the time of detection and amount of carcasses consumed are closely linked to the activities of invertebrates and decomposers, thereby determining the nutrients derived from carrion. The evaluation of which scavengers contribute to the detection and consumption of carrion (i.e., in combination with perspective of scavengers) could be an effective tool for identifying species that play an important role in the formation and function of scavenger assemblages. For example, anadromous salmon migrate from the ocean to rivers during spawning and die after spawning, and the majority of the salmon carcasses are consumed by brown bears (Ursus arctos), transporting marine-derived nutrients inland (Helfield and Naiman, 2006; Levi et al., 2015). Here, identifying the brown bear as the primary scavenger and quantifying how much salmon bears consume allows for the clarification of the bear’s role in the consumption and nutrient movement of salmon carcasses. As another example, quantifying the use and consumption (i.e., removal carrion) by various bird scavengers could help clarify the role of each species in preventing the spread of diseases within pastoral agroecosystems (Peisley et al., 2017). Thus, the roles of species in scavenger assemblages are not equal—as some species contribute significantly more to carrion consumption and community diversity.
Measurement of the mechanisms that maintain the carrion–scavenger interactions is one of the least understood topics in the study of scavenging dynamics. Within scavenger communities, facilitative and competitive species interactions occur through carrion acquisition (e.g., Naves-Alegre et al., 2022). These interactions are essential drivers for determining the role of species and species composition of the assemblage, and the loss of species that play a key role in these interactions could lead to the collapse of stable scavenging dynamics. Ecological networks such as a nestedness are useful for identifying these important species (e.g., Allen et al., 2014; Selva and Fortuna, 2007). Evaluating the diel patterns and sequence in which scavenger species visit a carcass can help in understanding intra- and inter-specific interactions. However, the identification of the interactions themselves is insufficient, and the remaining challenge is clarifying the specific interactions driving the scavenging dynamics.
3 Complexity of factors influencing scavenging dynamics
The scavenger assemblage and its functions are influenced by complex interactions of a variety of factors, and this has been a major research question in many studies. Supplementary Table S1 shows the variables selected as metrics of factors influencing scavenging dynamics (abiotic, biotic and anthropogenic factors). These variables have increased with the growing number of case studies, but there has been no consensus reached regarding their selection.
When examining factors that influence scavenging dynamics, it is important to select variables that consider the spatial and temporal scales of scavenging dynamics and carrion–scavenger properties which do not depend on the size of spatial and temporal scales. Pardo-Barquín et al. (2019) highlighted that the structure of scavenger assemblages is driven by different characteristics operating as ecological filters at different habitat scales (i.e. local, landscape, and biogeographical). Additionally, Sebastián-González et al. (2019) suggested that different climatic scales and anthropogenic factors impact scavenger assemblages. Of course, incorporating large scales requires the use of large-scale datasets, which pose challenges such as geographical imbalances and low spatial resolution. In addition, the variables are often interrelated and cannot always be clearly distinguished from each other. For example, temperature and season are often correlated, while habitat and vegetation are also closely linked. Nevertheless, understanding that various factors influence scavenging dynamics hierarchically at different scales could provide a guide for selecting the appropriate scales and variables.
In contrast to abiotic and anthropogenic factors, biotic factors must be considered as carrion–scavenger properties that influence scavenging dynamics at any spatial and temporal scale. The biotic factors can be understood from either the carcass or scavenger perspective. For example, carrion-related factors include carcass size, carcass type, cause of death, and time of death, while scavenger-related factors include the carrion importance (i.e., demand) and carrion preferences. Carrion consumption by invertebrates and decomposers, whose activities are greatly influenced by the environment, also impact scavenging dynamics through competition with vertebrate scavengers.
It is important to not lose sight of the goal of determining whether these factors influence the scavenger assemblage, carrion consumption patterns, or the underlying mechanisms themselves. Previous studies have tended to focus on the questions of what factors influence the scavenger assemblage and carrion consumption patterns, with little consideration of their impact on underlying mechanisms. For example, when a factor affects the foraging behavior of scavenger species at a given time, it suggests that the factor is influencing the mechanisms. Considering that interspecific information flow can influence community composition and its functions (Goodale and Magrath, 2024), identifying the factors that affect the mechanisms of scavenging dynamics is essential for future research.
4 Integrated conceptual model
We developed a conceptual model representing a series of processes linking scavenging dynamics and ecosystem functioning (Figure 1). Our model groups 13 metrics (Table 1) by four questions (Groups A–D: Figure 1) to consider in scavenging research and explains how scavenging dynamics are related to ecosystem functions. This model extends the multi-scale model proposed by Pardo-Barquín et al. (2019), which identifies the factors determining scavenger community composition, and integrates the ecological indicators of scavenging proposed by Newsome et al. (2021). By systematically presenting metrics for measuring the impact of the complex dynamics of scavenger communities on ecosystems, this model broadens the discussion of potential metrics and variables to be considered in future research. Our model is based on terrestrial vertebrate scavenger communities, where research has progressed the most (Newsome et al., 2021). However, our model can also theoretically be applied to different ecosystems such as marine or freshwater ecosystems, as well as to different invertebrate communities.
Our conceptual model is composed of three main components: ecosystem functions, scavenging dynamics, and drivers of scavenging dynamics. Scavenging dynamics are composed of Groups B–D in Figure 1, and by examining these three aspects, we can gain a comprehensive understanding of how scavenging provides ecosystem functions. However, it is important to note that these dynamics are influenced by complex and overarching set of driving factors (Group A in Figure 1), which are hierarchical and can be split into three different metrics (i.e., abiotic, biotic, and anthropogenic factors) across different spatial and temporal scales (global, regional, local, and inner-system properties). Therefore, it is important to consider at which spatiotemporal scales scavenging dynamics are evaluated when designing scavenging research. Tracking scavenging dynamics and their ecosystem functions with hierarchical scales, as shown in the conceptual model, requires enormous effort. On the other hand, the unraveling of these scavenging dynamics from different approaches has already begun in countries such as Spain (e.g., conservation: Margalida et al., 2010, Margalida et al., 2012; Aguilera-Alcalá et al., 2020) and Australia (e.g., invasive species: Newsome et al., 2024).
5 Conclusions
Recent reviews on carrion and scavenging have focused on topics such as the potential impacts of anthropogenic changes [e.g., global changes (Bartel et al., 2024) and introduced species (Newsome et al., 2024) on terrestrial scavenging processes, and proposals to integrate scavenging into wildlife management (Patterson et al., 2022)]—which are leading to cutting-edge research. There is also a recent review which focuses on inland aquatic ecosystems, where research is relatively underdeveloped (Orihuela-Torres et al., 2024). These trends suggested that scavenging studies of terrestrial ecosystems are entering a transitional phase toward applied research, and other less-studied ecosystems and regions are entering a phase of expanded basic research. Amid this rapid increase in the research of scavenging in ecosystems, we strongly advocate that those interested in carrion ecology should focus on contributing to the overall picture of scavenging dynamics. This is because, whether in basic or applied research, we ultimately need to combine indicators of scavenging and ecosystem dynamics to determine the actual ecosystem functions provided by scavenging. Since scavenging dynamics operate across different spatial and temporal scales, it is also necessary to evaluate each ecosystem function at different spatial and temporal scales. For example, the impact of scavenging dynamics on nutrient cycling can span several years in the case of mass mortality events (Subalusky et al., 2017), whereas it may be completed within hours for smaller carcasses (DeVault and Rhodes, 2002). The impact of scavenging dynamics on nutrient movement can sometimes cross ecosystems (Quinn et al., 2009), whereas it may at times be confined to only around the carcass. All of these functions are influenced by scavenging dynamics. The ecological indicators of ecosystem functions summarized by Newsome et al. (2021) are useful, and it is important to clearly state the conditions of scavenging dynamics under the derived results of metrics.
In conclusion, to compare and clarify the ecosystem functions provided by scavenging across ecosystems, further case studies are needed. Therefore, our conceptual model can be applied in several complementary tools throughout the research process: (1) identify the types of information currently lacking in the focal study system, allowing researchers to refine high-priority questions (Groups A–D in Figure 1) and clarify research goals; (2) determine the appropriate metrics and variables based on the spatial and temporal scales (Group A in Figure 1); (3) clarify the perspective (Groups B–D in Figure 1) for evaluating scavenging dynamics in the focal study and to design the research accordingly; and (4) interpret results and extract appropriate conclusions by understanding the flow of the scavenging process. Furthermore, establishing a consensus on the primary variables for metrics within each component of the conceptual model would facilitate more global and systematic comparisons of scavenging dynamics and their associated ecosystem functions, both inner- and inter-systems.
Data availability statement
The original contributions presented in the study are included in the article/supplementary material. Further inquiries can be directed to the corresponding author.
Author contributions
AI: Funding acquisition, Writing – original draft, Conceptualization. MA: Conceptualization, Writing – review & editing. SK: Writing – review & editing, Supervision.
Funding
The author(s) declare that financial support was received for the research and/or publication of this article. This study was supported partly by Japan Society for the Promotion of Science KAKENHI grants (no. JP24K23917), and the Institute of Global Innovation Research in Tokyo University of Agriculture and Technology.
Conflict of interest
The authors declare that the research was conducted in the absence of any commercial or financial relationships that could be construed as a potential conflict of interest.
Generative AI statement
The author(s) declare that no Generative AI was used in the creation of this manuscript.
Publisher’s note
All claims expressed in this article are solely those of the authors and do not necessarily represent those of their affiliated organizations, or those of the publisher, the editors and the reviewers. Any product that may be evaluated in this article, or claim that may be made by its manufacturer, is not guaranteed or endorsed by the publisher.
Supplementary material
The Supplementary Material for this article can be found online at: https://www.frontiersin.org/articles/10.3389/fevo.2025.1611982/full#supplementary-material
References
Aguilera-Alcalá N., Morales-Reyes Z., Martín-López B., Moleón M., and Sánchez-Zapata J. A. (2020). Role of scavengers in providing non-material contributions to people. Ecol. Indic 117, 106643. doi: 10.1016/j.ecolind.2020.106643
Allen M. L., Elbroch L. M., Wilmers C. C., and Wittmer H. U. (2014). Trophic facilitation or limitation? Comparative effects of pumas and black bears on the scavenger community. PloS One 9, e102257. doi: 10.1371/journal.pone.0102257
Bartel S. L., Stephenson T., Crowder D. W., Jones M. E., Storfer A., Strickland M. S., et al. (2024). Global change influences scavenging and carrion decomposition. Trends Ecol. Evol. 39, 152–164. doi: 10.1016/j.tree.2023.09.008
DeVault T. L. and Rhodes O. E. (2002). Identification of vertebrate scavengers of small mammal carcasses in a forested landscape. Acta Theriol (Warsz) 47, 185–192. doi: 10.1007/BF03192458
DeVault T. L., Rhodes O. E., and Shivik J. A. (2003). Scavenging by vertebrates: Behavioral, ecological, and evolutionary perspectives on an important energy transfer pathway in terrestrial ecosystems. Oikos 102, 225–234. doi: 10.1034/j.1600-0706.2003.12378.x
Gerke H. C., Hinton T. G., Okuda K., and Beasley J. C. (2022). Increased abundance of a common scavenger affects allocation of carrion but not efficiency of carcass removal in the Fukushima Exclusion Zone. Sci. Rep. 12, 1–14. doi: 10.1038/s41598-022-12921-y
Goodale E. and Magrath R. D. (2024). Species diversity and interspecific information flow. Biol. Rev. 99, 999–1014. doi: 10.1111/brv.13055
Helfield J. M. and Naiman R. J. (2006). Keystone interactions: Salmon and bear in riparian forests of Alaska. Ecosystems 9, 167–180. doi: 10.1007/s10021-004-0063-5
Levi T., Wheat R. E., Allen J. M., and Wilmers C. C. (2015). Differential use of salmon by vertebrate consumers: Implications for conservation. PeerJ 3, e1157. doi: 10.7717/peerj.1157
Margalida A., Carrete M., Sánchez-Zapata J. A., and Donázar J. A. (2012). Good news for European vultures. Science 335, 284. doi: 10.1126/science.335.6066.284-a
Margalida A., Donázar J. A., Carrete M., and Sánchez-Zapata J. A. (2010). Sanitary versus environmental policies: Fitting together two pieces of the puzzle of European vulture conservation. J. Appl. Ecol. 47, 931–935. doi: 10.1111/j.1365-2664.2010.01835.x
Moleón M. and Sánchez-Zapata J. (2021). The role of carrion in the landscapes of fear and disgust: A review and prospects. Diversity (Basel) 13, 28. doi: 10.3390/d13010028
Montenegro V. M., Mateo-Tomás P., Schneider J., Dent D. H., Crowther T., and Bello C. (2025). Linking functional traits to trophic roles in scavenger assemblages. Ecol. Evol. 15, e70485. doi: 10.1002/ece3.70485
Naves-Alegre L., Morales-Reyes Z., Sánchez-Zapata J. A., and Sebastián-González E. (2022). Scavenger assemblages are structured by complex competition and facilitation processes among vultures. J. Zool 318, 260–271. doi: 10.1111/jzo.13016
Newsome T. M., Barton B., Buck J. C., DeBruyn J., Spencer E., Ripple W. J., et al. (2021). Monitoring the dead as an ecosystem indicator. Ecol. Evol. 11, 5844–5856. doi: 10.1002/ece3.7542
Newsome T., Cairncross R., Cunningham C. X., Spencer E. E., Barton P. S., Ripple W. J., et al. (2024). Scavenging with invasive species. Biol. Rev. 99, 562–581. doi: 10.1111/brv.13035
Olea P P., Mateo-Tomás P., and Sánchez-Zapata J. A. (Eds.) (2019). Carrion ecology and management (Vol. 2). New York, NY: Springer. doi: 10.1007/978-3-030-16501-7
Orihuela-Torres A., Morales-Reyes Z., Hermoso V., Picazo F., Sánchez Fernández D., Pérez-García J. M., et al. (2024). Carrion ecology in inland aquatic ecosystems: a systematic review. Biol. Rev. 99, 1425–1443. doi: 10.1111/brv.13075
Pardo-Barquín E., Mateo-Tomás P., and Olea P. P. (2019). Habitat characteristics from local to landscape scales combine to shape vertebrate scavenging communities. Basic. Appl. Ecol. 34, 126–139. doi: 10.1016/j.baae.2018.08.005
Patterson J. R., DeVault T. L., and Beasley J. C. (2022). Integrating terrestrial scavenging ecology into contemporary wildlife conservation and management. Ecol. Evol. 12, e9122. doi: 10.1002/ece3.9122
Payne L. X. and Moore J. W. (2006). Mobile scavengers create hotspots of freshwater productivity. Oikos 115, 69–80. doi: 10.1111/j.2006.0030-1299.14899.x
Peisley R. K., Saunders M. E., Robinson W. A., and Luck G. W. (2017). The role of avian scavengers in the breakdown of carcasses in pastoral landscapes. Emu-Austral Ornithology 117, 68–77. doi: 10.1080/01584197.2016.1271990
Quaggiotto M. M., Barton P. S., Morris C. D., Moss S. E. W., Pomeroy P. P., McCafferty D. J., et al. (2018). Seal carrion is a predictab le resource for coastal ecosystems. Acta Oecologica 88, 41–51. doi: 10.1016/j.actao.2018.02.010
Quinn T. P., Carlson S. M., Gende S. M., and Rich H. B. (2009). Transportation of Pacific salmon carcasses from streams to riparian forests by bears. Can. J. Zool 87, 195–203. doi: 10.1139/Z09-004
Sebastián-González E., Barbosa J. M., Pérez-García J. M., Morales-Reyes Z., Botella F., Olea P. P., et al. (2019). Scavenging in the Anthropocene: Human impact drives vertebrate scavenger species richness at a global scale. Glob Chang Biol. 25, 3005–3017. doi: 10.1111/gcb.14708
Sebastián-González E., Morales-Reyes Z., Botella F., Naves-Alegre L., Pérez-García J. M., Mateo-Tomás P., et al. (2020). Network structure of vertebrate scavenger assemblages at the global scale: drivers and ecosystem functioning implications. Ecography 43, 1143–1155. doi: 10.1111/ecog.05083
Selva N. and Fortuna M. A. (2007). The nested structure of a scavenger community. Proc. R. Soc. B: Biol. Sci. 274, 1101–1108. doi: 10.1098/rspb.2006.0232
Smith J. B., Laatsch L. J., and Beasley J. C. (2017). Spatial complexity of carcass location influences vertebrate scavenger efficiency and species composition. Sci. Rep. 7, 10250. doi: 10.1038/s41598-017-10046-1
Steinbeiser C. M., Wawrzynowski C. A., Ramos X., and Olson Z. H. (2018). Scavenging and the ecology of fear: Do animal carcasses create Islands of risk on the landscape? Can. J. Zool 96, 229–236. doi: 10.1139/cjz-2016-0268
Subalusky A. L., Dutton C. L., Rosi E. J., and Post D. M. (2017). Annual mass drownings of the Serengeti wildebeest migration influence nutrient cycling and storage in the Mara River. Proc. Natl. Acad. Sci. U S A 114, 7647–7652. doi: 10.1073/pnas.1614778114
Vicente J. and VerCauteren K. (2019). “The role of scavenging in disease dynamics,” in Carrion ecology and management. Eds. Olea O. P., Mateo-Tomás P., and Sánchez-Zapata J. A. (Cham: Springer Cham), 161–182. doi: 10.1007/978-3-030-16501-7_7
Wenting E., Jansen P. A., Burggraeve S., Delsman D. F., Siepel H., and van Langevelde F. (2024). The influence of vertebrate scavengers on leakage of nutrients from carcasses. Oecologia. 206, 24–35. doi: 10.1007/s00442-024-05608-w
Keywords: carrion, carcass, ecosystem function, indicator, vertebrate scavenger
Citation: Inagaki A, Allen ML and Koike S (2025) Integration of specific metrics can promote the understanding of scavenging dynamics. Front. Ecol. Evol. 13:1611982. doi: 10.3389/fevo.2025.1611982
Received: 15 April 2025; Accepted: 05 May 2025;
Published: 22 May 2025.
Edited by:
Mathew Samuel Crowther, The University of Sydney, AustraliaReviewed by:
Torrey Stephenson, University of Idaho, United StatesMatthew Fielding, University of Tasmania, Australia
Copyright © 2025 Inagaki, Allen and Koike. This is an open-access article distributed under the terms of the Creative Commons Attribution License (CC BY). The use, distribution or reproduction in other forums is permitted, provided the original author(s) and the copyright owner(s) are credited and that the original publication in this journal is cited, in accordance with accepted academic practice. No use, distribution or reproduction is permitted which does not comply with these terms.
*Correspondence: Akino Inagaki, YWtpbm8uaW5hZ2FraUBnbWFpbC5jb20=