- 1School of Biological Sciences, University of Southampton, Southampton, United Kingdom
- 2SMART Research Institute, Jalan Teuku Umar, Pekanbaru, Indonesia
- 3Insect Ecology Group, Department of Zoology, University of Cambridge, Cambridge, United Kingdom
- 4School of Natural Sciences, Bangor University, Bangor, United Kingdom
- 5Conservation Science Group, Department of Zoology, University of Cambridge, Cambridge, United Kingdom
- 6School of Geography and Environmental Science, University of Southampton, Southampton, United Kingdom
Oil palm is the most productive vegetable oil crop per unit area and is crucial to the economy of developing countries such as Malaysia and Indonesia. However, it is also highly controversial due to the impact it has on biodiversity. Inputs of herbicides to control understory vegetation in plantations are high, which is likely to harm native biodiversity, but may be unnecessary in protecting oil palm yield. In this study we investigate the effects of understory manipulation using herbicides on soil fauna, litter decomposition rates, and soil abiotic variables: pH, soil organic carbon, soil water content, nitrogen, carbon/nitrogen ratio, potassium, and phosphorous. Understory vegetation was manipulated in three treatments: enhanced understory complexity (no herbicides, developed understory), normal understory complexity (intermediate herbicide use with some manual removal) and reduced understory complexity (heavy herbicide use, no understory vegetation). Two years after treatment, soil macrofauna diversity was higher in the enhanced than the normal, and reduced understory treatment. Furthermore, both macrofauna abundance and litter decomposition was higher in the enhanced than the reduced understory treatment. By contrast, soil fertility did not change between treatments, perhaps indicating there is little competition between oil palms, and understory vegetation. The reduction of herbicide use should be encouraged in oil palm plantations, this will not only reduce plantation costs, but improve soil biodiversity, and ecosystem functioning.
Introduction
Oil palm is the most productive vegetable oil crop per unit area (Zimmer, 2010) and is a crucial part of the economy in developing countries such as Indonesia and Malaysia (Koh and Wilcove, 2007). However, with over 21 million ha of plantations covering the tropics (FAOSTAT, 2016) oil palm cultivation is also one of the most controversial land uses. This is primarily due to the impacts on biodiversity and climate change caused by forest conversion to plantations (Carlson et al., 2013; Savilaakso et al., 2014). Therefore, improving the management of oil palm plantations to protect existing biodiversity and ecosystem functions is vital for agricultural sustainability and biodiversity conservation (Foster et al., 2011). Furthermore, it is in the interest of plantation managers to develop and apply sustainable practices, as this can lead to economic gain (Woittiez et al., 2017) and there is considerable market demand for palm oil to be certified as sustainable by the Round Table on Sustainable Palm Oil (RSPO) (Tayleur et al., 2018). Oil palm has the potential to implement relatively long-term sustainable management practices as it is a perennial crop with a ~25 year commercial lifespan. One of the core management criteria for plantations to be certified as sustainable by the RSPO is to improve soil sustainability (Roundtable on Sustainable Palm Oil., 2013).
Soil biodiversity plays a large part in the ecosystem functions that help maintain soil sustainability (Bardgett and van der Putten, 2014). Soil biota are important for many vital ecosystem functions such as: nutrient cycling; carbon sequestration; and nutrient uptake by plants. However, soil biodiversity is threatened by land use change and agricultural intensification (Tsiafouli et al., 2015; Franco et al., 2016) which can reduce ecosystem functioning (de Vries et al., 2013; Bardgett and van der Putten, 2014). For example, reductions in decomposer functional diversity has been shown to reduce decomposition rates and carbon and nutrient cycling (Handa et al., 2014), which are important ecosystem functions for soil formation and fertility (Nielsen et al., 2011).
While there has been a recent upsurge in research investigating the effects of oil palm plantation management on aboveground biodiversity and ecosystem function (Nurdiansyah et al., 2016; Syafiq et al., 2016; Teuscher et al., 2016), belowground biodiversity and soil functioning has been severely neglected (Bessou et al., 2017). Recent studies have found large declines in soil fertility and, in particular, soil organic carbon (SOC) in oil palm plantations after forest conversion, with continued declines as plantations age (Ashton-Butt et al., in review; Guillaume et al., 2018; Matysek et al., 2018). There are also changes to belowground biodiversity after forest conversion to oil palm; with termites, and litter feeding ants showing severe declines (Luke et al., 2014); and soil microbial communities have been found to alter in community composition and functional gene diversity (McGuire et al., 2015; Tripathi et al., 2016). However, the effect of these changes in biodiversity on ecosystem functioning is little known (Dislich et al., 2016). Recent research has found that the application of organic matter to the soil can improve soil quality and related biotic functions (Carron et al., 2016; Tao et al., 2016, 2018) and different zones around the palm hold varying amounts of soil fauna and nutrients as a result of standard management regimes (Carron et al., 2015).
Soil communities and their functioning are largely impacted by the diversity and abundance of plant communities (Eisenhauer et al., 2011; Thakur and Eisenhauer, 2015). Oil palm plantations can have a reasonably diverse plant understory (Foster et al., 2011). However, these plants are often seen as weeds thought to compete with oil palms for nutrients by some plantation managers and although understory vegetation management varies widely between different plantations, complete removal by herbicides, and weeding is common (Tohiran et al., 2017). A typical plantation uses up to 90% of its pesticide budget on herbicides such as paraquat, glufosinate ammonium, and glyphosphate (Page and Lord, 2006; Wibawa et al., 2010). This extensive use of herbicides can pollute water sources and pose a threat to natural ecosystems and human health (Schiesari and Grillitsch, 2011; Comte et al., 2012). Herbicides are also economically costly, especially to small-scale farmers (Lee et al., 2014). Furthermore, the use of pesticides in agriculture has been linked with mass biodiversity declines around the world (Geiger et al., 2010; Beketov et al., 2013) without consistent benefits to agricultural yield (Lechenet et al., 2017). In oil palm plantations, reduction in herbicide use, and a greater coverage of understory vegetation has been shown to improve avian biodiversity (Nájera and Simonetti, 2010; Tohiran et al., 2017). Furthermore, a greater developed understory benefits aboveground invertebrate communities, by providing additional habitat, and food resources (Chung et al., 2000; Ashraf et al., 2018; Spear et al., 2018). However, it is not known how the understory vegetation in oil palm plantations influences belowground invertebrate communities and related ecosystem functions.
In this study, we investigate the effect of experimentally manipulating understory vegetation in oil palm plantations on soil macrofauna abundance, diversity, and community composition, and litter decomposition rates, and soil abiotic properties in oil palm plantations. We hypothesized that macrofauna abundance and diversity would be positively affected by the amount of understory vegetation and that this would have correspondingly positive effects on soil processes. Our findings will have important implications for the sustainable management of oil palm plantations.
Methods
Study Area
Fieldwork took place in Sumatra, Indonesia, as part of the Biodiversity and Ecosystem Function in Tropical Agriculture (BEFTA) Programme. The BEFTA Vegetation Project is a large-scale, long-term ecological experiment testing the influence of different understory vegetation management strategies on oil palm biodiversity, ecosystem functioning, and yield (Foster et al., 2014). The project is located in oil palm estates owned and managed by Pt Ivo Mas Tunggal, a subsidiary of Golden Agro Resources (GAR) and with technical advice from Sinar Mas Agro Resources and Technology Research Institute (SMARTRI, the research and development center of GAR). The estates are located in the Siak regency of Riau Province, Sumatra (0°55′56″ N, 101°11′62″ E) [see Foster et al. (2014)]. This area receives an average rainfall of 2,400 mm/yr, with the natural landscape characterized by wet lowland forest on sedimentary soils. The soil type is ferralitic with gibbsite and kaolinite (Ferric Acrisol according to the FAO classification). Our study area was logged in the 1970s and the resulting logged forest was converted to oil palm from 1985 to 1995. The plantations included in this study were on average 25 years old (between 29 and 23 years old). The majority of the area around these estates is used to cultivate oil palm. There is no natural forest and few other crops are grown.
Standard fertilizer treatment of oil palm in our study site includes: 1.75 kg tree−1 yr−1 urea (46% N); 0.5 kg tree−1 yr−1 triple super phosphate (45% P2O5, 15% Ca); 2.5 kg tree−1 yr−1 muriate of potash (61% K2O, 46% Cl); and 0.5 kg tree−1 yr−1 Kieserite (16% Mg, S: 22%).
Understory Treatments
Eighteen study plots were established in October 2012. Oil palms on all plots were planted between 1987 and 1993, and so were mature at the time of the study. Plots were 150 × 150 m and are located on flat ground between 10 and 30 m above sea level and without adjacent human habitation. The plantations have a typical zonation of soil and vegetation management leading to 3 distinct zones, weeded circle, harvesting path, and windrow (Figure 1). The plots were arranged adjacently in triplets, with one plot in each triplet randomly assigned one of three understory vegetation management treatments (Figure 2). Treatments were implemented in February 2014, and involved the following management:
1) Normal understory complexity: standard company practice, consisting of intermediate understory vegetation management using herbicides, and some manual removal. The weeded circle (a circular zone around the palm) and harvesting paths were sprayed, and woody vegetation (shrubs and trees) was removed manually.
2) Reduced understory complexity: all understory vegetation was removed using herbicides.
3) Enhanced understory complexity: understory vegetation was allowed to grow with limited interference except for minimal manual clearance in the weeded circle and harvesting paths.
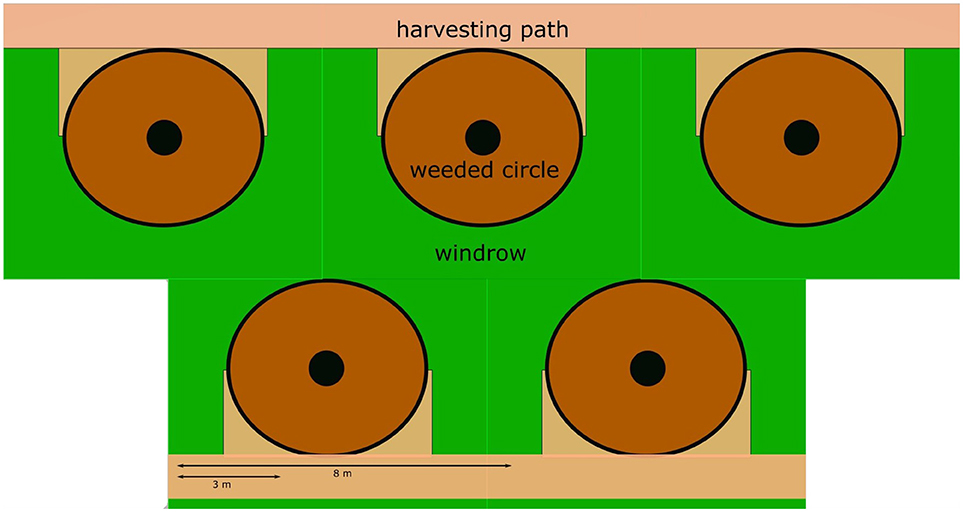
Figure 1. Diagram representing different management zones. The oil palms are the filled circles. The weeded circle is a circular zone with a radius of 1.8 m directly around the palm trunk, which is normally kept “clean” by chemical weed control to facilitate the collection of fruit bunches. The windrow is the zone where the palm fronds pruned during harvest (~18 fronds palm−1 year −1) are placed on the ground forming a U-shaped windrow around the palm. The harvesting path is a zone cleared for access in the alternate rows, with the windrows in-between.
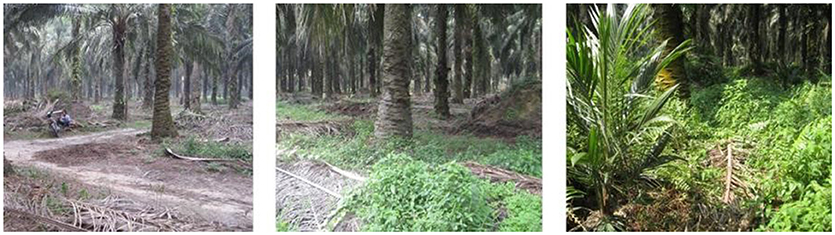
Figure 2. Photographs of the three understory treatments: Reduced complexity; Normal complexity; and Enhanced complexity (from left to right). Photographs courtesy of Edgar Turner.
The herbicides used in the establishment of the plots were Glyphosate (Rollup 480 SL), Paraquat Dichloride (Rolixone 276 SL), metsulfuron-methyl (Erkafuron 20 WG), and Fluroxypyr (Starane 290 EC).
Vegetation Sampling
Ground vegetation surveys were conducted (between April and June 2016, 2 years after the treatments were established)within each of the 6 replicate treatment blocks, at two sampling points (two palms) (12 palms from each treatment), totalling 36 points. At each sampling point, a 1 × 1 m quadrate was placed randomly, 4 times, within both the weeded circle, and windrow zones and the ground cover and bare ground estimated from an average of two observers. In addition, within each quadrat plants were identified to species level and abundance of each species recorded.
Soil Macrofauna Sampling
Soil macrofauna was sampled at the same points as the vegetation surveys, with samples being taken from both the circle, and the windrow, as these have been shown to hold different soil macrofauna abundance and composition (Carron et al., 2015). The harvesting path was not sampled, as this is known to contain a very low abundance of soil macrofauna (Carron et al., 2015). We used a standard Tropical Biology and Fertility Institute soil monolith method to sample invertebrates (Bignell et al., 2008), which involved excavating a 25 × 25 cm quadrat to a depth of 20 cm. All macrofauna, characterized as fauna visible to the naked eye (Kevan, 1968), were removed from soil samples in the field by hand-searching. Worms were placed immediately into formalin and all other arthropods were stored in 70% ethanol for later identification. Invertebrates were sorted to order, with the exception of termites, and ants, which were separated from Blattodea and Hymenoptera, owing to their abundance and distinct ecology, and Diplopoda and Chilopoda, which were identified to class.
Soil Abiotic Sampling
Soil abiotic samples were taken from the same sample locations as the vegetation and soil macrofauna surveys. Soil was collected from the weeded circle and windrow from 0 to 15 cm depth using a soil Dutch auger. At each sampling point, three samples were taken, and bulked from each of the weeded circle and windrow. The weeded circle and windrow have been found to have different soil nutrient contents in previous studies (Carron et al., 2015; Tao et al., 2016) and thus were kept separate.
The following soil chemical properties were measured: soil pH, soil organic carbon content (SOC), total nitrogen (N) content, carbon/nitrogen ratio (C/N ratio), total phosphorous content (P), and total potassium content (K). The soil pH was determined using a pH meter with a soil to water ratio of 1:1. The SOC concentration was measured by loss-on-ignition, using the Walkley–Black method (Nelson and Sommers, 1982). The total soil P concentration was analyzed using the hydrogen chloride extraction method. The total N was determined by the Kjeldahl method (McGill and Figueiredo, 1993). In addition to the chemical properties, soil aggregate stability (the ability of soil particles to resist disintegration) was measured on 3–5 mm aggregates according to the method proposed by Bissonais (1996) and soil water content were measured by the oven drying method.
Litter Decomposition Rates
We used litter decomposition bags, made of fine mesh, to calculate litter mass loss over time. Bags (10 × 10 cm) were filled with 4 g of freshly-cut oil palm fronds that had been dried to a constant weight in the oven. Bags were subject to two treatments: closed bag with no holes, excluding invertebrates, and open bags that had eight 1 cm holes cut into them, allowing access to invertebrates. Closed bags represent decomposition from microbes only and open bags decomposition from microbes and invertebrates. Both closed and open bags were stapled together and placed in each weeded circle and windrow at all sampling points (a total of 144 bags). Bags were left in the field for 30 days after which they were collected, dried at 70°C to a constant weight, and weighed to measure mass loss.
Statistical Analysis
All statistical analysis was performed in R 3.4.4 (R Core Team, 2018). We used linear mixed effects models (LMM) in R package “lme4” (Bates et al., 2014) to examine the effect of understory treatment on order richness and general linear mixed effects models (GLMM) to examine the effect on soil macrofauna abundance (as count data should not be modeled using a Gaussian distribution). We used a negative-binomial distribution to fit the GLMM to account for overdispersion. Understory treatment and sampling zone (weeded circle or windrow) were fitted as categorical fixed effects. Interaction effects were explored between sampling zone and understory treatment for both LMMs and GLMMs and were introduced into the GLMM based on model selection by the AICc value (Brewer et al., 2016). Sampling zone (weeded circle or windrow) was nested within the oil palm sampled and fitted as random effects. Model estimates for GLMMs were presented as incidence rate ratios (Tripepi et al., 2007) as these are more intuitive than the negative-binomially transformed model estimates.
A separate linear mixed effects model with plant species richness and vegetation cover was fitted with understory treatment and sampling location (windrow or weeded circle) as interacting categorical fixed effects to examine the effect of understory treatment on plant species richness and plant cover.
To determine whether understory treatment affected soil macrofauna community composition, we fitted multivariate generalized linear models to the macrofauna abundance data using R package “mvabund” (functions “manyglm” and “anova.manyglm”) (Wang et al., 2012). We used this model-based method to analyse community composition because, unlike distance-based methods (e.g., PRIMER), multivariate generalized linear models can account for the confounding mean–variance relationships that often exist in ecological count data by modeling multivariate abundance data with a negative-binomial distribution (Warton et al., 2016). Model terms were tested for significance with a likelihood ratio test and a Monte Carlo resampling scheme with 999 iterations. Tests were simultaneously performed for univariate (single-order) responses to treatment, adjusting these univariate p-values to correct for multiple testing (Wang et al., 2012).
To explore the effect of understory treatment on soil abiotic properties, LMMs were used with the same model structure as macrofauna order richness. C/N ratio, aggregate stability, and pH fitted a normal distribution, however, soil variables: C, N, P, K, and water content were log-transformed to correct for a non-normal distribution.
To determine the effect of understory treatment on decomposition rates we used a LMM. The model included understory treatment, sampling zone (weeded circle or windrow), and decomposition bag treatment as categorical fixed effects. Interaction effects were explored during model selection between the fixed effects, but were not included based on AICc values (Brewer et al., 2016). Sampling zone (windrow or weeded circle) was nested within the oil palm sampled and fitted as random effects. The model was: decomposition rate~understory treatment + sampling zone + bag treatment (1| oil palm/sample number). Significance of all LMMs and GLMMs were explored via p-values computed by Kenward-Rodger approximation (Luke, 2017).
Results
Vegetation
Vegetation cover did not differ between normal and enhanced understory treatments (estimate = −9.23, P = 0.306), but was higher than the reduced treatment for both weeded circle and windrow (Table 1 and Figure 2). Forty-five plant species were identified in the plantations. Asystasia micrantha was the most abundant species followed by Nephrolepis biserrata, Peperomia pellucida, and Asplenium longissimum. Plant species richness did not differ between normal and enhanced understory treatments, but was higher than the reduced treatment for both weeded circle and windrow (estimate = −2, P = 0.003) (Figure 3). Sampling zone had an interaction effect within treatment; the windrow of the enhanced understory treatment had a lower species richness than the weeded circle (estimate = −1.31, P = 0.035), whereas there was no difference between plant species richness of the weeded circle and windrow in the normal and reduced treatment.
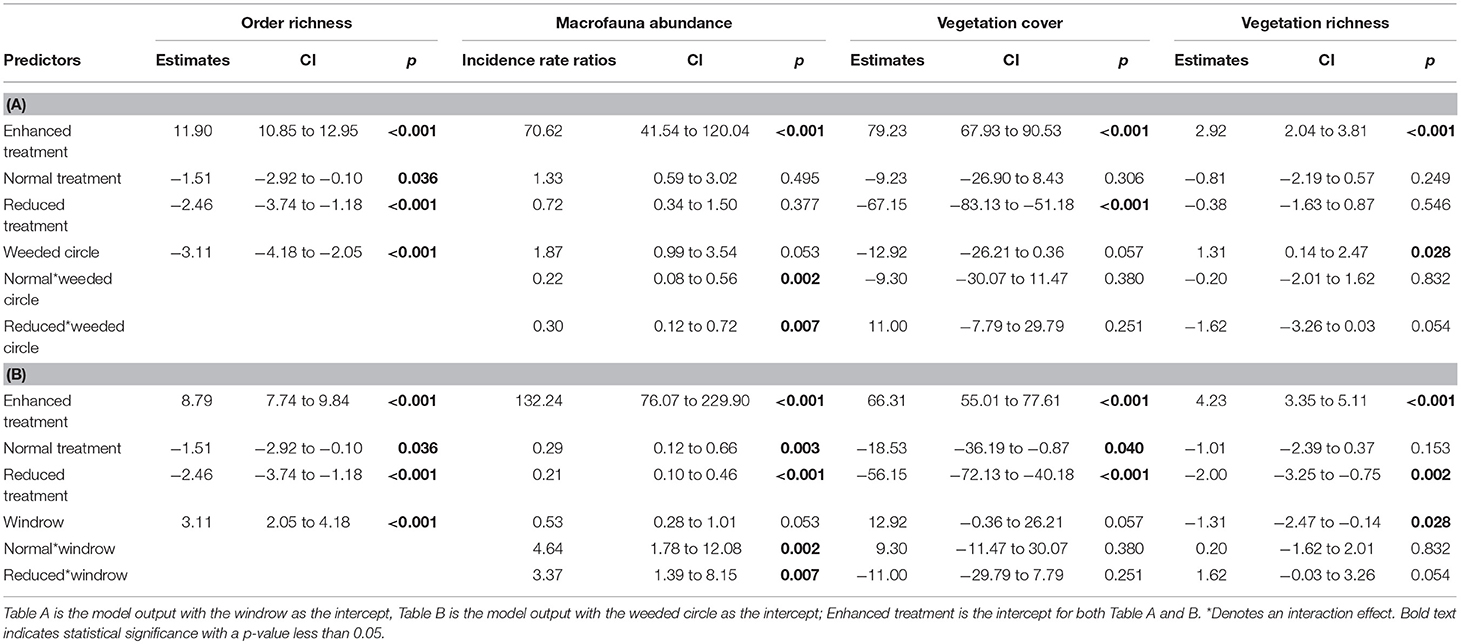
Table 1. Model outputs of LMMs and GLMM comparing macrofauna order richness, abundance, vegetation cover and vegetation richness between Enhanced, Normal, and Reduced treatment.
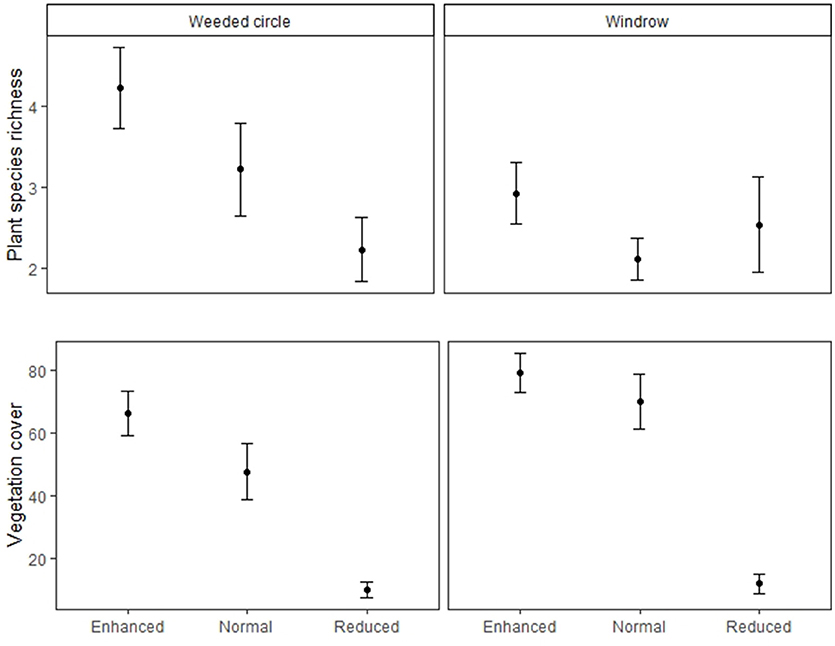
Figure 3. Plant species richness and vegetation cover of the weeded circle and windrow of the Enhanced, Normal and Reduced understory treatments. Filled circles indicate treatment means and bars standard errors.
Macrofauna Richness and Abundance
For the macrofauna survey, we sampled 6,417 individuals from 34 orders and taxonomic groups. Ants were the most abundant group found followed by: Dermaptera, Lumbricidae, Aranae, Isopoda, Diplopoda, Chilopoda, Blattodea, Diplura, Coleoptera, and Diptera. Order richness was higher in the enhanced understory treatment compared to the normal (estimate = −1.51, P < 0.05) and reduced understory treatments (estimate = −2.46, P < 0.001) (Table 1 and Figure 3). Order richness was also higher in the windrow (estimate = +3.11, P < 0.001) than the weeded circle in all treatments (Figure 4). Macrofauna abundance was higher in the weeded circle (but not the windrow) in areas with an enhanced understory than both areas with normal (IRR = 0.22, P < 0.005) and reduced understory (IRR = 0.3, P < 0.01) (Figure 4). In addition, abundance was higher in the windrow than the weeded circle of the normal (IRR = 4.64, P < 0.005); and reduced understory treatments (IRR = 3.37, P < 0.01). However, in the enhanced understory treatment, the windrow had a lower macrofauna abundance than the weeded circle, although, this was marginally non-significant (IRR = 0.53, P = 0.053).
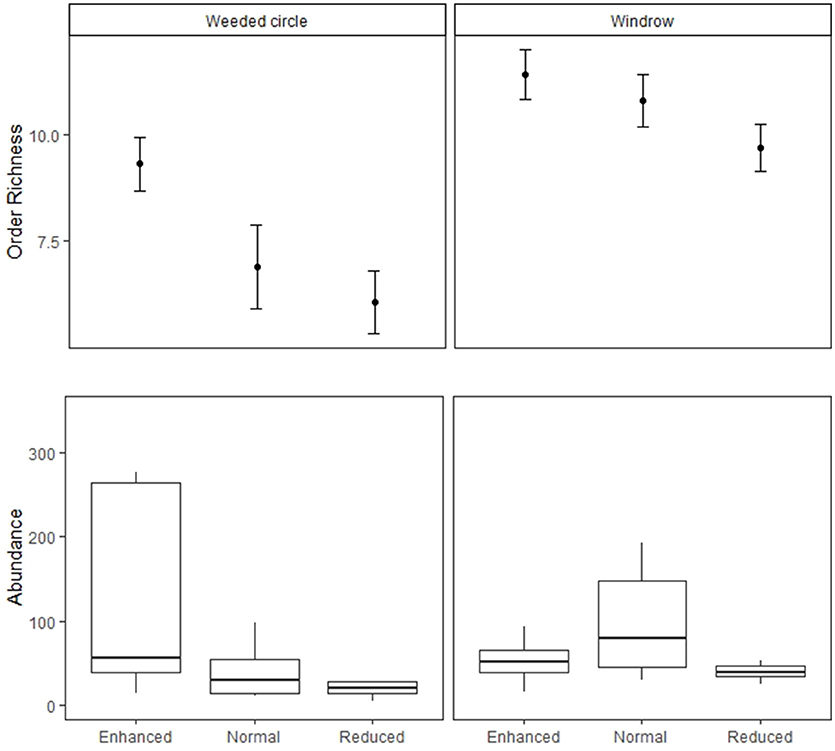
Figure 4. Soil macrofauna abundance and order richness in the weeded circle and windrow of the Enhanced, Normal and Reduced understory treatments. Filled circles indicate treatment means and bars standard errors.
Macrofauna Composition
Understory treatment had an effect on macrofauna composition (LR = 144.4, P < 0.001). The normal (LR = 52.69, P < 0.001) and reduced understory treatment (LR = 115.49, P < 0.001) differed in soil macrofauna composition from the enhanced treatment. The reduced understory treatment exhibited a larger difference in macrofauna composition from the enhanced treatment than the normal understory treatment. Zone of oil palm sampled (weeded circle or windrow) also had an interaction effect with treatment on macrofauna composition in the enhanced (LR = 69, P < 0.001), normal (LR = 38.93, P < 0.01), and reduced (LR = 115.49, P < 0.001) understory treatments. Ant (LR = 13.32, P = 0.02) Coleoptera (LR = 12.55, P = 0.038), Dermaptera (LR = 13.93, P = 0.012), Diplopoda (LR = 11.93, P = 0.048), Isopoda (LR = 13.8, P = 0.013) abundances were all affected by treatment, with lower abundances present in the reduced understory treatment than the enhanced or normal treatments (Figure 5).
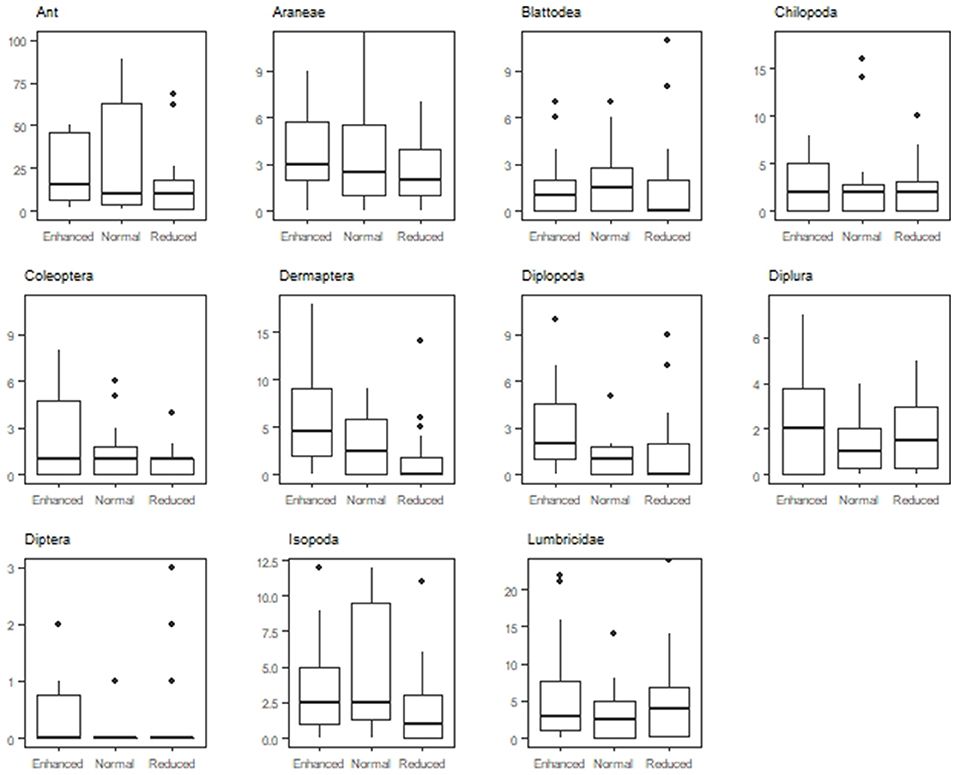
Figure 5. Abundance of the 11 most abundant orders found in the Enhanced, Normal and Reduced understory treatment.
Abiotic Variables
Understory treatment had no effect on SOC, N, P, K, SWC, C/N ratio, aggregate stability or pH (Figure 6 and Table 2). The zone of the oil palm sampled also had no effect on these variables apart from C/N ratio, where the windrow had a slightly higher C/N ratio than the weeded circle (model estimate = +2.65, P = 0.018) and total phosphorous where the windrow had a slightly lower total phosphorous level in the soil than the weeded circle (model estimate = −0.40, P = 0.045).
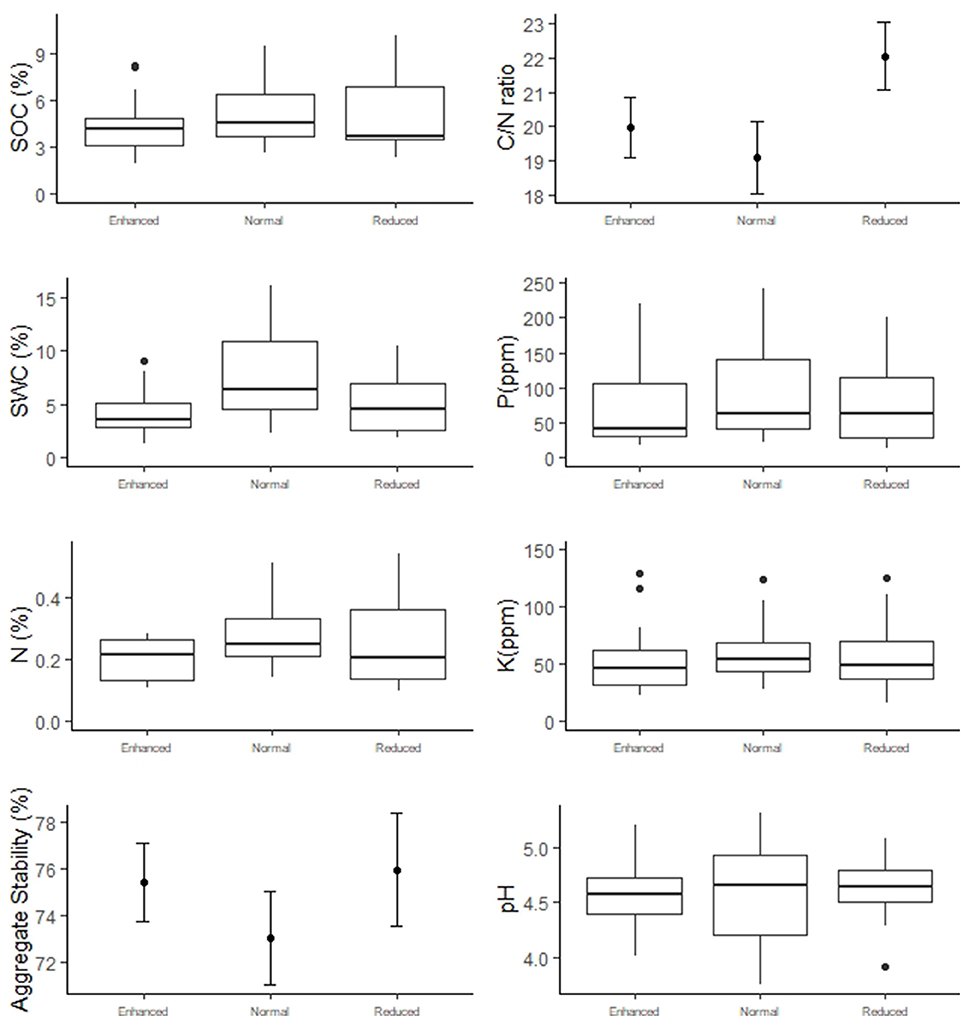
Figure 6. Soil abiotic properties of the Enhanced, Normal and Reduced understory treatments. Box-and-whisker plots present data with a non-normal distribution. Filled circles indicate treatment means and bars standard errors for normally distributed data.
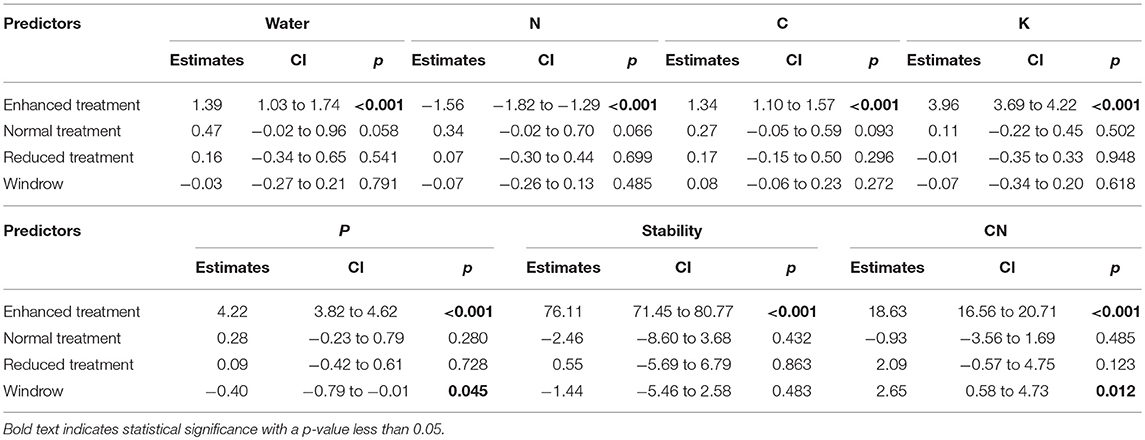
Table 2. Model outputs of LMMs soil abiotic variables between Enhanced, Normal, and Reduced treatment with the weeded circle as the model intercept.
Decomposition
Decomposition rate was higher in the enhanced treatment compared to the reduced understory treatment (estimate = −0.0068 g/day, P = 0.003) (Table 3 and Figure 7) and in the normal treatment compared to the reduced treatment (estimate = −0.0054 g/day, P = 0.028). Decomposition rate was marginally lower in the normal understory treatment compared to the enhanced understory treatment, although this was not statistically significant (estimate = −0.0014 g/day, P = 0.548). Bag treatment also had an effect on decomposition: open bags experienced a higher decomposition rate than closed bags (estimate = 0.0031 g/day, P = 0.042). Sampling zone also had a large effect on decomposition with bags in the windrow experiencing a higher decomposition rate than those in the weeded circle (estimate = 0.0074 g/day, P < 0.001).
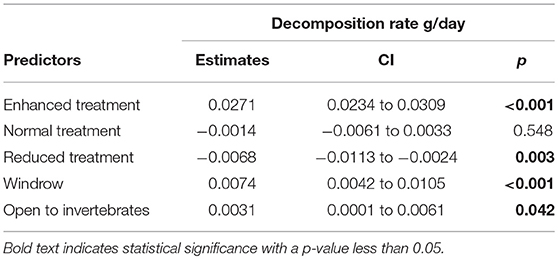
Table 3. Model outputs of LMM comparing litter decomposition rates between Enhanced, Normal, and Reduced treatment with the weeded circle as the intercept.
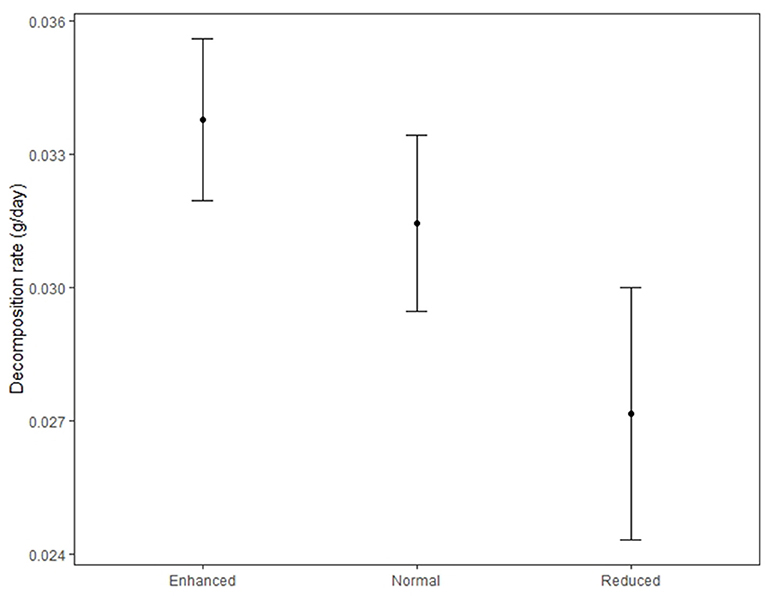
Figure 7. Decomposition rate of litter bags in the Enhanced, Normal, and Reduced understory treatment. Filled circles indicate treatment means and bars standard errors.
Discussion
Our findings show that diversity and abundance of soil macrofauna along with belowground ecosystem functioning can be improved in oil palm plantations by reducing herbicide applications and enhancing understory vegetation. Furthermore, soil nutrient levels were the same in the enhanced understory treatment compared to the other treatments, adding to evidence that understory vegetation is unlikely to compete for nutrients with oil palms.
Soil Macrofauna
Soil macrofauna order richness and abundance were higher in enhanced understory plots than the reduced plots and order richness (but not abundance) was higher in plots with an enhanced understory compared to normal understory plots. Increased plant diversity (characteristic of the enhanced understory plots) has been found to benefit soil biota in other systems (Scherber et al., 2010; Eisenhauer et al., 2011, 2012) and increased understory complexity can increase aboveground invertebrate abundance and food web complexity in oil palm plantations by providing greater resources (Spear et al., 2018). Furthermore, oil palm plantations suffer from hotter and drier microclimates than the natural habitat in the region (Luskin and Potts, 2011), which native soil invertebrates can be sensitive to (Fayle et al., 2010). An increased understory is likely to ameliorate this microclimate by preventing exposure of the soil to direct sunlight and by increasing water infiltration, thus benefitting soil invertebrates (Belsky et al., 1993; Ashraf et al., 2018). Soil macrofauna composition was different in the three understory treatments; taxa that include litter feeding organisms: Dermaptera; Diplopoda; Coleoptera; and Isopoda, all increased in abundance in the enhanced compared to the reduced understory treatment. This is likely due to the greater biomass and diversity of decaying vegetation and root matter provided by the understory plants (Wardle et al., 2004). These fauna are considered ecosystem engineers and are key in breaking down leaf litter and creating a wider availability of resources for microbial decomposers (Brussaard, 2012). Furthermore, the reported positive effects of the understory on soil biodiversity may be conservative in our study; benefits of plant diversity on soil biota can have a significant time delay (Eisenhauer et al., 2012). The enhanced understory treatment had only been installed for 2 years at the time of sampling, therefore, increased positive effects on the soil macrofauna community, and associated ecosystem functions can be expected over time. This is extremely pertinent in oil palm plantations, as they have a long commercial lifespan of more than 25 years. This study was conducted in mature plantations; enhanced understory vegetation could be even more important in young plantations where soil erosion and microclimate is more severe, as there is a reduced canopy cover and less organic matter available from decaying fronds (Luskin and Potts, 2011; Guillaume et al., 2015).
Soil Abiotic Properties
Our results show there was no impact of either treatment on soil fertility. This indicates that the changes in soil macrofauna community were caused by the direct impacts of vegetation. Furthermore, it suggests that the understory vegetation has little impact on nutrient availability for the oil palm, as there was no difference in nutrient levels between the treatments. If enhanced understory vegetation is maintained for an extended period of time, positive effects on soil fertility could be seen as undergrowth is likely to prevent soil erosion, loss of SOM, and leaching of other nutrients (Li et al., 2007; Lieskovský and Kenderessy, 2014).
Decomposition
Litter decomposition rates were substantially lower in reduced understory than in the normal and enhanced understory plots. Decomposition influences carbon storage and underlies soil formation (Swan and Kominoski, 2012). It is also a good indicator of the sensitivity of ecosystem processes to change in species richness (Hooper et al., 2012). The slowed rate of decomposition with reduced understory vegetation corresponds to the loss of macrofauna diversity and abundance (particularly litter feeders) in the reduced understory treatment. Bags that were closed to invertebrates also showed slower decomposition rates in all treatments. This is likely to be explained by a reduction in microbial litter decomposition. This could be a result of reduced macrofauna litter decomposition resulting in a lower availability of pre-digested material for microbes (Brussaard, 2012), and/or that the enhanced understory provides a more favorable microhabitat, and microclimate for microbial fauna, due to the increased soil cover and greater plant diversity. This could increase both microbial diversity and function (Eisenhauer, 2016). These findings have important impacts on soil sustainability and recovery after forest conversion to oil palm plantations and after replanting events, when soils lose large amounts of SOC (Guillaume et al., 2015; Matysek et al., 2018). Increased understory could help ameliorate these negative effects by biologically enhancing SOC sequestration, providing physical protection from soil erosion, and drying and providing a more amenable microclimate.
Conclusions
This study shows that a reduction in herbicide usage and the resulting improvement in understory vegetation diversity and coverage can be a key tool in improving within-plantation belowground biodiversity and ecosystem functioning. Furthermore, we stress that the reduced understory management scheme, that many oil palm plantations employ, has negative impacts on biodiversity, and ecosystem functioning. Reducing herbicide application can also benefit plantation owners by lowering operating costs and reducing health risks to plantation workers that are exposed to herbicides, sometimes without being equipped with the necessary protective equipment.
The improved soil quality realized by increasing understory vegetation in oil palm plantations could improve yield (Balasundram et al., 2006). It is thought that understory plants could compete for nutrients and water with oil palms and cause difficulty in harvesting fallen fruit, thus negatively impacting upon yield (Tohiran et al., 2017). However, we found no evidence for nutrient competition in this study. The impacts on yield are a priority for future research and are being addressed in the larger BEFTA project. However, as environmental conditions can take some time to effect yield, these findings are not published here. Further research into the long-term effects of understory management in oil palm plantations may also realize further benefits to soil sustainability. To support soil biodiversity and ecosystem functioning, increasing understory vegetation should be encouraged by certification schemes, such as the Round Table of Sustainable Palm Oil and other advisors of oil palm agriculture best practice.
Author Contributions
AA-B designed and conducted the study, performed the data analysis and wrote the manuscript. ET and WF designed the manipulation experiment and were involved in writing the manuscript. KP was integrally involved in the study design, and writing the manuscript. JS designed the manipulation experiment was integrally involved in the study design and writing the manuscript. GP was involved in the study design. AH helped with the data collection and writing the manuscript. MN, DP, S, RW, and J-PC helped with the data collection and study design. SW helped with the study design and writing of the manuscript.
Conflict of Interest Statement
The authors declare that the research was conducted in the absence of any commercial or financial relationships that could be construed as a potential conflict of interest.
Acknowledgments
We are grateful to Pt Ivo Mas Tunggal and Golden Agri Resources for allowing us to conduct research on their oil palm plantations, as well as The Isaac Newton Trust, Cambridge and Sinar Mas Agro Resources and Technology Corporation Research Institute (SMARTRI) for funding the BEFTA Project and providing the resources necessary to conduct all fieldwork. We are grateful to SMARTRI researchers and staff, particular thanks to the SMARTRI soil chemistry laboratory for their advice and support with all aspects of the field data collection and for assistance with sample preparations, and soil nutrient analysis. Furthermore, we thank the fantastic: Abbie Roach; Saskia Bloor; and Magnus Gornaja for helping to sort the invertebrate samples. AA-B was funded by the National Environmental Research Council (NERC) [grant number NE/L002531/1], ET and JS were supported by the Natural Environment Research Council [grant number NE/P00458X/1]. KP acknowledges support from the Institute for Life Sciences at Southampton University. AH acknowledges support from the Claire Barnes Studentship from the Department of Zoology, University of Cambridge. We thank RISTEK for research permission to set up and collect data from the BEFTA plots (426/SIP/FRP/SM/XI/2012, 72/EXT/SIP/FRP/SM/IX/2013, 44/EXT/SIP/FRP/SM/IX/2014). All data were collected by and in collaboration with Indonesian research staff from SMARTRI.
References
Ashraf, M., Zulkifli, R., Sanusi, R., Tohiran, K. A., Terhem, R., Moslim, R., et al. (2018). Alley-cropping system can boost arthropod biodiversity and ecosystem functions in oil palm plantations. Agric. Ecosyst. Environ. 260, 19–26. doi: 10.1016/j.agee.2018.03.017
Balasundram, S. K., Robert, P. C., Mulla, D. J., and Allan, D. L. (2006). Relationship between oil palm yield and soil fertility as affected by topography in an indonesian plantation. Commun. Soil Sci. Plant Anal. 37, 1321–1337. doi: 10.1080/00103620600626817
Bardgett, R. D., and van der Putten, W. H. (2014). Belowground biodiversity and ecosystem functioning. Nature 515, 505–511. doi: 10.1038/nature13855
Bates, D., Maechler, M., Bolker, B., and Walker, S. (2014). lme4: linear mixed-effects models using Eigen and S4. R Package Version 1, 1–23.
Beketov, M. A., Kefford, B. J., Schäfer, R. B., and Liess, M. (2013). Pesticides reduce regional biodiversity of stream invertebrates. Proc. Natl. Acad. Sci. U.S.A. 110, 11039–11043. doi: 10.1073/pnas.1305618110
Belsky, A. J., Mwonga, S. M., and Duxbury, J. M. (1993). Effects of widely spaced trees and livestock grazing on understory environments in tropical savannas. Agroforestry Syst. 24, 1–20. doi: 10.1007/BF00705265
Bessou, C., Verwilghen, A., Beaudoin-Ollivier, L., Marichal, R., Ollivier, J., Baron, V., et al. (2017). Agroecological practices in oil palm plantations: examples from the field. OCL 24:D305. doi: 10.1051/ocl/2017024
Bignell, D. E., Huising, J., Moreira, F. M. S., and Fattima, M. S. (2008). A Handbook of Tropical Soil Biology : Sampling and Characterization of Below-Ground Biodiversity. London: Earthscan.
Bissonais, Y. (1996). Aggregate stability and assessment of soil crustability and erodibility: I. theory and methodology. Eur. J. Soil Sci. 47, 425–437. doi: 10.1111/j.1365-2389.1996.tb01843.x
Brewer, M. J., Butler, A., and Cooksley, S. L. (2016). The relative performance of AIC, AIC C and BIC in the presence of unobserved heterogeneity. Methods Ecol. Evol. 7, 679–692. doi: 10.1111/2041-210X.12541
Brussaard, L. (2012). “Ecosystem services provided by the soil biota”, in Soil Ecology and Ecosystem Services, eds D. H. Wall, R. D. Bardgett, V. Behan-Pelletier, J. E. Herrick, T. H. Jones, K. Ritz, J. Six, D. R. Strong, and W. H. van der Putten (Oxford, UK: Oxford University Press), 45–58.
Carlson, K. M., Curran, L. M., Asner, G. P., Pittman, A. M., Trigg, S. N., and Marion Adeney, J. (2013). Carbon emissions from forest conversion by Kalimantan oil palm plantations. Nat. Clim. Chang. 3, 283–287. doi: 10.1038/nclimate1702
Carron, M. P., Auriac, Q., Snoeck, D., Villenave, C., Blanchart, E., Ribeyre, F., et al. (2015). Spatial heterogeneity of soil quality around mature oil palms receiving mineral fertilization. Eur. J. Soil Biol. 66, 24–31. doi: 10.1016/j.ejsobi.2014.11.005
Carron, M. P., Auriac, Q., Snoeck, D., Villenave, C., Blanchart, E., Ribeyre, F., et al. (2016). Do the impact of organic residues on soil quality extend beyond the deposition area under oil palm? Eur. J. Soil Biol. 75, 54–61. doi: 10.1016/j.ejsobi.2016.04.011
Chung, A. Y. C., Eggleton, P., Speight, M. R., Hammond, P. M., and Chey, V. K. (2000). The diversity of beetle assemblages in different habitat types in Sabah, Malaysia. Bull. Entomol. Res. 90, 475–496. doi: 10.1017/S0007485300000602
Comte, I., Colin, F., Whalen, J. K., Grünberger, O., and Caliman, J. P. (2012). Agricultural practices in oil palm plantations and their impact on hydrological changes, nutrient fluxes and water quality in indonesia: a review. Adv. Agron. 116, 71–124. doi: 10.1016/B978-0-12-394277-7.00003-8
de Vries, F. T., Thébault, E., Liiri, M., Birkhofer, K., Tsiafouli, M. A., Bjørnlund, L., et al. (2013). Soil food web properties explain ecosystem services across European land use systems. Proc. Natl. Acad. Sci. U.S.A. 110, 14296–14301. doi: 10.1073/pnas.1305198110
Dislich, C., Keyel, A. C., Salecker, J., Kisel, Y., Meyer, K. M., Auliya, M., et al. (2016). A review of the ecosystem functions in oil palm plantations, using forests as a reference system. Biol. Rev. 92, 1539–1569. doi: 10.1111/brv.12295
Eisenhauer, N. (2016). Plant diversity effects on soil microorganisms: spatial and temporal heterogeneity of plant inputs increase soil biodiversity. Pedobiologia 59, 175–177. doi: 10.1016/j.pedobi.2016.04.004
Eisenhauer, N., Milcu, A., Sabais, A. C. W., Bessler, H., Brenner, J., Engels, C., et al. (2011). Plant diversity surpasses plant functional groups and plant productivity as driver of soil biota in the long term. PLoS ONE 6:e16055. doi: 10.1371/journal.pone.0016055
Eisenhauer, N., Reich, P. B., and Scheu, S. (2012). Increasing plant diversity effects on productivity with time due to delayed soil biota effects on plants. Basic Appl. Ecol. 13, 571–578. doi: 10.1016/j.baae.2012.09.002
Fayle, T. M., Turner, E. C., Snaddon, J. L., Chey, V. K., Chung, A. Y. C., Eggleton, P., et al. (2010). Oil palm expansion into rain forest greatly reduces ant biodiversity in canopy, epiphytes and leaf-litter. Basic Appl. Ecol. 11, 337–345. doi: 10.1016/j.baae.2009.12.009
Foster, W. A., Snaddon, J. L., Advento, A. D., Barclay, H., Caliman, J. P., Rudi, E. C., et al. (2014). The biodiversity and ecosystem function in tropical Agriculture (BEFTA) project. Planter 90, 581–591.
Foster, W. A., Snaddon, J. L., Turner, E. C., Fayle, T. M., Cockerill, T. D., Farnon Ellwood, M. D., et al. (2011). Establishing the evidence base for maintaining biodiversity and ecosystem function in the oil palm landscapes of South East Asia. Philos. Trans. R. Soc. B Biol. Sci. 366, 3277–3291. doi: 10.1098/rstb.2011.0041
Franco, A. L. C., Bartz, M. L. C., Cherubin, M. R., Baretta, D., Cerri, C. E. P., Feigl, B. J., et al. (2016). Loss of soil (macro)fauna due to the expansion of Brazilian sugarcane acreage. Sci. Total Environ. 563–564, 160–168. doi: 10.1016/j.scitotenv.2016.04.116
Geiger, F., Bengtsson, J., Berendse, F., Weisser, W. W., Emmerson, M., Morales, M. B., et al. (2010). Persistent negative effects of pesticides on biodiversity and biological control potential on European farmland. Basic Appl. Ecol. 11, 97–105. doi: 10.1016/j.baae.2009.12.001
Guillaume, T., Damris, M., and Kuzyakov, Y. (2015). Losses of soil carbon by converting tropical forest to plantations: erosion and decomposition estimated by δ13C. Glob. Chang. Biol. 21, 3548–3560. doi: 10.1111/gcb.12907
Guillaume, T., Kotowska, M. M., Hertel, D., Knohl, A., Krashevska, V., Murtilaksono, K., et al. (2018). Carbon costs and benefits of Indonesian rainforest conversion to plantations. Nat. Commun. 9:2388. doi: 10.1038/s41467-018-04755-y
Handa, I. T., Aerts, R., Berendse, F., Berg, M. P., Bruder, A., Butenschoen, O., et al. (2014). Consequences of biodiversity loss for litter decomposition across biomes. Nature 509, 218–221. doi: 10.1038/nature13247
Hooper, D. U., Adair, E. C., Cardinale, B. J., Byrnes, J. E. K., Hungate, B. A., Matulich, K. L., et al. (2012). A global synthesis reveals biodiversity loss as a major driver of ecosystem change. Nature 486, 105–108. doi: 10.1038/nature11118
Koh, L. P., and Wilcove, D. S. (2007). Cashing in palm oil for conservation. Nature 448, 993–994. doi: 10.1038/448993a
Lechenet, M., Dessaint, F., Py, G., Makowski, D., and Munier-Jolain, N. (2017). Reducing pesticide use while preserving crop productivity and profitability on arable farms. Nat. Plants 3:17008. doi: 10.1038/nplants.2017.8
Lee, J. S. H., Garcia-Ulloa, J., Ghazoul, J., Obidzinski, K., and Koh, L. P. (2014). Modelling environmental and socio-economic trade-offs associated with land-sparing and land-sharing approaches to oil palm expansion. J. Appl. Ecol. 51, 1366–1377. doi: 10.1111/1365-2664.12286
Li, J., Okin, G. S., Alvarez, L., and Epstein, H. (2007). Quantitative effects of vegetation cover on wind erosion and soil nutrient loss in a desert grassland of southern New Mexico, USA. Biogeochemistry 85, 317–332. doi: 10.1007/s10533-007-9142-y
Lieskovský, J., and Kenderessy, P. (2014). Modelling the effect of vegetation cover and different tillage practices on soil erosion in vineyards: a case study in vráble (Slovakia) using WATEM/SEDEM. Land Degrad. Dev. 25, 288–296. doi: 10.1002/ldr.2162
Luke, S. G. (2017). Evaluating significance in linear mixed-effects models in R. Behav. Res. Methods 49, 1494–1502. doi: 10.3758/s13428-016-0809-y
Luke, S. H., Fayle, T. M., Eggleton, P., Turner, E. C., and Davies, R. G. (2014). Functional structure of ant and termite assemblages in old growth forest, logged forest and oil palm plantation in Malaysian Borneo. Biodivers. Conserv. 23, 2817–2832. doi: 10.1007/s10531-014-0750-2
Luskin, M. S., and Potts, M. D. (2011). Microclimate and habitat heterogeneity through the oil palm lifecycle. Basic Appl. Ecol. 12, 540–551. doi: 10.1016/j.baae.2011.06.004
Matysek, M., Evers, S., Samuel, M. K., and Sjogersten, S. (2018). High heterotrophic CO2 emissions from a Malaysian oil palm plantations during dry-season. Wetlands Ecol. Manage. 26, 415–424. doi: 10.1007/s11273-017-9583-6
McGill, W. B., and Figueiredo, C. T. (1993). “Total nitrogen,” in Soil Sampling and Methods of Analysis, ed M. R. Carter (Boca Raton, FL: CRC Press), 201–211.
McGuire, K. L., D'Angelo, H., Brearley, F. Q., Gedallovich, S. M., Babar, N., Yang, N., et al. (2015). Responses of soil fungi to logging and oil palm agriculture in Southeast Asian tropical forests. Microb. Ecol. 69, 733–747. doi: 10.1007/s00248-014-0468-4
Nájera, A., and Simonetti, J. A. (2010). Enhancing avifauna in commercial plantations: research note. Conserv. Biol. 24, 319–324. doi: 10.1111/j.1523-1739.2009.01350.x
Nelson, D. W., and Sommers, L. E. (1982). “Total carbon, organic carbon, and organic matter,” in Methods of Soil Analysis. Part 2. Chemical and Microbiological Properties, 2nd Edn, ed A. L. Page, (Madison, WI: ASA SSSA), 539–579.
Nielsen, U. N., Ayres, E., Wall, D. H., and Bardgett, R. D. (2011). Soil biodiversity and carbon cycling: a review and synthesis of studies examining diversity-function relationships. Eur. J. Soil Sci. 62, 105–116. doi: 10.1111/j.1365-2389.2010.01314.x
Nurdiansyah, F., Denmead, L. H., Clough, Y., Wiegand, K., and Tscharntke, T. (2016). Biological control in Indonesian oil palm potentially enhanced by landscape context. Agric. Ecosyst. Environ. 232, 141–149. doi: 10.1016/j.agee.2016.08.006
Page, B., and Lord, S. (2006). The oil palm industry's approach to the use of pesticides in Papua New Guinea. Planter 82, 13–21.
R Core Team (2018). R: A Language and Environment for Statistical Computing. R Foundation for Statistical Computing, Vienna. Available online at: https://www.r-project.org
Roundtable on Sustainable Palm Oil. (2013). RSPO Principles and Criteria for Sustainable Palm Oil Production. Available online at: https://rspo.org/key-documents/certification/rspo-principles-and-criteria (Accessed September 5, 2018).
Savilaakso, S., Garcia, C., Garcia-Ulloa, J., Ghazoul, J., Groom, M., Guariguata, M. R., et al. (2014). Systematic review of effects on biodiversity from oil palm production. Environ. Evid. 3:4. doi: 10.1186/2047-2382-3-4
Scherber, C., Eisenhauer, N., Weisser, W. W., Schmid, B., Voigt, W., Fischer, M., et al. (2010). Bottom-up effects of plant diversity on multitrophic interactions in a biodiversity experiment. Nature 468, 553–556. doi: 10.1038/nature09492
Schiesari, L., and Grillitsch, B. (2011). Pesticides meet megadiversity in the expansion of biofuel crops. Front. Ecol. Environ. 9:139. doi: 10.1890/090139
Spear, D. M., Foster, W. A., Advento, A. D., Naim, M., Caliman, J. P., Luke, S. H., et al. (2018). Simplifying understory complexity in oil palm plantations is associated with a reduction in the density of a cleptoparasitic spider, Argyrodes miniaceus (Araneae: Theridiidae), in host (Araneae: Nephilinae) webs. Ecol. Evol. 8, 1595–1603. doi: 10.1002/ece3.3772
Swan, C. M., and Kominoski, J. S. (2012). “Biodiversity and ecosystem function of decomposition,” in eLS (Chichester: John Wiley & Sons, Ltd). doi: 10.1002/9780470015902.a0023601
Syafiq, M., Nur Atiqah, A. R., Ghazali, A., Asmah, S., Yahya, M. S., Aziz, N., et al. (2016). Responses of tropical fruit bats to monoculture and polyculture farming in oil palm smallholdings. Acta Oecol. 74, 11–18. doi: 10.1016/j.actao.2016.06.005
Tao, H. H., Slade, E. M., Willis, K. J., Caliman, J. P., and Snaddon, J. L. (2016). Effects of soil management practices on soil fauna feeding activity in an Indonesian oil palm plantation. Agricul. Ecosyst. Environ. 218, 133–140. doi: 10.1016/j.agee.2015.11.012
Tao, H. H., Snaddon, J. L., Slade, E. M., Henneron, L., Caliman, J. P., and Willis, K. J. (2018). Application of oil palm empty fruit bunch effects on soil biota and functions: a case study in Sumatra, Indonesia. Agric. Ecosyst. Environ. 256, 105–113. doi: 10.1016/j.agee.2017.12.012
Tayleur, C., Balmford, A., Buchanan, G. M., Butchart, S. H. M., Corlet Walker, C., Ducharme, H., et al. (2018). Where are commodity crops certified, and what does it mean for conservation and poverty alleviation? Biol. Conserv. 217, 36–46. doi: 10.1016/j.biocon.2017.09.024
Teuscher, M., Gérard, A., Brose, U., Buchori, D., Clough, Y., Ehbrecht, M., et al. (2016). Experimental biodiversity enrichment in oil-palm-dominated landscapes in Indonesia. Front. Plant Sci. 7:1538. doi: 10.3389/fpls.2016.01538
Thakur, M. P., and Eisenhauer, N. (2015). Plant community composition determines the strength of top-down control in a soil food web motif. Sci. Rep. 5:9134. doi: 10.1038/srep09134
Tohiran, K. A., Nobilly, F., Zulkifli, R., Maxwell, T., Moslim, R., and Azhar, B. (2017). Targeted cattle grazing as an alternative to herbicides for controlling weeds in bird-friendly oil palm plantations. Agron. Sustain. Dev. 37:62. doi: 10.1007/s13593-017-0471-5
Tripathi, B. M., Edwards, D. P., Mendes, L. W., Kim, M., Dong, K., Kim, H., et al. (2016). The impact of tropical forest logging and oil palm agriculture on the soil microbiome. Mol. Ecol. 25, 2244–2257. doi: 10.1111/mec.13620
Tripepi, G., Jager, K. J., Dekker, F. W., Wanner, C., and Zoccali, C. (2007). Measures of effect: relative risks, odds ratios, risk difference, and ‘number needed to treat.' Kidney Int. 72, 789–791. doi: 10.1038/sj.ki.5002432
Tsiafouli, M. A., Thébault, E., Sgardelis, S. P., de Ruiter, P. C., van der Putten, W. H., Birkhofer, K., et al. (2015). Intensive agriculture reduces soil biodiversity across Europe. Glob. Chang. Biol. 21, 973–985. doi: 10.1111/gcb.12752
Wang, Y., Naumann, U., Wright, S. T., and Warton, D. I. (2012). Mvabund - an R package for model-based analysis of multivariate abundance data. Methods Ecol. Evol. 3, 471–474. doi: 10.1111/j.2041-210X.2012.00190.x
Wardle, D. A., Bardgett, R. D., Klironomos, J. N., Setälä, H., van der Putten, W. H., and Wall, D. H. (2004). Ecological linkages between aboveground and belowground biota. Science 304, 1629–1633. doi: 10.1126/science.1094875
Warton, D. I., Lyons, M., Stoklosa, J., and Ives, A. R. (2016). Three points to consider when choosing a LM or GLM test for count data. Methods Ecol. Evol. 7, 882–890. doi: 10.1111/2041-210X.12552
Wibawa, W., Mohayidin, M. G., Mohamad, R. B., Juraimi, A. S., and Omar, D. (2010). Efficacy and cost-effectiveness of three broad-spectrum herbicides to control weeds in immature oil palm plantation. Pertanika J. Trop. Agricul. Sci. 33, 233–241.
Woittiez, L. S., van Wijk, M. T., Slingerland, M., van Noordwijk, M., and Giller, K. E. (2017). Yield gaps in oil palm: a quantitative review of contributing factors. Eur. J. Agron. 83, 57–77. doi: 10.1016/j.eja.2016.11.002
Keywords: agricultural sustainability, herbicides, best practices, soil macrofauna, invertebrates, ecosystem function, litter decomposition
Citation: Ashton-Butt A, Aryawan AAK, Hood ASC, Naim M, Purnomo D, Suhardi, Wahyuningsih R, Willcock S, Poppy GM, Caliman J-P, Turner EC, Foster WA, Peh KS-H and Snaddon JL (2018) Understory Vegetation in Oil Palm Plantations Benefits Soil Biodiversity and Decomposition Rates. Front. For. Glob. Change 1:10. doi: 10.3389/ffgc.2018.00010
Received: 01 October 2018; Accepted: 30 November 2018;
Published: 14 December 2018.
Edited by:
Janice Ser Huay Lee, Nanyang Technological University, SingaporeReviewed by:
Stuart E. Hamilton, Salisbury University, United StatesGiuliano Maselli Locosselli, University of São Paulo, Brazil
Copyright © 2018 Ashton-Butt, Aryawan, Hood, Naim, Purnomo, Suhardi, Wahyuningsih, Willcock, Poppy, Caliman, Turner, Foster, Peh and Snaddon. This is an open-access article distributed under the terms of the Creative Commons Attribution License (CC BY). The use, distribution or reproduction in other forums is permitted, provided the original author(s) and the copyright owner(s) are credited and that the original publication in this journal is cited, in accordance with accepted academic practice. No use, distribution or reproduction is permitted which does not comply with these terms.
*Correspondence: Adham Ashton-Butt, YS5hc2h0b25idXR0QGdtYWlsLmNvbQ==
Anak A. K. Aryawan, YWp1bmsxMzkwNUBnbWFpbC5jb20=
Jake L. Snaddon, amxzbmFkZG9uQGdtYWlsLmNvbQ==