Time for a Plant Structural Economics Spectrum
- 1CAVElab – Computational and Applied Vegetation Ecology, Ghent University, Ghent, Belgium
- 2ISOFYS – Isotope Bioscience Laboratory, Ghent University, Ghent, Belgium
- 3Department of Plant Sciences, University of Cambridge, Cambridge, United Kingdom
- 4Environmental Change Institute, University of Oxford, Oxford, United Kingdom
- 5Department of Geography, University College London, London, United Kingdom
- 6NERC National Centre for Earth Observation, Leicester, United Kingdom
We argue that tree and crown structural diversity can and should be integrated in the whole-plant economics spectrum. Ecologists have found that certain functional trait combinations have been more viable than others during evolution, generating a trait trade-off continuum which can be summarized along a few axes of variation, such as the “worldwide leaf economics spectrum” and the “wood economics spectrum.” However, for woody plants the crown structural diversity should be included as well in the recently introduced “global spectrum of plant form and function,” which now merely focusses on plant height as structural factor. The recent revolution in terrestrial laser scanning (TLS) unlocks the possibility to describe the three dimensional structure of trees quantitatively with unprecedented detail. We demonstrate that based on TLS data, a multidimensional structural trait space can be constructed, which can be decomposed into a few descriptive axes or spectra. We conclude that the time has come to develop a “structural economics spectrum” for woody plants based on structural trait data across the globe. We make suggestions as to what structural features might lie on this spectrum and how these might help improve our understanding of tree form-function relationships.
Introduction
Here, we argue that tree and crown structural diversity can and should be central to a new, integrated whole-plant economic spectrum, to improve our understanding of the remarkable diversity of plants on Earth. This diversity includes a huge range of tree species that shape their environment and ecosystem, from boreal forests to tropical rainforests. The latter in particular contain more than 50,000 tree species (Slik et al., 2015). Each of these individual species have traits that are a result of their evolutionary development and are in turn also proxies for their function and form.
Ecologists have found that certain functional trait combinations have been more viable than others during evolution, generating a trait trade-off continuum which can be summarized along a few axes of variation. The first important step in this endeavor to capture critical traits underpinning plant diversity was the proposition of the “worldwide leaf economics spectrum” (LES) (Wright et al., 2004). Based on leaf chemical, morphological and physiological traits, this spectrum runs from acquisitive to conservative assimilation strategies in leaves, and operates largely independent of plant functional type and biome. However, debates about different aspects of this spectrum and its utility have not been without controversy (Osnas et al., 2013). Nevertheless, following this concept, a second spectrum was defined describing the trade-offs between woody tissues and tree function: “the wood economics spectrum” (WES) (Chave et al., 2009). Similar mechanisms of resource acquisition are driving the trade-offs of both the LES and the WES. Most recently, Díaz et al. (2015) proposed a two-dimensional global spectrum of plant form and function, in which a first dimension describes the size and seed mass of plants and a second dimension correlates with the leaf economics spectrum. This “global spectrum of plant form and function” (PES) integrates and expands the LES and the WES. However, we argue that for woody plants the crown structural diversity should be included as well in an integrative spectrum of plant form and function, which now merely focusses on plant/tree height as structural factor.
There are good reasons to assume that crown structure and architecture can be used to predict scaling of plant form and function across taxa, because it is known that structure and geometry of branches and roots directly affect resource acquisition, mechanical support, reproduction, and competitive ability (Bentley et al., 2013; Messier et al., 2017). The theory of metabolic scaling provides a synthesis of functioning and structure of plants as an economic trade-off and allows for example to predict growth of individuals (Enquist et al., 2009). However, observational studies on crown structural metrics have argued that the original metabolic scaling theory appears overly simplistic and needs to be extended to account for intra- and inter-species variability in allometric scaling (Pretzsch and Dieler, 2012). This latter study not only showed that crown plasticity is a prerequisite for plant competitiveness, but also illustrated the limitation of traditional tree biometric observations which only account for crude aspects of tree crown structure.
Indeed, until recently, the detailed description of tree or crown structure was not possible in a quantitative way and remained limited to estimates of relatively easy-to-observe metrics such as crown depth or crown projection area. Alternatively, qualitative descriptions of crown shapes have been used to group tree species in categories, for example based on the famous drawings of tropical trees by Hallé et al. (1978).
The recent revolution in terrestrial laser scanning (TLS) unlocks the possibility of describing the three dimensional structure of trees quantitatively and in unprecedented detail (Malhi et al., 2018). In the past few years, thousands of trees have been scanned in biomes across the globe and these information-rich point cloud data are currently being analyzed for a range of applications. We argue that with this revolution of new measurements and ways to analyze them, the time has come to develop a “structural economics spectrum” (SES) for woody plants.
SES Concept
An economic trade-off in ecology is an exchange of increased function or fitness in some aspect of life history strategy at the expense of decreased function or fitness in another. For example, thin leaves cost less carbon to deploy but are shorter-lived. A common test for the existence of such an economic trade-off is a correlation of otherwise unrelated traits. Evidence for a structural economics spectrum would thus constitute a suite of structural traits that are decomposed across fewer dimensions than traits. An economic spectrum of tree crown traits is not hard to imagine. Branching angles and crown widths, which may allow for great light interception, may be constrained by wood structural strength, carbon investment and mechanical risk.
Forests are characterized by a wide variety of tree crown shapes, which are extremely plastic and can be strongly influenced by habitat (e.g., the shape of an oak crown in a forest vs. in an open field). However, the species-specific crown shape is likely linked to a strategy to thrive in a certain abiotic and biotic environment. This strategy potentially links the structural and functional traits of a tree species. If we, for example, compare a Cecropia sp. tree in a tropical rainforest with an Abies sp. tree in a boreal forest, we know that the light demanding Cecropia has the strategy to grow up quickly and deploy a horizontal crown to compete for light, while the boreal fir has a conical shape, with shade adapted leaves (needles) at the slow return-on-investment end of the LES.
We define the SES as a paradigm describing a low-dimensionality space upon which plant structural types can be positioned against investment in other functional traits. This means that the SES represents the synthesis of variation in structural traits which can be positioned against other spectra as part of the whole plant economics spectrum (Figure 1).
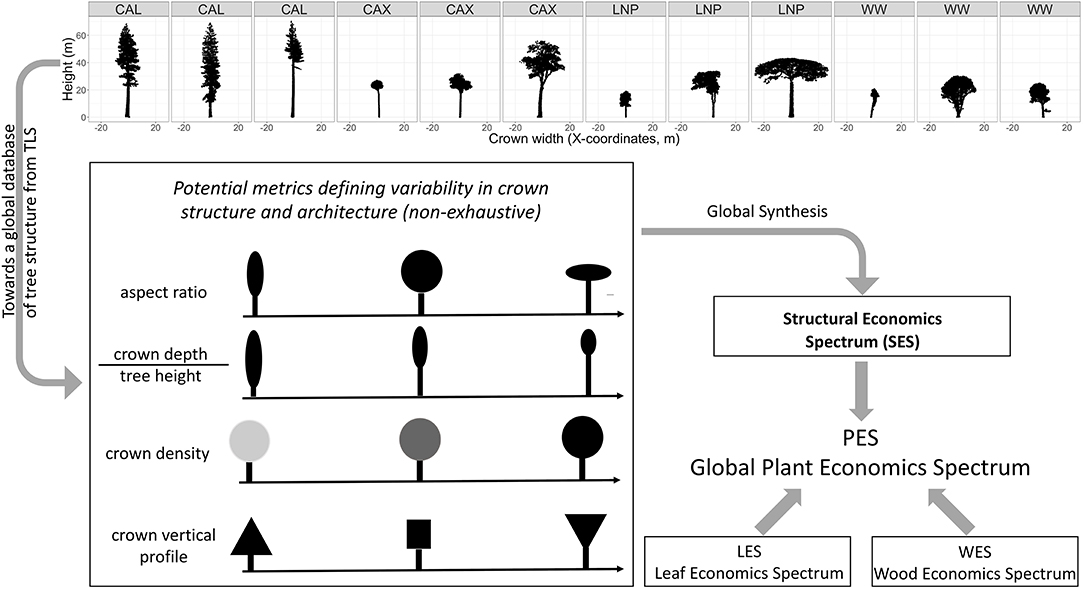
Figure 1. Concept for the development of a structural economics spectrum. Top panel: examples of structural diversity of trees captured by TLS with scans from: CAL, Giant redwoods at Grove of Old Trees site (Sequioa sempervirens) California (US); CAX, diverse tropical rainforest tree species in Caxiuanã drought experiment control plot (Brazil); LNP, diverse tropical tree species at Lopé National Park (Gabon); WW, temperate Acer pseudoplatanus trees from Wytham Woods (UK). The redwood (CAL) and sycamore (WW) TLS scans illustrate within-species variability, while the scans of tropical trees (CAX, LNP) illustrate crown shape variability within a diverse tropical forest plot of one hectare in Lopé and Caxiuanã. The bottom panel illustrates that a series of structural metrics could be synthesized in a low dimensionality space which defines the SES. The SES can potentially be integrated with the LES and WES into the PES.
The concept of a SES can be based on a series of tree structural and architectural metrics that can particularly be derived from trees reconstructed from TLS point clouds (Figure 1). This includes new metrics (such as branching topology and path length, i.e., the lengths of possible pathways through a tree from root to branch tip) which are only measurable by TLS and not with traditional observations or airborne laser observations. Furthermore, TLS provides measurements on a continuous scale, rather than using discrete categories of structure. A global analysis of these new metrics or other more familiar structural traits (e.g., crown aspect ratio, crown depth/tree height ratio, “density” of the crown i.e., the space-filling nature of the within-crown material, vertical crown density profiles, branch size, and angle distribution) should allow us to identify evolutionarily successful structural trait combinations.
Proof of Concept
Recently, a first synthesis effort (Jackson et al., 2019) on more than 1,000 TLS scanned trees from multiple locations across the globe was done to study wind-tree interactions and natural sway frequencies. Based on the structural metrics from that study, we can demonstrate the existence of a SES using a principle component analysis (PCA) (Figure 2).
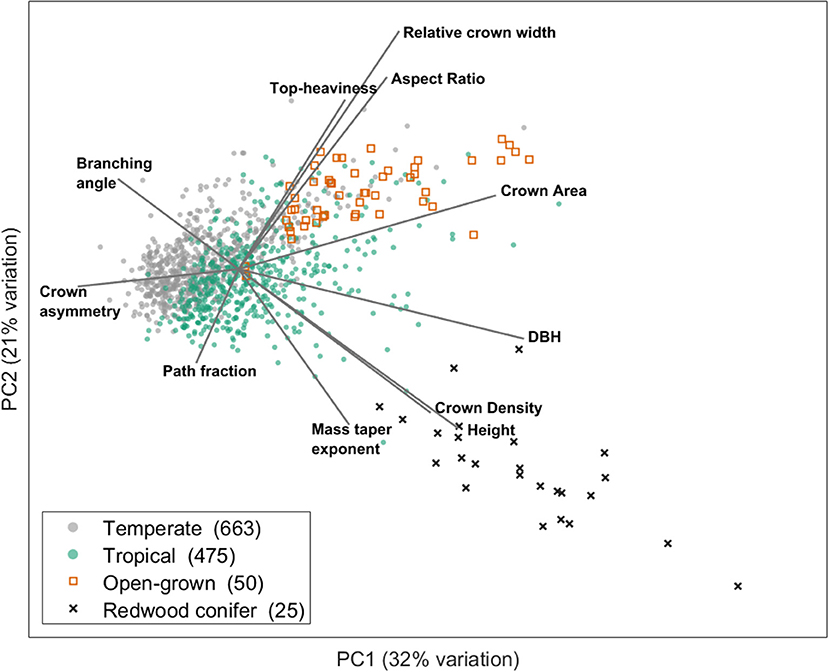
Figure 2. Illustration of how a multidimensional structural trait space can be decomposed in a few dominant axes which form the basis of a structural economics spectrum. This figure is modified from Jackson et al. (2019). The figure shows the two main axes of variation resulting from a principal component analysis executed on structural trait data extracted from cylinder models of TLS 3D point clouds from 1,213 trees from temperate and tropical ecosystems. Only trees taller than 10 m were included in the analysis for clarity. The included architectural metrics are: Top-heaviness (ratio of total woody volume in the crown to the stem woody volume), relative crown width (ratio of maximum crown width to tree height), aspect ratio (ratio of maximum crown width to crown height), crown area (maximum ground area covered by the crown viewed from above), DBH (stem diameter at breast height), crown density (ratio of crown area to woody volume in the crown), height (total tree height), mass taper exponent (exponent of a power law fit to the vertical profile of volume), path fraction (ratio of mean to maximum base-to-twig path length), crown asymmetry (the reatio of maximum to mean of 8 angular crown segments) and branching angle (the average angle between two cylinders at each branching point). More information on the underlying data can be found in the original study of Jackson et al. (2019).
This PCA shows that a multidimensional structural trait space can indeed be decomposed in a few axes (spectra) which describe dominant trade-offs in structural traits and form the basis of a structural economics spectrum. The two PCA axes in this example explain together 53% of the variation and are determined by structural traits related to tree size, crown shape and fine scale architectural metrics. Trees from temperate vs. tropical biomes can be positioned along these axes and it is clear that tropical trees span a wider range of structural types. The first PCA axis (explaining 32% of the variation) is clearly related to tree height (and other size related metrics such as diameter at breast height and crown area), a trait which is already included in the current PES. However, fine scale architectural traits such as branching angles are also correlated to this first axis. The second PCA axis (explaining 21% of the variation) exhibits structural traits that determine crown shape (e.g., crown aspect ratio and relative crown width) and fine scale architecture (e.g., path fraction), independent from the first axis. As such, large trees like Californian redwoods or open-grown city trees are both located at the right side of the first axis, but distinguished on the second axis by structural traits different from tree size as such.
Outlook and Conclusion
To construct a global SES the scientific community needs to expand the proof of concept we presented here, which was done only on a relatively limited number of trees. Therefore, structural metrics of a globally spread large number of trees needs to be analyzed in analogy to the global efforts that have been done to construct the LES. To achieve such an ambition, a community effort will be needed to standardize TLS data collection protocols [in line with other current similar efforts, e.g., CEOS (Duncanson et al., 2019)], and establish a repository for reconstructed trees with associated metadata to ensure reproducibility. Challenges remain to accurately extract individual trees from scans of 3D forest point clouds and reconstruct tree architecture. However, extracting structural information is a problem that lends itself very well to approaches based on recent rapid advances in big data science, particularly artificial intelligence and machine learning approaches to pattern recognition. A standardization and synthesis effort should allow to construct a global dataset of structural traits, guided by the example of existing community efforts for plants functional traits (e.g., Kattge et al., 2011).
A global analysis of matched functional and structural trait datasets, could further elaborate on the interaction between functional and structural spectra and test to what extent these spectra are independent or correlated. We have focused in this perspective paper on woody plants, but we suggest that including non-woody plants in a global analysis could allow to test if the SES can be generalized to all plants beyond woody plants alone.
In conclusion, we expect that the structural economics spectrum will help to functionally describe tree species and woody ecosystems in a much more general way, crucially allowing testable predictions to be made about the relationships between ecosystem structure and function and vice versa. A successful SES would also enable tree structural diversity to be addressed in global vegetation models and models of forest demography. Moreover, the SES will allow for a better understanding of habitat diversity essential for species survival and biodiversity conservation (WWF, 2018) and to monitor forest degradation.
Data Availability
The datasets generated for this study are available on request to the corresponding author.
Author Contributions
All authors listed have made a substantial, direct and intellectual contribution to the work, and approved it for publication.
Conflict of Interest Statement
The authors declare that the research was conducted in the absence of any commercial or financial relationships that could be construed as a potential conflict of interest.
Acknowledgments
HV was funded by the European Research Council Starting Grant 637643 (TREECLIMBERS). KC was funded by BELSPO (Belgian Science Policy Office) in the frame of the STEREO III programme – project 3D-FOREST (SR/02/355). TJ was funded by NERC grant NE/S010750/1. MD acknowledges capital funding for TLS equipment from NERC National Centre for Earth Observation (NCEO) as well as funding from NERC grant NE/P011780/1 Understanding tree architecture, form and function in the tropics, and NASA Carbon Monitoring System Grant #15-CMS15-0055. We thank partners for supporting TLS data collection in their sites. For Danum Valley in Malaysia, the Sabah Forestry Department and the Sabah Biodiversity Council. For Lopé in Gabon, the Agence National de Parcs du Gabon. For Caxiuanã in Brazil, Museu Paraense Emílio Goeldi. For Wineperu in Guyana, the Guyana Forestry Commission. We thank D. Culvenor and G. Newnham for supporting the TLS data collection at Rushworth Forest. We also acknowledge help with TLS data collection in California from Laura Duncanson, John Armston, and Andy Burt. TLS data collection at Wytham Woods was funded through the Metrology for Earth Observation and Climate project (MetEOC-2), grant no. ENV55 within the European Metrology Research Programme (EMRP). The EMRP was jointly funded by the EMRP participating countries within EURAMET and the European Union.
References
Bentley, L. P., Stegen, J. C., Savage, V. M., Smith, D. D., von Allmen, E. I., Sperry, J. S., et al. (2013). An empirical assessment of tree branching networks and implications for plant allometric scaling models. Ecol. Lett. 16, 1069–1078. doi: 10.1111/ele.12127
Chave, J., Coomes, D., Jansen, S., Lewis, S. L., Swenson, N. G., and Zanne, A. E. (2009). Towards a worldwide wood economics spectrum. Ecol. Lett. 12, 351–366. doi: 10.1111/j.1461-0248.2009.01285.x
Díaz, S., Kattge, J., Cornelissen, J. H., Wright, I. J., Lavorel, S., Dray, S., et al. (2015). The global spectrum of plant form and function. Nature 529:167. doi: 10.1038/nature16489
Duncanson, L., Armston, J., Disney, M., Avitabile, V., Barbier, N., Calders, K., et al. (2019). The importance of consistent global forest aboveground biomass product validation. Surv. Geophys. 40, 979–999. doi: 10.1007/s10712-019-09538-8
Enquist, B. J., West, G. B., and Brown, J. H. (2009). Extensions and evaluations of a general quantitative theory of forest structure and dynamics. Proc. Natl. Acad. Sci.U.S.A. 106, 7046–7051. doi: 10.1073/pnas.0812303106
Hallé, F., Oldeman, R. A., and Tomlinson, P. B. (1978). Tropical Trees and Forests. An Architectural Analysis. Berlin: Springer. doi: 10.1007/978-3-642-81190-6
Jackson, T., Shenkin, A., Moore, J., Bunce, A., van Emmerik, T., Kane, B., et al. (2019). An architectural understanding of natural sway frequencies in trees. J. R. Soc. Interf. 16:20190116. doi: 10.1098/rsif.2019.0116
Kattge, J., Díaz, S., Lavorel, S., Prentice, I. C., Leadley, P., Bönisch, G., et al. (2011). TRY—a global database of plant traits. Global Change Biol. 17, 2905–2935. doi: 10.1111/j.1365-2486.2011.02451.x
Malhi, Y., Jackson, T., Patrick Bentley, L., Lau, A., Shenkin, A., Herold, M., et al. (2018). New perspectives on the ecology of tree structure and tree communities through terrestrial laser scanning. Interface Focus 8:52. doi: 10.1098/rsfs.2017.0052
Messier, J., Lechowicz, M. J., McGill, B. J., Violle, C., and Enquist, B. J. (2017). Interspecific integration of trait dimensions at local scales: the plant phenotype as an integrated network. J. Ecol. 105, 1775–1790. doi: 10.1111/1365-2745.12755
Osnas, J. L., Lichstein, J. W., Reich, P. B., and Pacala, S. W. (2013). Global leaf trait relationships: mass, area, and the leaf economics spectrum. Science 340, 741–744. doi: 10.1126/science.1231574
Pretzsch, H., and Dieler, J. (2012). Evidence of variant intra- and interspecific scaling of tree crown structure and relevance for allometric theory. Oecologia 169, 637–649. doi: 10.1007/s00442-011-2240-5
Slik, J. W. F., Arroyo-Rodríguez, V., Aiba, S. I., Alvarez-Loayza, P., Alves, L. F., Ashton, P., et al. (2015). An estimate of the number of tropical tree species. Proc. Natl. Acad. Sci.U.S.A. 112, 7472–7477. doi: 10.1073/pnas.1423147112
Wright, I. J., Reich, P. B., Westoby, M., Ackerly, D. D., Baruch, Z., Bongers, F., et al. (2004). The worldwide leaf economics spectrum. Nature 428, 821–827. doi: 10.1038/nature02403
Keywords: laser scanning (LiDAR), canopy 3D structure, forest, plant functional trait, plant structural trait
Citation: Verbeeck H, Bauters M, Jackson T, Shenkin A, Disney M and Calders K (2019) Time for a Plant Structural Economics Spectrum. Front. For. Glob. Change 2:43. doi: 10.3389/ffgc.2019.00043
Received: 23 April 2019; Accepted: 19 July 2019;
Published: 06 August 2019.
Edited by:
Nikolaos M. Fyllas, University of the Aegean, GreeceReviewed by:
Xiangzhong Remi Luo, Lawrence Berkeley National Laboratory, United StatesChristopher Benjamin Anderson, Stanford University, United States
Copyright © 2019 Verbeeck, Bauters, Jackson, Shenkin, Disney and Calders. This is an open-access article distributed under the terms of the Creative Commons Attribution License (CC BY). The use, distribution or reproduction in other forums is permitted, provided the original author(s) and the copyright owner(s) are credited and that the original publication in this journal is cited, in accordance with accepted academic practice. No use, distribution or reproduction is permitted which does not comply with these terms.
*Correspondence: Hans Verbeeck, hans.verbeeck@ugent.be