- 1Griffith School of Environment and Science, Australian Rivers Institute, Griffith University, Brisbane, QLD, Australia
- 2Smithsonian Tropical Research Institute, Ancon, Panama
- 3Department of Agriculture and Fisheries, University of the Sunshine Coast, Sippy Downs, QLD, Australia
The changes in fire regimes expected under climate change are likely to disrupt the biogeochemical cycling of carbon (C) and nutrients in forest ecosystems. Plant litter decomposition is a critical step in the terrestrial biogeochemical cycle, and is an important determinant of fire fuel load and forest C balance. We conducted a 277-day leaf litter decomposition experiment in an Australian eucalypt forest to test whether three contrasting, long-term fire regimes (no burning [NB], 4-yearly burning, and 2-yearly burning) were associated with different C and nutrient dynamics during litter decomposition. Fire regime had strong effects on many litter properties, including overall rates of decomposition and C loss, which were greatest in the NB treatment, suggesting that fire regime can modify the rate at which C is returned from litter to soil or the atmosphere. This has potentially important implications for soil C storage and atmospheric CO2 concentrations under a changing climate. High-frequency fire was associated with litter nutrient depletion and high microbial nutrient demand, but did not affect nutrient loss rates from decomposing litter, suggesting conservative use and retention of nutrients by the litter microbial biomass. These effects differed qualitatively between 2- and 4-yearly burning regimes, and they show how decadal-scale increases in fire frequency might contribute to soil nutrient depletion by disrupting decomposition. Many effects of fire regime on litter properties throughout decomposition were sensitive to litter bag re-collection date, suggesting that seasonal factors moderate the effects of fire regime, and that the role of fire regime-altered litter chemistry in shaping decomposition may be secondary to that of fire regime-altered environmental variables. Together, our findings highlight the potential consequences of long-term increases in prescribed fire frequency for litter decomposition and the storage and cycling of C and nutrients in eucalypt forests, and reveal the specific importance of average burn frequency in this context.
Introduction
Vegetation fires currently affect 360–380 million hectares of the Earth's terrestrial surface each year (Alonso-Canas and Chuvieco, 2015). However, fire regimes are shifting worldwide due to climate change such that the extent, severity, and frequency of wild and prescribed fires are increasing in many ecosystems (Liu et al., 2010; Abatzoglou and Williams, 2016; Boer et al., 2016). These changes in fire regime are likely to have various consequences for ecosystem structure and function, yet uncertainties remain. In particular, while individual fire events often have marked impacts on the biogeochemical cycling of elements within ecosystems (e.g., Fioretto et al., 2005; Holden and Treseder, 2013; Butler et al., 2018), it is less clear how biogeochemical cycling will respond to the changes in fire regime that manifest over longer (i.e., decadal) timescales, such as shifts in average fire frequency or return interval. Long-term changes in ecosystem C cycling processes associated with decadal-scale changes in fire regime can increase or reduce an ecosystem's tendency to store C in soil and vegetation (e.g., Carney et al., 2007; Pellegrini et al., 2018), while changes in ecosystem nutrient cycling and availability can affect ecosystem productivity (e.g., Dijkstra et al., 2017) and, in doing so, alter the ability of vegetation to fix atmospheric CO2 (Conroy et al., 1992; McGuire et al., 1995). Thus, the effects of long-term fire regime on C and nutrient cycling have strong potential to feed back into the global climate system (Bowman et al., 2009).
An aspect of C and nutrient cycling that has particular relevance to fire regime and climate change feedbacks is decomposition, the process by which dead organic material breaks down into its constituent components via physical, chemical, and biological processes (Chapin et al., 2002). By regulating the rate of accumulation of dead plant biomass in an ecosystem, decomposition processes exert important influence over the availability of fuel for combustion, thus influencing the intensity, severity, and the rate and direction of spread of fires (Birk and Bridges, 1989; Brennan et al., 2009; Bradstock et al., 2010). At the same time, decomposition represents the primary flux of C and nutrients from plant biomass to soil and can thus act as a critical bottleneck on rates of soil C sequestration and ecosystem productivity (Chapin et al., 2002; Prescott, 2010). A strong understanding of decomposition and elemental fluxes under different fire regimes is, therefore, particularly important for forests dominated by Eucalyptus species or other sclerophyllous Myrtaceae (hereafter “eucalypt forests”). This is because eucalypt forests are highly fire prone, particularly during periods of drought (Gill and Zylstra, 2005), and because there is ongoing debate around the efficacy of fuel reduction burning in lowering wildfire risk (e.g., Bradstock et al., 2012; McCaw, 2013; Zylstra, 2018) and around the effects of prescribed surface fires on soil and ecosystem C storage in eucalypt forests (Murphy et al., 2010; Bennett et al., 2013; Krishnaraj et al., 2016; Chen et al., 2019).
The effects of fire and, in particular, changes in fire regime on litter decomposition processes are varied and likely depend on site and fire regime characteristics. Indeed, fire events and increases in fire frequency have been associated with both faster and slower decomposition in prior studies (e.g., Davies et al., 2013; Toberman et al., 2014; Throop et al., 2017). This variation is not suprising, because the decomposition process is influenced by a large number of interacting biological, chemical, and abiotic variables, many of which are potentially affected by fire regime (Chapin et al., 2002). For instance, eucalypt forest fire regime has been shown as a substantial source of variation in the composition and activities of the microbial and invertebrate communities that drive decomposition through their demand for C and nutrients (Springett, 1979; Brennan et al., 2009; Shen et al., 2016). Micro-organisms such as fungi and bacteria contribute to decomposition by releasing extra-cellular enzymes that hydrolyse organic molecules to release soluble, labile forms of C and nutrients which are subsequently absorbed and metabolized by the microbial biomass (Chapin et al., 2002). Litter and soil-dwelling invertebrates complement and usually enhance these processes by displacing, consuming, and fragmenting the litter and through their top-down effects on the microbial community (Hättenschwiler et al., 2005; García-Palacios et al., 2013). Although micro-organisms generally make a larger contribution to decomposition than invertebrates (Chapin et al., 2002; García-Palacios et al., 2013), prior studies have shown that the relative contributions of micro-organisms and invertebrates to decomposition in eucalypt forests can be significantly altered by fire regime (Brennan et al., 2009; Butler et al., 2019b). At the same time, by altering the structure of eucalypt forests, changes in fire regime can affect the degree to which sunlight penetrates to the litter layer, which in turn influences the temperature and moisture of the decompositional environment (e.g., Heisler et al., 2004). These abiotic factors can exert strong influence over litter decomposition rates through their effects on biological processes (Meentemeyer, 1978; Zhang et al., 2008).
However, fire regime can also shape the inherent chemical properties of litter in eucalypt forests on a community level by regulating plant species composition and on a species level by altering the relative supplies of energy and nutrients available to plants (Lewis et al., 2012; Toberman et al., 2014). Litter chemistry can influence the overall rate of litter mass loss (Prescott, 2010), but can also influence the cycling dynamics and rates of loss of specific elements during decomposition (Güsewell and Gessner, 2009; Mooshammer et al., 2012). Thus, from the perspective of ecosystem C cycling and storage, fire regime-altered litter chemistry is likely to be a particularly important driver of fire regime-altered decomposition.
There are patterns in the biogeochemical trajectory of ecosystems that enable basic prediction about how changes in fire regime might affect litter chemistry and thus decomposition processes. In particular, C and N tend to accumulate in soil over time in the absence of fire as these elements are taken from the atmosphere by plants and subsequently transferred to soil through decomposition (Attiwill and Adams, 1993; Turner et al., 2008). On the other hand, soil P availability tends to increase following fires (Butler et al., 2018) due largely to ash deposition and mineralization of organic P (Chambers and Attiwill, 1994; Certini, 2005). These effects are often subsequently paralleled in the chemistry of plant litter and organic matter in general (Lagerström et al., 2009; Hume et al., 2016). However, during fire events, losses of ecosystem C and N can occur when organic forms of these elements are converted to their gaseous forms (i.e., CO2 and NOx) during combustion (Raison et al., 1984; Urbanski et al., 2008). These effects are likely to be compounded when novel fire regimes are introduced and maintained for long periods of time (i.e., over decades; Muqaddas et al., 2015; Pellegrini et al., 2018), yet various uncertainties remain regarding the effects of specific fire regime characteristics (frequency, severity, and season of burns, duration of the regime, etc.).
Thus, we conducted a 277-day, field-based decomposition experiment with Eucalyptus pilularis leaf litter within a long-term fire regime manipulation experiment at Peachester State Forest, south-east Queensland, Australia. Prior studies have shown that soil and leaf litter at Peachester State Forest differ among the three experimental fire regimes, with no burning (NB) and 4-yearly prescribed burning (4yB) associated with markedly higher levels of C and N in soil, and of N in leaf litter, than biennial prescribed burning (2yB; Toberman et al., 2014; Muqaddas et al., 2015). We therefore expected that litter mass and element loss rates would vary significantly among the fire regimes, but that these differences would be strongest between the 2yB treatment and the NB treatment, with the 4yB treatment intermediate. More specifically, we expected that decomposition would be most rapid in the NB treatment, with correspondingly high rates of C and P loss throughout decomposition, and that rates of N loss would be highest in the 2yB treatment due to high microbial N demand in the N-depleted 2yB litter.
Materials and Methods
Study Site and Fire Regimes
We performed a field-based decomposition trial within a long-running prescribed fire experiment at Peachester State Forest (26°52′S, 152°51′E; hereafter referred to as “Peachester”), which is located near the town of Beerwah in south-east Queensland, Australia. The forest at Peachester is broadly characterized as ‘wet sclerophyll' forest dominated by Eucalyptus pilularis, with several other Myrtaceous species present in the canopy. The climate is sub-tropical, with an average annual precipitation of 1,684 mm and an average daily temperature of 23.3°C (Bureau of Meteorology, 2020). The prescribed fire experiment at Peachester consists of three fire frequency treatments, namely, burned every 2 years since 1972 (“2yB”), burned every 4 years since 1972 (“4yB”), and unburned since 1969 (“NB”). These treatments are each replicated four times, and each replicate plot has an area of ~27 × 30 m. The prescribed burns have been carried out between June and September (Australian winter to early Spring). These fires were spatially-patchy surface fires and were of low or low-to-moderate intensity by design, with energy release normally <2,500 kW m−1 (Lewis et al., 2012). We note here that this experimental design unavoidably confounds burn frequency with time since fire for comparisons of NB against the 2yB and 4yB treatments. Similarly, differences in understorey vegetation composition and time between fires for the 2yB and 4yB treatments may have led to differences in fuel load and fuel distribution, such that there could have been subtle differences in fire intensity, severity, and patchiness between the 2yB and 4yB treatments. It can be argued, however, that in our study these effects are ultimately attributable to fire frequency, which in turn influences fire intensity and severity. Nevertheless, the potentially important confounding influence of fire intensity and severity over litter decomposition dynamics should be considered when interpreting the results of our study.
The soils at Peachester are classified as Alfisols (Soil Survey Staff., 1999), equivalent to red to yellow Kandosols in the Australian soil classification, with a uniformly sandy texture until around 60 cm depth (Guinto et al., 2001). The soil is acidic to strongly acidic, with soil surface (0–10 cm) pH values ranging between 3.81 and 5.34 (mean soil pH = 4.5). Most soil chemical properties vary significantly among the fire regime treatments at Peachester. In particular, surface soil (0–10 cm) total N concentrations in the NB and 4yB treatments are around double those of the 2yB treatment (0.26 and 0.27% vs. 0.13%, respectively; Butler et al., 2019a). Total and soluble C concentrations are also substantially higher in NB and 4yB soils than in the 2yB soils (Muqaddas et al., 2015).
Decomposition Experiment
We collected samples of mixed-age E. pilularis leaf litter from each replicate plot between the 10th and the 13th of November, 2015, at which time it had been 28 months since the most recent fire in both the 2yB and 4yB treatments. We chose intact litter that had undergone minimal, if any, prior decomposition or microbial colonization, and had not been subjected to herbivory. A sub-sample of litter was taken for laboratory analyses, and the remaining litter was stored at 4°C. In the week subsequent to litter collection, we constructed 144 litter decomposition bags. These bags were 18 × 18 cm in area and made from fine-aperture (0.3 mm) nylon mesh. Each decomposition bag was individually labeled and contained ~10.0 g (dry mass equivalent) of litter. On the 20th of November, 2015, 12 decomposition bags were installed within 5 × 5 m areas within the same replicate plots from which litter samples originated (12 bags × 12 plots = 144 bags in total). There was no crossing of litter within or between treatments in our experimental design. Bags were held in place with steel pegs, covered lightly with additional litter, and left to decompose. We then retrieved three bags from each replicate plot after 24, 95, 188, and 277 days. We were unable to leave the litter bags in the field for a full year because of scheduled prescribed burns in the 2yB and 4yB treatment in September of 2016, although the scheduled burns did not eventuate due to inappropriate weather and fuel conditions.
Laboratory Analyses
The partially-decomposed leaf litter from each plot was bulked together to form one composite litter bag sample per plot per litter re-collection date. These samples were weighed fresh and then cut into pieces that were roughly 1 cm2 in area. Sub-samples of litter were oven-dried for 3 days at 65°C to calculate litter moisture content, and the remaining litter was kept “fresh” at 4°C. These oven-dried litter samples were subsequently finely-ground with a dish and puck mill before being used for measurement of litter total C, N, and P content. Litter total C and N content were measured via dry combustion (LECO Total CN Determinator, TruMac no. 830-300-400). Litter total P content was measured with molybdenum-blue spectrophotometry after the litter had been digested in nitric and perchloric acids (Jackson, 1958; Murphy and Riley, 1962).
We used the “fresh” litter samples to measure litter pH, electrical conductivity (EC), soluble and microbial biomass C, N, and P, and the potential activities of three extra-cellular enzymes: β-glucosidase (GLU), N-acetyl-β-D-glucosaminidase (also known as “chitinase;” CHN), and acid phosphatase (AP). Litter pH and EC were measured in a 1:5 litter: water solution. These variables were measured because each is a useful proxy for the properties and processes that influence or drive decomposition. Litter pH, EC, and soluble C, N, and P give insight into litter nutrient loading and C and nutrient “lability,” and can also affect microbial community composition. Microbial biomass represents an important sink for litter-derived C and nutrients and is a source of extra-cellular enzymes, while extra-cellular enzymes hydrolyse organic forms of litter C, N, and P and, thus, play a direct, mechanistic role in decomposition. All of these variables can, therefore, give useful insights into the effects of prescribed fire regime on the decomposition of eucalyptus litter.
Litter microbial biomass C (MBC), N (MBN), and P (MBP) were measured following the chloroform fumigation-extraction method (Brookes et al., 1982, 1985; Vance et al., 1987). Fumigations and extractions of litter samples occurred within 28 days of litter sampling or litter bag re-collection. In brief, soluble organic C (hereafter “soluble C”) and soluble total N (hereafter “soluble N”) were extracted from fumigated and non-fumigated litter samples using 0.5 M K2SO4 and soluble (hereafter “soluble P”) was extracted from a separate set of fumigated and non-fumigated litter samples using 0.5 M NaHCO3 (pH = 8.5). Soluble C and N concentrations in litter extracts were then measured using high-temperature catalytic oxidation with a Shimadzu TOC-VCPH/CPN analyser that included a total N unit (Shimadzu Scientific Instruments, Sydney, Australia), while soluble P concentrations in litter extracts were measured with spectrophotometry as for total litter P. Concentrations of litter microbial biomass C, N, and P were then calculated based on the difference between soluble C, N, and P concentrations in fumigated and non-fumigated litter extracts and conversion factors of 2.64 for MBC, 2.22 for MBN, and 0.4 for MBP. Hereafter, litter soluble C, N, and P refer to the concentrations of C, N, and P in the non-fumigated litter extracts. Finally, we used the p-nitrophenol spectrophotometric method to measure the potential activities of GLU, CHN, and PHP (Tabatabai and Bremner, 1972; Eivazi and Tabatabai, 1977, 1988). Enzyme assays were done within 15 days of litter bag re-collection in all cases.
Statistical Analyses
We used R software for all statistical analyses (R Core Team, 2019; version 3.5.3). We used factorial analysis of variance to evaluate the effects of fire regime treatment and litter re-collection date on litter mass loss and on litter chemical and biological properties. Most litter chemical and biological properties were analyzed across the entire period, from initially collected samples (i.e., “day 0” samples) to day 277, but mass loss and enzyme activities (absolute activities as well as activities standardized to microbial biomass C) were only measured (and thus only analyzed) for days 24 through to 277. We also used analysis of variance to evaluate the effect of fire regime on cumulative losses of C, N, and P during the 277-day decomposition period. Where required, we used Tukey's Honestly Significant Difference as a post-hoc test of treatment differences.
In addition, we used the “vegan” package (Oksanen et al., 2018) in R to carry out a Principal Component Analysis (PCA) to explore the effects fire regime treatment and litter re-collection date on all litter chemical and biological properties simultaneously. Variables included in the PCA were litter moisture, pH, EC; total, soluble, and microbial biomass C; total, soluble, and microbial biomass N, total, soluble, and microbial biomass P, and absolute potential activities of GLU, CHN, and AP. The PCA ordinations were used to visually assess the primary patterns of variation in litter properties, and these were used in conjunction with permutational analysis of variance (based on Bray-Curtis dissimilarities) to test for treatment effects. The PCA results were then used to select the three litter variables that were most strongly correlated with the first three Principal Components (PCs). We then used Pearson's correlation to evaluate the relationships between these three litter variables, litter mass loss, and cumulative element loss. This was done to give some insight into which litter properties, and therefore what sorts of processes, are likely to be coupled with, or drivers of, variation in the dynamics of C and nutrients throughout litter decomposition.
Results
Patterns of rainfall and temperature during the litter decomposition period are presented in Figure 1. The effects of fire regime on the rates of E. pilularis litter mass loss as determined by our litter bag experiment have already been described by Butler et al. (2019b), who showed that the rates of microbially-driven litter decomposition were 42.1 and 23.6% slower in the 4yB and 2yB treatments, respectively, than in the NB treatment. The results of our ANOVA of litter mass loss over the four bag re-collection dates were consistent with this (Figure 2); however, the significant interaction between fire regime and litter bag re-collection date also indicates that the effect of fire regime was particularly strong at the end of the experimental decomposition period (i.e., at day 277; Figure 2). The cumulative loss of C from litter followed a similar pattern to that of overall mass loss, with NB treatment litter losing significantly more C per day than 4yB treatment litter (2.2 vs. 1.3 mg of C lost per g of initial litter C per day; Figure 3A). However, cumulative losses of C did not differ significantly between the 2yB and NB treatment, and cumulative losses of litter N and P did not differ significantly among any of the fire regime treatments (Figures 3B,C). The effects of fire regime on the cumulative loss of specific elements were the same regardless of whether losses were calculated relative to the initial amount of the element in the litter or calculated as absolute losses (i.e., total mg of element lost over 277 days).
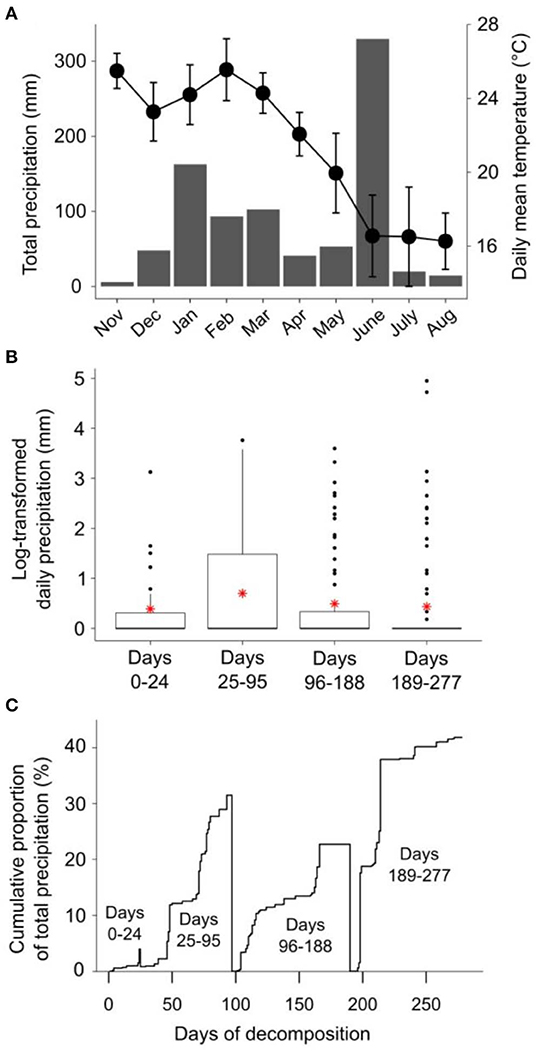
Figure 1. (A) Climodiagram showing total monthly rainfall (mm; gray bars) and average daily mean temperature (± standard deviation; black circles) during the litter decomposition period; (B) daily rainfall (log-transformed, ln[x+1]) for the period between each litter decomposition bag retreival date, with means shown as red asterisks; and (C) the cumulative proportion of the total precipitation between each litter decomposition bag retreival date, expressed on a percentage basis. Rainfall and temperature data were obtained from the Australian Bureau of Meteorology online data portal (Bureau of Meteorology, 2020); rainfall data were collected at Peachester meteorological station (26.84° S, 152.88° E), temperature data were collected at Beerburrum Forest meteorological station (26.96° S, 152.96° E).
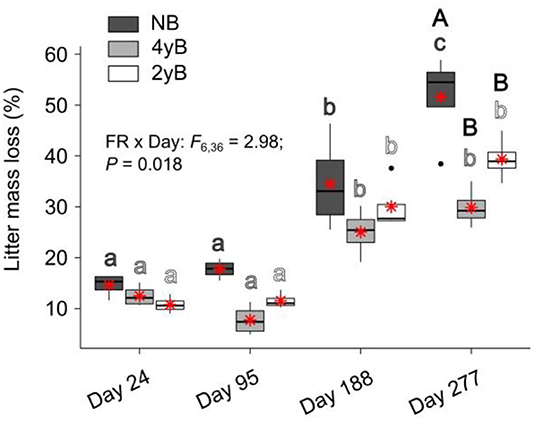
Figure 2. Percentage of Eucalyptus pilularis leaf litter mass lost throughout decomposition on four litter bag re-collection dates, compared among dates and fire regime treatments (NB = no burning; 4yB = four-yearly burning; 2yB = two-yearly burning); treatment means are displayed as red asterisks; results of two-way factorial analysis of variance and Tukey's Honestly Significant Difference post-hoc comparisons of treatment combination means shown (different lowercase letters of the same color indicate significant differences between date means for a given fire regime treatment, different uppercase letters indicate significant differences between fire regime means for a given date).
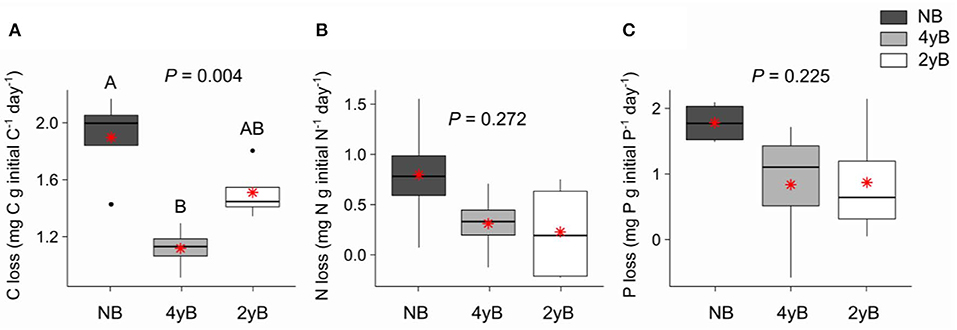
Figure 3. Rates of cumulative loss of (A) carbon (C), (B) nitrogen (N), and (C) phosphorus (P) from decomposing Eucalyptus pilularis leaf litter after 277 days of decomposition, compared among fire regime treatments (NB = no burning; 4yB = four-yearly burning; 2yB = two-yearly burning); means are displayed as red asterisks; P-values from one-way analyses of variance and Tukey's Honestly Significant Difference post-hoc comparisons of fire regime treatment means shown (different uppercase letters indicate significant differences between fire regime treatment means).
Sampling date and fire regime interacted to affect litter moisture content, with moisture tending to be higher at later stages of decomposition in the 2yB and particularly the NB treatment, but not in the 4yB treatment, in which litter moisture was stable throughout decomposition (Figure 4A). Litter pH declined throughout decomposition and was significantly higher in NB litter than in the 2yB litter (Figure 4B). Litter electrical conductivity (EC) was affected by the interaction between sampling date and fire regime; in general, EC was lower on day 277 than other days, but this effect was clearer for the NB and particularly the 2yB treatment than it was for the 4yB treatment (Figure 4C).
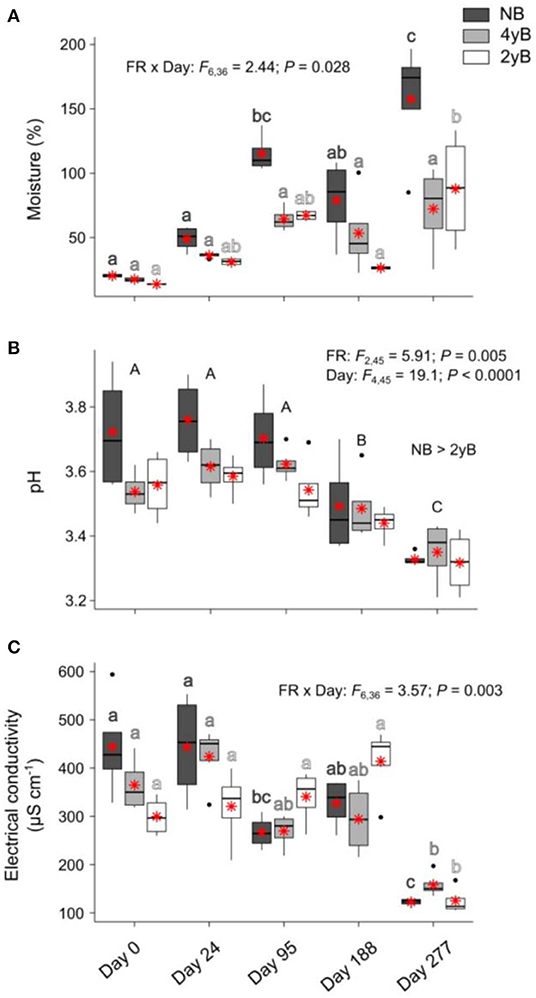
Figure 4. Values of (A) litter moisture content, (B) litter pH, and (C) litter electrical conductivity compared among dates and fire regime treatments (NB = no burning; 4yB = four-yearly burning; 2yB = two-yearly burning); means are displayed as red asterisks; results of two-way factorial analysis of variance and Tukey's Honestly Significant Difference post-hoc comparisons of treatment combination means shown (different lowercase letters of the same color indicate significant differences between date means for a given fire regime treatment, different uppercase letters indicate significant differences among date means).
Litter total C content did not differ among fire regimes at any point during our study (Figure 5A). When averaged across fire regime treatments, litter total C declined slightly yet consistently and significantly over the 277 days, from 51.9 to 50.6% (Figure 5A). Soluble C concentrations of 4yB litter did not change significantly throughout decomposition, while soluble C concentrations of 2yB and NB litter tended to be higher on day 188 than at earlier stages of decomposition (Figure 5B). Soluble C concentrations of 2yB litter spiked on day 188, at which time they were significantly higher than those of 4yB and NB litter. Litter soluble C concentrations did not vary among fire regimes on other dates (Figure 5B). Litter MBC concentrations fluctuated widely throughout decomposition, declining sharply after initial litter bag re-installation (i.e., on day 24), increasing strongly from day 24 to 188, and subsequently declining by 105% from day 188 to 277 (Figure 5C). However, fire regime did not affect litter MBC concentrations or their dynamics throughout decomposition.
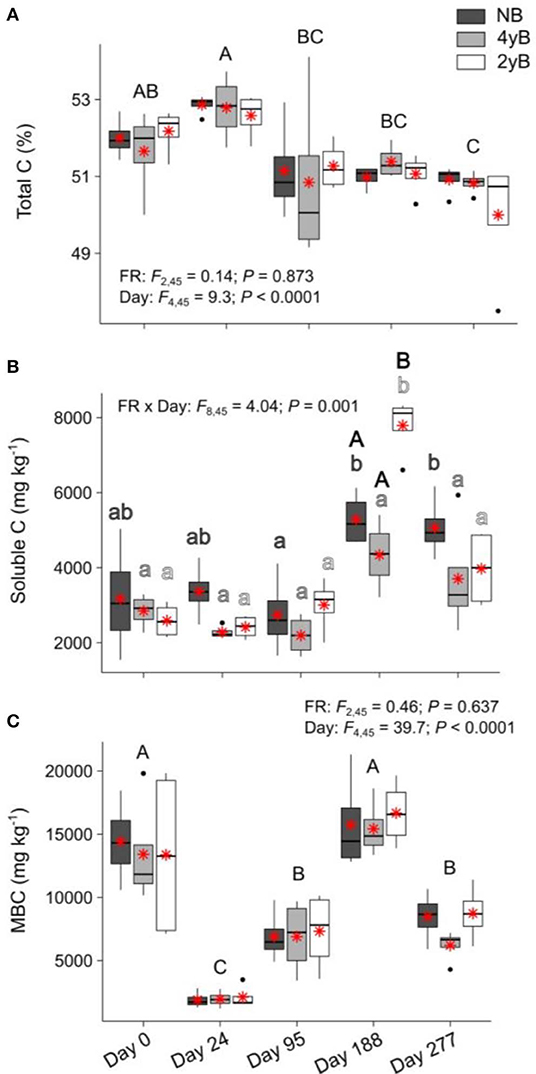
Figure 5. Concentrations of (A) total carbon (C), (B) soluble C, and (C) microbial biomass C (MBC) compared among dates and fire regime treatments (NB = no burning; 4yB = four-yearly burning; 2yB = two-yearly burning); means are displayed as red asterisks; results of two-way factorial analysis of variance and Tukey's Honestly Significant Difference post-hoc comparisons of treatment combination means shown (different lowercase letters of the same color indicate significant differences between date means for a given fire regime treatment, different uppercase letters indicate significant differences among date means for panels A and C, and between fire regime treatment means on day 188 for panel B).
Litter total N content was strongly affected by fire regime and litter bag re-collection date, but not by the interaction between these two factors (Figure 6A). Specifically, litter total N concentrations were higher in NB than 4yB and 2yB litter, and litter N concentrations tended to increase throughout decomposition in all fire regime treatments. Thus, the total N contents of 2yB and 4yB litter on days 188 and 277 were similar to those of NB litter on day 0. Soluble N concentrations did not vary among fire regime treatments, but temporal dynamics were qualitatively similar to those of soluble C (Figure 6C). The effect of fire regime on litter MBN was similar to that on litter total N, with NB litter having higher MBN concentrations than 4yB and 2yB litter (Figure 6E). Litter MBN concentrations followed a similar temporal pattern to that of MBC, with an initial decline following litter bag installation and a subsequent increase up until day 188 (Figure 6E). Litter P concentrations were strongly affected by fire regime, with NB and 2yB litter having significantly higher concentrations of total, soluble, and microbial biomass P than 4yB litter (Figures 6B,D,F). Litter total P concentrations did not change significantly throughout decomposition in any fire regime treatment (Figure 6B), but soluble P concentrations peaked on day 24 and subsequently declined (Figure 6D), while MBP concentrations tended to increase from day 24 to 277 (Figure 6F).
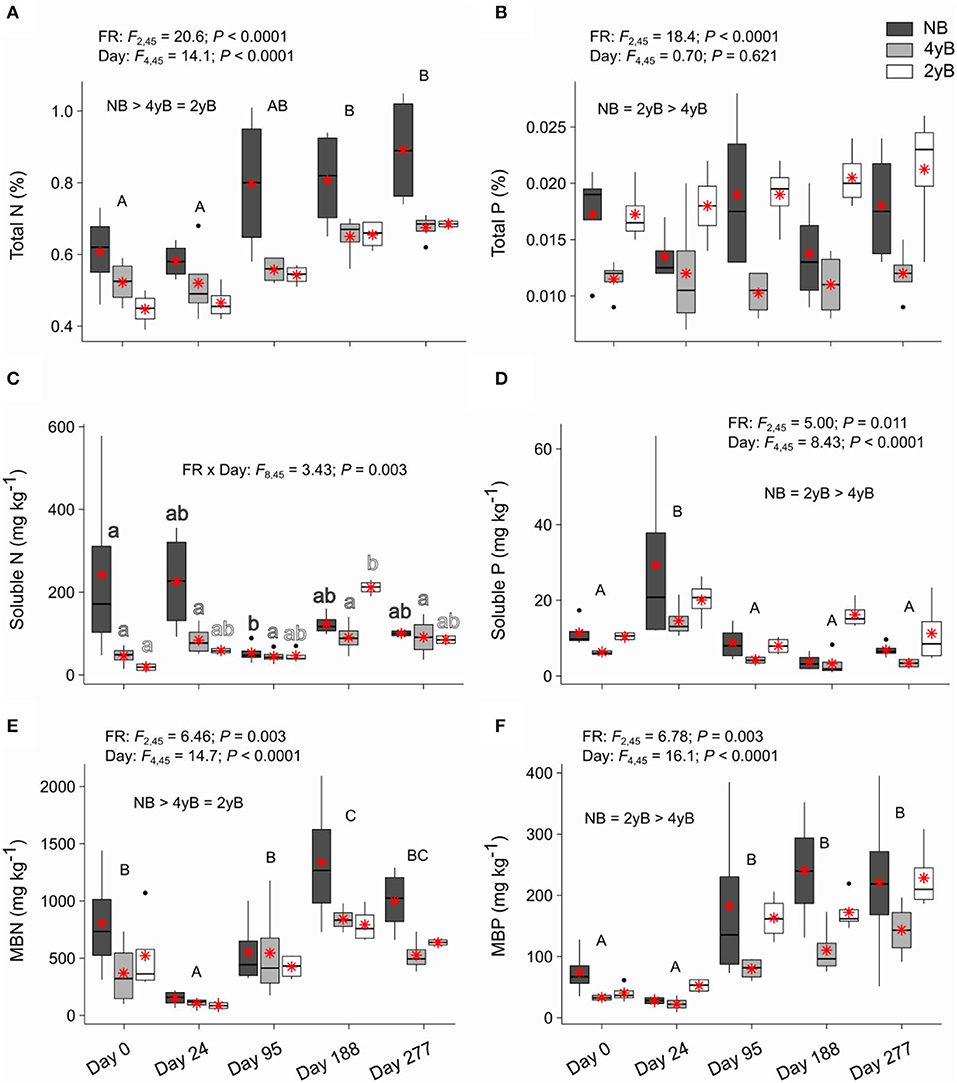
Figure 6. Concentrations of (A) total nitrogen (N), (B) total phosphorus (P), (C) soluble N, (D) soluble P, (E) microbial biomass N (MBN), and (F) microbial biomass P (MBP) compared among dates and fire regime treatments (NB = no burning; 4yB = four-yearly burning; 2yB = two-yearly burning); means are displayed as red asterisks; results of two-way factorial analysis of variance and Tukey's Honestly Significant Difference post-hoc comparisons of treatment combination means shown (different lowercase letters of the same color indicate significant differences among date means for a given fire regime treatment; different uppercase letters indicate significant differences among date means).
Fire regime had a strong, complex influence over the potential activities of litter eco-enzymes that varied based on litter bag re-collection date (Figure 7). Biennial and quadrennial burning were associated with a significant increase in litter potential GLU activity at day 277, with this effect most pronounced for the 2yB treatment (Figure 7A). Potential GLU activity also tended to be higher in NB litter than in 4yB and 2yB litter, but this effect was only statistically significant for the contrast between NB and 4yB on day 95. The potential activity of CHN followed similar patterns (Figure 7C), but 2yB litter CHN activities were also significantly higher than those of NB litter on day 277. The potential activity of PHP was significantly higher in NB litter than in 4yB and particularly 2yB litter, but these effects were restricted to days 95 and 188 of our experiment (Figure 7E). Potential PHP activities of NB litter were significantly higher on days 95 and 188 than on day 24, but on day 277 this effect was no longer significant (Figure 7E). On the other hand, there was limited temporal variation in potential PHP activities for 4yB and 2yB litter until day 277, when PHP activities increased by 137% for 4yB and 245% for 2yB, relative to day 188 levels. However, when enzyme activities are expressed relative to the litter microbial biomass C concentration, the main effect of litter bag re-collection date becomes the most important source of variation. Thus, GLU:MBC and CHN:MBC ratios declined strongly from day 24 to 188, and increased from day 188 to 277. A similar pattern was evident for AP:MBC ratios, although the decline on or around day 188 occurred more abruptly. Additionally, AP:MBC ratios were significantly lower for 2yB litter than NB litter.
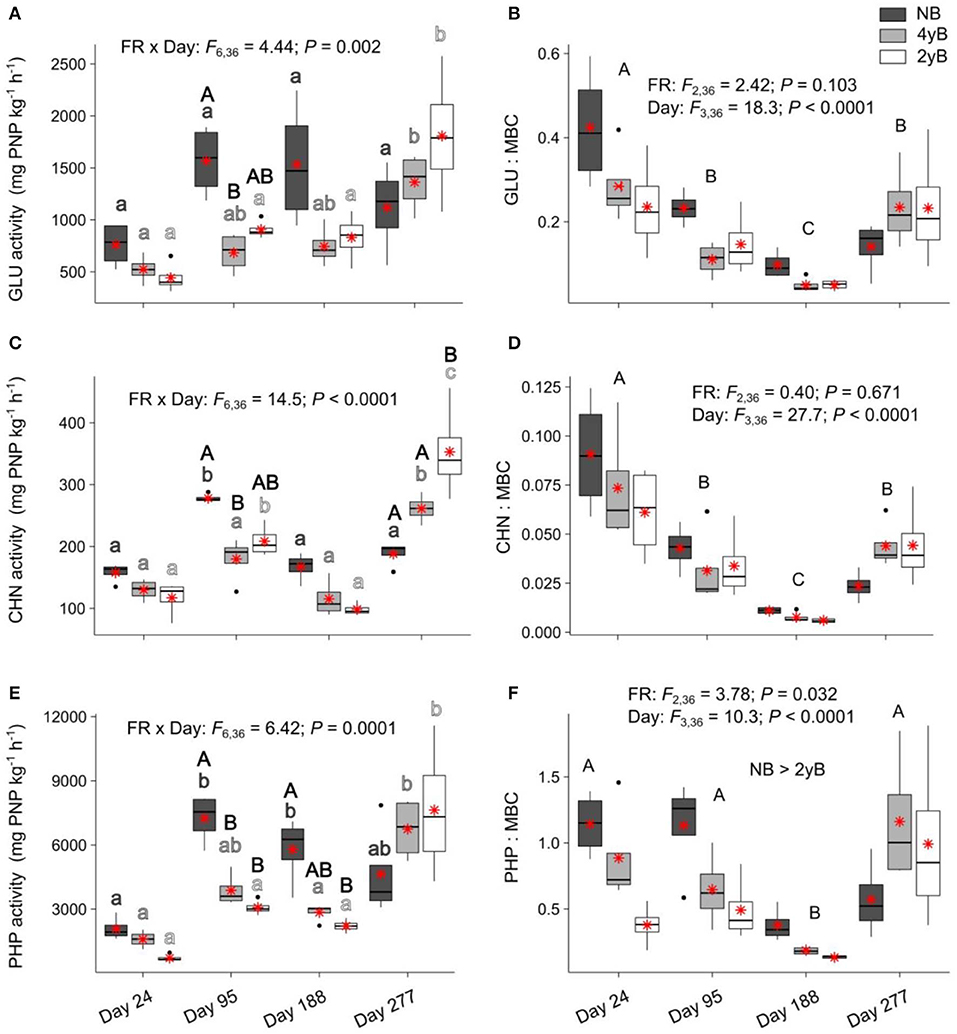
Figure 7. Potential activities of (A) β-glucosidase (GLU), (B) GLU relative to microbial biomass carbon (GLU:MBC), (C) chitinase (CHN), (D) CHN relative to MBC (CHN:MBC), (E) acid phosphatase (PHP), and (F) PHP relative to MBC (PHP:MBC) compared among dates and fire regime treatments (NB = no burning; 4yB = four-yearly burning; 2yB = two-yearly burning); means are displayed as red asterisks; results of two-way factorial analysis of variance and Tukey's Honestly Significant Difference post-hoc comparisons of treatment combination means shown (different lowercase letters of the same color indicate significant differences among date means for a given fire regime treatment, different uppercase letters indicate significant differences among fire regime means for a given date for panels A, C, and E, and among dates for panels B, D, and F).
Principal Component Analysis and PERMANOVA revealed additional effects of fire regime on the dynamics of litter C and nutrients throughout litter decomposition (Figure 8). The first three PCs represented 40.5, 18.4, and 12.0% of variation in the dataset, respectively. Principal Component 1 was most strongly correlated with MBP (r = 0.34). Principal components 2 and 3 were strongly negatively correlated with litter soluble C (r = −0.48) litter soluble P (r = −0.50). The biplot generated based on PC 1 and PC 2 indicated that fire regime and litter bag re-collection date both exerted strong influence over litter properties in general (Figure 8). However, the differences among fire regime treatments were not consistent among litter bag re-collection dates, and this is reflected by the significant interaction between fire regime treatment and litter bag re-collection date in the PERMANOVA (P = 0.005). For instance, the difference between the NB and 4yB treatment was stronger in the later stages of decomposition than on day 24, while the 2yB and NB treatments tended to be more similar at earlier stages (i.e., on days 24 and 95) than later stages (i.e., days 188 and 277) of decomposition (Figure 8). Finally, among the variables that best represented PCs 1, 2, and 3 (i.e., MBP, soluble C, and soluble P, respectively), litter MBP and soluble C were both strongly and positively correlated with litter mass loss and litter C loss (Figure 8). Litter N and P loss were not significantly correlated with any of the variables that best represented the first three PCs. However, P loss was strongly positively correlated with N loss, and N loss was strongly positively correlated with C loss. Mass loss was strongly positively correlated with C loss, but not with N or P loss.
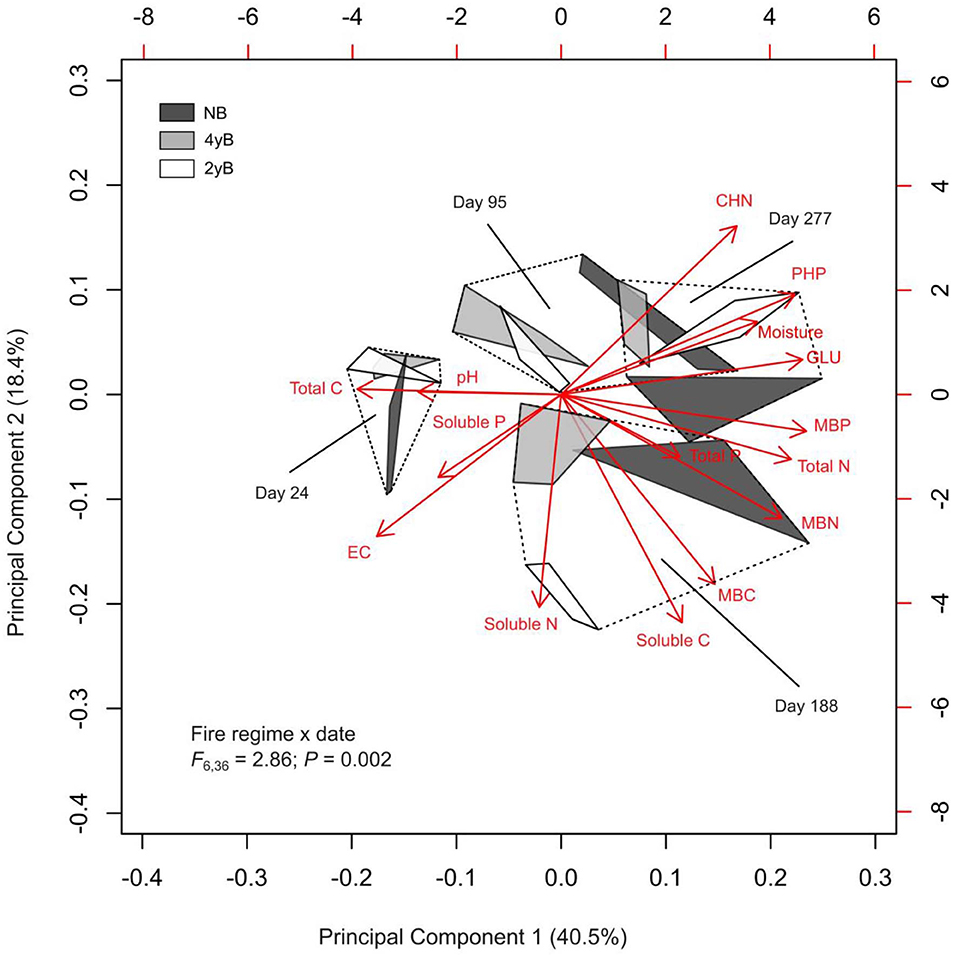
Figure 8. Biplot generated from Principal Component Analysis of chemical and biological properties of Eucalyptus pilularis leaf litter during 277-days of decomposition, with hulls based on fire regime treatment; statistics for fire regime by date interaction from factorial permutational analysis of variance provided.
Discussion
Our results indicate that long-term prescribed fire regime had a strong and statistically significant effect on many of the biological and chemical properties of decomposing E. pilularis leaf litter (i.e., Figures 5–7). The finding that fire exclusion was associated with higher levels of litter N than were quadrennial and particularly biennial burning regimes is consistent with our expectations, along with general biogeochemical theory and prior studies at Peachester (Toberman et al., 2014). This effect is likely due in part to long-term, uninterrupted accumulation of N in NB soils via fixation of atmospheric N. In 2yB soils the accumulation of soil N has presumably been impeded by repeated burns (Muqaddas et al., 2015), which can volatilise a non-trivial amount of forest floor and surface soil N (Raison et al., 1984; Urbanski et al., 2008). The consequent differences in soil N levels between the 2yB and NB treatments (Muqaddas et al., 2015) likely contributed to the strong differences in litter N content between these treatments. This is because foliar resorption is often sensitive to soil nutrient availability, with high availability of a given nutrient in soil theoretically causing plants to dedicate less energy to resorption of that nutrient (Wright and Westoby, 2003; Rejmánková, 2005).
However, prior studies at Peachester also show that soil N levels in the 4yB treatment do not differ from those of the NB treatment (Muqaddas et al., 2015; Butler et al., 2019a), so the effect of soil N levels on foliar N resorption does not fully explain the patterns of litter N evident in our study. The same is likely true for P, given that 4yB litter was depleted of both P and N relative to NB and 2yB litter, but 4yB soils are not similarly depleted of P (e.g., Butler et al., 2019a). Given that these effects were roughly consistent among all litter bag re-collection dates, it seems likely that they are due to E. pilularis resorption processes rather than to post-abscission and decomposition processes. In this case, our data suggest that E. pilularis trees in the 4yB treatment had higher N and P resorption proficiencies (Killingbeck, 1996) than trees in the 2yB and NB treatments. In other words, 4yB E. pilularis trees were using N and P more conservatively than their 2yB and NB counterparts. This result is somewhat surprising, as we expected that properties of decomposing litter would contrast most strongly between NB and 2yB treatments, with the 4yB litter somewhere in between. The reason for this result is also not obvious; however, because the 2yB and 4yB treatments were both burned 28 months prior to our initial litter sample collection, we can attribute the effect to fire frequency specifically, rather than fire regime more generally. We note that this could include the effects of fire frequency on fire intensity and severity as mediated by the 2-years difference in the fuel accumulation period between 2yB and 4yB. Using a meta-analysis of long-term fire frequency manipulation experiments, Pellegrini et al. (2018) showed that increases in fire frequency generally have clearly negative effects on soil N compared to fire exclusion, and have positive effects on soil P concentrations during the first 10 years that disappear over longer time-scales (i.e., > 30 years of increased fire frequency). Thus, while our results complement the findings of Pellegrini et al. (2018) by showing that the nutrient depletion associated with increased fire frequency can occur in litter as well as in soil, they also complicate matters by showing how different levels of fire frequency increase can have markedly and qualitatively different effects on litter chemistry.
The strong differences in litter chemical properties among fire regimes coincided with faster overall decomposition of NB litter compared to 2yB and particularly the 4yB litter (Figure 2). This difference in decomposition rates was paralleled by rates of overall C loss from litter that were 20 and 40% lower in the 2yB and 4yB treatments, respectively, than those in the NB treatment. This result was partially consistent with our expectations, although we expected differences in decomposition rate to be greatest between 2yB and NB, not between 4yB and NB. At least some of this effect can likely be attributed to the low P concentration of 4yB litter, which has suppressed microbial C demand in favor of P acquisition (Butler et al., 2019b). An implication of this finding is that the rate of accumulation of soil C could be significantly lower in frequently burned eucalypt forest due to constrained decomposition rates. This is supported by prior studies at Peachester, which have shown that rates of leaf litter input differ between the NB and frequently burned treatments by only around 13% (2yB) and 7.4% (4yB; Muqaddas and Lewis, 2020), such that differences in leaf litter inputs are unlikely to compensate for differences in decomposition rates. Thus, the effects observed in our study may contribute to a positive feedback between climate change and fire regime, wherein the increased prescribed fire frequencies associated with heightened wildfire risk lead to lower C storage and C sequestration rates in eucalypt forests. However, the nature of such a feedback strongly depends on the fate of the lost litter C. For instance, if litter C is being lost primarily in the form of respired CO2, then high-frequency fire might serve to constrain CO2 emissions from decomposing litter. On the other hand, if a substantial proportion of litter C is being transferred and sequestered in soil, our results suggest that high frequency prescribed fire can both drive losses of soil C through volatilisation and reduce the rate at which soil C stocks recover from such losses in the post-fire environment. This would be complicated further if the fate of decomposing litter C is dependent upon fire regime. Further studies will be required to trace and determine the fate of litter C during decomposition in fire-affected ecosystems.
Although patterns of mass and C loss from decomposing litter were reasonably consistent with our expectations, the depletion of litter nutrients in the 2yB and 4yB treatments did not exert correspondingly strong influence over the rate at which nutrients are lost from decomposing E. pilularis litter (Figure 3). We had initially expected that the low N content of 2yB litter would lead to high rates of N loss from 2yB litter via the activities of N-deprived decomposer organisms. Under nutrient depleted conditions, it seems plausible that litter-associated micro-organisms tend to retain or immobilize nutrients rather than release them to the environment (e.g., Qui et al., 2008). Measurements of total litter N and P would not necessarily reflect such re-distributions of N and P within the litter-microbial complex. The tendency for litter microbial biomass N and P concentrations to increase throughout decomposition seems consistent with N and P redistribution from litter to the litter microbial biomass as the litter decomposes (Figures 6E,F), as does the related effect of the positive coupling between mass (and C) loss and microbial biomass P (Figure 9). Prior study indicates that microbial biomass associated with low P soils can serve as a sink for mobile soil P in post-fire environments (Huang et al., 2013), and Butler et al. (2019b) found that a significant portion of high-frequency fire's effect on litter mass loss was caused by microbial nutrient stress as evidenced by eco-enzymatic stoichiometry (Sinsabaugh and Follstad Shah, 2012). If nutrient-deprived or nutrient-limited micro-organisms in frequently burned eucalypt forests immobilize a large proportion of litter-bound nutrients before those nutrients reach the soil, the nutritional status and productivity of the associated vegetation may be affected as a consequence, although this is speculative.
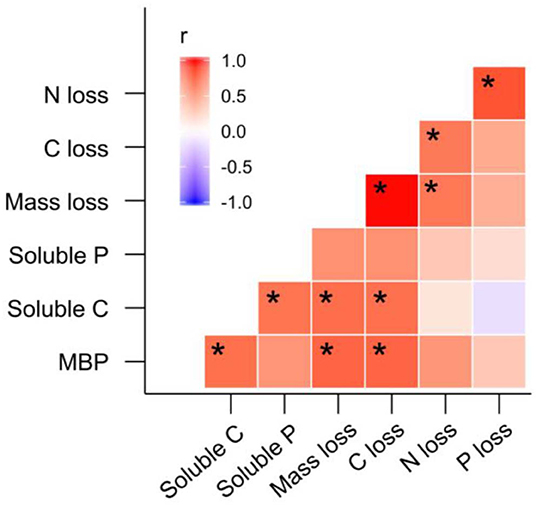
Figure 9. Matrix of Pearson's correlation coefficients for correlations among litter microbial biomass phosphorus (MBP), litter soluble carbon (C), litter soluble phosphorus (P), litter mass loss, C loss, N loss, and P loss; significant correlations are denoted “*”.
Long-term fire regime has clearly had substantial effects on the decomposition and biological and chemical properties of E. pilularis leaf litter; however, in most cases these effects were superimposed over or dependent upon the effect of time (i.e., litter bag re-collection date). Indeed, the effects of time on decomposition tended to be much greater in magnitude than the effects of fire regime. This finding is similar to those of Liu et al. (2017), who argued that variation in soil microbial community characteristics at Peachester was dominated by seasonal factors, with fire regime playing a secondary albeit significant role. This is likely the case for the leaf litter properties measured in our study, as there was substantial variation in rainfall and temperature throughout the decomposition period and among the four litter bag re-collection dates (Figure 1). Initial differences in the chemical properties among different litters often become smaller as decomposition progresses (e.g., Preston et al., 2009; Wickings et al., 2012). As a consequence, the importance of abiotic factors such as temperature and rainfall as drivers of local-scale variation in decomposition rates are sometimes predicted to increase throughout decomposition, while the importance of litter chemistry is predicted to decline (e.g., García-Palacios et al., 2016). Although our 9-month study was short relative to these processes, which often manifest over multiple years (Melillo et al., 1989), our results suggest that the chemical and biological properties of NB, 4yB, and 2yB litter were not converging after 277 days, at which point 40% of initial litter mass had been lost, on average (Figure 2). On the contrary, temporal dynamics were complex and litter properties tended to be more similar among fire regime treatments on day 24 than at later litter bag re-collection dates (e.g., Figure 8). This suggests that the relative importance of litter properties vs. abiotic factors probably did not shift substantially during our experiment. Given that changes in fire regime can lead to significant changes in microclimate conditions, the effects of seasonality on litter decomposition processes are likely to interact with fire regime in ways that we were unable to detect in our experiment. For example, potential differences in evaporation rates due to differences in vegetation structure among the treatments might affect the sensitivity of decomposition to temporal rainfall trends.
From this perspective, it may also be relevant that the potential activities of GLU, CHN, and PHP (absolute and relative to MBC) tended to be highest in the NB treatment, but that this effect was effectively reversed on day 277 (Figure 7). This effect seems due more to increases in enzyme activities for 4yB and 2yB litter at day 277 than to a decrease in enzyme activities for NB litter. The reason for this is not clear from our experiment, but we suggest that the substantial increase in total rainfall during the period between days 188 and 277 might have allowed 2yB and 4yB micro-organisms to “overcome” moisture deficits that may have existed at earlier stages of decomposition. Indeed, 2yB and 4yB litter moisture content on day 277 tended to be higher than on prior litter bag re-collection dates (Figure 4A). Relief from moisture limitation might have prompted a rapid spike in enzyme activity. In this sense, the normal trajectory of decomposition was perhaps inhibited by the dry environmental conditions in the 2yB and 4yB treatments. This reinforces the view that nutritional or chemical drivers of decomposition responses to fire regime are superimposed over and secondary to abiotic drivers. Such a view is consistent with the broader hierarchy of decomposition drivers, wherein climatic factors override litter properties at global or continental scales, while litter properties are dominant at local scales (Lavelle et al., 1993; Chapin et al., 2002). Changes in fire regime introduce a complex and likely interactive gradient of both microclimatic and litter chemical conditions. Our study was limited in its ability to disentangle the interplay among the chemical, biological, and abiotic drivers, because we were focused primarily on the general effect of fire regime on the rates of mass and element loss from decomposing litter and the associated variation in litter properties over time. Given the clear consequences of increases in fire frequency for decomposition shown by our study, and the potential feedbacks to climate change associated with these consequences, further studies are warranted.
Data Availability Statement
The data associated with this study will be made available upon request to the corresponding author.
Author Contributions
OB and CC designed the study, with input from TL. OB carried out the experiment and laboratory analyses with support from MR and CC. OB carried out statistical analyses and wrote the first draft of the manuscript. All authors contributed to interpretation of results at various stages and contributed to subsequent drafts.
Funding
This research project was supported by a grant of Australian Research Council Future Fellowship project (FT0990547). OB is a recipient of the SE Queensland Fire & Biodiversity Consortium scholarship.
Conflict of Interest
The authors declare that the research was conducted in the absence of any commercial or financial relationships that could be construed as a potential conflict of interest.
Acknowledgments
The authors would like to acknowledge the support of the Griffith University Environmental Biogeochemistry Research Laboratory. The authors respectfully acknowledge the traditional owners of the lands on which this project was conducted, at Peachester State Forest the Jinibara People, and at Griffith University's Nathan Campus the Jagera and Turrbal Peoples.
References
Abatzoglou, J. T., and Williams, A. P. (2016). Impact of anthropogenic climate change on wildfire across western US forests. Proc. Natl. Acad. Sci. U. S. A. 113, 11770–11775. doi: 10.1073/pnas.1607171113
Alonso-Canas, I., and Chuvieco, E. (2015). Global burned area mapping from ENVISAT-MERIS and MODIS active fire data. Remote Sens. Environ. 163, 140–152. doi: 10.1016/j.rse.2015.03.011
Attiwill, P. M., and Adams, M. A. (1993). Tansley Review No. 50: Nutrient cycling in forests. New Phytol. 124, 561–582. doi: 10.1111/j.1469-8137.1993.tb03847.x
Bennett, L. T., Aponte, C., Tolhurst, K. G., Löw, M., and Baker, T. G. (2013). Decreases in standing tree-based carbon stocks associated with repeated prescribed fires in a temperate mixed-species eucalypt forest. For. Ecol. Manage. 306, 243–255. doi: 10.1016/j.foreco.2013.06.036
Birk, E. M., and Bridges, R. G. (1989). Recurrent fires and fuel accumulation in even-aged Blackbutt (Eucalyptus pilularis) forests. For. Ecol. Manage. 29, 59–79. doi: 10.1016/0378-1127(89)90056-X
Boer, M. M., Bowman, D. M. J. S., Murphy, B. P., Cary, G. J., Cochrane, M. A., Fensham, R. J., et al. (2016). Future changes in climatic water balance determine potential for transformational shifts in Australian fire regimes. Environ. Res. Lett. 11. doi: 10.1088/1748-9326/11/6/065002
Bowman, D. M. J. S., Balch, J. K., Artaxo, P., Bond, W. J., Carlson, J. M., Cochrane, M. A., et al. (2009). Fire in the earth system. Science 324, 481–484. doi: 10.1126/science.1163886
Bradstock, R. A., Cary, G. J., Davies, I., Lindenmayer, D. B., Price, O. F., and Williams, R. J. (2012). Wildfires, fuel treatment and risk mitigation in Australian eucalypt forests: insights from landscape-scale simulation. J. Environ. Manage. 105, 66–75. doi: 10.1016/j.jenvman.2012.03.050
Bradstock, R. A., Hammill, K. A., Collins, L., and Price, O. (2010). Effects of weather, fuel and terrain on fire severity in topographically diverse landscapes of south-eastern Australia. Landsc. Ecol. 25, 607–619. doi: 10.1007/s10980-009-9443-8
Brennan, K. E. C., Christie, F. J., and York, A. (2009). Global climate change and litter decomposition: more frequent fire slows decomposition and increases the functional importance of invertebrates. Glob. Change Biol. 15, 2958–2971. doi: 10.1111/j.1365-2486.2009.02011.x
Brookes, P. C., Landman, A., Pruden, G., and Jenkinson, D. S. (1985). Chloroform fumigation and the release of soil nitrogen: a rapid direct extraction method to measure microbial biomass in soil. Soil Biol. Biochem. 17, 837–842. doi: 10.1016/0038-0717(85)90144-0
Brookes, P. C., Powlson, D. S., and Jenkinson, D. S. (1982). Measurement of microbial biomass phosphorus in soil. Soil Biol. Biochem. 14, 319–329. doi: 10.1016/0038-0717(82)90001-3
Bureau of Meteorology (2020). Climate Data Online. Available online at: http://www.bom.gov.au/climate/data/ (accessed January 28, 2020).
Butler, O. M., Elser, J. J., Lewis, T., Mackey, B., and Chen, C. (2018). The phosphorus-rich signature of fire in the soil–plant system: a global meta-analysis. Ecol. Lett. 21, 335–344. doi: 10.1111/ele.12896
Butler, O. M., Lewis, T., Rashti, M. R., and Chen, C. (2019a). Energetic efficiency and temperature sensitivity of soil heterotrophic respiration vary with decadal-scale fire history in a wet sclerophyll forest. Soil Biol. Biochem. 134, 62–71. doi: 10.1016/j.soilbio.2019.03.022
Butler, O. M., Lewis, T., Rezaei Rashti, M., Maunsell, S. C., Elser, J. J., and Chen, C. (2019b). The stoichiometric legacy of fire regime regulates the roles of micro-organisms and invertebrates in decomposition. Ecology 100, 1–12. doi: 10.1002/ecy.2732
Carney, K. M., Hungate, B. A., Drake, B. G., and Megonigal, J. P. (2007). Altered soil microbial community at elevated CO2 leads to loss of soil carbon. Proc. Natl. Acad. Sci. U. S. A. 104, 4990–4995. doi: 10.1073/pnas.0610045104
Certini, G. (2005). Effects of fire on properties of forest soils: a review. Oecologia 143, 1–10. doi: 10.1007/s00442-004-1788-8
Chambers, D. P., and Attiwill, P. M. (1994). The ash-bed effect in Eucalyptus regnans forest: Chemical, physical, and microbiological changes in soil after heating or partial sterilisation. Aust. J. Bot. 42, 739–749. doi: 10.1071/BT9940739
Chapin, F. S., Matson, P. A., and Vitousek, P. M. (2002). Principles of Terrestrial Ecosystem Ecology. New York, NY: Springer-Verlag. doi: 10.1007/b97397
Chen, Y., Cao, J., Zhou, L., Li, F., and Fu, S. (2019). Effects of prescribed burning on carbon accumulation in two paired vegetation sites in subtropical china. For. Ecosyst. 6:26. doi: 10.1186/s40663-019-0185-z
Conroy, J. P., Milham, P. J., and Barlow, E. W. R. (1992). Effect of nitrogen and phosphorus availability on the growth response of Eucalyptus grandis to high CO2. Plant. Cell Environ. 15, 843–847. doi: 10.1111/j.1365-3040.1992.tb02152.x
Davies, A. B., van Rensburg, B. J., Eggleton, P., and Parr, C. L. (2013). Interactive effects of fire, rainfall, and litter quality on decomposition in savannas: frequent fire leads to contrasting effects. Ecosystems 16, 866–880. doi: 10.1007/s10021-013-9657-0
Dijkstra, F. A., Jenkins, M., de Rémy de Courcelles, V., Keitel, C., Barbour, M. M., Kayler, Z. E., et al. (2017). Enhanced decomposition and nitrogen mineralization sustain rapid growth of Eucalyptus regnans after wildfire. J. Ecol. 105, 229–236. doi: 10.1111/1365-2745.12663
Eivazi, F., and Tabatabai, M. A. (1977). Phosphatases in soils. Soil Biol. Biochem. 9, 167–172. doi: 10.1016/0038-0717(77)90070-0
Eivazi, F., and Tabatabai, M. A. (1988). Glucosidases and galactosidases in soils. Soil Biol. Biochem. 20, 601–606. doi: 10.1016/0038-0717(88)90141-1
Fioretto, A., Papa, S., and Pellegrino, A. (2005). Effects of fire on soil respiration, ATP content and enzyme activities in Mediterranean maquis. Appl. Veg. Sci. 8, 13–20. doi: 10.1111/j.1654-109X.2005.tb00624.x
García-Palacios, P., Maestre, F. T., Kattge, J., and Wall, D. H. (2013). Climate and litter quality differently modulate the effects of soil fauna on litter decomposition across biomes. Ecol. Lett. 16, 1045–1053. doi: 10.1111/ele.12137
García-Palacios, P., Shaw, E. A., Wall, D. H., and Hättenschwiler, S. (2016). Temporal dynamics of biotic and abiotic drivers of litter decomposition. Ecol. Lett. 19, 554–563. doi: 10.1111/ele.12590
Gill, A. M., and Zylstra, P. (2005). Flammability of Australian forests. Aust. For. 68, 87–93. doi: 10.1080/00049158.2005.10674951
Guinto, D. F., Xu, Z. H., House, A. P. N., and Saffigna, P. G. (2001). Soil chemical properties and forest floor nutrients under repeated prescribed burning in eucalypt forests of south-east Queensland, Australia. New Zeal. J. For. Sci. 31, 170–187.
Güsewell, S., and Gessner, M. O. (2009). N:P ratios influence litter decomposition and colonization by fungi and bacteria in microcosms. Funct. Ecol. 23, 211–219. doi: 10.1111/j.1365-2435.2008.01478.x
Hättenschwiler, S., Tiunov, A. V., and Scheu, S. (2005). Biodiversity and litter decomposition in terrestrial ecosystems. Annu. Rev. Ecol. Evol. Syst. 36, 191–218. doi: 10.1146/annurev.ecolsys.36.112904.151932
Heisler, J. L., Briggs, J. M., Knapp, A. K., Blair, J. M., and Seery, A. (2004). Direct and indirect effects of fire on shrub density and aboveground productivity in a mesic grassland. Ecology 85, 2245–2257. doi: 10.1890/03-0574
Holden, S. R., and Treseder, K. K. (2013). A meta-analysis of soil microbial biomass responses to forest disturbances. Front. Microbiol. 4, 1–17. doi: 10.3389/fmicb.2013.00163
Huang, W., Xu, Z., Chen, C., Zhou, G., Liu, J., Abdullah, K. M., et al. (2013). Short-term effects of prescribed burning on phosphorus availability in a suburban native forest of subtropical Australia. J. Soils Sediments 13, 869–876. doi: 10.1007/s11368-013-0660-z
Hume, A., Chen, H. Y. H., Taylor, A. R., Kayahara, G. J., and Man, R. (2016). Soil C : N : P dynamics during secondary succession following fire in the boreal forest of central Canada. For. Ecol. Manage. 369, 1–9. doi: 10.1016/j.foreco.2016.03.033
Killingbeck, K. T. (1996). Nutrients in senesced leaves: keys to the search for potential resorption and resorption proficiency. Ecology 77, 1716–1727. doi: 10.2307/2265777
Krishnaraj, S. J., Baker, T. G., Polglase, P. J., Volkova, L., and Weston, C. J. (2016). Prescribed fire increases pyrogenic carbon in litter and surface soil in lowland Eucalyptus forests of south-eastern Australia. For. Ecol. Manage. 366, 98–105. doi: 10.1016/j.foreco.2016.01.038
Lagerström, A., Esberg, C., Wardle, D. A., and Giesler, R. (2009). Soil phosphorus and microbial response to a long-term wildfire chronosequence in northern Sweden. Biogeochemistry 95, 199–213. doi: 10.1007/s10533-009-9331-y
Lavelle, P., Blanchart, E., Martin, A., Martin, S., and Schaefer, R. (1993). A hierarchical model for decomposition in terrestrial ecosystems: Application to soils of the humid tropics. Biotropica 25, 130–150. doi: 10.2307/2389178
Lewis, T., Reif, M., Prendergast, E., and Tran, C. (2012). The effect of long-term repeated burning and fire exclusion on above- and below-ground Blackbutt (Eucalyptus pilularis) forest vegetation assemblages. Austral Ecol. 37, 767–778. doi: 10.1111/j.1442-9993.2011.02337.x
Liu, X., Chen, C. R., Hughes, J. M., Wang, W. J., and Lewis, T. (2017). Temporal changes rather than long-term repeated burning predominately control the shift in the abundance of soil denitrifying community in an Australian sclerophyll forest. Microb. Ecol. 73, 177–187. doi: 10.1007/s00248-016-0894-6
Liu, Y., Stanturf, J., and Goodrick, S. (2010). Trends in global wildfire potential in a changing climate. For. Ecol. Manage. 259, 685–697. doi: 10.1016/j.foreco.2009.09.002
McCaw, W. L. (2013). Managing forest fuels using prescribed fire—a perspective from southern Australia. For. Ecol. Manage. 294, 217–224. doi: 10.1016/j.foreco.2012.09.012
McGuire, D. A., Melillo, J. M., and Joyce, L. A. (1995). The role of nitrogen in the response of forest net primary production to elevated atmostpheric carbon dioxide. Annu. Rev. Ecol. Syst. 26, 473–503. doi: 10.1146/annurev.es.26.110195.002353
Meentemeyer, V. (1978). Macroclimate and lignin control of litter decomposition rates. Ecology 59, 465–472. doi: 10.2307/1936576
Melillo, J. M., Aber, J. D., Linkins, A. E., Ricca, A., Melillo, J. M., Aber, J. D., et al. (1989). Carbon and nitrogen dynamics along the decay continuum: plant litter to soil organic matter. Plant Soil 115, 189–198. doi: 10.1007/BF02202587
Mooshammer, M., Wanek, W., Schnecker, J., Wild, B., Leitner, S., Hofhansl, F., et al. (2012). Stoichiometric controls of nitrogen and phosphorus cycling in decomposing beech leaf litter. Ecology 93, 770–782. doi: 10.1890/11-0721.1
Muqaddas, B., and Lewis, T. (2020). Temporal variations in litterfall biomass input and nutrient return under long-term prescribed burning in a wet sclerophyll forest, Queensland, Australia. Sci. Total Environ. 706:136035. doi: 10.1016/j.scitotenv.2019.136035
Muqaddas, B., Zhou, X., Lewis, T., Wild, C., and Chen, C. (2015). Long-term frequent prescribed fire decreases surface soil carbon and nitrogen pools in a wet sclerophyll forest of Southeast Queensland, Australia. Sci. Total Environ. 536, 39–47. doi: 10.1016/j.scitotenv.2015.07.023
Murphy, B. P., Russell-Smith, J., and Prior, L. D. (2010). Frequent fires reduce tree growth in northern Australian savannas: implications for tree demography and carbon sequestration. Glob. Chang. Biol. 16, 331–343. doi: 10.1111/j.1365-2486.2009.01933.x
Murphy, J., and Riley, J. P. (1962). A modified single solution method for the determination of phosphate in natural waters. Anal. Chim. Acta 27, 31–36. doi: 10.1016/S0003-2670(00)88444-5
Oksanen, A. J., Blanchet, F. G., Kindt, R., Legendre, P., Minchin, P. R., Hara, R. B. O., et al. (2018). Package ‘vegan' version 2.5-2. Commun. Ecol. Package 10, 631–637. Available online at: https://CRAN.R-project.org/package=vegan
Pellegrini, A. F. A., Ahlström, A., Hobbie, S. E., Reich, P. B., Nieradzik, L. P., Staver, A. C., et al. (2018). Fire frequency drives decadal changes in soil carbon and nitrogen and ecosystem productivity. Nature 553, 194–198. doi: 10.1038/nature24668
Prescott, C. E. (2010). Litter decomposition: what controls it and how can we alter it to sequester more carbon in forest soils? Biogeochemistry 101, 133–149. doi: 10.1007/s10533-010-9439-0
Preston, C. M., Nault, J. R., Trofymow, J. A., and Smyth, C. (2009). Chemical changes during 6 years of decomposition of 11 litters in some Canadian forest sites. Part 1. Elemental composition, tannins, phenolics, and proximate fractions. Ecosystems 12, 1053–1077. doi: 10.1007/s10021-009-9266-0
Qui, S., McComb, A. J., and Bell, R. W. (2008). Ratios of C, N and P in soil water direct microbial immobilisation-mineralisation and N availability in nutrient amended sandy soils in southwestern Australia. Agr. Ecosyst. Environ. 127, 93–99. doi: 10.1016/j.agee.2008.03.002
Raison, R. J., Khanna, P. K., and Woods, P. V. (1984). Mechanisms of element transfer to the atmosphere during vegetation fires. Can. J. For. Res. 15, 1–5. doi: 10.1139/x85-022
Rejmánková, E. (2005). Nutrient resorption in wetland macrophytes: Comparison across several regions of different nutrient status. New Phytol. 167, 471–482. doi: 10.1111/j.1469-8137.2005.01449.x
Shen, J. P., Chen, C. R., and Lewis, T. (2016). Long term repeated fire disturbance alters soil bacterial diversity but not the abundance in an Australian wet sclerophyll forest. Sci. Rep. 619639. doi: 10.1038/srep19639
Sinsabaugh, R. L., and Follstad Shah, J. J. (2012). Ecoenzymatic stoichiometry and ecological theory. Annu. Rev. Ecol. Evol. Syst. 43, 313–343. doi: 10.1146/annurev-ecolsys-071112-124414
Soil Survey Staff. (1999). Soil Taxonomy: A Basic System of Soil Classification for Making and Interpreting Soil Surveys. Washington DC: USDA, Soil Conservation Service.
Springett, J. A. (1979). The effects of a single hot summer fire on soil fauna and on litter decomposition in jarrah (Eucalyptus marginata) forest in Western Australia. Aust. J. Ecol. 4, 279–291. doi: 10.1111/j.1442-9993.1979.tb01219.x
Tabatabai, M. A., and Bremner, J. M. (1972). Assay of urease activity in soils. Soil Biol. Biochem. 4, 479–487. doi: 10.1016/0038-0717(72)90064-8
Throop, H. L., Salem, M. A., and Whitford, W. G. (2017). Fire enhances litter decomposition and reduces vegetation cover influences on decomposition in a dry woodland. Plant Ecol. 218, 799–811. doi: 10.1007/s11258-017-0730-1
Toberman, H., Chen, C., Lewis, T., and Elser, J. J. (2014). High-frequency fire alters C : N : P stoichiometry in forest litter. Glob. Chang. Biol. 20, 2321–2331. doi: 10.1111/gcb.12432
Turner, J., Lambert, M., Jurskis, V., and Bi, H. (2008). Long term accumulation of nitrogen in soils of dry mixed eucalypt forest in the absence of fire. For. Ecol. Manage. 256, 1133–1142. doi: 10.1016/j.foreco.2008.06.021
Urbanski, S. P., Hao, W. M., and Baker, S. (2008). Chemical composition of wildland fire emissions, Dev. Environ. Sci. 8, 79–107. doi: 10.1016/S1474-8177(08)00004-1
Vance, E. D., Brookes, P. C., and Jenkinson, D. S. (1987). An extraction method for measuring soil microbial biomass C. Soil Biol. Biochem. 19, 703–707. doi: 10.1016/0038-0717(87)90052-6
Wickings, K., Grandy, A. S., Reed, S. C., and Cleveland, C. C. (2012). The origin of litter chemical complexity during decomposition. Ecol. Lett. 15, 1180–1188. doi: 10.1111/j.1461-0248.2012.01837.x
Wright, I. J., and Westoby, M. (2003). Nutrient concentration, resorption, and lifespan: leaf traits of Australian sclerophyll species. Funct. Ecol. 17, 10–19. doi: 10.1046/j.1365-2435.2003.00694.x
Zhang, D., Hui, D., Luo, Y., and Zhou, G. (2008). Rates of litter decomposition in terrestrial ecosystems: global patterns and controlling factors. J. Plant Ecol. 1, 85–93. doi: 10.1093/jpe/rtn002
Keywords: prescribed burning, decomposition, nitrogen, phosphorus, eco-enzymes
Citation: Butler OM, Lewis T, Rezaei Rashti M and Chen C (2020) Long-Term Fire Regime Modifies Carbon and Nutrient Dynamics in Decomposing Eucalyptus pilularis Leaf Litter. Front. For. Glob. Change 3:22. doi: 10.3389/ffgc.2020.00022
Received: 07 November 2019; Accepted: 24 February 2020;
Published: 17 March 2020.
Edited by:
Kirsten Thonicke, Potsdam-Institut für Klimafolgenforschung (PIK), GermanyReviewed by:
Daniel Moya, University of Castilla La Mancha, SpainDavid Bowman, University of Tasmania, Australia
Copyright © 2020 Butler, Lewis, Rezaei Rashti and Chen. This is an open-access article distributed under the terms of the Creative Commons Attribution License (CC BY). The use, distribution or reproduction in other forums is permitted, provided the original author(s) and the copyright owner(s) are credited and that the original publication in this journal is cited, in accordance with accepted academic practice. No use, distribution or reproduction is permitted which does not comply with these terms.
*Correspondence: Chengrong Chen, Yy5jaGVuQGdyaWZmaXRoLmVkdS5hdQ==