Responses of Plant Biomass in the Brazilian Savanna to Frequent Fires
- 1Departmento de Ecologia, Universidade de Brasília, Brasília, Brazil
- 2Centro de Sensoriamento Remoto, Universidade Federal de Minas Gerais, Belo Horizonte, Brazil
Fire has been a natural feature of the ecosystem for million years. Still, currently fire regimes have been increasingly altered by human activities and climate change, causing economic losses, air pollution, and environmental damage. In Brazil, savannas (locally known as the Cerrado) occupy almost 25% of the area of the country and contain 70% of the concentrated burned area. Fire frequency is related to the use of biannual fire in agricultural practices, aiming at cleaning cattle pastures, which act as ignition sources for the surrounding natural vegetation. Here, we present an ecological model to demonstrate how biennial fire affects plant biomass and carbon release from fine fuel in the Cerrado. The BEFIRE model (Behavior and Effect of Fire) is the first quantitative model to simulate the relationships between fire frequency, plant biomass, and fire-associated emissions based on the synthesis of knowledge about fire behavior and the effects on ecosystems compiled from experimental burnings in the Cerrado. Our model uses microclimate variables and vegetation structure (the amount of the aboveground biomass of trees, shrubs, herbs, and grasses) as inputs, and generates outputs related to the fire behavior (fire spread rate, fire intensity, and heat released) and the fire effects on the dynamic of plant biomass and post-fire carbon emissions. The BEFIRE model predicts that biennial fires allow for the recovery of the biomass of herbs and grasses, due to its fast growth. However, this fire interval does not allow for the recovery of the biomass of shrubs and trees. These growth limitations alter the co-existence of trees/shrubs and herbs/grasses and prevent the uptake of the total amount of emitted carbon from the combustion of fine fuel. Based on the model results, we proposed some recommendations for fire management in this threatened biome.
Introduction
Fire is a historical and frequent event that plays a key role in the processes and functions of global ecosystems, influencing the dynamics of vegetation, biogeochemical cycles, and climate (Beerling and Osborne, 2006; Pausas and Bond, 2020). In recent history, fire has increased in its frequency mainly due to climate change, such as rising temperatures and intensified droughts, and due to human activities (Enright et al., 2015; Bowman et al., 2020). Notably, the Brazilian savanna (locally known as the Cerrado and occupying almost 25% of the area of the country) contains 70% of the concentrated burned area (Araújo et al., 2012; Araújo and Ferreira, 2015). A high frequency of fire events occurred in the northern portion of the biome (Santana et al., 2020), where the remaining native vegetation grows (Sano et al., 2010). This high frequency is mainly related to the use of fire as a management tool in agricultural practices like cleaning converted areas and stimulating the resprout of pastures (Mistry, 1998; Miranda et al., 2002). These practices use fire biennially and are important sources of ignition for the spread of fire in the surrounding native vegetation (Medeiros and Fiedler, 2003; França et al., 2007; Dias and Miranda, 2010), especially during the dry season (August–September) when the vegetation is more flammable (Miranda et al., 2010). The biennial fire regime is associated with ecological impacts, such as the reduction of tree biomass (Garda, 2018; da Silva Rios et al., 2018; Montenegro, 2019), species diversity (Silva, 1999; Ribeiro et al., 2012; da Silva Rios and Sousa-Silva, 2017), and the uptake of carbon emitted by fire (Sato, 2003; Sato et al., 2010).
Additionally, prescribed burning—the intentional ignition of controlled fire in the landscape—has been conducted in ecosystems worldwide for environmental management purposes (Knapp et al., 2009; Penman et al., 2011; Collins et al., 2019). These burns are mainly carried out to reduce the risk of fire by reducing the biomass available for burning and to conserve the species of fire-prone ecosystems (Fernandes and Botelho, 2003; Kolden, 2019). However, studies have shown that the application of prescribed fires can shorten the fire interval and potentially reduce carbon stocks (Peterson and Reich, 2001; Collins et al., 2019). The main Brazilian environmental regulation, the Forest Code, allows fire management in protected areas aiming at the conservationist management of native savannas. Accordingly, integrated fire management programs have been implemented in some protected areas of the Cerrado since 2014 (Schmidt et al., 2018). These programs consider ecological, economic, and cultural aspects of fire use, to propose prescribed burns, firefighting, and prevention (Schmidt et al., 2018; Moura et al., 2019). However, these programs are recent and there is still a lot of uncertainty associated with management decisions (Schmidt et al., 2018; Moura et al., 2019), because of fragmented knowledge on the relationships between fire behavior, effects on multiple ecological processes, and different fire regimes (Gomes et al., 2018). Fire ecology involves various aspects that are not usually quantified together, such as fire behavior (fire spread, fire intensity, and heat released), impacts on different vegetation components (herbs and woody layers), and trace gas emissions (Gomes et al., 2018, 2020). The lack of connection between these studies hinders the understanding of the Cerrado‘s ecological processes associated with the different fire regimes.
System Dynamics (SD) is a modeling tool that integrates multiple mathematical equations to describe the general behavior of a system and is used with a view to unifying empirical knowledge (Angerhofer and Angelides, 2000; Duggan, 2016). SD can be used as a quantitative modeling tool for analyzing the impact of recurrent disturbances such as fire, in complex dynamic systems over time (Collins et al., 2013; Yan et al., 2016; Godde et al., 2019; Thompson et al., 2019). It also considers essential fire-related processes, such as interdependence between system components, temporal feedback, and the non-immediate responses of each component (Angerhofer and Angelides, 2000; Duggan, 2016). The use of this modeling tool has contributed to our synthesis of knowledge, decision-making capacity in relation to fire management strategies, and provides a useful basis for improving dynamic global vegetation models (DGVMs) (Harris et al., 2016; Drüke et al., 2019). However, conceptual and quantitative regional fire models in the Cerrado are still incipient in systemic terms, relying only on simplified models that do not consider the relationships between fire behavior and effects on ecosystem processes (Gomes et al., 2018).
Despite the lack of connection between studies about fire behavior and its effects in the Cerrado (Gomes et al., 2018), the information generated by studies using experimental burning allow us to construct SD models. Thus, after an extensive literature review about experimental fires in the Brazilian savanna, we developed BEFIRE (Behavior and Effect of Fire)—the first quantitative model based on SD for the Cerrado. The model simulates the relationships between fire frequency, plant biomass, and fire-associated emissions to improve the understanding of fire effects on ecosystems and support decision making related to fire management. In this study we simulated two scenarios of fire frequency: I) one with a single fire (1F) in the dry season (September), to represent a fire regime with a longer time interval (4 years) favoring the recovery of plant biomass, and II) a scenario with two biennial fires (2BF) in the dry season, to represent the most common fire regime. The dynamics of plant biomass and fire-associated emissions by the fire were simulated over a period of 4 years post-fire to allow for the validation of the model by field data.
Materials and Methods
Study Area
The Cerrado biome is classified as an Aw tropical savanna climate (Alvares et al., 2013). There is a high seasonality in the precipitation distribution, with a rainy season (October–March) and a dry season (April–September) (Silva et al., 2008). The Cerrado biome is characterized by a mosaic of heterogeneously distributed vegetation formations (grassland, savanna, and forest) (Figure 1), mainly defined by a tree cover gradient (Ribeiro and Walter, 2008). Fire occurrence in the Cerrado is also highly seasonal, as during the rainy season there is a substantial increase in the amount of plant biomass, while in the dry season this biomass becomes highly inflammable (Miranda et al., 2010; Hoffmann et al., 2012).
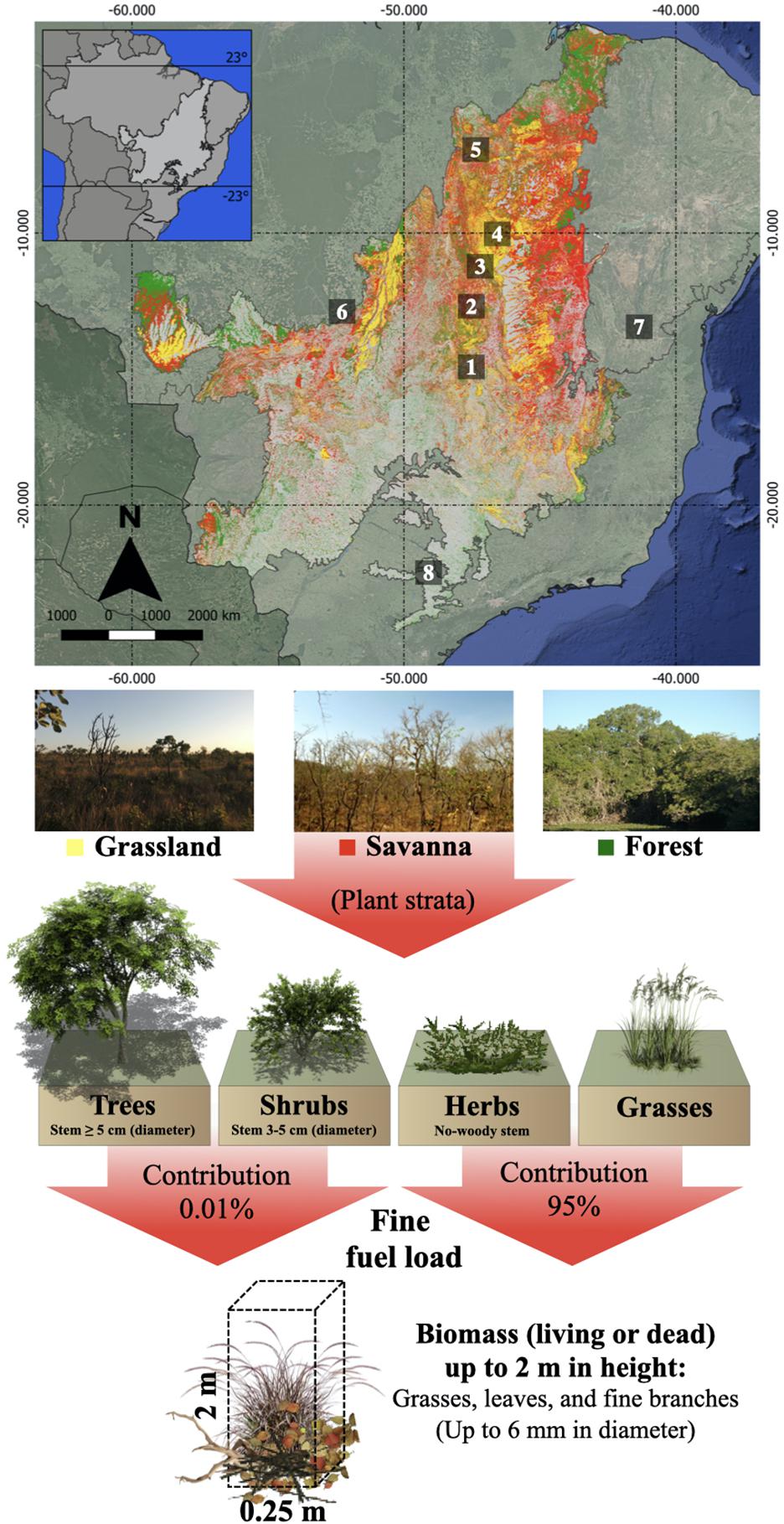
Figure 1. Location of experimental fires carried out in the Brazilian Cerrado and distribution of the main types of vegetation in the Cerrado from MapBiomas (http://mapbiomas.org/), classification of vegetation strata in the savanna, and their contributions to the fine fuel composition (online version in color). (1) Ecological Reserve, Distrito Federal; (2) Serra do Tombador Nature Reserve, Goiás; (3) Água Limpa Ranch, Tocantins; (4) Jalapão State Park, Tocantins; (5) Chapada das Mesas National Park, Maranhão; (6) Tanguro Ranch, Mato Grosso; (7) Serra do Sincorá, Bahia, and (8) Ecological Station of Santa Bárbara, São Paulo. Descriptions of the study areas are shown in Supplementary Table S1.
We used the savanna formation to parameterize the developing SD model, as this type of vegetation is the most representative of the biome (in millions of hectares, savanna ∼ 76, forest ∼ 40, and grassland ∼ 8) (Sano et al., 2010). Savanna formation is characterized by the coexistence of different vegetation strata, such as trees, shrubs, herbs, and grasses (Ribeiro and Walter, 2008), which are differently affected by fire (Sato, 2003; Sato et al., 2010). Thus, we considered these vegetation strata and their respective biomass dynamic (before and after fire).
Database Construction
Information regarding ecological processes related to the structure and function of this vegetation type was obtained from an extensive literature review from 1994 to 2020 (see Gomes et al., 2018, 2020), using the following platforms: Web of Science, Science Direct, Google Scholar, and the Brazilian Digital Library of Theses, and Dissertations. We used the following key words: (prescribed burns∗ OR fire) AND (behavior∗ OR effect∗ OR management∗ OR regime∗ OR emissions ∗ OR frequency). We included research papers, theses, and dissertations that used prescribed burns to characterize the fire behavior and effect in the Cerrado. We identified eight experimental burn studies distributed throughout the Cerrado biome (Figure 1 and Supplementary Table S1): (1) IBGE Ecological Reserve, Distrito Federal (Kauffman et al., 1994; Miranda et al., 1996; Castro and Kauffman, 1998; Silva, 1999; Castro-Neves, 2000; Medeiros, 2002; Sato et al., 2010), (2) Serra do Tombador Nature Reserve, Goiás (Fidelis et al., 2013; Gorgone-Barbosa et al., 2015; Rissi et al., 2017), (3) Água limpa Ranch, Totacantins (Cachoeira et al., 2020), (4) Jalapão State Park, Tocantins (Schmidt et al., 2016), (5) Chapada das Mesas National Park, Maranhão (Schmidt et al., 2016; Montenegro, 2019; Santos, 2019), (6) Tanguro Ranch, Mato Grosso (Balch et al., 2008; Brando et al., 2014), (7) Serra do Sincorá, Bahia (Conceição and Pivello, 2011), and (8) Ecological Station of Santa Bárbara, São Paulo (Brooklynn et al., 2020).
Heading Principles of the BEFIRE Model
We developed the BEFIRE model to support the decision-making process in protected areas determining the frequency of prescribed burns in the Cerrado. We use the method of System Dynamics to combine qualitative analysis with quantitative analysis (Angerhofer and Angelides, 2000; Duggan, 2016). This method is widely used to describe the mechanism of a disturbance using a dynamic view, thus analyzing the relationships and links between various factors caused by the disturbance, as well as the consequences of these factors on the event over time (Godde et al., 2019; Thompson et al., 2019). We used the system dynamics simulation software Vensim (2017) to simulate these processes. Detailed descriptions on the relationships and equations used are described below and in the supporting information.
The BEFIRE model simulates the effect of fire on vegetation and emissions (Figure 2). Each of these compartments is represented in the model as stocks. The stocks represent the balance of resource (biomass and carbon, respectively) based on losses and gains over time. This value depends on what has happened in the past (Angerhofer and Angelides, 2000; Duggan, 2016). In this model the plants biomass varies monthly, depending on the monthly rates of the defined increases or decreases. The loss and gain rates were based on literature from long-term experiments and were specific to the Brazilian savanna (Figure 1 and Supplementary Tables S1, S2), where it was possible to obtain the monthly rates of plant biomass variations (with and without fire) (Supplementary Tables S2, S3). Since reductions in biomass represent plant mortality and increases in biomass represent plant recruitment, we calculated the plant biomass using an allometric equation specific for Brazilian savannas (Roitman et al., 2018; Supplementary Figures S1, S2).
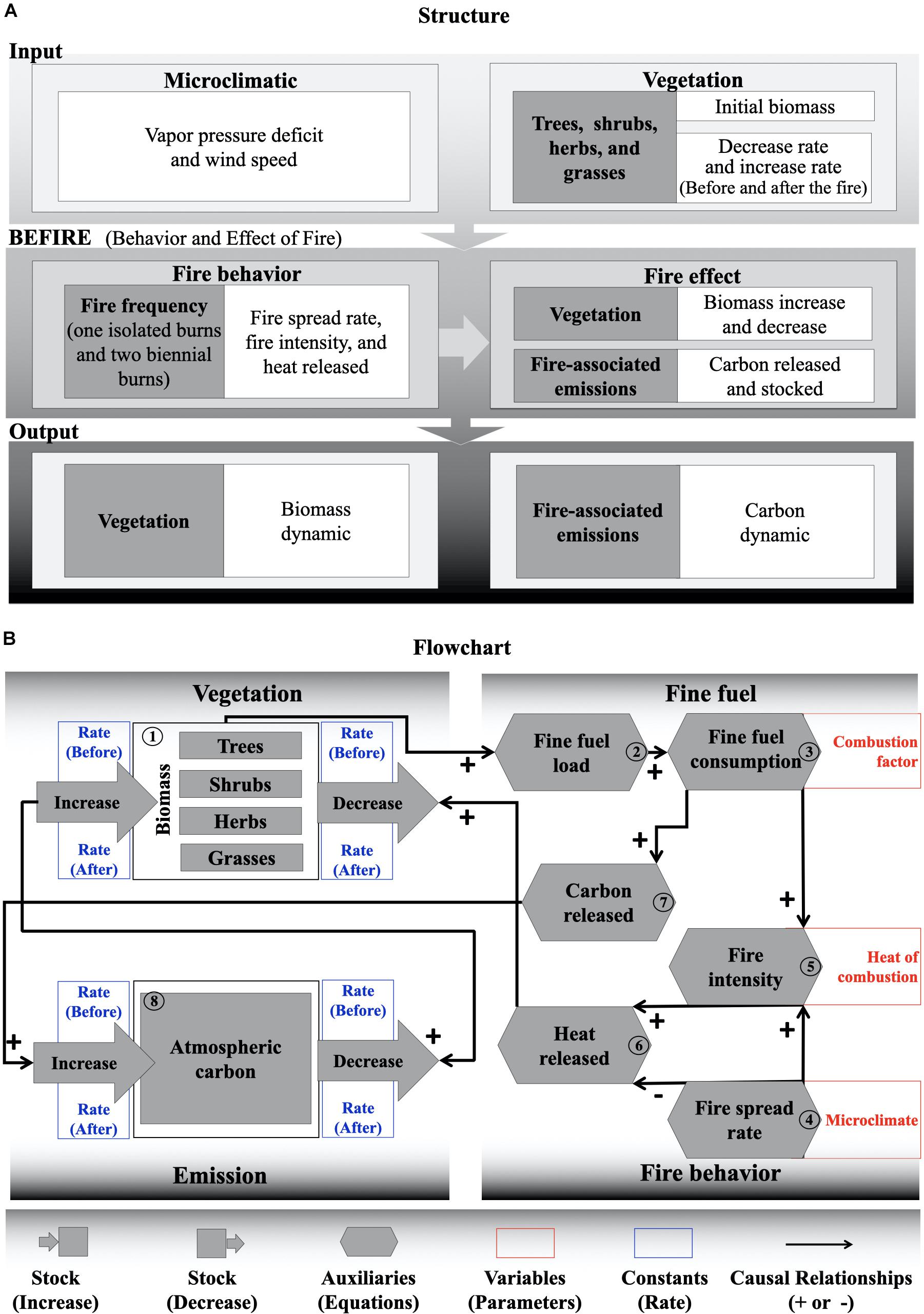
Figure 2. (A) Structure and (B) flowchart of the BEFIRE model. The numbers represent the steps of the model (online version in color).
The first step of the model (Figure 2—Step 1) was given by the initial biomass values (inputs) for each plant strata (Supplementary Table S3), which can vary according to the characteristics of each site. We calculated the contribution of each of these plant strata to the fine fuel load (Figure 2—Step 2), because the composition of fine fuel available is an important predictor of the fire behavior and its effect in the Cerrado (Hoffmann et al., 2012; Brooklynn et al., 2020; Gomes et al., 2020). The fine fuel load corresponds to biomass (living or dead) on the soil surface, made up of grasses, leaves, and fine branches with diameters up to 6 mm (Luke and McArthur, 1978) and, estimated up to a height of 2 m (MCT, 2002). Approximately 95% of the biomass of the herbs-grasses stratum is available for burning (Batmanian and Haridasan, 1985; Miranda et al., 2010; Hoffmann et al., 2012), while only 0.01% of the trees-shrub biomass is available (Nardoto et al., 2006; Hoffmann et al., 2012; Figure 1). Next, we quantified the fuel material consumption rates (Figure 2—Step 3), which can vary from 80 to 90% according to microclimatic variables (MCT, 2002; Miranda et al., 2010; Gomes et al., 2020).
The fire spread rate, fire intensity, and heat released equations defined the fire behavior subsystem (Table 1). These parameters have been widely used in the Cerrado biome, and have been considered satisfactory in representing the fire behavior (Miranda et al., 2010; Hoffmann et al., 2012; Gomes et al., 2018, 2020; Brooklynn et al., 2020; Cachoeira et al., 2020). The fire spread rate equation (Figure 2—Step 4) was obtained from a selection of models, specific for the Brazilian savanna (Table 1; Eq. 1), where the vapor pressure deficit (VPD) and wind speed were the most important variables in determining the fire spread rate (Gomes et al., 2020), then the microclimate characteristics in the model were represented by two input variables: VPD and wind. Actually, the VPD (Table 1; Eq. 2) has also been used in global models of fire behavior (Drüke et al., 2019) as an important metric by considering both the relative averages of temperature and humidity in its equation (Allen et al., 1998). It represents a measure of the evaporative demand that drives water loss from fine fuels (Brooklynn et al., 2020).
We used the equation of Byram (1959) to calculate fire intensity from the fire spread rate and fuel consumed (Figure 2—Step 5), which corresponds to the rate of energy release per unit of length (Table 1; Eq. 3). The heat release (Figure 2—Step 6) was also used according to Rothermel and Deeming (1980), which corresponds to the released heat per unit area and is a product of the intensity over the fire spread rate (Table 1; Eq. 4). These two parameters have been used in most fire behavior studies (Gomes et al., 2020) and have been considered strong predictors of the severity of fires in the biome (Miranda et al., 2010; Hoffmann et al., 2012; Brooklynn et al., 2020; Gomes et al., 2020).
The carbon emissions (Figure 2—Step 7) were calculated according to the Brazilian inventory of anthropic emissions of greenhouse gas (MCT, 2002), based on the Intergovernmental Panel on Climate Change (IPCC) guidelines (Table 1; Eq. 5). This methodology consists of estimating carbon emissions (with and without fire) from the amount of biomass (living and dead) of vegetation (Table 1 and Supplementary Table S1). The last step corresponds to the carbon stock in the atmosphere (Figure 2—Step 8) where the carbon dynamic is given by the carbon emitted by the fire (input) and the carbon that is being absorbed (output) by the vegetation over time, thus closing the systemic relationship between vegetation, climate, and fire.
We also created two fire frequency scenarios. The first involves a single fire (1F) to simulate the recuperation of the plants biomass and the carbon dynamic 4 years after the fire. In the second, we used two biennial fires (2BF) to simulate the effect of consecutive burning, similar to the burning regime of the biome (Dias and Miranda, 2010; Santana et al., 2020). All these simulations refer to the fire period at the end of the dry season (specifically, in September), when climatic conditions increase susceptibility of wildfires (Miranda et al., 2002).
We also tested different scenarios (moderate, medium, and extreme) for VPD, wind, and fine fuel in order to simulate their effects on fire behavior and carbon emissions defined according to their range of variations in the studies compiled (Supplementary Table S4). All input variables of the BEFIRE model, both vegetation (as fuel load and recovery rate) and microclimate (VPD and wind), allow the BEFIRE model’s parametrization for other regions of the Cerrado.
The BEFIRE model contains the following assumptions: (a) vegetation is a native Cerrado savanna; (b) the prescribed burning is carried out in September; (c) the terrain is flat; (d) fine fuel follows a continuous spatial distribution; (e) fires are at the surface; (f) fire mainly consumes fine fuel up to 2 m in height, and (g) fire follows the direction of the wind. The BEFIRE model does not consider: (a) ignition risk and patterns of fire spreading; (b) influence of species composition on fire behavior and effects, and (c) influence of topography on fire behavior. All these parameters have been highlighted as important drivers of fire behavior, but operating at larger spatial scales (Jin, 2010). The current version of the BEFIRE model does not yet present a spatial component (i.e., its simulations represent only one pixel of an image). Moreover, these limitations can be justified by the lack of sufficient empirical knowledge for the calibration and validation of the BEFIRE model. However, the BEFIRE model can become an important basis for future models and studies that consider these relationships between fire behavior and landscape.
Results
Our simulations demonstrate different patterns of biomass variation over time between plant strata (trees, shrubs, herbs, and grasses) and between burning scenarios (1F and 2BF) (Figure 3). In the simulation 1F, the biomass of trees, herbs, and grasses recovered more rapidly than the biomass of shrubs. After the fire occurrence the biomass of trees declined (initial = 0.90; post-fire = 0.54 kg m–2) and recovered its initial biomass in approximately 12 months. Shrubs also lost much of their biomass after the fire (initial = 0.13; post-fire = 0.07 kg m–2). However, their biomass recovery rate was slower than trees, and they did not regain their initial values before fire (end = 0.09 kg m–2). Although herbs (initial = 0.08; post-fire = 0.01 kg m–2) and grasses (initial = 0.20; post-fire = 0.03 kg m–2) lost much of their biomass after the fire, they regained their initial biomass quickly 15 months after the fire, maintaining its seasonal cycles of biomass variation. At 48 months post-fire, the amount of carbon released from fine fuel consumption (0.23 kg m–2) was gradually absorbed by the vegetation in recovery.
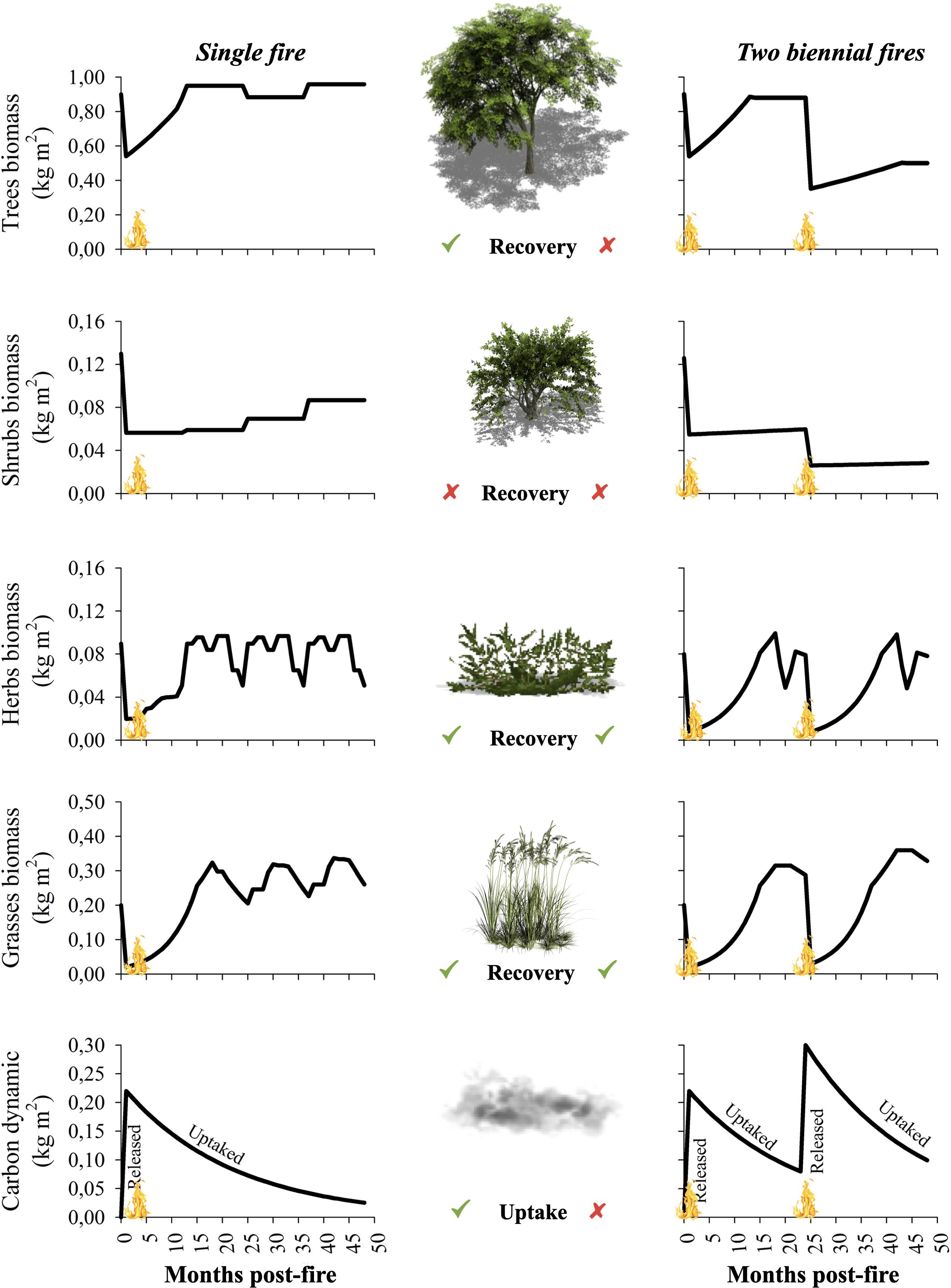
Figure 3. Post-fire recovery simulations for biomass of trees, shrubs, herbs, grasses, and atmospheric carbon dynamic (uncommitted emissions) for the Brazilian savanna, using the BEFIRE model. ✓ = Yes; x = No (online version in color).
In scenario 2BF, the pattern of simulated biomass recovery also varied among plant strata. However, it showed a greater decline in biomass recovery rates compared to scenario 1F (Figure 3). These simulations showed that over a 2 year interval, the biomass of herbs and grasses also recovered and maintained their seasonal cycles of biomass variation. However, the proportion of tree biomass lost by fire increased (1st fire = 40%; 2nd fire = 60%) after the second fire, while the proportion of biomass recovered over time decreased relative to the first fire, recovering only 60% of their initial biomass (initial = 0.90; end = 0.54 kg m–2) after 24 months. The decline in bush shrubs was even more pronounced after the second burning, recovering only 23% of their initial biomass before the fire (initial = 0.13; end = 0.03 kg m–2) after 24 months. These trees and shrubs biomass reductions were reflected in the atmospheric carbon dynamic, resulting in less carbon being absorbed by the vegetation regrowth during this time interval.
During the experimental fires studied, the environmental variables of microclimate (VPD [mim = 1; max = 5 kPa]; wind [mim = 0; max = 2 m s–1); and fine fuel (mim = 0.2; max = 1.1 kg m2) were shown to vary widely (Supplementary Table S2). Our simulations also showed that variations in VPD values, wind speed, and fine fuel influenced the fire spread rate, fire intensity, heat, and carbon released (Table 2). Extremes values of fine fuel (0.8 kg m2), VPD (5 kPa), and wind speed (4 m s–1) resulted in increased fire spread rate (0.94 m s–1), fire intensity (11,124 (kJ m), heat released (11,780 (kJ m–2), fine fuel consumed (0.76 kg m2), and carbon released (0.26 kg m2).
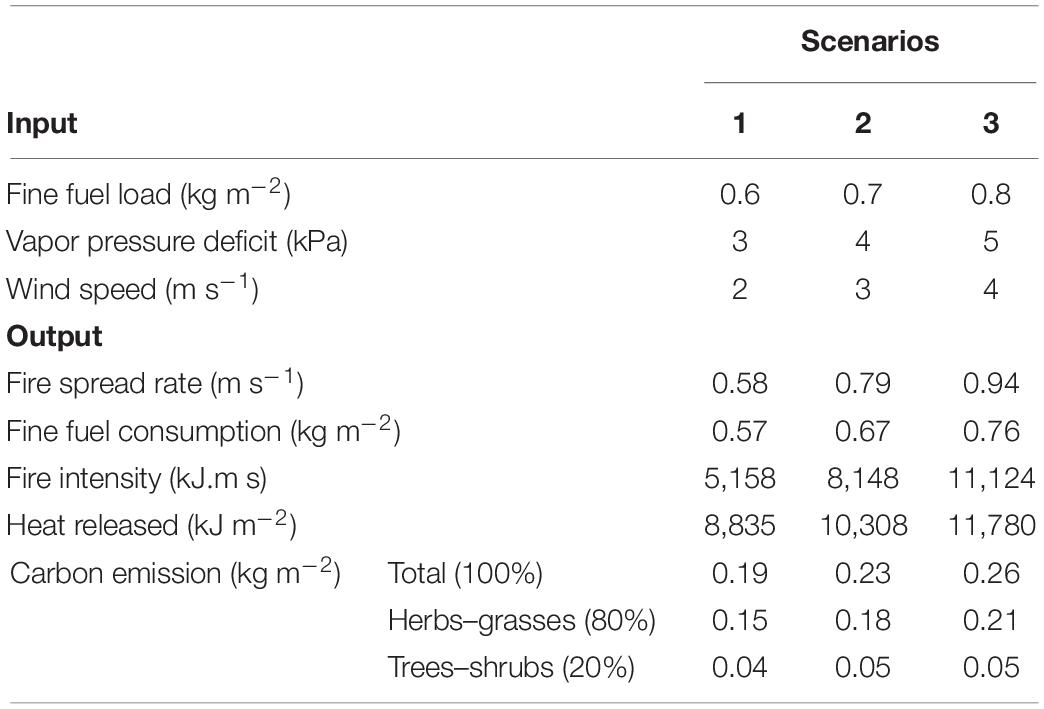
Table 2. Fire behavior and carbon emission simulations for the Brazilian savanna using the BEFIRE model with three scenarios (inputs: 1 moderate, 2 medium, 3 extreme) for fine fuel and microclimate.
Discussion
Tree biomass recovers more rapidly after one fire event (1F) than other vegetation strata, as tree individuals are generally more protected from fire damage, because of higher height, trunk diameter, and bark thickness (Hoffmann et al., 2003; Souchie et al., 2017). Therefore, damage caused by fires is generally associated with partial damage to individual trees, such as topkill (Hoffmann and Solbrig, 2003; Hoffmann et al., 2009; Souchie et al., 2017) and this permits more rapid regeneration through sprouts produced from the trunk or tree crown (Moreira et al., 2008; Souchie et al., 2017). However, for smaller individuals (< 2 m), such as shrubs, fire can cause complete death of the trunk due to greater exposure to higher temperatures, making regeneration more difficult (Moreira et al., 2008; Hoffmann et al., 2009; Gomes et al., 2014).
On the other hand, the recovery of tree biomass declines with increased fire frequency, as the 2 year fire intervals (2 BF) may not be sufficient for the thickening of bark or for trunk growth above the height of greatest exposure to flames (Souchie et al., 2017; Keeley and Pausas, 2019). Other studies also demonstrate the decline in tree biomass in Brazilian savanna after three (Rios, 2016; da Silva Rios et al., 2018) and five biennial fires (Sato, 2003; Sato et al., 2010). This decline in the biomass of trees and shrubs due to successive fires impedes the absorption, over time, of carbon emitted during burns (Sato, 2003; Sato et al., 2010). Furthermore, recurrent fires impact carbon emission not only as a consequence of the combustion of plant biomass, but also in relation to emissions from the soil after burning (Santos, 1999; Pinto et al., 2002). Burnt areas of the Brazilian savanna show greater soil respiration compared with non-burnt areas, especially during the rainy season (Pinto et al., 2002). It is also important to consider that in the case of tree and shrub death, other biomass compartments such as woody material and roots become carbon sources to the atmosphere through decomposition (committed emissions) (Davidson et al., 2002). Additionally, fire intervals shorter than 3 years may impede the vegetation from reabsorbing the nutrients that were lost during burns (Pivello and Coutinho, 1992). The predicted changes in the BEFIRE model demand new approaches to fire management that will maximize the adaptive capacity of these strata to recover the initial biomass.
The biomass of herbs and grasses recover rapidly, even after biennial fires. Fire reduces tree cover, promoting a microclimate with greater temperatures and sun exposure, which favors the germination of these strata (Musso et al., 2015). The non-occurrence of fire makes the soil temperature more stable, which hinders the germination of Cerrado species that require temperature fluctuations to interrupt the dormancy of their seeds (Kolb et al., 2016). Studies have also demonstrated that the biomass of herbs and grasses in the Brazilian savanna is maintained even after five biennial fires (Andrade, 1998; Sato, 2003; Neto, 2005; Miranda et al., 2010; Sato et al., 2010). Furthermore, the proportions of living and dead biomass in fine fuel are the same after 1 year (Andrade, 1998). In this case, fire management, aiming to reduce fine fuel in order to reduce the occurrence of wildland fire (uncontrolled fire) may not be effective due to the rapid recuperation of biomass in these strata while damaging trees and shrubs.
Grasses correspond to approximately 70% of the biomass of fine fuel (Andrade, 1998; Hoffmann et al., 2012). As such, this stratum is responsible for the greater part of the carbon emissions during fire. Also, the proportion of oxidized biomass after fire corresponds to 100% of the dead biomass and 62% of the living biomass of the fine fuel in the Brazilian savanna (MCT, 2002). In this case, we can presume that fires in the late dry season would cause greater carbon emissions, since the amount of dead grasses biomass is greater in this period than in the rainy season (Silva and Haridasan, 2007).
The use of mathematical models to predict the behavior and effects of fire is an important step for fire management in the Cerrado (Gomes et al., 2020). The BEFIRE model can be used to help in the decision making process associated with recent fire management policies for the region. In this case, we suggest monitoring VPD and wind in order to carry out prescribed burns with higher fire spread so as to cause a lower impact on woody savanna vegetation. A lower fire spread rate may cause the ignition of branches and cause severe damage to the vascular tissues of the plant, resulting in death (Kayll, 1968; Silva and Miranda, 1996). We also recommend monitoring the amount of fine fuel to avoid wildland fire in the managed areas reaching high intensity, or the release of great quantities of heat.
On the one hand, biennial fires negatively affect tree and shrub biomass, on the other hand herbs and grasses need to be managed with fire in order to maintain the biodiversity and functioning of the ecosystem (Pinheiro and Durigan, 2009; Abreu et al., 2017; Durigan et al., 2020). As such, we suggest the use of prescribed burns in mosaic configurations with different fire frequencies (no fire and quadrennial fires). In this way, at some locations there would be quadrennial fires to maintain the functioning of the ecosystem, while at other locations there would be the prevention of fire and fighting of non-planned fires at intervals lower than 4 years, aiming to preserve the structure of the woody vegetation, allowing the persistence of species exclusive to each fire regime. As well as this, areas with variation in structure and floristic composition can serve as a refuge for fauna in the case of wildland fire. These factors suggest that a careful evaluation of the multiple aspects and consequences of fire management for the different vegetation strata are essential to guarantee the conservation and functioning of the Cerrado ecosystems.
Concerning the impacts of the fire in the Cerrado, previous studies have shown that when considering isolated fire events, the timing of the fire has little effect on the woody vegetation (Sato, 1996, 2003; Rissi et al., 2017). However, in the case of frequent fires these effects may be intensified, resulting in reductions in the carbon stocks in woody vegetation, with these reductions reaching 9% for early dry season burns, 39% for mid-dry season burns, and 55% for late-dry season burns (Sato, 2003). As such, we suggest that future models and management strategies should consider the interactions between frequency and period of fire.
Data Availability Statement
The datasets analyzed in this study are not publicly available. Requests to access the datasets should be directed to LG, leticiagomesbio@gmail.com.
Author Contributions
LG, MB, HM, and BS-F led the development of the research design, analysis, and manuscript writing. LG, BS-F, LR, and UO contributed to the model implementation and analysis of the model results. All authors revised the draft and gave final approval for publication.
Funding
This research was supported by the National Council for Scientific and Technological Development (CNPq), the Coordination for the Improvement of Higher Education Personnel (CAPES), the World Bank Group (WBG), the Forest Investment Program (FIP), the Rede Clima and INCT Mudanças Climáticas, and the Federal District Research Support Foundation (FAPDF).
Conflict of Interest
The authors declare that the research was conducted in the absence of any commercial or financial relationships that could be construed as a potential conflict of interest.
Acknowledgments
We thank P. Brando, E. Lenza, and their reviewers for helpful comments on the manuscript.
Supplementary Material
The Supplementary Material for this article can be found online at: https://www.frontiersin.org/articles/10.3389/ffgc.2020.507710/full#supplementary-material
References
Abreu, R. C. R., Hoffmann, W. A., Vasconcelos, H. L., Pilon, N. A., Rossatto, D. R., and Durigan, G. (2017). The biodiversity cost of carbon sequestration in tropical savanna. Sci. Adv. 3, 1–8. doi: 10.1126/sciadv.1701284
Allen, R. G., Pereira, L. S., Raes, D., and Smith, M. (1998). Crop Evapotranspiration. Rome: FAO - Food and Agriculture Organization of the United Nations.
Alvares, C. A., Stape, J. L., Sentelhas, P. C., Gonçalves, J. L. M., and Sparovek, G. (2013). Köppen’s climate classification map for Brazil. Meteorol. Zeitschrift. 22, 711–728. doi: 10.1127/0941-2948/2013/0507
Andrade, S. M. A. (1998). Dinâmica do Combustível Fino e Produção Primária do Estrato Rasteiro de áreas de Campo sujo de Cerrado Submetidas a Diferentes Regimes de Queimas. Ph. D thesis, Universidade de Brasília, Brasília.
Angerhofer, B., and Angelides, M. (2000). “System dynamics modelling in supply chain management: research review,” in Proceedings of the 2000 Winter Simulation Conference Proceedings (Cat. No.00CH37165), Orlando, FL.
Araújo, F. M., and Ferreira, L. G. (2015). Satellite-based automated burned area detection: a performance assessment of the MODIS MCD45A1 in the Brazilian Savanna. Int. J. Appl. Earth Obs. Geoinf. 36, 94–102. doi: 10.1016/j.jag.2014.10.009
Araújo, F. M., Ferreira, L. G., and Arantes, A. E. (2012). Distribution patterns of burned areas in the Brazilian Biomes: an analysis based on satellite data for the 2002-2010 period. Remote Sens. 4, 1929–1946. doi: 10.3390/rs4071929
Balch, J. R. K., Nepstad, D. C., Brando, P. M., Curran, L. M., Portela, O., de Carvalho, O., et al. (2008). Negative fire feedback in a transitional forest of southeastern Amazonia. Glob. Chang. Biol. 14, 2276–2287. doi: 10.1111/j.1365-2486.2008.01655.x
Batmanian, G. J., and Haridasan, M. (1985). Primary production and accumulation of nutrients by the ground layer community of cerrado vegetation of central Brazil. Plant Soil 88, 437–440. doi: 10.1007/BF02197500
Beerling, D. J., and Osborne, C. P. (2006). The origin of the savanna biome. Glob. Chang. Biol. 12, 2023–2031. doi: 10.1111/j.1365-2486.2006.01239.x
Bowman, D. M. J. S., Kolden, C. A., Abatzoglou, J. T., Johnston, F. H., van der Werf, G. R., and Flannigan, M. (2020). Vegetation fires in the anthropocene. Nat. Rev. Earth Environ. 1, 500–515. doi: 10.1038/s43017-020-0085-3
Brando, P. M., Balch, J. K., Nepstad, D. C., Morton, D. C., Putz, F. E., Coe, M. T., et al. (2014). Abrupt increases in Amazonian tree mortality due to drought-fire interactions. Proc. Natl. Acad. Sci. U.S.A. 111, 6347–6352. doi: 10.1073/pnas.1305499111
Brooklynn, M. N., Power, N. C. R., Abreu, R. C. R., Durigan, G., Rossatto, D., and Hoffmann, W. A. (2020). Flammability thresholds or flammability gradients? Determinants of fire across savanna-forest transitions. New Phytol. 228, 910–921. doi: 10.1111/nph.16742
Byram, G. M. (1959). “Combustion of fuels,” in Forest Fire Control and Use, ed. K. Davis (New York, NY: McGraw-Hill Book), 61–89.
Cachoeira, J. N., Silva, D. P., Souza-Junior, M. R., Batista, A. C., Giongo, M., Neto, E. G., et al. (2020). Prediction of the variables of fire behavior at sensu stricto Cerrado in southern Tocantins. Adv. For. Sci. 7, 939–946. doi: 10.34062/afs.v7i2.6854
Castro, E. A., and Kauffman, J. B. (1998). Ecosystem structure in the Brazilian Cerrado: a vegetation gradient of aboveground biomass, root mass and consumption by fire. J. Trop. Ecol. 14, 263–283. doi: 10.1017/S0266467498000212
Castro-Neves, B. M. (2000). Comportamento de Queimadas, Temperaturas do Solo e Recuperação da Biomassa Aérea em Campo sujo Nativo e em capim Gordura (Melinis Minutiflora). Ph. D thesis, Universidade de Brasília, Brasília.
Collins, L., Bradstock, R., Ximenes, F., Horsey, B., Sawyer, R., and Penman, T. (2019). Aboveground forest carbon shows different responses to fire frequency in harvested and unharvested forests. Ecol. Appl. 29, 1–14. doi: 10.1002/eap.1815
Collins, R. D., Neufville, R., Claro, J., Oliveira, T., and Pacheco, A. P. (2013). Forest fire management to avoid unintended consequences: a case study of Portugal using system dynamics. J. Environ. Manag. 130, 1–9. doi: 10.1016/j.jenvman.2013.08.033
Conceição, A., and Pivello, V. R. (2011). Biomassa combustível em campo sujo no entorno do parque nacional da chapada diamantina, Bahia, Brasil. Biodivers. Bras. 2, 146–160.
da Silva Rios, M. N., and Sousa-Silva, J. C. (2017). Grupos funcionais em áreas com histórico de queimadas em cerrado sentido restrito no Distrito Federal. Pesqui. Florest. Bras. 37:285. doi: 10.4336/2017.pfb.37.91.1386
da Silva Rios, M. N. S., Sousa-Silva, J. C., and Malaquias, J. V. (2018). Mudanças pós-fogo na florística e estrutura da vegetação arborea-arbustiva de um cerrado sentido restrito em Planaltina-DF. Ciência Florest. 28, 469–482. doi: 10.5902/1980509832028
Davidson, E. A., Savage, K., Bolstad, P., Clark, D. A., Curtis, P. S., Ellsworth, D. S., et al. (2002). Belowground carbon allocation in forests estimated from litterfall and IRGA-based soil respiration measurements. Agric. For. Meteorol. 113, 39–51. doi: 10.1016/S0168-1923(02)00101-6
Dias, B. F. S., and Miranda, H. S. (2010). “O Projeto fogo,” in Efeitos do Regime do Fogo sobre a Estrutura de Comunidades de Cerrado: Resultados do Projeto Fogo, ed. H. S. Miranda (Brasília: IBAMA), 15–22.
Drüke, M., Forkel, M., von Bloh, W., Sakschewski, B., Cardoso, M., Bustamante, M., et al. (2019). Improving the LPJmL4-SPITFIRE vegetation-fire model for South America using satellite data. Geosci. Model. Dev. Discuss. 12, 5029–5054. doi: 10.5194/gmd-2019-92
Duggan, J. (2016). System Dynamics Modeling with R. Cham: Springer International Publishing. doi: 10.1007/978-3-319-34043-2
Durigan, G., Pilon, N. A. L., Abreu, R. C. R., Hoffmann, W. A., Martins, M., Fiorillo, B. F., et al. (2020). No net loss of species diversity after prescribed fires in the Brazilian Savanna. Front. For. Glob. Chang. 3:13. doi: 10.3389/ffgc.2020.00013
Enright, N. J., Fontaine, J. B., Bowman, D. M. J. S., Bradstock, R. A., and Williams, R. J. (2015). Interval squeeze: altered fire regimes and demographic responses interact to threaten woody species persistence as climate changes. Front. Ecol. Environ. 13, 265–272. doi: 10.1890/140231
Fernandes, P. M., and Botelho, H. S. (2003). A review of prescribed burning effectiveness in fire hazard reduction. Int. J. Wildl. Fire 12, 117–128. doi: 10.1071/WF02042
Fidelis, A., Lyra, M. F., di, S., and Pivello, V. R. (2013). Above- and below-ground biomass and carbon dynamics in Brazilian Cerrado wet grasslands. J. Veg. Sci. 24, 356–364. doi: 10.1111/j.1654-1103.2012.01465.x
França, H., Ramos Neto, M. B., and Setzer, A. (2007). O fogo no Parque Nacional das Emas. Brasília: Ministério do Meio Ambiente.
Garda, A. B. (2018). Dano e Recuperação pós-fogo em Espécies Lenhosas do Cerrado: Fogo Após 18 anos de Proteção Versus Queimadas Bienais em três Épocas Distintas. Ph. D thesis, Universidade de Brasília, Brazíl.
Godde, C., Dizyee, K., Ash, A., Thornton, P., Sloat, L., Roura, E., et al. (2019). Climate change and variability impacts on grazing herds: insights from a system dynamics approach for semi-arid Australian rangelands. Glob. Chang. Biol. 25, 3091–3109. doi: 10.1111/gcb.14669
Gomes, L., Maracahipes, L., Marimon, B. S., Reis, S. M., Elias, F., Maracahipes-Santos, L., et al. (2014). Post-fire recovery of savanna vegetation from rocky outcrops. Flora 209, 201–208. doi: 10.1016/j.flora.2014.02.006
Gomes, L., Miranda, H. S., and Bustamante, M. (2018). How can we advance the knowledge on the behavior and effects of fire in the Cerrado biome? For. Ecol. Manag. 417, 281–290. doi: 10.1016/j.foreco.2018.02.032
Gomes, L., Miranda, H. S., Silvério, D. V., and Bustamante, M. M. C. (2020). Effects and behaviour of experimental fires in grasslands, savannas, and forests of the Brazilian Cerrado. For. Ecol. Manag. 458:117804. doi: 10.1016/j.foreco.2019.117804
Gorgone-Barbosa, E., Pivello, V. R., Bautista, S., Zupo, T., Rissi, M. N., and Fidelis, A. (2015). How can an invasive grass affect fire behavior in a tropical savanna? A community and individual plant level approach. Biol. Invas. 17, 423–431. doi: 10.1007/s10530-014-0740-z
Harris, R. M. B., Remenyi, T. A., Williamson, G. J., Bindoff, N. L., and Bowman, D. M. J. S. (2016). Climate-vegetation-fire interactions and feedbacks: trivial detail or major barrier to projecting the future of the Earth system? Wiley Interdiscip. Rev. Clim. Chang. 7, 910–931. doi: 10.1002/wcc.428
Hoffmann, W. A., Adasme, R., Haridasan, M., De Carvalho, M. T., Geiger, E. L., Pereira, M. A. B., et al. (2009). Tree topkill, not mortality, governs the dynamics of savanna-forest boundaries under frequent fire in central Brazil. Ecology 90, 1326–1337. doi: 10.1890/08-0741.1
Hoffmann, W. A., Jaconis, S., McKinley, K., Geiger, E., Gotsh, S., and Franco, A. C. (2012). Fuels or microclimate? Understanding the drivers of fire feedbacks at savanna-forest boundaries. Austr. Ecol. 37, 634–643. doi: 10.1111/j.1442-9993.2011.02324.x
Hoffmann, W. A., Orthen, B., and Nascimento, P. K. V. (2003). Comparative fire ecology of tropical savanna and forest trees. Funct. Ecol. 17, 720–726. doi: 10.1111/j.1365-2435.2003.00796.x
Hoffmann, W. A., and Solbrig, O. T. (2003). The role of topkill in the differential response of savanna woody species to fire. For. Ecol. Manag. 180, 273–286. doi: 10.1016/S0378-1127(02)00566-2
Jin, H. (2010). Drivers of Global Wildfires- Statistical Analyses. Thesis, Department of Earth and Ecosystem Sciences, Lund University.
Kauffman, J. B., Cummings, D. L., and Ward, D. E. (1994). Relationships of fire, biomass and nutrient dynamics along a vegetation gradient in the Brazilian Cerrado. J. Ecol. 82, 519–531. doi: 10.2307/2261261
Kayll, A. J. (1968). “Heat tolerance of tree seedlings,” in Proceedings of the Tall Timbers Fire Ecology Conference, Tallahassee, FL.
Keeley, J. E., and Pausas, J. G. (2019). Distinguishing disturbance from perturbations in fire-prone ecosystems. Int. J. Wildl. Fire 28:282. doi: 10.1071/wf18203
Knapp, E. E., Estes, B. L., and Skinner, C. N. (2009). Ecological Effects of Prescribed Fire Season: a Literature Review and Synthesis for Managers. Darby, PA: DIANE Publishing. doi: 10.2737/PSW-GTR-224
Kolb, R. M., Pilon, N. A. L., and Durigan, G. (2016). Factors influencing seed germination in Cerrado grasses. Acta Bot. Brasil. 30, 87–92. doi: 10.1590/0102-33062015abb0199
Kolden, C. A. (2019). We’re not doing enough prescribed fire in the western united states to mitigate wildfire risk. Fire 2:30. doi: 10.3390/fire2020030
Luke, R., and McArthur, A. (1978). Bushfire in Australia. Canberra: Australian Government Publishing Service.
MCT (2002). Emissões de Gases de efeito Estufa da Queima de Biomassa do Cerrado Não-Antrópico Utilizando Dados Orbitais. 53. Available online at: https://cetesb.sp.gov.br/proclima/wp-content/uploads/sites/36/2014/05/101.pdf (accessed November 11, 2020).
Medeiros, M. B. (2002). Efeitos do fogo nos Padrões de Rebrotamento em Plantas Lenhosas, em Campo Sujo, Após Queimadas Prescritas. Ph. D thesis, Universidade de Brasília, Brazíl.
Medeiros, M. B., and Fiedler, N. C. (2003). Incêndios florestais no parque nacional da Serra da Canastra: desafios para a conservação da biodiversidade. Ciência Florest. 14, 157–168. doi: 10.5902/198050981815
Miranda, H. S., Bustamante, M. M. C., and Miranda, A. C. (2002). “The fire factor,” in The Cerrados of Brazil: Ecology and Natural History of a Neotropical Savanna, eds P. Oliveira and R. Marquis (New York, NY: Columbia University Press), 53–68.
Miranda, H. S., Neto, W. N., and Castro-Neves, B. M. (2010). “Caracterização das queimadas de Cerrado,” in Efeitos do Regime do fogo sobre a Estrutura de Comunidades de Cerrado: Resultados do Projeto Fogo, ed. H. S. Miranda (Brasília: IBAMA), 23–33.
Miranda, H. S., Silva, E. R., and Miranda, A. C. (1996). “Comportamento do fogo em queimadas de campo sujo,” in Impactos das Queimadas em Áreas de Cerrado E Restinga, eds H. S. Miranda, C. H. Saito, and B. F. S. Dias (Brasília: ECL), 1–10.
Mistry, J. (1998). Fire in the cerrado (savannas) of Brazil: an ecological review. Prog. Phys. Geogr. 22, 425–448. doi: 10.1191/030913398668494359
Montenegro, S. R. (2019). Efeitos do Manejo do fogo na Estrutura de Comunidades Lenhosas em Formações Savânicas do Cerrado. Dissertation, Universidade de Brasília.
Moreira, F., Catry, F., Duarte, I., Acácio, V., and Silva, J. S. (2008). A conceptual model of sprouting responses in relation to fire damage: an example with cork oak (Quercus suber L.) trees in Southern Portugal. Plant Ecol. 201, 77–85. doi: 10.1007/s11258-008-9476-0
Moura, L. C., Scariot, A. O., Schmidt, I. B., Beatty, R., and Russell-Smith, J. (2019). The legacy of colonial fire management policies on traditional livelihoods and ecological sustainability in savannas: impacts, consequences, new directions. J. Environ. Manag. 232, 600–606. doi: 10.1016/j.jenvman.2018.11.057
Musso, C., Miranda, H. S., Aires, S. S., Bastos, A. C., Soares, A. M. V. M., and Loureiro, S. (2015). Simulated post-fire temperature affects germination of native and invasive grasses in cerrado (Brazilian savanna). Plant Ecol. Divers. 8, 219–227. doi: 10.1080/17550874.2014.910714
Nardoto, G. B., Bustamante, M. M., da, C., Pinto, A. S., and Klink, C. A. (2006). Nutrient use efficiency at ecosystem and species level in savanna areas of Central Brazil and impacts of fire. J. Trop. Ecol. 22, 191–201. doi: 10.1017/S0266467405002865
Neto, W. N. (2005). Modelagem Ecológica de Queimadas Usando Inteligência Artificial. Brasília: Universidade de Brasília.
Pausas, J. G., and Bond, W. J. (2020). Alternative biome states in terrestrial ecosystems. Trends Plant Sci. 25, 250–263. doi: 10.1016/j.tplants.2019.11.003
Penman, T. D., Christie, F. J., Andersen, A. N., Bradstock, R. A., Cary, G. J., Henderson, M. K., et al. (2011). Prescribed burning: how can it work to conserve the things we value? Int. J. Wildl. Fire 20, 721–733. doi: 10.1071/WF09131
Peterson, D. W., and Reich, P. B. (2001). Prescribed fire in oak savanna: fire frequency effects on stand structure and dynamics. Ecol. Appl. 11, 914–927. doi: 10.1890/1051-0761(2001)011[0914:pfiosf]2.0.co;2
Pinheiro, E. D. S., and Durigan, G. (2009). Dinâmica espaço-temporal (1962-2006) das fitofisionomias em unidade de conservação do Cerrado no sudeste do Brasil. Rev. Bras. Bot. 32, 441–454. doi: 10.1590/S0100-84042009000300005
Pinto, A. S., Bustamante, M., Kisselle, K., Burke, R., Zepp, R., Viana, L., et al. (2002). Soil emissions of N2O, NO, and CO2 in Brazilian savannas: effects of vegetation type, seasonality, and prescribed fires. J. Geophys. Res. 107, 1–9. doi: 10.1029/2001JD000342
Pivello, V. R., and Coutinho, L. M. (1992). Transfer of macro-nutrients to the atmosphere during experimental burnings in an Open Cerrado (Brazilian Savanna). J. Trop. Ecol. 8, 487–497. doi: 10.1017/s0266467400006829
Ribeiro, J. F., and Walter, B. M. (2008). “As principais fitofisionomias do bioma cerrado,” in Cerrado: Ecologia e Flora, eds S. M. Sano, S. P. Almeida, and J. F. Ribeiro (Planaltina: Embrapa Cerrados), 151–199.
Ribeiro, M. N., Sanchez, M., Pedroni, F., Peixoto, K., and da, S. (2012). Fogo e dinâmica da comunidade lenhosa em cerrado sentido restrito, Barra do Garças, Mato Grosso. Acta Bot. Brasil. 26, 203–217. doi: 10.1590/S0102-33062012000100020
Rios, M. N. S. (2016). Dinâmica de Comunidades Vegetais em Cerrado Típico com Histórico de Fogo no Distrito Federal. Ph. D thesis, Universidade de Brasilia, Brazil.
Rissi, M. N., Baeza, M. J., Gorgone-Barbosa, E., Zupo, T., and Fidelis, A. (2017). Does season affect fire behaviour in the Cerrado? Int. J. Wildl. Fire 26, 427–433. doi: 10.1071/WF14210
Roitman, I., Bustamante, M. M. C., Haidar, R. F., Shimbo, J. Z., Abdala, G. C., Eiten, G., et al. (2018). Optimizing biomass estimates of savanna woodland at different spatial scales in the Brazilian Cerrado: re-evaluating allometric equations and environmental influences. PLoS One 13:e0196742. doi: 10.1371/journal.pone.0196742
Rothermel, R. C., and Deeming, J. E. (1980). Measuring and Interpreting Fire Behavior for Correlation with Fire Effects. Ogden: UT: USDA Forest Service, Intermountain Forest and Range Experiment Station.
Sano, E. E., Rosa, R., Brito, J. L. S., and Ferreira, L. G. (2010). Land cover mapping of the tropical savanna region in Brazil. Environ. Monit. Assess. 166, 113–124. doi: 10.1007/s10661-009-0988-4
Santana, N. C., de Carvalho, O. A., Gomes, R. A. T., and Guimarães, R. F. (2020). Accuracy and spatiotemporal distribution of fire in the Brazilian biomes from the MODIS burned-area products. Int. J. Wildl. Fire 29, 907–918. doi: 10.1071/WF19044
Santos, A. C. (2019). Efeitos de Diferentes Regimes de Queima no Estrato Herbáceo-Arbustivo da Vegetação em Áreas de Manejo Integrado do Fogo no Cerrado. Thesis, Universidade de Brasília, Brazíl.
Santos, A. J. B. (1999). Fluxos de Energia, Carbono e Água em Vegetação de Campo Sujo. Thesis, Universidade de Brasilia, Brasil.
Sato, M. N. (1996). Mortalidade de Plantas do Cerrado Submetidas a Diferentes Regimes de Queima. Thesis, Universidade de Brasilia, Brazil.
Sato, M. N. (2003). Efeito a Longo Prazo de Queimadas Prescritas na Estrutura de Comunidade de Lenhosas da Vegetação do Cerrado Sensu Stricto. Thesis, Universidade de Brasília, Brazil.
Sato, M. N., Miranda, H. S., and Maia, J. M. F. (2010). “O fogo e o estrato arbóreo do Cerrado: efeitos imediatos e a longo prazo,” in Efeitos do Regime do Fogo Sobre a Estrutura de Comunidades de Cerrado: Resultados do Projeto Fogo, ed. H. S. Miranda (Brasília: IBAMA), 77–91.
Schmidt, I. B., Fonseca, C. B., Ferreira, M., and Sato, M. N. (2016). Implementação do programa piloto de Manejo Integrado do Fogo em três Unidades de Conservação do Cerrado. Biodivers. Bras. 6, 55–70.
Schmidt, I. B., Moura, L. C., Ferreira, M., Eloy, L., Sampaio, A. B., Dias, P. A., et al. (2018). Fire management in the Brazilian savanna: first steps and the way forward. J. Appl. Ecol. 55, 2094–2101. doi: 10.1111/1365-2664.13118
Silva, E. R. (1999). Efeito do Regime de Queima na Taxa de Mortalidade e Estrutura da Vegetação Lenhosa de Campo sujo de Cerrado. Thesis, Universidade de Brasília, Brazil.
Silva, E. R., and Miranda, H. S. (1996). “Temperatura do câmbio de espécies lenhosas do cerrado durante queimadas prescritas,” in Biodiversidade e Produção Sustentável de Alimentos e Fibras nos Cerrados: Proceedings of the VIII Simpósio sobre o Cerrado, eds R. C. Pereira and L. C. B. Nasser (Brasília: Embrapa), 253–257.
Silva, F. M., Assad, E. D., and Evangelista, B. A. (2008). “Caracterização climática do Bioma Cerrado,” in Cerrado: Ecologia e Flora, eds S. M. Sano, S. P. Almeida, and J. P. Ribeiro (Planaltina: Embrapa), 69–88.
Silva, J. S. O., and Haridasan, M. (2007). Acúmulo de biomassa aérea e concentração de nutrientes em Melinis minutiflora P. beauv. e gramíneas nativas do cerrado. Rev. Bras. Bot. 30, 337–344. doi: 10.1590/S0100-84042007000200016
Souchie, F. F., Pinto, J. R. R., Lenza, E., Gomes, L., Maracahipes-Santos, L., and Silvério, D. V. (2017). Post-fire resprouting strategies of woody vegetation in the Brazilian savanna. Acta Bot. Brasil. 31, 260–266. doi: 10.1590/0102-33062016abb0376
Thompson, M. P., Wei, Y., Dunn, C. J., and O’Connor, C. D. (2019). Wildfire response policies. Systems 7:25. doi: 10.3390/systems7040049
Keywords: aboveground biomass, climate change, carbon emissions, Cerrado, co-existence, fire behavior, fire frequency, management
Citation: Gomes L, Miranda HS, Soares-Filho B, Rodrigues L, Oliveira U and Bustamante MMC (2020) Responses of Plant Biomass in the Brazilian Savanna to Frequent Fires. Front. For. Glob. Change 3:507710. doi: 10.3389/ffgc.2020.507710
Received: 27 October 2019; Accepted: 18 September 2020;
Published: 24 November 2020.
Edited by:
Gitta Lasslop, Senckenberg Biodiversity and Climate Research Centre, GermanyReviewed by:
Luciana Ghermandi, Consejo Nacional de Investigaciones Científicas y Técnicas (CONICET), ArgentinaRenata Libonati, Federal University of Rio de Janeiro, Brazil
Copyright © 2020 Gomes, Miranda, Soares-Filho, Rodrigues, Oliveira and Bustamante. This is an open-access article distributed under the terms of the Creative Commons Attribution License (CC BY). The use, distribution or reproduction in other forums is permitted, provided the original author(s) and the copyright owner(s) are credited and that the original publication in this journal is cited, in accordance with accepted academic practice. No use, distribution or reproduction is permitted which does not comply with these terms.
*Correspondence: Letícia Gomes, leticiagomesbio@gmail.com