- 1Wildlife Conservation Society, Global Conservation Program, Bronx, NY, United States
- 2World Wildlife Fund, Oslo, Norway
- 3World Wildlife Fund, Washington, DC, United States
- 4School of Earth and Environmental Sciences, University of Queensland, Brisbane, QLD, Australia
As demand for commodities increases globally, resource-rich countries are focal areas for industrial expansion. The extractives sector is driving unprecedented growth of infrastructure (e.g., roads, railways and pipelines) into sparsely populated regions, which contain some of the world’s remaining intact forests. Yet, the extent of the threat posed by an increased footprint of extractive industries is not well understood. Here we provide a novel global assessment of the potential threat posed to tropical Intact Forest Landscapes (IFLs), continuous patches of forest that have no remotely sensed signs of human activity or forest fragmentation, from the extractives sector including mining, oil and gas projects, using one of the most extensive global spatial datasets on extractives concessions to date. We found ∼20% of the global tropical IFL estate is designated as extractive’s concessions, presenting an enormous threat to these forests. Oil and gas concessions overlap with 408,000 km2 of tropical IFLs (7.85% of total IFL area), with the biggest overlap in the Democratic Republic of the Congo and Papua New Guinea. Mining concessions overlap with 589,000 km2 (11.33%) of tropical IFL area, mainly in Brazil and Democratic Republic of Congo. As the majority of concessions overlapping IFLs are currently at the exploration stages for all types of extractives, now is the time to plan for reducing the potential threat through strategies such as identifying “no go” areas and applying the mitigation hierarchy. Nations and investors should undertake regional strategic environmental assessments to reduce impacts, including potential indirect and cumulative impacts of multiple projects and other threats in the landscape. We also call for banks that funders of extractives projects to apply global best practice industry environmental safeguards that recognize the value of intact forests and the risk of cumulative impacts to these unique sites.
Introduction
A rapidly increasing global population and consequent demand for commodities are driving industrial expansion across the biodiverse tropical areas of South America, Asia-Pacific and Central Africa (Harfoot et al., 2018). Extractive industries such as oil and gas, in addition to mining, are growing at an increasing pace, and this industry boom has led to an unprecedented expansion of infrastructure (e.g., roads, railways and pipelines) into sparsely populated regions (Lessmann et al., 2016; Kleinschroth et al., 2019; Johnson et al., 2020). These developments provide fiscal revenues and economic opportunities in rural areas for many less-developed countries, but can be especially problematic when they occur in areas of high-value biodiversity regions such as wilderness areas and intact forests, which also provide critical ecosystems services for people (Potapov et al., 2008; Watson et al., 2018a,b).
An IFL is a large patch of forest, including naturally treeless ecosystems, that has no remotely sensed signs of human activity and is at least 500 km2 in size that can have low-intensity and historic human influence, such as hunting, scatter small scale shifting cultivation, and preindustrial selective logging (Potapov et al., 2017). IFLs are considered globally important for biodiversity conservation. They represent some of the last places on Earth that still contain species assemblages at near-natural levels of abundance, especially for wide-ranging species (Mittermeier et al., 2003; Ripple et al., 2014). They are also vitally important for stabilizing terrestrial carbon storage, with the Earth’s forests absorbing around a quarter of anthropogenic carbon emissions each year (Pan et al., 2011; Houghton, 2013; Maxwell et al., 2019), and for regulating hydrological regimes by generating rainfall (Sheil and Murdiyarso, 2009). Intact forests are also home to some of the most politically and economically marginalized indigenous communities, many of whom still have strong bio-cultural connections to their land (Kormos et al., 2017).
Despite their extraordinary value for biodiversity and humanity, many IFLs are declining rapidly. Over 7% of the total IFL area (919,000 km2) was lost between 2000 and 2013, with 60% of this loss occurring in the tropics (Potapov et al., 2017). The drivers of this loss are complex and varied, but natural resource extraction projects make a large contribution (Harfoot et al., 2018). Seismic survey lines and access roads (e.g., to drill pads) for resource exploration, pipelines, power lines, and linear infrastructure for transport (roads, railways, pipelines etc.) are all key drivers of habitat fragmentation (Parish et al., 2013). In general, linear infrastructure increases accessibility and leads to increases in hunting, logging, artisanal mining, charcoal and fuelwood extraction, and small-scale agriculture (Suárez et al., 2009; Finer and Orta-Martínez, 2010). These indirect causes of biodiversity loss are often far greater in spatial extent and overall impact than the original footprint of infrastructure from development (Sonter et al., 2018; Johnson et al., 2020). Forest loss and degradation have substantial and well-documented impacts on biodiversity (Newbold et al., 2015), but can also compromise ecosystem service provision (Sutton et al., 2016) and even bring people into closer contact with potentially disastrous disease vectors (Watson et al., 2018a). Oil spills and the tailings from dam walls breaking can have devastating impacts downstream as was the case with the Brumadinho iron mine in Brazil, which killed 259 people and destroyed huge areas of forests and other ecosystems (Silva Rotta et al., 2020).
Here we explore the potential threat posed by the extractives sector to tropical IFLs in the three major tropical forest regions: South America, Asia-Pacific and Central Africa. We quantify this using one of the most comprehensive global spatial datasets on extractive concessions (mining and oil and gas) to identify IFLs that are at elevated risk of forest loss and degradation from exposure to extractive projects. Our results aim to improve understanding of the overall threat that extractive projects pose to intact tropical forests and to help guide the establishment of policies and processes that can minimize their environmental damage.
Materials and Methods
In this study, we calculated the spatial overlap of extractive concessions specifically mining, and oil and gas, with the IFL dataset for 2016. The spatial scope of the study is the three tropical regions: (1) South America, (2) Asia-Pacific (excluding Australia), and (3) Central Africa (Figures 1, 2). There were several countries within these regions for which we could not obtain data. For mining, these were Angola, Bangladesh, Brunei, China, Equatorial Guinea, India, Malaysia, Nigeria, Singapore, São Tomé and Principe, Suriname, Timor-Leste, Thailand, Venezuela, and Vietnam. For oil and gas, it was only Singapore.
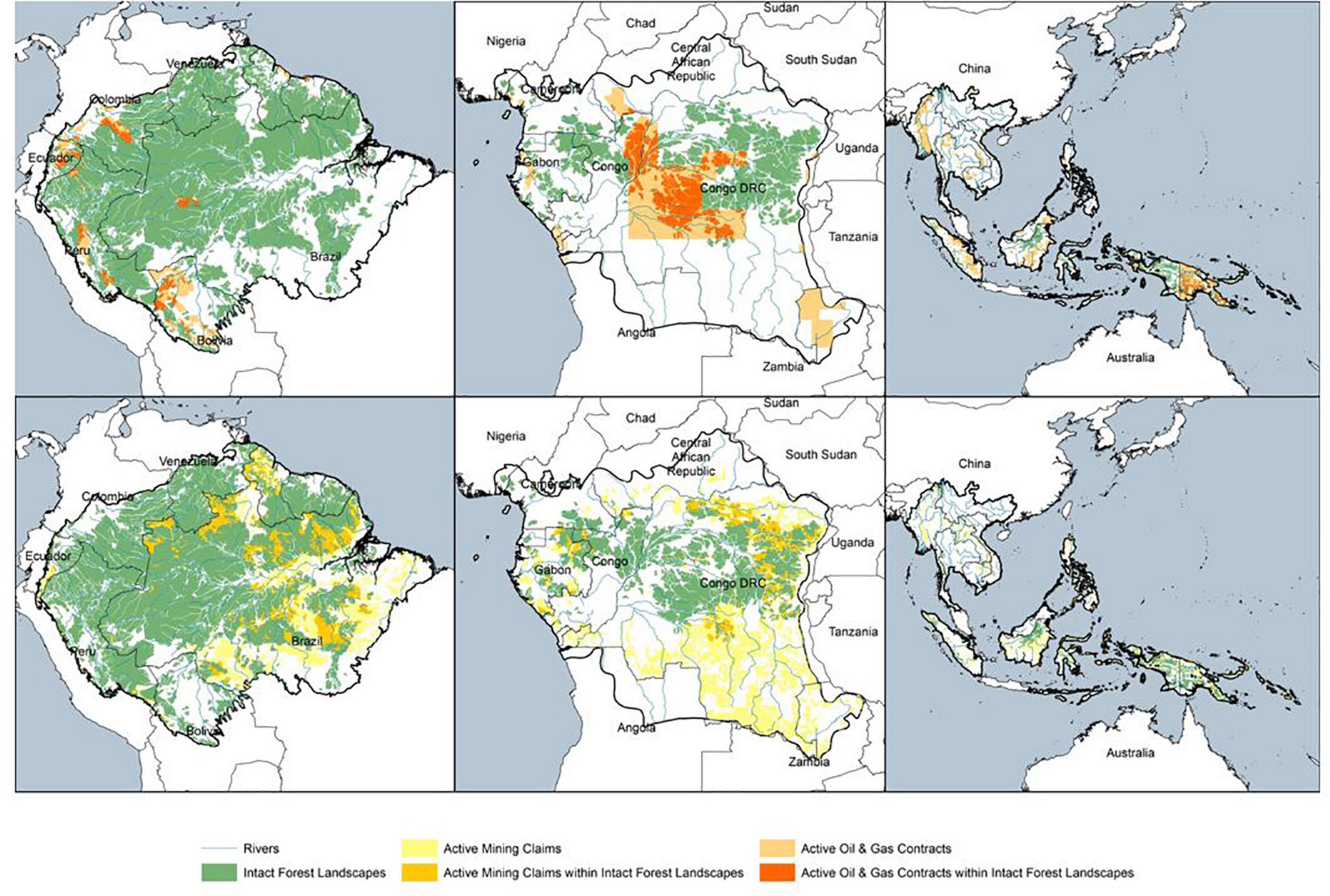
Figure 1. The distribution of IFLs and oil and gas contracts (top) and mining leases (bottom) for the three regions of interest: South America (left), Central Africa (middle) and Asia-Pacific (right).
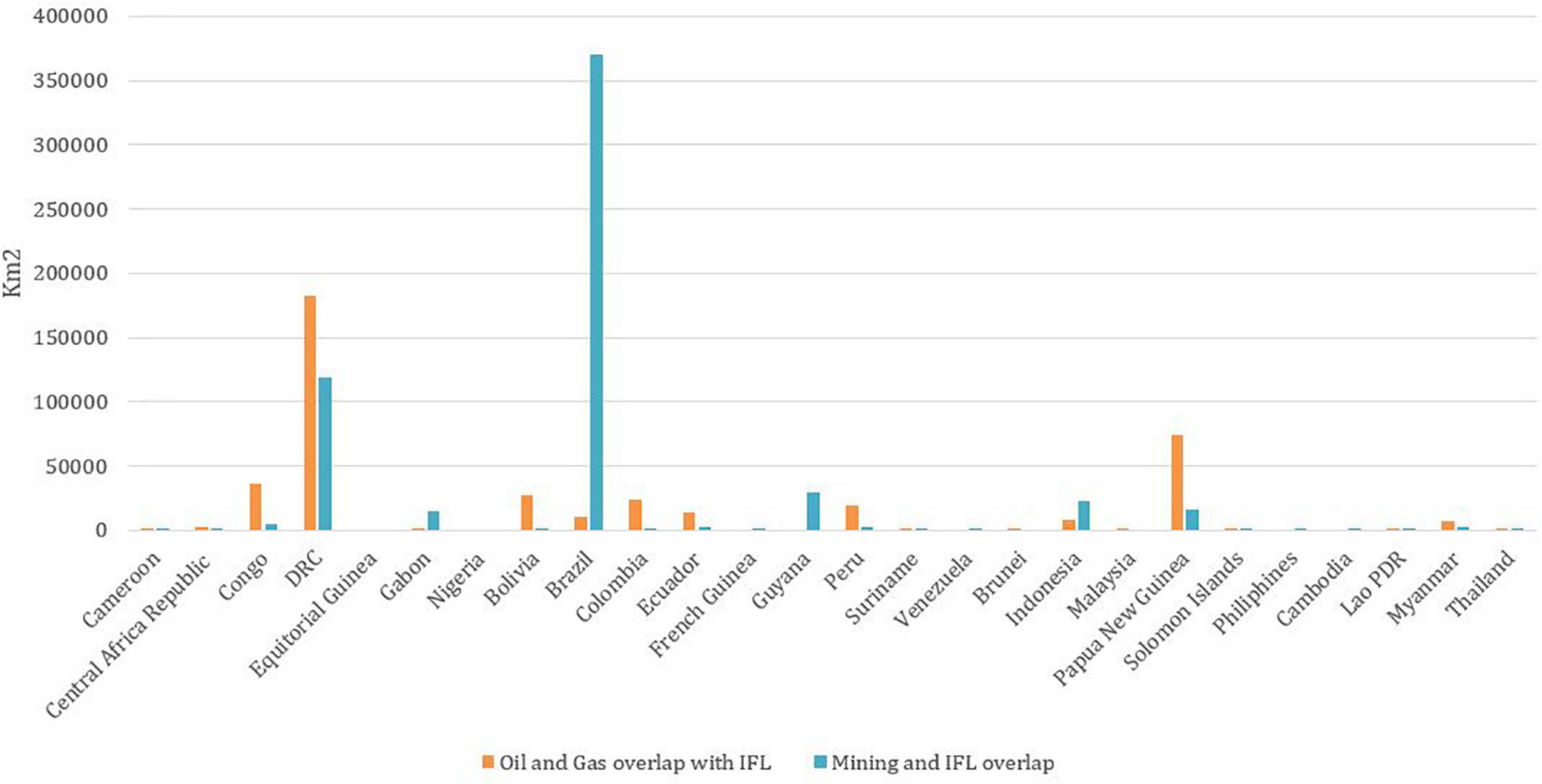
Figure 2. The area of overlap of Oil and Gas contracts, and mining claims and IFLs within each country in the study region.
Data
The IFL map depicts the extent of IFLs for 20161. Data defining sub-basins was based on HydroBASINS Level 4 which provides a global coverage of consistently sized and hierarchically nested sub-basins at different scales (Lehner and Grill, 2013). The data used for the spatial location of mining concessions was sourced from the SNL Metals and Mining, an offering of S&P SNL Metals and Mining (2018) and contains copyrighted and trade secret material distributed under license from SNL (accessed January 2018) and therefore are not publicly available. The data defining the spatial location of oil and gas concessions was sourced from Drilling Info (2018) and contains copyrighted material distributed under license (accessed January 2018). We screened the database by applying the following filters. For oil and gas, we included claims with the status of “Application,” “Contract”, “Reserved areas,” and “under negotiation.” We excluded contracts with status “Force majeure” or “Open” as these are not active/not assigned to a company, removed contracts that as expired before 01.01.2018, those not linked to any company (“Not operated”), and contracts with overlap area smaller than 5 km2 with an IFL. For mining claims, we include all claims with the status of “Granted” and “Application.” We removed claims with the status of “Not operated” or not linked to any company, registered as expired before 01.01.2018 or had an overlap area smaller than 1 km2 with IFLs. Results were then combined to provide an overall summary of extractive activities overlapping IFLs. Maps were generated using these filtered results. There were a few places that oil and gas contracts and mining claims overlap. This is taken into account in the combined statistics to avoid double counting.
Analysis
The spatial overlap analysis was conducted using ArcGIS 10.7 accessing the World Wildlife Fund (WWF)-Sight server2, which is a platform developed by WWF that provides a global overview of major sectoral developments (e.g., extractives) against major environmental assets (e.g., Intact Forest Landscapes) in order to monitor and understand the environmental and social implications of human development around the world.
Results
Within the study regions, there are ∼5.2 million km2 of IFLs, of which 74% in South America, 16% in Central Africa, and 10% in Asia-Pacific (Table 1). Within these tropical IFLs, there are 16,000+ oil and gas contracts and mining claims covering 19% of IFL area (975,000 km2), with oil and gas contracts covering 408,000 km2 (8%), mining claims covering 590,000 km2 (11%), and a small area covered by overlapping claims (Table 1). Central Africa region has the highest share of IFLs overlapping with oil and gas contracts, with ∼ 26% of IFL covered by oil and gas contracts, followed by Asia-Pacific (18%) and South America (3%) (Table 1). Central Africa is again the region with the highest share of IFLs overlapping with mining claims with 16.5% of IFL covered by mining claims, followed by Amazon (11%), and Asia-Pacific (9%) (Table 1). Measured by areas, South America has the highest overlap with 421,746 km2, followed by Central Africa (142,840 km2) and Asia-Pacific (46,704 km2) (Table 1). Brazil has the highest overlap of extractives and IFLs primarily due to mining, and DRC is second with a mixture of oil and gas and mining (Figure 2). Oil extraction on its own is the biggest IFL overlap driver within the oil and gas sector (Figure 3), and gold is the biggest driver for mining (Figure 4). We found overwhelmingly that both oil and gas contracts and mining leases that overlap with IFLs are in the exploration stage (Figures 5, 6).
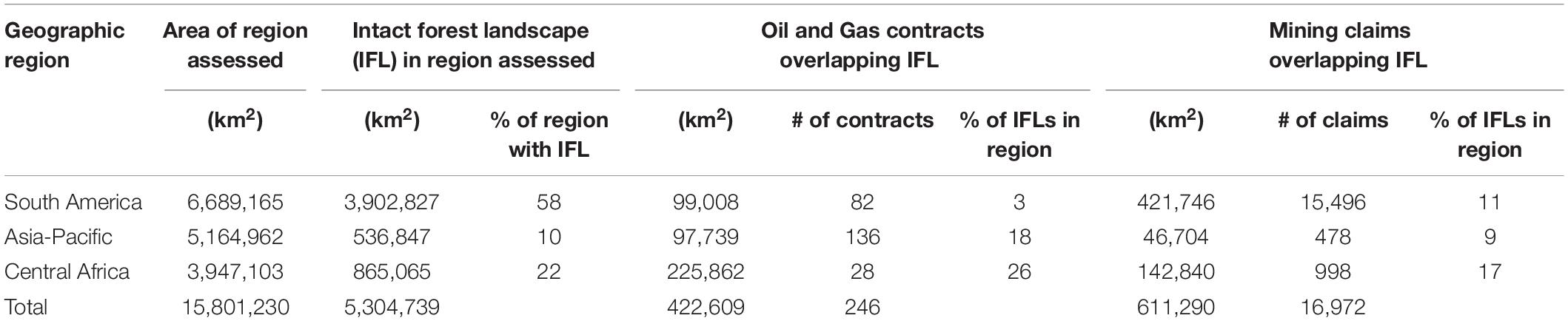
Table 1. A summary of the extent and both Oil and Gas contracts and Mining claims overlapping with IFLs for each region and overall.
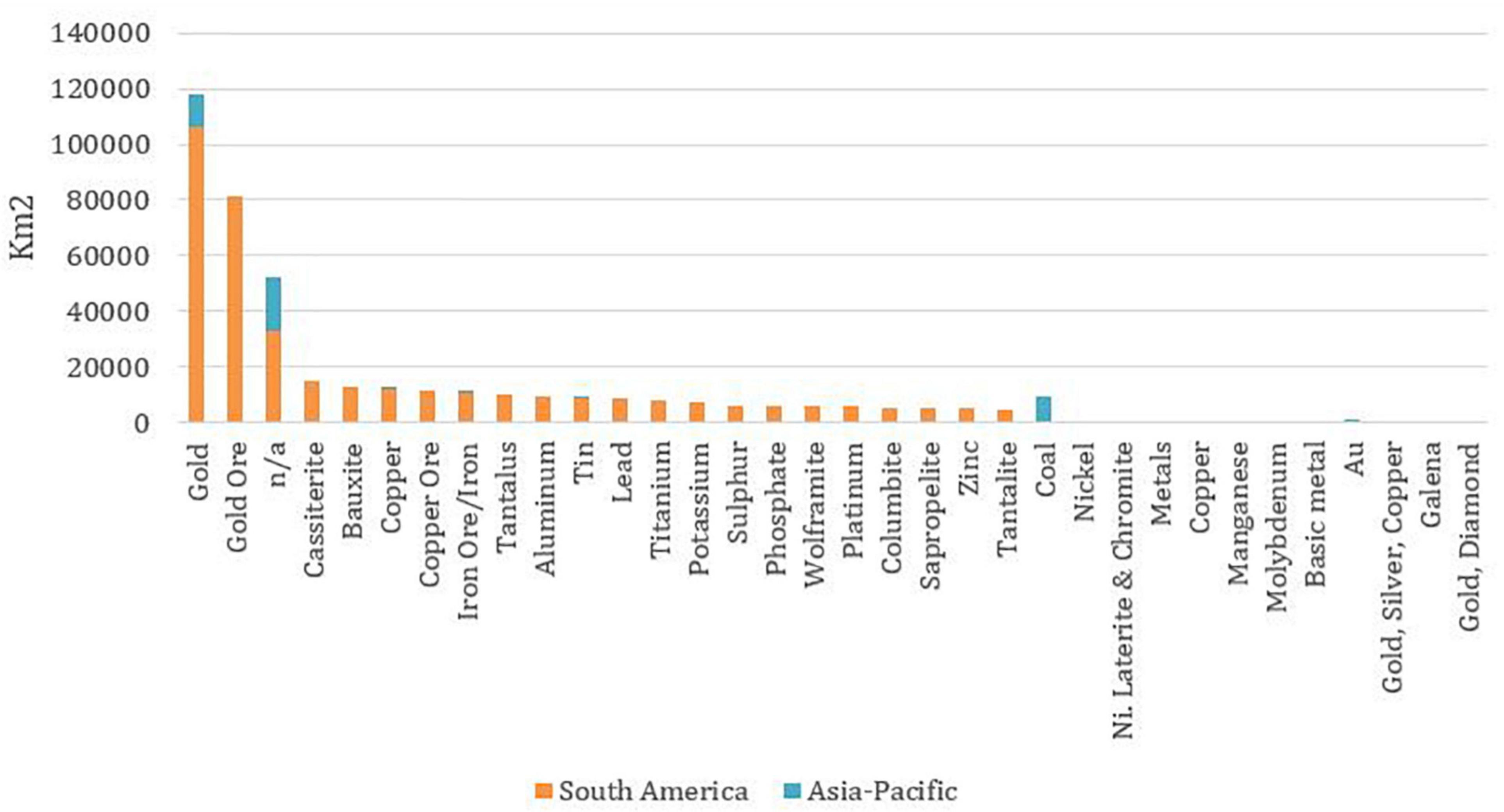
Figure 4. The type of mining commodity where mining claims overlap IFLs. Central Africa is missing as the data was unclear and could not be reasonably summarized.
South America
There are ∼4 million km2 of IFLs in the South America study region, covering 58% of the region’s land area (Table 1). The total overlap of oil and gas contracts and IFLs is ∼95,500 km2, which includes 82 contracts and covers 2.5% of IFLs (Table 1). Oil and gas contracts within IFLs are concentrated in the western part of the region and in the central Amazon (Figure 1). The country with the highest proportional overlap is Bolivia, followed by Colombia, then Peru (Figure 2). Combined oil and gas contracts are the most common overlap, consisting of 33 contracts covering ∼40,000 km2 (Figure 3). Around half of these contracts are at the stage of exploration and production, and one-fifth are reserved by the governments for potential future exploration (Figure 5).
There is a total of 589,000 Km2 of overlap between IFLs and mining claims in this region, which consists of 15,832 claims covering nearly 11% of IFLs (Table 1), with most claims located in the eastern part of the region (Figure 1). Mining claim overlaps are overwhelmingly within Brazil (∼370,000 km2, 6,483 contracts), followed by Guyana (29,000 km2, 7,285) (Figure 1). The country with the highest number of contracts overlapping with IFLs is Guyana (7,285), followed by Brazil, which makes up the majority of all the contracts, and are scattered across the region (Figures 1, 2). The type of mining is mainly for gold (Figure 4), and the vast majority of these mining claims are at the exploration permit stage (just above 89%, ∼364,000 km2), followed by exploration lease/licence stage (Figure 6).
Asia-Pacific
There are nearly 510,000 km2 of IFL within the Asia-Pacific study region, which covers 10% of the land area within the region assessed. Within this region, oil and gas contracts overlap with IFLs by 18% or 91,000 km2 (Table 1), mainly found in New Guinea and its offshore islands and significant overlaps on the island of Borneo and northwestern Myanmar (Figure 1). The country with the highest overlap is Papua New Guinea (PNG) with over 73,000 km2, followed by Indonesia with 8,000 km2, and Myanmar with 7,500 km2 (Figure 2). Of the 134 contracts overlapping IFLs, the majority are combined oil and gas, with 76 contracts covering 47,000 km2 (52%) of overlap area (Figure 3). The remainder is mostly gas-only, with 39 contracts covering 41,000 km2 of overlap area (Figure 3). The majority of all contracts (87 out of 133) are at the exploration stage (Figure 5), with only 9% of overlap area (82,000 km) currently licensed either for production or for exploration/production (Figure 5).
The highest overlap of mining claims and IFLs is within New Guinea and its offshore islands. The country with the highest overlap is Indonesia with over 23,000 km2, followed by PNG with over 15,000 km2 (Figure 3). The mining claim commodity with the highest overlap is “unknown” for most, followed by gold (11,500 km2), and coal (∼ 9,000 km2) (Figure 4). The stage of mining is mostly exploration lease/license with just over 43,000 km2 (Figure 6).
Central Africa
Central Africa has ∼840,000 km2 of IFLs, of which ∼ 221,000 km2 is covered by 27 oil and gas contracts (26% of the IFL area) (Table 1), which are mostly in the center of the region (Figure 1). DRC accounts for 82% of overlap, covering around 182,000 km2 (Figure 2). The country with the second highest overlap by area is the Republic of Congo, covering around 36,000 km2 (Figure 2). The majority of contracts are for oil (17 contracts covering 81.5% of the overlap area, 180,000 km2) (Figure 4). Combined oil and gas contracts cover a much smaller area of 42,000 km2 of overlap (Figure 4). Almost all of these contracts are in the exploration stage (Figure 5), with only 5 contracts currently allowing some level of production (Figure 5).
The overlap between IFLs and mining claims in the region cover ∼139,000 km2 and include 988 contracts covering 16.5% of the overlap area (Table 1), mostly in the eastern part (Figure 1). DRC again accounts for the majority (84%) of total overlap with ∼118,500 km and has nearly all the contracts that overlap (991 out of 1,038) (Figure 2). The remaining mining claims are distributed in Gabon, followed by the Republic of Congo (Figure 2). Within this region, the combinations of gold and diamond cover just over 50% of the overlap area (Figure 4). The production stage of most mining claims is not clear, with almost all classified as “other” (Figure 6). These “other” claims make up nearly 80% of the IFL overlap area (111,000 km2), followed by exploration lease/license with just over 20,000 km2 (15%; Figure 6).
Discussion
Our results show that the extractive sector now poses a significant threat to tropical IFLs, with nearly a fifth of tropical IFL area (975,000 km2) currently designated as some form of extractives concession. Mining claims cover 590,000 km2 (11%), while oil and gas contracts cover 408,000 km2 (8%), and a small area is covered by overlapping contracts and claims. These results are concerning, as extractive industries have historically been a major driver of IFL loss, especially in northern latitudes (e.g., Canada) and in Latin America, where extractive projects are widespread (Potapov et al., 2017). With recent research showing that mining-driven deforestation occurs up to 70 km beyond mining lease boundaries (Sonter et al., 2017), and other studies predicting substantial impacts on biodiversity from extractive projects in tropical regions (Butt et al., 2013), our results show that there is a significant potential threat of further tropical IFL loss due to the extractive sector and this is likely at the early stages given most companies are at the exploration stage.
While our data shows large overlaps between extractive concessions and tropical IFLs, any mining operation’s direct footprint (e.g., drill pads, roads) is likely to be far smaller than the total concession area. For example, Maus et al. (2020) predict the global mining footprint at around 57,000 km2, which in itself is an order of magnitude smaller than the mining claims within this analysis. However, it is clear that the indirect impacts of extractive projects almost always extend far beyond their direct footprint (Suárez et al., 2009; Sonter et al., 2018; Johnson et al., 2020). For example, roads or pipelines can attract human populations to previously remote areas, driving new threats to biodiversity (Sonter et al., 2017), or exacerbate pre-existing threats such as over-exploitation (e.g., hunting, fishing), invasive species, and habitat loss (Sonter et al., 2018). Recent research shows that seismic survey lines for resource exploration are becoming a key driver of habitat fragmentation in North American boreal forests (Dabros et al., 2018) and eastern Africa (UNEP-WCMC, 2011). Furthermore, pollutants generated by mining projects can travel vast distances, especially within river systems (Hettler et al., 1997). Concerningly, exploration phase activities are not usually subject to the same level of regulatory licensing and Environmental and Social Impact Assessment (ESIA) as production phase activities. As such, protecting IFLs will require environmental assessments and licensing to consider adequately both on-lease and off-lease impacts on biodiversity, as well as the impacts of pre-production exploration activities early in project development.
When assessing the impacts of extractive projects on IFLs, it is critical to consider that the impacts of a single project cannot be measured in isolation. We found that concessions tend to concentrate in particular areas, for example right in the middle of the Central African region (Figure 1). When numerous development projects and other human pressures occur within a region, cumulative impacts may be overlooked if concession applications are considered individually (Whitehead et al., 2017). This is particularly true if developments are from different sectors (Raiter et al., 2014). Within many of the IFLs and countries assessed here, cumulative impacts are already occurring, with ongoing forest loss and degradation due to infrastructure, industrial logging, poaching and agriculture (Lane et al., 2012; Barber et al., 2014; Benítez-López et al., 2017; Laurance, 2018). New extractives operations within IFLs might risk opening the forest frontier and lead to other industries moving in causing further impact to these forests (Molinario et al., 2020). Protecting IFLs from cumulative impacts will therefore require careful multi-sectoral and multi-stakeholder planning of infrastructure, which can be informed by conservation planning approaches and tools that consider cumulative impacts (e.g., Whitehead et al., 2017).
Many of the extractive projects that threaten IFLs will likely be required to apply the mitigation hierarchy, a decision-making framework designed to address the environmental impacts of development projects through first seeking to avoid impacts wherever possible, then minimizing or restoring impacts, and finally by offsetting any unavoidable impacts (Business and Biodiversity Offsets Programme [BBOP], 2012; Ekstrom et al., 2015). For any development project, but especially those likely to affect IFLs, the avoidance phase of the mitigation hierarchy will be most crucial as IFLs are typically old growth forests that can take thousands of years to recover and are therefore not offsetable (Jones et al., 2018; Watson et al., 2018a). Effective avoidance planning must take place during the project design phase, so that information on the most important intact forest areas can inform project design. Ideally, coordination with and preferably by governments will allow for landscape scale planning, including Strategic Environmental Assessments that consider numerous projects and environmental values at once (Whitehead et al., 2017). While IFLs are recognized as an important avoidance value in some industry and financial lending environment safeguards (e.g., Forest Stewardship Council, national import policy on supply chains), there is a need for them to be more widely recognized directly (e.g., International Finance Corporation, World Bank). Finally, where possible we encourage governments not to allocate extractives concessions within IFLs where possible to avoid potential impacts in these locations in the future.
There is still time to act to ensure future extractive efforts can minimize harm. We found that the majority of extractive concessions that overlap with IFLs are at the exploration stage, meaning the mitigation hierarchy can still be used to avoid or minimize the impacts of any projects that arise from these exploration leases. However, it is crucial to recognize that there are limitations to the effectiveness of the mitigation hierarchy, especially with regard to IFLs and the great difficulties of offsetting impacts on them. For example, it is almost impossible to restore the myriad values of intact forests once they are lost (Lamb et al., 2005; Crouzeilles et al., 2016), and the offsetability of intact forests is also debated given their irreplaceability for biodiversity (Watson et al., 2018a). Given that there is a time series on IFL distribution (Potapov et al., 2017) and likely future updates, future work will be able to better understand the dynamics and effectiveness mitigation strategies to reduce the impacts of extractives on IFLs.
While we found considerable overlap between extractive concessions and tropical IFLs, our results are affected by a number of limitations. The concessions data is not publicly available making it difficult for others to use and assess the accuracy of the data. We were unable to obtain extractive concession data for all countries within the regions of focus (see methods for a list of missing data), which means the true overlap between concessions and IFLs is likely higher than we estimate. Concession data may not be up-to-date within the countries assessed with updates variable (e.g., DRC updates around 6 months, Australia every week) and there were some temporal differences between the datasets. We were also only able to assess commercial extractive concessions and did not consider artisanal or illegal resource extraction activities, which can have a fairly large footprint (e.g., Matthysen et al., 2019; Molinario et al., 2020) and also cause substantial and widespread impacts on biodiversity (Asner et al., 2013). Finally, it is important to note that despite the large areal overlap between extractive concessions and IFLs, only a small fraction of this total area is ever likely to be explored or developed for production. However, despite being limited in area, development projects and their linear infrastructure often drive indirect impacts which extend far beyond the project footprint, and also cause increased fragmentation of intact areas. Indeed, fragmentation from logging, infrastructure projects, and fire was responsible for 86% of IFL loss between 2001 and 2013 (Potapov et al., 2017).
Conclusion
This study is the first to combine data on tropical IFLs with a novel extensive dataset on extractives. The extractives sector is an important economic development driver in many less-developed countries, but with this study finding around 20% of tropical IFLs being designated with extractives concessions, it poses a significant potential hurdle for the conservation community to achieve its global climate and biodiversity ambition. It is not possible to restore or offset IFLs, so they are only being lost in their extent globally with dire ramifications for biodiversity and ecosystem services like climate mitigation, which consequently is a loss of economic gains from maintaining these forests (e.g., finance through REDD+). Our analysis shows there is an urgency, and opportunity, to address the risk the extractive sector has on IFLs given that the majority of concessions are at the exploratory stage providing the opportunity to mitigate potential impacts before they occur at the avoidance stage, which is particularly important given it is unlikely that IFLs anywhere can be offset. Careful planning of extractive projects by both governments and the private sector could lead to the successful implementation of the mitigation hierarchy, landscape-level planning of infrastructure and other associated developments with projects, such as housing and agriculture, strategic environmental assessment and identification of avoidance and no-go zones. Financial lending safeguards and industry environmental safeguards need to be updated to better account for intact forests values, as currently this is missing within most of them.
Data Availability Statement
We are not able to share the extractives data given contractual obligations to data custodians. Intact Forest Landscapes data can be downloaded at http://www.intactforests.org.
Author Contributions
PT and PI concieved the idea. HG, PT, and PI analyzed the data. HG and KJ drafted the text. All authors listed have made a substantial, direct and intellectual contribution to the work, and approved it for publication.
Funding
This study was funded by IKEA via WWF’s forest practice and undertaken in partnership with WCS.
Conflict of Interest
The authors declare that the research was conducted in the absence of any commercial or financial relationships that could be construed as a potential conflict of interest.
The handling editor declared a past co-authorship with the authors HG and JW.
Footnotes
References
Asner, G. P., Llactayo, W., Tupayachi, R., and Luna, E. R. (2013). Elevated rates of gold mining in the Amazon revealed through high-resolution monitoring. Proc. Natl. Acad. Sci. U.S.A. 110, 18454–18459. doi: 10.1073/pnas.1318271110
Barber, C. P., Cochrane, M. A., Souza, C. M., and Laurance, W. F. (2014). Roads, deforestation, and the mitigating effect of protected areas in the Amazon. Biol. Conserv. 177, 203–209. doi: 10.1016/j.biocon.2014.07.004
Benítez-López, A., Alkemade, R., Schipper, A. M., Ingram, D. J., Verweij, P. A., Eikelboom, J. A. J., et al. (2017). The impact of hunting on tropical mammal and bird populations. Science 356, 180–183. doi: 10.1126/science.aaj1891
Business and Biodiversity Offsets Programme [BBOP], (2012). Standard on Biodiversity Offsets. Washington, DC: Business and Biodiversity Offsets Programme.
Butt, N., Beyer, H. L., Bennett, J. R., Biggs, D., Maggini, R., Mills, M., et al. (2013). Biodiversity risks from fossil fuel extraction. Science 342, 425–426. doi: 10.1126/science.1237261
Crouzeilles, R., Curran, M., Ferreira, M. S., Lindenmayer, D. B., Grelle, C. E. V., and Rey Benayas, J. M. (2016). A global meta-analysis on the ecological drivers of forest restoration success. Nat. Commun. 7:11666. doi: 10.1038/ncomms11666
Dabros, A., Pyper, M., and Castilla, G. (2018). Seismic lines in the boreal and arctic ecosystems of North America: environmental impacts, challenges, and opportunities. Environ. Rev. 26, 214–229. doi: 10.1139/er-2017-0080
Ekstrom, J., Bennun, L., and Mitchell, R. (2015). A Cross-Sector Guide for Implementing the Mitigation Hierarchy. Cambridge: The Biodiversity Consultancy.
Finer, M., and Orta-Martínez, M. (2010). A second hydrocarbon boom threatens the Peruvian Amazon: trends, projections, and policy implications. Environ. Res. Lett. 5:014012. doi: 10.1088/1748-9326/5/1/014012
Harfoot, M. B. J., Tittensor, D. P., Knight, S., Arnell, A. P., Blyth, S., Brooks, S., et al. (2018). Present and future biodiversity risks from fossil fuel exploitation. Conserv. Lett. 11:e12448. doi: 10.1111/conl.12448
Hettler, J., Irion, G., and Lehmann, B. (1997). Environmental impact of mining waste disposal on a tropical lowland river system: a case study on the Ok Tedi Mine, Papua New Guinea. Mineral. Deposita 32, 280–291. doi: 10.1007/s001260050093
Houghton, R. A. (2013). The emissions of carbon from deforestation and degradation in the tropics: past trends and future potential. Carbon Manag. 4, 539–546. doi: 10.4155/cmt.13.41
Johnson, C. J., Venter, O., Ray, J. C., and Watson, J. E. M. (2020). Growth-inducing infrastructure represents transformative yet ignored keystone environmental decisions. Conserv. Lett. 13:e12696. doi: 10.1111/conl.12696
Jones, H. P., Jones, P. C., Barbier, E. B., Blackburn, R. C., Rey Benayas, J. M., Holl, K. D., et al. (2018). Restoration and repair of Earth’s damaged ecosystems. Proc. R. Soc. B Biol. Sci. 285:20172577. doi: 10.1098/rspb.2017.2577
Kleinschroth, F., Laporte, N., Laurance, W. F., Goetz, S. J., and Ghazoul, J. (2019). Road expansion and persistence in forests of the Congo Basin. Nat. Sustain. 2, 628–634. doi: 10.1038/s41893-019-0310-6
Kormos, C. F., Badman, T., Bertzky, B., Jaeger, T., Larsen, P. B., van Merm, R., et al. (2017). World Heritage, Wilderness and Large Landscapes and Seascapes. Gland: International Union for Conservation of Nature.
Lamb, D., Erskine, P. D., and Parrotta, J. A. (2005). Restoration of degraded tropical forest landscapes. Science 310, 1628–1632. doi: 10.1126/science.1111773
Lane, J. E., Kruuk, L. E. B., Charmantier, A., Murie, J. O., and Dobson, F. S. (2012). Delayed phenology and reduced fitness associated with climate change in a wild hibernator. Nature 489, 554–557.
Laurance, W. F. (2018). Conservation and the global infrastructure tsunami: disclose, debate, delay!. Trends Ecol. Evol. 33, 568–571. doi: 10.1016/j.tree.2018.05.007
Lehner, B., and Grill, G. (2013). Global river hydrography and network routing: baseline data and new approaches to study the world’s large river systems. Hydrol. Process. 27, 2171–2186. doi: 10.1002/hyp.9740
Lessmann, J., Fajardo, J., Muñoz, J., and Bonaccorso, E. (2016). Large expansion of oil industry in the Ecuadorian Amazon: biodiversity vulnerability and conservation alternatives. Ecol. Evol. 6, 4997–5012. doi: 10.1002/ece3.2099
Matthysen, K., Spittaels, S., and Schouten, P. (2019). Mapping Artisanal Mining Areas and Mineral Supply Chains in Eastern DR Congo. Antwerp: International Peace Information Service, 288–295.
Maus, V., Giljum, S., Gutschlhofer, J., da Silva, D. M., Probst, M., Gass, S. L. B., et al. (2020). A global-scale data set of mining areas. Sci. Data 7:289. doi: 10.1038/s41597-020-00624-w
Maxwell, S. L., Evans, T., Watson, J. E. M., Morel, A., Grantham, H., Duncan, A., et al. (2019). Degradation and forgone removals increase the carbon impact of intact forest loss by 626%. Sci. Adv. 5:eaax2546. doi: 10.1126/sciadv.aax2546
Mittermeier, R. A., Mittermeier, C. G., Brooks, T. M., Pilgrim, J. D., Konstant, W. R., and Fonseca, G. A. B. (2003). Wilderness and biodiversity conservation. Proc. Natl. Acad. Sci. U.S.A. 100, 10309–10313. doi: 10.1073/pnas.1732458100
Molinario, G., Hansen, M., Potapov, P., Tyukavina, A., and Stehman, S. (2020). Contextualizing landscape-scale forest cover loss in the Democratic Republic of Congo (DRC) between 2000 and 2015. Land 9:23.
Newbold, T., Hudson, L. N., Hill, S. L. L., Contu, S., Lysenko, I., Senior, R. A., et al. (2015). Global effects of land use on local terrestrial biodiversity. Nature 520, 45–50. doi: 10.1038/nature14324
Pan, Y., Birdsey, R. A., Fang, J., Houghton, R., Kauppi, P. E., Kurz, W. A., et al. (2011). A large and persistent carbon sink in the world’s forests. Science 333, 988–993. doi: 10.1126/science.1201609
Parish, E. S., Kline, K. L., Dale, V. H., Efroymson, R. A., McBride, A. C., Johnson, T. L., et al. (2013). Comparing scales of environmental effects from gasoline and ethanol production. Environ. Manage. 51, 307–338. doi: 10.1007/s00267-012-9983-6
Potapov, P., Hansen, M. C., Laestadius, L., Turubanova, S., Yaroshenko, A., Thies, C., et al. (2017). The last frontiers of wilderness: tracking loss of intact forest landscapes from 2000 to 2013. Sci. Adv. 3:e1600821. doi: 10.1126/sciadv.1600821
Potapov, P., Yaroshenko, A., Turubanova, S., Dubinin, M., Laestadius, L., Thies, C., et al. (2008). Mapping the world’s intact forest landscapes by remote sensing. Ecol. Soc. 13:51. doi: 10.5751/ES-02670-130251
Raiter, K. G., Possingham, H. P., Prober, S. M., and Hobbs, R. J. (2014). Under the radar: mitigating enigmatic ecological impacts. Trends Ecol. Evol. 29, 635–644. doi: 10.1016/j.tree.2014.09.003
Ripple, W. J., Estes, J. A., Beschta, R. L., Wilmers, C. C., Ritchie, E. G., Hebblewhite, M., et al. (2014). Status and ecological effects of the world’s largest carnivores. Science 343:1241484. doi: 10.1126/science.1241484
Sheil, D., and Murdiyarso, D. (2009). How forests attract rain: an examination of a new hypothesis. BioScience 59, 341–347. doi: 10.1525/bio.2009.59.4.12
Silva Rotta, L. H., Alcântara, E., Park, E., Negri, R. G., Lin, Y. N., Bernardo, N., et al. (2020). The 2019 Brumadinho tailings dam collapse: possible cause and impacts of the worst human and environmental disaster in Brazil. Int. J. Appl. Earth Obs. Geoinf. 90:102119. doi: 10.1016/j.jag.2020.102119
Sonter, L. J., Ali, S. H., and Watson, J. E. M. (2018). Mining and biodiversity: key issues and research needs in conservation science. Proc. R. Soc. B Biol. Sci. 285:20181926. doi: 10.1098/rspb.2018.1926
Sonter, L. J., Herrera, D., Barrett, D. J., Galford, G. L., Moran, C. J., and Soares-Filho, B. S. (2017). Mining drives extensive deforestation in the Brazilian Amazon. Nat. Commun. 8:1013. doi: 10.1038/s41467-017-00557-w
Suárez, E., Morales, M., Cueva, R., Bucheli, V. U., Zapata-Ríos, G., Toral, E., et al. (2009). Oil industry, wild meat trade and roads: indirect effects of oil extraction activities in a protected area in north-eastern Ecuador. Anim. Conserv. 12, 364–373. doi: 10.1111/j.1469-1795.2009.00262.x
Sutton, P. C., Anderson, S. J., Costanza, R., and Kubiszewski, I. (2016). The ecological economics of land degradation: impacts on ecosystem service values. Ecol. Econ. 129, 182–192. doi: 10.1016/j.ecolecon.2016.06.016
UNEP-WCMC (2011). Selous Game Reserve. UNEP-WCMC World Heritage Information Sheets. Cambridge: UNEP-WCMC.
Watson, J. E. M., Evans, T., Venter, O., Williams, B., Tulloch, A., Stewart, C., et al. (2018a). The exceptional value of intact forest ecosystems. Nat. Ecol. Evol. 2, 599–610. doi: 10.1038/s41559-018-0490-x
Watson, J. E. M., Venter, O., Lee, J., Jones, K. R., Robinson, J. G., Possingham, H. P., et al. (2018b). Protect the last of the wild. Nature 563, 27–30. doi: 10.1038/d41586-018-07183-6
Keywords: intact forest landscape, mining, emerging threat, oil and gas, spatial analysis, mining commodity, forest
Citation: Grantham HS, Tibaldeschi P, Izquierdo P, Mo K, Patterson DJ, Rainey H, Watson JEM and Jones KR (2021) The Emerging Threat of Extractives Sector to Intact Forest Landscapes. Front. For. Glob. Change 4:692338. doi: 10.3389/ffgc.2021.692338
Received: 08 April 2021; Accepted: 26 May 2021;
Published: 16 July 2021.
Edited by:
Peter Potapov, University of Maryland, College Park, United StatesReviewed by:
Giuseppe Molinario, World Bank Group, United StatesClaudia Azevedo-Ramos, Federal University of Pará, Brazil
Copyright © 2021 Grantham, Tibaldeschi, Izquierdo, Mo, Patterson, Rainey, Watson and Jones. This is an open-access article distributed under the terms of the Creative Commons Attribution License (CC BY). The use, distribution or reproduction in other forums is permitted, provided the original author(s) and the copyright owner(s) are credited and that the original publication in this journal is cited, in accordance with accepted academic practice. No use, distribution or reproduction is permitted which does not comply with these terms.
*Correspondence: Hedley S. Grantham, hgrantham@wcs.org