- 1Department of Botany, Govt. Vijay Bhushan Singh Deo Girls PG College, Jashpur, India
- 2Department of Botany, Guru Ghasidas Vishwavidyalaya, Bilaspur, India
- 3Botany Department, Deen Dayal Upadhyay Gorakhpur University, Gorakhpur, India
- 4Center for Integrative Conservation, Xishuangbanna Tropical Botanical Garden, Mengla, China
Due to overexploitation for forest resources, many important medicinal plants are disappearing from the tropical dry forest (TDF) regions. The present study is aimed to investigate the diversity of medicinal plants exposed to different levels of anthropogenic disturbance in TDFs. For this study, we selected six forest regions, covering a radius of 25 km, and exposed to variable intensity of disturbances, in the form of illegal logging, cattle grazing and fire. Based on the intensity of three disturbances, we categorized the study sites as low (LDS) and high (HDS) disturbed sites, and analyzed differences in soil properties and vegetation parameters in the two disturbance categories. We recorded tree density, tree species richness and herbaceous species richness in 100 m2 quadrats at the selected regions. Results exhibited significantly greater soil organic C (LDS, 4.6%; HDS, 0.9%; P < 0.001), water holding capacity (LDS, 47.4%; HDS, 33.6%; P < 0.001), and sand content (LDS, 53.7%; HDS, 48.3%; P < 0.001) across LDS, whereas significantly higher bulk density (LDS, 1.30 gcm–3; HDS, 1.34 gcm–3; P < 0.05) and gravel content (LDS, 14.6%; HDS, 20.0%; P < 0.001) across HDS. We found considerable shift in relationships between vegetation parameters under the two disturbance regimes. The results indicated that many of the important medicinal plants (e.g., Adina cordifolia, Bauhinia vahlii, Holarrhena antidysenterica, Asparagus racemosus, Curculigo orchioides, Hemidesmus indicus, etc.) which are potential constituents of several ayurvedic preparations as well as of traditional medical systems are lacking from the HDS, either due to direct impact of disturbances or indirectly due to disturbance induced changes in soil properties. For instance, the high grazing pressure increased soil compaction, leading to increase in bulk density and decline in water holding capacity of soil. We suggest that forest managers take into consideration the presence of anthropogenic disturbances in their management and conservation efforts of medicinal plants in TDFs.
Introduction
India accounts for 8% of the world’s total biodiversity (Shaikh and D Parveen, 2017), and is among 12 megadiversity countries, with 38.2% of tropical dry forest (TDF) cover (MoEF, 1999; Singh and Chaturvedi, 2017). TDFs across the globe are potentially rich in biodiversity, however these forests are the less protected ecosystems, facing high degree of disturbances, leading to considerable loss in species richness (Rivas et al., 2020; Jhariya and Singh, 2021a,b,c). These forests are known to harbor numerous medicinal plants (Rosero-Toro et al., 2018), which are currently disappearing at alarming rates due to natural and/or man made disturbances, and overexploitation of forest resources (Shanley and Luz, 2003; Prajapati et al., 2018). Forest conversion and alteration in species composition are expected to be governed by many factors (Klinge et al., 1995; Wittmann and Junk, 2003), although prominent among these are the anthropogenic disturbances, playing crucial role in modification of canopy structure, and thus significantly affecting ecosystem processes (Chaturvedi et al., 2017a,2021). Studies in TDFs have shown that the anthropogenic forest disturbances negatively affect the forest flora and fauna, ultimately resulting into loss of biological diversity (Rai and Chakrabarti, 2001; Ramírez-Marcial et al., 2001; Arjunan et al., 2005; Shahabuddin and Kumar, 2007). Among anthropogenic disturbances, land use change is one of the important factors influencing biodiversity of TDFs, for instance, the forests near human settlements are under continuous threat due to unsustainable resource use and clearing of forest for agriculture (Trejo and Dirzo, 2000). Moreover, severity of forest disturbances is also due to the absence of adequate recovery phase required for the regeneration of plants or ecosystems experiencing continuous anthropogenic disturbances (Singh, 1998).
Tropical dry forest exhibits a relatively suitable climate and soil for supporting agriculture, which attracts high density of human population (Sánchez-Azofeifa and Portillo-Quintero, 2011). However, it also brings a wide range of disturbances of variable frequency, intensity and duration, which leads to forest degradation and shift in species composition (Chaturvedi et al., 2017a). Besides a long dry period and occasional wildfires, TDFs are highly threatened by human induced disturbances such as harvesting and cattle grazing. TDFs exhibit a wide variety of landscapes, with a continuum of disturbances (or environmental drivers) and plant survival strategies (Chaturvedi et al., 2021). At one end, the TDF landscapes, such as moist forests tend to absorb or dampen the spread of disturbances, while at the other end, the landscapes, such as dry forests, fragmented or young forests magnify the spread of disturbances (Chaturvedi et al., 2017a). During the dry season, forest floor in TDFs are loaded with dry litter which act as fuel for wildfires causing mortality of many plant species which do not have defense mechanisms against fire (Markesteijn and Poorter, 2009). Disturbances such as fire and grazing usually lead to mortality of small sized plants, which do not have protection against these drivers, while mortality of large trees is mostly due to harvesting by humans (Eriksson et al., 2003; Chaturvedi et al., 2017a). Studies have reported top-killing of herbs and shrubs even due to low intensity of surface fires (Morgan and Neuenschwander, 1988). However, despite the loss of aboveground parts, plants growing in fire-prone ecosystems have evolved strategies, such as resprouting, seed heat tolerance and fire stimulated germination, which allow their persistence in such conditions (Paula and Pausas, 2008). In TDfs, many understory species also possess capacity of vegetative regeneration from the surviving buds on plant organs, either located on soil surface (e.g., stolons), or belowground litter and mineral soils (e.g., roots, rhizomes, bulbs, corms, and lignotubers) (Paula et al., 2009; Stephan et al., 2010).
The continued degradation of forests, and changes in the structural attributes of overstory trees highly influences the diversity of understory plants including medicinally important plant species (Gilliam, 2007; Wyatt and Silman, 2010). In fact, the above ecological concepts relating biodiversity and vegetation structure with ecosystem processes, although have been developed, these are not known to common people. In the modern world, conservation of medicinal plants and their sustainable utilization is lagging behind due to lack of ecological awareness among the people (McGeoch et al., 2008). Therefore, community awareness programs should be organized to inculcate the idea of sustainable use of forest resources (Singh et al., 2010; Jhariya et al., 2019).
During the last couple of decades, assessment of medicinal plant diversity in response to anthropogenic disturbance has been conducted by different workers across the globe (e.g., Shanley and Luz, 2003; McGeoch et al., 2008; Adnan and Hölscher, 2012; Adnan et al., 2014; Shaikh and D Parveen, 2017). In India, a lot of work has been conducted in TDFs regarding the impact of anthropogenic disturbances on ecosystem processes, nutrient dynamics, species diversity, carbon sequestration, and functional traits (Singh et al., 1989, 1991; Singh and Singh, 1991, Singh and Singh, 1992; Lal et al., 2001; Sagar et al., 2003a,b, 2008; Saha and Howe, 2003; Chaturvedi et al., 2011a,b, Chaturvedi et al., 2012, 2017a,2017b,2021, 2013; Chaturvedi and Raghubanshi, 2014, 2015), however specific study focusing on the diversity of medicinal plants in these forests is still lacking. The present study is aimed to investigate the diversity of medicinal plants in a TDF exposed to different levels of anthropogenic disturbance.
In the Indian subcontinent, around 350 million rural population and 84% of the ethnic communities living in and/or around forests (Mehta and Shah, 2003), still depend on the forest resources for their livelihood (Poffenberger and McGean, 1996; Hegde and Enters, 2000; Kutty and Kothari, 2001; Harris and Mohammed, 2003). These human settlements generally follow traditional medical practices, and highly depend on medicinal plants growing in nearby forests. WHO has listed more than 21,000 medicinal plants which are in use across the globe, particularly in developing countries, where around 80% people still rely on traditional medicines for the treatment of various ailments (WCMC, 1992). According to an estimate, about 15–30% of household income of the poor people in developing countries comes from the forest resources, and medicinal plants are the significant source in this direction (Hamilton, 2004). Particularly, due to lack of health facilities, and also limited purchasing capacity, for most of the rural communities, herbal based medicines are an alternative to the modern allopathic medicines (Adnan et al., 2015). The present work was conducted in Jashpur, a human tribe dominating district of Chhattisgarh with 62.27% of tribal population (2011 census). The land area of the district accounts for 42% of forest cover and majority of the human population still depend on forest resources for their livelihood (Painkra et al., 2015). Subsequently, the use of forest resources unsustainably has resulted into the forest fragmentation and loss of biological diversity in the forest region. According to local tribes, this forest region is potentially rich in medicinal plants, and it has considerable impact on their livelihood. However, systematic evaluation of the anthropogenic pressure on these forests is still lacking. Therefore aim of the present work is to evaluate the impact of anthropogenic disturbance on the diversity of medicinal plants on the selected forest sites of the region.
Materials and Methods
Study Site
The proposed work was conducted in a dry tropical forest of Jashpur district (22°17′ and 23°15′N and 83°30′ and 84°24′E) of Chhattisgarh with a geographical area of 6,205 km2. The forest is tropical dry deciduous type dominated by Shorea robusta. The climate is monsoon type with 3–4 months of critical dry periods. For this study, we selected six forest regions, covering a radius of 25 km. The idea behind the selection of these forest regions was to get a gradient of disturbance, mainly determined by the density of trees and visual observations of the intensity of disturbances in the form of logging, cattle grazing and fire scars. The selected forest regions are: (1) Chandan Tongari (22°55.59′N and 84°7.36′E, 814 m.a.s.l.), (2) Bel Pahar-1 (22°53.34′N and 84°7.46′E, 894 m.a.s.l.), (3) Luikona-1 (22°52.38′N and 84°5.89′E, 808 m.a.s.l.), (4) Marga (22°55.58′N and 84°7.88′E, 801 m.a.s.l.), (5) Bel Pahar-2 (22°53.43′N and 84°7.49′E, 867 m.a.s.l.), and (6) Luikona-2 (22°52.42′N and 84°5.91′E, 805 m.a.s.l.). Based on our preliminary survey, Chandan Tongari, Bel Pahar-1 and Luikona-1 were considered as low disturbed forest regions, while Marga, Bel Pahar-2 and Luikona-2 were listed as high disturbed forest regions. The differences in disturbance among forest regions was mainly determined by their distance from human settlements, as we observed that the low disturbed forest regions were at least 5 km from the nearest village, and were not easily accessible, while the high disturbed forest regions were much close to human settlements and frequently visited by the inhabitants.
Data Collection
The data was collected by quadrat method with a quadrat size of 100 m2 (20 m × 5 m). At each selected forest region, nine random sites were chosen depending on the feasibility of sampling. So, we had a total of 54 sites for vegetation sampling and data collection. The minimum distance between the sites at each region was at least 2 km. For each site, plant species were counted in the three random quadrats separated by a distance of at least 100 m between the two nearest quadrats. For the tree species, diameter at breast height (DBH) was noted for each individual in the quadrat, at each site. Woody species were categorized as adult (DBH ≥10 cm), and saplings (DBH <10 and ≥1 cm). In the center of each 100 m2 quadrat, at each site, the stem count of each herbaceous species was recorded in 10 m2 (2 m × 0.5 m) quadrat. During sampling of herbaceous species, we neglected the ephemerals of short life span, and exhibiting less than 5 cm height. For 2 years, we recorded tree harvesting in the form of logged stumps, and observed grazing pressure in the form of dung pellets in each 100 m2 quadrat, at each site. During this period we also recorded frequency of fire occurrences and the quadrat area under fire, for each quadrat.
Composite surface (0–30 cm) soil samples were collected at five random locations from each quadrat, at each site, in September, for the physico-chemical analysis. These samples were analyzed for texture (Sheldrick and Wang, 1993), water holding capacity (Schlichting and Blume, 1966), and organic C (Walkley and Black, 1934) contents. To get the actual organic carbon content, values obtained by Walkley–Black method were multiplied by a correction factor (1.95) given by Krishan et al. (2009) for similar soils of central India. The correction factor is based on the relationship between Walkley–Black estimate and that from oxidative combustion-infrared analysis method using total organic carbon analyzer. Soil bulk density at each quadrat, at each site was determined by the core method (Krzic et al., 2000). For chemical analysis, we pooled the soil samples collected from the three quadrats and obtained one value for each soil parameter, per site.
Statistical Analysis
We calculated percent frequency, density, and abundance of tree and herbaceous species at each site based on the data collected from the three quadrats at each site. The importance value index (IVI) for all species was calculated by averaging relative frequency, relative density and relative dominance (i.e., basal area for trees and cover for herbaceous species), following Curtis and McIntosh (1951). Disturbance index for all study sites was calculated following Chaturvedi et al. (2017b).
The statistical analyses were done in R version 4.0.5 (R Foundation for Statistical Computing, Vienna, Austria) (R Development Core Team, 2018). We used likelihood ratio tests (LRTs) for testing interactions and main effects. We also used Wald tests for evaluating the parameter estimates. We observed site groupings by using harvest index, grazing index, and fire intensity data for each site through PCA, hierarchical clustering, and partitioning clustering particularly through k-means method by HCPC (hierarchical clustering on principal components). For this analysis, we used the R package “FactoMineR” and “factoextra” (Kassambara and Mundt, 2016). We used functions prcomp() and PCA() (FactoMine package) for PCA analysis. After clustering, all 54 study sites were separated into two disturbance categories (viz., low and high disturbance sites).
Through step-wise regression, we identified the best predictors of tree density, tree species richness and herbaceous species richness across the low (LDS) and high (HDS) disturbed sites in the forest region. For predicting the three vegetation parameters, we used harvest index, grazing index, fire intensity, and soil parameters across 54 study sites in the six selected forest regions. For step-wise regression, we used the R package “tidyverse” (Wickham et al., 2019), “caret” (Kuhn et al., 2016), and “leaps” (Lumley and Miller, 2009). We used the function stepAIC() (MASS package) (Venables and Ripley, 2010), for choosing the best model by AIC.
We calculated Student’s t-test for analyzing differences between LDS and HDS for tree density, tree species richness, herbaceous species richness, harvest index, grazing index, fire intensity, and soil properties by using “vegan” package (Oksanen et al., 2018). Pearson’s correlation was observed on tree density, tree species richness, herbaceous species richness, harvest index, grazing index, fire intensity, and soil properties across the 54 study sites by using “Hmisc” (Harrell, 2017) and “xtable” (Dahl, 2016) package. For plotting boxplots, we also used “ggplot2” package (Wickham, 2009).
Results
PCA and Hierarchical Clustering on Principal Components Analyses
The PCA biplot (Figure 1) explained the variability among the 54 study sites on the basis of disturbances in the form of tree harvesting, cattle grazing and wild fires. The eigenvalue of the first two PCA axes were 1.768 and 0.855, respectively. The individual variance explained by the first two PCA axes were 58.9 and 28.5%, while the two axes together accounted 87.4% of the total multivariate variation during PCA. The first PCA axis exhibited stronger associations with fire intensity (R = 0.768), while the second PCA axis indicated stronger correlations with grazing index (R = 0.887) and harvest index (R = 0.780). The HCPC separated the total 54 sites into two separate clusters as shown in Figure 1, where the sites inside the high disturbance eclipse (blue color) are in cluster 1, and the sites inside the low disturbance eclipse (red color) are in cluster 2. Description of quantitative variables of HCPC, including V-test indicated that the sites in cluster 1 exhibit significant positive influence for grazing index and harvest index (Supplementary Table 1). However, in cluster 2, the sites were observed to have significant negative influence of harvest index and grazing index (Supplementary Table 2).
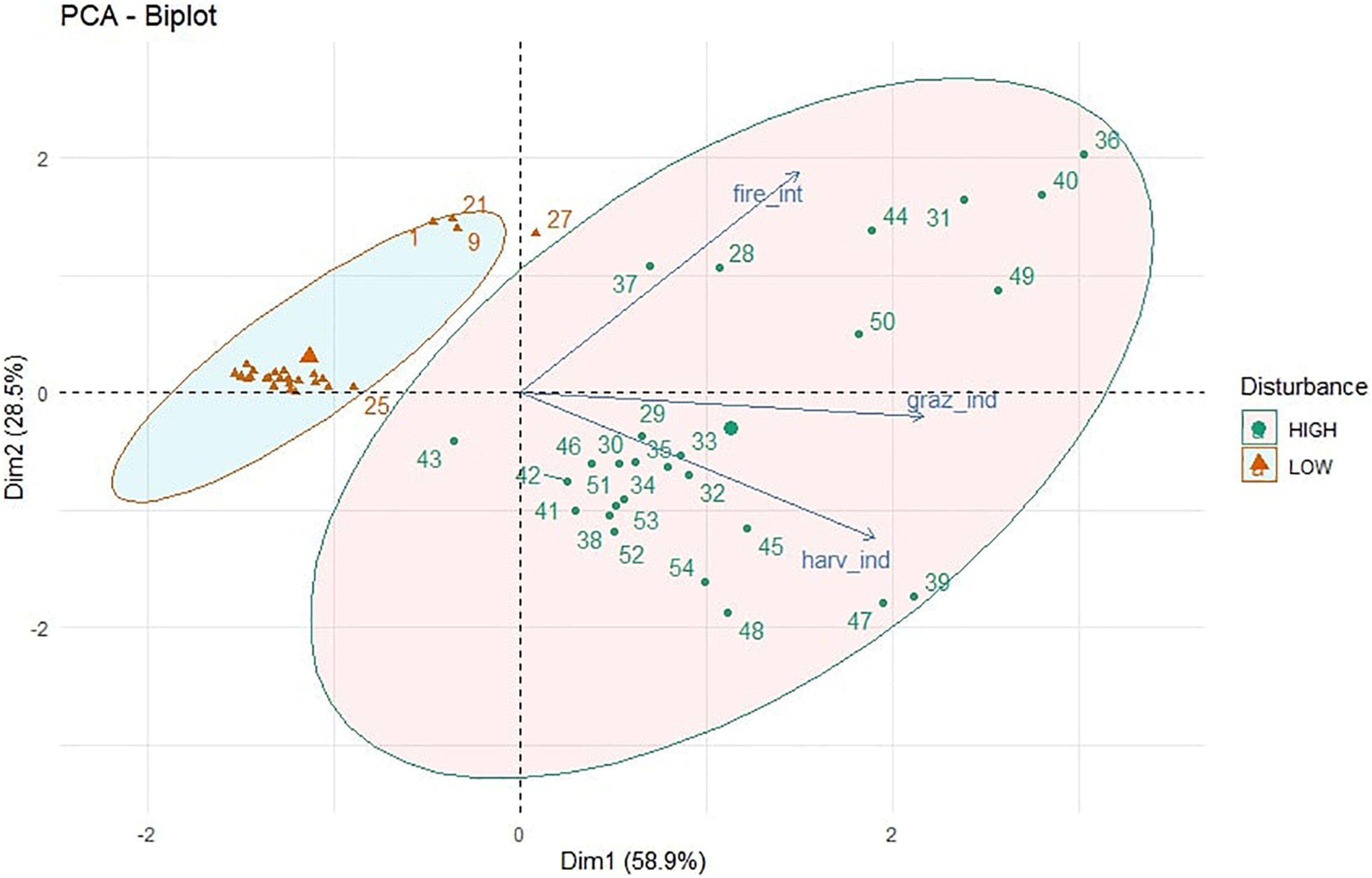
Figure 1. PCA showing clusters of low and high disturbed study sites based on harvest index, grazing index, and fire intensity at those sites.
Impact of Disturbances on Vegetation Properties
Vegetation features including tree stem count, tree species count, herbaceous species count, stump count, harvest index, grazing index, and fire intensity in the study sites with low and high disturbance are listed in Supplementary Table 3. In terms of disturbances, the low and high disturbance category sites showed significant differences for tree harvesting and cattle grazing, while the fire occurrences for the two categories of sites were not significant (Figure 2). Similarly, soil properties also exhibited significant differences between the LDS and HDS, although clay content was not significantly different between the two categories (Figure 3). We observed soil organic C, water holding capacity, and sand content significantly greater across LDS, whereas bulk density and gravel content was significantly higher across HDS.
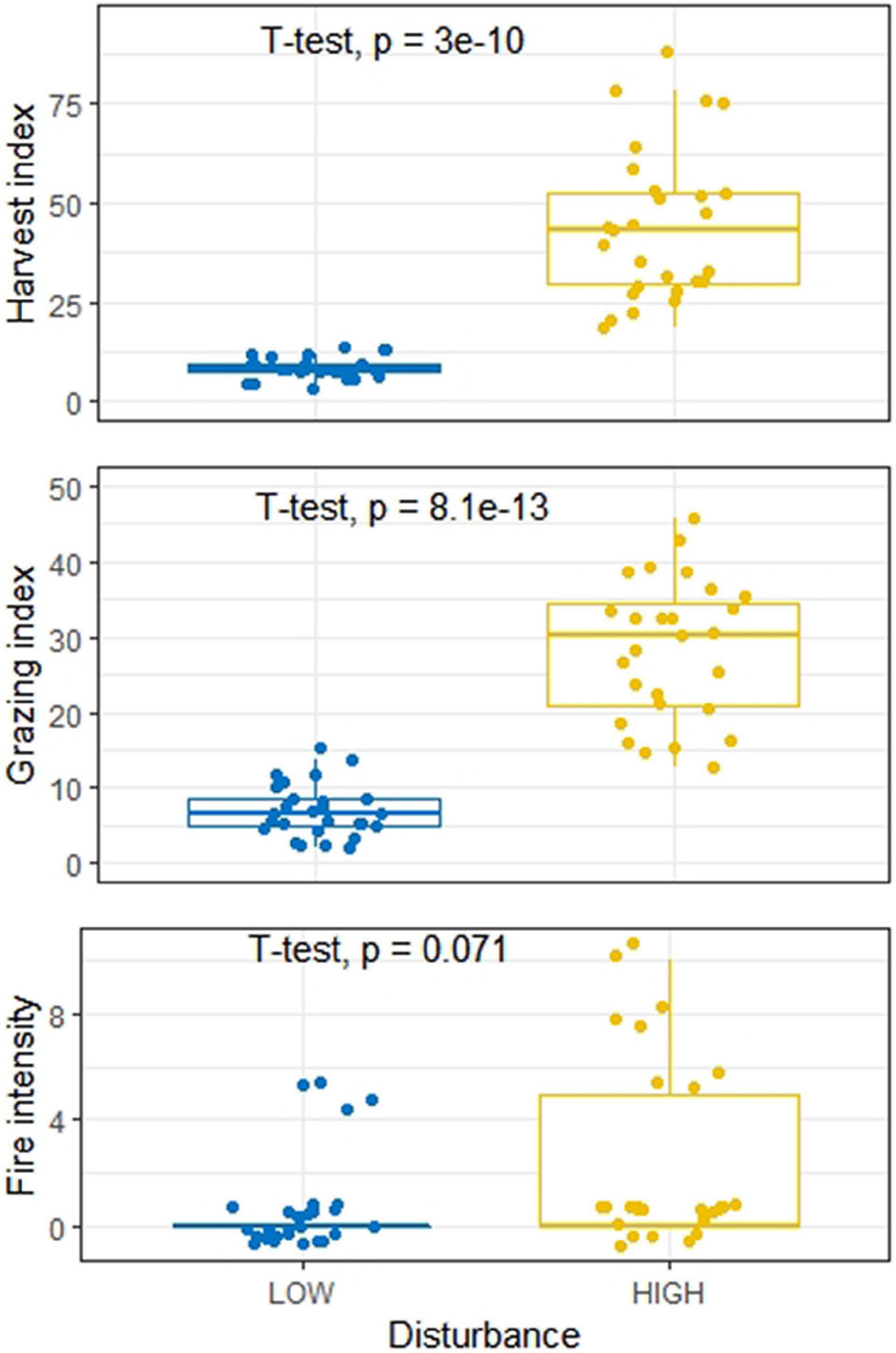
Figure 2. Boxplot showing distribution of harvest index, grazing index, and fire intensity at low and high disturbed sites.
Pearson’s correlation exhibited considerable impact of disturbances on vegetation parameters including soil properties (Table 1). Among the three disturbances, grazing index exhibited significant positive correlations with harvest index and fire index, whereas the relationship between harvest index and fire index was not significant. We found that the harvest index in the study region exhibited significantly positive correlation only with gravel content, while with other vegetation parameters, viz., tree density, tree species richness, soil organic C, water holding capacity, and sand content was significantly negative (Table 1). The relationships of grazing index were observed significantly positive with herbaceous species richness and gravel content, across the study region, whereas the negative associations of grazing index were similar to harvest index, i.e., significant for tree density, tree species richness, soil organic C, water holding capacity, and sand content (Table 1). Regarding fire intensity, the correlation across the study region was significantly negative with tree species richness, soil organic C, and water holding capacity (Table 1).
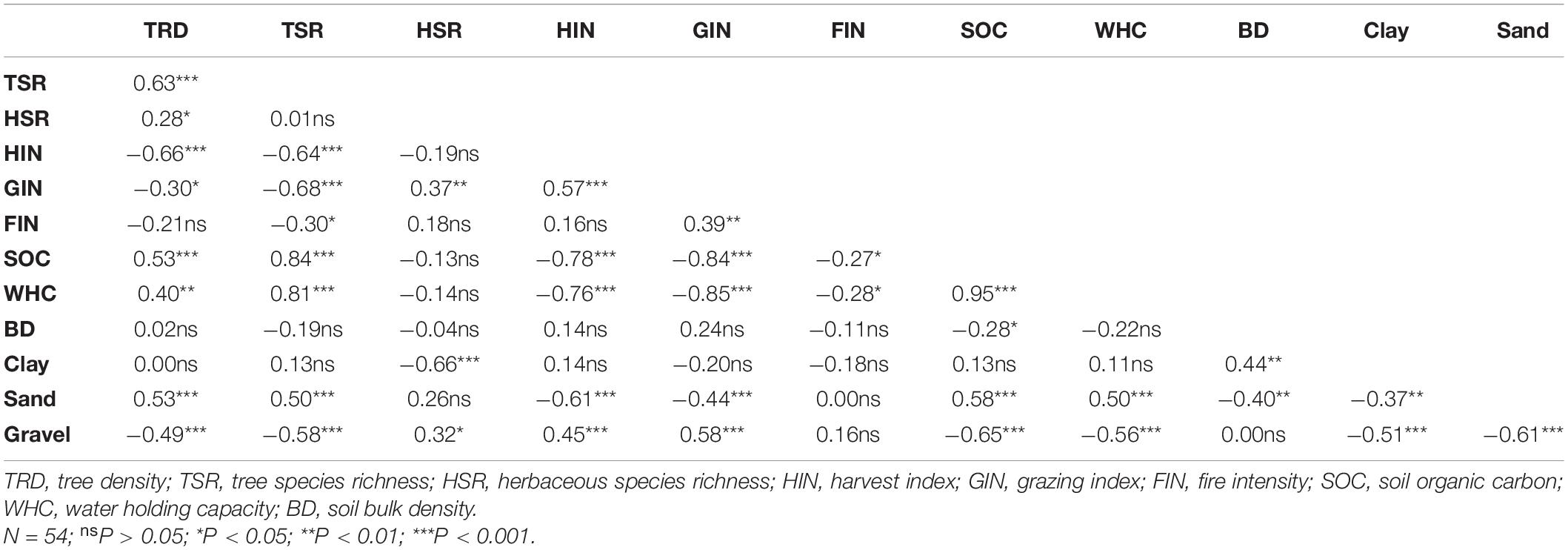
Table 1. Summary of Pearson’s correlation among various habitat characteristics across the low and high disturbance sites.
We observed that, for other than disturbances, correlations of tree density was significantly positive for tree species richness, herbaceous species richness, soil organic C, water holding capacity, and sand content, while the association was significantly negative for gravel content (Table 1). Across the study region, the relationship of tree species richness was significantly negative for gravel content, whereas significantly positive with soil organic C, water holding capacity, and sand content. Across the study region, herbaceous species richness exhibited significantly positive correlations with gravel content, whereas significantly negative association with clay content (Table 1).
We found that the correlation of soil organic C was significantly positive with water holding capacity and sand content, while significantly negative with bulk density and gravel content, across the study region (Table 1). Further, correlations among other soil physico-chemical properties indicated significant positive association of water holding capacity with sand content, whereas significantly negative relationship with gravel content. Bulk density exhibited significantly positive correlation with clay content, while significantly negative with sand content. Among the soil particles, the correlations of clay content was significantly negative with both sand content as well as gravel content, while the sand content also exhibited significantly negative association with gravel content, across the study region (Table 1).
We recorded 21 tree species across the 54 study sites in the forest region, among which the LDS showed the occurrence of 17 tree species, while the HDS exhibited only 11 tree species (Supplementary Table 4). Between the two disturbance categories, we recorded only six tree species growing across both LDS and HDS (Supplementary Table 4). According to IVI, S. robusta was the most dominant tree species across both low (IVI = 59.6) as well as HDS (IVI = 55.1), where this species constituted more than half of the tree composition. Among the other common species occurring across low as well as HDS, the dominance of Buchanania cochinchinensis and Syzygium cumini was greater across LDS, whereas the dominance of Diospyros melanoxylon, Randia diversiloba, and Vitex negundo was higher across the HDS. Among the 17 tree species across the LDS, 16 species exhibit medicinal properties, while out of 11 tree species across HDS, 10 have medicinal importance. Moreover, based on IVI, 90.5% of tree cover in the forest region contain medicinal properties.
Across the 54 study sites, we recorded 12 herbaceous species, in which the LDS and HDS exhibited eight and seven species, respectively (Supplementary Table 5). Only three herbaceous species were common to both categories of disturbances. According to the IVI values, Cynodon dactylon (IVI = 41.1) was the dominant species across the sites in low disturbance category, while Dichanthium annulatum (IVI = 34.9) was dominant species across the sites in high disturbance category. Interestingly, we observed that the composition of species belonging to Poaceae family was greater across the HDS (three species), as compared across the LDS (one species). It was found that around 56% of the herbaceous species community across the sites for high disturbed category was dominated by Poaceae family, whereas across LDS, Poaceae family accounted for 41% of the herbaceous species community. Among the eight herbaceous species across the LDS, seven species exhibit medicinal properties, while out of seven herbaceous species across HDS, only three species have medicinal importance. Moreover, based on IVI, 63.6% of herbaceous cover contain species of medicinal importance. When both medicinal and non-medicinal species were included in the analyses, we observed significant differences in tree density and tree species richness between the LDS and HDS, however herbaceous species richness was not significantly different (Figure 4). While on the other hand, when we analyzed the differences for only medicinal plants between the LDS and HDS, we found that besides tree species density and tree species richness, the herbaceous species richness was also significantly different (Figure 5). This result indicates that the impact of disturbances is more on medicinal plants. Across the LDS adult tree (≥10 cm DBH) density, as well as sapling tree (<10 to ≥ 1 cm DBH) density were significantly greater compared to the HDS. Moreover, adult:sapling ratio was also recorded significantly higher across the LDS compared to the HDS (Figure 4). We observed 16.9% of tree population across the LDS at adult stage, while 11.6% of tree population across the HDS at adult stage (Supplementary Table 3). We also noticed that across all LDS, both adult and sapling growth stages were recorded, however across the HDS there were five sites, where we were not able to get adult trees, although there was also one site, where we were not able to find sapling trees (Supplementary Table 3).
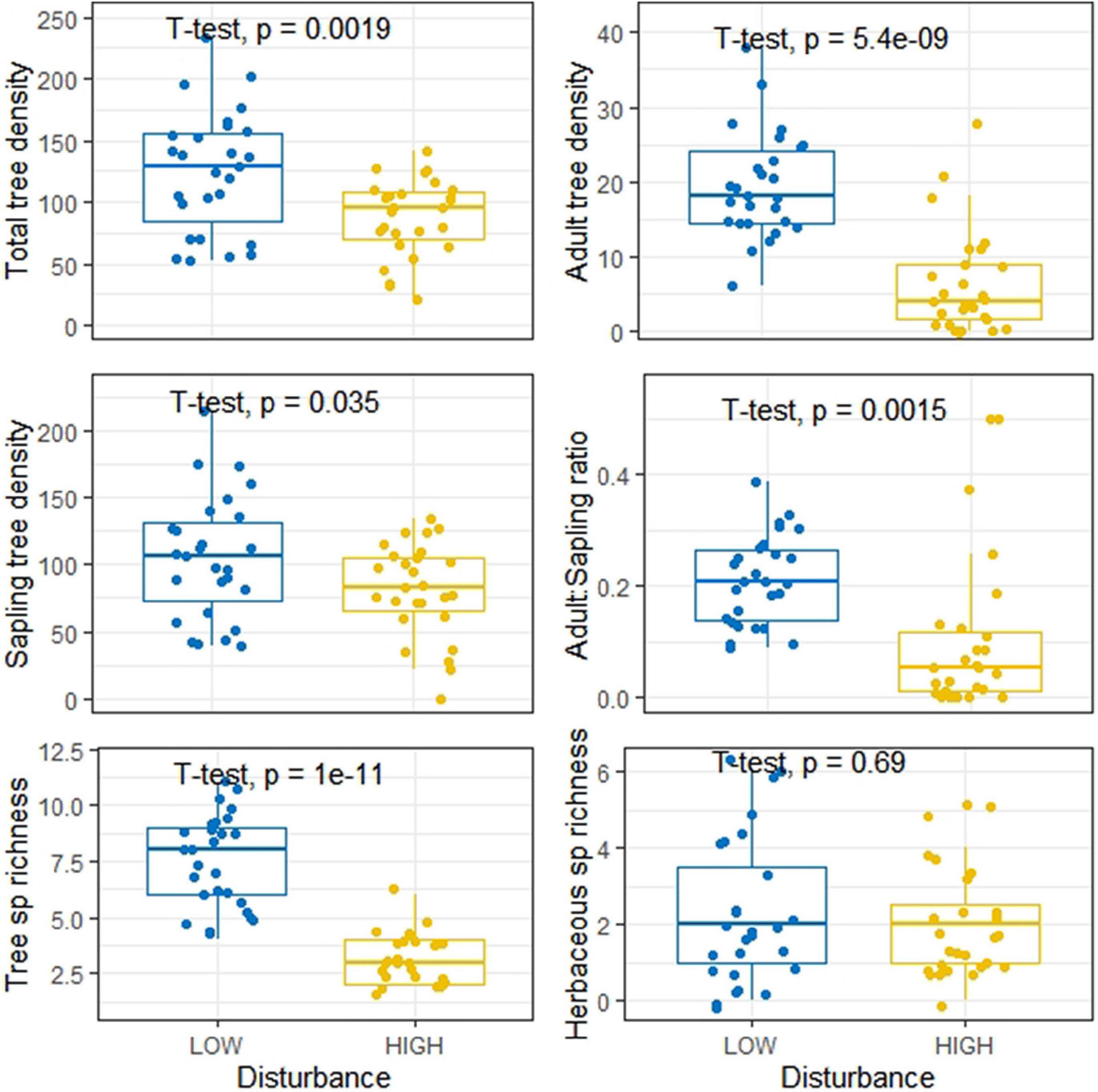
Figure 4. Boxplot showing distribution of total tree density, adult tree density, sapling tree density, adult:sapling ratio, tree species richness, and herbaceous species richness at low and high disturbed sites.
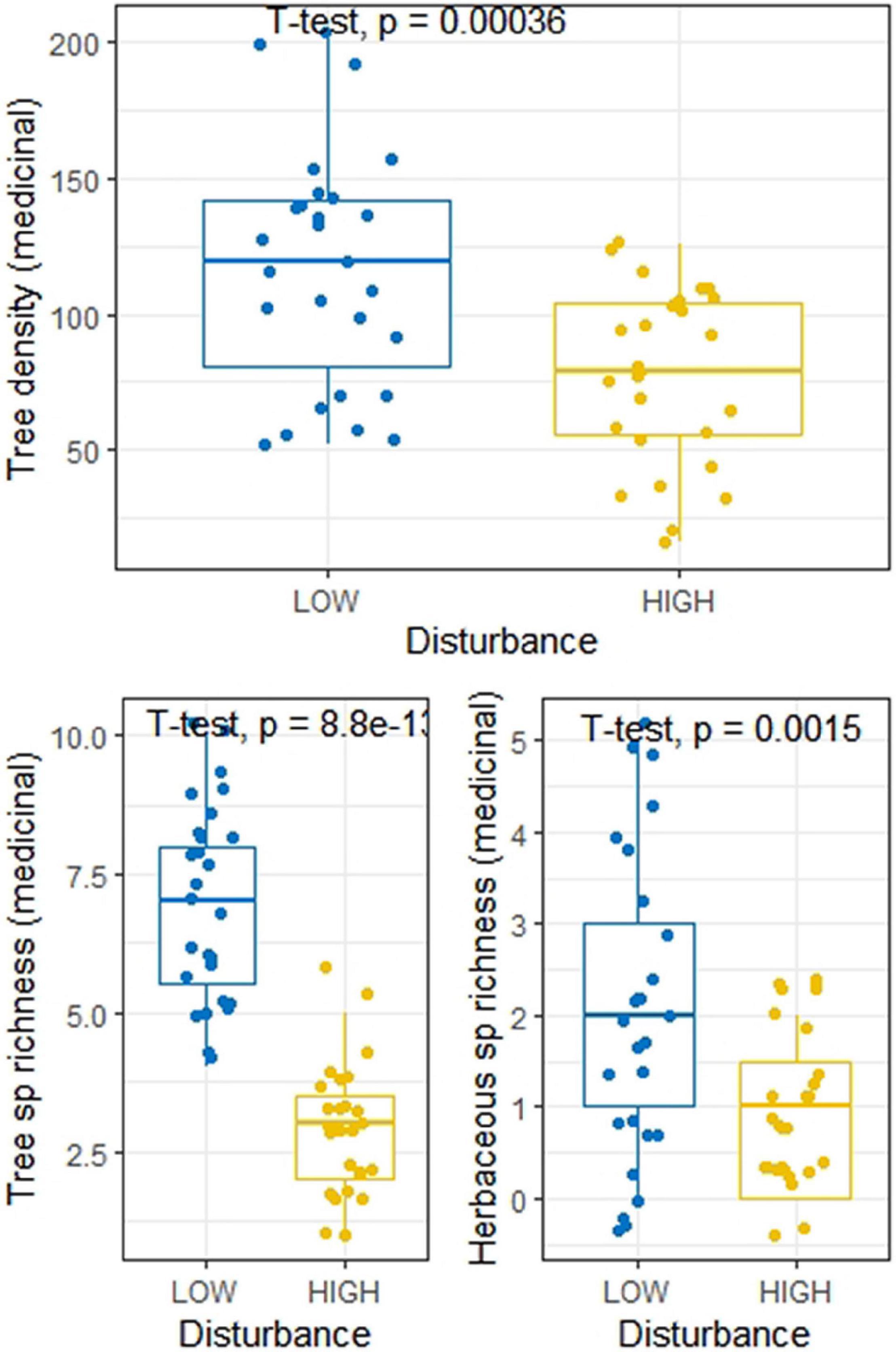
Figure 5. Boxplot showing distribution of tree density, tree species richness, and herbaceous species richness of medicinal plants at low and high disturbed sites.
Table 2 summarizes the stepwise regression results relating the three disturbing factors and soil properties with tree density, tree species richness, and herbaceous species richness, across the LDS and HDS in the forest region. Here we have produced the models exhibiting maximum variance and lowest AIC. According to stepwise regression results across LDS, maximum variance for tree density (80.6%) was observed for the model containing harvest index, sand content, soil organic C, bulk density, and water holding capacity. For the tree species richness, stepwise regression selected the model containing sand content, water holding capacity, clay content, grazing index, and fire intensity, exhibiting 53.6% variance in the model, while for the herbaceous species richness maximum variance (70.3%) was recorded for the model containing clay content, grazing index, sand content, and bulk density. Across the HDS, for tree density, the stepwise regression selected the model containing harvest index, grazing index, and sand content exhibiting variance of 96.1%. For tree species richness, maximum variance (48.7%) was recorded for the model containing clay content, bulk density, harvest index, and gravel content, while for herbaceou species richness the most efficient model contained clay content, grazing index, bulk density, water holding capacity, and soil organic C, exhibiting variance of 81.2% in the model.
Discussion
Across the study sites, we reported significant positive associations between harvest index and grazing index, at one hand, while grazing index with fire intensity, at the other hand. These relationships suggest that there is indirect associations among the three disturbances. As a resistance against disturbances, TDF species generally possess functional adaptations against drought, grazing and fire. After harvesting, open spaces develop in the forest where herbaceous species grow abundantly, which attract grazing animals to frequently visit these places. The fire adaptive C4 grasses also grow in the gaps, thereby increasing the fuel load helping in fire occurrences (Chaturvedi et al., 2017a). Most plant species are deciduous in TDF (Chaturvedi et al., 2011b), and these species are having numerous traits providing resistance against fire, such as thick bark, basal resprouting ability, and underground storage (Ratnam et al., 2016; Jhariya, 2017a,b). However, intense cattle grazing induces adverse impacts on species diversity, which is harmful especially for small sized trees, as compared to the herbaceous species (Chaturvedi et al., 2017a). Due to high grazing pressure, many tree saplings including important medicinal plants get damaged, and after the impact of drought and fire, these species are removed from the forest site (Chaturvedi et al., 2012, 2017b).
Among the three disturbances considered in our study, the step-wise regression results indicated harvest index as negatively influencing tree density across the LDS, and tree density as well as tree species richness across the HDS, while fire intensity was negatively impacting tree species richness, only across the LDS. Besides, the impact of grazing index was interesting, where it showed negative influence on tree density across the HDS, whereas for tree species richness at LDS, and for herbaceous species richness, both across LDS as well across HDS, the impact of grazing index was positive. Across the HDS, open spaces are more abundant (or more frequent) which attract grazing animals, and makes their movement easy. This leads to frequent damage, and mortality of tree saplings coming on their way (Chaturvedi et al., 2012, 2017a,2017b). Also, during the dry season, when grasses dry out, grazing animals are attracted toward tree saplings, which leads to high mortality and shift in the composition of tree species. In our study, we found some sites across the high disturbed category, where adult trees were absent, while at one site saplings were absent. For herbaceous species, situation is different, because in our forest region, herbs and grasses shed their aboveground structure and survive in the form of underground storage organ during the dry period, while they are available for grazing animals only during favorable wet season, when grazing pressure is less, compared to dry season. Moreover, the grazing animals damage the potential overstory trees and make open spaces for better growth of light demanding tree saplings and herbaceous vegetation, particularly palatable grasses which are more tolerant to grazing. Although, our results did not show significant difference in the herbaceous species richness between LDS and HDS, graminoids were more abundant, and medicinal herbaceous species significantly less abundant among HDS, compared to the LDS. Thus, we can argue that the intensity of grazing by cattle and wild animals, at our study sites could be the potential mechanism responsible for determining shift in herbaceous species composition in near future. Our findings in this study are supported by Lin et al. (2010) and Fenetahun et al. (2020), where they reported significant impact of intensity and distribution of grazing on composition and productivity of herbaceous species.
Disruption of soil organic C in TDFs is mostly due to disturbances, such as overgrazing and occasional fires, however it is generally neglected in scientific studies (Abril et al., 2005). Across the study sites, we observed that the soil organic C was positively associated with tree density and tree species richness, whereas negatively related with harvest index, grazing index, and fire intensity. The results are in accordance with other studies; for example, in one of those, higher soil organic C induces positive feedback on species richness (Chaturvedi et al., 2011a). We observed significantly high soil organic C, across LDS, compared to the HDS. Among other factors, the decline in soil organic C across the study sites could also be due to grazing by cattle, since due to increasing grazing pressure, ground layer vegetation gets eaten and removed by grazing animals, leading to reduction in soil organic C (Chaturvedi et al., 2012). In a study by Chen et al. (2015) moderate grazing accumulated more soil organic C due to high root production and turnover. They reported that consistent high grazing pressure reduces above and belowground plant production, increases soil N losses, and creates unfavorable microbial environment, which leads to decline in organic C input in soil. Moreover, the soil organic C at HDS could also be influenced by greater gravel content, since large soil particles are not able to hold organic C. Earlier studies have suggested that soil organic C increases productivity by increasing water holding capacity of soil in TDFs (Tiessen et al., 1994; Chaturvedi and Singh, 2017), therefore understory vegetation, particularly herbaceous species needs to be conserved and protected from excessive grazing.
Studies made for the assessment of plant diversity in the dry tropical regions have reported decreasing pattern of diversity due to anthropogenic pressure (Chaturvedi et al., 2011a,Chaturvedi et al., 2012, 2017a,2017b). Similarly, the diversity of medicinal plants in our study is also affected negatively due to forest disturbances (Figure 5). For instance, the important medicinal plants like Adina cordifolia, Anogeissus latifolia, Asparagus racemosus, Bauhinia vahlii, Curculigo orchioides, Hemidesmus indicus, Holarrhena antidysenterica, Lygodium flexuosum, Nyctanthes arbor-tristis, Schleichera oleosa, Terminalia tomentosa, Woodfordia fruticosa, and Ziziphus nummularia were not recorded from the HDS, and the probable reason behind their absence from our study sites might be enormous anthropogenic pressure. Supporting our observations, similar studies for the assessment of medicinal plant diversity exposed to anthropogenic pressure, for instance, in Himalayan region, conducted by Adnan et al. (2014, 2015), in Eastern Amazonia by Shanley and Luz (2003), in Western Nepal by Kunwar et al. (2016) have also reported similar results in their forest regions.
Among the herbaceous species recorded in this study, A. racemosus, C. dactylon, and H. indicus exhibit clonal propagation where plant population is derived from a single individual through asexual reproduction. Therefore, these species produce large clones, and might be under or over represented in our data set. Further, although we found greater impact of disturbances in the form of harvesting, grazing and fire on declining composition of medicinal plants in the forest region, some of the differences between HDS and LDS might potentially, already be there before the disturbance. For example, differences in clay, sand, and gravel. As soil structure influence water retention, nutrient content and bulk density, which themselves influence which species can grow or determine the abundance of some of the species, and it is difficult to separate the impact of the disturbance from the differences between sites that existed prior to the disturbances. Considering the difference in soil texture between high and low disturbance, we could expect some difference in both tree and herbaceous species composition due to soil properties, as well. The other soil properties could have been modified by the disturbance or were already present and exacerbated by the disturbances. Therefore, we emphasize further investigations on the potential role of anthropogenic disturbances in modulating habitat properties, community structure and diversity of medicinal plants. We suggest that forest managers take into consideration the presence of anthropogenic disturbances in their management and conservation efforts for medicinal plants in TDFs.
Data Availability Statement
The original contributions presented in the study are included in the article/Supplementary Material, further inquiries can be directed to the corresponding author.
Author Contributions
PS and SP collected the field data and prepared the tables for the primary data and its calculations. KS helped in manuscript writing. RC did the statistical analysis and gave the manuscript its final shape. All authors contributed to the article and approved the submitted version.
Funding
University Grants Commission, Central Regional Office, Bhopal was deeply acknowledged for providing financial assistance under Minor Research Project scheme (MS-24/201027/XII/14-15/CRO) to PS. National Natural Science Foundation of China (NSFC), Chinese Academy of Science, China (award No. 31750110466) is acknowledged for financial support to RC.
Conflict of Interest
The authors declare that the research was conducted in the absence of any commercial or financial relationships that could be construed as a potential conflict of interest.
Publisher’s Note
All claims expressed in this article are solely those of the authors and do not necessarily represent those of their affiliated organizations, or those of the publisher, the editors and the reviewers. Any product that may be evaluated in this article, or claim that may be made by its manufacturer, is not guaranteed or endorsed by the publisher.
Acknowledgments
The authors are thankful to M. L. Nayak (Retd., Pt. R.S. University, Raipur) for helping in identification of species. The authors also appreciate the insightful comments of the reviewers for improving the structure of manuscript.
Supplementary Material
The Supplementary Material for this article can be found online at: https://www.frontiersin.org/articles/10.3389/ffgc.2021.718930/full#supplementary-material
References
Abril, A., Barttfeld, P., and Bucher, E. H. (2005). The effect of fire and overgrazing disturbes on soil carbon balance in the Dry Chaco forest. For. Ecol. Manage. 206, 399–405. doi: 10.1016/j.foreco.2004.11.014
Adnan, M., and Hölscher, D. (2012). Diversity of Medicinal Plants among Different Forest-use Types of the Pakistani Himalaya. Econ. Bot. 66, 344–356. doi: 10.1007/s12231-012-9213-4
Adnan, M., Tariq, A., and Shinwari, Z. K. (2015). Effects of human proximity and nomadic grazing on the diversityof medicinal plants in Temperate Hindukush. Pak. J. Bot. 47, 149–157.
Adnan, M., Tariq, A., Begum, S., Ullah, A., and Mussarat, S. (2014). Medicinal plants after forest disturbance restoration and cultivation in Pakistani Himalaya. Int. J. Agric. Biol. 16, 1006–1010.
Arjunan, M., Puyravaud, J.-P., and Davidar, P. (2005). The impact of resource collection by local communities on the dry forests of the Kalakad-Mundanthurai Tiger Reserve, India. Trop. Ecol. 46, 135–143.
Chaturvedi, R. K., and Raghubanshi, A. S. (2014). Species Composition, Distribution, and Diversity of Woody Species in a Tropical Dry Forest of India. J. Sustain. For. 33, 729–756. doi: 10.1080/10549811.2014.925402
Chaturvedi, R. K., and Raghubanshi, A. S. (2015). Assessment of carbon density and accumulation in mono- and multi-specific stands in tropical dry forests of India. For. Ecol. Manage. 339, 11–21. doi: 10.1016/j.foreco.2014.12.002
Chaturvedi, R. K., and Singh, J. S. (2017). Restoration of mine spoil in a dry tropical region: a review. Proc. Indian Natl. Sci. Acad. INSA 83, 789–844.
Chaturvedi, R. K., Prasad, S., Rana, S., Obaidullah, S. M., Pandey, V., and Singh, H. (2013). Effect of dust load on the leaf attributes of the tree species growing along the roadside. Environ. Monit. Assess. 185, 383–391. doi: 10.1007/s10661-012-2560-x
Chaturvedi, R. K., Raghubanshi, A. S., and Singh, J. S. (2011a). Carbon density and accumulation in woody species of tropical dry forest in India. For. Ecol. Manage. 262, 1576–1588. doi: 10.1007/s10661-017-5899-1
Chaturvedi, R. K., Raghubanshi, A. S., and Singh, J. S. (2011b). Plant functional traits with particular reference to dry deciduous forests: a review. J. Biosci. 36, 963–981. doi: 10.1007/s12038-011-9159-1
Chaturvedi, R. K., Raghubanshi, A. S., and Singh, J. S. (2012). Effect of grazing and harvesting on diversity, recruitment and carbon accumulation of juvenile trees in tropical dry forests. For. Ecol. Manage. 284, 152–162. doi: 10.1016/j.foreco.2012.07.053
Chaturvedi, R. K., Raghubanshi, A. S., Tomlinson, K. W., and Singh, J. S. (2017a). Impacts of human disturbance in tropical dry forests increase with soil moisture stress. J. Veg. Sci. 28, 997–1007. doi: 10.1111/jvs.12547
Chaturvedi, R. K., Raghubanshi, A. S., and Singh, J. S. (2017b). Sapling harvest: a predominant factor affecting future composition of tropical dry forests. For. Ecol. Manage. 38, 221–235. doi: 10.1016/j.foreco.2016.10.026
Chaturvedi, R. K., Tripathi, A., Raghubanshi, A. S., and Singh, J. S. (2021). Functional traits indicate a continuum of tree drought strategies across a soil water availability gradient in a tropical dry forest. For. Ecol. Manage. 482, 1–18.
Chen, W., Huang, D., Liu, N., Zhang, Y., Badgery, W. B., Wang, X., et al. (2015). Improved grazing management may increase soil carbon sequestration in temperate steppe. Sci. Rep. 5:10892. doi: 10.1038/srep10892
Curtis, J. T., and McIntosh, R. P. (1951). An upland forest continuum in the prairie-forest border Region of Wisconsin. Ecology 32, 476–496.
Eriksson, I., Teketay, D., and Granström, A. (2003). Response of plant communities to fire in an Acacia woodland and a dry Afromontane forest, southern Ethiopia. For. Ecol. Manage. 177, 39–50.
Fenetahun, Y., Xu, X., You, Y., and Wang, Y. (2020). Effects of Vegetation Cover, Grazing and Season on Herbage Species Composition and Biomass: in Case of Yabello Rangeland, Southern Ethiopia. J. Resour. Ecol. 11, 159–170. doi: 10.5814/j.issn.1674-764x.2020.02.004
Gilliam, F. S. (2007). The ecological significance of the herbaceous layer in temperate forest ecosystems. Bioscience 57, 845–858. doi: 10.1890/12-1582.1
Hamilton, A. C. (2004). Medicinal plants, conservation and livelihoods. Biodivers. Conserv. 13, 1477–1517.
Harris, F., and Mohammed, S. (2003). Relying on nature: wild foods in Northern Nigeria. Ambio 32, 24–29. doi: 10.1579/0044-7447-32.1.24
Hegde, R., and Enters, T. (2000). Forest products and household economy: a case study from Mudumalai Wildlife sanctuary, Southern India. Environ. Conserv. 27, 250–259.
Jhariya, M. K. (2017a). Vegetation ecology and carbon sequestration potential of shrubs in tropics of Chhattisgarh, India. Environ. Monit. Assess. 189:518. doi: 10.1007/s10661-017-6246-2
Jhariya, M. K. (2017b). Influences of forest fire on forest floor and litterfall dynamics in Bhoramdeo Wildlife Sanctuary (C.G.), India. J. For. Environ. Sci. 33, 330–341. doi: 10.7747/JFES.2017.33.4.330
Jhariya, M. K., and Singh, L. (2021a). Herbaceous diversity and biomass under different fire regimes in a seasonally dry forest ecosystem. Environ. Dev. Sustain. 23, 6800–6818. doi: 10.1007/s10668-020-00892-x
Jhariya, M. K., and Singh, L. (2021b). Effect of fire severity on soil properties in a seasonally dry forest ecosystem of Central India. Int. J. Environ. Sci. Technol. 18, 3967–3978. doi: 10.1007/s13762-020-03062-8
Jhariya, M. K., and Singh, L. (2021c). “Productivity and Carbon Accumulation in Tropical Dry Deciduous Forest Ecosystem,” in Tropical Dry Forests: emerging Features and Ecological Perspectives, eds R. K. Chaturvedi, R. Singh, and R. Bhadouria (NY USA: Nova Science), 269–287.
Jhariya, M. K., Banerjee, A., Meena, R. S., and Yadav, D. K. (eds) (2019). Sustainable Agriculture, Forest and Environmental Management. Singapore: Springer Nature Singapore, 606. doi: 10.1007/978-981-13-6830-1
Kassambara, A., and Mundt, F. (2016). Factoextra: Extract and Visualize the Results of Multivariate Data Analyses, 1–74.
Klinge, H., Adis, J., and Worbes, M. (1995). The vegetation of a seasonal várzea in the lower Solimões river, Brazilian Amazon. Acta Amazon. 25, 201–220. doi: 10.1590/1809-43921995253220
Krishan, G., Srivastav, S. K., Kumar, S., Saha, S. K., and Dadhwal, V. K. (2009). Quantifying the underestimation of soil organic carbon by the Walkley and Black technique uexamples from Himalayan and Central Indian soils. Curr. Sci. 96, 1133–1136.
Krzic, M., Fortin, M.-C., and Bomke, A. A. (2000). Short-term responses of soil physical properties to corn tillage-planting systems in a humid maritime climate. Soil Tillage Res. 54, 171–178.
Kuhn, M., Wing, J., Wenston, S., Williams, A., Keefer, C., Engelhardt, A., et al. (2016). Caret: Classifification and Regression Training. R Package Version 6.0–78.
Kunwar, R. M., Baral, K., Paudel, P., Acharya, R. P., Thapa-Magar, K. B., Cameron, M., et al. (2016). Land-Use and Socioeconomic Change, Medicinal Plant Selection and Biodiversity Resilience in Far Western Nepal. PLoS One 11:e0167812. doi: 10.1371/journal.pone.0167812
Kutty, R., and Kothari, A. (2001). Protected Areas in India: a Profile. New Delhi: Kalpavriksh Publications.
Lal, C. B., Annapurna, C., Raghubanshi, A. S., and Singh, J. S. (2001). Foliar demand and resource economy of nutrients in dry tropical forest species. J. Veg. Sci. 12, 5–14.
Lin, Y., Hong, M., Han, G., Zhao, M. L., Bai, Y. F., Chang, S. X., et al. (2010). Grazing intensity affected spatial patterns of vegetation and soil fertility in a desert steppe. Agric. Ecosyst. Environ. 138, 282–292. doi: 10.1016/j.agee.2010.05.013
Markesteijn, L., and Poorter, L. (2009). Seedling root morphology and biomass allocation of 62 tropical tree species in relation to drought and shade tolerance. J. Ecol. 97, 311–325. doi: 10.1111/j.1365-2745.2008.01466.x
McGeoch, L., Gordon, I., and Schmitt, J. (2008). Impacts of land use, anthropogenic disturbance, and harvesting on an African medicinal liana. Biol. Conserv. 141, 2218–2229. doi: 10.1016/j.biocon.2008.06.021
Mehta, A. K., and Shah, A. (2003). Chronic poverty in India: incidence, causes and policies. World Dev. 31, 491–511.
MoEF (1999). National Forestry Action Plan. New Delhi, India: Ministry of Environment and Forests, Government of India.
Morgan, P., and Neuenschwander, L. F. (1988). Shrub response to high and low severity burns following clearcutting in northern Idaho. West. J. Appl. For. 3, 5–9. doi: 10.1093/wjaf/3.1.5
Oksanen, J., Guillaume Blanchet, F., Friendly, M., Kindt, R., Legendre, P., McGlinn, D., et al. (2018). vegan: Community Ecology Package. R Package. Version 2.5-2. Available online at: https://CRAN.R-project.org/package=vegan
Painkra, V. K., Jhariya, M. K., and Raj, A. (2015). Assessment of Knowledge of Medicinal Plants and their use in Tribal Region of Jashpur District of Chhattisgarh, India. J. Appl. Nat. Sci. 7, 434–442.
Paula, S., and Pausas, J. G. (2008). Burning seeds: germinative response to heat treatments in relation to resprouting ability. J. Ecol. 96, 543–552. doi: 10.1111/j.1365-
Paula, S., Arianoutsou, M., Kazanis, D., Tavsanoglu, C., Lloret, F., Buhk, C., et al. (2009). Fire-related traits for plant species of the Mediterranean basin. Ecology 90:1420. doi: 10.1890/08-1309.1
Poffenberger, M., and McGean, B. (eds) (1996). Village Voices, Forest Choices: joint Forest Management in India. Delhi: Oxford University Press.
Prajapati, S. K., Sharma, K., and Singh, P. K. (2018). Plant diversity in tropical dry deciduous forests of Jashpur, Chhattisgarh with special reference to their ethnomedicinal uses. Trop. Ecol. 59, 505–514.
R Development Core Team (2018). R: a Language and Environment for Statistical Computing. Vienna, Austria: R Foundation for Statistical Computing.
Rai, S. N., and Chakrabarti, S. K. (2001). Demand and supply of fuel-wood and timber in India. Indian For. 3, 263–279.
Ramírez-Marcial, N., González-Espinosa, M., and Williams- Linera, G. (2001). Anthropogenic disturbance and tree diversity in montane rain forests in Chiapas, Mexico. For. Ecol. Manage. 154, 311–326.
Ratnam, J., Tomlinson, K. W., Rasquinha, D. N., and Sankaran, M. (2016). Savannas of Asia: evidence for antiquity, biogeography, and an uncertain future. Philos. Trans. R. Soc. B Biol. Sci. 371:20150305. doi: 10.1098/rstb.2015.0305
Rivas, C., Navarro-Cerillo, R., Johnston, J., and Guerrero-Casado, J. (2020). Dry forest is more threatened but less protected than evergreen forest in Ecuador’s coastal region. Environ. Conserv. 47, 79–83. doi: 10.1017/S0376892920000077
Rosero-Toro, J. H., Romero-Duque, L. P., Santos-Fita, D., and Ruan-Soto, F. (2018). Cultural significance of the flora of a tropical dry forest in the Doche vereda (Villavieja, Huila, Colombia). J. Ethnobiol. Ethnomed. 14, 1–16. doi: 10.1186/s13002-018-0220-0
Sagar, R., Raghubanshi, A. S., and Singh, J. S. (2003a). Tree species composition, dispersion and diversity along a disturbance gradient in a dry tropical forest region of India. For. Ecol. Manage. 186, 61–71. doi: 10.1016/s0378-1127(03)00235-4
Sagar, R., Raghubanshi, A. S., and Singh, J. S. (2003b). Asymptotic models of species–area curve for measuring diversity of dry tropical forest tree species. Curr. Sci. 84, 1555–1560.
Sagar, R., Raghubanshi, A. S., and Singh, J. S. (2008). Comparison of community composition and species diversity of understorey and overstorey tree species in a dry tropical forest of northern India. J. Environ. Manage. 88, 1037–1046. doi: 10.1016/j.jenvman.2007.05.013
Saha, S., and Howe, H. F. (2003). Species composition and fire in a dry deciduous forest. Ecology 84, 3118–3123.
Sánchez-Azofeifa, G. A., and Portillo-Quintero, C. (2011). “Extent and drivers of change of Neotropical seasonally dry tropical forests,” in Seasonally dry tropical forests, eds R. Dirzo, H. S. Young, H. A. Mooney, and G. Ceballos (London: Island Press), 45–58. doi: 10.1371/journal.pone.0034074
Sheldrick, B. H., and Wang, C. (1993). “Particle-size distribution,” in Soil Sampling and Methods of Analysis. Canadian Society of Soil Science, ed. M. R. Carter (Ann Arbor, MI: Lewis Publishers), 499.
Shahabuddin, G., and Kumar, R. (2007). Effects of extractive disturbance on bird assemblages, vegetation structure and floristic in tropical scrub forest, Sariska Tiger Reserve, India. For. Ecol. Manage. 206, 175–185. doi: 10.1016/j.foreco.2007.03.061
Shaikh, K. B., and D Parveen, N. (2017). Floristic and medicinal plant survey at ahobilam forest of Kurnool district, Andhra Pradesh, India. J. Med. Plants Stud. 5, 28–30.
Shanley, P., and Luz, L. (2003). The impacts of forest degradation on medicinal plant use & implications for health care in Eastern Amazonia. Bioscience 53, 573–584.
Singh, J. S., and Chaturvedi, R. K. (2017). Diversity of ecosystem types in India: a review. Proc. Indian Natl. Sci. Acad. INSA 83, 569–594.
Singh, J. S., and Singh, V. K. (1992). Phenology of seasonally dry tropical forest. Curr. Sci. 63, 684–689.
Singh, J. S., Raghubanshi, A. S., Singh, R. S., and Srivastava, S. C. (1989). Microbial biomass acts as a source of plant nutrients in dry tropical forests and savanna. Nature 338, 499–500. doi: 10.1038/338499a0
Singh, J. S., Singh, K. P., and Agrawal, M. (1991). Environmental degradation of the Obra-Renukoot-Singrauli area, India, and its impact on natural and derived ecosystems. Environmentalist 11, 171–180. doi: 10.1007/bf01263230
Singh, L., and Singh, J. S. (1991). Storage and flux of nutrients in a dry tropical forest in India. Ann. Bot. 68, 275–284. doi: 10.1093/oxfordjournals.aob.a088253
Singh, P. K., Kumar, V., Tiwari, R. K., Sharma, A., Rao, C. V., and Singh, R. H. (2010). Medico-Ethnobotany of ‘Chatara’ Block of District Sonebhadra, Uttar Pradesh, India. Adv. Biol. Res. 4, 65–80.
Singh, S. P. (1998). Chronic disturbance, a principal cause of environmental degradation in developing countries (Editorial). Environ. Conserv. 25, 1–2.
Stephan, K., Miller, M., and Dickinson, M. B. (2010). First-Order Fire Effects on Herbs and Shrubs: present Knowledge and Process Modeling Needs. Fire Ecol. 6, 95–114. doi: 10.4996/fireecology.0601095
Tiessen, H., Cuevas, E., and Chacon, P. (1994). The role of soil organic matter in sustaining soil fertility. Nature 371, 783–785. doi: 10.1038/371783a0
Trejo, I., and Dirzo, R. (2000). Deforestation of seasonally dry tropical forest: a national and local analysis in Mexico. Biol. Conserv. 94, 133–142. doi: 10.1016/s0006-3207(99)00188-3
Venables, W. N., and Ripley, B. D. (2010). Modern Applied Statistics With S, 4th Edn. New York, NY: Springer-Verlag.
Walkley, A., and Black, I. A. (1934). An examination of the Degtjareff method for determining soil organic matter, and a proposed modification of the chromic acid titration method. Soil Sci. 37, 29–38. doi: 10.1097/00010694-193401000-00003
Wickham, H., Averick, M., Bryan, J., Chang, W., McGowan, L., François, R., et al. (2019). Welcome to the Tidyverse. J. Open Source Softw. 4:1686.
Wittmann, F., and Junk, W. J. (2003). Sapling communities in Amazonian whitewater forests. J. Biogeogr. 30, 1533–1544. doi: 10.1046/j.1365-2699.2003.00966.x
Keywords: medicinal plants, harvesting, grazing, fire, tropical dry forest
Citation: Singh PK, Prajapati SK, Sunita K and Chaturvedi RK (2022) Disturbance Induced Changes in Diversity of Medicinal Plants in a Dry Tropical Forest of India. Front. For. Glob. Change 4:718930. doi: 10.3389/ffgc.2021.718930
Received: 01 June 2021; Accepted: 26 October 2021;
Published: 14 February 2022.
Edited by:
Jean-Claude Ruel, Laval University, CanadaReviewed by:
Line Lapointe, Laval University, CanadaManoj Kumar Jhariya, Sant Gahira Guru Vishwavidyalaya, India
Copyright © 2022 Singh, Prajapati, Sunita and Chaturvedi. This is an open-access article distributed under the terms of the Creative Commons Attribution License (CC BY). The use, distribution or reproduction in other forums is permitted, provided the original author(s) and the copyright owner(s) are credited and that the original publication in this journal is cited, in accordance with accepted academic practice. No use, distribution or reproduction is permitted which does not comply with these terms.
*Correspondence: Ravi Kant Chaturvedi, cmF2aWthbnRjaGF0dXJ2ZWRpMTBAZ21haWwuY29t