Leaf Anatomical Traits of Lianas and Trees at the Canopy of Two Contrasting Lowland Tropical Forests in the Context of Leaf Economic Spectrum
- Centre for Earth Observation Science, Department of Earth and Atmospheric Sciences, University of Alberta, Edmonton, AB, Canada
The leaf economic spectrum describes a comprehensive framework of how the surrounding environment modulates leaf functional traits (LFT) and how these are associated between them. This framework has traditionally focused on physiological, chemical, and biomass assignation traits, but rarely in leaf anatomical traits. Here we compare leaf anatomical metrics and traits of 40 liana and tree species from two lowland tropical forests in Panama with contrasting rainfall regimes: Parque Natural Metropolitano (dry-forest) and Parque Nacional San Lorenzo (wet-forest). Then we evaluate how anatomical traits are associated with well-established LFTs. Anatomical metrics were collected from leaf cross-section images estimating the area, thickness, cell count, and size of the upper and lower epidermis and palisade and spongy mesophyll. Ratios between metrics were performed as potential anatomical traits to reduce the leaf size effect between species. Our results suggest that anatomical changes between life forms are associated with increases in the palisade area and thickness of trees in comparison with lianas, while anatomical changes between forest type species are related to increases in the spongy area and thickness of wet-forest species than dry-forest. These differences could be associated with the high photosynthetic rates of trees or the need to enhance the gas exchange in humid environments. Our results also suggest that anatomical traits are related to well-established LFT; however, the degree of association between them may depend on the life forms and forest type. For example, our results suggest that reductions in the palisade and spongy cell density are associated with increases in leaf mass area and maximum photosynthetic capacity, but this association was not observed when we compared life forms or forest types. The use of leaf anatomical information may facilitate to describe the mechanism that drives the leaf economy, improving our understanding of the resource allocation strategies embedded in functional groups.
Introduction
Leaf economic spectrum (LES) provides a useful framework to describe how leaf traits are inter-correlated and how they are modulated by their surrounding environment (Wright et al., 2004). This theory attempts to transform discrete groups of plant functional types to a more continuum-oriented approach to assess plant functional diversity (Reich, 2014). Currently, the LES has been expanded towards other traits related to wood (Chave et al., 2009), organs such as roots or stems (Reich, 2014; Kong et al., 2019), plant structure (Verbeeck et al., 2019), or even to decomposition rates (Santiago, 2007). Overall, the traits founded in this spectrum are directly associated with the acquisition, processing, and conservation of resources (Reich, 2014); herein, the economic perspective. There are several traits that integrate the current economic spectrum, but those normalized by leaf area or mass have been used predominantly to group functional types based off of resource-investment strategies (Osnas et al., 2013). The most common traits used are leaf mass per area, nitrogen concentration, or maximum photosynthetic capacity that are strongly modulated by climate, latitude, or biome (Reich et al., 1997, 1999; Reich and Oleksyn, 2004; Wright et al., 2005; Reich, 2014). This modulation tends to be a consequence of internal adaptations of the leaf anatomy (upper epidermis, palisade mesophyll, spongy mesophyll, and lower epidermis) responsible for particular processes (i.e., protective cells, light capture, or conductance) (Villar et al., 2013).
The conceptual framework of the LES is rooted in the selective pressures associated with trade-off between the acquisition and conservation of carbon, water, and nutrients (Reich, 2014). Co-variances between the common functional traits, such as Leaf-Mass Area (LMA), leaf nitrogen concentration by mass (N) or photosynthetic capacity (Amax), have been used to explore the broader implications of natural selection at the community level (Osnas et al., 2013; Reich, 2014). For instance, the positive relationships between the mass-based traits Nmass and Amax (mass-based) are used to understand and equalize the productivity per leaf area or the unit of mass investment (Reich, 2014; John et al., 2017). This suggests there is a selective advantage of increased nitrogen leaf content to photosynthetic capacity across species and ecosystems. A deeper exploration of anatomical limitations and facilitations associated with resource acquisition to this spectrum-wide analysis could provide a structural and mechanical perspective of such generalized co-variances driven by selection.
Previous investigations have focused on specific functional traits’ relationship and correlation with anatomy. For instance, Villar et al. (2013) and John et al. (2017) looked at co-variances between anatomy and LMA or Pyankov et al. (1999) compared anatomy to specific-leaf area and specific-leaf mass. There appears to be a gap in the literature that broadens the scope of multiple functional traits against multiple anatomical traits that relates to LES. Like the leaf, plant, or wood traits, leaf anatomy is likely to face physiological, structural, and defensive trade-offs (Javelle et al., 2011; Sack and Scoffoni, 2013; Somavilla et al., 2014; Onoda et al., 2017). At any given time, the variability of leaf anatomy depends on the species nature and the surrounding pressure on their growth and development (Tichá, 1985; Oguchi et al., 2003; Xiao et al., 2016). The lack of systematic anatomical deconstruction associated with leaf traits creates an incomplete representation of the mechanics by how the LES varies between plants and environments. For example, several anatomical features appear to be important for photosynthesis, such as the palisade thickness, mesophyll cell density, or air spaces that mediate photosynthetic rates, to mention some (Kenzo et al., 2004; Lehmeier et al., 2017). Currently, studies by Liu et al. (2019) suggest that anatomical traits tend to present a weak correlation with leaf economic traits in temperate and subtropical species. However, in tropical species, there is little information regarding how anatomical traits influence leaf traits in different growth forms and how these relationships vary according to ecosystems. The latter is crucial because as a theory LES must be applied equally to different species within the same ecosystem; even those that coexist together and present distinguishing physiological, biochemical, and optical traits such as the case of lianas and trees (Sánchez-Azofeifa et al., 2009; Asner and Martin, 2012; Slot and Winter, 2017; Werden et al., 2017; Guzmán et al., 2018; Guzmán and Sanchez-Azofeifa, 2021).
Lianas –woody vines– are non-self-supporting plants that use host trees to access canopy light, probably affecting the availability of resources around the tree host (Stewart and Schnitzer, 2017). Typically during their development, lianas produce a higher proportion of their biomass in foliage above their host affecting the tree growth, survival, and reproduction (Phillips et al., 2005; Peña-Claros et al., 2008; Ingwell et al., 2010; Wright et al., 2015; Martínez-Izquierdo et al., 2016). Many investigations throughout the tropics have revealed dramatic increases in liana abundance in recent decades, particularly in seasonal ecosystems and dry climatic conditions (Schnitzer and Bongers, 2011; Schnitzer, 2015; Wright et al., 2015). Moreover, some investigations have attributed this recent proliferation to the resource investment strategies of lianas, namely, light capture and growth traits (Asner and Martin, 2012; Wyka et al., 2013). This appears to be a viable investment without the burden of costs associated with self-supporting structures provided by their host tree. Conversely, trees have been reported to invest in traits associated with defence, maintenance, and metabolism (Asner and Martin, 2012; Wyka et al., 2013; Liu et al., 2021).
The aim of this study is to determine whether associations between anatomical and well-established leaf functional traits provide a mechanistic point of view to the trade-off theory of the LES. The associations between different anatomical traits and functional traits are the result of evolutionary and ecological pressures driving patterns along the spectrum as a continuous entity. Here we consider leaf functional traits as those morpho-physiological and structural traits that modulate the fitness of the individual (e.g., growth, reproduction, and survival) (Violle et al., 2007). The leaf anatomical traits studied here, which could also be functional, are focused on fractions of each tissue type and their density (i.e., epidermis, palisade, and spongy mesophyll) based on the cellular organization (i.e., number of cells, cell size, air spaces, and tissue cross area and thickness) in order to normalize the potential differences in leaf size. We attempt to address this by examining the LES using two perspectives: the spectrum as a single axis (i.e., investment patterns regardless of relatedness or climate) and a comparison of potential end members along the spectrum. Overall, the liana–tree co-existence and their contrasting traits make for a convenient comparison as contrasting endmembers of the spectrum. This is also explored at two different forest types (tropical wet forest and tropical dry forest) as a potential descriptor of environmental drivers that modulate the variation in leaf traits. Specifically, here we address two hypotheses: (i) liana and tree species present contrasting leaf anatomical traits exhibiting of resource acquisitive-conservative (fast-slow) strategies according to the forest type, and (ii) the trade-off investment strategies of the leaf anatomical traits are associated with those described in well-established leaf traits. For the first hypothesis, we expect that liana investment in leaf anatomical traits resemble those related to resource acquisitive strategies (fast), while trees to resource conservative (slow) (List of species Table 1). For the second hypothesis, we predict that the variability in leaf anatomical traits have a meaningful correlation, positive or negative, with the variability of well-establish traits, in accordance with investment payoff of a particular trait (Table 2). For example, as palisade cell density increases, Amax and Rd should also increase; this is because palisade anatomical traits which have light capture and growth strategies should exhibit positive relationships with Amax and Rd because they also exhibit light capture and growth functional strategies; or as spongy mesophyll density decreases nitrogen leaf content should increase because there two sets of traits have opposing strategic investments, maintenance and metabolism vs. light capture and growth, respectively (Table 2); The negative association is because there is an inherent energy requirement to maintain the leaf at the cost of resource acquisition ability. The anatomical traits studied here may represent the mechanism by which LES strategies are achieved, improving our understanding of the resource allocation hypothesis embedded in the LES framework.
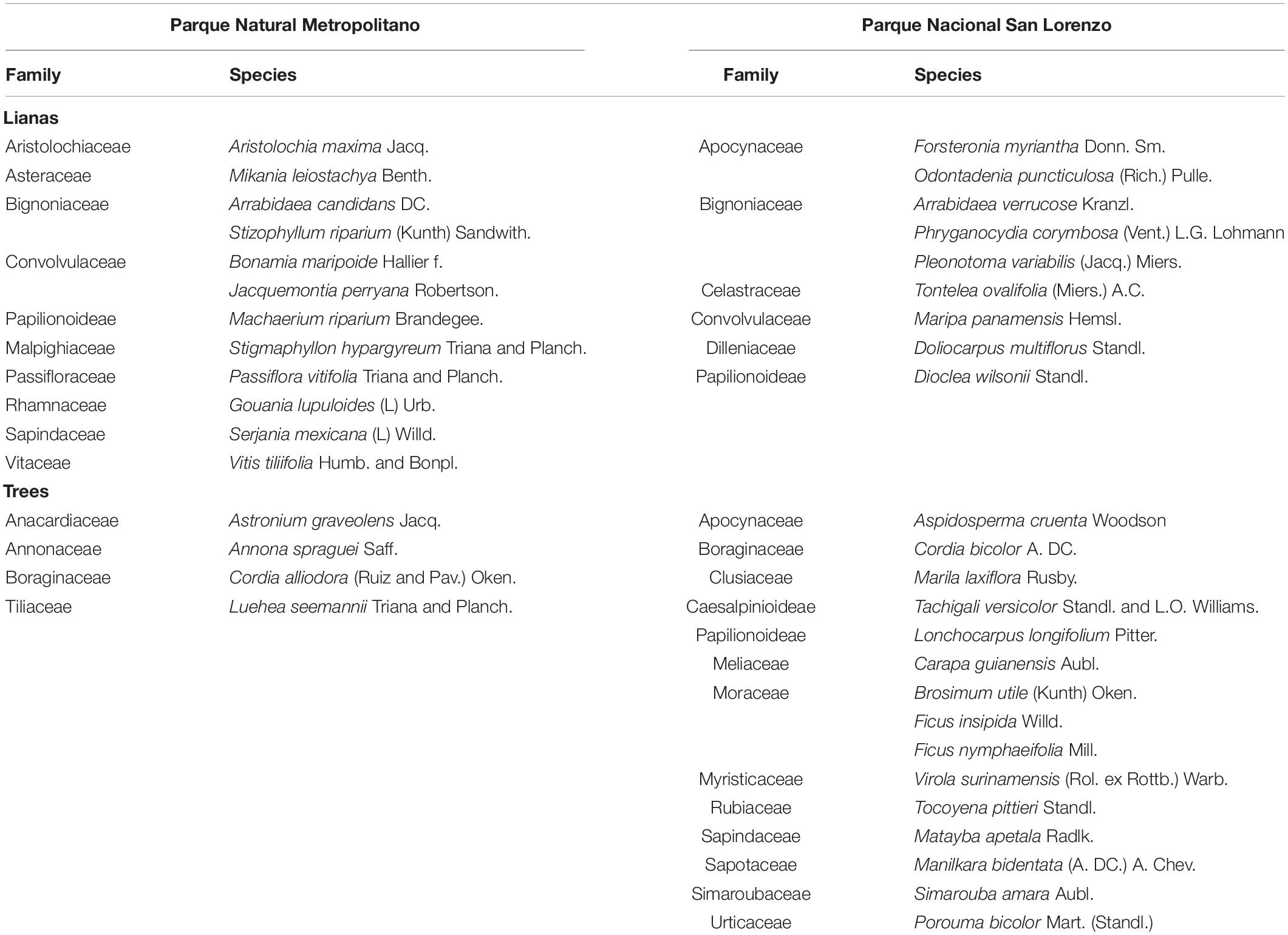
Table 1. List of species of lianas and trees collected at the Parque Natural Metropolitano and Parque Nacional San Lorenzo, Panama.
Materials and Methods
Study Area
This research took place at two canopy crane systems in Panama during the rainy season, August 2004: (i) Parque Natural Metropolitano (PNM, 8° 59′ 39.95″ N, 79° 32′ 34.68″ W, 150 m a.s.l.), and (ii) Parque Nacional San Lorenzo (SL, 9° 16′ 51.71″ N, 79° 58′ 28.27″ W, 130 m a.s.l.). The PNM is located in Panama City on the Pacific coast of the Republic of Panama. This site presents a seasonally dry tropical forest with a mean annual temperature of 26.5°C and an annual rainfall of 1740 mm. The PNM contains a 265 ha of a natural reserve with an old secondary forest of 80–150 years with tree heights up to 40 m (Wright et al., 2003). The PNM site has a 42 m standing crane with a 51 m long jib with a suspended cage that can be used to access the top of the canopy. The crane covers approximately 0.85 ha of forest in which more than 60 species of trees and lianas can be reached (Wright et al., 2003). On the other hand, the SL is a wet evergreen forest located on the Caribbean coast of Panama with a mean annual temperature of 25.3°C and an annual rainfall of 3000 mm. This site presents a weak dry season from January to March with rainfalls below 45 mm per month. The SL contains a 12,000 ha of old forest (∼300 years) that is part of the Mesoamerican Biological Corridor (Wright et al., 2003). This site also presents a standing crane of 52 m tall with a 54 m jib that can be used to access the top of the canopy. This crane provides access to 0.92 ha of the forest where more than 240 species of trees and lianas can be found (Wright et al., 2003).
Sample Collection and Leaf Histology
Three individuals (i.e., trees or lianas) were selected to collect three sun leaves from the top of the canopy using both cranes in the wet season of 2004; therefore, nine leaves per species were sampled. Leaves were collected from 40 species in total (Table 1). Twelve liana species and four tree species were collected from the PNM crane, while nine liana species and fifteen tree species were collected from the SL crane. Each leaf sample immediately upon clipping (early morning) was placed in a plastic bag with a moist paper towel and then transferred within 1-h to a dry ice cooler for transportation to a laboratory. Three small sections (5 mm in width and 20 mm in length) were cut from each leaf while avoiding the presence of prominent vascular areas. The cut-out sections were treated for 2 weeks with formalin aceto-alcohol in a vacuum oven. After the 2 weeks, the samples were run through an ethanol processing centre and transferred to a hot paraffin bath for embedding into paraffin blocks. A detailed description of the process can be found in Sánchez-Azofeifa et al. (2009).
Slides were prepared following Bancroft and Cook (1984) histological preparation protocols. On these samples, seven cross-sections were prepared with a Leica microtome from a paraffin block and mounted onto microscope slides. Each cross-section has a thickness of 7 μm and a width and height of 22 by 22 mm. The slides were placed in an oven at 37°C overnight for roughly 10–12 h. The following morning, slides were de-waxed with a series of diluted ethanol concentrations and then soaked in toluene for 2 min. The slides were then submerged in a toluene blue stain for 22 s. Coverslips were placed over stained samples using a DPX mounting medium. Slides were again placed in 37°C oven for 10–12 h to dry and set the DPX. Finally, cross-sectional photos were taken using a Zeiss M1 Axio Imager microscope at 400 times magnification. All samples had a field of view of 450 μm by 450 μm. For each species, nine leaves were mounted, stained, and photographed.
Estimation of Anatomical Metrics and Traits
The cross-section photographs previously obtained were used to derive metrics in order to quantify the anatomy of leaf tissues. Specifically, the used metrics are focused on describing the area, thickness, number of cells, and cell size of four-leaf sections: (i) upper epidermis (EU), (ii) lower epidermis (EL), (iii) palisade mesophyll (MP), and (iv) spongy mesophyll (MS). These anatomical metrics derived from photos that were previously enhanced by colour and contrast and transformed to black and white using ImageJ 1.46 (Schneider et al., 2012). The area and percentage of the blackened and non-blackened region were used to estimate the cell-occupied space and leaf airspace using the “analysed particles” tools. Likewise, the area and thickness of each leaf section were estimated, making a manual selection of each blackened section using the “region of interest” tool. The area and thickness of each section were calculated by taking the average of 5 measurements per sample. On the other hand, the number of cells was estimated using the edge effect rules with a counting frame (Mbf Bioscience, 2015). For this, a counting frame of 3,000 μm2 central area was established from the centre of the photograph with a 1,500 μm2 buffer zone surrounding the perimeter of the cross-section. Next, each cell at each layer was counted in the selected area. For cells to be included, they needed to be entirely encompassed in the central area and the buffer zone. Once the cell count was established, the number of cells was estimated per mesophyll layer (MP and MS). The estimated cell count was accomplished by multiplying the total area of the mesophyll layers by the estimates cell counts. Likewise, the individual cell size was then estimated with the cell count results. This was accomplished by dividing the cell counts by the area of the mesophyll layers. To eliminate pseudo-replication bias, the sample mean for the individual (three individuals per species) was used as a descriptor of the anatomical metrics.
Once the anatomical metrics were done, we calculated anatomical relationships between metrics to reduce the collinearity among them for future analysis. In general, these relationships are a group of ratios or fractions that can be used to normalize the variation of anatomical metrics, which could be affected by the leaf size. These anatomical relationships are called hereinafter as leaf anatomical traits. We calculated eight anatomical traits based on their potential significance to describe physiological, structural, and defensive trade-offs. Specifically, we calculated (Table 3): (i) palisade area fraction (fMParea), (ii) spongy area fraction (fMSarea), (iii) airspace area fraction (fAIRarea), (iv) palisade density (ρMPcell; number of cells per μm2), (v) spongy density (ρMScell; number of cells per μm2), (vi) epidermis thickness fraction (fEthickness), (vii) palisade thickness fraction (fMPthickness), and (viii) palisade/spongy cell count ratio (MPcount/MScount). For the following traits, fMParea, fMSarea, fAIRarea, fEthickness, fMPthickness, and MPcount/MScount they do not have units because they are fractions. However, area and thickness are measured in μm and cell counts are the number of cells in a given area. Descriptions on how to derive these traits and their potential relevance are described in Table 3.
Well-Established Leaf Functional Traits
We used eight well-established LFTs for each species and forest type obtained from published records from Osnas et al. (2018). Specifically, we used: maximum net photosynthetic rate (Amax, μmol CO2 m–2s–1), dark respiration rate (Rdark, μmol CO2 m–2s–1), nitrogen concentration (N, g m–2), and phosphorus concentration (P, g m–2), leaf mass per area (LMA, g m–2), leaf thickness (LT, mm), leaf density (LD, g cm–3) and leaf lifespan (LL, days). In general, these traits were collected at the same sites under two canopy positions (canopy and understory) from different species, including trees, treelets, lianas, vines, epiphytes, and hemi-epiphytes. For this research, only sun leaves were used to compare against leaf anatomical traits. A detailed description of the data collection and trait calculations can be found at Osnas et al. (2018).
Data Analysis
We first compared the effect of life forms and forest type using MANOVAs at three levels of evaluation: (i) anatomical metrics, (ii) leaf anatomical traits, and (iii) well-established LFTs. For each MANOVA, we extracted the univariate analysis of variance (ANOVA) to describe the effects on each trait. For these analyses, we considered each species as the sample unit using the average of leaves per species, and thus meet the assumption of independent observations. In addition, for these analysis leaf traits variables were initially transformed using Box-Cox or log transformation in order to reach the univariate normality and homogeneity of variance assumptions. The application of the Mahalanobis distance tests did not reveal multivariate outliers at the three levels of evaluation. However, the multivariate normality assumption was not meet in all the cases; probably to the sample size. Therefore, Pillai trace was used as measure of variance given that it is relative robust to multivariate normality deviations, assumptions of homogeneity of the variance–covariance matrices across groups, and unbalanced datasets (Johnson and Field, 1993; Quinn and Keough, 2002). These analyses were performed following the recommendations of Quinn and Keough (2002). On the other hand, we performed bootstrapped pairwise Pearson’s correlation analysis between the leaf anatomical traits and the well-established LFTs to see their association. These correlations were first performed using all the species, and then on separate by life forms and forest type. The previous analyses were conducted in R software version 3.6.1 (R Core Team, 2020) using the “manova” and “aov” functions to compute the MANOVAs and ANOVAs, respectively, the “corr.test” function of the psych package (Revelle, 2017) to estimate the Pearson correlations and their significance, and the boot package for bootstrapping (Canty and Ripley, 2019). Each trait was previously transformed using the logarithmic transformation when the normality of the data was not reached.
Results
Anatomical Metrics
According to the MANOVA, the comparisons of the anatomical metrics between life forms, forest type, and their interactions suggested that the multivariate effect do not contribute to the differences between life forms (Pillai trace(15, 21) = 0.55; p = 0.12), forest types (Pillai trace(15, 21) = 0.56; p = 0.11), or the life form × forest type interaction (Pillai trace(15, 21) = 0.41; p = 0.53). Despite this, the univariate analyses of these comparisons suggested the several anatomical metrics tend to differ between life forms as well as forest types (Table 4). Between life forms specially, the results revealed that liana leaves tend to have less cross-sectional area and are thinner than trees. Likewise, lianas presented lower palisade cross-section area and thickness than trees. However, at the cellular level, lianas seemed to have larger cells and fewer cells in the palisade and spongy leaf tissues. Between forest types on the other hand, species at PNM showed leaves with the less cross-sectional area and thinner that species at SL. Species at SL seemed to have higher spongy cross-section area and thickness than species at PNM. Likewise, the cross-section area of airspaces and the cell count of the spongy leaf tissue seemed to be higher in species at SL than PNM. The univariate comparisons did not reveal an effect of the interaction of life form and forest type in any anatomical metric.
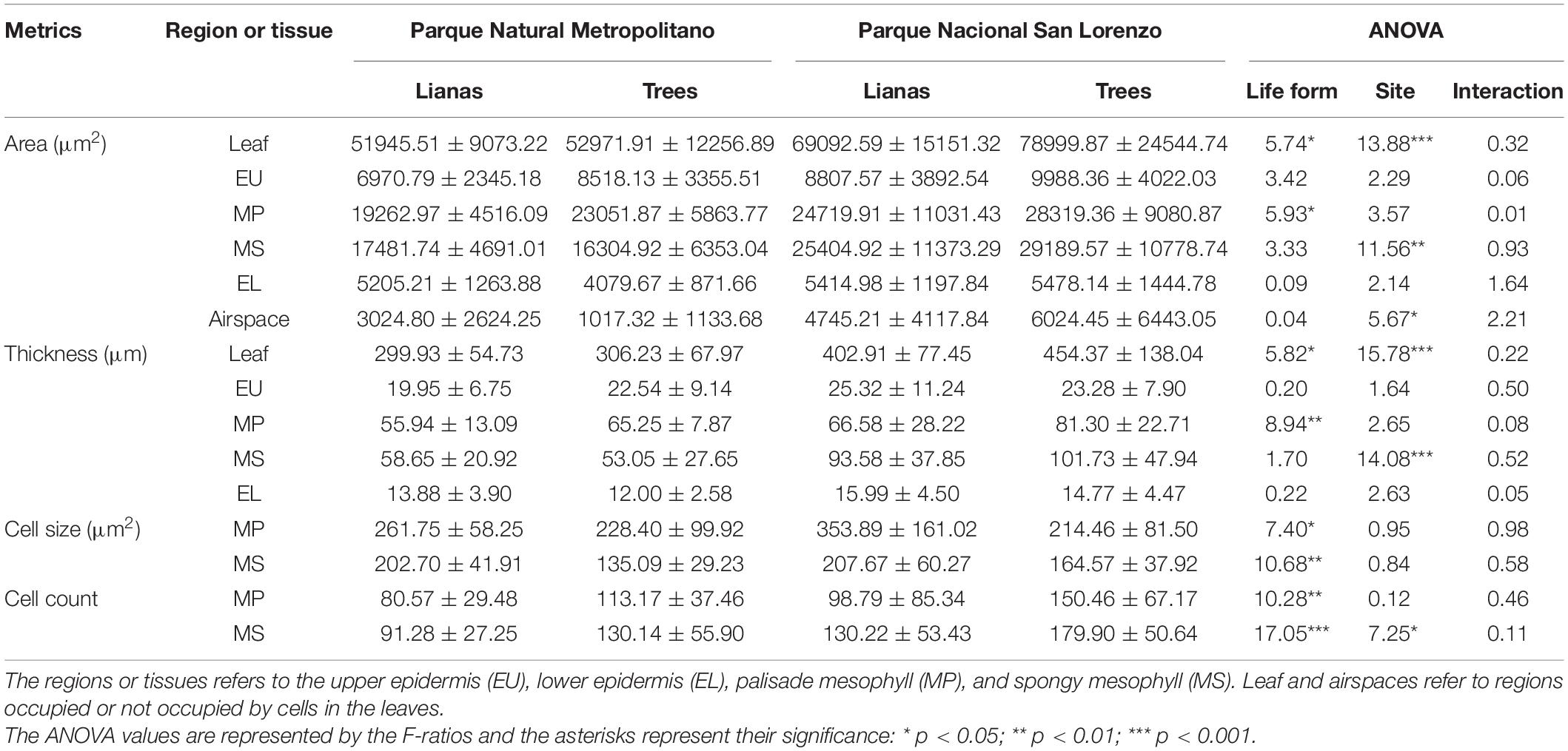
Table 4. Comparison of anatomical metrics between lianas and trees summarized by forest type: seasonally dry forest (Parque Natural Metropolitano) and wet forest (Parque Nacional San Lorenzo).
Leaf Anatomical Traits
The comparisons on the anatomical traits between life forms, forest type, and their interactions using a MANOVA revealed that these traits tend to be affected by life form (Pillai trace(8, 28) = 0.46; p = 0.01), but not by the forest type (Pillai trace(8, 28) = 0.26; p = 0.31) or interaction of life form and forest type (Pillai trace(8, 28) = 0.22; p = 0.45). The univariate comparisons showed that only ρMPcell and ρMScell were affected by the life forms (Table 5), where lianas tended to present higher values than trees (Figures 1D,E). Likewise, the ρMScell is the only trait that seemed to be affected by forest type, where species at PNM presented higher values than species at SL (Figure 1E). Overall, lianas seemed to show higher values of fMSarea, fAIRarea, and fEthickness and lower values of fMParea, fMPthickness, and MPcount/MScount than trees (Figures 1A–C,F–H); however, these differences did not appear to be statistically supported (Table 5).
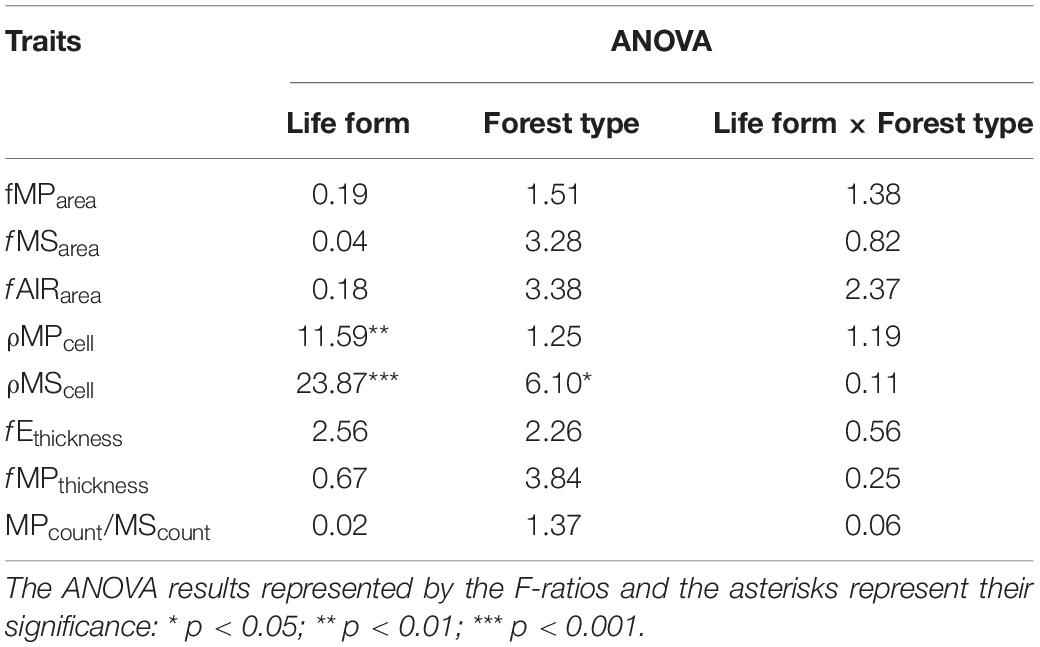
Table 5. Results of the ANOVA comparison of the effect of life forms, forest type, and their interaction on the derived leaf anatomical traits.
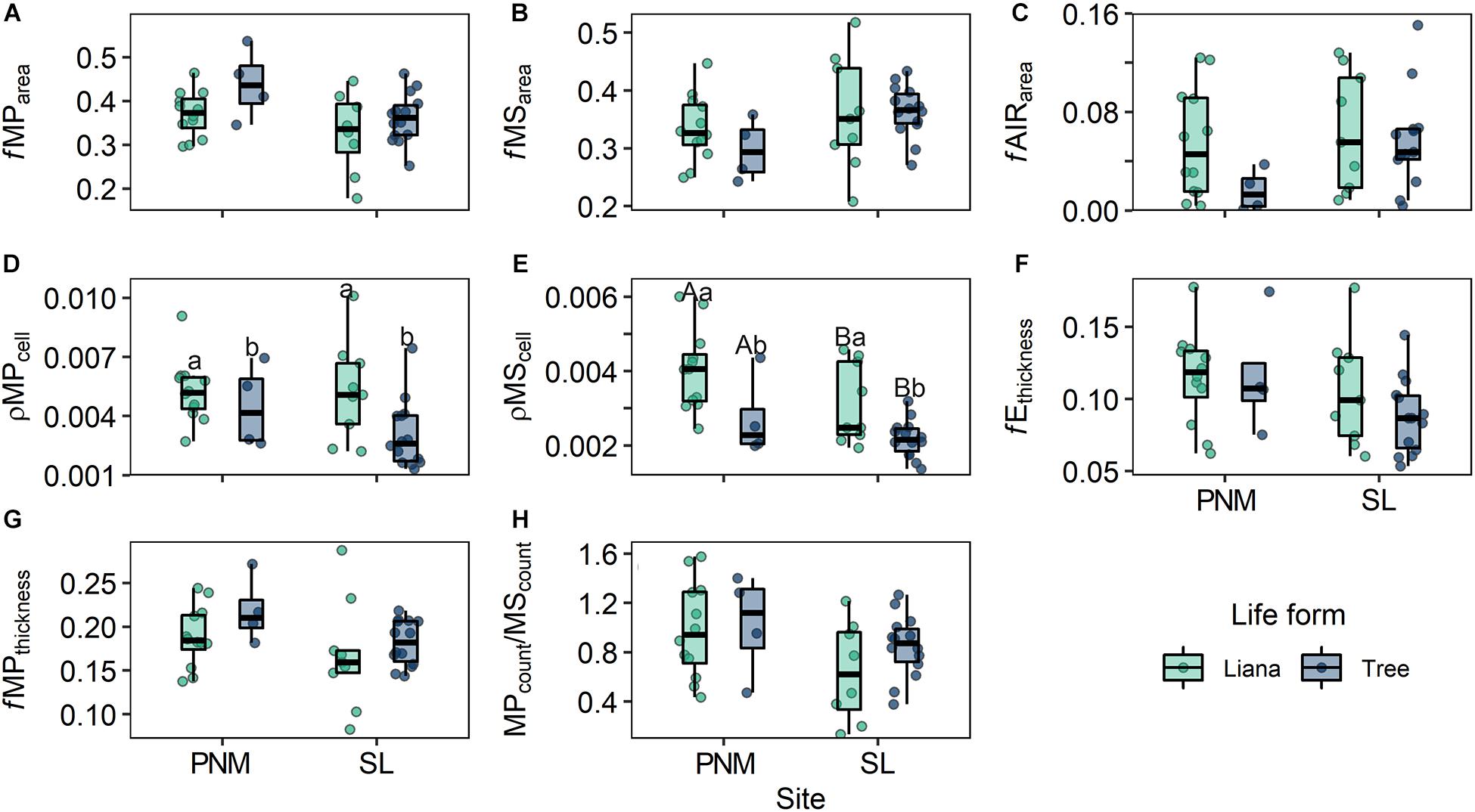
Figure 1. Comparisons of the leaf anatomical traits of lianas and trees at Parque Natural Metropolitano (PNM) and Parque Nacional San Lorenzo (SL). Different capital letters represent a statistical difference between forest types, while different lowercase letters represent a statistical difference between life forms. Each point represents the mean value per species. Each fractional anatomical trait (fMParea, fMSarea, fAIRarea, fEthickness, fMPthickness, and MPcount/MScount) does not have units, however, area and thickness are measured in μm. While the units for, ρMPcell and ρMScell are the number of cells per μm. ANOVA results are described in Table 4. See Table 3 for abbreviations of traits.
Well-Established Leaf Functional Traits
On the other hand, the comparisons of LFT between life forms, forest type, and their interactions revealed that the LFT were affected by the life forms (Pillai trace(8, 21) = 0.66; p < 0.01), forest type (Pillai trace(8, 21) = 0.69; p < 0.001), and their interaction (Pillai trace(8, 21) = 0.58; p < 0.05). The univariate effects of this analysis suggest that only Amax, N, P, LMA, LD, and LL were affected by life forms, where lianas showed lower values than trees (Table 6). Likewise, the univariate effect of this analysis suggested that only P, LMA, and LT were affected by the forest type, where species at PNM showed higher values of P and lower values of LMA and LT than species at SL (Table 6). Despite the presence of multivariate interaction, the univariate analysis did not reveal statistically significant interaction within LFTs.
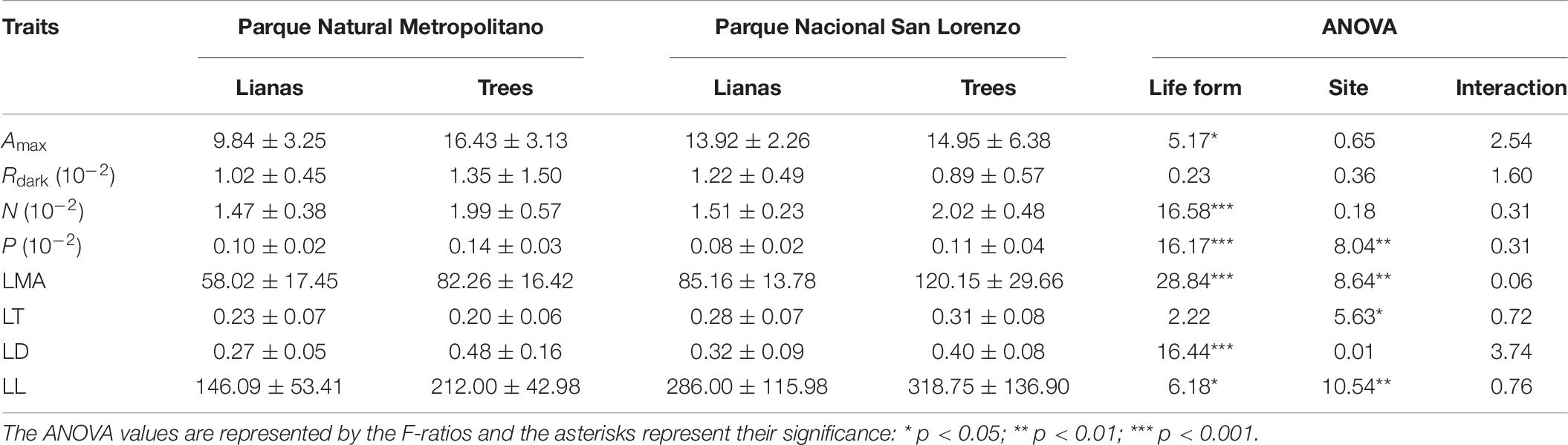
Table 6. Comparison of the well-established leaf traits between lianas and trees summarized by forest type: seasonally dry forest, Parque Natural Metropolitano, and wet forest, Parque Nacional San Lorenzo.
Association Between Anatomical Traits and Well-Established Leaf Functional Traits
The correlations revealed that some leaf anatomical traits were associated with well-established traits (Figure 2A); however, the presence or degree of correlations seemed to be dependent on the life forms and forest type (Figures 2B,C). For instance, reductions in ρMPcell, ρMScell, and fEthickness seemed to be associated with increases in LMA regardless of the life form or forest type (Figure 2A). Likewise, increases in fEthickness tended to be correlated with reductions in LD and LL. Increases in ρMPcell also tended to be associated with reductions in N, while increases in ρMScell tended to be correlated with reductions of Amax and LT. Between life forms (Figure 2B), fMParea and fMPthickness were positively correlated with N, P, and LD in trees, but not in lianas. On the other hand, in liana species increases in ρMPcell, ρMScell, and fEthickness seemed to be correlated with reductions in N, LT, and LD, respectively, but not in trees species. When comparing forest types, the correlations revealed that reductions in ρMPcell, ρMScell of PNM species seemed to be associated with increases in LT, but not for SL species. Likewise, increases of fMParea of PNM species tended to be correlated with increases in LT. In SL species, reductions in fEthickness seemed to be associated with increases in N and LD, while reductions in fAIRarea seemed to be associated with increases in Rdark. A complete figure with all the correlations (i.e., significant or not) was added in Supplementary Figure 1.
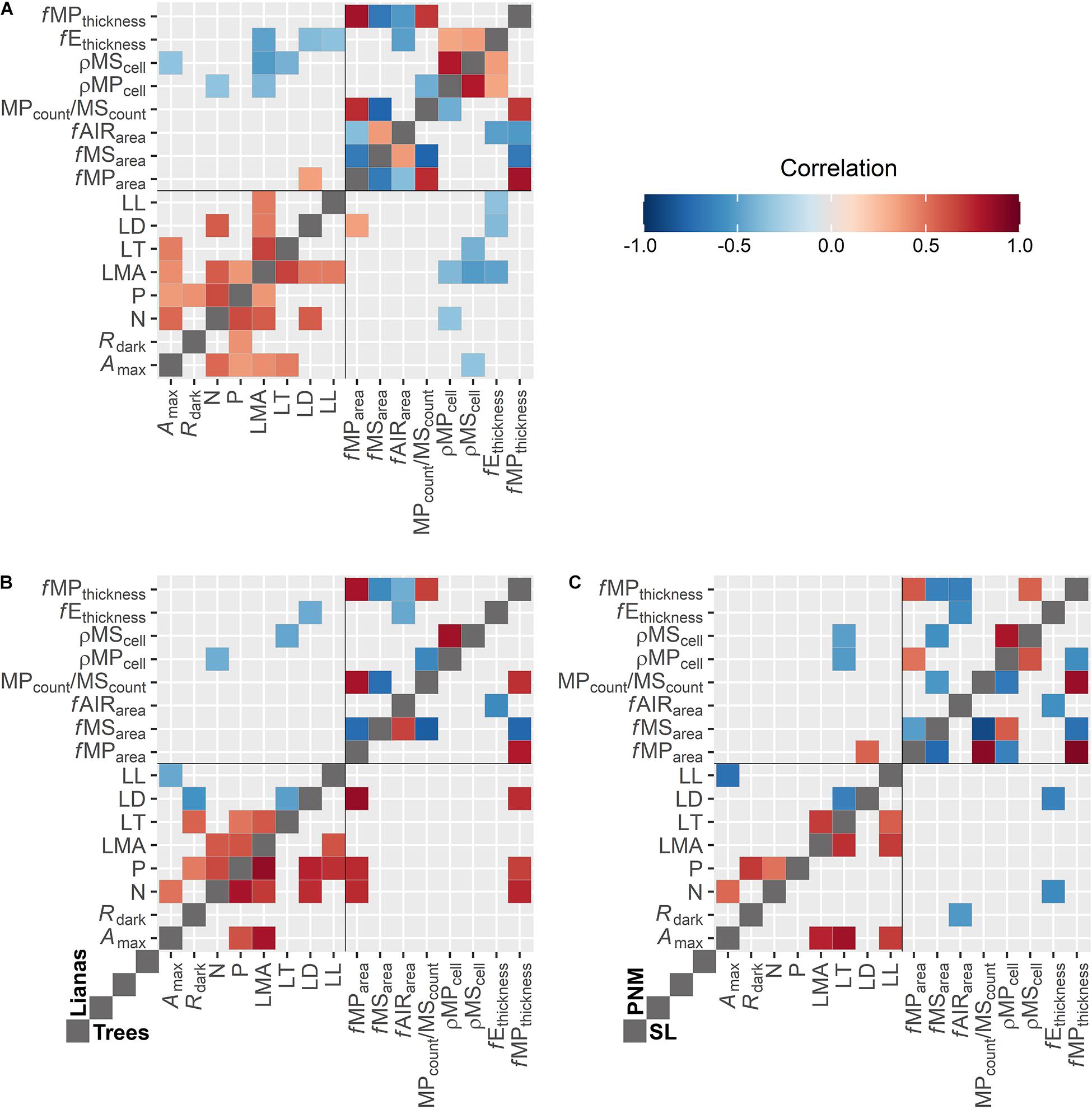
Figure 2. Correlation analysis between leaf anatomical traits and well-established leaf functional traits. All species (A), life forms (B), and forest type (C; PNM: Parque Natural Metropolitano and, and SL: Parque Nacional San Lorenzo). The colours show the bootstrapped pairwise Pearson correlation that are significant (p > 0.05). The grey grid of the middle divides the upper and lower the correlation results between life forms (A) or forest type (B). An extended figure with all the correlation was attached as Supplementary Figure 1.
Discussion
This research reveals several anatomical traits that tend to be contrasting between life forms and forest types as well as the mechanics that drive some of the well-established LFTs. Here, we focus on highlighting the interactions between anatomical traits and LFT, and how these differences reveal potential trade-off strategies between species and environments. Albeit the discussion regarding anatomy and LES has been had before, our finding advances this argument in that are cell and tissue mechanisms associated with morpho-physiological trait investment strategies. Moreover, our results also have merit to the idea that leaf anatomical traits, as an additional scientific exploration tool, can help us better understand the functional ecology of species. From these findings, we argue that anatomical traits could complement the framework of the LES.
End of Spectrum Case Study Comparison- Growth Form and Site
The objective of the endmember perspective of this investigation was to compare groups of species that exhibit fast vs. slow functional strategies. Therefore, our first hypothesis was supported by the association between anatomical traits and LFT may depend on the life forms and forest type. For example, species with higher LMA seem to be associated with reductions in ρMPcell, ρMScell, and fMPthickness, but this association is not observed when comparing life forms or forest types. Despite this, on some occasions, ρMPcell and ρMScell and appear to have a negative association with the variation of LD and LT in lianas, and both forest types. These correlations interestingly mirror the breakdown of LMA as the product of LD and LT (LMA = Leaf volume area × Leaf density) (Poorter et al., 2009).
Trade-offs in investment strategies such as the conservative-acquisitive trade-off hypothesis between lianas and trees (Asner and Martin, 2012; Wyka et al., 2013) could be dissected with the correlative exploration between leaf anatomical traits and the well-established LFT. For example, trees tend to have more in N and P per unit leaf area and higher LD as a conservative strategy. These LFTs mentioned above seem to be positively correlated with fMParea and fMPthickness; probably to boost carbon assimilation for woody structures. On the other hand, with lower metabolic demand (compared to trees) of support structures, lianas traits are generally related to acquisitive strategies with low investment in nutrients and structures for fast growth and high light capture. This strategy appears to be reflected in the increasing cellular infrastructure (ρMPcell, ρMScell, and fEthickness) with high turnover of nutrients as investment and leaf volume demands (LT and LD). In addition, trade-offs in investment strategies between forest types may also reveal how species modify their leaf anatomy to deal with contrasting rainfall regimes. For example, it appears that species at the PNM tend to increase the density of palisade and spongy cells with lower LT which would suggest a loss of airspace. This strategy of dry-forest species may prevent to break the water tension between cells avoiding the leaf hydraulic failure. A negative pressure is formed between mesophyll cells when small menisci of water are formed through adhesion to the outside of the spongy mesophyll cells creating tension and suction as water is lost through the stomata. Conversely, species at the wet-forest seem to increase fAIRarea; to be more metabolically efficient to reduce of Rdark.
The LES as a Continuous Single Axis- an Anatomical Perspective
There is convincing evidence for leaf investment strategies along the LES to exhibit the same patterning of trait correlation across taxonomy and climatic gradients (Wright et al., 2004). The LES maps these universal patterns in which traits that are not energetically unfavourable are lost and thus allows for the LES to be placed along single axis. Our second perspective to the LES takes a closer look at these patterns and dissects the compositional origin. Broadly speaking, we find cellular mechanisms and organization associated with the function of a particular tissue. The anatomical traits that seem to have the most casual impacts are mesophyll densities and epidermal thickness (ρMPcell, ρMScell and fEthickness). For example, decreases in ρMPcell, ρMScell, and fEthickness were found to be associated with an increase in LMA (Figure 2). This cellular structure demonstrates the trade off between metabolic function and the structural integrity of the leaf. Although LMA is thought to be a black box in the literature, John et al. (2017) complementarily found that an increase in cell size (thus decrease in density) drives greater LMA. Moreover, other compositional studies have found a breakdown of LMA by anatomy to be affected equally by protoplast and cell wall components (metabolism and structure, respectively; Shipley et al., 2006; John et al., 2017). A more specific example can be seen in the negative correlation between ρMPcell and N. Given that nitrogen is an important component of chloroplast, more dense palisade results in lower N and thus there is a restriction on photosynthetic capacity. This is supported by Pyankov et al. (1999), who found that mesophyll size and numbers were negatively correlated and that leaves with large cells have more chloroplasts per cell.
When comparing investment strategies types, we found support for our second hypothesis. We found higher epidermal thickness with lower LD and LL. These correlations reflect the carbon allocation investment costs of structural defence. The cuticle and epidermis are considered the first line of defence in plants, this trade off can be seen regardless of phylogenetic and environmental factors. Simply put, this pattern arose from blanketed selective pressures on plants as stationary organisms and the of cost of protection. Moreover, Flexas et al. (2013) have found that across diverse range of species mesophyll conductance is strongly linked to gas exchange traits, stomatal conductance and leaf vein density. Supporting the idea that at the foliar traits can be linked to tissue-level dissection of these traits and there is evolution consistency in these trends.
Moreover, within the spongy mesophyll we found negative relationships between ρMScell and Amax which supports the trade-off hypothesis of different functional strategies (maintenance and metabolism against light capture and growth traits) (Table 2). Increasing spongy mesophyll density with lower Amax is indicative of increased gas diffusion resistance. Less intracellular space reduces the diffusion capacity of the spongy mesophyll and this shows photosynthesis capacity’s limitation associated with the rate of gas diffusion. Liu et al. (2019) found high dry matter content with low SLA suggesting an increase diffusion resistance. Our findings take this one step further and show the allocation of spongy mesophyll tissue to come at a cost of decreased transpiration and photosynthetic capacity despite the wide variability around life form and site type. The application to the LES spectrum is that photosynthetic capacity is limited by its reactants’ and products’ the distance travelled and rate of travel across the spongy anatomy, quite literally, it is subjected to fast-slow continuum.
Future Perspectives of Leaf Anatomical Traits
Currently, there are a few studies that address the role of leaf anatomical traits on the functional ecology of the species or communities, however, they seem to focus on only snap-shot perspectives of the LES; for instance solely discussing the usefulness of LMA (Pyankov et al., 1999; John et al., 2017), or finding a weak correlation between anatomical traits and economic and hydraulic traits (Liu et al., 2019).
The LES was a paradigm shift in plant ecology. This study provides further justification to add anatomical traits to the LES, however, we only focused on leaf traits. The future of this research should focus on anatomical deconstruction at all levels to match the grandiose nature of the LES: cellular, tissue, organ, and systems as well as root, stem, and leaf. Moreover, it seems clear that the degree of correlation of the anatomical traits depends on the different functional groups and environments. Therefore, the differentiation of the co-variation of anatomical traits between species, functional groups, or communities could reflect the different trade-off strategies of species in face to a given environment. Based on this, the use of anatomical decomposition of leaves may provide a deeper understanding of the leaf economy variation along ecological evolutionary and climatic gradients.
Conclusion
The discussion of traits as an explanation to the trade-off between growth and survival is long and continuous. Moreover, the discussion of including anatomy has been recited before, we have pushed this discussion in a direction that shows that leaf anatomy plays specific roles between growth and survival. The novel approach in this investigation was focusing on the LES as a spectrum, in a continuous vs. endmember dissection of the covariances of LFT and anatomy. These covariances are a result of ecological and evolutionary selective pressures. We found mesophyll density and epidermal thickness (ρMPcell, ρMScell, and fEthickness) have a more casual impact when summarizing the LES along singular axis. Mesophyll density is the proverbial stock to the currency of photosynthetic capacity traits as the currencies and epidermal thickness come at a cost of longevity and density. Such trade offs reflect unifying selective pressures along the LES: i-maintenance and metabolism for light capture and growth and ii- structure and defence for maintenance and metabolism, respectively. In contrast, our endmember case study found a group of traits selected for a specific life history. For example, lianas, resource acquisitive or at the “fast” end of the spectrum show positive covariance strategies both favourable to growth such as increase nutrients in their palisade mesophyll. The results where water was the comparative metric demonstrated that when resource was limited (water; PMN) the anatomy reflected this source of selective pressure by reducing thickness and ultimately airspaces. Future studies should investigate deeper in the harmonization of anatomical traits to better explain the leaf anatomy and current LFTs. Likewise, future studies should address the leaf anatomical trait co-variation of different taxonomic groups and how these are adapted to different environments.
Data Availability Statement
Data collected by the authors of leaf anatomical metrics and traits per species and forest types are available at the Tropi-Dry dataverse through https://doi.org/10.7910/DVN/ESLKNT.
Author Contributions
GS-A performed the leaf collection, helped to the interpretation of the results, editing of the manuscript, and got the funding. DH carried out the leaf histology and the measurements of anatomical metrics. JG analysed the data and performed the Figures. DH and JG drafted the manuscript. All the authors agreed with the final version of the manuscript.
Funding
This work was supported by the Natural Science and Engineering Research Council of Canada (NSERC) Discovery grant program. JG was a Vanier Scholar supported by NSERC.
Conflict of Interest
The authors declare that the research was conducted in the absence of any commercial or financial relationships that could be construed as a potential conflict of interest.
Publisher’s Note
All claims expressed in this article are solely those of the authors and do not necessarily represent those of their affiliated organizations, or those of the publisher, the editors and the reviewers. Any product that may be evaluated in this article, or claim that may be made by its manufacturer, is not guaranteed or endorsed by the publisher.
Acknowledgments
We are grateful to the Smithsonian Tropical Research Institute in Panama that provides the access to the canopy crane at both sites. Likewise, we thank S. Joseph Wright for previous discussions of the manuscript and the access to the well-established traits database. Our warm thanks to Janice Cooke for her guide and insights on the leaf anatomical measurements and results.
Supplementary Material
The Supplementary Material for this article can be found online at: https://www.frontiersin.org/articles/10.3389/ffgc.2021.720813/full#supplementary-material
References
Asner, G. P., and Martin, R. E. (2012). Contrasting leaf chemical traits in tropical lianas and trees: implications for future forest composition. Ecol. Lett. 15, 1001–1007. doi: 10.1111/j.1461-0248.2012.01821.x
Bancroft, J., and Cook, H. (1984). Manual of histological techniques. Edinburgh: Churchill Livingstone.
Canty, A., and Ripley, B. (2019). boot: Bootstrap R (S-Plus) Functions. Cambridge: Cambridge University Press.
Chave, J., Coomes, D., Jansen, S., Lewis, S. L., Swenson, N. G., and Zanne, A. E. (2009). Towards a worldwide wood economics spectrum. Ecol. Lett. 12, 351–366. doi: 10.1111/j.1461-0248.2009.01285.x
Flexas, J., Scoffoni, C., Gago, J., and Sack, L. (2013). Leaf mesophyll conductance and leaf hydraulic conductance: an introduction to their measurement and coordination. J. Exp. Bot. 64, 3965–3981. doi: 10.1093/jxb/ert319
Guzmán, J. A., Rivard, B., and Sánchez-Azofeifa, G. A. (2018). Discrimination of liana and tree leaves from a Neotropical Dry Forest using visible-near infrared and longwave infrared reflectance spectra. Remote Sens. Environ. 219, 135–144. doi: 10.1016/j.rse.2018.10.014
Guzmán, J. A., and Sanchez-Azofeifa, G. A. (2021). Prediction of leaf traits of lianas and trees via the integration of wavelet spectra in the visible-near infrared and thermal infrared domains. Remote Sens. Environ. 259:112406. doi: 10.1016/j.rse.2021.112406
Ingwell, L. L., Joseph Wright, S., Becklund, K. K., Hubbell, S. P., and Schnitzer, S. A. (2010). The impact of lianas on 10 years of tree growth and mortality on Barro Colorado Island, Panama. J. Ecol. 98, 879–887. doi: 10.1111/j.1365-2745.2010.01676.x
Javelle, M., Vernoud, V., Rogowsky, P. M., and Ingram, G. C. (2011). Epidermis: the formation and functions of a fundamental plant tissue. New Phytol. 189, 17–39. doi: 10.1111/j.1469-8137.2010.03514.x
John, G. P., Scoffoni, C., Buckley, T. N., Villar, R., Poorter, H., and Sack, L. (2017). The anatomical and compositional basis of leaf mass per area. Ecol. Lett. 20, 412–425. doi: 10.1111/ele.12739
Johnson, C., and Field, C. (1993). Using fixed-effects model multivariate analysis of variance in marine biology and ecology. Oceanogr. Mar. Biol. Annu. Rev. 31, 177–221.
Kenzo, T., Ichie, T., Yoneda, R., Kitahashi, Y., Watanabe, Y., Ninomiya, I., et al. (2004). Interspecific variation of photosynthesis and leaf characteristics in canopy trees of five species of Dipterocarpaceae in a tropical rain forest. Tree Physiol. 24, 1187–1192. doi: 10.1093/treephys/24.10.1187
Kong, D., Wang, J., Wu, H., Valverde-Barrantes, O. J., Wang, R., Zeng, H., et al. (2019). Nonlinearity of root trait relationships and the root economics spectrum. Nat. Commun. 10:2203. doi: 10.1038/s41467-019-10245-6
Lehmeier, C., Pajor, R., Lundgren, M. R., Mathers, A., Sloan, J., Bauch, M., et al. (2017). Cell density and airspace patterning in the leaf can be manipulated to increase leaf photosynthetic capacity. Plant J. 92, 981–994. doi: 10.1111/tpj.13727
Liu, C., Li, Y., Xu, L., Chen, Z., and He, N. (2019). Variation in leaf morphological, stomatal, and anatomical traits and their relationships in temperate and subtropical forests. Sci. Rep. 9:5803. doi: 10.1038/s41598-019-42335-2
Liu, Q., Sterck, F. J., Zhang, J.-L., Scheire, A., Konings, E., Cao, M., et al. (2021). Traits, strategies, and niches of liana species in a tropical seasonal rainforest. Oecologia 196, 499–514. doi: 10.1007/s00442-021-04937-4
Martínez-Izquierdo, L., García, M. M., Powers, J. S., and Schnitzer, S. A. (2016). Lianas suppress seedling growth and survival of 14 tree species in a Panamanian tropical forest. Ecology 97, 215–224. doi: 10.1890/14-2261.1
Mbf Bioscience. (2015). The edge effect with counting frame. Available Online at: http://www.stereology.info/the-edge-effect-with-counting-frame/ [Accessed November 12, 2020]
Oguchi, R., Hikosaka, K., and Hirose, T. (2003). Does the photosynthetic light-acclimation need change in leaf anatomy? Plant. Cell. Environ. 26, 505–512. doi: 10.1046/j.1365-3040.2003.00981.x
Onoda, Y., Wright, I. J., Evans, J. R., Hikosaka, K., Kitajima, K., Niinemets, Ü, et al. (2017). Physiological and structural tradeoffs underlying the leaf economics spectrum. New Phytol. 214, 1447–1463. doi: 10.1111/nph.14496
Osnas, J. L. D., Katabuchi, M., Kitajima, K., Wright, S. J., Reich, P. B., Van Bael, S. A., et al. (2018). Divergent drivers of leaf trait variation within species, among species, and among functional groups. Proc. Natl. Acad. Sci. 115, 5480–5485. doi: 10.1073/pnas.1803989115
Osnas, J. L. D., Lichstein, J. W., Reich, P. B., and Pacala, S. W. (2013). Global leaf trait relationships: mass, area, and the leaf economics spectrum. Science 340, 741–744. doi: 10.1126/science.1231574
Peña-Claros, M., Fredericksen, T. S., Alarcón, A., Blate, G. M., Choque, U., Leaño, C., et al. (2008). Beyond reduced-impact logging: silvicultural treatments to increase growth rates of tropical trees. For. Ecol. Manage. 256, 1458–1467. doi: 10.1016/j.foreco.2007.11.013
Phillips, O. L., Vásquez Martínez, R., Monteagudo Mendoza, A., Baker, T. R., and Núñez Vargas, P. (2005). Large lianas are hyperdynamic elements of the tropical forest canopy. Ecology 86, 1250–1258. doi: 10.1890/04-1446
Poorter, H., Niinemets, Ü, Poorter, L., Wright, I. J., and Villar, R. (2009). Causes and consequences of variation in leaf mass per area (LMA): a meta-analysis. New Phytol. 182, 565–588. doi: 10.1111/j.1469-8137.2009.02830.x
Pyankov, V. I., Kondractchuk, A. V., and Shipley, B. (1999). Leaf structure and specific leaf mass: the alpine desert plants of the Eastern Pamirs, Tadjikistan. New Phytol. 143, 131–142. doi: 10.1046/j.1469-8137.1999.00435.x
Quinn, G., and Keough, M. (2002). Experimental design and data analysis for biologists. New York, USA: Cambridge University Press.
R Core Team. (2020). R: A Language and Environment for Statistical Computing. R Found. Stat. Comput. Available online at: https://www.r-project.org/ (accessed July 29, 2021).
Reich, P. B. (2014). The world-wide ‘fast–slow’ plant economics spectrum: a traits manifesto. J. Ecol. 102, 275–301. doi: 10.1111/1365-2745.12211
Reich, P. B., Ellsworth, D. S., Walters, M. B., Vose, J. M., Gresham, C., Volin, J. C., et al. (1999). Generality of leaf trait relationships: a test across six biomes. Ecology 80, 1955–1969.
Reich, P. B., and Oleksyn, J. (2004). Global patterns of plant leaf N and P in relation to temperature and latitude. Proc. Natl. Acad. Sci. U S A. 101, 11001–11006. doi: 10.1073/pnas.0403588101
Reich, P. B., Walters, M. B., and Ellsworth, D. S. (1997). From tropics to tundra: Global convergence in plant functioning. Proc. Natl. Acad. Sci. 94, 13730–13734.
Revelle, W. (2017). psych: Procedures for Personality and Psychological Research. Available Online at: https://cran.r-project.org/package=psych (accessed July 29, 2021).
Sack, L., and Scoffoni, C. (2013). Leaf venation: structure, function, development, evolution, ecology and applications in the past, present and future. New Phytol. 198, 983–1000. doi: 10.1111/nph.12253
Sánchez-Azofeifa, G. A., Castro, K., Wright, S. J., Gamon, J., Kalacska, M., Rivard, B., et al. (2009). Differences in leaf traits, leaf internal structure, and spectral reflectance between two communities of lianas and trees: Implications for remote sensing in tropical environments. Remote Sens. Environ. 113, 2076–2088. doi: 10.1016/j.rse.2009.05.013
Santiago, L. S. (2007). Extending the leaf economics spectrum to decomposition: evidence from a tropical forest. Ecology 88, 1126–1131. doi: 10.1890/06-1841
Schneider, C. A., Rasband, W. S., and Eliceiri, K. W. (2012). NIH Image to ImageJ: 25 years of image analysis. Nat. Methods 9, 671–675. doi: 10.1038/nmeth.2089
Schnitzer, S. (2015). “Increasing liana abundance in neotropical forests: causes and consequences,” in Ecology of Lianas. Hoboken, NJ: John Wiley & Sons, Ltd, 451–464.
Schnitzer, S., and Bongers, F. (2011). Increasing liana abundance and biomass in tropical forests: emerging patterns and putative mechanisms. Ecol. Lett. 14, 397–406. doi: 10.1111/j.1461-0248.2011.01590.x
Shipley, B., Lechowicz, M., Wright, I., and Reich, P. (2006). Fundamental trade-offs generating the worldwide leaf economics spectrum. Ecology 87, 535–541. doi: 10.1890/05-1051
Slot, M., and Winter, K. (2017). In situ temperature response of photosynthesis of 42 tree and liana species in the canopy of two Panamanian lowland tropical forests with contrasting rainfall regimes. New Phytol. 214, 1103–1117. doi: 10.1111/nph.14469
Somavilla, N. S., Kolb, R. M., and Rossatto, D. R. (2014). Leaf anatomical traits corroborate the leaf economic spectrum: a case study with deciduous forest tree species. Brazil. J. Bot. 37, 69–82. doi: 10.1007/s40415-013-0038-x
Stewart, T. E., and Schnitzer, S. A. (2017). Blurred lines between competition and parasitism. Biotropica 49, 433–438. doi: 10.1111/btp.12444
Tichá, I. (1985). “Ontogeny of leaf morphology and anatomy,” in Photosynthesis during leaf development, ed. Z. Šestăk (Dordrecht: Springer Netherlands), 16–50.
Verbeeck, H., Bauters, M., Jackson, T., Shenkin, A., Disney, M., and Calders, K. (2019). Time for a plant structural economics spectrum. Front. For. Glob. Chang. 2:43. doi: 10.3389/ffgc.2019.00043
Villar, R., Ruiz-Robleto, J., Ubera, J. L., and Poorter, H. (2013). Exploring variation in leaf mass per area (LMA) from leaf to cell: An anatomical analysis of 26 woody species. Am. J. Bot. 100, 1969–1980. doi: 10.3732/ajb.1200562
Violle, C., Navas, M.-L., Vile, D., Kazakou, E., Fortunel, C., Hummel, I., et al. (2007). Let the concept of trait be functional! Oikos 116, 882–892. doi: 10.1111/j.0030-1299.2007.15559.x
Werden, L. K., Waring, B. G., Smith-Martin, C. M., and Powers, J. S. (2017). Tropical dry forest trees and lianas differ in leaf economic spectrum traits but have overlapping water-use strategies. Tree Physiol. 38, 517–530. doi: 10.1093/treephys/tpx135
Wright, I. J., Reich, P. B., Cornelissen, J. H. C., Falster, D. S., Groom, P. K., Hikosaka, K., et al. (2005). Modulation of leaf economic traits and trait relationships by climate. Glob. Ecol. Biogeogr. 14, 411–421. doi: 10.1111/j.1466-822x.2005.00172.x
Wright, I. J., Reich, P. B., Westoby, M., Ackerly, D. D., Baruch, Z., Bongers, F., et al. (2004). The worldwide leaf economics spectrum. Nature 428, 821–827. doi: 10.1038/nature02403
Wright, S. J., Horlyck, V., Basset, Y., Barrios, H., Bethancourt, A., Bohlman, S. A., et al. (2003). “Tropical canopy biology program, Republic of Panama,” in Studying Forest Canopies from Above: The International Canopy Crane Network, eds Y. Basset, V. Horlyck, and S. Wright (Balboa, Panama: Smithsonian Tropical Research Institute, Panama and the United Nations Environmental Programme), 137–155.
Wright, S. J., Sun, I.-F., Pickering, M., Fletcher, C. D., and Chen, Y.-Y. (2015). Long-term changes in liana loads and tree dynamics in a Malaysian forest. Ecology 96, 2748–2757. doi: 10.1890/14-1985.1
Wyka, T. P., Oleksyn, J., Karolewski, P., and Schnitzer, S. A. (2013). Phenotypic correlates of the lianescent growth form: a review. Ann. Bot. 112, 1667–1681. doi: 10.1093/aob/mct236
Keywords: life forms, functional traits, leaf mass per area, leaf anatomy, leaf economic spectrum
Citation: Harrison D, Guzmán Q JA and Sánchez-Azofeifa GA (2021) Leaf Anatomical Traits of Lianas and Trees at the Canopy of Two Contrasting Lowland Tropical Forests in the Context of Leaf Economic Spectrum. Front. For. Glob. Change 4:720813. doi: 10.3389/ffgc.2021.720813
Received: 05 June 2021; Accepted: 28 July 2021;
Published: 17 August 2021.
Edited by:
Geertje M. F. Van Der Heijden, University of Nottingham, United KingdomReviewed by:
Louis S. Santiago, University of California, Riverside, United StatesChris Chandler, University of Nottingham, United Kingdom
Copyright © 2021 Harrison, Guzmán Q and Sánchez-Azofeifa. This is an open-access article distributed under the terms of the Creative Commons Attribution License (CC BY). The use, distribution or reproduction in other forums is permitted, provided the original author(s) and the copyright owner(s) are credited and that the original publication in this journal is cited, in accordance with accepted academic practice. No use, distribution or reproduction is permitted which does not comply with these terms.
*Correspondence: G. Arturo Sánchez-Azofeifa, arturo.sanchez@ualberta.ca