- 1Division of Biogeochemistry of Agroecosystems, Georg-August University of Göttingen, Göttingen, Germany
- 2Department of Water and Soil, Faculty of Agricultural Engineering, Shahrood University of Technology, Shahrood, Iran
- 3Chair of Soil Physics, Bayreuth Center of Ecology and Environmental Research (BayCEER), University of Bayreuth, Bayreuth, Germany
- 4Department of Agroecosystems, Faculty of Agriculture, University of South Bohemia, České Budějovice, Czechia
- 5Department of Soil and Plant Microbiome, Institute of Phytopathology, Christian-Albrechts-University of Kiel, Kiel, Germany
- 6Department of Soil Science, Institute for Plant Nutrition and Soil Science, Christian-Albrechts-University Kiel, Kiel, Germany
- 7Soil Science of Temperate Ecosystems, University of Göttingen, Göttingen, Germany
- 8School of Geographical Sciences, Nanjing University of Information Science and Technology, Nanjing, China
Soil compaction associated with mechanized wood harvesting can long-lastingly disturb forest soils, ecosystem function, and productivity. Sustainable forest management requires precise and deep knowledge of logging operation impacts on forest soils, which can be attained by meta-analysis studies covering representative forest datasets. We performed a meta-analysis on the impact of logging-associated compaction on forest soils microbial biomass carbon (MBC), bulk density, total porosity, and saturated hydraulic conductivity (Ksat) affected by two management factors (machine weight and passage frequency), two soil factors (texture and depth), and the time passed since the compaction event. Compaction significantly decreased soil MBC by −29.5% only in subsoils (>30 cm). Overall, compaction increased soil bulk density by 8.9% and reduced total porosity and Ksat by −10.1 and −40.2%, respectively. The most striking finding of this meta-analysis is that the greatest disturbance to soil bulk density, total porosity, and Ksat occurs after very frequent (>20) machine passages. This contradicts the existing claims that most damage to forest soils happens after a few machine passages. Furthermore, the analyzed physical variables did not recover to the normal level within a period of 3–6 years. Thus, altering these physical properties can disturb forest ecosystem function and productivity, because they play important roles in water and air supply as well as in biogeochemical cycling in forest ecosystems. To minimize the impact, we recommend the selection of suitable logging machines and decreasing the frequency of machine passages as well as logging out of rainy seasons especially in clayey soils. It is also very important to minimize total skid trail coverage for sustainable forest management.
Introduction
Forests cover approximately 30% of the earth’s terrestrial area, providing several important ecosystem services such as food, water, raw materials, climate regulation, erosion prevention, nutrient cycling, biodiversity conservation, and recreation (Groot et al., 2012; Holden and Treseder, 2013). However, demand for forest products is increasing, which can severely affect these ecosystem services and result in a set of massive problems for the environment and human welfare (Lidskog et al., 2013; Acharya et al., 2019).
Soils, as non-renewable resources, are crucial components of forest ecosystems that ensure forest productivity and biodiversity conservation by regulating water and nutrients cycles (Dominati et al., 2010). For instance, forest soils store more than 40% of the total organic carbon in terrestrial ecosystems and are consequently important regulators of global carbon dioxide (Certini et al., 2003; Bonan, 2008; Neruda et al., 2010; Wei et al., 2014).
Abiotic forest-disturbances factors are categorized as fire, storm with windthrow, and anthropogenic tree harvesting (Holden and Treseder, 2013). Tree harvesting generally leads to a reduction in soil carbon stocks (Mayer et al., 2020). Moreover, mechanized logging and log transport by skidders, forwarders, and tractors can remarkably damage forest soils and reduce forest productivity (Horn et al., 2007; Picchio et al., 2011; Hartmann et al., 2014). Although the efficiency and power of logging machines have been increased in recent years, they have also become heavier (Vossbrink and Horn, 2004; Horn et al., 2007). This suggests that disturbance to forest soils and thereby to the forest ecosystem function caused by logging operations can be more severe and long-lasting (Hartmann et al., 2014; Cambi et al., 2015).
Soil compaction is one of the most pronounced consequences of mechanized logging operations, caused by imposed vertical and horizontal stresses on the soil (McNabb et al., 2001; Ares et al., 2005; Cambi et al., 2015). Created skid trails and ruts by logging operations (Figure 1) can considerably disturb soils physical, chemical, and biological properties and severely alter the forest ecosystem function (Heninger et al., 2002; Powers et al., 2004; Agherkakli et al., 2010; Cambi et al., 2015). For example, soil compaction influences between 10 and 70% of the logged stand, indicating its substantial potential to disturb the soil ecosystem and forest (Frey et al., 2009; Picchio et al., 2012).
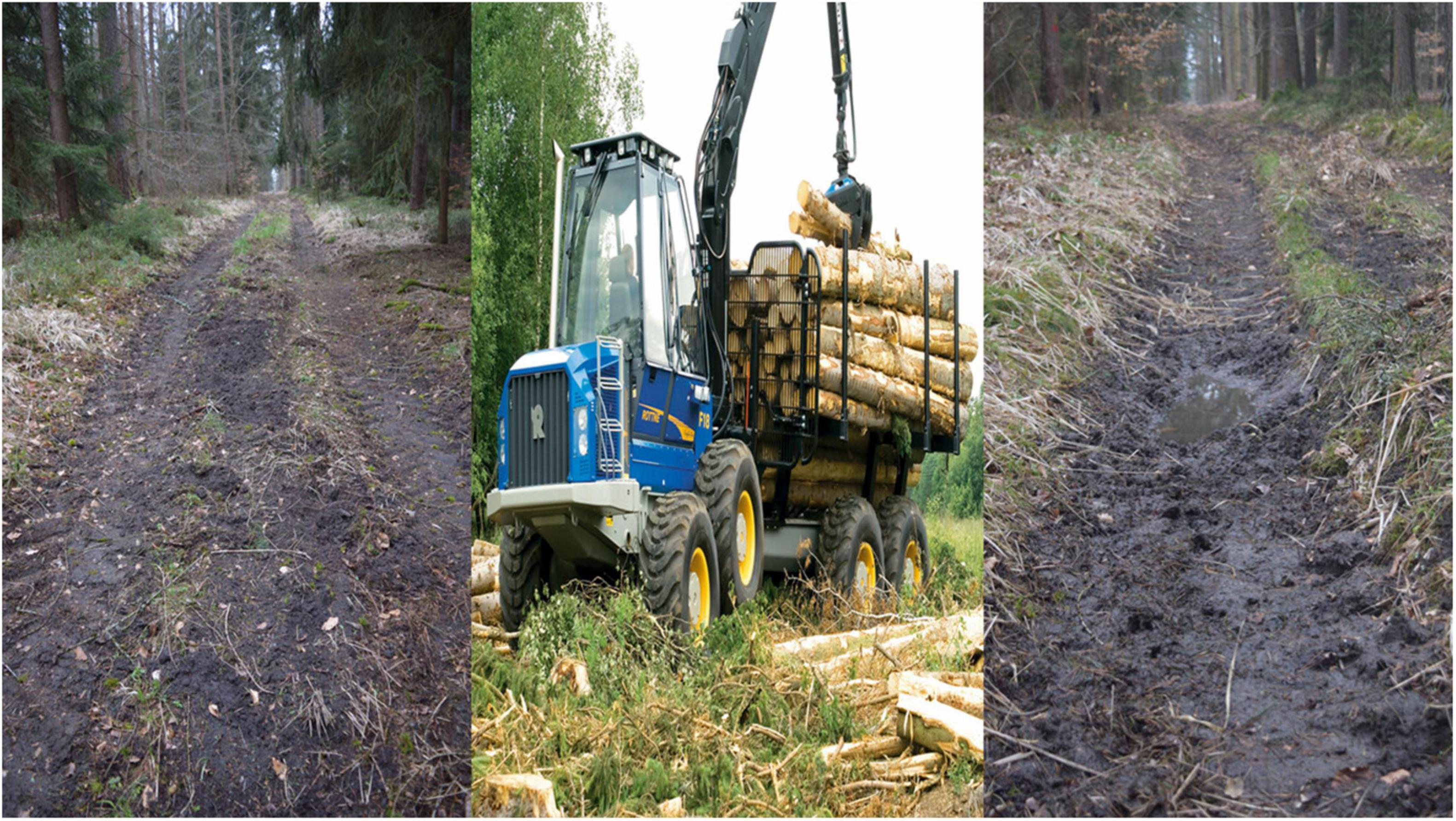
Figure 1. Skid trail (left) and rut (right) created by a wheeled skidder (Rottne Solid F14 8WD, middle) during logging operations in a mixed spruce (Picea abies L.), beech (Fagus sylvatica L.), and fir (Abies alba L.) forest in Kulmbach, Bavaria, Germany. The images clearly illustrate soil compaction and displacement. The image of the wheeled skidder was adopted from https://www.rottne.com.
Forest soils usually have low bulk density values due to their richness in organic matter, specifically in the upper layers (Corti et al., 2002). Commonly, a low soil bulk density makes the soil more prone to compaction (Williamson and Neilsen, 2000; Powers et al., 2004). An increased bulk density of forest soils due to compaction can reduce the total porosity by 50–60% (Frey et al., 2009; Picchio et al., 2012; Solgi and Najafi, 2014). Reduction in soil total porosity dramatically disturbs the soil oxygen diffusion and water balance, the latter causing waterlogging, runoff, and erosion (Jansson and Johansson, 1998; Startsev and McNabb, 2000; Grace et al., 2006; Ampoorter et al., 2007; Schack-Kirchner et al., 2007). Consequently, oxygen and water availability to tree roots and soil microorganisms are reduced, leading to disturbed forest productivity and ecosystem services (Kozlowski, 1999; Ares et al., 2005; Agherkakli et al., 2010).
Compaction may also affect microbial biomass carbon (MBC) in forest soils. In general, soil compaction remarkably reduces soil MBC, due to reduction in total porosity, pore connectivity, and air and water supply (Wronski and Murphy, 1994; Breland and Hansen, 1996; Startsev et al., 1998; Tan et al., 2008; Frey et al., 2009), reducing the availability of key growth resources (oxygen, dissolved organic carbon, mineral nutrients, etc.) to soil microorganisms. In contrast, some other studies observed no significant impact of compaction on MBC (Ponder and Tadros, 2002; Jordan et al., 2003; Nazari et al., 2020). These contrasting results might be attributed to site-specific properties and experimental settings like timespan between the compaction event and soil sampling (Nazari et al., 2020). It is very plausible that sampling soil after a short timespan will not result in a significantly reduced MBC, because compaction does not directly kill microorganisms but indirectly affects their habitat and resources availability.
The degree of soil deformation by compaction depends on several properties such as initial bulk density, texture, depth, organic matter content, and water content and also on management factors such as weight and passage frequency of harvesting machines as well as the time after the harvesting event (Ballard, 2000; Jamshidi et al., 2008; Cambi et al., 2015; Nazari et al., 2020). For instance, coarse-textured soils are commonly more resistant to compaction compared to fined-textured soils (McNabb et al., 2001; Sakai et al., 2008; Magagnotti et al., 2012). Fined-textured soils generally hold more water than coarse-textured ones and thereby make the soil more susceptible to compaction (Cambi et al., 2015). Another example is that logging machines affect soil mostly after a few passages and the later more machine passages have minor impacts on the soil (Wang, 1997; Wallbrink et al., 2002; Han et al., 2006). Nonetheless, the progressive impact of machine passages also depends on several factors such as soil texture and depth as well as on machine configuration (Williamson and Neilsen, 2000; Cambi et al., 2015). The weight of logging machines is another important controlling factor of soil compaction. For instance, lighter machines were found to have lower impacts on soil than heavier ones (Jansson and Wästerlund, 1999; Picchio et al., 2012).
Forest managers have to minimize the impact of logging operations on soil for sustainable forestry. A necessary prerequisite for achieving this goal is the access to precise and profound knowledge of the influence of logging operations on forest soils. So far, there have been a few review studies on the impacts of logging operations on forest soils (i.e., Cambi et al., 2015; Picchio et al., 2020). Although these review studies provide readers with valuable, comprehensive and profound information, they are rather theoretical. Meanwhile, the increasing number of published papers in recent years on the influence of harvesting machines on forest soils calls for conducting meta-analysis studies using all available datasets to provide a universal picture, which were missing so far (Cambi et al., 2015).
Therefore, this meta-analysis aimed at investigating the influence of logging machines management factors (machine weight and passage frequency) and some controlling factors (soil texture, depth, time passed since compaction) on MBC, bulk density, total porosity, and saturated hydraulic conductivity (Ksat) in forest soils. This study can be regarded as guidance for forest managers for their decision-making between alternative management practices.
Methods
Literature Survey and Selection Criteria
This meta-analysis was conducted using previously published peer-reviewed studies (1983–2021). We identified papers that reported bulk density, total porosity, saturated hydraulic conductivity (Ksat), and MBC in undisturbed (control) and compacted (treatment) forest soils using the online database search engines Web of Science and Google Scholar. Keywords used for literature search were combinations of terms such as forest, soil, compaction, logging, bulk density, porosity, hydraulic conductivity, and microbial biomass. The following main criteria were applied to select appropriate studies: (i) all papers were based on field experiments rather than laboratory trials, (ii) all studies were conducted in forest environments, and (iii) all studies reported results from an undisturbed (control) and compacted (treatment) soil.
Collection of Data
We reviewed over 500 papers and found that 67 of them met our selection criteria (Gent et al., 1983; Froehlich et al., 1985; Allbrook, 1986; Dick et al., 1988; Gayoso and Iroume, 1991; Shepperd, 1993; Aust et al., 1995; Startsev et al., 1998; Jordan et al., 2000; Startsev and McNabb, 2000; Williamson and Neilsen, 2000; Brais, 2001; McNabb et al., 2001; Gomez et al., 2002; Chen et al., 2003; Li et al., 2004; Teepe et al., 2004; Eliasson, 2005; Shestak and Busse, 2005; Tan et al., 2005; Busse et al., 2006; Grace et al., 2006; Mariani et al., 2006; Schnurr-Pütz et al., 2006; Ampoorter et al., 2007; Makineci et al., 2007; Schack-Kirchner et al., 2007; Tan and Chang, 2007; Wang et al., 2007; Ziegler et al., 2007; Jamshidi et al., 2008; Tan et al., 2008; Frey et al., 2009; Agherkakli et al., 2010; Bagheri et al., 2012; Brevik and Fenton, 2012; Picchio et al., 2012; Soltanpour and Jourgholami, 2013; Hartmann et al., 2014; Naghdi and Solgi, 2014; Solgi and Najafi, 2014; Naghdi et al., 2015, 2016; Cambi et al., 2016a,b, 2017a,b; Eroğlu et al., 2016; Naghdi and Mousavi, 2016; Proto et al., 2016; Abdi et al., 2017; Etehadi Abari et al., 2017; Shabaga et al., 2017; Tavankar et al., 2017, 2021; Bigelow et al., 2018; Hansson et al., 2018; Sohrabi et al., 2019, 2020; Picchio et al., 2018, 2019, 2020; Venanzi et al., 2019, 2020a,b; Nazari et al., 2020; Jourgholami et al., 2021). The studies included in this meta-analysis are indicated by an asterisk in the references list. In total, 1496 observations or 748 pairs of observations (effect sizes) were extracted from those studies meeting our criteria and were used in this meta-analysis. These datasets consisted of the bulk density, total porosity, and Ksat of undisturbed and compacted forest soils affected by different machine weights, machine passage frequency, soil textures and depths, as well as the time passed since the compaction event (sampling time). They also consisted of the MBC of undisturbed and compacted forest soils affected by different soil textures and depths as well as the time passed since the compaction event. It is important to note that most of the studies on soil MBC did not mention machine weight and passage frequency, whereby these two management factors were excluded from the analysis of soil MBC.
Machine weights were categorized as light (<13 Mg), medium (13–20 Mg), and heavy (>20 Mg). Machine passage frequencies were classified as very few (<4), few (4–7), medium (8–11), frequent (11–20), and very frequent (>20). Soil textures were grouped as sandy, loam, and clayey based on the dominant particle fraction. Four depth groups were considered including surface (<10 cm), shallow (10–19 cm), medium (20–30 cm), and deep (>30 cm). Time periods passed since the compaction event were categorized as immediate (sampling on day zero), short-term (sampling after <1 year), medium-term (sampling after 1–3 years), long-term (sampling after 3–6 years) for the soil physical characteristics and as short-term (<1 year), medium-term (1–6 years), and long-term (6–10 years) for soil MBC.
Meta-Data Analyses
Meta-analysis calculates the magnitude of change of a variable and the significance of this change in response to a treatment. The magnitude of change is called the effect size. The natural logarithm of the response ratio (RR) was used to estimate the effect size (Hedges et al., 1999):
where XC and XT are the means of the variable in the control and compaction treatment, respectively. The RR can be conceptualized as the percentage change after back-transformation as (eln(RR) −1) × 100 (Nave et al., 2010). For generating confidence intervals (CIs) around effect sizes, we recorded standard deviation (SD) and number of replicates (n) of soil bulk density, total porosity, Ksat, and MBC for the control and compaction treatment. Data presented in graphs were digitized using WebPlotDigitizer 4.4.1 If a study reported standard error (SE) or coefficient of variation (CV), the SD was calculated by the formulas SD = SE × √n and SD = CV × mean. The statistical significance was tested using 95% CI for each effect size. If the 95% CIs did not overlap with the zero line, the response was statistically significant at P ≤ 0.05. The group means were significantly different from each other if their 95% CIs did not overlap. In some of the investigated studies, soil bulk density, total porosity, and Ksat were reported simultaneously, based on which regression tests were conducted to detect any interrelationships. A non-linear regression test was used for soil total porosity versus bulk density and for Ksat versus bulk density, because the data were not normally distributed. Data collection and organization as well as all calculations (i.e., effect size and CIs) were performed by Microsoft Excel 2019. Regression analyses and creation of forest plots were conducted using IBM SPSS Statistics for Windows, version 25 (IBM Corp., Armonk, NY, United States).
For assessing the presence of publication bias, we implemented the Spearman rank correlation test in order to test for the relationship between the replicate number of each study and the effect size (Holden and Treseder, 2013). In addition, we visually evaluated funnel plots of the SE versus the effect size (Egger et al., 1997). Publication bias was assessed in all soil physical data for all factors except time. No publication bias was inspected in the soil MBC data for any of the factors. It is worth noting that the assessed publication bias for the physical properties could be due to the nature of the findings (Egger et al., 1997), i.e., compaction probably always has a direct and significant effect on the physical properties.
Results and Discussion
Soil Microbial Biomass Carbon
Across all studies, soil MBC was not significantly affected by compaction at α = 0.05 (Figure 2). Compaction neither significantly affected MBC in soils of different textures (sandy, loam, clayey) nor at the shallower soil depths (surface 0–9 cm, shallow 10–19 cm, and medium 20–30 cm), but it significantly negatively affected MBC at deep (>30 cm) depth (by −29.5%). Moreover, compaction induced a higher reduction in MBC (−35.9 and −21.8%) at deep depth than surface and medium depths, respectively. Time periods passed since compaction (short-term <1 year, medium-term 1–6 years, long-term 6–10 years) had no significant impact on soil MBC.
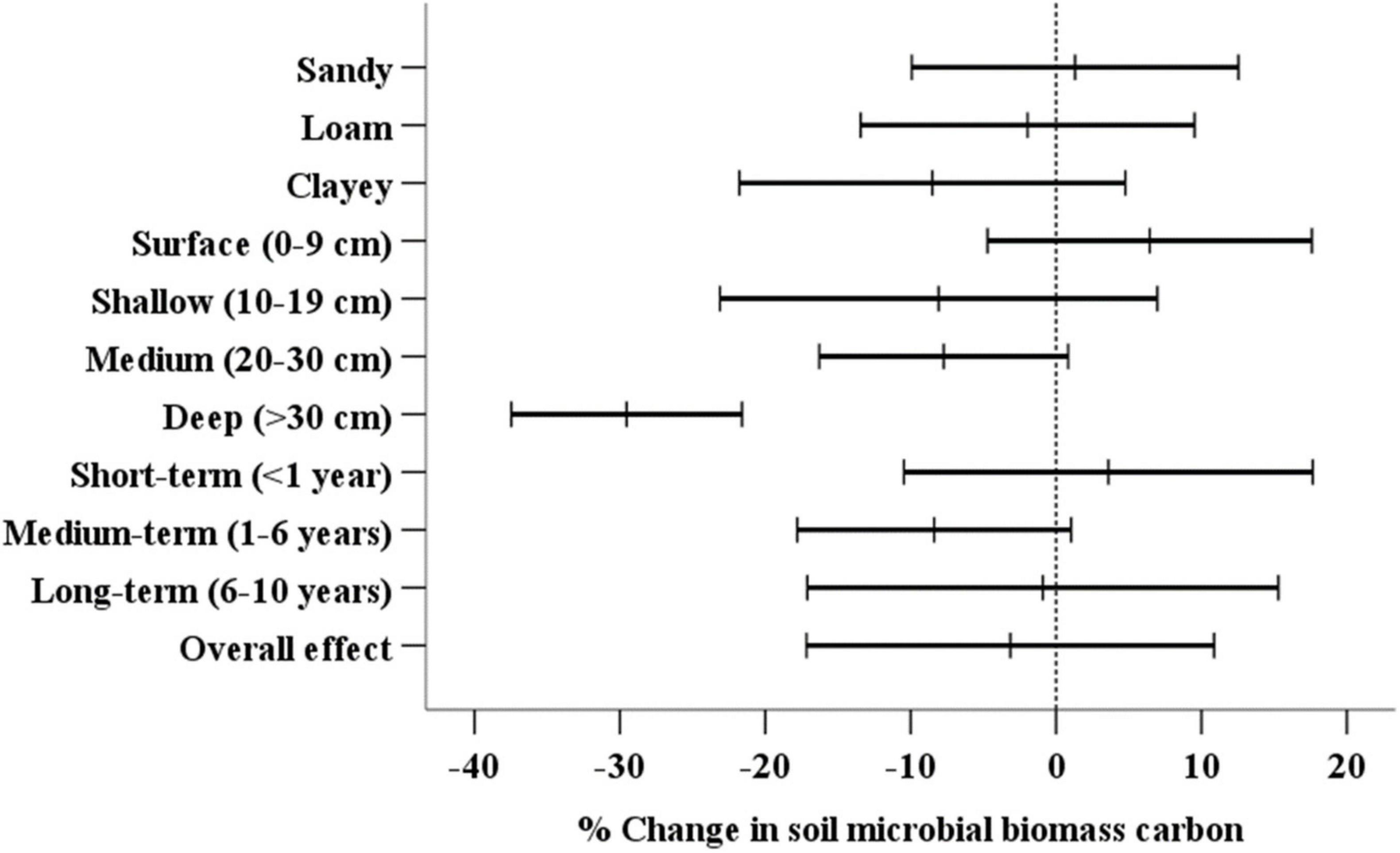
Figure 2. Soil microbial biomass carbon (MBC) changes due to compaction, influenced by texture, depth, and time passed since compaction. Results are presented as mean effect sizes ± 95% confidence intervals. Groups with confidence intervals overlapping the dashed reference line (0% change) indicate no statistically significant change in soil MBC due to compaction at α = 0.05. Groups with confidence intervals overlapping each other are not significantly different at α = 0.05.
The result of this meta-analysis is in contrast with the existing common viewpoint that soil MBC is dramatically decreased by compaction (Torbert and Wood, 1992; Marshall, 2000; Li et al., 2004; Frey et al., 2009), due to negative alternations in the soil total porosity and particle connectivity and therefore in air and water supply (Wronski and Murphy, 1994; Breland and Hansen, 1996; Startsev et al., 1998). However, in agreement with the results of our meta-analysis, a few other studies found no significant impact of compaction on soil MBC at different forest sites with various soil textures (Hassink et al., 1993; Shestak and Busse, 2005; Busse et al., 2006). Since microorganisms inhabit a small portion (about 0.5%) of the surface area in available soil pores, a decline in porosity may not disturb such minor habitable areas (Hassink et al., 1993). For instance, bacteria and fungi prefer to inhabit pores between 0.2 and 30 μm, which still remain habitable even after large reductions in porosity at the expense of large pores by compaction (Hassink et al., 1993; Paz, 2001). Another reason for the lack of change in soil MBC after compaction is the reduced activity of faunal predators such as nematodes and protozoa grazing on soil microorganisms (Paz, 2001; Beylich et al., 2010). In summary, compaction can reduce porosity without disturbing the habitat of microorganisms, causing no significant change in soil MBC.
In subsoils (>30 cm), the concentration of organic carbon and nutrients steeply declines in comparison to upper soil layers (Turner et al., 2014; Stone et al., 2015). In addition, the content of iron and aluminum hydrous oxides and clay minerals is higher in subsoils than in upper soil layers (Mikutta et al., 2009; Turner et al., 2014), intensifying the sorption of organic matter and nutrients to reactive minerals. Thus, subsoil microorganisms have to adapt themselves to such energy and carbon limited conditions. One major source of growth resources for microorganisms is the transport of dissolved organic matter derived through preferential flow paths from the organic layer to top soil layers and, especially to subsoils in forest ecosystems (Kalks et al., 2020; Nazari et al., 2020). This major nutritional source for subsoil microorganisms may substantially decrease in its availability to subsoil microorganisms, due to the destruction of preferential flow paths by compaction. Compaction can further increase the physical protection of already stable subsoil organic matter and lock it away from microbial decomposition (Gartzia-Bengoetxea et al., 2011). Another important reason can be the inability of soil fauna to incorporate topsoil litter and organic matter into the subsoil (bioturbation), further limiting energy and nutrients availability to subsoil microorganisms. Last but not least, decreased subsoil MBC can also be caused by the less presence of ectomycorrhizal fungi due to decline in tree rooting following compaction (Hartmann et al., 2014).
Soil MBC is a sensitive indicator of changing soil conditions, serving as an early warning for many aspects related to soil health (Powlson and Jenkinson, 1976). Although no significant effect could be found in upper soil layers, potentially due to counteracting processes, it needs to be considered that subsoils contain more than 50% of the global terrestrial organic carbon and therefore are very important in global carbon storage (Salomé et al., 2010; Rumpel and Kögel-Knabner, 2011). Thus, special attention has to be paid to MBC reductions of forest subsoils by logging operations, which may in the long-term have even more detrimental implications.
Soil Bulk Density
Influence of Soil Factors and Time
Overall, compaction significantly increased soil bulk density up to 8.9% at α = 0.05 (Figure 3). In detail, compaction caused significantly different bulk density changes across all investigated influencing factors. Bulk density increased by 12.6, 8.6, and 6.4% in clayey, sandy, and loam soils, respectively, due to compaction, which were significantly different from each other. Compaction enhanced bulk density at shallow (10–19 cm) and surface (0–9 cm) depths by 11.9 and 7.4%, respectively, which were significantly different from each other and also from bulk density at deep (>30 cm) and medium (20–30 cm) depths. However, compaction increased bulk density by 5.4 and 4.7% at deep (>30 cm) and medium (20–30 cm) depths, respectively, which were not significantly different from each other. Time passed since compaction affected bulk densities significantly, with highest and lowest bulk density changes of 13.2 and 5.9% at medium-term (1–3 years) and short-term (<1 year) time periods since the compaction event took place.
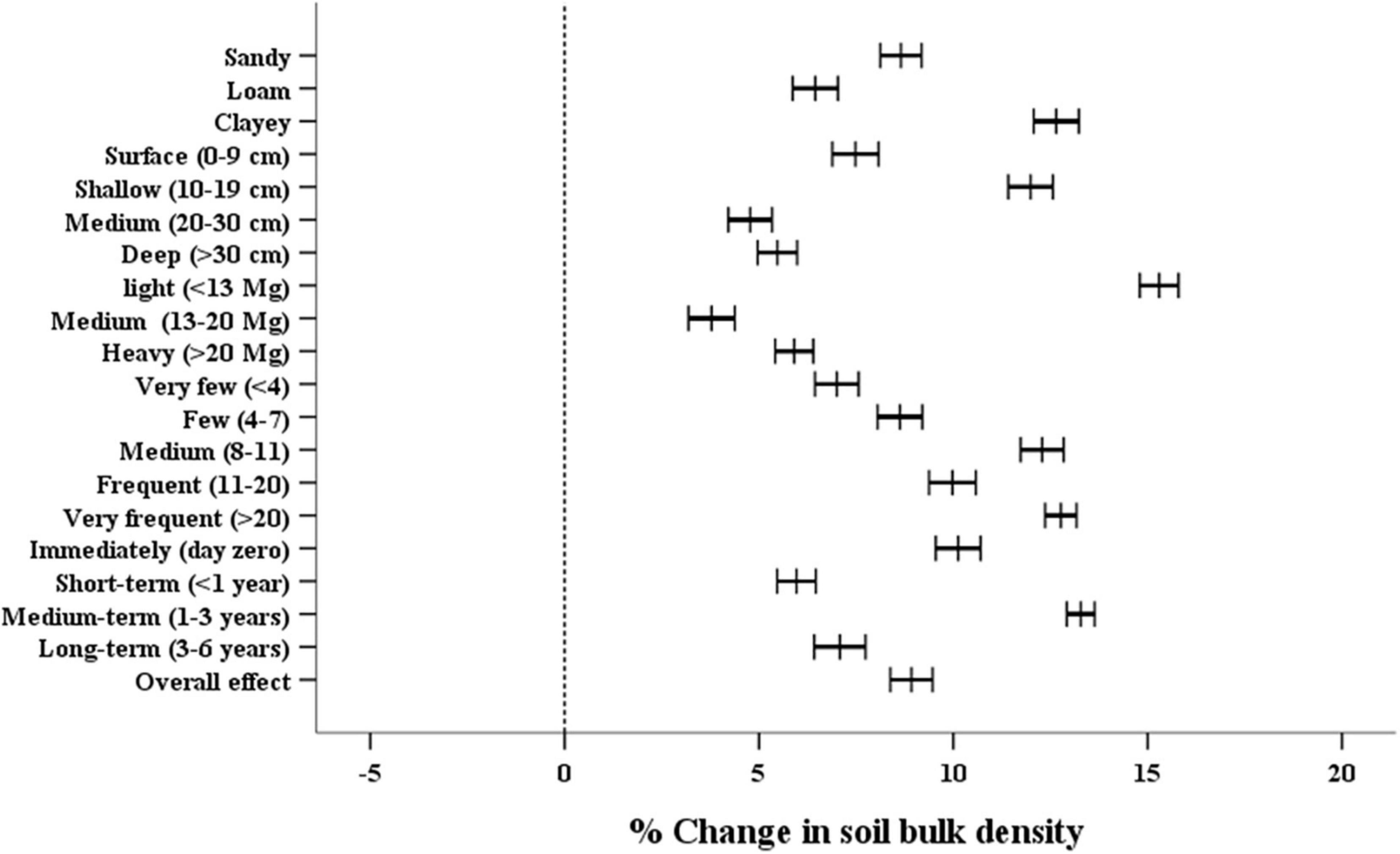
Figure 3. Soil bulk density changes due to compaction, influenced by texture, depth, time passed since compaction, machine weight, and machine passage frequency. Results are presented as mean effect sizes ± 95% confidence intervals. Groups with confidence intervals overlapping the dashed reference line (0% change) indicate no statistically significant change in soil bulk density due to compaction at α = 0.05. Groups with confidence intervals overlapping each other are not significantly different at α = 0.05.
Increased bulk density is the direct outcome of vertical and horizontal pressure on soil (Ares et al., 2005). The degree of enhancement in bulk density by compaction depends on several factors such as soil initial bulk density, organic matter content, water content, and texture (Ballard, 2000; Jamshidi et al., 2008). A higher bulk density enhancement in clayey and sandy than loam soils could be attributed to the loose nature of sandy forest soils (Williamson and Neilsen, 2000; Powers et al., 2004). Clayey soils have a higher water holding capacity and therefore a greater water content than sandy and loam soils. Increase in soil water content leads to a decrease in frictional forces between soil particles, making the soil more susceptible to compaction (McNabb et al., 2001). It can be deduced that logging operations have to be performed cautiously in clayey forest soils, especially in regions with high precipitation (e.g., Europe). An optimum management practice can be the implementation of logging operations out of rainy seasons when the soil moisture is minimum. In case of mechanized coppicing interventions carried out in autumn and winter (e.g., rainy seasons in Europe), we recommend checking the soil moisture content of the coppicing area and selecting days on which the soil moisture is minimum. Another practical way is to conduct coppicing interventions in winter when water in the soil is frozen, strengthening the soil structure and minimizing compaction-associated damage to the soil (De Schrijver et al., 2018).
Bulk density increased more in the upper soil layers compared to the lower soil layers. Soils resistance to compaction depends on soil structure, organic matter content, and the degree of aggregation in order to withstand compaction-induced pressure (Page-Dumroese et al., 2006). Thus, organic matter may enhance the resistance of soil to deformation by compaction. Although there is a higher proportion of organic matter in upper soil layers than in lower ones (Arthur et al., 2013), the lower layers often contain stronger cementing agents than organic matter such as iron and aluminum oxides or carbonates, improving the soil structure and increasing its resistance to compaction (Yee and Harr, 1977).
Bulk density was not a function of the time passed since the compaction event and did not recover to the control level (the zero line) after 3–6 years. It is plausible that the bulk density recovery of forest soils requires a longer period of time. For example, it was shown that the bulk density of forest soils did not significantly recover 5 years (Croke et al., 2001), 10 years (Rab, 2004), and even 30 years (DeArmond et al., 2019) after logging operations. We strongly recommend the performance of long-term studies (i.e., >10 years) in order to shed more light on the recovery time of bulk density in forest soils after logging operations.
Influence of Management Factors
Light machines (<13 Mg) increased soil bulk density by 15.2%, which was significantly higher than the bulk density enhancement of 5.9 and 3.7% caused by heavy (>20 Mg) and medium machines (13–20 Mg), respectively (Figure 3). Bulk densities differed significantly from each other depending on machine passage frequency except medium (8–11) and very frequent (>20) machine passages. Very few (<4), few (4–7), medium (8–11), frequent (11–20), and very frequent (>20) machine passages led to 7, 8.6, 12.2, 9.9, and 12.7% change in bulk density, respectively.
A significantly higher bulk density increase by heavy machines (>20 Mg) than by medium machines (13–20 Mg) is in agreement with the results of several other studies (Jansson and Wästerlund, 1999; Han et al., 2009; Picchio et al., 2012). However, 9.3 and 11.5% greater bulk density caused by light machines (<13 Mg) compared to heavy (>20 Mg), and medium (13–20 Mg) machines, respectively, contradicts these findings. The degree of soil compaction by logging machines depends on several factors such as contact area of machines with soil, type of soil-contacting device (i.e., wheel or track), width of soil-contacting device, logging speed, and machine weight (Bygdén et al., 2003; Cambi et al., 2015). For instance, wheeled machines, due to their lower contact area, usually compact and damage forest soils more severely than tracked ones (Johnson et al., 1991; Jansson and Johansson, 1998; Neruda et al., 2010). It seems that other machine and management factors (e.g., soil-contacting device, machine speed, operator’s expertise) than solely machine weight play a part in increasing bulk density, which need to be evaluated in prospective studies. We also suggest that future studies compare the impacts of different machineries (i.e., skidders, forwarders, tractors, etc.), harvesting systems, and forest types.
Medium (8–11), frequent (11–20), and very frequent (>20) machine passages increased bulk density significantly and more dramatically than very few (<4) and few (4–7) machine passages. This is clearly in contrast with the existing claim that most of the effect on soil bulk density usually happens in the first few machine passages, whereas next machine passages slightly increase bulk density (Wang, 1997; Wallbrink et al., 2002; Han et al., 2006; Cambi et al., 2015). However, very frequent machine passages can keep compacting soil and increasing the bulk density if machine pressure on the soil is greater than the soil strength (Ampoorter et al., 2012). It is possible that initial machine passages reduce the quality of soil physical properties such as aggregate stability, particle connectivity and preferential flow paths, making the soil vulnerable to further compaction.
Soil Total Porosity
Influence of Soil Factors and Time
In total, compaction significantly decreased soil total porosity by −10.1% at α = 0.05 (Figure 4). Total porosity significantly declined in all textures, at all depths, and at all time periods due to compaction, but there was no significant difference between the textures, between the depths, and between the time periods passed since compaction.
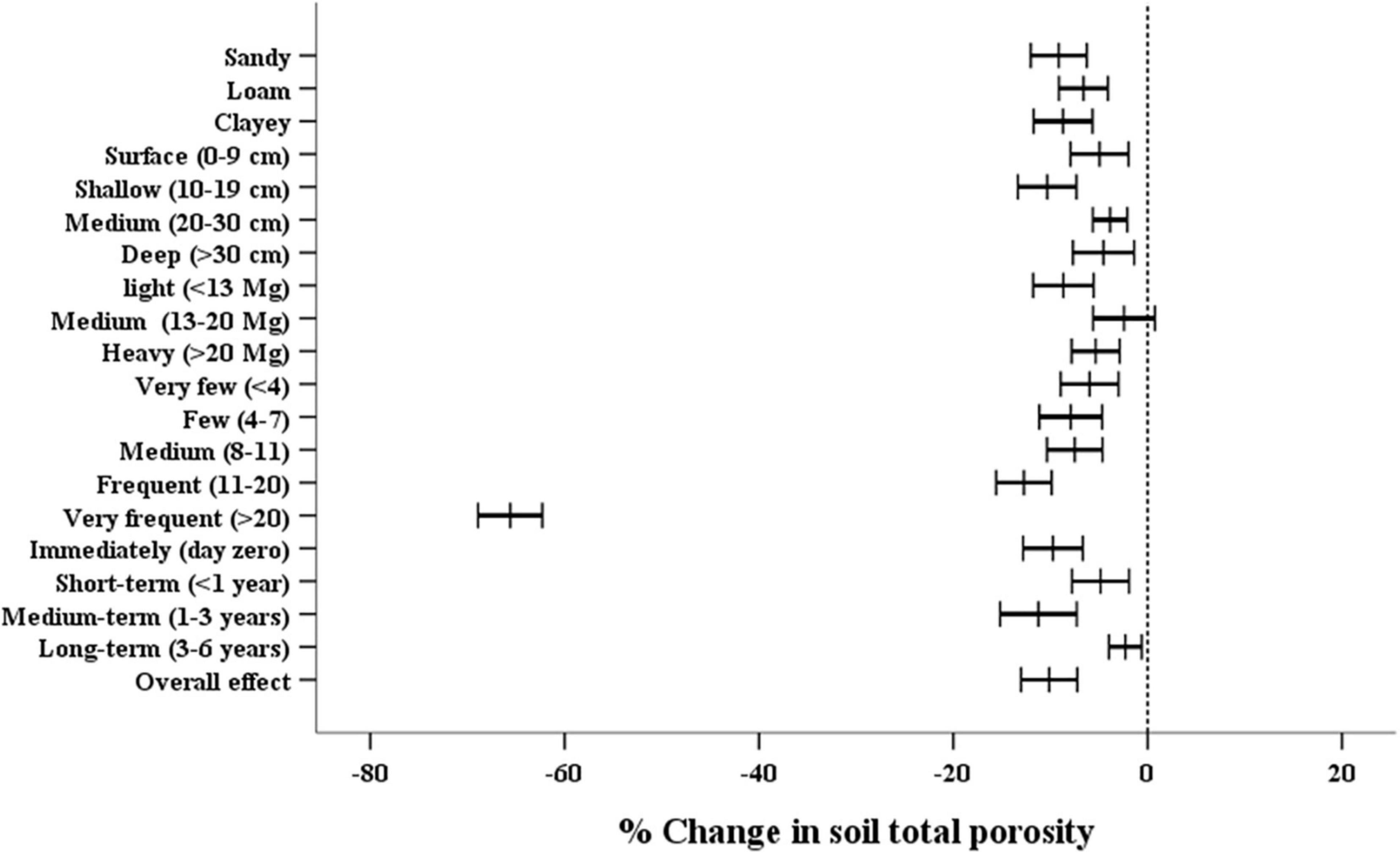
Figure 4. Soil total porosity changes due to compaction, influenced by texture, depth, time passed since compaction, machine weight, and machine passage frequency. Results are presented as mean effect sizes ± 95% confidence intervals. Groups with confidence intervals overlapping the dashed reference line (0% change) indicate no statistically significant change in soil total porosity due to compaction at α = 0.05. Groups with confidence intervals overlapping each other are not significantly different at α = 0.05.
Compaction imposed by logging operations may lead to a soil total porosity reduction between −50 and −60% (Ares et al., 2005; Ampoorter et al., 2007; Demir et al., 2007; Frey et al., 2009; Picchio et al., 2012; Solgi and Najafi, 2014). This reduction is much higher than the reduction derived from our meta-analysis. The difference can be due to the lower number of studies and replicates used for evaluation of total porosity in the already mentioned studies in comparison to the huge dataset used in our meta-analysis. Soil total porosity is a crucial factor for the functioning of forest ecosystem and also for maintaining forest productivity, as it determines the amount of air and water but also dissolved organic matter delivery to the soil. Thus, it is essential for the life of tree roots and soil organisms. Reduction in total porosity can disturb soil water balance, resulting in increased waterlogging, runoff, and erosion (Grace et al., 2006; Ampoorter et al., 2007; Schack-Kirchner et al., 2007).
Compaction similarly reduced total porosity in the different textures and also at the different soil depths. This indicates the independency of total porosity from texture and depth, probably due to the homogenizing impact of high pressure imposed by logging machines. All time periods passed since compaction were indicated to similarly affect total porosity. Moreover, the reduced total porosity, similar to the increased bulk density, did not recover to the control level within the investigated time periods in this meta-analysis. Rab (2004) indicated that soil total porosity reduction in native forests in the Victorian Central Highlands of Australia did not recover after 10 years from compaction imposed by logging operations.
There was a significant negative relationship between percentage changes in bulk density and total porosity in response to compaction (Figure 5). Overall, total porosity is reduced by −10% with 17.8% increase in bulk density due to logging-associated compaction. This implies an increase in the ratio of soil solids to pore space. Organic matter constitutes about 5% of mineral soil solids in forests, which can be physically protected by compaction (Gartzia-Bengoetxea et al., 2011), increasing the ratio of soil solids to pore space. Possible benefits and drawbacks of soil organic matter protection by compaction should be targeted in prospective studies. Considering the importance of total porosity as a crucial pillar of forest ecosystem health and productivity, special attention has to be paid to it by forest managers in order to minimize the disturbance.
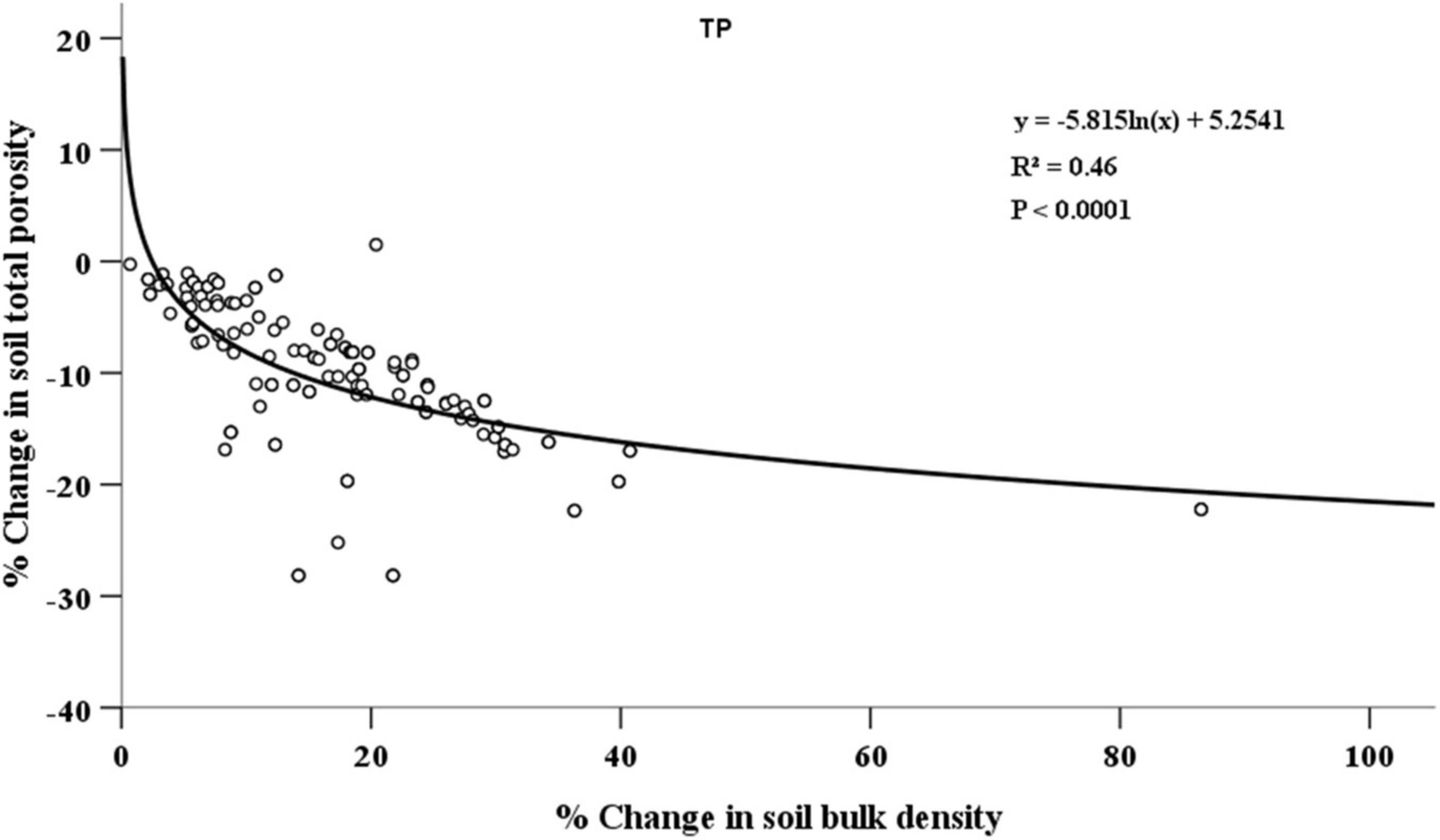
Figure 5. Relationship between percentage change in soil total porosity and percentage change in soil bulk density (n = 272). The relationship was statistically significant at α = 0.05.
Influence of Management Factors
Light machines (<13 Mg) significantly reduced soil total porosity by −9.1%, which was greater than the change caused by medium machines (13–20 Mg) but did not significantly differ from the change caused by heavy machines (>20 Mg) (Figure 4). Heavy machines (>20 Mg) also led to a significantly reduced total porosity (−5.3%), but this change was not significantly different from that caused by medium machines (13–20 Mg). Medium machines (13–20 Mg) did not significantly influence total porosity. Very frequent (>20) machine passages led to the highest soil total porosity reduction by −65%, which was significantly −59.6, −58.1, −57.6, and −51.4% greater than the total porosity change caused by very few (<4), medium (8–11), few (4–7), and frequent (11–20) machine passages, respectively. Furthermore, frequent machine passages (11–20) resulted in −8.1 and −6.6% greater reduction in total porosity in comparison to very few (<4) and medium (8–11) machine passages, respectively.
In addition to a lack of significant impact on the total porosity of forest soils by medium machines (13–20 Mg), logging operations with medium machines (13–20 Mg) also led to the lowest disturbance to the bulk density. This indicates that such machines might possess specific configurations that minimize the damage to soil. It has already been shown that soil disturbance by logging machines depends on several factors such as contact area and contacting device of machines (Bygdén et al., 2003; Cambi et al., 2015). Another possible reason can be the small size and high number of wheels in medium-weight machines, distributing the logging-induced pressure over a larger soil area. However, these assumptions require further experimental evaluation using medium-weight machines with various wheel and track configurations in different soils and forest types. Such experiments can shed light on possible reasons of a non-significant disturbance to total porosity after logging operations by medium-weight machines.
The greatest reduction in total porosity (−65.6%) occurred by very frequently (>20) passing via logging machines. This finding is in contrast with the already existing claims that the majority of total porosity reduction happens after less than 5 machine passages (Jourgholami et al., 2014; Cambi et al., 2015). It seems that initial machine passages reduce the ability of soils to withstand the impact of further compaction. For instance, initial machine passages can impose adequate pressure to break down and disrupt soil aggregates (Cambi et al., 2015), exposing the soil to subsequent machine passages and damage. Since −65.6% is a considerably huge reduction, serious measures have to be taken into account in order to diminish the frequency of machine passages in logging operations. A lack of attention along with the fact that total porosity does not recover in a short time span after compaction can cause serious hazards to global forest ecosystems.
Soil Saturated Hydraulic Conductivity
Influence of Soil Factors and Time
Compaction decreased soil saturated hydraulic conductivity (Ksat) by −40.2% at α = 0.05 across all studies (Figure 6). Ksat significantly responded to all soil textures, depths, and time periods passed since compaction. However, there was no significant difference between the magnitude of Ksat reduction between the soil textures in response to compaction. Compaction resulted in the highest Ksat reduction (−55.3%) at deep depth (>30 cm), which was a significantly greater reduction of Ksat than at shallower soil layers. Ksat reduction was significantly affected by the time passed since the compaction event, with medium-term (1–3 years) and long-term (3–6 years) time periods passed since compaction leading to the highest Ksat reductions of −63.2 and −50.3%, respectively, and immediate (day zero) time passed since compaction resulting in the lowest Ksat reduction of −31.7%.
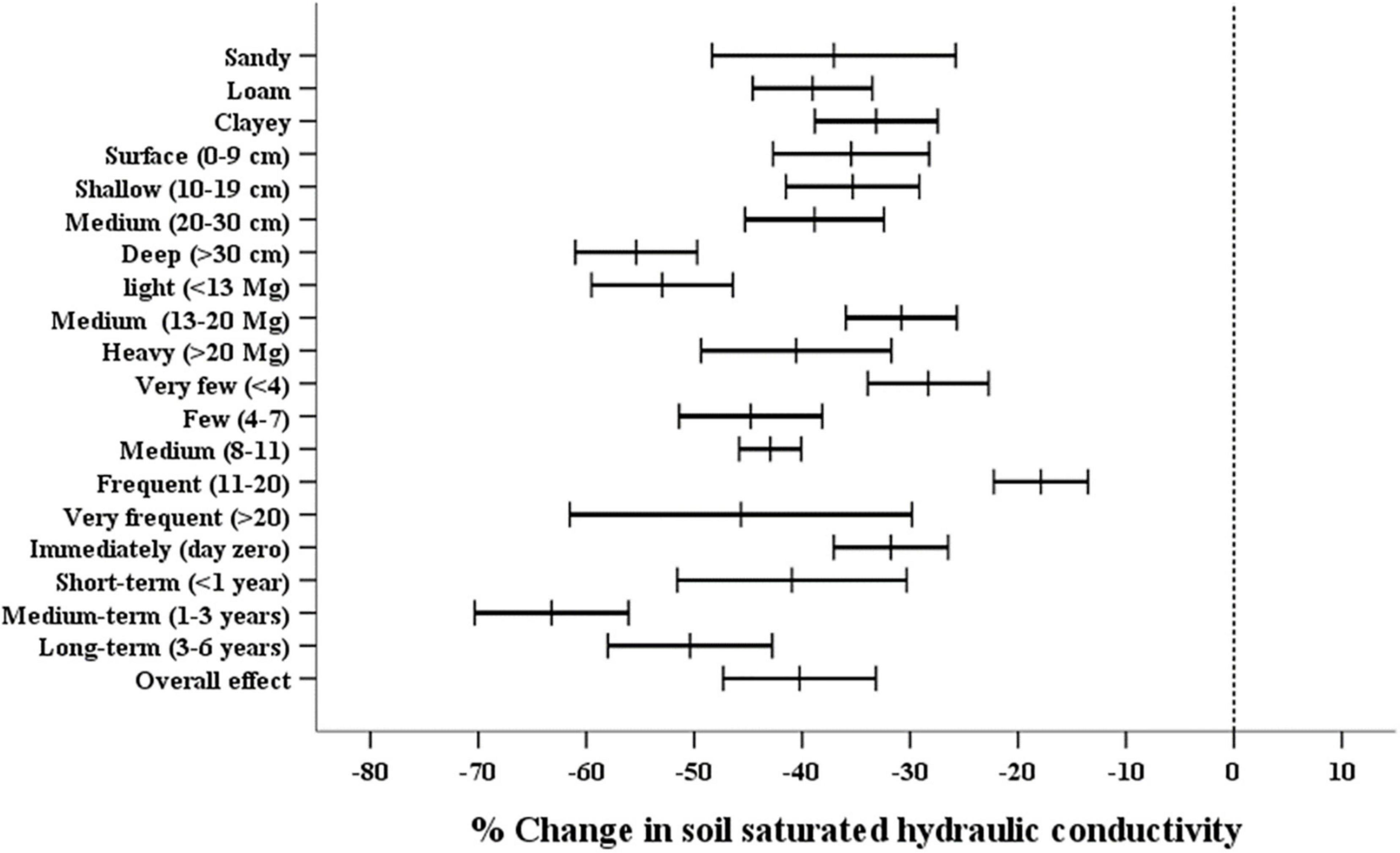
Figure 6. Soil saturated hydraulic conductivity changes due to compaction, influenced by texture, depth, time passed since compaction, machine weight, and machine passage frequency. Results are presented as mean effect sizes ± 95% confidence intervals. Groups with confidence intervals overlapping the dashed reference line (0% change) indicate no statistically significant change in soil saturated hydraulic conductivity due to compaction at α = 0.05. Groups with confidence intervals overlapping each other are not significantly different at α = 0.05.
A −40.2% reduction in soil saturated hydraulic conductivity (Ksat) is much larger than the bulk density enhancement and total porosity reduction due to compaction in our meta-analysis, indicating that Ksat is highly sensitive and susceptible to logging-associated compaction. A major reason for the decrease of Ksat by compaction is reduction in macropores, which play a key role in soil drainage (Ampoorter et al., 2007). The Ksat reduction was not significantly different between the investigated textures, but the reduction was significantly greater at deep soil layers (>30 cm) than at shallower soil depths. This can be due to the higher clay content coupled with the high cementation ability of subsoils, those that become more compact after compaction and therefore conduct less water. Another reason can be the decrease of bio-pores due to reduction in the rooting of trees and other plant species. The Ksat reduction was significantly different between the time periods passed since compaction, with medium-term (1–3 years, −63.2%) and long-term (3–6 years, −50.3%) time periods passed since compaction leading to a significantly higher Ksat reduction than immediate (day zero, −31.7%) time passed since compaction. This indicates that the Ksat response to logging-associated compaction is not a direct effect of compaction but rather an indirect impact, resulting from a chain of altering processes. Lack of a significant relationship between the Ksat and bulk density (Figure 7) and also between the Ksat and total porosity (Figure 8) proves the indirect impact of compaction on Ksat. Two main indirect drivers of the Ksat reduction seem to be less faunal activity and tree rooting caused by compaction (Beylich et al., 2010; Hartmann et al., 2014). Moreover, Ksat did not recover to the control level within the investigated time period in our meta-analysis, empowering the assumption that, like soil bulk density and total porosity, it also requires a longer period of time to recover or maybe even a much longer time than bulk density and total porosity, if the recovery follows the same indirect pathway.
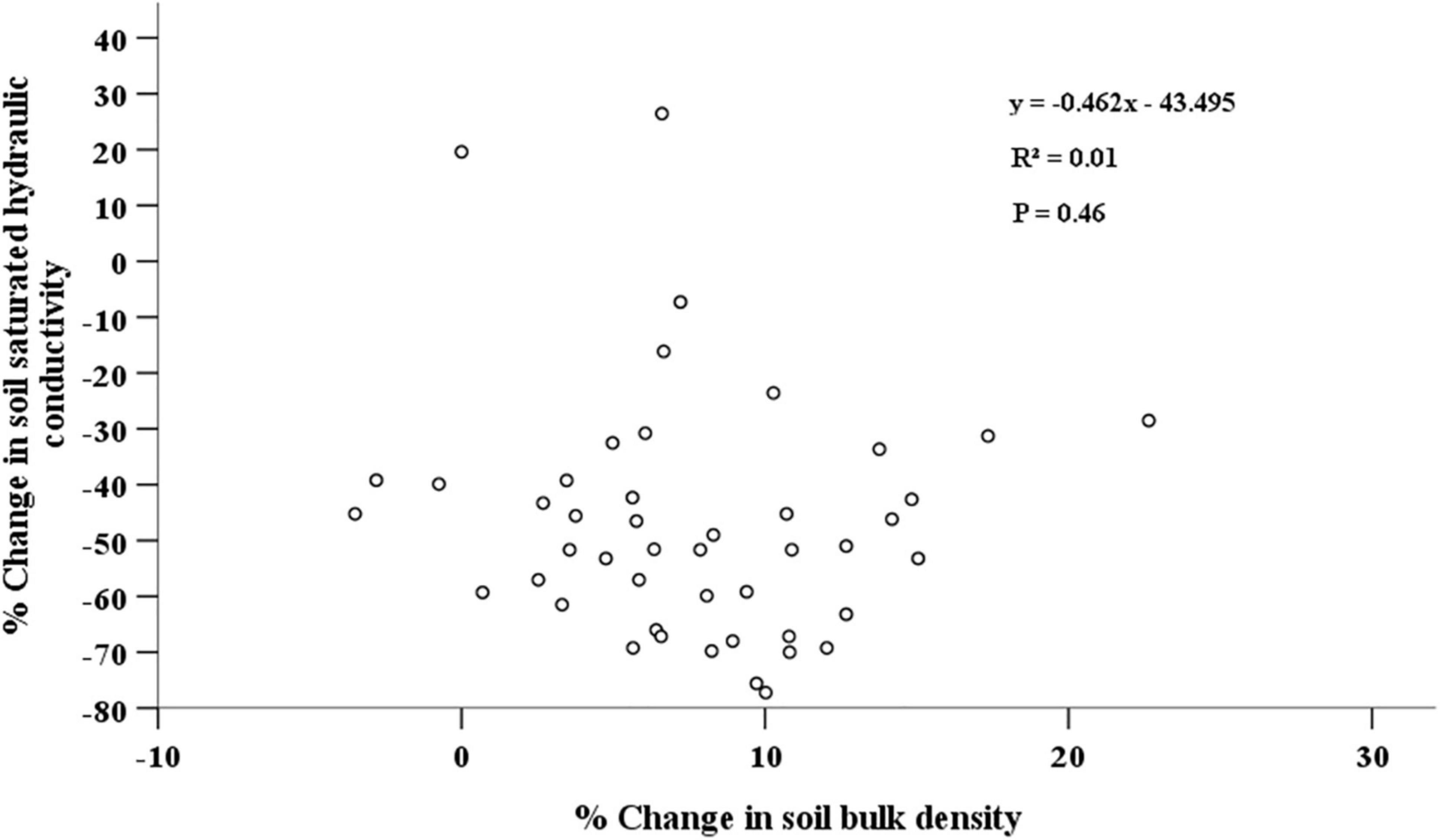
Figure 7. Relationship between percentage change in soil saturated hydraulic conductivity and percentage change in soil bulk density (n = 92). The relationship was not statistically significant at α = 0.05.
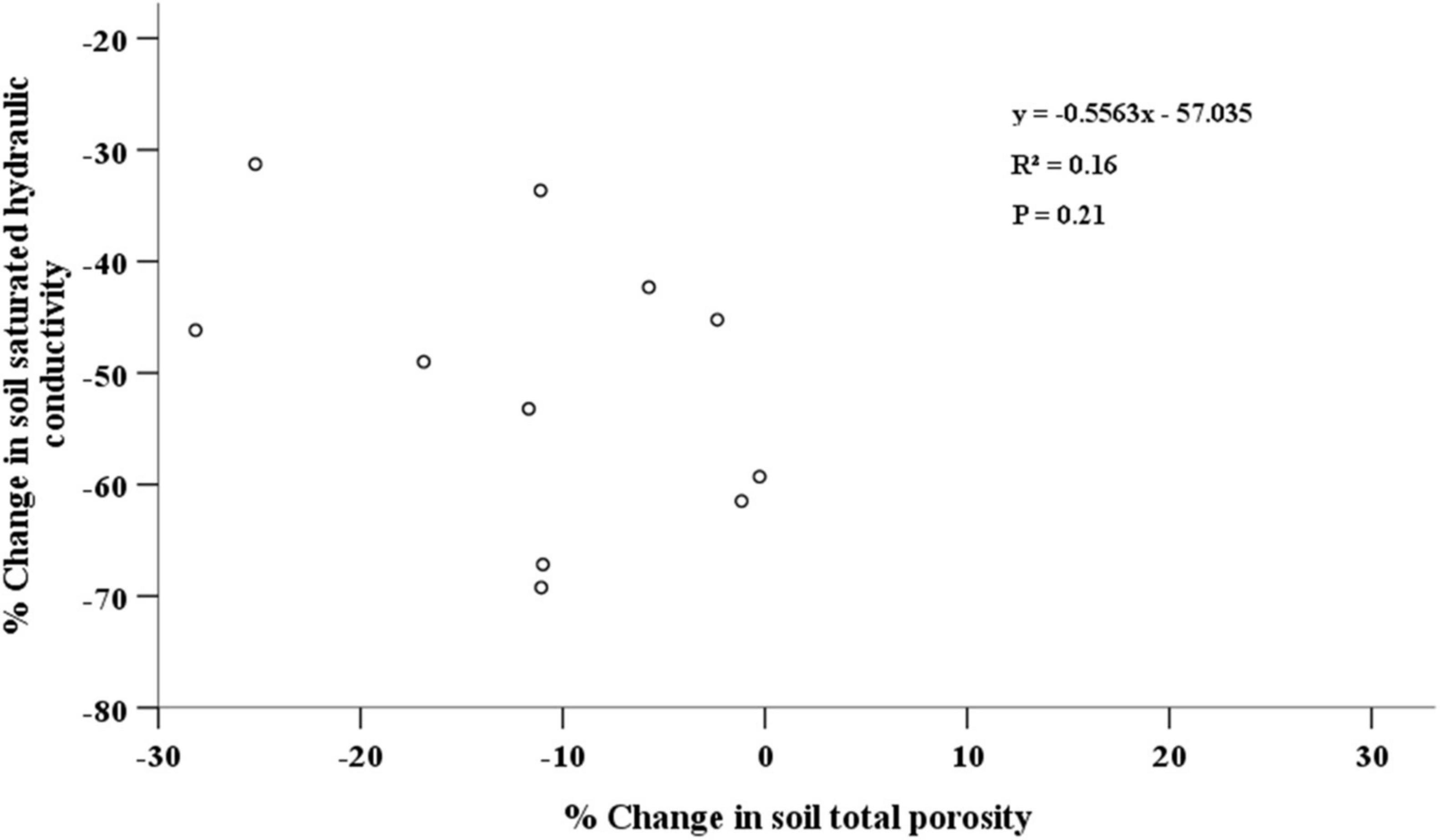
Figure 8. Relationship between percentage change in soil saturated hydraulic conductivity and percentage change in soil total porosity (n = 22). The relationship was not statistically significant at α = 0.05.
Trees require water as a major metabolic agent for growth and development, implying the importance of soil hydraulic conductivity in forests. Soil Ksat determines the proportion of precipitation entering the soil and is also an indicator of soil infiltration capacity (Zimmermann et al., 2006; Tetzlaff et al., 2007; Price et al., 2010), whose reduction may contribute to enhanced waterlogging in flat areas or runoff and erosion on slopes (Jansson and Johansson, 1998; Grace et al., 2006). Therefore, a severe reduction in Ksat can seriously disturb forest ecosystem function and productivity.
Influence of Management Factors
Soil Ksat significantly responded to all management factors (Figure 6). Light machines (<13 Mg) led to −22.1% significantly higher reduction in Ksat than medium machines (13–20 Mg). However, there was no significant difference between light (<13 Mg) and heavy (>20 Mg) machines and between heavy (>20 Mg) and medium (13–20 Mg) machines in the reduction of Ksat. The lowest reduction in Ksat belonged to frequent (11–20) machine passages (−17.8%), while the highest reduction was related to very frequent (> 20) machine passages (−45.6). Very frequent (> 20) machine passages resulted in −27.7% significantly greater reduction in Ksat compared to frequent (11–20) machine passages. Moreover, few (4–7) and medium (8–11) machine passages resulted in significantly −16.4 and −14.6% higher reduction in Ksat than very few (<4) machine passages, respectively.
It seems that other machine characteristics than solely machine weight influences the disturbance degree of soil Ksat by compaction. Such characteristics may include wheel or track width, type, and contact area, which require experimental proof in future studies. Nevertheless, it has been reported that wheeled machines more dramatically damage forest soils than tracked ones (Johnson et al., 1991; Jansson and Johansson, 1998; Neruda et al., 2010).
On the one hand, it seems that very few (<4) machine passages do not impose adequate pressure on soil that can lead to huge declines in the proportion of macropores and bio-pores. On the other hand, very frequent (>20) machine passages probably impose a strong pressure on soil, by which not only macropores and bio-pores are dramatically disturbed and declined but also are soil preferential flow paths. However, these assumptions require experimental proof in prospective studies. In regards to the importance of Ksat for supplying water to tree roots and soil microorganisms, special endeavors are needed by forest managers and operators to reduce the frequency of machine passages beside the selection of appropriate logging machines.
Ecological and Economic Consequences of Forest Soil Compaction
Logging-associated soil compaction can have several negative effects on forest ecosystem and function. Compaction noticeably reduces the volume of macropores and also destroys pore continuity, hampering regular soil-atmosphere gas exchanges (Cambi et al., 2015; De Schrijver et al., 2018). This can cause serious problems for soil organisms and disturbance of biochemical processes. For instance, oxygen cannot properly enter the compacted soil, thus limiting soil life and root growth of plants and trees (Ampoorter et al., 2012). Compaction also decreases the number of capillary pores in soil and thereby water conductivity and infiltration and increases surface runoff and erosion (Lousier, 1990; Malmer and Grip, 1990; Lüscher, 2010). Moreover, compaction can diminish the biodiversity of forest soils fauna (i.e., mites, earthworms), due to increased soil bulk density and limited oxygen diffusion (Siepel, 2015; Cambi et al., 2017b). Mycorrhizal fungi, as important symbionts of tree roots, are also negatively affected by decreased air supply due to compaction, limiting nutrient uptake by tree roots (Smeltzer et al., 1986; De Schrijver et al., 2018).
Soil compaction harms the above-mentioned complex components of forest soil ecosystem and can thereby negatively affect forest productivity (Kozlowski, 1999). For example, trees close their leaf stomata in case of compaction-derived water shortage, reducing photosynthesis and tree growth. Specifically, compaction limits the growth of young seedlings, because it is difficult for them to penetrate compacted soils for taking up water and nutrients (Bassett et al., 2005; Cambi et al., 2017a). The negative impact of compaction on seedlings are also visible in later stages, i.e., after 10 and 32 years since the logging event (Wert and Thomas, 1981; Heninger et al., 2002).
Although there is little research on the economic impacts of logging-associated soil compaction, compaction can potentially influence the quantity and quality of harvestable timber. For instance, Potvliet (2016) modeled the economic impact of compaction for a Scots pine (Pinus sylvestris) forest in the Netherlands and found an increased revenue per hectare by 14.3% caused by the scenario of avoiding soil compaction. Moreover, Borchert et al. (2008) reported that soil compaction caused root damage and thereby timber rotting, decreasing timber prices by −20% in 2007 in Germany. We suggest conducting future interdisciplinary studies to investigate economic consequences of forest soil compaction in order to expand our understanding of the context.
Conclusion
This meta-analysis evaluated the influence of logging-associated compaction on the physical properties and MBC in forest soils affected by two management factors (machine weight and passage frequency), two soil factors (texture and depth), and the time passed since the compaction event. Despite an overall insignificant effect, MBC is significantly decreased in subsoils (>30 cm) due to compaction. Since forest subsoils play an important part in global terrestrial carbon cycle and generally show slow recovery kinetics, specific measures have to be taken by forest managers and operators for minimizing the impact.
Compaction leads to an overall increase of 8.9% in soil bulk density, which is significantly greater in clayey soils than in sandy and loam soils. Accompanied with the fact that clayey soils hold more water and are more susceptible to compaction, specific attention should be paid when logging operations are implemented on clayey soils in regions with high precipitation (e.g., Europe). Compaction results in −10.1 and −40.2% reduction in soil total porosity and saturated hydraulic conductivity, respectively. Very frequent (>20) machine passages cause the greatest change to soil bulk density, total porosity, and saturated hydraulic conductivity, indicating the need for a serious action to minimize the frequency of machine passages in logging operations. Since these physical variables play important roles in water and air supply as well as nutrient cycling in forest soils, the consideration by forest managers and operators can prevent long-term disturbance to forest ecosystems and productivity.
For sustainable forest management, it is essential to minimize total skid trail coverage. However, to minimize the logging impact, we recommend the selection of appropriate logging machines that can diminish the disturbance to soil and forest ecosystem. Otherwise, when the current logging machines are used, decreasing the frequency of machine passages and logging out of rainy seasons especially in clayey soils are recommended.
Data Availability Statement
The original contributions presented in the study are included in the article/supplementary material, further inquiries can be directed to the corresponding author.
Author Contributions
MN and ME reviewed the manuscript and collected the data. MN, ME, and MG performed the meta-analysis. MZ, MD, NB, and KZ commented on the manuscript and shared their knowledge. KZ supervised the meta-analysis process. MN prepared the manuscript draft. All authors reviewed the manuscript before submission.
Conflict of Interest
The authors declare that the research was conducted in the absence of any commercial or financial relationships that could be construed as a potential conflict of interest.
Publisher’s Note
All claims expressed in this article are solely those of the authors and do not necessarily represent those of their affiliated organizations, or those of the publisher, the editors and the reviewers. Any product that may be evaluated in this article, or claim that may be made by its manufacturer, is not guaranteed or endorsed by the publisher.
Acknowledgments
The German Federal Environmental Foundation (Deutsche Bundesstiftung Umwelt, DBU) was acknowledged for funding MN. We also thank the National Natural Science Foundation of China (NSFC grant number 42050410320 to KZ) for their support.
Footnote
References
Abdi, E., Moghadamirad, M., Hayati, E., and Jaeger, D. (2017). Soil hydrophysical degradation associated with forest operations. For. Sci. Technol. 13, 152–157. doi: 10.1080/21580103.2017.1387611
Acharya, R. P., Maraseni, T., and Cockfield, G. (2019). Global trend of forest ecosystem services valuation – An analysis of publications. Ecosyst. Serv. 39:100979. doi: 10.1016/j.ecoser.2019.100979
Agherkakli, B., Najafi, A., and Sadeghi, S. H. (2010). Ground based operation effects on soil disturbance by steel tracked skidder in a steep slope of forest. J. For. Sci. 56, 278–284. doi: 10.17221/93/2009-jfs
Allbrook, R. F. (1986). Effect of skid trail compaction on a volcanic soil in central oregon. Soil Sci. Soc. Am. J. 50, 1344–1346. doi: 10.2136/sssaj1986.03615995005000050052x
Ampoorter, E., De Schrijver, A., Van Nevel, L., Hermy, M., and Verheyen, K. (2012). Impact of mechanized harvesting on compaction of sandy and clayey forest soilsesults of a meta-analysis. Ann. For. Sci. 69, 533–542. doi: 10.1007/s13595-012-0199-y
Ampoorter, E., Goris, R., Cornelis, W. M., and Verheyen, K. (2007). Impact of mechanized logging on compaction status of sandy forest soils. For. Ecol. Manag. 241, 162–174. doi: 10.1016/j.foreco.2007.01.019
Ares, A., Terry, T. A., Miller, R. E., Anderson, H. W., and Flaming, B. L. (2005). Ground-based forest harvesting effects on soil physical properties and douglas-fir growth. Soil Sci. Soc. Am. J. 69, 1822–1832. doi: 10.2136/sssaj2004.0331
Arthur, E., Schjønning, P., Moldrup, P., Tuller, M., and de Jonge, L. W. (2013). Density and permeability of a loess soil: long-term organic matter effect and the response to compressive stress. Geoderma 194, 236–245. doi: 10.1016/j.geoderma.2012.09.001
Aust, W. M., Tippett, M. D., Burger, J. A., and McKee, W. H. (1995). Compaction and rutting during harvesting affect better drained soils more than poorly drained soils on wet pine flats. South. J. Appl. For. 19, 72–77. doi: 10.1093/sjaf/19.2.72
Bagheri, I., Kalhori, S. B., Akef, M., and Khormali, F. (2012). Effect of compaction on physical and micromorphological properties of forest soils. Am. J. Plant Sci. 3, 159–163. doi: 10.4236/ajps.2012.31018
Ballard, T. M. (2000). Impacts of forest management on northern forest soils. For. Ecol. Manag. 133, 37–42. doi: 10.1016/S0378-1127(99)00296-0
Bassett, I. E., Simcock, R. C., and Mitchell, N. D. (2005). Consequences of soil compaction for seedling establishment: implications for natural regeneration and restoration. Austr. Ecol. 30, 827–833. doi: 10.1111/j.1442-9993.2005.01525.x
Beylich, A., Oberholzer, H. R., Schrader, S., Höper, H., and Wilke, B. M. (2010). Evaluation of soil compaction effects on soil biota and soil biological processes in soils. Soil Tillage Res. 109, 133–143. doi: 10.1016/j.still.2010.05.010
Bigelow, S. W., Jansen, N. A., Jack, S. B., and Staudhammer, C. L. (2018). Influence of selection method on skidder-trail soil compaction in longleaf pine forest. For. Sci. 64, 641–652. doi: 10.1093/forsci/fxy023
Bonan, G. B. (2008). Forests and climate change: forcings, feedbacks, and the climate benefits of forests. Science 320, 1444–1449. doi: 10.1126/science.1155121
Borchert, H., Blaschke, M., and Metan, M. (2008). Wurzelverletzungen under Raupe und Rad. Trotzschwerer Wurzelverletcungen bei Raupenfahrwerken schneinden Raupenfahrzeuge in Vergleich zu Radmaschinen nicht schlechter ab. LWF Aktuell. 16–18.
Brais, S. (2001). Persistence of Soil compaction and effects on seedling growth in northwestern quebec. Soil Sci. Soc. Am. J. 65, 1263–1271. doi: 10.2136/sssaj2001.6541263x
Breland, T. A., and Hansen, S. (1996). Nitrogen mineralization and microbial biomass as affected by soil compaction. Soil Biol. Biochem. 28, 655–663. doi: 10.1016/0038-0717(95)00154-9
Brevik, E. C., and Fenton, T. E. (2012). Long-term effects of compaction on soil properties along the mormon trail. South-Central Iowa, USA. Soil Horizons 53, 37–42. doi: 10.2136/sh12-03-0011
Busse, M. D., Beattie, S. E., Powers, R. F., Sanchez, F. G., and Tiarks, A. E. (2006). Microbial community responses in forest mineral soil to compaction, organic matter removal, and vegetation control. Can. J. For. Res. 36, 577–588. doi: 10.1139/x05-294
Bygdén, G., Eliasson, L., and Wästerlund, I. (2003). Rut depth, soil compaction and rolling resistance when using bogie tracks. J. Terramech. 40, 179–190. doi: 10.1016/j.jterra.2003.12.001
Cambi, M., Certini, G., Fabiano, F., Foderi, C., Laschi, A., and Picchio, R. (2016a). Impact of wheeled and tracked tractors on soil physical properties in a mixed conifer stand. IForest 9, 89–94. doi: 10.3832/ifor1382-008
Cambi, M., Grigolato, S., Neri, F., Picchio, R., and Marchi, E. (2016b). Effects of forwarder operation on soil physical characteristics: a case study in the Italian alps. Croat. J. For. Eng. 37, 233–239.
Cambi, M., Certini, G., Neri, F., and Marchi, E. (2015). The impact of heavy traffic on forest soils: a review. For. Ecol. Manag. 338, 124–138. doi: 10.1016/j.foreco.2014.11.022
Cambi, M., Hoshika, Y., Mariotti, B., Paoletti, E., Picchio, R., Venanzi, R., et al. (2017a). Compaction by a forest machine affects soil quality and Quercus robur L. seedling performance in an experimental field. For. Ecol. Manag. 384, 406–414. doi: 10.1016/j.foreco.2016.10.045
Cambi, M., Paffetti, D., Vettori, C., Picchio, R., Venanzi, R., and Marchi, E. (2017b). Assessment of the impact of forest harvesting operations on the physical parameters and microbiological components on a Mediterranean sandy soil in an Italian stone pine stand. Eur. J. For. Res. 136, 205–215. doi: 10.1007/s10342-016-1020-5
Certini, G., Corti, G., Agnelli, A., and Sanesi, G. (2003). Carbon dioxide efflux and concentrations in two soils under temperate forests. Biol. Fertil. Soils 37, 39–46. doi: 10.1007/s00374-002-0560-7
Chen, C. R., Xu, Z. H., Blumfield, T. J., and Hughes, J. M. (2003). Soil microbial biomass during the early establishment of hoop pine plantation: seasonal variation and impacts of site preparation. For. Ecol. Manag. 186, 213–225. doi: 10.1016/S0378-1127(03)00275-5
Corti, G., Ugolini, F. C., Agnelli, A., Certini, G., Cuniglio, R., Berna, F., et al. (2002). The soil skeleton, a forgotten pool of carbon and nitrogen in soil. Eur. J. Soil Sci. 53, 283–298. doi: 10.1046/j.1365-2389.2002.00442.x
Croke, J., Hairsine, P., and Fogarty, P. (2001). Soil recovery from track construction and harvesting changes in surface infiltration, erosion and delivery rates with time. For. Ecol. Manag. 143, 3–12. doi: 10.1016/S0378-1127(00)00500-4
De Schrijver, A., de, E., Ampoorter, R., van der Burg, A., Demey, S., Schelfhout, A., et al. (2018). “Groeiplaatsverbetering,” in Praktijkboek Bosbeheer, eds P. Jansen, M. Boosten, M. Cassaert, E. Thomassen, and M. Winnock (Wageningen: Stichting Probos & Inverde), 64–97. (Red.).
DeArmond, D., Emmert, F., Lima, A. J. N., and Higuchi, N. (2019). Impacts of soil compaction persist 30 years after logging operations in the Amazon Basin. Soil a Tillage Res. 189, 207–216. doi: 10.1016/j.still.2019.01.010
Demir, M., Makineci, E., and Yilmaz, E. (2007). Investigation of timber harvesting impacts on herbaceous cover, forest floor and surface soil properties on skid road in an oak (Quercus petrea L.) stand. Build Environ. 42, 1194–1199. doi: 10.1016/j.buildenv.2005.11.008
Dick, R. P., Myrold, D. D., and Kerle, E. A. (1988). Microbial biomass and soil enzyme activities in compacted and rehabilitated skid trail soils. Soil Sci. Soc. Am. J. 52, 512–516. doi: 10.2136/sssaj1988.03615995005200020038x
Dominati, E., Patterson, M., and Mackay, A. (2010). A framework for classifying and quantifying the natural capital and ecosystem services of soils. Ecol. Econ. 69, 1858–1868. doi: 10.1016/j.ecolecon.2010.05.002
Egger, M., Smith, G. D., Schneider, M., and Minder, C. (1997). Bias in meta-analysis detected by a simple, graphical test. Br. Med. J. 315, 629–634. doi: 10.1136/bmj.315.7109.629
Eliasson, L. (2005). Effects of forwarder tyre pressure on rut formation and soil compaction. Silva Fennica Monographs 39, 549–557. doi: 10.14214/sf.366
Eroğlu, H., Sariyildiz, T., Küçük, M., and Sancal, E. (2016). The effects of different logging techniques on the physical and chemical characteristics of forest soil. Balt. For. 22, 139–147.
Etehadi Abari, M., Majnounian, B., Malekian, A., and Jourgholami, M. (2017). Effects of forest harvesting on runoff and sediment characteristics in the Hyrcanian forests, northern Iran. Eur. J. For. Res. 136, 375–386. doi: 10.1007/s10342-017-1038-3
Frey, B., Kremer, J., Rüdt, A., Sciacca, S., Matthies, D., and Lüscher, P. (2009). Compaction of forest soils with heavy logging machinery affects soil bacterial community structure. Eur. J. Soil Biol. 45, 312–320. doi: 10.1016/j.ejsobi.2009.05.006
Froehlich, H. A., Miles, D. W. R., and Robbins, R. W. (1985). Soil bulk density recovery on compacted skid trails in central idaho. Soil Sci. Soc. Am. J. 49, 1015–1017. doi: 10.2136/sssaj1985.03615995004900040045x
Gartzia-Bengoetxea, N., Arbestain, M. C., Mandiola, E., and Martínez de Arano, I. (2011). Physical protection of soil organic matter following mechanized forest operations in Pinus radiata D.Don plantations. Soil Biol. Biochem. 43, 141–149. doi: 10.1016/j.soilbio.2010.09.025
Gayoso, J., and Iroume, A. (1991). Compaction and soil disturbances from logging in southern Chile. Ann. Sci. For. 48, 63–71. doi: 10.1051/forest:19910105
Gent, J. A., Ballard, R., and Hassan, A. E. (1983). The impact of harvesting and site preparation on the physical properties of lower coastal plain forest soils. Soil Sci. Soc. Am. J. 47, 595–598. doi: 10.2136/sssaj1983.03615995004700030041x
Gomez, A., Powers, R. F., Singer, M. J., and Horwath, W. R. (2002). Soil compaction effects on growth of young ponderosa pine following litter removal in california’s sierra nevada. Soil Sci. Soc. Am. J. 66, 1334–1343. doi: 10.2136/sssaj2002.1334
Grace, J., Skaggs, R. W., and Cassel, D. K. (2006). Soil physical changes associated with forest harvesting operations on an organic soil. Soil Sci. Soc. Am. J. 70, 503–509. doi: 10.2136/sssaj2005.0154
Groot, D. R., Brander, L., van der Ploeg, S., Costanza, R., Bernard, F., Braat, L., et al. (2012). Global estimates of the value of ecosystems and their services in monetary units. Ecosyst. Serv. 1, 50–61. doi: 10.1016/j.ecoser.2012.07.005
Han, H.-S., Page-Dumroese, D., Han, S.-K., and Tirocke, J. (2006). Effects of slash, machine passes, and soil moisture on penetration resistance in a cut-to-length harvesting. Int. J. For. Eng. 17, 11–17. doi: 10.1080/14942119.2006.10702532
Han, S. K., Han, H. S., Page-Dumroese, D. S., and Johnson, L. R. (2009). Soil compaction associated with cut-to-length and whole-tree harvesting of a coniferous forest. Can. J. For. Res. 39, 976–989. doi: 10.1139/X09-027
Hansson, L. J., Koestel, J., Ring, E., and Gärdenäs, A. I. (2018). Impacts of off-road traffic on soil physical properties of forest clear-cuts: X-ray and laboratory analysis. Scand. J. For. Res. 33, 166–177. doi: 10.1080/02827581.2017.1339121
Hartmann, M., Niklaus, P. A., Zimmermann, S., Schmutz, S., Kremer, J., Abarenkov, K., et al. (2014). Resistance and resilience of the forest soil microbiome to logging-associated compaction. ISME J. 8, 226–244. doi: 10.1038/ismej.2013.141
Hassink, J., Bouwman, L. A., Zwart, K. B., Bloem, J., and Brussaard, L. (1993). Relationships between soil texture, physical protection of organic matter, soil biota, and c and n mineralization in grassland soils. Geoderma 57, 105–128. doi: 10.1016/0016-7061(93)90150-J
Hedges, L. V., Gurevitch, J., and Curtis, P. S. (1999). The meta-analysis of response ratios in experimental ecology. Ecology 80, 1150–1156.
Heninger, R., Scott, W., Dobkowski, A., Miller, R., Anderson, H., and Duke, S. (2002). Soil disturbance and 10-year growth response of coast Douglas-fir on nontilled and tilled skid trails in the Oregon Cascades. Can. J. For. Res. 32, 233–246. doi: 10.1139/x01-195
Holden, S. R., and Treseder, K. K. (2013). A meta-analysis of soil microbial biomass responses to forest disturbances. Front. Microbiol. 4:163. doi: 10.3389/fmicb.2013.00163
Horn, R., Vossbrink, J., Peth, S., and Becker, S. (2007). Impact of modern forest vehicles on soil physical properties. For. Ecol. Manag. 248, 56–63. doi: 10.1016/j.foreco.2007.02.037
Jamshidi, R., Jaeger, D., Raafatnia, N., and Tabari, M. (2008). Influence of two ground-based skidding systems on soil compaction under different slope and gradient conditions. Int. J. For. Eng. 19, 9–16. doi: 10.1080/14942119.2008.10702554
Jansson, K. J., and Johansson, J. (1998). Soil changes after traffic with a tracked and a wheeled forest machine: a case study on a silt loam in Sweden. Forestry 71, 57–66. doi: 10.1093/forestry/71.1.57
Jansson, K. J., and Wästerlund, I. (1999). Effect of traffic by lightweight forest machinery on the growth of young picea abies trees. Scand. J. For. Res. 14, 581–588. doi: 10.1080/02827589908540823
Johnson, C. E., Johnson, A. H., Huntington, T. G., and Siccama, T. G. (1991). Whole-tree clear-cutting effects on soil horizons and organic-matter pools. Soil Sci. Soc. Am. J. 55, 497–502. doi: 10.2136/sssaj1991.03615995005500020034x
Jordan, D., Hubbard, V. C., Ponder, F., and Berry, E. C. (2000). The influence of soil compaction and the removal of organic matter on two native earthworms and soil properties in an oak-hickory forest. Biol. Fertil. Soils 31, 323–328. doi: 10.1007/s003740050663
Jordan, D., Ponder, F., and Hubbard, V. C. (2003). Effects of soil compaction, forest leaf litter and nitrogen fertilizer on two oak species and microbial activity. Appl. Soil Ecol. 23, 33–41. doi: 10.1016/S0929-1393(03)00003-9
Jourgholami, M., Feghhi, J., Tavankar, F., Latterini, F., Venanzi, R., and Picchio, R. (2021). Short-term effects in canopy gap area on the recovery of compacted soil caused by forest harvesting in old-growth oriental beech (Fagus orientalis lipsky) stands. iForest 14, 370–377. doi: 10.3832/ifor3432-014
Jourgholami, M., Soltanpour, S., Abari, M. E., and Zenner, E. K. (2014). Influence of slope on physical soil disturbance due to farm tractor forwarding in a Hyrcanian forest of Northern Iran. iForest 7, 342–348. doi: 10.3832/ifor1141-007
Kalks, F., Liebmann, P., Wordell-Dietrich, P., Guggenberger, G., Kalbitz, K., Mikutta, R., et al. (2020). Fate and stability of dissolved organic carbon in topsoils and subsoils under beech forests. Biogeochemistry 148, 111–128. doi: 10.1007/s10533-020-00649-8
Kozlowski, T. T. (1999). Soil compaction and growth of woody plants. Scand. J. For. Res. 14, 596–619. doi: 10.1080/02827589950154087
Li, Q., Lee Allen, H., and Wollum, A. G. (2004). Microbial biomass and bacterial functional diversity in forest soils: effects of organic matter removal, compaction, and vegetation control. Soil Biol. Biochem. 36, 571–579. doi: 10.1016/j.soilbio.2003.12.001
Lidskog, R., Sundqvist, G., Kall, A. S., Sandin, P., and Larsson, S. (2013). Intensive forestry in Sweden: stakeholders’ evaluation of benefits and risk. J. Int. Environ. Sci. 10, 145–160. doi: 10.1080/1943815X.2013.841261
Lousier, J. D. (1990). Impacts of Forest Harvesting and Regeneration on Forest Sites. Victoria B.C: BC Ministry of Forests, 70–92.
Lüscher, P. (2010). Bodenveränderungen und Typisierung von Fahrspuren nach physikalischer Belastung. Schweiz Z. Forstwes. 161, 504–509.
Magagnotti, N., Spinelli, R., Güldner, O., and Erler, J. (2012). Site impact after motor-manual and mechanised thinning in Mediterranean pine plantations. Biosyst. Eng. 113, 140–147. doi: 10.1016/j.biosystemseng.2012.07.001
Makineci, E., Demir, M., Comez, A., and Yilmaz, E. (2007). Chemical characteristics of the surface soil, herbaceous cover and organic layer of a compacted skid road in a fir (Abies bornmulleriana Mattf.) forest. Trans. Res. Part D 12, 453–459. doi: 10.1016/j.trd.2007.06.001
Malmer, A., and Grip, H. (1990). Soil disturbance and loss of infiltrability caused by mechanized and manual extraction of tropical rainforest in Sabah. Malaysia. For. Ecol. Manag. 38, 1–12. doi: 10.1016/0378-1127(90)90081-L
Mariani, L., Chang, S. X., and Kabzems, R. (2006). Effects of tree harvesting, forest floor removal, and compaction on soil microbial biomass, microbial respiration, and N availability in a boreal aspen forest in British Columbia. Soil Biol. Biochem. 38, 1734–1744. doi: 10.1016/j.soilbio.2005.11.029
Marshall, V. G. (2000). Impacts of forest harvesting on biological processes in northern forest soils. For. Ecol. Manag. 133, 43–60. doi: 10.1016/S0378-1127(99)00297-2
Mayer, M., Prescott, C. E., Abaker, W. E. A., Augusto, L., Cécillon, L., Ferreira, G. W. D., et al. (2020). Influence of forest management activities on soil organic carbon stocks: a knowledge synthesis. For. Ecol. Manag. 466:118127. doi: 10.1016/j.foreco.2020.118127
McNabb, D. H., Startsev, A. D., and Nguyen, H. (2001). Soil wetness and traffic level effects on bulk density and air-filled porosity of compacted boreal forest soils. Soil Sci. Soc. Am. J. 65, 1238–1247. doi: 10.2136/sssaj2001.6541238x
Mikutta, R., Schaumann, G. E., Gildemeister, D., Bonneville, S., Kramer, M. G., Chorover, J., et al. (2009). Biogeochemistry of mineral-organic associations across a long-term mineralogical soil gradient (0.3-4100 kyr). Hawaiian Islands. Geochim. Cosmochim. Acta 73, 2034–2060. doi: 10.1016/j.gca.2008.12.028
Naghdi, R., and Mousavi, S. R. (2016). Impacts of rubber-tired skidder and crawler tractor on forest soil in the mountainous forests of Northern Iran. Balt. For. 22, 375–381.
Naghdi, R., and Solgi, A. (2014). Effects of skidder passes and slope on soil disturbance in two soil water contents. Croat. J. For. Eng. 35, 73–80.
Naghdi, R., Solgi, A., and Ilstedt, U. (2016). Soil chemical and physical properties after skidding by rubber-tired skidder in Hyrcanian forest. Iran. Geoderma 265, 12–18. doi: 10.1016/j.geoderma.2015.11.009
Naghdi, R., Solgi, A., and Zenner, E. K. (2015). Soil disturbance caused by different skidding methods in mountainous forests of Northern Iran. Int. J. For. Eng. 26, 212–224. doi: 10.1080/14942119.2015.1099814
Nave, L. E., Vance, E. D., Swanston, C. W., and Curtis, P. S. (2010). Harvest impacts on soil carbon storage in temperate forests. For. Ecol. Manag. 259, 857–866. doi: 10.1016/j.foreco.2009.12.009
Nazari, M., Horvat, M., Joergensen, R. G., and Peth, S. (2020). Soil organic matter mobilization by re-compaction of old forest skid trails. Eur. J. Soil Biol. 98:103173. doi: 10.1016/j.ejsobi.2020.103173
Neruda, J., Kadlec, J., Ulrich, R., and Cudzik, A. (2010). “Soil carbon dioxide concentration and efflux changes in ruts after heavy machine passes,” in FORMEC 2010-Forest Engineering: Meeting the Needs of the Society and the Environment, Padova
Page-Dumroese, D. S., Jurgensen, M. F., Tiarks, A. E., Ponder, F., Sanchez, F. G., Fleming, R. L., et al. (2006). Soil physical property changes at the North American long-term soil productivity study sites: 1 and 5 years after compaction. Can. J. For. Res. 36, 551–564. doi: 10.1139/x05-273
Paz, L. W. (2001). Soil-Water Characteristics and Hydrologic Implications Following Forest Soil Disturbance: The Relative Influence of Organic Residue and Soil Compaction on Permeability and Moisture Capacity. Ph.D. dissertation. Berkeley, CA: University of California.
Picchio, R., Mederski, P. S., and Tavankar, F. (2020). How and how much, do harvesting activities affect forest soil, regeneration and stands? Cur. For. Rep. 6, 115–128. doi: 10.1007/s40725-020-00113-8
Picchio, R., Mercurio, R., Venanzi, R., Gratani, L., Giallonardo, T., Monaco, L. A., et al. (2018). Strip clear-cutting application and logging typologies for renaturalization of pine afforestation-A case study. Forests 9:366. doi: 10.3390/f9060366
Picchio, R., Neri, F., Maesano, M., Savelli, S., Sirna, A., Blasi, S., et al. (2011). Growth effects of thinning damage in a Corsican pine (Pinus laricio Poiret) stand in central Italy. For. Ecol. Manag. 262, 237–243. doi: 10.1016/j.foreco.2011.03.028
Picchio, R., Neri, F., Petrini, E., Verani, S., Marchi, E., and Certini, G. (2012). Machinery-induced soil compaction in thinning two pine stands in central Italy. For. Ecol. Manag. 285, 38–43. doi: 10.1016/j.foreco.2012.08.008
Picchio, R., Venanzi, R., Tavankar, F., Luchenti, I., Iranparast Bodaghi, A., Latterini, F., et al. (2019). Changes in soil parameters of forests after windstorms and timber extraction. Eur. J. For. Res. 138, 875–888. doi: 10.1007/s10342-019-01210-5
Ponder, F., and Tadros, M. (2002). Phospholipid fatty acids in forest soil four years after Organic matter removal and Soil compaction. Appl. Soil Ecol. 19, 173–182. doi: 10.1016/S0929-1393(01)00182-2
Potvliet, G. (2016). Impact van Gemachineerde Houtoogst op de Bosbodem & Effecten van het Werken met Dunningspaden. s.l. LL Ede: Bosgroep Midden Nederland.
Powers, R., Sanchez, F., Scott, D. A., and Page-Dumrosese, D. (2004). “The North American long-term soil productivity experiment: coast-to-coast findings from the first decade,” in USDA Forest Service Proceedings of RMRS-P-34, (Fort Collins, CO: U.S. Department of Agriculture), 191–206.
Powlson, D. S., and Jenkinson, D. S. (1976). The effects of biocidal treatments on metabolism in soil-II. Gamma irradiation, autoclaving, air-drying and fumigation. Soil Biol. Biochem. 8, 179–188. doi: 10.1016/0038-0717(76)90002-X
Price, K., Jackson, C. R., and Parker, A. J. (2010). Variation of surficial soil hydraulic properties across land uses in the southern Blue Ridge Mountains, North Carolina, USA. J. Hydrol. 383, 256–268. doi: 10.1016/j.jhydrol.2009.12.041
Proto, A. R., Macrì, G., Sorgonà, A., and Zimbalatti, G. (2016). Impact of skidding operations on soil physical properties in southern Italy. Contemp. Engi. Sci. 9, 1095–1104. doi: 10.12988/ces.2016.68132
Rab, M. A. (2004). Recovery of soil physical properties from compaction and soil profile disturbance caused by logging of native forest in Victorian Central Highlands, Australia. For. Ecol. Manag. 191, 329–340. doi: 10.1016/j.foreco.2003.12.010
Rumpel, C., and Kögel-Knabner, I. (2011). Deep soil organic matter-a key but poorly understood component of terrestrial C cycle. Plant Soil 338, 143–158. doi: 10.1007/s11104-010-0391-5
Sakai, H., Nordfjell, T., Suadicani, K., Talbot, B., and Bollehuus, E. (2008). Soil compaction on forest soils from different kinds of tires and tracks and possibility of accurate estimate. Croat. J. For. Eng. 29, 15–27.
Salomé, C., Nunan, N., Pouteau, V., Lerch, T. Z., and Chenu, C. (2010). Carbon dynamics in topsoil and in subsoil may be controlled by different regulatory mechanisms. Glob. Change Biol. 16, 416–426. doi: 10.1111/j.1365-2486.2009.01884.x
Schack-Kirchner, H., Fenner, P. T., and Hildebrand, E. E. (2007). Different responses in bulk density and saturated hydraulic conductivity to soil deformation by logging machinery on a Ferralsol under native forest. Soil Manag. 23, 286–293. doi: 10.1111/j.1475-2743.2007.00096.x
Schnurr-Pütz, S., Bååth, E., Guggenberger, G., Drake, H. L., and Küsel, K. (2006). Compaction of forest soil by logging machinery favours occurrence of prokaryotes. FEMS Microbiol. Ecol. 58, 503–516. doi: 10.1111/j.1574-6941.2006.00175.x
Shabaga, J. A., Basiliko, N., Caspersen, J. P., and Jones, T. A. (2017). Skid trail use influences soil carbon flux and nutrient pools in a temperate hardwood forest. For. Ecol. Manag. 402, 51–62. doi: 10.1016/j.foreco.2017.07.024
Shepperd, W. D. (1993). The effect of harvesting activities on soil compaction, root damage, and suckering in colorado aspen. West. J. Appl. For. 8, 62–66. doi: 10.1093/wjaf/8.2.62
Shestak, C. J., and Busse, M. D. (2005). Compaction alters physical but not biological indices of soil health. Soil Sci. Soc. Am. J. 69, 236–246. doi: 10.2136/sssaj2005.0236
Siepel, H. (2015). De rol van bodemfauna in oude bosbodems. Vakblad Natuur Bos Landschap. 12, 23–25.
Smeltzer, D. L. K., Bergdahl, D. R., and Donnelly, J. R. (1986). Forest ecosystem responses to artificially induced soil compaction. II. Selected soil microorganism populations. Can. J. For. Res. 16, 870–872. doi: 10.1139/x86-154
Sohrabi, H., Jourgholami, M., Jafari, M., Shabanian, N., Venanzi, R., Tavankar, F., et al. (2020). Soil recovery assessment after timber harvesting based on the sustainable forest operation (SFO) perspective in Iranian temperate forests. Sustainability 12:2874. doi: 10.3390/su12072874
Sohrabi, H., Jourgholami, M., Tavankar, F., Venanzi, R., and Picchio, R. (2019). Post-harvest evaluation of soil physical properties and natural regeneration growth in steep-slope terrains. Forests 10:1034. doi: 10.3390/f10111034
Solgi, A., and Najafi, A. (2014). The impacts of ground-based logging equipment on forest soil. J. For. Sci. 60, 28–34. doi: 10.17221/76/2013-jfs
Soltanpour, S., and Jourgholami, M. (2013). soil bulk density and porosity changes due to ground-based timber extraction in the hyrcanian forest. Not. Sci. Biol. 5, 263–269. doi: 10.15835/nsb528951
Startsev, A. D., and McNabb, D. H. (2000). Effects of skidding on forest soil infiltration in west-central Alberta. Can. J. Soil Sci. 80, 617–624. doi: 10.4141/S99-092
Startsev, N. A., McNabb, D. H., and Startsev, A. D. (1998). Soil biological activity in recent clearcuts in west-central Alberta. Can. J. Soil Sci. 78, 69–76. doi: 10.4141/S97-028
Stone, M. M., Kan, J., and Plante, A. F. (2015). Parent material and vegetation influence bacterial community structure and nitrogen functional genes along deep tropical soil profiles at the Luquillo Critical Zone Observatory. Soil Biol. Biochem. 80, 273–282. doi: 10.1016/j.soilbio.2014.10.019
Tan, X., and Chang, S. X. (2007). Soil compaction and forest litter amendment affect carbon and net nitrogen mineralization in a boreal forest soil. Soil Tillage Res. 93, 77–86. doi: 10.1016/j.still.2006.03.017
Tan, X., Chang, S. X., and Kabzems, R. (2005). Effects of soil compaction and forest floor removal on soil microbial properties and N transformations in a boreal forest long-term soil productivity study. For. Ecol. Manag. 217, 158–170. doi: 10.1016/j.foreco.2005.05.061
Tan, X., Chang, S. X., and Kabzems, R. (2008). Soil compaction and forest floor removal reduced microbial biomass and enzyme activities in a boreal aspen forest soil. Biol. Fertil. Soils 44, 471–479. doi: 10.1007/s00374-007-0229-3
Tavankar, F., Bonyad, A. E., Nikooy, M., Picchio, R., Venanzi, R., and Calienno, L. (2017). Damages to soil and tree species by cable-skidding in caspian forests of Iran. For. Syst. 26:e009. doi: 10.5424/fs/2017261-09100
Tavankar, F., Picchio, R., Nikooy, M., Jourgholami, M., Naghdi, R., Latterini, F., et al. (2021). Soil natural recovery process and Fagus orientalis lipsky seedling growth after timber extraction by wheeled skidder. Land 10:113. doi: 10.3390/land10020113
Teepe, R., Brumme, R., Beese, F., and Ludwig, B. (2004). Nitrous oxide emission and methane consumption following compaction of forest soils. Soil Sci. Soc. Am. J. 68, 605–611. doi: 10.2136/sssaj2004.6050
Tetzlaff, D., Soulsby, C., Waldron, S., Malcolm, I. A., Bacon, P. J., Dunn, S. M., et al. (2007). Conceptualization of runoff processes using a geographical information system and tracers in a nested mesoscale catchment. Hydrol. Process. 21, 1289–1307. doi: 10.1002/hyp.6309
Torbert, H. A., and Wood, C. W. (1992). Effects of Soil Compaction and Water-Filled Pore Space on Soil Microbial Activity and N Losses. Commun. Soil Sci. Plant Anal. 23, 1321–1331. doi: 10.1080/00103629209368668
Turner, S., Schippers, A., Meyer-Stüve, S., Guggenberger, G., Gentsch, N., Dohrmann, R., et al. (2014). Mineralogical impact on long-term patterns of soil nitrogen and phosphorus enzyme activities. Soil Biol. Biochem. 68, 31–43. doi: 10.1016/j.soilbio.2013.09.016
Venanzi, R., Picchio, R., Grigolato, S., and Latterini, F. (2019). Soil and forest regeneration after different extraction methods in coppice forests. For. Ecol. Manag. 454:117666. doi: 10.1016/j.foreco.2019.117666
Venanzi, R., Picchio, R., Grigolato, S., and Spinelli, R. (2020a). Soil disturbance induced by silvicultural treatment in chestnut (Castanea sativa mill.) coppice and post-disturbance recovery. Forests 11:1053. doi: 10.3390/f11101053
Venanzi, R., Picchio, R., Spinelli, R., and Grigolato, S. (2020b). Soil disturbance and recovery after coppicing a mediterranean oak stand: the effects of silviculture and technology. Sustainability 12:4074. doi: 10.3390/SU12104074
Vossbrink, J., and Horn, R. (2004). Modern forestry vehicles and their impact on soil physical properties. Eur. J. For. Res. 123, 259–267. doi: 10.1007/s10342-004-0040-8
Wallbrink, P. J., Roddy, B. P., and Olley, J. M. (2002). A tracer budget quantifying soil redistribution on hillslopes after forest harvesting. Catena 47, 179–201. doi: 10.1016/S0341-8162(01)00185-0
Wang, J., LeDoux, C. B., and Edwards, P. (2007). Changes in soil bulk density resulting from construction and conventional cable skidding using preplanned skid trails. North. J. Appl. For. 24, 5–8. doi: 10.1093/njaf/24.1.5
Wang, L. (1997). Assessment of animal skidding and ground machine skidding under mountain conditions. (1997). J. For. Eng. 7, 63–72. doi: 10.1080/08435243.1997.10702704
Wei, X., Shao, M., Gale, W., and Li, L. (2014). Global pattern of soil carbon losses due to the conversion of forests to agricultural land. Sci. Rep. 4:4062. doi: 10.1038/srep04062
Wert, S., and Thomas, B. R. (1981). Effects of skid roads on diameter. Height, and Volume Growth in Douglas-fir. Soil Sci. Soc. Am. J. 45, 629–632. doi: 10.2136/sssaj1981.03615995004500030038x
Williamson, J. R., and Neilsen, W. A. (2000). The influence of forest site on rate and extent of soil compaction and profile disturbance of skid trails during ground-based harvesting. Can. J. For. Res. 30, 1196–1205. doi: 10.1139/x00-041
Wronski, E. B., and Murphy, G. (1994). Responses of Forest Crops to Soil Compaction. Dev. Agric. Eng. 11, 317–342. doi: 10.1016/B978-0-444-88286-8.50022-2
Yee, C. S., and Harr, R. D. (1977). Effect of Wetting Mode on Shear Strength of Two Aggregated Soils in the Oregon Coast Ranges. USDA Forest Service Research Note PNW-303. Portland, OR: Pacific Northwest Forest and Range Experiment Station.
Ziegler, A. D., Negishi, J. N., Sidle, R. C., Gomi, T., Noguchi, S., and Nik, A. R. (2007). Persistence of road runoff generation in a logged catchment in Peninsular Malaysia. Earth Surf. Process. Landf. 32, 1947–1970. doi: 10.1002/esp.1508
Keywords: soil compaction, forest soils, logging, microbial biomass carbon, soil physical properties
Citation: Nazari M, Eteghadipour M, Zarebanadkouki M, Ghorbani M, Dippold MA, Bilyera N and Zamanian K (2021) Impacts of Logging-Associated Compaction on Forest Soils: A Meta-Analysis. Front. For. Glob. Change 4:780074. doi: 10.3389/ffgc.2021.780074
Received: 20 September 2021; Accepted: 03 November 2021;
Published: 03 December 2021.
Edited by:
Jess K. Zimmerman, University of Puerto Rico, Puerto RicoReviewed by:
Miren Lorente, Environment and Climate Change Canada, CanadaRodolfo Picchio, University of Tuscia, Italy
Copyright © 2021 Nazari, Eteghadipour, Zarebanadkouki, Ghorbani, Dippold, Bilyera and Zamanian. This is an open-access article distributed under the terms of the Creative Commons Attribution License (CC BY). The use, distribution or reproduction in other forums is permitted, provided the original author(s) and the copyright owner(s) are credited and that the original publication in this journal is cited, in accordance with accepted academic practice. No use, distribution or reproduction is permitted which does not comply with these terms.
*Correspondence: Meisam Nazari, TWVpc2FtLm5hemFyaUBmb3JzdC51bmktZ29ldHRpbmdlbi5kZQ==; TWVpc2FtLm5hemFyaTE5OTFAZ21haWwuY29t