- 1Centre for Tropical Environmental and Sustainability Science, College of Science and Engineering, James Cook University, Cairns, QLD, Australia
- 2Skyrail Rainforest Cableway, Cairns, QLD, Australia
Lianas are increasing in abundance in many tropical forests. This increase can alter forest structure and decrease both carbon storage and tree diversity via antagonistic relationships between lianas and their host trees. Climate change is postulated as an underlying driver of increasing liana abundances, via increases in dry-season length, forest-disturbance events, and atmospheric CO2 concentrations; all factors thought to favour lianas. However, the impact of climate change on liana reproductive phenology, an underlying determinant of liana abundance, has been little studied, particularly outside of Neotropical forests. Over a 15-year period (2000–2014), we examined the phenological patterns of a liana community in intact rainforests of the Wet Tropics bioregion of Australia; a World Heritage Area and hotspot of floral diversity. Specifically, we assessed (1) flowering and fruiting patterns of liana species; (2) potential climate drivers of flowering and fruiting activity; and (3) the influence of El Niño-related climatic disturbances on liana phenology. We found that flowering and fruiting of the studied liana species increased over time. Liana reproduction, moreover, rose in apparent response to higher temperatures and reduced rainfall. Finally, we found flowering and fruiting of the liana species increased following El Niño events. These results suggest that liana reproduction and abundance are likely to increase under predicted future climate regimes, with potentially important impacts on the survival, growth, and reproduction of resident trees and thus the overall health of Australian tropical rainforests.
Introduction
The combined pressures of global climate change and anthropogenic land modification are having profound impacts on the structure and function of tropical forests (Laurance et al., 2002; Phillips et al., 2002; Lewis et al., 2004). Losses of old-growth trees and the proliferation of pioneers and lianas have been documented in degraded and fragmented forests across the tropics (Laurance et al., 2014; Marshall et al., 2020). However, even fully intact forests may be subjected to changes in forest structure and composition resulting from large-scale drivers such as global climate change or rising atmospheric CO2 levels, as has been observed in the Brazilian Amazon (Phillips et al., 2002; Laurance et al., 2014). Natural disturbances such as cyclones and droughts also shape tropical forest ecosystems by controlling their structure, species composition, and functional processes (Dale et al., 2001). Changes to the structure and composition of tropical forests will have important implications for biodiversity conservation and ecosystem services (Rice et al., 2004; Hector et al., 2011).
An important driver of forest structure and function is the change in timing and intensity of floral phenological activity due to variations in climate conditions (Gray and Ewers, 2021). Global and regional models of climate change project substantial change in temperature and rainfall patterns, as well as future increases in extreme climatic events, such as cyclones and ENSO, as well as natural disturbance events (IPCC, 2021). Plant phenology is primarily regulated by climate with current expectations suggesting a shift in the timing and intensity of flower and fruit production as a response to climate change (Menzel et al., 2006; Cleland et al., 2007; Richardson et al., 2013; Tang et al., 2016). Modification of rainfall patterns and increases in the frequency of El Niño events will result in more regular drought events in tropical forests (Cai et al., 2014) also impacting phenological patterns. Additionally, the stresses of higher ambient temperatures will result in more leaf-shedding events, higher tree mortality rates, and an increase in tropical forest fires (Cochrane and Laurance, 2008). Disruption to phenological patterns, as well as natural disturbances, may favour the proliferation of early successional tree species and other disturbance-adapted plant guilds such as lianas.
Previous studies have shown that liana abundance is increasing in intact tropical forests in response to large-scale drivers (Laurance et al., 2001a; Schnitzer and Bongers, 2002, 2011; Schnitzer, 2005; Campbell et al., 2015, 2018). Climate-related changes in the phenology and reproduction of lianas and trees could further influence their relative abundance in tropical forests. For example, increasing reproduction of liana species has been observed in Panama (Wright and Calderón, 2006, 2018; Chapman et al., 2018) and Mexico (Cortés-Flores et al., 2015), and may relate to rising levels of atmospheric CO2 (Granados and Korner, 2002), dry-season intensity, or temperature (Marvin et al., 2015). Additionally, lianas have structural and physiological adaptations that make them resilient to, and allow them to benefit from, many types of disturbances (Oliveira et al., 1997; Laurance et al., 2006, 2011; Schnitzer and Carson, 2010; Paul and Yavitt, 2011; Ledo and Schnitzer, 2014). Schnitzer et al. (2021), for example, proposed that the ability of lianas to increase and capitalise on forest disturbance and the spiralling interaction between disturbance and climate change, could be behind increasing liana abundance in Neotropical forests. As such, predicted increases in the rates of disturbance and drier conditions pantropically will likely increase liana abundances relative to old-growth trees and other plant species adapted to undisturbed tropical forests.
Potential increases in liana abundance are important for forest management and conservation as the proliferation of lianas can have a detrimental impact on host trees (Schnitzer and Bongers, 2002). Lianas are a structural parasite of trees and compete for nutrients, water, and sunlight; severely impacting tree growth and survival (Putz, 1984; Laurance et al., 2001a). Increased liana loads have been found to negatively impact tree reproduction in Uganda (Babweteera et al., 2018), Brazil (Kainer et al., 2006), Panama (Wright, 2005; García León et al., 2018), and Malaysia (Wright et al., 2015). Evidence also indicates that lianas may have higher comparative pollination success when compared to trees (Boulter et al., 2009). Hence, a proliferation of lianas could lead to both higher mortality and reduced recruitment of tropical trees, with important implications for forest biodiversity and carbon storage.
Here we present a long-term phenological study of the liana community found across an elevational gradient (ca. 5–540 m elevation) in the Australian wet tropics, as well as an analysis of the relationship between climate drivers and liana phenology. Specifically, we examined: (1) flowering and fruiting patterns and seasonality of the liana community; (2) the potential climate drivers of these flowering and fruiting patterns; and (3) assessed how liana phenology was responding to observed changes in temperature and rainfall through time, with the aim of projecting future changes in phenological timing and duration for the liana species assemblage we studied.
Materials and Methods
Study Area
Phenological observations of the canopy of a tropical rain forest were conducted for 15 years (2000–2014 inclusive) using the Skyrail Rainforest Cableway,1 a 7.5 km scenic gondola cableway running above the Barron Gorge National Park (Figure 1). Barron Gorge National Park comprises part of the Wet Tropics World Heritage Area of Australia (16.84°S, 145.64°E), whose listing was partially due to its recognition as a hotspot of plant and animal biodiversity. The vegetation community of the park, part of the traditional lands of the Djabugandji people (traditional owners), is composed of a closed-canopy mesophyll/notophyll vine forest (Tracey, 1982).
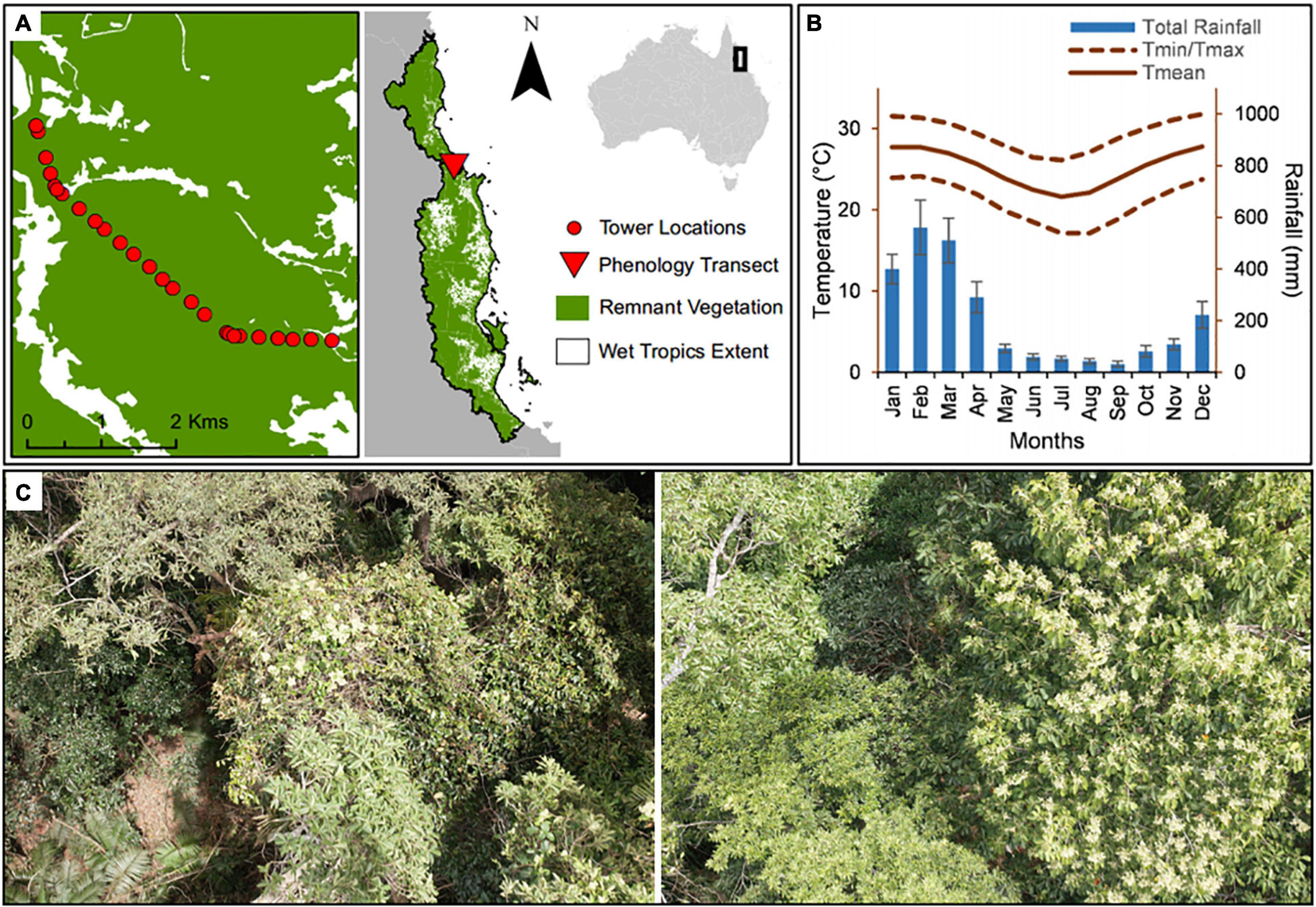
Figure 1. Location and mean annual climate for the study region. (A) Wet Tropics World Heritage Area extent with phenology transect located in Barron Gorge National Park, Far North Queensland, Australia. (B) Monthly general meteorological patterns of rainfall (mm), maximum temperature (Tmax °C), minimum temperature (Tmin °C), and mean temperature (Tmean °C) from January 2000 to December 2014, and (C) View from the forest canopy as seen from the gondola.
The study region experiences climatic seasonality, with a drier season from June to November and a wetter season from December to May (Figure 1). The forests in the Wet tropics are frequently subjected to disturbances including cyclones and El Niño-related droughts and heat-waves; making them highly vulnerable to climate change impacts (Hughes, 2003; Turton, 2012). Climatic information for the study area was obtained through the Australian Bureau of Meteorology (BOM) for the Kuranda Railway Station weather station (BOM, n.d., station numbers 031036 and 031011) (Figure 2) located at the upper end of the cableway.
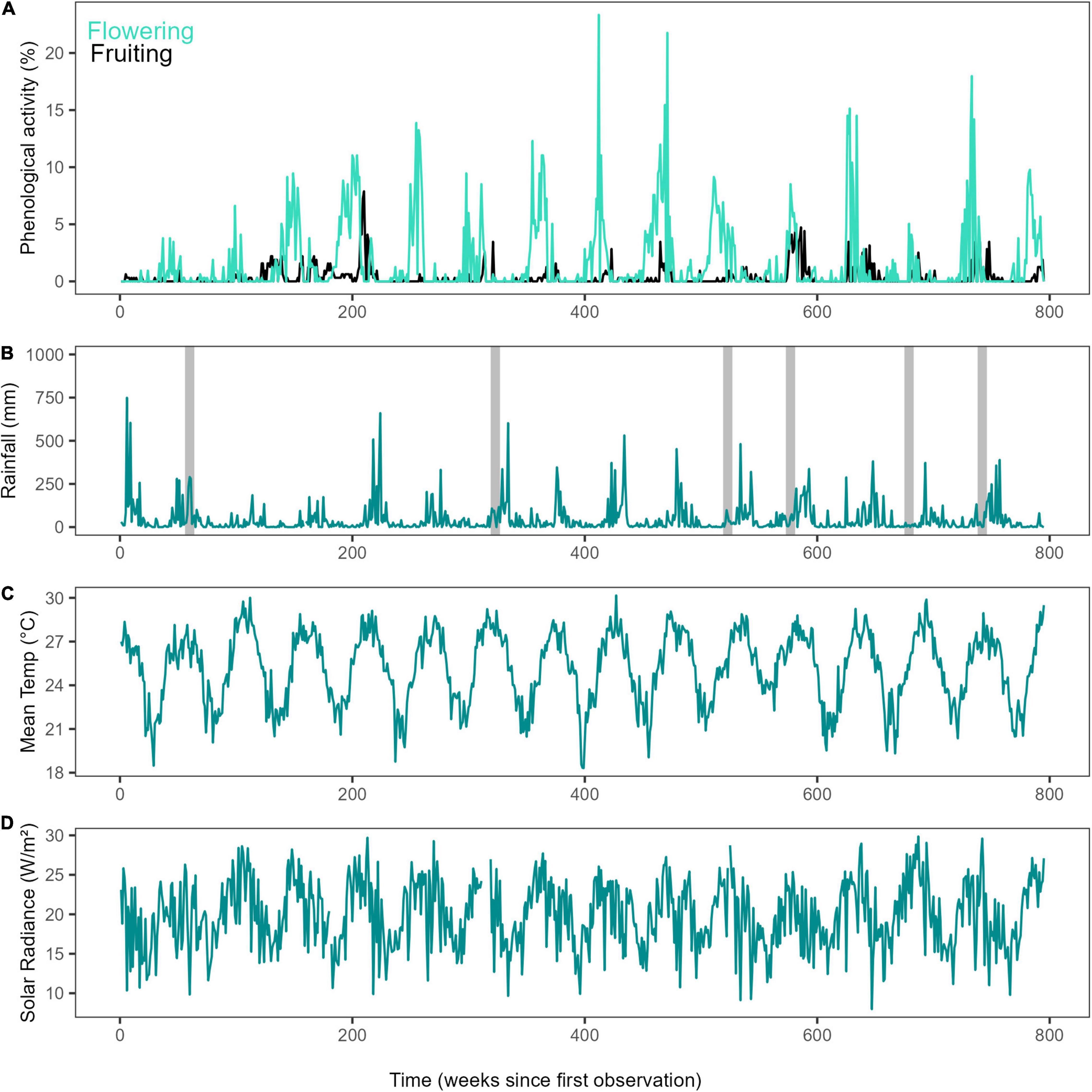
Figure 2. Time series of long-term phenology and climate. (A) Weekly proportion of plots with flowering and fruiting activity for the studied period (2000–2014) in Barron Gorge National Park, Far North Queensland, Australia, (B) Time series for weekly rainfall (mm) and cyclones (grey bars), (C) Mean daily temperature (°C), and (D) Mean daily solar irradiance (W/m2).
Phenological Observations
Flowering and fruiting activity along a 7.5 km transect of the Skyrail gondola were recorded weekly from January 2000 to December 2014 by the same observer. The observer scored phenology from above using a maintenance gondola with unimpeded visual access to the canopy. To quantify observation records, the total area sampled was divided into 10 subplots of ∼100 m width and ∼25 m length, between each pair of towers, for a total of 320 subplots. The total area sampled using the 320 subplots was estimated to be approximately 0.75 km2. We recorded presence or absence of flowering and fruiting activity within each subplot for the conspicuous liana community, composed of 17 species (Supplementary Table 1).
Visual identification of species from the gondola was cross-checked against plant ground samples (flowers, fruits, and leaves) that were identified using the Australian Rainforest Key (Zich et al., 2018), developed by the Australian Tropical Herbarium. The botanical observer was trained by professional botanists (Australian Tropical Herbarium, Cairns Regional Council) and independent verification of plant identification was carried out using plant samples collected on the ground. In addition high resolution digital photographs (Sony DSLR α-700P 12MP) were collected along the 7.5 km transect and these were then examined against the Australian Rainforest Key to further cross-check the ability of the observer to correctly identify liana activity to the species level from the gondola. We considered presence of phenological activity (flowers or fruits) in a single subplot as a single observation. We then calculated, for each week and month, the mean percentage of phenological activity for the transect. To calculate the percentage activity for the transect, we found, for each species, the maximum number of subplots (from a total of 320) presenting phenological activity at one time. We then summed these maximum values to estimate the potential maximum activity for the entire transect, and calculated the percentage activity by comparing the total activity at each time point to this maximum value (Figure 2). Percentage activity was calculated using the weekly observations of the original records, and aggregated to monthly values by taking the maximum percentage.
Species-specific phenological patterns were assessed for the eight most dominant species, which together accounted for 80% of the phenological records. We assessed the long-term weekly patterns for each species individually and the summed percent of weekly activity. The most dominant species were: Austrosteenisia blackii (F.Muell.), Austrosteenisia stipularis (C.T.White) Jessup, Cissus penninervis (F.Muell.) Planch, Dichapetalum papuanum (Becc.) Boerl, Embelia caulialata S.T.Reynolds, Faradaya splendida F.Muell., Neosepicaea jucunda (F.Muell.) Steenis, and Parsonsia latifolia (Benth.) S.T.Blake.
Statistical Analysis
To assess year-to-year phenological patterns and seasonality, we conducted circular statistical analyses using the monthly percentage of activity for flowering and fruiting. To calculate the circular statistical parameters, months were converted to angles, where the first month, January, is transformed into angle 0° and subsequent months are added in intervals of 30° until December at 330° (Morellato et al., 2010). We then calculated the mean angle, circular standard deviation (SD), and vector length (r). Vector length (r) indicates the degree of seasonality, varying from 0 (aseasonal) to 1 (seasonal) (Zar, 1996), while the circular standard deviation can be an indicator of season length (Ting et al., 2008). To determine if phenological patterns were significantly seasonal, we used Rayleigh tests (Zar, 1999).
We assessed long-term patterns of flowering and fruiting using generalised additive models (GAMs) with monthly phenology (maximum weekly percentage of activity for the month) as the response variable and date, temperature, rainfall, and solar radiation as the explanatory variables. Phenological and climate time series were detrended in order to remove the intra-annual seasonal and random components using the R package “forecast” (Hyndman et al., 2021).
We identified the potential climate drivers of liana phenology based on both seasonal patterns (prior to detrending) and long-term trends (post detrending). As relationships between phenological activity and drivers are often reported as being non-linear, we used Generalised Additive Models for Location, Scale and Shape (GAMLSS) to model relationships (Hudson et al., 2010). We used GAMLSS as they accommodate unconventional distributions and the temporal autocorrelation inherent to phenological series. Model distributions were chosen to maximise fit of each model. We assumed a Beta distribution, however, the model fit and residuals were substantially better when a Gaussian distribution was used for both flowering and fruiting (with a log link function). We developed the GAMLSS models using a cubic spline smoothing function, and included an auto-regressive AR(1) correlation structure for the response variable (term of 1 month). Collinearity between explanatory variables was assessed with concurvity, a generalisation of collinearity appropriate for GAMs. Proportion of individual subplots in flowering or fruiting activity were used as response variables, and monthly rainfall (mm), monthly mean temperature (°C), monthly mean photoperiod (h/day), and monthly mean solar radiation (W/m2) were used as predictor variables. Optimal models were selected using the Aikaike Information Criteria (AIC).
To assess possible influence of climatic disturbance events, ENSO variation and cyclones, as well as inter-annual influence of climate variables on phenological activity, we conducted cross-correlation analyses against the phenological activity time series. We decomposed the phenological activity time series and removed the seasonal component prior to cross-correlation using the “stl” function in base R. Influence of ENSO was modelled using the multivariate ENSO index (MEI) and cyclones were treated as a discrete event with values of 1 for months when cyclones occurred and 0 when they did not occur. Time series analysis was conducted using the “timeSeries” R package (Wuertz, 2020). All analyses have been conducted in R studio (R Core Team, 2019).
Results
We identified a long-term increase in flowering activity of the liana community in the study area and two supra-annual peaks in fruiting activity, in November 2004 and January 2011 (Figure 2). Overall visualisation of annual total rainfall and mean maximum temperature showed that although rainfall may be variable in the region, temperature has been increasing since 1950 (Supplementary Figure 3). The studied period was not anomalous to the general trends and patterns. Time in number of months since first observation was found to have a significant positive effect on both flowering and fruiting activity (F = 31.74, P < 0.0001 and F = 38.54, P < 0.001, respectively) as assessed using GAMs performed on seasonally detrended data (trend component of time series) of monthly maximum values. Long-term patterns of flowering noted a continuous increase through time, but fruiting increased, decreased and increased again, forming two peaks (Figure 3) when assessing the data visually. Rainfall showed a linear decrease, while mean temperature showed a linear increase over time (Supplementary Figure 4). Additionally, mean temperature (flowering: F = 5.62, P < 0.001, and fruiting: F = 3.58, P < 0.01), rainfall (flowering: F = 2.85, P < 0.01 and fruiting: F = 7.32, P < 0.0001), solar irradiance (flowering: F = 9.01, P < 0.0001 and fruiting: F = 5.61, P < 0.001), and MEI (flowering: F = 4.41, P < 0.01 and fruiting: F = 22.46, P < 0.0001) all influenced long-term flowering and fruiting trends.
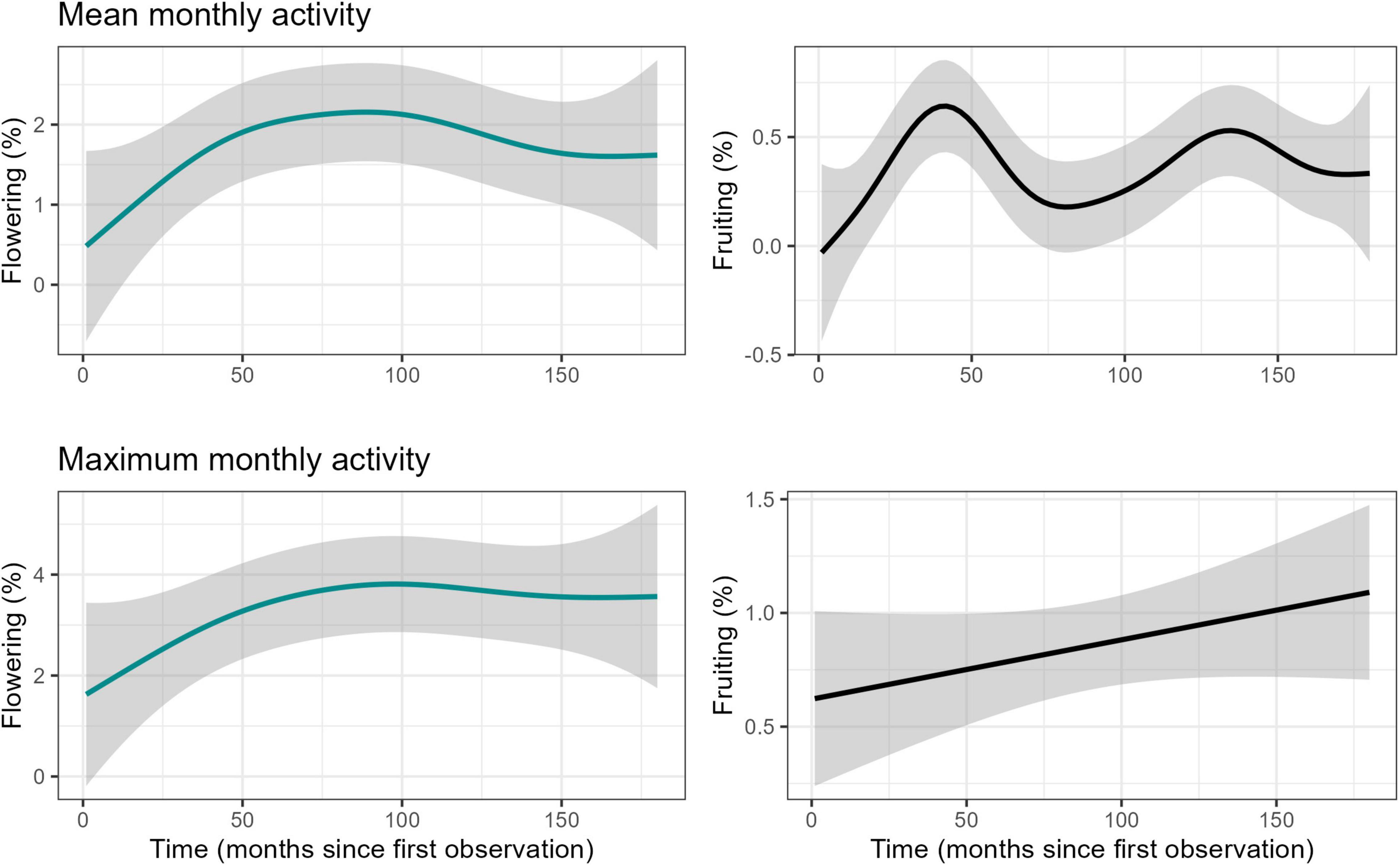
Figure 3. Fitted GAM-smoother for long-term relationship between monthly mean and maximum phenological activity and time (months since first observation), for the liana community in Barron Gorge National Park, Far North Queensland, Australia.
When analysing intra-annual patterns, GAMLSS smoothers for temperature, rainfall and solar radiation were significant (P < 0.001) for flowering activity (Figure 4 and Supplementary Table 3). Conversely, smoothers for temperature and solar radiation were significant for fruiting activity (Figure 4 and Supplementary Table 3). The seasonal peak of fruiting activity was significantly related to higher mean temperatures and low levels of solar radiation (Figure 4). Similarly, flowering activity increased with an increase in temperature, peaking when the temperature was between 28 and 30 degrees Celsius. Additionally, flowering activity was negatively correlated with rainfall (Figure 4). Fruiting presented a negative relationship with solar radiation, peaking at the minimum levels of solar radiation (Figure 4).
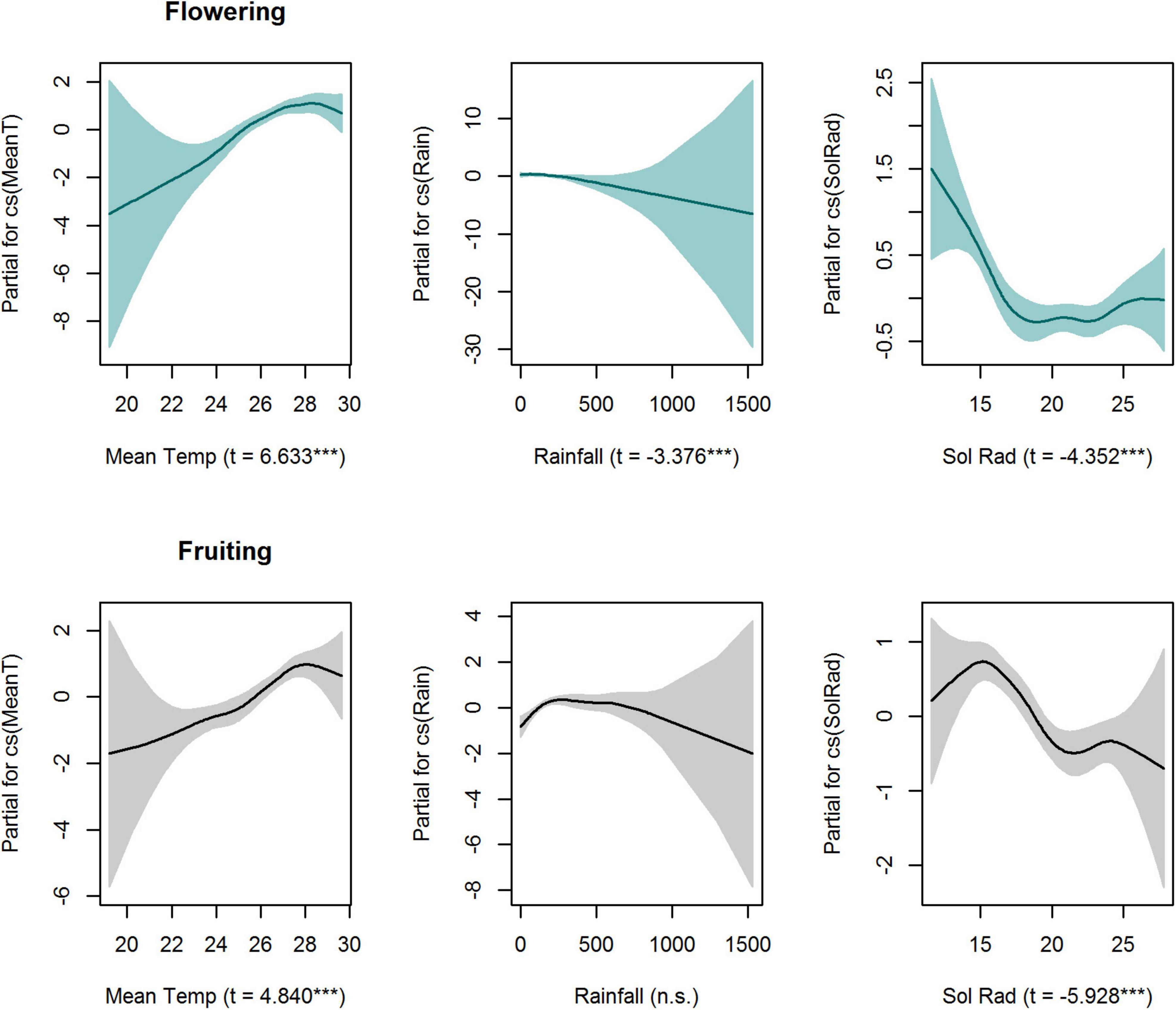
Figure 4. Partial effects of GAMLSS explanatory variables on flowering and fruiting seasonal trends in a liana community (17 species) (Cox Snell R2 0.48 and R2 0.26, respectively), in Barron Gorge National Park, Far North Queensland, Australia. The shaded areas indicate the 95% confidence intervals. GAMLSS-smoother terms for total monthly rainfall (mm), mean monthly temperature (°C), and mean monthly solar radiation (MJ/m2) were significant for flowering (P < 0.05) whereas mean temperature (°C) and mean monthly solar radiation (MJ/m2) were significant for fruiting. T-values and significance (***P < 0.001) are presented within parenthesis in x-axis title.
MEI (ENSO index) had a significant influence on both flowering and fruiting activity (as assessed using cross-correlation analyses), and a significant influence of cyclones on flowering. Flowering increased at a lag of 19 months following increased MEI values, indicating an El Niño event (r = 0.20), and fruiting activity was found to decrease 4 months after an El Niño event (r = −0.18), and then increased 9 months after an El Niño event (r = 0.24). As the relationship between phenological activity and MEI was related to increases in MEI, the results suggest the relationship was caused by El Niño events. In tropical Australia, El Niño events lead to dry, hot weather, and this relationship is supported by cross-correlation analyses between MEI and rainfall, temperature, and solar radiation (Table 1A). Moreover, these cross-correlation analyses between MEI and climate variables indicated that solar radiation increased 4 months before increase in MEI, with mean temperature increasing 4 months after increase in MEI. MEI and rainfall had concurrent changes, with lag of 0 being the significant one, indicating a significant decrease in rainfall (Table 1A). Flowering activity was also found to significantly decrease 4 months before a cyclone (r = -0.27), while fruiting was not significantly affected (Table 1B).
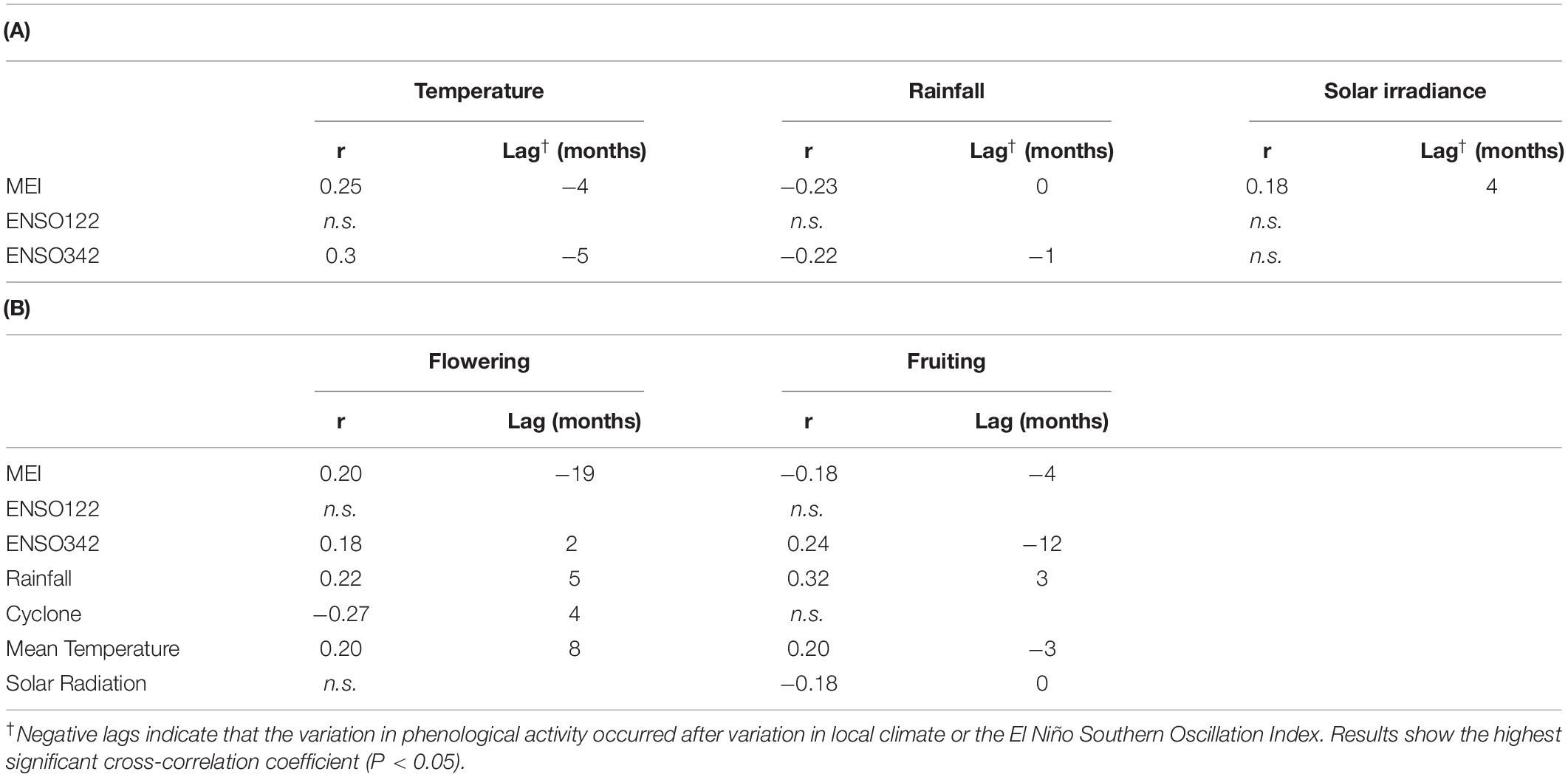
Table 1. Cross correlations among monthly values of flowering and fruiting activity (percentage of subplots showing phenological activity) on Barron Gorge National Park, Far North Queensland; (A) Between three indices of the El Niño Southern Oscillation and climate variables (rainfall, mean temperature, and solar irradiance) recorded the study area, and (B) Between climate variables, including El Niño Southern Oscillation index and cyclone, and phenological activity (flowering and fruiting).
In general, the studied liana community presented intra-annual seasonality, with a peak of flowering activity at the end of the dry season (October) and a peak of fruiting activity in the middle of the wet season (January) (Supplementary Figure 1) when the weekly circular phenological data was analysed over the whole study period. Seasonal phenological patterns were consistent among species, and community patterns were not dependent on any dominant species (Figure 5). A general pattern of increase in flowering was found for the community with five out of the eight species presenting an increase. Further, the majority of species increased fruiting activity. Two clear exceptions were present, however, with Parsonsia latifolia presenting a decrease in fruiting activity and Cissus penninervis, displaying a decrease in activity of both phenophases over time. Flowering at community level was seasonal in all years, and fruiting was mostly seasonal years with the exception of 2000 and 2009 (Rayleigh test P < 0.05; Supplementary Table 2) as determined through assessing the weekly phenological data using circular statistics.
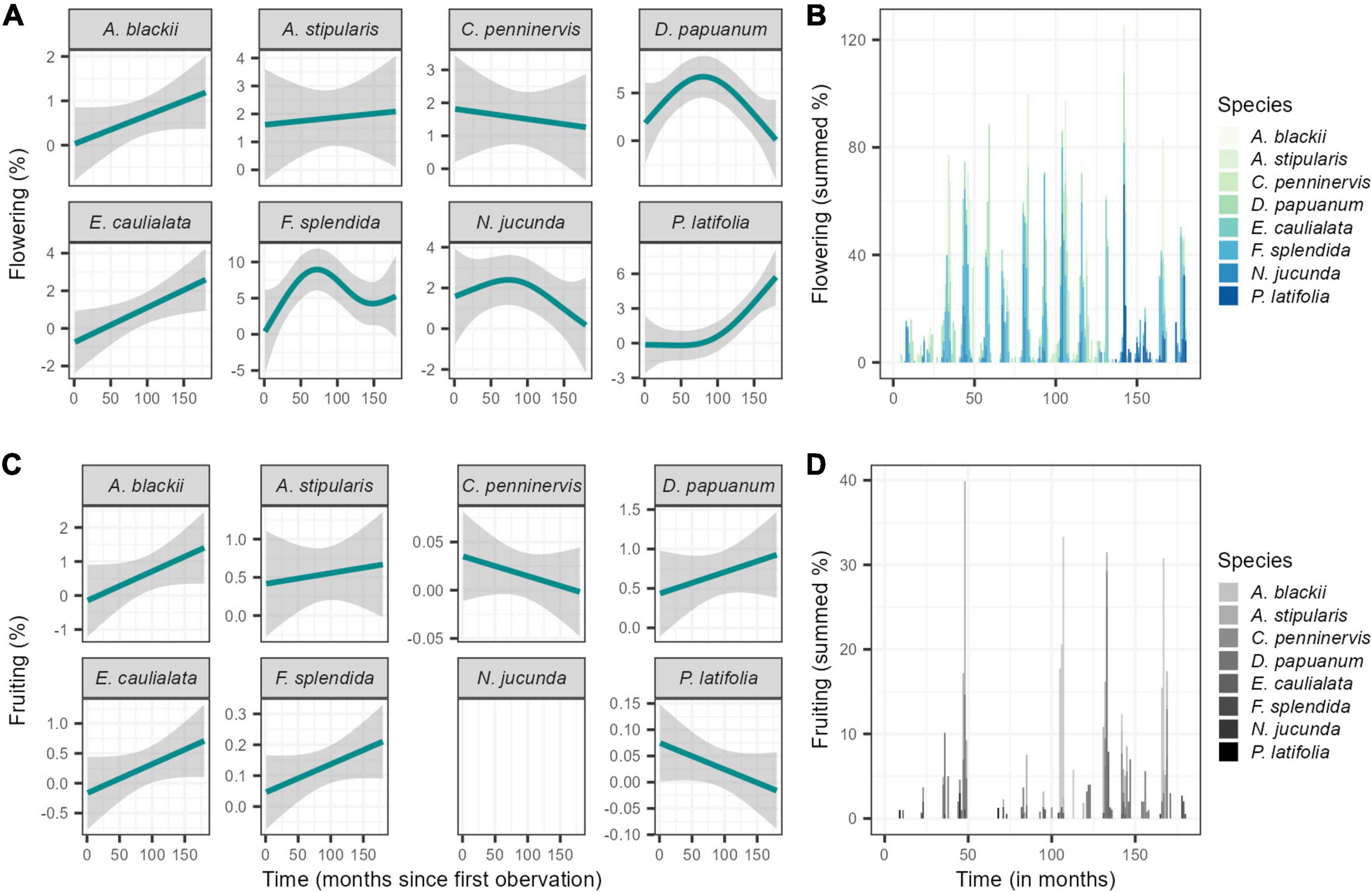
Figure 5. (A) Fitted GAM-smoother for monthly mean percentage of overall flowering and fruiting trends for each of the dominant species and (B) Summed monthly mean activity of flowering and fruiting for the dominant species in Barron Gorge National Park, Far North Queensland, Australia.
Although presenting clear seasonality in the flowering and fruiting activity for most years (Supplementary Table 2), the synchronicity of activity was low (below 20%). However, the percentage of flowering activity occurred in supra-annual peaks–activity above 15%, in October 2007 (23.3%), November 2008 (21.8%), November 2011 (15.1%), and November 2013 (18%). When comparing differences among years, fruiting showed increased activity in 2003 (2.04-fold) and in 2011 (1.6-fold).
We found a significant delay in timing of flowering for the years of 2001, 2010, 2011, and 2012 with delays of 18, 33, 25, and 25 days, respectively, when comparing mean dates from each year to the general mean date for the whole studied period. The years of 2010 and 2011 endured concurrent La Niña and cyclones events. Fruiting activity was highly variable, but mean dates were mainly found to occur between late November and January (Supplementary Table 2).
Discussion
Reproductive Activity of Lianas Is Increasing Through Time
Reproductive activity (flowering and fruiting) of lianas in our study site in the Australian wet tropics showed an increase over the study period from 2000 to 2014. Species-specific patterns, although variable, suggest community patterns were not significantly influenced by any individual species. In fact, five of the eight dominant species showed an increase in flowering activity over the examined time. However, one species, Cissus penninervis, displayed a decrease in both flowering and fruiting activity. Moreover, the species Parsonsia latifolia, displayed an interesting sharp increase in flowering activity, but a decrease in fruiting. These finding suggest, that at least in part, some responses to climate and climate change may be species dependent, though whether the responses are due to physiological or ecological limitations (or a synergism of the two) requires further investigation. Overall, an increase in liana phenological activity over time, particularly flowering, has also been found in other tropical regions such as Panama (Wright and Calderón, 2006; Pau et al., 2018). This increase may be linked to an increase in temperature, seasonality, or higher fertilisation of plant species from rising atmospheric CO2 levels (Granados and Korner, 2002; Körner, 2009), as suggested by Manzanedo et al. (2018) and Pau et al. (2018). Additionally, both fruiting and flowering activity in liana species increased with higher temperatures, both between and within years. This fertilisation response, coupled with rising mean temperatures due to apparent climate change (Australia has warmed by 1.44 ± 0.24°C since 1910; Commonwealth of Australia, 2020), may be triggering faster growth and reproduction of lianas in the Australian wet tropics.
Our findings also demonstrate that increased flowering activity of liana species was associated with lower rainfall, both between and within different years. In Ghana, Swaine and Grace (2007) found that liana richness was higher in drier forests, suggesting an adaptation of lianas to habitats with high evapotranspirative demand. Cai et al. (2009) and Medina-Vega et al. (2021) likewise found that physiological and trait differences give lianas an adaptive advantage over trees in more seasonal and drier forests. Australia’s Wet tropics region is expected to see higher mean temperatures and an increase in rainfall seasonality, and hence altered dry-season length and drier conditions, under climate change (IPCC, 2021). These results suggest liana reproductive activity and abundance are likely to continue increasing in the Australian wet tropics (Syktus et al., 2020), and presumably elsewhere in the global tropics as well.
Phenological Activity Related to Cyclones and El Niño Disturbances
Supra-annual variations in liana reproduction, with fruit production increasing in specific years, suggests they may be responding to disturbance events such as El Niños and cyclones (Laurance and Curran, 2008). El Niños and cyclones both occur with some regularity in tropical Australia (Turton and Stork, 2009), making it difficult to untangle the influence of such events on plant phenology without multi-decadal studies. Despite this challenge, we were able to identify an increase in both liana fruiting and flowering following El Niño events—observations that align with findings in the Neotropics and African tropics (e.g., Panama: Wright and Calderón, 2006; Puerto Rico: Zimmerman et al., 2007; Uganda: Chapman et al., 2018).
Various studies have demonstrated that increasing forest disturbances, tree mortality, and forest turnover lead to an increase in liana abundance (Putz, 1984; Schnitzer and Bongers, 2011; Hogan et al., 2017; Umaña et al., 2019). As El Niño events often increase tree mortality and leaf shedding by drought-stressed trees, they can increase canopy openness and allow lianas to rapidly colonise forests (Laurance et al., 2001a) via their seeds, advance regeneration, or lateral growth. This facilitates large-scale recruitment of lianas in disturbed forests, which is then followed by fast vertical growth towards the canopy (Ingwell et al., 2010; Schnitzer and Bongers, 2011). There is also evidence that lianas are more physiologically capable than trees under drier conditions (Medina-Vega et al., 2021). Increasing liana reproduction related to disturbance events, coupled with their strong ability to colonise disturbed forests, is expected to increase liana abundance in tropical forests under climate change.
Increased Liana Reproduction and Changing Tropical Forests
The observed increase in liana reproduction may contribute to changes in forest composition and structure in the future. An increase in abundance of vines and lianas has already been observed in the Amazon (Laurance et al., 2014) and other Neotropical forests (Schnitzer et al., 2014) and in Southeast Asia (Wright et al., 2015). However, long-term studies on the competitive balance between trees and lianas in Australia are lacking. These results may provide preliminary evidence of increasing liana abundance in the tropical forests of Australia.
A greater abundance of lianas, and the associated increase in mortality of infested trees may result in important compositional and structural changes in tropical rainforests, including reduced tree diversity and forest-carbon storage (Laurance et al., 2001b). Moreover, an increase in liana abundance at the expense of climax tree species in Australian rain forests may have cascading impacts such as an increase in insect diversity (Odell et al., 2019) and a reduction in fruit resources for keystone species such as the southern cassowary – due to a proportionate increase in wind-dispersed seeds and decrease in per unit area fruit production (Zich et al., 2020). Liana species are often more adept than trees at responding to large-scale disturbance events, such as cyclones and El Niño droughts, and small-scale disturbances such as tree falls (Putz, 1984; Schnitzer and van der Heijden, 2019). The ability of lianas to grow faster and use water more efficiently than trees, particularly during the dry season (Schnitzer, 2005; Cai et al., 2009), facilitates their colonisation of treefall gaps after disturbance. Additionally, physiological adaptations such as higher hydraulic conductivity, maximum carbon-assimilation rate, increased water-use efficiency and decrease susceptibility to cavitation in the dry season (Zhu and Cao, 2010), could provide liana species further advantages under higher temperature and rainfall seasonality expected due to climate change.
Changes in Seasonality
The period 2010–2012 endured concurrent cyclones and La Niñas, and presented a significant delay in mean flowering date (33, 25, and 25 days, respectively). Contrastingly, years when El Niños occurred had an advance in the mean date of flowering when compared to the mean date for the study period. In regions where multiple extreme events can occur at the same time, as is the case for the Wet Tropics, it can be challenging to determine which event is the main influence. However, our results suggest that extreme events in the region may cause changes to the mean dates of flowering periods.
Changes to timing of flowering or fruiting may constitute a change to resource availability for pollinators and frugivores. Mismatches in activity can happen between flowering plants and their pollinators, which can decrease fruiting (Morellato et al., 2016), and resource availability for pollinators and nectivores. Hence, the delays in the timing of liana flowering observed in relation to extreme events might also have adverse impacts on forest communities.
Observed liana fruiting activity was generally low, a pattern previously observed in liana species in Africa (Ley and Claßen-Bockhoff, 2013) and Asia (Nakonechnaya et al., 2021). Interestingly, the supra-annual peaks we observed for flowering were not accompanied by a proportional increase in fruit availability. Previous work in tree and shrub species has shown that reduced fruit set in relation to flower production may be due to resource limitation or reduced pollinator activity (Rathcke, 2000; Cunningham et al., 2020). When combined with the observed overall increase in liana activity and potential changes to floristic composition and structure, these extreme events are also unlikely to provide any benefit to frugivore communities.
Conclusion
We found that liana reproductive activity has been increasing through time (2000–2014) in rainforests of the Australian wet tropics. The increased activity was associated with increases in temperatures, rainfall seasonality, and climatic disturbances such as El Niño events. The observed increase in liana fruiting was also coupled with a change in timing and contraction of fruiting-season duration. Such changes may mitigate any potential advantages for frugivore species associated with increased liana fecundity. These results suggest that liana reproduction, and hence abundance, is likely to increase in the Australian wet tropics region in response to future climate change, negatively impacting resident tree species and degrading forest structure.
Data Availability Statement
The raw data supporting the conclusions of this article will be made available by the authors, without undue reservation.
Author Contributions
NV conceived and wrote the manuscript with conceptual input from JE and ML, and editorial input from JE, MC, WL, and ML. TL collected the data. NV and JE prepared all figures. All authors contributed to the article and approved the submitted version.
Conflict of Interest
The authors declare that the research was conducted in the absence of any commercial or financial relationships that could be construed as a potential conflict of interest.
Publisher’s Note
All claims expressed in this article are solely those of the authors and do not necessarily represent those of their affiliated organizations, or those of the publisher, the editors and the reviewers. Any product that may be evaluated in this article, or claim that may be made by its manufacturer, is not guaranteed or endorsed by the publisher.
Acknowledgments
We acknowledge the traditional owners of the study region, and thank Skyrail Rainforest Cableway staff for their help and support with data collection.
Supplementary Material
The Supplementary Material for this article can be found online at: https://www.frontiersin.org/articles/10.3389/ffgc.2022.787950/full#supplementary-material
Footnotes
References
Babweteera, F., Plumptre, A. J., Adamescu, G. S., Shoo, L. P., Beale, C. M., Reynolds, V., et al. (2018). The ecology of tree reproduction in an African medium altitude rain forest. Biotropica. 50, 405–417. doi: 10.1111/btp.12563
BOM (n.d.). Climate Data Online: Kuranda Railway Station. Available online at: http://www.bom.gov.au/jsp/ncc/cdio/weatherData/av?p_nccObsCode=139&p_display_type=dataFile&p_startYear=&p_c=&p_stn_num=031036 (accessed November 09, 2021).
Boulter, S. L., Kitching, R. L., Gross, C. L., Goodall, K. L., and Howlett, B. G. (2009). “Floral morphology, phenology and pollination in the Wet tropics,” in Living in a Dynamic Tropical Forest Landscape, eds S. L. Turton and N. E. Stork (Oxford: Blackwell Publishing, Ltd), 224–239. doi: 10.1186/1999-3110-55-15
Cai, W., Borlace, S., Lengaigne, M., van Rensch, P., Collins, M., Vecchi, G., et al. (2014). Increasing frequency of extreme El Niño events due to greenhouse warming. Nat. Clim. Chang. 4, 111–116.
Cai, Z., Schnitzer, S. A., and Bongers, F. (2009). Seasonal differences in leaf-level physiology give lianas a competitive advantage over trees in a tropical seasonal forest. Oecologia 161, 25–33. doi: 10.1007/s00442-009-1355-4
Campbell, M., Magrach, A., and Laurance, W. F. (2015). “Liana diversity and the future of tropical forests,” in Biodiversity of Lianas, ed. N. Parthasarathy (Cham: Springer International Publishing), 255–274. doi: 10.1007/978-3-319-14592-1_13
Campbell, M. J., Edwards, W., Magrach, A., Alamgir, M., Porolak, G., Mohandass, D., et al. (2018). Edge disturbance drives liana abundance increase and alteration of liana–host tree interactions in tropical forest fragments. Ecol. Evol. 8, 4237–4251. doi: 10.1002/ece3.3959
Chapman, C. A., Valenta, K., Bonnell, T. R., Brown, K. A., and Chapman, L. J. (2018). Solar radiation and ENSO predict fruiting phenology patterns in a 15-year record from Kibale National Park, Uganda. Biotropica. 50, 384–395. doi: 10.1111/btp.12559
Cleland, E. E., Chuine, I., Menzel, A., Mooney, H. A., and Schwartz, M. D. (2007). Shifting plant phenology in response to global change. Trends Ecol. Evol. 22, 357–365. doi: 10.1016/j.tree.2007.04.003
Cochrane, M. A., and Laurance, W. F. (2008). Synergisms among fire, land use, and climate change in the Amazon. Ambio 37, 522–527. doi: 10.1579/0044-7447-37.7.522
Cortés-Flores, J., Cornejo-Tenorio, G., and Ibarra-Manríquez, G. (2015). Flowering phenology and pollination syndromes in species with different growth forms in a neotropical temperate forest of Mexico. Botany 93, 361–367. doi: 10.1139/cjb-2014-0218
Cunningham, S. A., Evans, M. J., Neave, M., Armstrong, J., and Barton, P. S. (2020). Pollination and resource limitation as interacting constraints on almond fruit set. Plant Biol. 22, 112–113. doi: 10.1111/plb.13045
Dale, V. H., Joyce, L. A., McNulty, S., Neilson, R. P., Ayres, M. P., Flannigan, M. D., et al. (2001). Climate change and forest disturbances: climate change can affect forests by altering the frequency, intensity, duration, and timing of fire, drought, introduced species, insect and pathogen outbreaks, hurricanes, windstorms, ice storms, or landslides. Bioscience 51, 723–734.
García León, M. M., Izquierdo, L. M., Mello, F. N. A., Powers, J. S., and Schnitzer, S. A. (2018). Lianas reduce community-level canopy tree reproduction in a Panamanian forest. J. Ecol. 106, 737–745. doi: 10.1111/1365-2745.12807
Granados, J., and Korner, C. (2002). In deep shade, elevated CO2 increases the vigor of tropical climbing plants. Glob. Chang. Biol. 8, 1109–1117. doi: 10.1046/j.1365-2486.2002.00533.x
Gray, R. E. J., and Ewers, R. M. (2021). Monitoring forest phenology in a changing world. Forests 12:297. doi: 10.3390/f12030297
Hector, A., Philipson, C., Saner, P., Chamagne, J., Dzulkifli, D., O’Brien, M., et al. (2011). The Sabah biodiversity experiment: a long-term test of the role of tree diversity in restoring tropical forest structure and functioning. Phil. Trans. R. Soc. B. 366, 3303–3315. doi: 10.1098/rstb.2011.0094
Hogan, J., Mayorquín, S., Rice, K., Thompson, J., Zimmerman, J., and Brokaw, N. (2017). Liana dynamics reflect land-use history and hurricane response in a Puerto Rican forest. J. Trop. Ecol. 33, 155–164. doi: 10.1017/s0266467417000049
Hudson, I. L., Kim, S. W., and Keatley, M. R. (2010). “Climatic influences on the flowering phenology of four eucalypts: a GAMLSS approach phenological research,” in Phenological Research, eds I. L. Hudson and M. R. Keatley (Dordrecht: Springer Netherlands Link).
Hughes, L. (2003). Climate change and Australia: trends, projections and impacts. Austral Ecol. 28, 423–443. doi: 10.1046/j.1442-9993.2003.01300.x
Hyndman, R., Athanasopoulos, G., Bergmeir, C., Caceres, G., Chhay, L., O’Hara-Wild, M., et al. (2021). Forecast: Forecasting Functions for Time Series and Linear Models. R Package Version 8.15. Available online at: https://pkg.robjhyndman.com/forecast/ (accessed November 09, 2021).
Ingwell, L. L., Joseph Wright, S., Becklund, K. K., Hubbell, S. P., and Schnitzer, S. A. (2010). The impact of lianas on 10 years of tree growth and mortality on Barro Colorado Island, Panama. J. Ecol. 98, 879–887. doi: 10.1111/j.1365-2745.2010.01676.x
IPCC (2021). Climate Change 2021: The Physical Science Basis. Contribution of Working Group I to the Sixth Assessment Report of the Intergovernmental Panel on Climate Change, eds V. Masson-Delmotte, P. Zhai, A. Pirani, S. L. Connors, C. Péan, S. Berger, et al. (Cambridge: Cambridge University Press).
Kainer, K. A., Wadt, L. H. O., Gomes-Silva, D. A. P., and Capanu, M. (2006). Liana loads and their association with Bertholletial excelsa fruit and nut production, diameter growth and crown attributes. J. Trop. Ecol. 22, 147–154. doi: 10.1017/s0266467405002981
Körner, C. (2009). Responses of humid tropical trees to rising CO2. Annu. Rev. Ecol. Evol. Syst. 40, 61–79. doi: 10.1073/pnas.1704811114
Laurance, W. F., Andrade, A. S., Magrach, A., Camargo, J. L. C., Valsko, J. J., Campbell, M., et al. (2014). Long-term changes in liana abundance and forest dynamics in undisturbed Amazonian forests. Ecology 95, 1604–1611. doi: 10.1890/13-1571.1
Laurance, W. F., Camargo, J. L. C., Luizao, R. C. C., Laurance, S. G., Pimm, S. L., Bruna, E. M., et al. (2011). The fate of Amazonian forest fragments: a 32-year investigation. Biol. Conserv. 144, 56–67. doi: 10.1016/j.biocon.2010.09.021
Laurance, W. F., and Curran, T. J. (2008). Impacts of wind disturbance on fragmented tropical forests: a review and synthesis. Austral Ecol. 33, 399–408. doi: 10.1111/j.1442-9993.2008.01895.x
Laurance, W. F., Lovejoy, T. E., Vasconcelos, H. L., Bruna, E. M., Didham, R. K., Stouffer, P. C., et al. (2002). Ecosystem decay of Amazonian Forest fragments: a 22-year investigation. Conserv. Biol. 16, 605–618. doi: 10.1046/j.1523-1739.2002.01025.x
Laurance, W. F., Nascimento, H. E. M., Laurance, S. G., Andrade, A. C., Fearnside, P. M., Ribeiro, J. E. L., et al. (2006). Rain forest fragmentation and the proliferation of successional trees. Ecology 87, 469–482. doi: 10.1890/05-0064
Laurance, W. F., Perez-Salicrup, D., Delamonica, P., Fearnside, P. M., D’Angelo, S., Jerozolinski, A., et al. (2001a). Rain forest fragmentation and the structure of Amazonian liana communities. Ecology 82, 105–116. doi: 10.1890/0012-9658(2001)082[0105:rffats]2.0.co;2
Laurance, W. F., Williamson, G. B., Delamonica, P., Oliveira, A., Lovejoy, T. E., Gascon, C., et al. (2001b). Effects of a strong drought on Amazonian forest fragments and edges. J. Trop. Ecol. 17, 771–785. doi: 10.1017/s0266467401001596
Ledo, A., and Schnitzer, S. A. (2014). Disturbance and clonal reproduction determine liana distribution and maintain liana diversity in a tropical forest. Ecology 95, 2169–2178. doi: 10.1890/13-1775.1
Lewis, S. L., Phillips, O. L., Baker, T. R., Lloyd, J., Malhi, Y., Almeida, S., et al. (2004). Concerted changes in tropical forest structure and dynamics: evidence from 50 South American long-term plots. Philos. Trans. R. Soc. Lond. B Biol. Sci. 359, 421–436. doi: 10.1098/rstb.2003.1431
Ley, A. C., and Claßen-Bockhoff, R. (2013). Breeding system and fruit set in African Marantaceae. Flora Morphol. Distrib. Funct. Ecol. Plants 208, 532–537. doi: 10.1016/j.flora.2013.07.011
Manzanedo, R. D., Ballesteros-Cánovas, J., Schenk, F., Stoffel, M., Fischer, M., and Allan, E. (2018). Increase in CO2 concentration could alter the response of Hedera helix to climate change. Ecol. Evol. 8, 8598–8606. doi: 10.1002/ece3.4388
Marshall, A. R., Platts, P. J., Chazdon, R. L., Seki, H., Campbell, M. J., Phillips, O. L., et al. (2020). Conceptualising the global forest response to liana proliferation. Front. For. Glob. Change. 3:35.
Marvin, D. C., Winter, K., Burnham, R. J., and Schnitzer, S. A. (2015). No evidence that elevated CO2 gives tropical lianas an advantage over tropical trees. Glob. Chang. Biol. 21, 2055–2069. doi: 10.1111/gcb.12820
Medina-Vega, J. A., Bongers, F., Poorter, L., Schnitzer, S. A., and Sterck, F. J. (2021). Lianas have more acquisitive traits than trees in a dry but not in a wet forest. J Ecol. 109, 2367–2384. doi: 10.1111/1365-2745.13644
Menzel, A., Sparks, T. H., Estrella, N., and Roy, D. B. (2006). Altered geographic and temporal variability in phenology in response to climate change. Glob. Ecol. Biogeogr. 15, 498–504. doi: 10.1111/j.1469-8137.2004.01003.x
Morellato, L. P. C., Alberti, L., and Hudson, I. L. (2010). “Applications of circular statistics in plant phenology: a case studies approach,” in Phenological Research, eds I. Hudson and M. Keatley (Dordrecht: Springer), 339–359. doi: 10.1007/978-90-481-3335-2_16
Morellato, L. P. C., Alberton, B., Alvarado, S. T., Borges, B., Buisson, E., Camargo, M. G. G., et al. (2016). Linking plant phenology to conservation biology. Biol. Conserv. 195, 60–72. doi: 10.1016/j.biocon.2015.12.033
Nakonechnaya, O., Koren, O., Sidorenko, V., Shabalin, S., Markova, T., and Kalachev, A. (2021). Poor fruit set due to lack of pollinators in Aristolochia manshuriensis (Aristolochiaceae). Plant Ecol. Evol. 154, 39–48. doi: 10.5091/plecevo.2021.1747
Odell, E. H., Stork, N. E., and Kitching, R. L. (2019). Lianas as a food resource for herbivorous insects: a comparison with trees. Biol. Rev. 94, 1416–1429. doi: 10.1111/brv.12508
Oliveira, A. T., deMello, J. M., and Scolforo, J. R. S. (1997). Effects of past disturbance and edges on tree community structure and dynamics within a fragment of tropical semideciduous forest in south-eastern Brazil over a five-year period (1987-1992). Plant Ecol. 131, 45–66. doi: 10.1023/a:1009744207641
Pau, S., Okamoto, D. K., Calderón, O., and Wright, S. J. (2018). Long-term increases in tropical flowering activity across growth forms in response to rising CO2 and climate change. Glob. Change Biol. 24, 2105–2116. doi: 10.1111/gcb.14004
Paul, G. S., and Yavitt, J. B. (2011). Tropical vine growth and the effects on forest succession: a review of the ecology and management of tropical climbing plants. Bot. Rev. 77, 11–30. doi: 10.1007/s12229-010-9059-3
Phillips, O., Vásquez Martínez, R., Arroyo, L., Baker, T., Killeen, T., Lewis, S., et al. (2002). Increasing dominance of large lianas in Amazonian forests. Nature 418, 770–774. doi: 10.1038/nature00926
Putz, F. E. (1984). The natural history of lianas on Barro-Colorado island, Panama. Ecology 65, 1713–1724. doi: 10.1890/12-1012.1
R Core Team (2019). R: A Language and Environment for Statistical Computing. Vienna: R Foundation for Statistical Computing.
Rathcke, B. J. (2000). Hurricane causes resource and pollination limitation of fruit set in a bird-pollinated shrub. Ecology 81, 1951–1958. doi: 10.1890/0012-9658(2000)081[1951:hcrapl]2.0.co;2
Rice, A. H., Pyle, E. H., Saleska, S. R., Hutyra, L., Palace, M., Keller, M., et al. (2004). Carbon balance and vegetation dynamics in an old-growth Amazonian forest. Ecol. Appl. 14, 55–71. doi: 10.1890/02-6006
Richardson, A. D., Keenan, T. F., Migliavacca, M., Ryu, Y., Sonnentag, O., and Toomey, M. (2013). Climate change, phenology, and phenological control of vegetation feedbacks to the climate system. Agric. For. Meteorol. 169, 156–173.
Schnitzer, S. A. (2005). A mechanistic explanation for global patterns of liana abundance and distribution. Am. Nat. 166, 262–276. doi: 10.1086/431250
Schnitzer, S. A., and Bongers, F. (2002). The ecology of lianas and their role in forests. Trends Ecol. Evol. 17, 223–230. doi: 10.1016/s0169-5347(02)02491-6
Schnitzer, S. A., and Bongers, F. (2011). Increasing liana abundance and biomass in tropical forests: emerging patterns and putative mechanisms. Ecol. Lett. 14, 397–406. doi: 10.1111/j.1461-0248.2011.01590.x
Schnitzer, S. A., and Carson, W. P. (2010). Lianas suppress tree regeneration and diversity in treefall gaps. Ecol. Lett. 13, 849–857. doi: 10.1111/j.1461-0248.2010.01480.x
Schnitzer, S. A., DeFilippis, D. M., Visser, M., Estrada-Villegas, S., Rivera-Camaña, R., Bernal, B., et al. (2021). Local canopy disturbance as an explanation for long-term increases in liana abundance. Ecol. Lett. 24, 2635–2647. doi: 10.1111/ele.13881
Schnitzer, S. A., and van der Heijden, G. M. F. (2019). Lianas have a seasonal growth advantage over co-occurring trees. Ecology 100:e02655. doi: 10.1002/ecy.2655
Schnitzer, S. A., van der Heijden, G., Mascaro, J., and Carson, W. P. (2014). Lianas in gaps reduce carbon accumulation in a tropical forest. Ecology 95, 3008–3017.
Swaine, M. D., and Grace, J. (2007). Lianas may be favoured by low rainfall: evidence from Ghana. Plant Ecol. 192, 271–276. doi: 10.1007/s11258-007-9319-4
Syktus, J., Trancoso, R., Ahrens, D., Toombs, N., and Wong, K. (2020). Queensland Future Climate Dashboard: Downscaled CMIP5 climate projections for Queensland. Available online at: https://www.longpaddock.qld.gov.au/qld-future-climate/ (accessed November 09, 2021).
Tang, J., Körner, C., Muraoka, H., Piao, S., Shen, M., Thackeray, S. J., et al. (2016). Emerging opportunities and challenges in phenology: a review. Ecosphere 7:e01436.
Ting, S., Hartley, S., and Burns, K. C. (2008). Global patterns in fruiting seasons. Glob. Ecol. Biogeogr. 17, 648–657. doi: 10.1111/j.1466-8238.2008.00408.x
Tracey, J. G. (1982). The Vegetation of the Humid Tropical Region of North Queensland. Melbourne, VIC: CSIRO.
Turton, S. M., and Stork, N. E. (2009). “Impacts of tropical cyclones on forests in the wet tropics of Australia,” in Living in a Dynamic Tropical Forest Landscape, eds N. E. Stork and S. M. Turton (Carlton, VIC: Blackwell Publishing, Ltd), 47–58. doi: 10.1016/j.scitotenv.2016.05.107
Turton, S. M. (2012). Securing landscape resilience to tropical cyclones in Australia’s Wet Tropics under a changing climate: lessons from Cyclones Larry (and Yasi). Geogr. Res. 50, 15–30.
Umaña, M. N., Forero-Montaña, J., Nytch, C. J., Thompson, J., Uriarte, M., Zimmerman, J. K., et al. (2019). Dry conditions and disturbance promote liana seedling survival and abundance. Ecology 100, 1–9. doi: 10.1002/ecy.2556
Wright, S., and Calderón, O. (2006). Seasonal, El Niño and longer term changes in flower and seed production in a moist tropical forest. Ecol. Lett. 9, 35–44.
Wright, S. J. (2005). Tropical forests in a changing environment. Trends Ecol. Evol. 20, 553–560. doi: 10.1016/j.tree.2005.07.009
Wright, S. J., and Calderón, O. (2018). Solar irradiance as the proximate cue for flowering in a tropical moist forest. Biotropica 50, 374–383. doi: 10.1111/btp.12522
Wright, S. J., Sun, I. F., Pickering, M., Fletcher, C. D., and Chen, Y. Y. (2015). Long-term changes in liana loads and tree dynamics in a Malaysian forest. Ecology 96, 2748–2757. doi: 10.1890/14-1985.1
Wuertz, D. (2020). timeSeries: Financial Time Series Objects (Rmetrics). R package version 3062.100.
Zar, J. H. (1996). “Circular distributions: descriptive statistics,” in Biostatistical Analysis, 519–611.
Zhu, S. D., and Cao, K. F. (2010). Contrasting cost-benefit strategy between lianas and trees in a tropical seasonal rain forest in southwestern China. Oecologia 163, 591–599. doi: 10.1007/s00442-010-1579-3
Zich, F. A., Hyland, B. P. M., Whiffin, T., and Kerrigan, R. A. (2018). Australian Tropical Rainforest Plants, 7th Edn. Available online at: https://apps.lucidcentral.org/rainforest/text/intro/index.html (accessed November 09, 2021).
Zich, F. A., Hyland, B. P. M., Whiffin, T., and Kerrigan, R. A. (2020). ‘Taxon Name’. Australian Tropical Rainforest Plants, 8th Edn. Available online at: https://apps.lucidcentral.org/rainforest/ (accessed November 09, 2021).
Keywords: flowering, fruiting, rainforest, ENSO (El Niño Southern Oscillation), forest disturbance, woody vines
Citation: Vogado NO, Engert JE, Linde TL, Campbell MJ, Laurance WF and Liddell MJ (2022) Climate Change Affects Reproductive Phenology in Lianas of Australia’s Wet Tropics. Front. For. Glob. Change 5:787950. doi: 10.3389/ffgc.2022.787950
Received: 01 October 2021; Accepted: 25 March 2022;
Published: 09 June 2022.
Edited by:
Geertje M. F. Van Der Heijden, University of Nottingham, United KingdomReviewed by:
Francis Edward Putz, University of Florida, United StatesRachael Gallagher, Western Sydney University, Australia
Copyright © 2022 Vogado, Engert, Linde, Campbell, Laurance and Liddell. This is an open-access article distributed under the terms of the Creative Commons Attribution License (CC BY). The use, distribution or reproduction in other forums is permitted, provided the original author(s) and the copyright owner(s) are credited and that the original publication in this journal is cited, in accordance with accepted academic practice. No use, distribution or reproduction is permitted which does not comply with these terms.
*Correspondence: Nara O. Vogado, dm9nYWRvLm5hcmFAZ21haWwuY29t