- 1Environmental Studies, Colby College, Waterville, ME, United States
- 2School of Biological Sciences, The University of Utah, Salt Lake City, UT, United States
- 3Department of Biological Sciences, University of Notre Dame, Notre Dame, IN, United States
- 4Department of Ecology, Evolution and Behavior, University of Minnesota, St. Paul, MN, United States
- 5Department of Plant and Microbial Biology, University of Minnesota, St. Paul, MN, United States
Lianas are thought to be increasing and altering tree growth and ecosystem productivity in tropical forests, but less research has focused on secondary or seasonally dry tropical forest. We report on an 11-year study of tree growth and liana presence from Guanacaste, Costa Rica, where we measured the diameter growth and liana presence on more than 1,700 trees in regenerating forest of different ages. We find that the proportion of trees without lianas is decreasing and the number of trees with lianas occupying more than 10% of tree’s crowns is increasing. We also find that lianas are affecting the diameter growth of trees. The 11-year average relative growth rates of trees with lianas in more than 10% of the tree’s crown are lower than the relative growth of trees with no lianas or lianas in less than 10% of their crown. Year-to-year, tree relative growth rate is related to annual precipitation and tree diameter. However, trees that were heavily infested with lianas (i.e., with lianas in more than 50% of their crowns) had lower relative growth and a weaker precipitation-growth relationship. This work underscores the value of long-term longitudinal data in secondary forest and adds critical data on dry forest liana abundance change.
Introduction
Lianas (woody vines) are an important component in forest ecosystems around the planet that can provide habitat and foster biodiversity, all while parasitizing trees (Putz, 1984b,1991; Gentry and Dodson, 1987; Clark and Clark, 1990). In recent decades, lianas appear to be increasing in tropical forests in abundance and biomass (Phillips et al., 2002; Yorke et al., 2013; Laurance et al., 2014). While reports of this increase are widespread, the cause for increasing liana abundance in particular and the general controls over liana distribution remain a matter of intense study (Muller-Landau and Pacala, 2020; Schnitzer et al., 2021). This increase likely has ecosystem consequences because lianas have long been known to affect the demographic rates their host trees (Clark and Clark, 1990; Putz, 1991). A decrease in growth and increase in mortality associated with increasin liana abundance has the potential to alter tropical forest carbon uptake and storage, although many aspects of these dynamics are unclear (van der Heijden et al., 2013).
A variety of intrinsic and extrinsic factors have been investigated to explain the increase in tropical forest liana abundance, though increasing evidence suggests that disturbances and the resulting post-disturbance forest structure may play an important role (Schnitzer et al., 2021). In general, lianas may be more resistant and resilient to common tropical disturbances than their host trees. The distinct physiology of lianas means that they can often capitalize on drought disturbances with typically high water-use efficiency and hydraulic conductivity relative to tree hosts and competitors (Cai et al., 2009; Chen et al., 2015; Smith-Martin et al., 2019; van der Sande et al., 2019). Marginal shifts in water availability due to increases in drought severity and frequency have been found to contribute to the rise in liana abundance particularly in the seasonal tropics where water can be more of a limiting factor than light (Schnitzer, 2005). Lianas are also poised to take advantage of gaps and or open patches created by anthropogenic disturbances, thus altering light availability and facilitating rapid and aggressive liana growth (Schnitzer and Bongers, 2011; Schnitzer et al., 2021). Lianas require trees for support and use small tree or other lianas as trellises to move toward the canopy, which could result in existing lianas facilitating further liana colonization (Putz, 1984b; Balfour and Bond, 1993; Campbell et al., 2018). Both climate and disturbance patterns (including anthropogenic and other disturbances) are changing in ways that promote environments that are more suited to thriving lianas.
There are different mechanisms through which lianas interact with host trees. Lianas are aggressive competitors that, in high numbers, can affect the growth, fecundity, and mortality patterns of trees (Putz, 1984a,1990; Schnitzer and Bongers, 2002; Reis et al., 2020). For example, some lianas colonize canopy tree crowns and block large portions of light from reaching their host’s leaves leading to lower growth rates (Clark and Clark, 1990; van der Heijden and Phillips, 2009). There is evidence of tree growth reductions associated with lianas (Putz, 1984b; Clark and Clark, 1990; Schnitzer and Bongers, 2002; Grauel and Putz, 2004) or increases in tree growth following liana removal (Estrada-Villegas and Schnitzer, 2018). Others have documented the complex causes of these reductions that may go beyond light interception and structural damage. For example, the below-ground resource acquisition of lianas can limit tree sapling growth even in environments with high light availability and in gaps lianas can slow or inhibit the growth of seedlings (Schnitzer and Carson, 2010; Pasquini et al., 2015).
Even though lianas appear to be more abundant in seasonally dry tropical forest (SDTF) compared to wet forest (Gentry, 1991; Schnitzer, 2005; DeWalt et al., 2015), we know less about SDTF liana dynamics compared to areas that receive more rainfall, particularly in secondary SDTF. On the one hand, SDTFs may have a climate and disturbance regime that is more hospitable to lianas relative to a seasonal wet forests (Schnitzer, 2005), and many liana species experience fewer growth reductions with seasonal drought compared to co-occurring trees (Smith-Martin et al., 2019). On the other hand, research on SDTF lianas has shown that they occupy a similar range of water use physiologies as host trees (Werden et al., 2018) and do not differ in hydraulic traits like hydraulic safety margin or water potential at 50% loss of hydraulic conductivity (Smith-Martin et al., 2022). Similarly, a recent large-scale liana removal experiment in a dry forest in Panama showed that many trees did not experience increased growth rates when lianas were cut, and some species even showed decreases in growth rates (Estrada-Villegas et al., 2021). These unusual results remain unexplained. But, Estrada-Villegas et al. (2021) suggest they may be the result of tree allocation of resources to roots, a lack of root competition between lianas and trees, an increase of solar radiation and soil drying after liana removal, or water limitation in dry forests outweighing the negative effects of liana presence. Resolving whether liana abundance is increasing in SDTF and whether this leads to decreased tree growth rates is a high priority as both changing disturbance and climatic regimes may favor trees over lianas in this biome.
In this 11-year study in Guanacaste, Costa Rica, we annually measured tree growth and recorded liana presence using a four-level ordinal scale in secondary seasonally dry tropical forest plots ranging from 5 to 60 years since agricultural abandonment (largely pasture with rare remnant trees). We used these longitudinal data to evaluate changes in liana presence and the potential for effects of lianas on tree growth and mortality. Specifically, we ask, (1) Do the number of trees with lianas change over time? (2) Does the degree liana crown presence change over time? (3) Does liana presence affect tree growth? (4) Does liana presence impact tree mortality?
Materials and Methods
Site Description
Our study was conducted in seasonally dry tropical forests (SDTF) of Guanacaste Province, Costa Rica. In 2008, 18 permanent research plots (0.1 ha each) were established in Área de Conservación Guanacaste, Sector Santa Rosa (Santa Rosa National Park) and Área de Conservación Arenal Tempisque (Palo Verde National Park; Becknell et al., 2021). Both of these parks are a patchwork of forests in different successional stages recovering from agricultural abandonment at different times over the past century (Powers et al., 2009). The mean annual rainfall is 1,575 mm in Santa Rosa (1980–2020) and 1,445 mm in Palo Verde (1995–2018)1,2. The majority of precipitation at both sites occurs between May and late November leading to a ∼6-month dry season with little or no rainfall. Our 18 20 m × 50 m (0.1 ha) plots were established to document forest succession. At the start of the study plots spanned an age range from 5 to 60 years since agricultural abandonment. Six sites were in forests initially dominated by a single oak species, Quercus oleoides (“evergreen oak”). The other twelve sites represented the more common diverse and majority deciduous SDTF in the lowlands of Santa Rosa and Palo Verde. These same plots have been described in several other studies of succession, ecosystem function, and drought disturbance (Powers and Peréz-Aviles, 2013; Schilling et al., 2016; Becknell et al., 2021).
Field Measurements
Steel dendrometer bands were affixed to all trees within the plot with DBH >10 cm starting in 2008. We then added dendrometers to trees >7 cm DBH in 2013. Dendrometers were read each year at the start of the dry season (typically in late December or early January). When dendrometers were read each year, visual observations of lianas were also made on an ordinal scale. We coded each tree into one of four liana index categories: 0: no lianas, 1: lianas present on the tree’s trunk and/or in <10% of the tree’s crown, 2: lianas present in 10–50% of a tree’s crown, and 3: lianas present in more than 50% of the tree’s crown. Dendrometer measurements and liana observations were made separately for each stem of multi-stem trees which divided below 1.3 m. To keep observations and measurements consistent, the same field manager was present for all censuses and trained new field workers on dendrometer measurements and liana index observations.
Data Analysis
To examine changes in liana abundance, we calculated the number and proportion of trees in each liana category for each year. Then we used simple linear regression on the proportion of trees in each category as a function of time. To examine the growth effects of lianas, we took two approaches. First, we calculated the study-long mean relative growth rate for all trees with more than 4 years in our record and compared this across liana presence categories. Because the liana presence categories changed for many trees over the course of the study, we averaged the liana index and rounded it to the nearest value. We also omitted trees with less than 4 years of observed growth (1,408 trees remained). Next, we compared the distributions of mean relative growth rates across liana index categories with the non-parametric Kruskal–Wallis test. Then, to compare growth effects of lianas among years and include annual rainfall and tree size we used a linear mixed-effects model to evaluate the effects of liana index on relative growth rates each year while including tree size and annual precipitation as fixed effects and individual tree identifier and tree species as random effects to account for repeated measures, and the likely important but unbalanced effect of different tree species. Because tree species differences were not the focus of this analysis, we included species as a random effect, which meant that individual intercept values were calculated for each species, but fixed effects were determined using all species. We also included two interaction terms: one between the liana index and tree diameter and the other between liana index and annual rainfall. Before running this analysis, we removed data where relative growth rates were more than 4 standard deviations from the mean as has been done in similar analyses (Visser et al., 2018). We also included interactions between liana index and annual rainfall as well as an interaction term between annual rainfall and tree size. Our model is as follows.
To examine tree mortality, we calculated the annual mortality rate (λ) for each year for trees in each of the four liana categories using the method explained by Sheil et al. (1995):
Where N0 is number of live trees at the start of the census period, Nt is the number of surviving trees at the end of the census period, and t time in years between observations. Then we compared these mortality rates across liana categories using the non-parametric Kruskal–Wallis test.
We also attempted to determine if our data showed evidence of trees shedding lianas. To do this, we compared the liana index for each tree at the start and end of their record in our study looking for decreases in the liana index. For each tree, we took the mean liana index in the first and second halves of our record. We then tallied which of those trees with a mean liana index greater than 1 in the first half of the record had a liana index decline of more than 0.5 in the mean liana index by the second half of the study. We used only the trees for which we had 4 or more consecutive years of observations. All analyses were conducted using the R statistical computing language (R Core Team, 2021). All data were pooled over the 18 plots for this analysis.
Results
Our data included annual growth measurements and liana observations on 1,745 unique stems with DBHs from 7 to 96 cm, representing 102 tree species from 86 genera. Of the 1,745 stems, 623 (35%) were observed in all 12 years. Another 493 were observed for 7 years due to the fact that in the first 5 years of the study we included only trees 10 cm DBH and above. In, 2013 we added dendrometers to trees between 7 and 10 cm DBH which were observed for the first time in 2014. Averaged across all years, 49% of trees in our study had no lianas, 16% of trees had lianas that occupied less than 10% of the tree’s crown, 18% of trees had lianas which occupied 10–50% of the tree’s crown, and 17% of trees had lianas which occupied more than 50% of a tree’s crown.
Excluding trees >7 cm DBH that were added to the survey in 2014, the proportion of trees in each liana presence category changed over time (Figure 1). The number of trees with no lianas declined over time from 52% in 2010 to 45% in 2017. Linear regression fit to this proportion by year suggests that the proportion of trees with no lianas declines at a rate of 1.3% per year (p = 0.0066, R2 = 0.53). The number of trees with a liana index of 2 or 3 (i.e., those with lianas occupying more than 10% of the tree’s crown) increased over time from 21% in 2010 to 41% in 2020 increasing at a rate of 2.1% per year (p = 0.0023, R2 = 0.62).
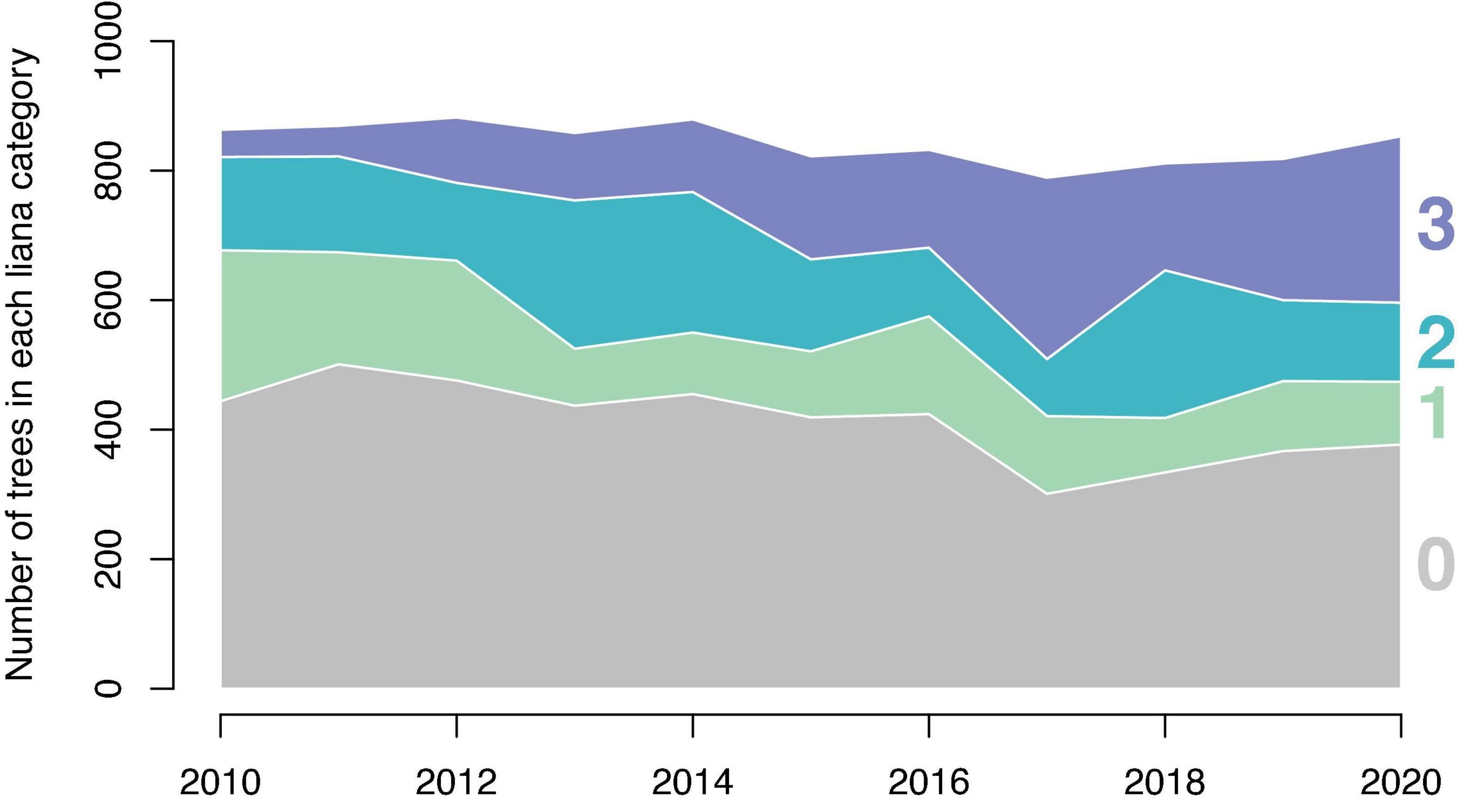
Figure 1. The change in the number of trees per liana presence category by year. The overall number of trees in the study changes little among years. The number of trees in categories 2 and 3 increase while the number of trees in categories 0 and 1 decrease over time. This analysis omits trees under 10 cm DBH which were first observed in 2014.
With respect to liana shedding, of the 490 stems with a mean liana index over 1 in the first half of the record (35% of stems with more than 4 years of observations), 13.7% (67) had a mean liana index that decreased by more than 0.5 in the second half of the study. We examined the liana index records of each of these 67 stems and found that the liana declines in 28 of these records could be explained by a likely mis-code in the liana index, i.e., a single high liana value toward the start of the record or a single low liana value toward the end of the record. Or, in several cases, a liana record that increased and decreased dramatically throughout the record, indicated a tree with a liana that was difficult to code. This analysis leaves 39 of 490 stems (8% of stems starting with high liana values) that show evidence of liana shedding over the length of our study. This suggests a liana shedding rate of 0.7% of trees shedding lianas per year. The 39 trees included four trees which died, but in all four cases, the death occurred two or more years after the liana index had gone to zero.
In our analysis of study-long average relative growth rates, we found that trees with liana index of 0 or 1 (no liana, or liana present in less than 10% of the tree’s crown) had higher mean relative growth rates than trees with liana index of 2 or more (i.e., lianas present in more than 10% of the crown; Figure 2; Kruskal–Wallis p-value = 3.563⋅10–08). The Bonferroni adjusted Dunn Q test showed that trees with liana index of 3 had significantly lower growth rates than trees with no lianas (p = 0.00042) and trees with liana index of 1 (p = 0.00074). Trees with liana index of 2 also had significantly lower relative growth rates compared to those without lianas (p = 0.000012) and trees with liana index of 1 (p = 0.000063). Relative growth rates did not differ between liana index of 0 and 1 nor did they differ between trees with liana index of 2 and 3. Median relative growth rates for trees with liana index of 0, 1, 2, and 3 were 0.0137, 0.0140, 0.00983, and 0.00735, respectively. Trees with lianas in more than 10% of trees’ crowns had 34% lower median relative growth rates compared to trees with no lianas and lianas occupying less than 10% of trees’ crowns.
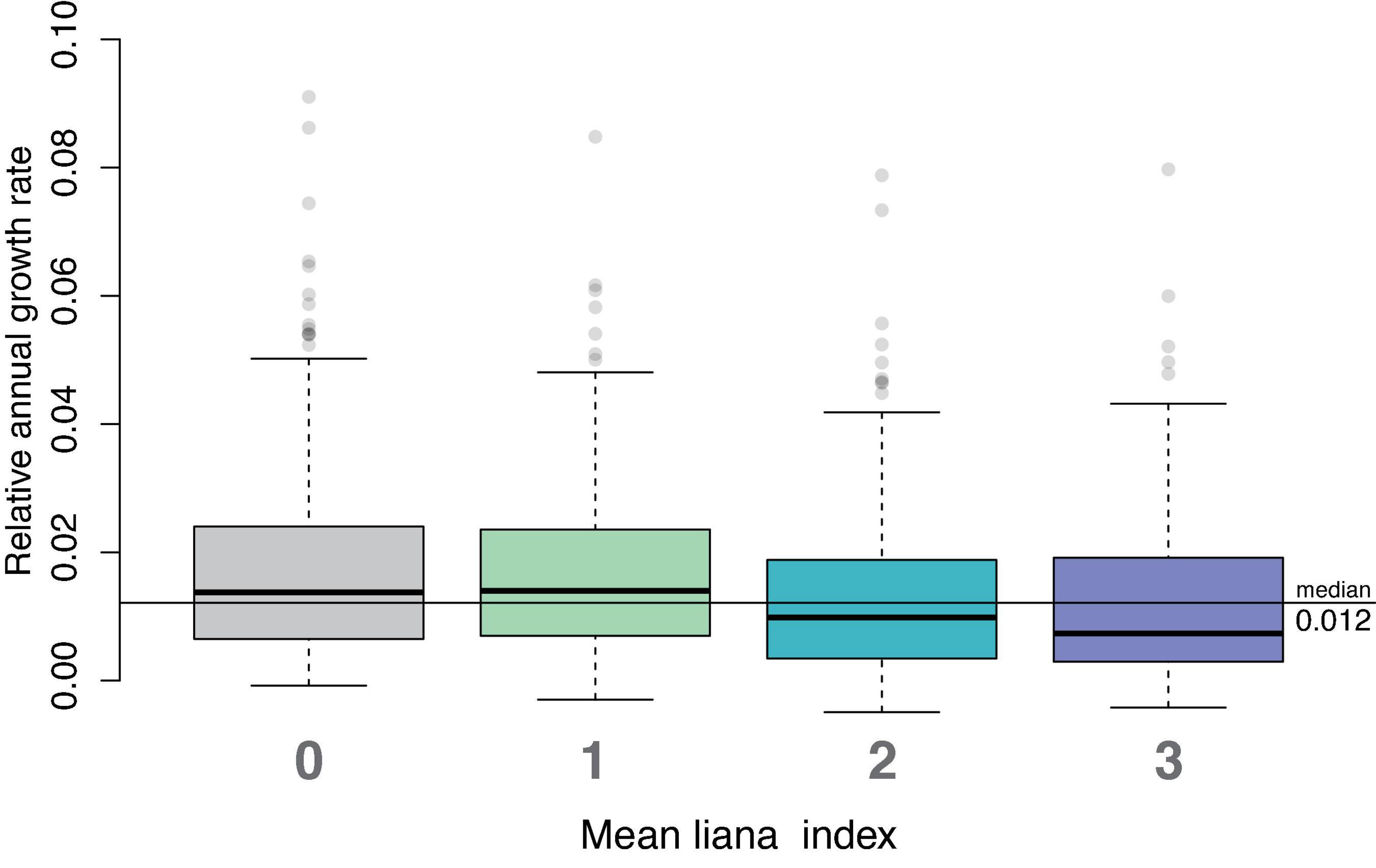
Figure 2. Overall median relative growth rates for trees with different average liana index over all study years. Trees with liana index of 0 and 1 had significantly higher relative growth than trees with lianas present in more than 10% of the tree’s crowns.
Next, we used a linear mixed-effects model to evaluate the effects of liana index on relative growth rates each year while including tree size, annual precipitation, as fixed effects and individual tree identifier and tree species as random effects to account for repeated measures and the likely important but imbalanced effect of different tree species (Table 1 and Figure 3). Relative growth was significantly higher for liana index of 1 and lower for liana index of 3. Both annual rainfall and tree diameter had significant effects on relative growth rate. There was an interaction between rainfall and liana index where the effect of rainfall on relative growth rate was lower for trees with a liana index of 3.
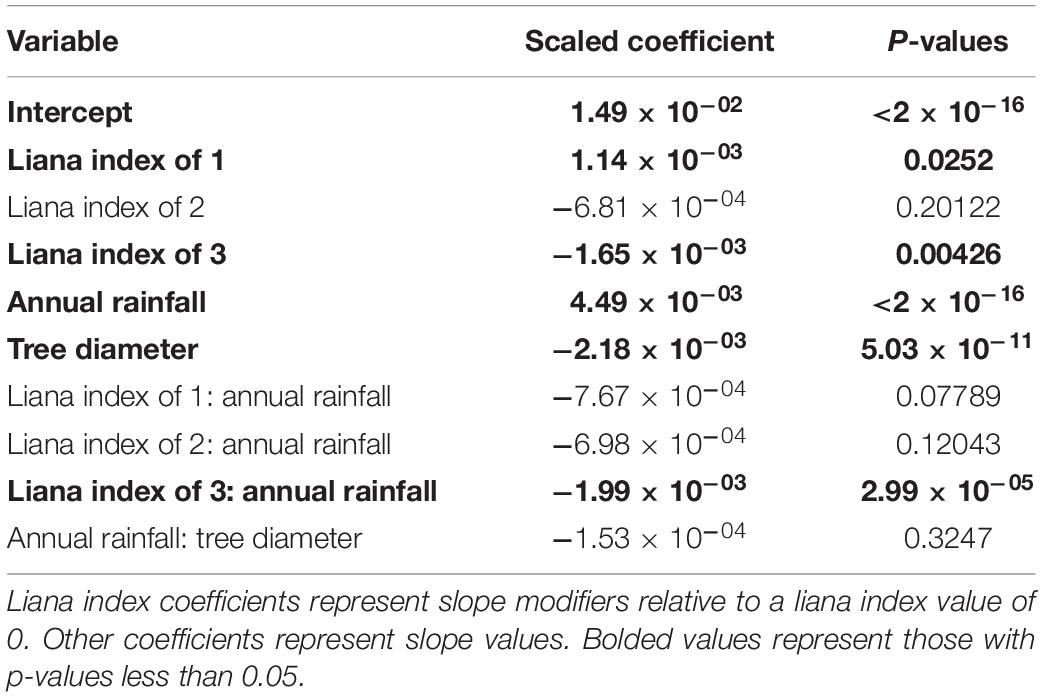
Table 1. Results from linear mixed effects model relating tree growth to liana index, annual rainfall, and tree diameter.
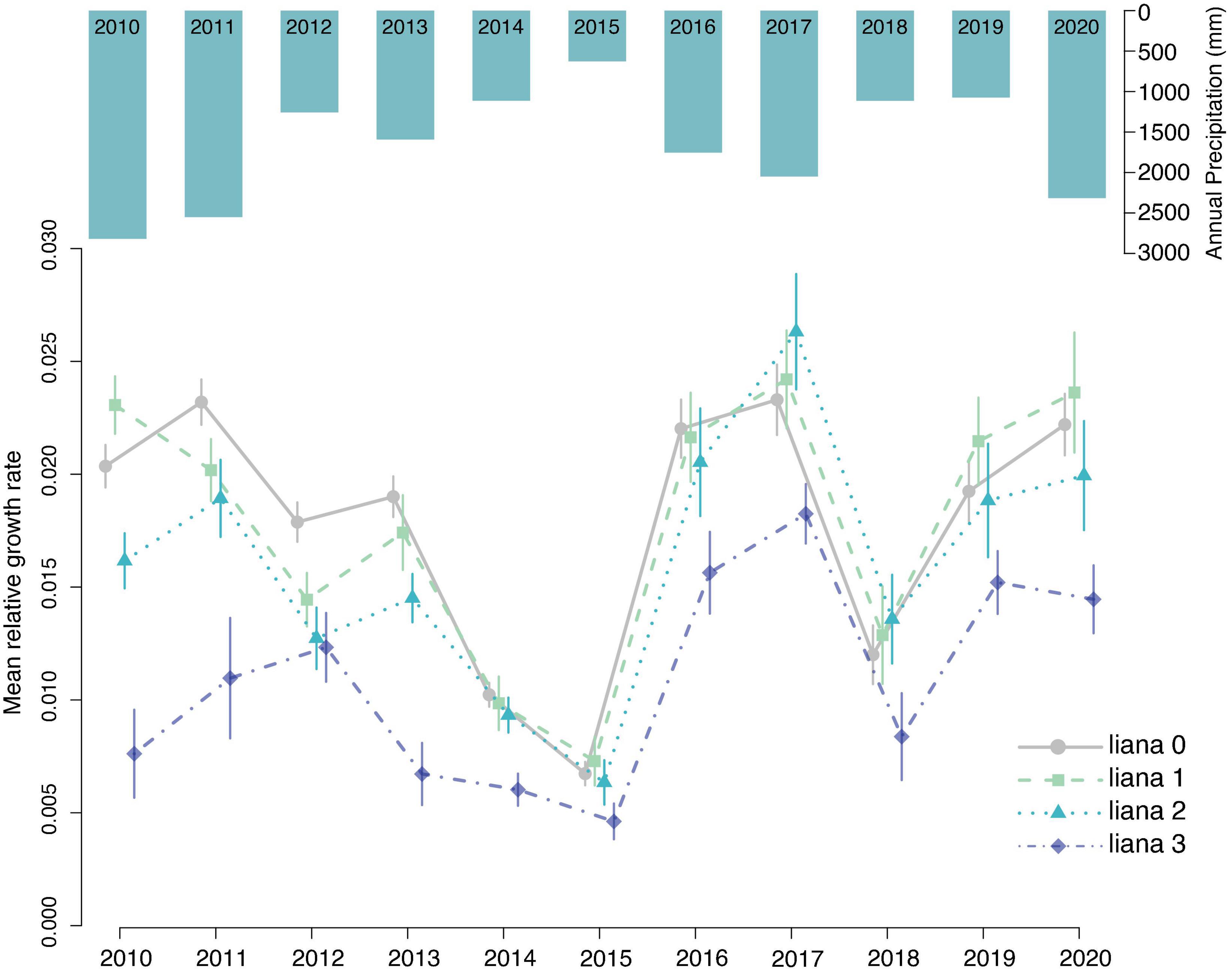
Figure 3. Annual mean relative growth rate for all trees in each liana category. Error bars represent standard error. Bars at the top show annual precipitation, which is positively correlated with annual growth. While growth in all categories appears to rise and fall with precipitation, trees in category 3 show significantly lower relative growth rates and growth in these trees responded less to annual precipitation.
We calculated the annual mortality rate of trees in each liana category that died each year. While the trend is for trees with liana values of 3 to have higher mortality rates (Figure 4), there was no significant difference among categories (Kruskal–Wallis p-value = 0.5211).
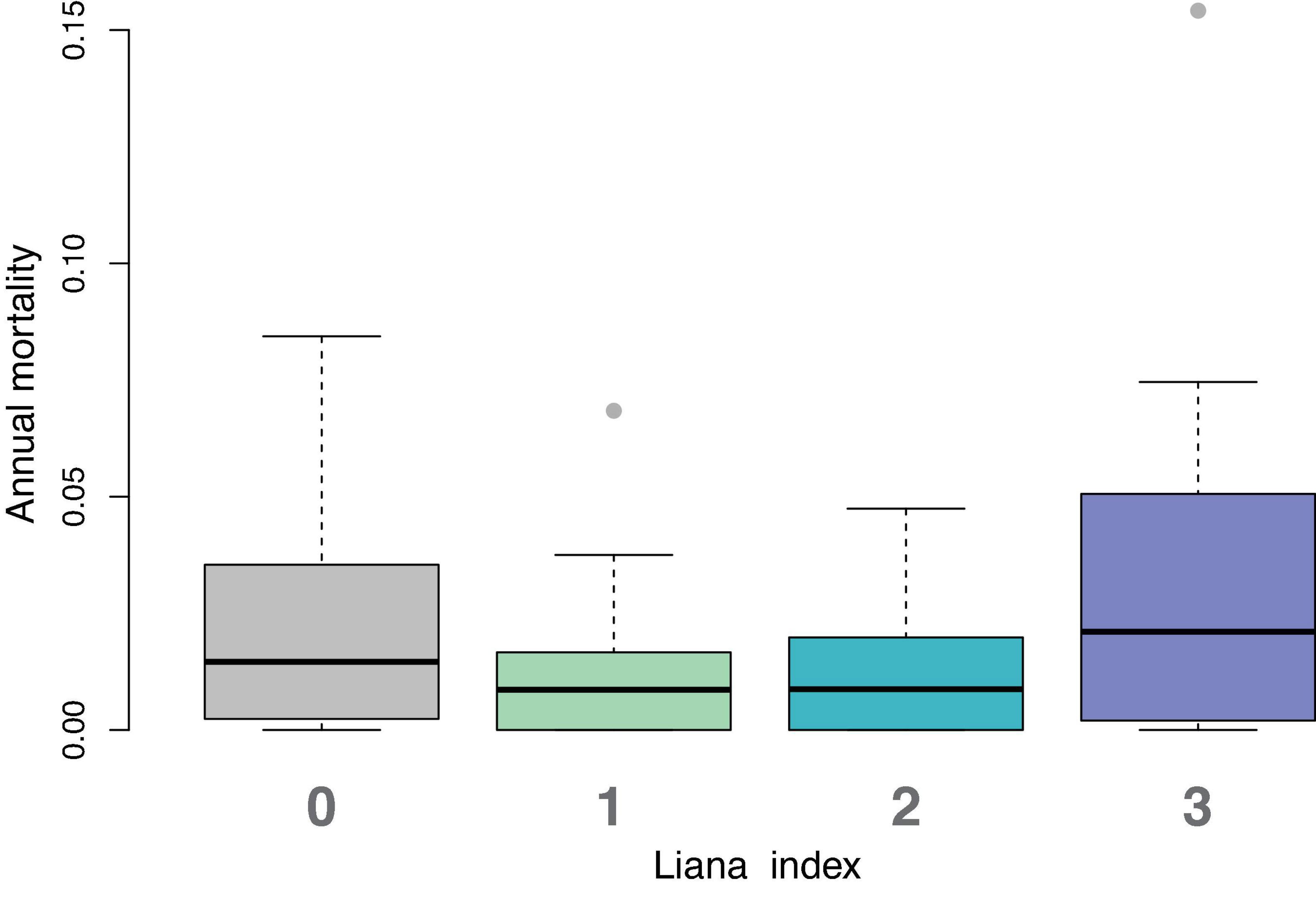
Figure 4. Distributions of annual mortality rate for each liana index value. Each box and whisker plot represents 11 points, one from each year. The median annual mortality rate for liana level 3 (lianas present in more than 50% of the tree’s crown) is highest. But non-parametric comparison of means test shows no significant differences among factors.
Discussion
Our 11-year study of lianas in Costa Rica provides evidence that lianas are increasing in abundance in a secondary seasonally dry tropical forest and that lianas negatively impact the growth of trees. This result represents one of a small number of studies on liana increase and growth effects in a seasonally dry forest. This result is also unique as it provides annually resolved records of the effects of liana index on growth in each year of our study. Many studies that census lianas and/or tree growth use less frequent intervals. One of our novel results was the interaction between liana presence index and annual rainfall; i.e., the liana-related reductions in annual growth rates were similar among liana presence classes in years with low rainfall, but varied more among liana presence classes during years of high rainfall. This liana-related suppression of growth over a decade of observations and the post-drought increase in lianas implies that lianas have a strong ongoing impact on ecosystem function and successional trajectories in these secondary SDTFs.
The number of trees with lianas increased over time in our study as did the number of trees in each liana index category, with more trees severely impacted by lianas by the end of the study. More than half of the trees in our study had lianas present which, on an annual basis increased, to 55% by the end of our study. This liana increase was evident in all 18 study plots, which included very early secondary successional sites (as young as 5 years at the start of the study) as well as plots with mid to late successional secondary forest (70 years by the end of the study). While some liana increase was evident early in the study, the liana index of trees increased markedly after the 2015 drought and its associated tree mortality (Powers et al., 2020). Whether these increases in lianas are a result of successional processes, drought and other gap-scale disturbances, or some other factor is difficult to determine.
Understanding the degree to which host trees can shed lianas is an important parameter for efforts to incorporate lianas into simulation models. Our data show some evidence of liana shedding among a small percentage of trees. Approximately 8% of trees starting with high liana values showed decreases in liana index over the course of our study which amounts to less than 1% of trees shedding lianas per year. This 8% represents a small number of trees and it is difficult to determine the degree to which lianas are actually “shed” using our data. Some tree species have been observed to be free of lianas while others are able to shed lianas (Putz, 1984a). It is not clear from our work whether this regularly occurs at our site or if particular species are common liana shedders. The most represented species in our list of trees that appear to shed lianas are the most common species in our dataset and the number of shedding trees we found was too low to allow further analysis of species.
Liana increases have been reported in many tropical forest regions over the past decades (Phillips et al., 2002; Wright et al., 2004; Schnitzer and Bongers, 2011), but the mechanism behind this increase remains a topic of research. Several important factors may be combining to facilitate liana expansion. These include past disturbances or ongoing minor disturbances and gap dynamics as well as extrinsic factors like CO2 fertilization, climate warming, or nutrient status (Schnitzer et al., 2011, 2020, 2021). In disturbed, fragmenting, or regenerating forests, the factors leading to liana increases likely include the structure of these forests including small trees and lianas acting as trellises and facilitating further liana colonization and growth (Putz, 1984b; Balfour and Bond, 1993; Campbell et al., 2018). Declines in liana abundance with succession have also been observed (Dewalt et al., 2000). While succession and disturbance are likely important factors at our study site, we cannot eliminate other possible drivers of liana increase. All of our sites are recovering from agricultural abandonment to differing degrees. Further, halfway through our study was a major drought leading to unusually high tree mortality (Powers et al., 2020) and followed by a large uptick in liana abundance. A continuation of liana increase combined with more frequent droughts could severely impact the productivity, carbon uptake, and carbon storage of this region.
Liana increases have also been documented in other tropical dry forest regions. A decade-long survey of lianas in Indian tropical dry forests shows liana increases in some but not all study sites (Pandian and Parthasarathy, 2003). Moist seasonal forests of the Americas show liana increases in several studies (Ingwell et al., 2010; Schnitzer and Bongers, 2011), and there is a general pattern toward higher liana density with lower precipitation (Parolari et al., 2020). But whether or not there is widespread liana increase in dry forests has not been sufficiently studied. Further, if this liana increase is common in dry forests, it’s not clear which mechanisms might be at play. But the likely explanation would seem to be the same successional and disturbance response patterns observed in liana increases in moist and wet forests (Schnitzer et al., 2021).
Our data firmly establish that tree growth rates are being negatively impacted by high liana crown presence at our study site. From our study-long average of growth rates, lianas slowed growth by 34% in trees with lianas present in more than 10% of the tree’s crown. When considering years separately and including the effects of tree size and annual rainfall, only the trees with lianas in more than 50% of their crowns appear to have reduced growth. This difference in result between study-long average versus annual growth suggests that while high liana presence (lianas in >50% of crown) reduces growth in all years, liana index 2 (lianas in 10–50% of crown) only reduced growth in some years. This could be because liana index 2 is a category that includes much more variation in the degree to which the liana is affecting the tree. Or this pattern may represent a real but unnoticed ecological or physiological effects such as differences among liana species in crown presence or effects on tree growth. Trees with lianas in 20–50% of their crowns had higher growth rates in 2017 and 2018, 2 years following a major drought which led to higher than normal tree mortality. Perhaps this was driven by trees whose growth was “released” by the death of adjacent liana-laden trees. These surviving neighbors may have been able to increase their growth as canopy gaps opened despite their moderate liana loads. Despite these differences in growth effects with analytical approach, it is clear that lianas are reducing growth in many trees in our study area. This reduced growth effect is persistent in wet and dry years and across our sites of varied age and tree species composition.
These growth effects are mirrored by the patterns we can observe in our mortality data. However, our small sample size limits our ability to show clear differences in mortality among liana categories. Despite this, the pattern we can observe in our data combined with evidence from other studies of liana-driven mortality leads us to conclude that lianas are impacting mortality here. Expanding our study to include more trees and continuing it for more years will likely make this pattern clearer.
Negative effects of lianas on tree growth have been documented in a variety of secondary and old growth forests, but early successional forests are less studied (Estrada-Villegas and Schnitzer, 2018). A recent secondary SDTF liana removal experiment showed no impact of lianas (Estrada-Villegas et al., 2021). In contrast, our results that lianas do indeed reduce growth directly contradict this work. Taken together, these results suggest a complicated picture of liana impacts on tree growth in secondary SDTF. Both sites had similar climate, similar land-use history, and both sites included historically low precipitation in 2015 (Estrada-Villegas et al., 2021). Estrada-Villegas et al. (2021) have more very early successional sites, suggesting that growth in mid-successional forests may be more affected by lianas. Other possible explanations for this difference in result include, species differences, past land-use history, disturbance history, or edaphic differences.
We acknowledge that a liana index resulting from a rapid visual assessment and can produce inconsistent data. We have minimized inconsistency by using the same field personnel throughout our study. That said, ground-based assessment of the amount of crown area taken up by lianas is difficult leading to both over and underestimates in liana index. Though, there is no evidence that these errors should be greater in the direction of over or under estimating lianas. This analysis excludes consideration of tree species or liana species both of which are likely important factors in explaining liana abundance changes and their impacts on tree growth. Tree species differentially respond to liana presence (Visser et al., 2018) and liana species have different effects on trees due to differences in morphology and life-history traits (Smith-Martin et al., 2020). We also acknowledge that assessing growth impacts of lianas using diameter growth alone leaves out important aspects of tree growth.
These findings have implications for understanding seasonally dry tropical forest function, restoration, and disturbance dynamics. More than half of trees had lianas and 35% of trees had levels of liana presence that reduced diameter growth. These trees were competitively disadvantaged and had reduced carbon uptake. Assuming these sites accurately represent liana levels across the landscape in this region, regional carbon uptake could be impacted by lianas.
Now that liana increases and tree growth impacts have been established in this landscape, further research is needed to fully understand this phenomenon’s impact on regional ecology and ecosystem function. Surveys of liana biomass or liana removal experiments may help to understand their impact on ecosystem carbon cycling more accurately (Laurance et al., 2014; Estrada-Villegas and Schnitzer, 2018). Studies of liana morphology and physiology will help to unravel the mechanisms behind these phenomena (Smith-Martin et al., 2020; Meunier et al., 2021). Continued longitudinal studies that include more detailed liana data may help to illustrate the mechanisms behind the liana increase. Remote sensing and modeling work are needed to extend this work beyond what is possible in the field (di Porcia e Brugnera et al., 2019). Comparative work on different forest types, across soil gradients, elevation gradients, and rainfall gradients may help to discern where in the landscape liana expansion is occurring and where those lianas impact growth. Along with studies in other systems these extensions of liana research can help explain the ecological role of lianas secondary SDTFs.
In summary, this work provides two key results: (1) lianas are increasing in abundance in secondary SDTFs we studied, and (2) these lianas have clear, negative effects on the growth of trees. This work demonstrates liana increases in secondary SDTF and adds support to other findings that lianas negatively impact tree growth in this forest type. Our observed liana increases may be explained by a combination of successional patterns, forest structural patterns, and tree mortality disturbance relating to the drought in the middle of our study. However, more work is needed to confirm this hypothesis and uncover the mechanisms behind liana impacts on growth, and whether these relationships are tree and liana-species specific.
Data Availability Statement
The raw data supporting the conclusions of this article will be made available by the authors, without undue reservation.
Author Contributions
JB conceived of the study, conducted initial field work, analyzed the data, and wrote the manuscript. GV collected field data, advised on the analysis, and edited the manuscript. LW and N-FW entered data, analyzed the data, and helped to write the manuscript. DM advised on the study and edited the manuscript. JP conceived of the study, conducted field work, and edited the manuscript. All authors contributed to the article and approved the submitted version.
Conflict of Interest
The authors declare that the research was conducted in the absence of any commercial or financial relationships that could be construed as a potential conflict of interest.
Publisher’s Note
All claims expressed in this article are solely those of the authors and do not necessarily represent those of their affiliated organizations, or those of the publisher, the editors and the reviewers. Any product that may be evaluated in this article, or claim that may be made by its manufacturer, is not guaranteed or endorsed by the publisher.
Acknowledgments
We thank Department of Energy Terrestrial Ecosystem Science Program grants DE-SC0014363 and DE-SC0020344 and National Science Foundation Grant DEB-1053237 for funding. We also thank Daniel Pérez-Aviles, Erick Schilling, and Damaris Pereira for help in the field. We thank the staff of Area de Conservacioìn Guanacaste, the staff of Palo Verde, and the Palo Verde OTS research station for facilitating this work.
Footnotes
References
Balfour, D. A., and Bond, W. J. (1993). Factors limiting climber distribution and abundance in a southern african. Source: J. Ecol. 81, 93–100. doi: 10.2307/2261227
Becknell, J. M., Vargas, G., Pérez-Aviles, D., Medvigy, D., and Powers, J. S. (2021). Above-ground net primary productivity in regenerating seasonally dry tropical forest: contributions of rainfall, forest age and soil. J. Ecol. 109, 3903–3915. doi: 10.1111/1365-2745.13767
Cai, Z. Q., Schnitzer, S. A., and Bongers, F. (2009). Seasonal differences in leaf-level physiology give lianas a competitive advantage over trees in a tropical seasonal forest. Oecologia 161, 25–33. doi: 10.1007/s00442-009-1355-4
Campbell, M. J., Edwards, W., Magrach, A., Alamgir, M., Porolak, G., Mohandass, D., et al. (2018). Edge disturbance drives liana abundance increase and alteration of liana-host tree interactions in tropical forest fragments. Ecol. Evol. 8, 4237–4251. doi: 10.1002/ECE3.3959
Chen, Y. J., Cao, K. F., Schnitzer, S. A., Fan, Z. X., Zhang, J. L., and Bongers, F. (2015). Water-use advantage for lianas over trees in tropical seasonal forests. New Phytol. 205, 128–136. doi: 10.1111/NPH.13036
Clark, D. B., and Clark, D. A. (1990). Distribution and effects on tree growth of lianas and woody hemiepiphytes in a Costa Rican tropical wet forest. J. Tropical Ecol. 6, 321–331. doi: 10.1017/S0266467400004570
DeWalt, S. J., Schnitzer, S. A., Alves, L. F., Bongers, F., Burnham, R. J., Cai, Z., et al. (2015). “Biogeographical patterns of liana abundance and diversity,” in Ecology of Lianas, eds S. Schnitzer, F. Bongers, R. J. Burnham, and F. E. Putz (West Sussex, SXW: John Wiley & Sons), 131–146. doi: 10.1111/brv.12508
Dewalt, S. J., Schnitzer, S. A., and Denslow, J. S. (2000). Density and diversity of lianas along a chronosequence in a central Panamanian lowland forest. J. Trop. Ecol. 16, 1–19. doi: 10.1017/S0266467400001231
di Porcia e Brugnera, M., Meunier, F., Longo, M., Krishna Moorthy, S. M., de Deurwaerder, H., Schnitzer, S. A., et al. (2019). Modeling the impact of liana infestation on the demography and carbon cycle of tropical forests. Global Change Biol. 25, 3767–3780. doi: 10.1111/GCB.14769
Estrada-Villegas, S., and Schnitzer, S. A. (2018). A comprehensive synthesis of liana removal experiments in tropical forests. Biotropica 50, 729–739. doi: 10.1111/BTP.12571
Estrada-Villegas, S., Hall, J. S., van Breugel, M., and Schnitzer, S. A. (2021). Lianas do not reduce tree biomass accumulation in young successional tropical dry forests. Oecologia 195, 1019–1029. doi: 10.1007/s00442-021-04877-z
Gentry, A. H. (1991). “Chapter 1: The distribution and evolution of climbing plants” in The Biology of Vines, eds F. E. Putz and H. A. Mooney (Cambridge: Cambridge University Press), 3–50. doi: 10.1017/CBO9780511897658.003
Gentry, A. H., and Dodson, C. (1987). Contribution of nontrees to species richness of a tropical rain forest. Biotropica 19, 149–156. doi: 10.2307/2388737
Grauel, W. T., and Putz, F. E. (2004). Effects of lianas on growth and regeneration of Prioria copaifera in Darien, Panama. Forest Ecol. Manag. 190, 99–108. doi: 10.1016/J.FORECO.2003.10.009
Ingwell, L. L., Joseph Wright, S., Becklund, K. K., Hubbell, S. P., and Schnitzer, S. A. (2010). The impact of lianas on 10 years of tree growth and mortality on Barro Colorado Island, Panama. J. Ecol. 98, 879–887. doi: 10.1111/j.1365-2745.2010.01676.x
Laurance, W. F., Andrade, A. S., Magrach, A., Camargo, J. L. C., Valsko, J. J., Campbell, M., et al. (2014). Long-term changes in liana abundance and forest dynamics in undisturbed Amazonian forests. Ecology 95, 1604–1611. doi: 10.1890/13-1571.1
Meunier, F., Verbeeck, H., Cowdery, B., Schnitzer, S. A., Smith-Martin, C. M., Powers, J. S., et al. (2021). Unraveling the relative role of light and water competition between lianas and trees in tropical forests: a vegetation model analysis. J. Ecol. 109, 519–540. doi: 10.1111/1365-2745.13540
Muller-Landau, H. C., and Pacala, S. W. (2020). “What determines the abundance of lianas and vines?,” in Unsolved Problems in Ecology, eds A. Dobson, D. Tilman, and R. D. Holt (Princeton, NJ: Princeton University Press).
Pandian, E., and Parthasarathy, N. (2003). Decadal (2003-2013) changes in liana diversity, abundance and aboveground biomass in four inland tropical dry evergreen forest sites of peninsular India. J. Forestry Res. 27, 133–146. doi: 10.1007/s11676-015-0146-145
Parolari, A. J., Paul, K., Griffing, A., Condit, R., Perez, R., Aguilar, S., et al. (2020). Liana abundance and diversity increase with rainfall seasonality along a precipitation gradient in Panama. Ecography 43, 25–33. doi: 10.1111/ECOG.04678
Pasquini, S. C., Wright, S. J., Santiago, L. S., and Uriarte, M. (2015). Lianas always outperform tree seedlings regardless of soil nutrients: results from a long-term fertilization experiment. Ecology 96, 1866–1876. doi: 10.1890/14-1660.1
Phillips, O. L., Vésquez Martínez, R., Arroyo, L., Baker, T. R., Killeen, T., Lewis, S. L., et al. (2002). Increasing dominance of large lianas in Amazonian forests. Nature 418, 770–774. doi: 10.1038/nature00926
Powers, J. S., and Peréz-Aviles, D. (2013). Edaphic factors are a more important control on surface fine roots than stand age in secondary tropical dry forests. Biotropica 45, 1–9. doi: 10.1111/J.1744-7429.2012.00881.X
Powers, J. S., Becknell, J. M., Irving, J., and Pèrez-Aviles, D. (2009). Diversity and structure of regenerating tropical dry forests in Costa Rica: geographic patterns and environmental drivers. Forest Ecol. Manag. 258, 959–970. doi: 10.1016/j.foreco.2008.10.036
Powers, J. S., Vargas, G., Brodribb, T. J., Schwartz, N. B., Pérez-Aviles, D., et al. (2020). A catastrophic tropical drought kills hydraulically vulnerable tree species. Global Change Biol. 26, 3122–3133. doi: 10.1111/GCB.15037
Putz, F. E. (1990). Liana stem diameter growth and mortality rates on Barro Colorado Island. Panama. Biotropica 22:103. doi: 10.2307/2388725
Putz, F. E. (1991). “Silvicultural effects of lianas,” in Biology of vines, eds F. E. Putz and H. A. Mooney (Cambridge: Cambridge University Press), 493–501. doi: 10.1017/cbo9780511897658.020
R Core Team (2021). R: A Language and Environment for Statistical Computing. Vienna: R Foundation for Statistical Computing.
Reis, S. M., Marimon, B. S., Morandi, P. S., Elias, F., Esquivel-Muelbert, A., Marimon Junior, B. H., et al. (2020). Causes and consequences of liana infestation in southern Amazonia. J. Ecol. 108, 2184–2197. doi: 10.1111/1365-2745.13470
Schilling, E. M., Waring, B. G., Schilling, J. S., and Powers, J. S. (2016). Forest composition modifies litter dynamics and decomposition in regenerating tropical dry forest. Oecologia 182, 287–297. doi: 10.1007/s00442-016-3662-x
Schnitzer, S. A. (2005). A mechanistic explanation for global patterns of liana abundance and distribution. Ame. Natural. 166, 262–276. doi: 10.1086/431250
Schnitzer, S. A., and Bongers, F. (2002). The ecology of lianas and their role in forests. Trends Ecol. Evol. 17, 223–230. doi: 10.1016/s0169-5347(02)02491-6
Schnitzer, S. A., and Bongers, F. (2011). Increasing liana abundance and biomass in tropical forests: emerging patterns and putative mechanisms. Ecol. Lett. 14, 397–406. doi: 10.1111/j.1461-0248.2011.01590.x
Schnitzer, S. A., and Carson, W. P. (2010). Lianas suppress tree regeneration and diversity in treefall gaps. Ecol. Lett. 13, 849–857. doi: 10.1111/J.1461-0248.2010.01480.X
Schnitzer, S. A., Bongers, F., and Wright, S. J. (2011). Community and ecosystem ramifications of increasing lianas in neotropical forests. Plant Signal Behav. 6, 598–600. doi: 10.4161/PSB.6.4.15373
Schnitzer, S. A., DeFilippis, D. M., Visser, M., Estrada-Villegas, S., Rivera-Camaña, R., Bernal, B., et al. (2021). Local canopy disturbance as an explanation for long-term increases in liana abundance. Ecol. Lett. 24, 2635–2647. doi: 10.1111/ele.13881
Schnitzer, S. A., Estrada-Villegas, S., and Wright, S. J. (2020). The response of lianas to 20 yr of nutrient addition in a Panamanian forest. Ecology 101:e03190. doi: 10.1002/ECY.3190
Sheil, D., Burslem, D. F. R. P., and Alder, D. (1995). The interpretation and misinterpretation of mortality rate measures. J. Ecol. 83, 331–333. doi: 10.2307/2261571
Smith-Martin, C. M., Bastos, C. L., Lopez, O. R., Powers, J. S., and Schnitzer, S. A. (2019). Effects of dry-season irrigation on leaf physiology and biomass allocation in tropical lianas and trees. Ecology 100:e02827. doi: 10.1002/ECY.2827
Smith-Martin, C. M., Jansen, S., Brodribb, T. J., Medina-Vega, J. A., Lucani, C., Huppenberger, A., et al. (2022). Lianas and trees from a seasonally dry and a wet tropical forest did not differ in embolism resistance but did differ in xylem anatomical traits in the dry forest. Front. For. Glob. Change 5:834891. doi: 10.3389/ffgc.2022.834891
Smith-Martin, C. M., Xu, X., Medvigy, D., Schnitzer, S. A., and Powers, J. S. (2020). Allometric scaling laws linking biomass and rooting depth vary across ontogeny and functional groups in tropical dry forest lianas and trees. New Phytol. 226, 714–726. doi: 10.1111/NPH.16275
van der Heijden, G. M. F., and Phillips, O. L. (2009). Liana infestation impacts tree growth in a lowland tropical moist Forest. Biogeosciences 6, 2217–2226. doi: 10.5194/BG-6-2217-2009
van der Heijden, G. M., Schnitzer, S. A., Powers, J. S., and Phillips, O. L. (2013). Liana impacts on carbon cycling, storage and sequestration in tropical forests. Biotropica 45, 682–692. doi: 10.1111/btp.12060
van der Sande, M. T., Poorter, L., Schnitzer, S. A., Engelbrecht, B. M. J., and Markesteijn, L. (2019). The hydraulic efficiency-safety trade-off differs between lianas and trees. Ecology 100:e02666. doi: 10.1002/ECY.2666
Visser, M. D., Schnitzer, S. A., Muller-Landau, H. C., Jongejans, E., de Kroon, H., Comita, L. S., et al. (2018). Tree species vary widely in their tolerance for liana infestation: a case study of differential host response to generalist parasites. J. Ecol. 106, 781–794. doi: 10.1111/1365-2745.12815
Werden, L. K., Waring, B. G., Smith-Martin, C. M., and Powers, J. S. (2018). Tropical dry forest trees and lianas differ in leaf economic spectrum traits but have overlapping water-use strategies. Tree Physiol. 38, 517–530. doi: 10.1093/treephys/tpx135
Wright, S. J., Calderón, O., Hernandéz, A., and Paton, S. (2004). Are lianas increasing in importance in tropical forests? A 17-year record from panama. Ecology 85, 484–489. doi: 10.1890/02-0757
Keywords: liana abundance, seasonally dry tropical forest, tree growth, secondary forest, dendrometers
Citation: Becknell JM, Vargas G G, Wright LA, Woods NF, Medvigy D and Powers JS (2022) Increasing Liana Abundance and Associated Reductions in Tree Growth in Secondary Seasonally Dry Tropical Forest. Front. For. Glob. Change 5:838357. doi: 10.3389/ffgc.2022.838357
Received: 17 December 2021; Accepted: 25 March 2022;
Published: 18 April 2022.
Edited by:
Geertje M. F. Van Der Heijden, University of Nottingham, United KingdomReviewed by:
Francis Edward Putz, University of Florida, United StatesRomà Ogaya, Centre for Ecological Research and Forestry Applications (CREAF), Spain
Copyright © 2022 Becknell, Vargas G, Wright, Woods, Medvigy and Powers. This is an open-access article distributed under the terms of the Creative Commons Attribution License (CC BY). The use, distribution or reproduction in other forums is permitted, provided the original author(s) and the copyright owner(s) are credited and that the original publication in this journal is cited, in accordance with accepted academic practice. No use, distribution or reproduction is permitted which does not comply with these terms.
*Correspondence: Justin M. Becknell, anVzdGluLmJlY2tuZWxsQGNvbGJ5LmVkdQ==