- 1Institute of Research in Biodiversity and Environment (National Scientific and Technical Research Council, National University of Comahue), Río Negro, Argentina
- 2University of Buenos Aires, Buenos Aires, Argentina
Wildland-urban interface (WUI) fires have increased in the last decades, putting lives and homes at risk, and fire hazard assessment is a useful tool to develop plans for prevention and fire management. In northwestern Patagonia, the WUI areas are principally located around the urbanized zones that are not only cities or towns but settlements surrounded by the natural environment. In Patagonia, there are the largest and most ancient national parks of Argentina with areas where former settlers develop their livestock activities. We assessed the fire hazard in the Laguna Blanca National Park (LBNP) located in Neuquén province (Patagonia, Argentina) dominated by steppe vegetation. We performed the study at two scales: community and species. Community scale comprised the variables vegetation cover, slope, and rock fragments, whereas species scale included flammability variables of dominant species (tussock grasses and shrubs) at leaf- and plant-level. We integrated all variables at different scales and grouped the vegetation units into three classes using multivariate analysis. Finally, we established three fire hazard categories for each vegetation unit: low, moderate, and high, to elaborate a fire hazard map. Three vegetation units, which represented 37% of the area of the park, were categorized with high fire hazard because of the high cover and horizontal continuity of dominant vegetation. The tussock grass Pappostipa speciosa and the shrubs Mulinum spinosum, Nassauvia axillaris, and Anarthorphyllum rigidum were the most flammable species and the most frequent species in the park. Land uses in the park (i.e., transhumance and tourism) would be regulated with the collaboration of settlers because increase the vulnerability to wildfires. Our fire hazard map constitutes a valuable tool because it identified the most vulnerable WUI in the LBNP. This study emphasizes the need to include flammability and fuel load studies in fire management plans to better protect human lives and natural resources in protected areas.
Introduction
Wildland-urban interface (WUI) areas are present in the protected areas of Argentina because in the park sectors that have low category of conservation (reserve) there are settlements and infrastructures (buildings related to park administration and touristic uses). In the protected areas of Argentina, fire management consists of the suppression of all fires without considering their origin. The consequence of this policy is the accumulation of fuel loads that increase the probability of large wildfires (Bridge et al., 2005; Miller et al., 2009; Godoy et al., 2019; Moreira et al., 2020). In addition, climate change models show a clear drought trend in Patagonia (Garreaud et al., 2013; Holz et al., 2017) that increases the fire hazard by drying the biomass. These conditions increase the wildfire risk in the WUI of the Patagonian parks. In Argentina, 172,326 ha of protected areas were burned in the period 1998–2016 (Ministry of Environment and Sustainable Development, 2018) and, for this reason, it is important to carry out scientific assessments to the elaboration of plans of prevention and fire management.
The coexistence between fire and people is not easy and the discussion between suppressing all fires in protected areas vs. letting natural fires burn and managing areas with prescribed fire is not closed (Ghermandi et al., 2005; Pereira et al., 2012; Huffman et al., 2020). The decision not fighting fires can provoke the death of people and the destruction of infrastructure inside and outside protected areas. There is also resistance to prevention measures that involve the fuel load reduction by prescribed fire (Defossé et al., 2011).
Fire hazard refers to the amount, composition, and distribution of vegetation (fuel) in the landscape that could affect the potential behavior and spread of fire, and its assessment, together with fire risk, is useful in fire prevention and management (Hardy, 2005; Dentoni and Muñoz, 2013). The elaboration of fire hazard maps helps to identify the most vulnerable areas (Ghermandi et al., 2016). The large majority of fire hazard and risk mapping use fire simulation modeling (Aragoneses and Chuvieco, 2021). Fire hazard studies include estimation of fuel load and analysis of the spatial arrangement and flammability of vegetation (Hardy, 2005).
In particular, flammability measurement is complex, because it is a property that combines four scale of analysis: leaf, plant, community and landscape (Pausas and Moreira, 2012; Ghermandi et al., 2016; Pausas et al., 2017). Leaves contain different moisture and content of flammable substances (resins, terpenes) and variables of leaf flammability as ignition frequency, ignition and combustion times, and flame temperature (proxy of heat release) are measured in the laboratory using an epiradiator (Valette, 1992: Ghermandi et al., 2016; Blackhall and Raffaele, 2019). Very flammable leaves are characterized by low moisture content and ignition time, high ignition frequency, combustion duration, and flame temperature. In addition, plants differ in their bulk density (dry biomass per unit volume) and proportions of live, dead, fine, and coarse material (Pausas et al., 2012). Very flammable plants have a high proportion of dead fine fuel load and low bulk density (the presence of air among branches and leaves guarantees combustion). These characteristics of leaves and plants of different species cause them to burn more or less easily when a fire occurs. Nevertheless, high leaf flammability of species present in communities is not the unique explanation of what occurs during wildfires (Schwilk, 2015). After the plant ignites, community variables come into play: vegetation cover, slope, and rock fragments. High vegetation cover implies high horizontal continuity and therefore high fire spread. Finally, when an entire plant community burns, landscape factors such as corridors that facilitate fire spread and barriers (water bodies, rocky outcrops, roads) that stop or reduce fire spread come into play (Littell and Gwozdz, 2011). For example, corridors can facilitate fire spread between areas inside and outside of the protected areas.
Laguna Blanca National Park (LBNP) (Patagonia, Argentina) has areas within the reserve category of the protected areas system that are considered as WUI because of the presence of human settlements and anthropic activities compatible with the conservation (Administration of National Parks, 2022). The LBNP is located in a semi-arid region, where the accumulation of fine fuel, dry summer conditions, high frequency of thunderstorms, and the presence of strong winds increase fire frequency. Wildfires constitute a problem to park management, leading them to elaborate a Laguna Blanca National Park fire management plan (2017). Our general objective was to contribute to this plan by assessing the fire hazard at two interconnected scales (community and species), through the analysis of environmental variables, and flammability at the level of leaf and plant with the final elaboration of a fire hazard map that integrated all the variables.
Materials and methods
Study area
The study area is the LBNP (11,250 ha), located in the Neuquén province, Argentine (30°02’S, 70°21’W) (Figure 1). The park was created in 1945 mainly to protect migratory water bird (Laguna Blanca National Park fire management plan, 2017). The park is considered a Ramsar site since 1992 (i.e., important wetland for conserving biological diversity by United Nations Educational, Scientific, and Cultural Organization [UNESCO], 2022) due to the presence of lagoons inhabited by numerous species of birds. The park is divided into areas with different protection categories: reserve (30%), and park (70%). Within the park category, the wild nature reserve constitutes 10%, and the strict nature reserve constitutes 60% (Figure 1). The more strictly conserved area is named “strict nature reserve” whereas in the “reserve” area settlements and stockbreeding are permitted. The climate is arid with dry summers, 180 mm of annual precipitation accumulated in autumn-winter, and a mean annual temperature of 14°C (Meteorological station of Zapala airport). Strong winds come from the west with gusts up to 160 km/h (Hoermann, 2004). The relief is dominated by plateaus and volcanic cones and the soil is sandy-clay loam, with components of basaltic origin and low organic matter content (Laguna Blanca National Park fire management plan, 2017).
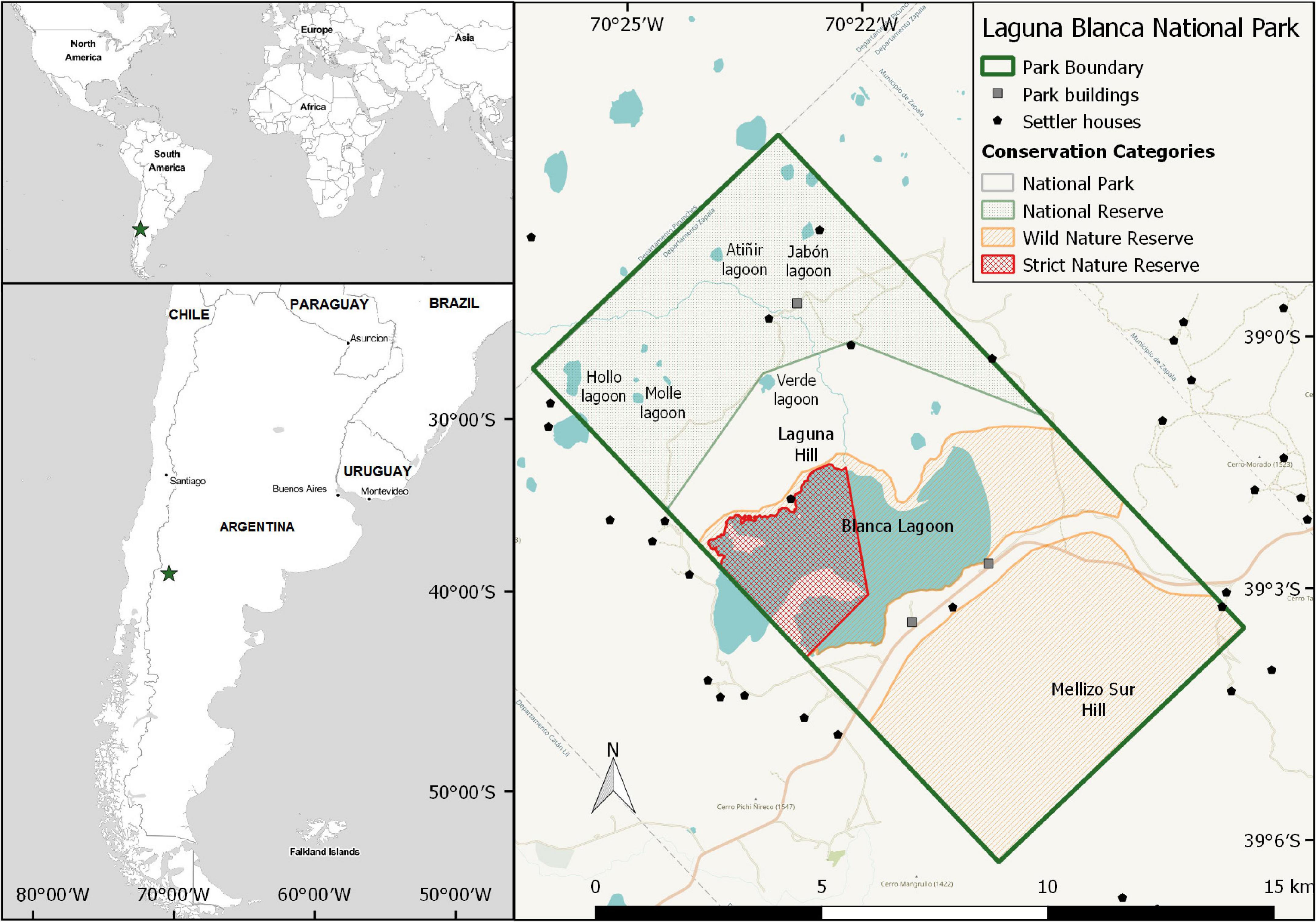
Figure 1. Location of the Laguna Blanca National park and settlements inside and outside of the park. The limits of the different management categories of the park were also showed (modified from LBNP management plan).
Vegetation corresponds to the Patagonian phytogeographic Province (Cabrera, 1976) and is dominated by the tussock grass Pappostipa speciosa and the shrubs Mulinum spinosum and Nassauvia axillaris. Shrublands of Anartrophyllum rigidum, Lycium chilense, and Colliguaja integerrima are present on slopes (Villamil and Testoni, 2012).
Areas of the park are used for recreation, tourism, and by rural settlers (Figure 1) that breed livestock, mainly goats and sheep. There are transhumance routes utilized by the neighbor settlers because the park is not fenced. Hardwood harvesting is prohibited (Laguna Blanca National Park fire management plan, 2017).
During the period 1965–2018, 28 fires were recorded in the park (Figure 2), 61% of which were anthropic, 11% were natural fires (by lightning storms), and 28% of unknown causes (Table 1). This incomplete fire record was provided by the rangers of the park. The mean burned area was 9.6 ± 16.8 ha, being the highest burned area 46 ha, and the smallest of two initial fires (0.002 and 0.004 ha) quickly extinguished by firefighters (Table 1).
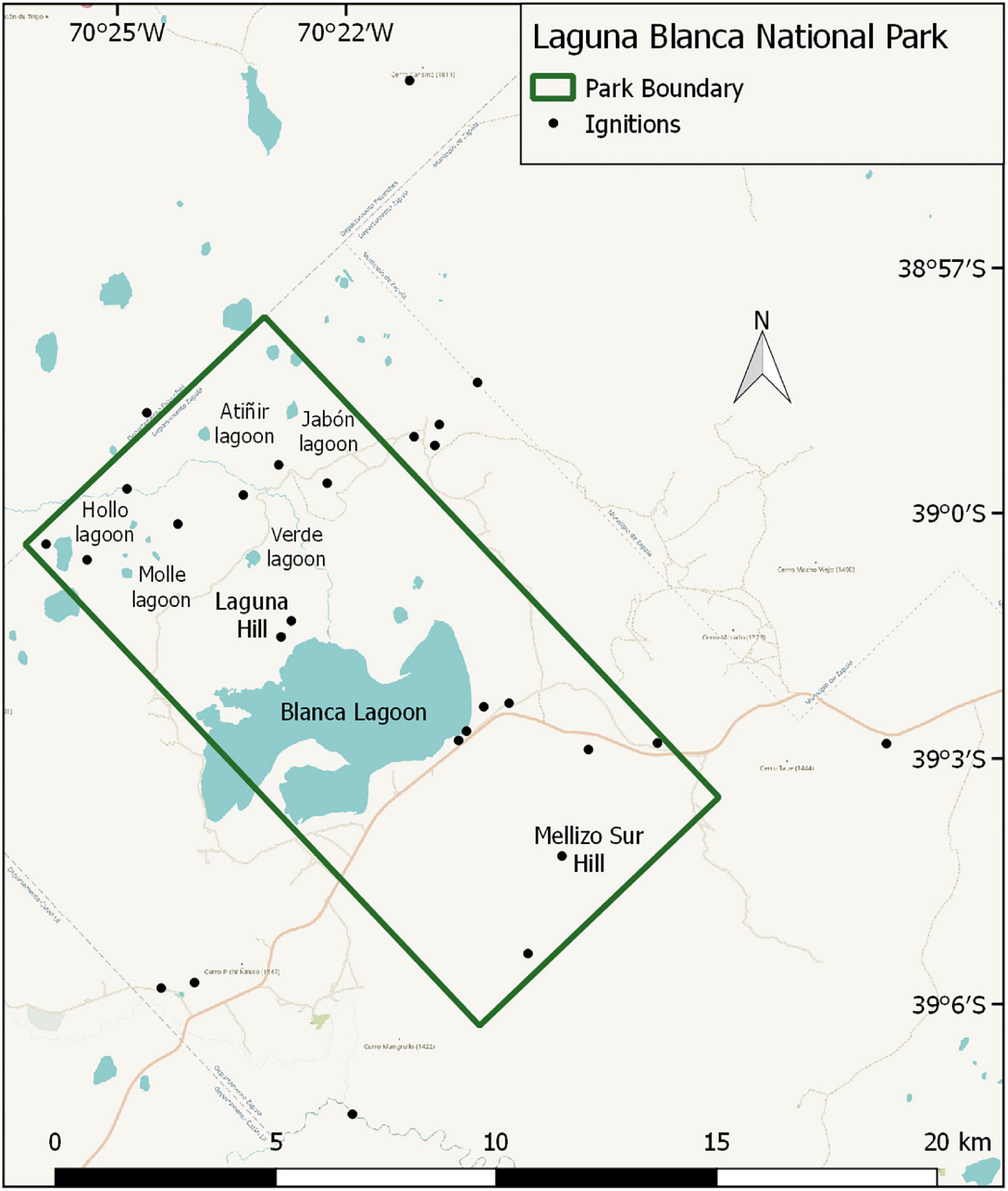
Figure 2. Location of fire ignitions inside and outside of the park (modified from Laguna Blanca National Park fire management plan, 2017).
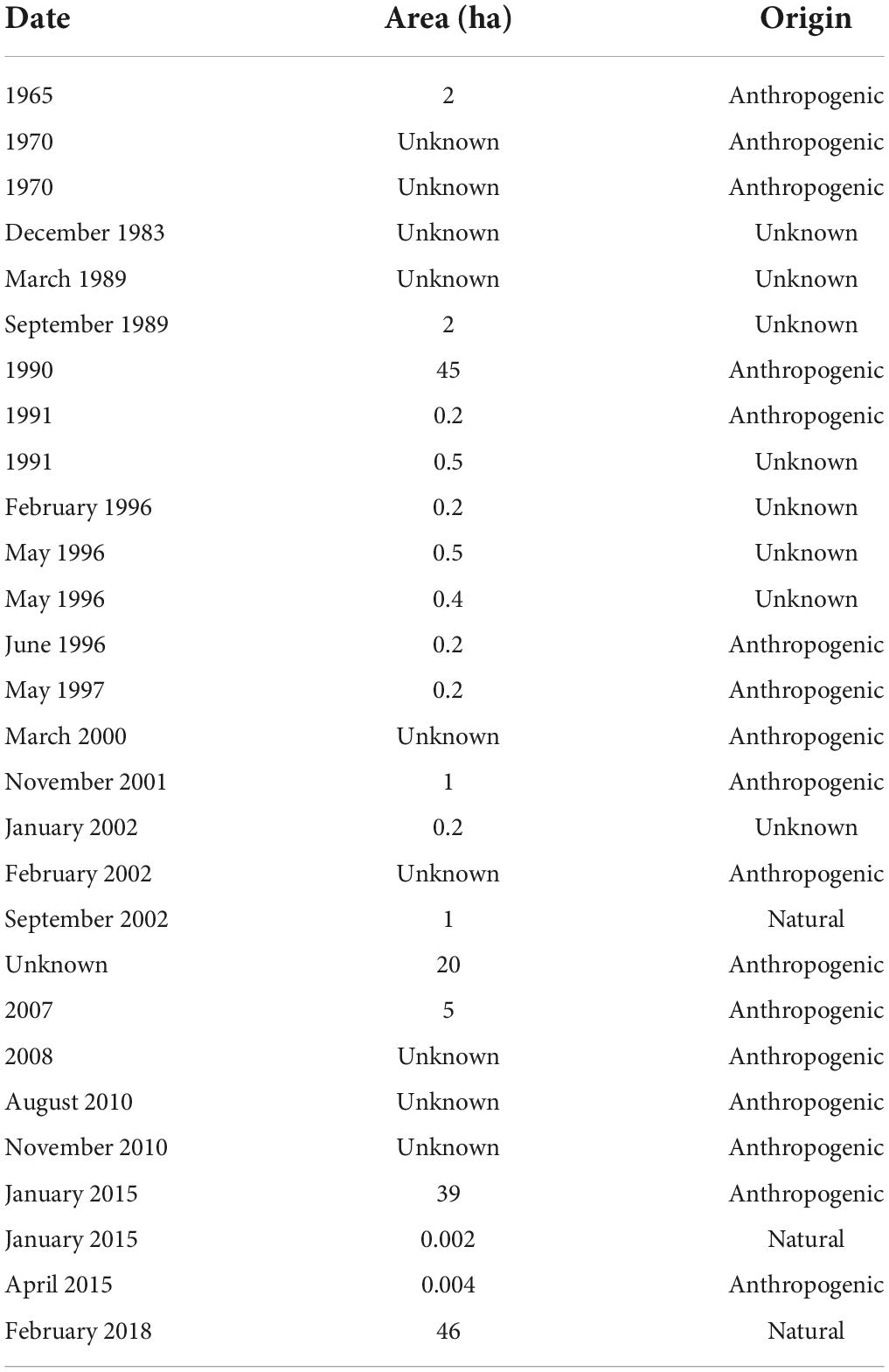
Table 1. Fires (1965–2018) recorded in the park (modified from Laguna Blanca National Park fire management plan, 2017).
Data collection
We performed the study at two scales: community and species. The community scale comprised the following variables: slope, rock fragments, and vegetation cover, whereas the species scale comprised flammability variables at leaf-level (moisture content, ignition frequency, ignition time, combustion duration, flame height, flame temperature) and at plant-level (fuel load, bulk density).
At the community scale, we used the same vegetation units recorded in the Laguna Blanca National Park fire management plan (2017) and we added a new vegetation unit (Retanilla patagonica-Colliguaja integerrrima). In some cases, we changed the name of the vegetation units to highlight the presence of the dominant species.
In November 2018, we performed field surveys in vegetation units and we measured dominant species cover using the line intercept method on five 50-m long transects randomly located and separated by at least 10 m (Mueller-Dombois and Ellenberg, 1974). We obtained the data of slope, rock fragments, and horizontal continuity of biomass from the Laguna Blanca National Park fire management plan (2017).
At the species scale, in January 2019 we collected 40 samples from 20 individuals of Pappostipa speciosa (Poaceae), Nassauvia axillaris (Asteraceae), Anartrophyllum rigidum (Fabaceae), Retanilla patagonica (Rhamnaceae), Colliguaja integerrima (Euphorbiaceae), Senecio filaginoides (Asteraceae), Schinus marchandii (Anarcadiaceae), and Lycium chilense (Solanaceae). The samples were kept cool in plastic bags to avoid moisture loss.
We estimated the moisture content with the gravimetric method weighing 20 samples of 2 g before and after to put them in an oven at 80°C for 48 h. Relative moisture content was calculated using the formula: (fresh weight – dry weight/dry weight) × 100.
For flammability testing, a 500 W epiradiator with 10 cm diameter radiant disc and nominal surface temperature of 420°C was used as a heat source. We burned 20 samples of 1 g and we measured the flammability parameters (Valette, 1992): ignition frequency (percentage of ignition occurrence respect to the total), ignition time (time lapsed until flame appearance), combustion duration; flame height, and flame temperature. Flame temperature was recorded at 2 cm height with a type K thermocouple connected to a datalogger.
To estimate the fuel load of the dominant species we randomly selected five individuals in each vegetation unit (March 2019). We measured the height and the two perpendicular hemi-axes to calculate the plant volume. We harvested 1/4 of each plant, and we separated the biomass in live fine, live coarse, dead fine and dead coarse fractions (fine: < 6 mm diameter; coarse > 6 mm diameter) which were dried in an oven at 80°C for 48 h and then weighed (Pausas et al., 2012). Then, we estimated the percentage of all the fractions and the bulk density (dry biomass per unit volume, g/cm3) (Pausas et al., 2012; De Cáceres et al., 2019).
We did not perform the flammability tests for Mulinum spinosum (Apiaceae) because we used previous data about this species (Blackhall and Raffaele, 2019), and we did not estimate fuel load and bulk density of S. marchandii because it is a protected species in the LBNP.
Statistics
We used one-way Anova and Kruskall-Wallis tests to compare data flammability variables at leaf and plant-level in the same species and among species and post-hoc multiple comparisons when the results were significant (P < 0.05).
We performed a Multiple Correspondence Analysis (MCA) followed by an Ascendant Hierarchical Classification Analysis (AHCA) (Lebart et al., 1995) to group the vegetation units in classes taking into account the representative community and flammability variables. Previous to the analysis; we divided the range of the data of community and flammability variables into three categories. We calculated the minimum value plus one-third of the difference (category 1), and the maximum value minus one- third of the difference (category 3). Category 2 was between both categories (Table 2). For the flammability variables, we calculated weighted averages by the relative cover of the tussock and shrub species present in each vegetation unit using the following equation (Ghermandi et al., 2016):
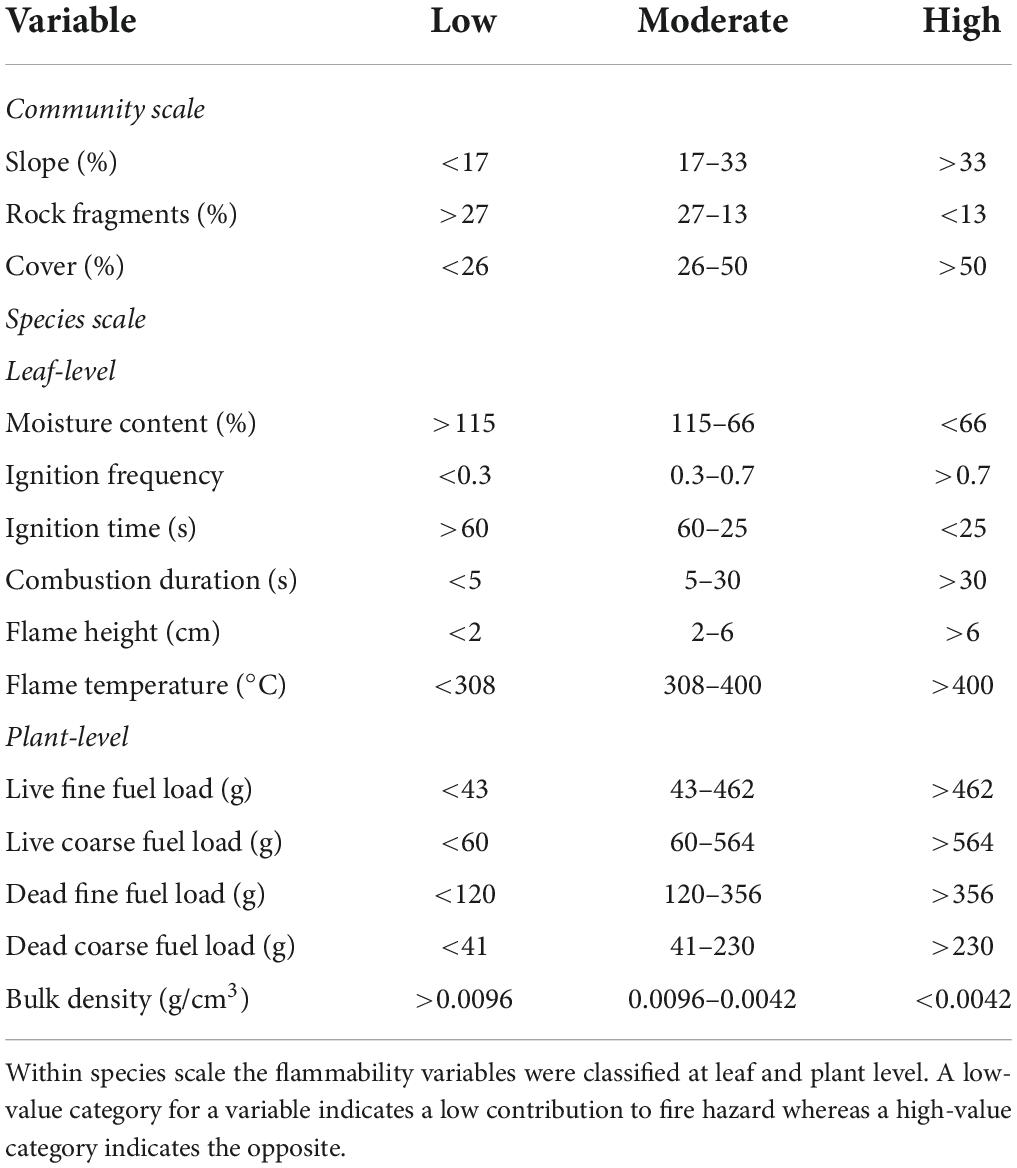
Table 2. Categories of the variables used in the multiple correspondence analysis and the ascendant hierarchical classification analysis at community and species scale.
where n is the maximum number of tussock grass and shrub species considered in a given vegetation unit, FV(i) represents a given flammability variable for the species i and, RC (i) represents the relative cover percentage estimated for this species in the vegetation unit. The resulting value for each vegetation unit was assigned to one of the three categories transforming the continuous variables into categorical variables. A low-value category for a variable indicates a low contribution to fire hazard whereas a high-value category indicates the opposite in a determined vegetation unit.
Then, we elaborated a single database with the vegetation units and the categories for flammability and community variables and analyzed using the MCA and AHCA. Together, the MFA and the AHCA select the most representative categories of all variables which were used to describe the classes (de Torres Curth et al., 2012). The classes comprised by vegetation units were classified into three fire hazard categories: low, moderate, and high. Finally, using these categories, we elaborated a WUI fire hazard map for the park. We described each category integrating the results of the two scales.
Results
Community scale variables
The 12 vegetation units in LBNP were grouped following the geomorphology. The volcano cones are occupied by N. axillaris, Colliguaja integerrima, Retanilla patagonica, and M. spinosum; the basaltic plateaus by Nassauvia axillaris, Pappostipa speciosa, Anarthrophyllum rigidum, and R. patagonica; the basaltic escarpments by P. speciosa, M. spinosum, N. axillaris, A. rigidum, and C. integerrima, and the valley bottoms by N. axillaris, Grindelia chiloensis, and M. spinosum (Figure 3 and Table 3).
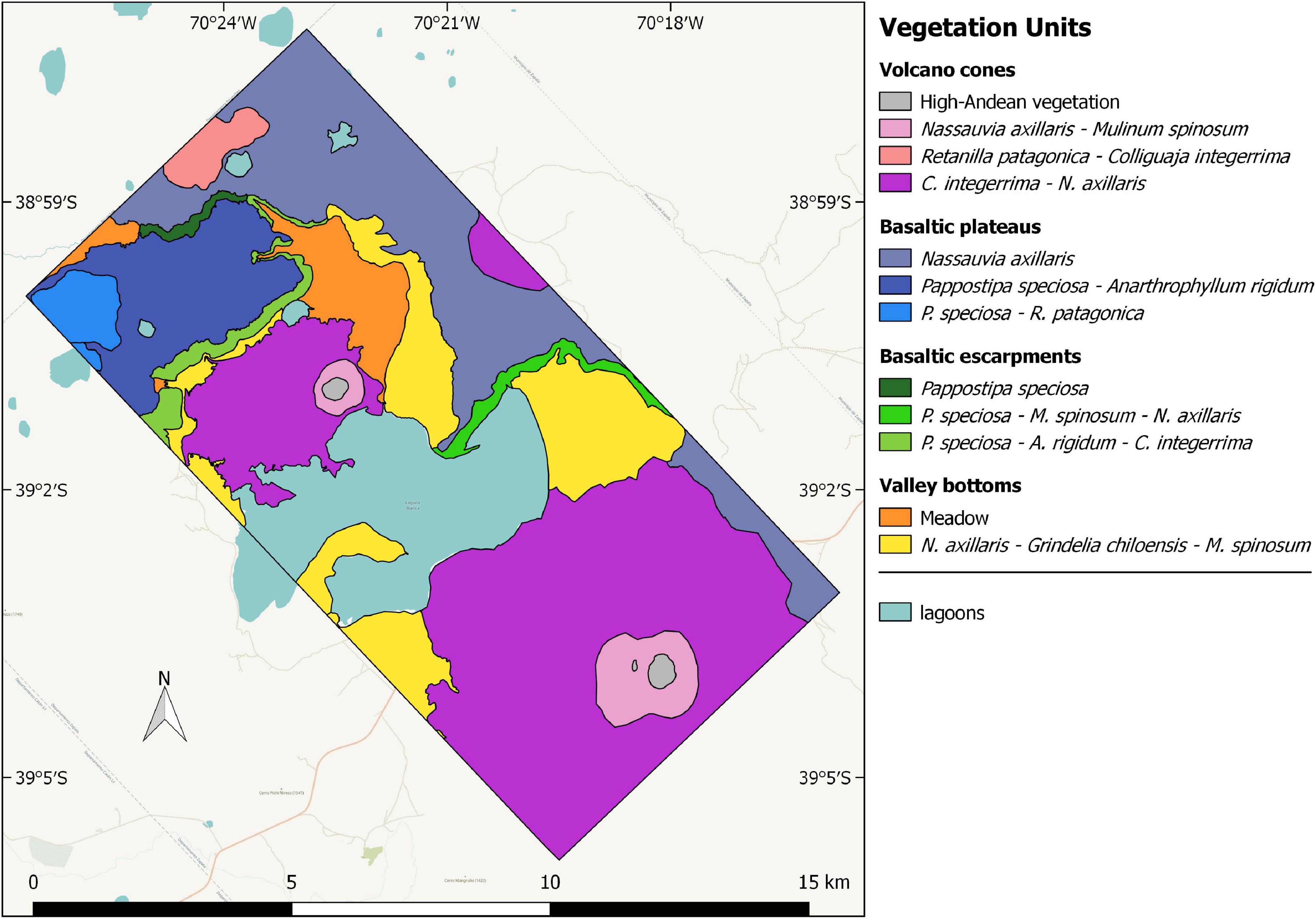
Figure 3. Vegetation units of the LBNP [modified from Laguna Blanca National Park fire management plan (2017)].
The cover in the high-Andean vegetation unit was the lowest (10%), whereas cover in the meadow (a mixture of Poaceae, Juncaceae, and Cyperaceae) was the highest (65%). Rock fragments percentage ranged from 0 to 40%, whereas the slope values ranged from 0 to 50% (Table 3).
Species scale variables
Leaf flammability
The moisture content ranged from 55.2 ± 14.9% (Pappostipa speciosa) and 193.9 ± 29% (Lycium chilense) and was different among species (H = 159, P < 0.005) (Figure 4A).
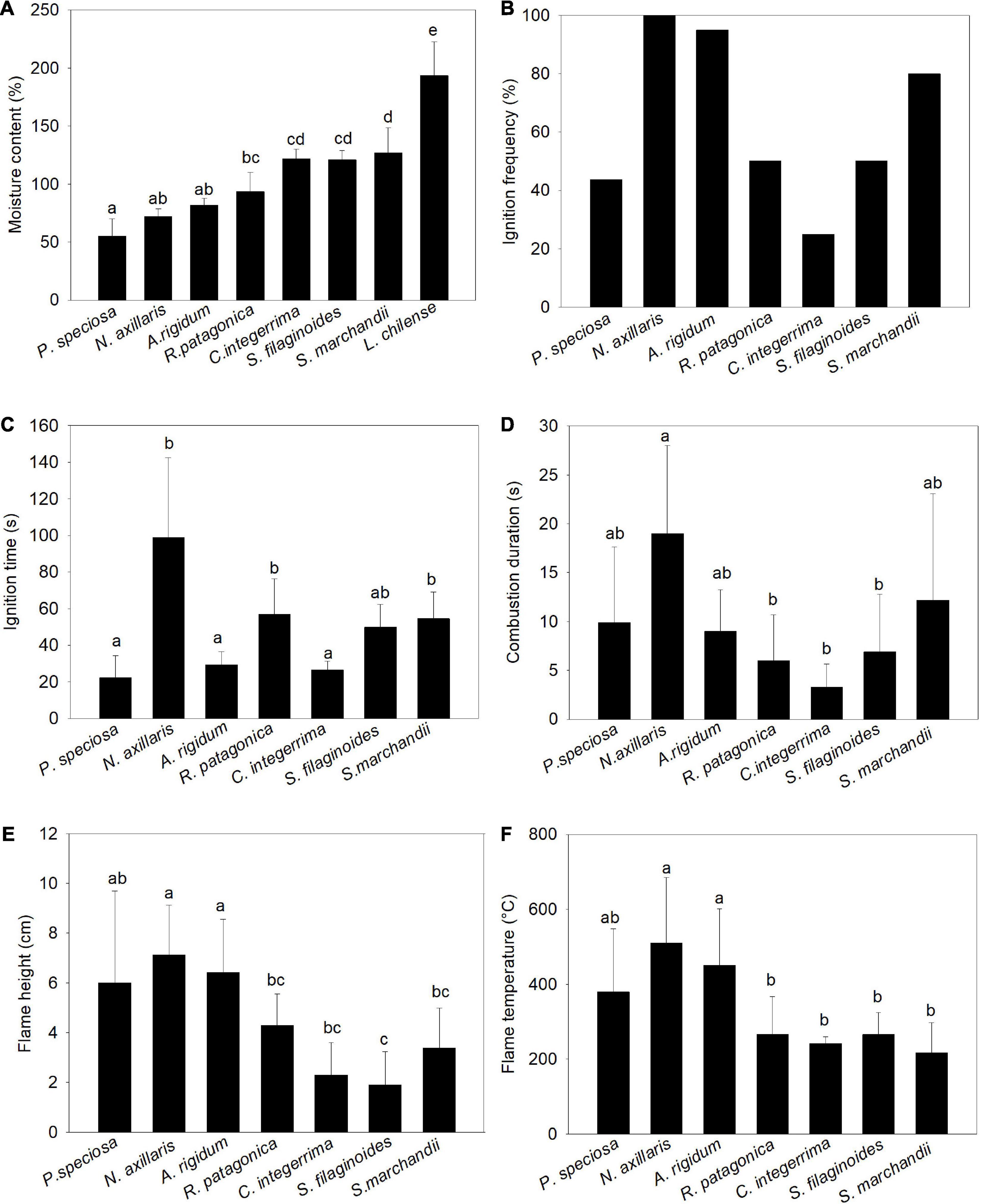
Figure 4. Mean ± (SD) of flammability variables (at leaf-level) of the dominant species present in the vegetation units. (A) Moisture content (%), (B) ignition frequency, (C) ignition time, (D) combustion duration (s), (E) flame height (cm), and (F) flame temperature (°C). Different letters indicate significant differences among species (P < 0.005).
The ignition frequency was high (>80%) for Schinus marchandii, Nassauvia axillaris, and Anarthrophyllum rigidum, whereas Senecio filaginoides, Retanilla patagonica, and P. speciosa showed intermediate values, being the lowest (25%) in Colliguaja integerrima. Lycium chilense was not analyzed because the samples never burned (Figure 4B).
We found differences among species for all flammability variables at leaf-level (ignition time: H = 57.5, P < 0.0001; combustion duration; H = 26.5, P < 0.0001; flame height: H = 49, P < 0.0001; flame temperature (H = 42.5, P < 0.0001) (Figures 4C–F). Nassauvia axillaris leaves had the longest ignition time and combustion duration, and produced the tallest flame and highest flame temperature (Figures 4C–F). The A. rigidum, C. integerrima, and P. speciosa leaves had the shortest ignition time (Figure 4C) whereas Colliguaja integerrima leaves had the lowest combustion duration (Figure 4D). Senecio filaginoides leaves had the shortest flame (Figure 4E) whereas the leaves of this shrub and R. patagonica, C. integerrima, and S. marchandii had the lowest flame temperature (Figure 4F).
Plant flammability
Plants of P. speciosa and M. spinosum had only fine fuel load mostly dead (83 and 62%, respectively) whereas N. axillaris had only coarse fuel load, of which 60% was live (Figure 5). Anarthrophyllym rigidum and S. filaginoides had high percentage of live fuel load (Figure 5).
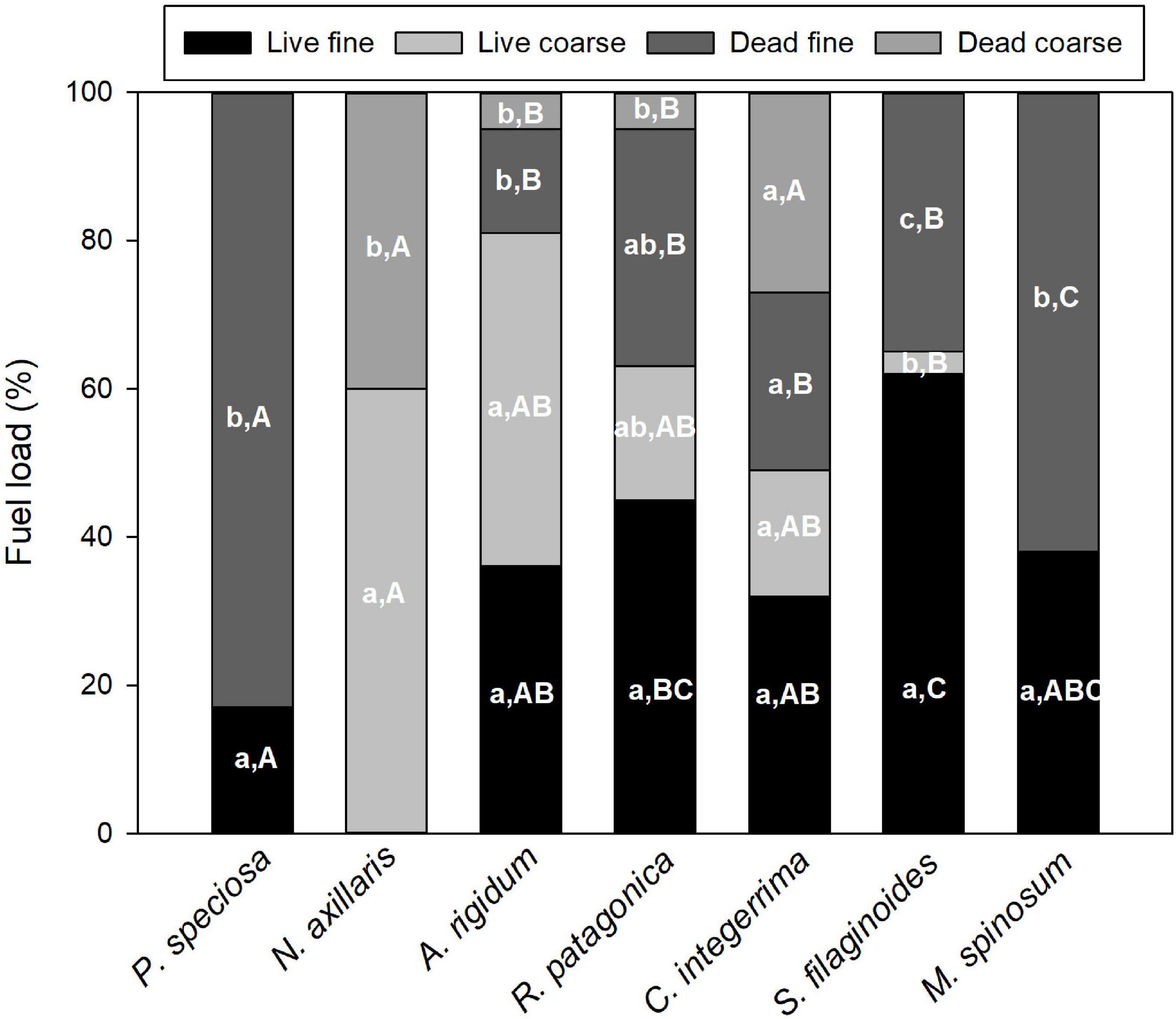
Figure 5. Mean percentage ± SD of live fine and coarse, and dead fine and coarse fuel load of dominant species. Different lower-case letters indicate significant differences in the same species, whereas upper-case letters represent significant differences among species (P < 0.005).
When we compared fuel load among species, we found that P. speciosa had the highest dead fine fuel load [F(5, 31) = 17.46, P < 0.001] whereas S. filaginoides has the highest values of live fine fuel load [F(5, 31) = 94.5, P < 0.001], although were not found significant differences with R. patagonica and M. spinosum (Figure 4). N. axillaris and C. integerrima had high percentage values of dead coarse fuel load than other species (H = 26.7, P < 0.001) (Figure 5).
Nassauvia axillaris had the highest bulk density, whereas C. integerrima, R. patagonica, and S. bracteolatus showed the lowest bulk density (H = 20.6, P < 0.01) (Figure 6).
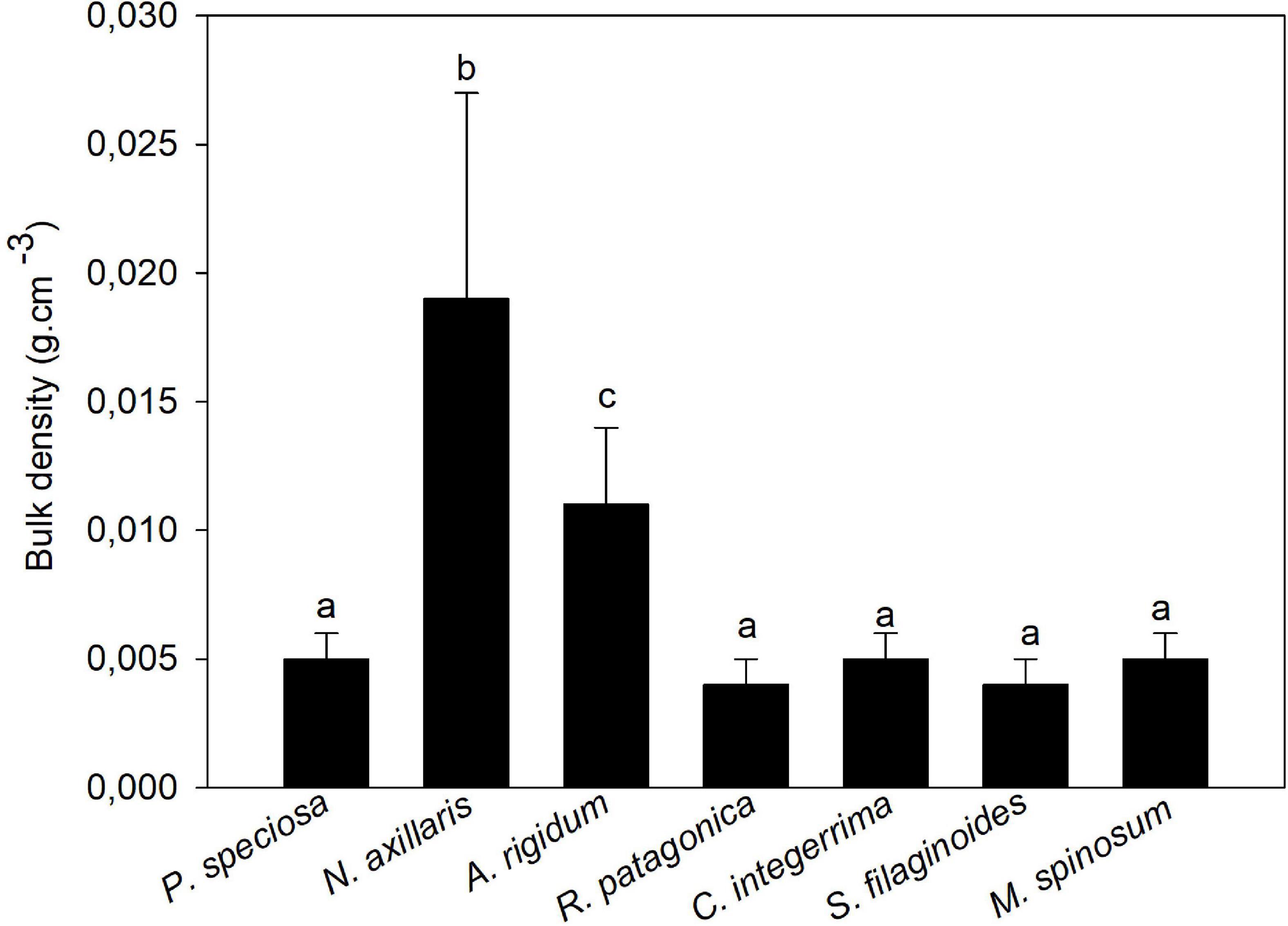
Figure 6. Mean bulk density ± SD of dominant species. Different letters indicate significant differences among species (P < 0.005).
Integration of community and species scale variables
In the multivariate correspondence analysis, the first axis explained 56.4% and the second axis explained 41.8% of the total variation. All the variables are represented in both axes. Axis 1 is mainly explained by the variables cover, moisture content, ignition time, flame height, and live coarse fuel load; while axis 2 is mainly explained by the variables ignition frequency, live fine fuel, and dead coarse fuel load (Supplementary Table 1). The AHCA allowed the identification of three classes (Figure 7). Class characterization was carried out on the basis of the results of the posteriori comparison of means (Supplementary Table 2). Class 1 includes two vegetation units of basaltic plateaus: N. axillaris and P. speciosa-R. patagonica; and one of the valley bottoms: N. axillaris-Grindelia chiloensis-M. spinosum. These vegetation units have intermediate cover and low percentage of rock fragments and slope. With regard to flammability variables, these vegetation units have high ignition times, intermediate values of moisture content, combustion duration, flame height, flame temperature, ignition frequency, amount of live fine and coarse fuel load and dead coarse fuel load; and low bulk density and amount of dead fine fuel load. Class 2 includes all the vegetation units of volcano cones: Nassauvia axillaris-Mulinum spinosum, Retanilla patagonica-Colliguaja integerrima, and C. integerrima-N. axillaris; and of basaltic escarpments: Pappostipa speciosa, P. speciosa-M. spinosum-N. axillaris, P. speciosa- Anarthrophyllum rigidum -C. integerrima, and one vegetation unit of basaltic plateaus: P. speciosa-A. rigidum. These vegetation units have intermediate plant cover and high percentage of rock fragments and slope. All flammability (leaves and individual plants) variables have intermediate values. Finally, the class 3 consists of a single vegetation unit: meadow, which is characterized by high cover and low percentage of rock fragments and slope. This vegetation unit has high values for the flammability variables moisture content and combustion duration; low values for flame height, flame temperature, frequency ignition, coarse (live and dead) fuel load; and intermediate bulk density and fine (live and dead) fuel load (Supplementary Table 2).
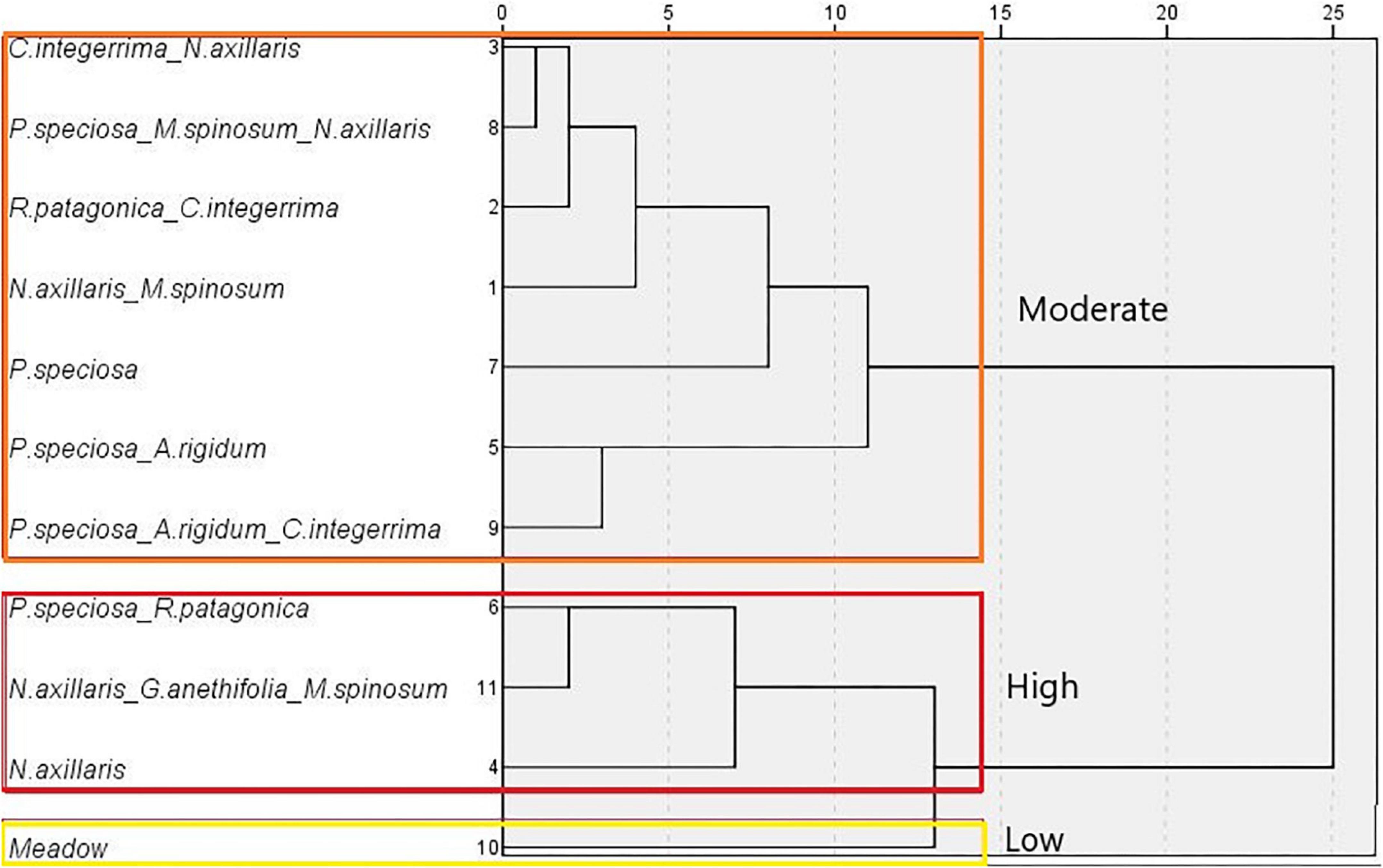
Figure 7. Dendrogram from the ascendant hierarchical classification analysis. The fire hazard category (low, moderate, and high) for each vegetation unit is showed.
We assigned three fire hazard categories (high, moderate, and low) to the vegetation units. The category high fire hazard was assigned to class 1 (58% of the park), the moderate fire hazard to class 2 (37% of the park), and low fire hazard to class 3 (5% of the park). High-Andean vegetation was not included in the multivariate analysis because the very low cover and the type of vegetation allowed the inclusion of this unit in the low fire hazard category. We used these categories to create a fire hazard map (Figure 8).
Discussion
The aim of the work was to elaborate a wildfire hazard map of the LBNP, integrating variables at community and species scale, to identify the most vulnerable wildland urban-natural areas. The park is inhabited by settlers and rangers and it is located relatively near the city of Zapala (30 km north-east, 40,000 inhabitants). The high fire hazard category occupies more than one-third of the total area of the park (37%) and it is composed by three vegetation units with the dominant presence of the very flammable shrub Nassauvia axillaris. In the units of vegetation of this fire hazard category, high fuel load and horizontal continuity contribute to fire spread. The moderate category (58%) brings together seven vegetation units principally present in the volcano cones and basaltic escarpments with the dominance of tussock grasses with a relatively low fuel load. Based on fire records within the park, the 33 and 66% of the total fires occurred in vegetation units categorized as high and moderate fire hazard. Finally, the low category is represented by high-Andean vegetation and the meadow (5%) for opposite reasons: the high-Andean vegetation, at the top of the volcano cones, is scattered and short whereas in the meadow the high cover is composed by short grasses and sedges (with low fuel load), and the local moisture is relatively high along the year, due to the shallow water table.
In Patagonia, most studies were performed about the flammability of plant species of forest and shrubland species, but not of steppe species (Bianchi and Defossé, 2015; Ghermandi et al., 2016; Bianchi et al., 2018; Blackhall and Raffaele, 2019). The species P. speciosa, M. spinosum, N. axillaris, and A. rigidum, frequent in the park, were the most flammable. Pappostipa speciosa showed the lowest leaf moisture content and time to ignition. Flammability leaf data for Mulinum spinosum (short time to ignition and high heat released) were recorded in another study (Blackhall and Raffaele, 2019). Both, P. speciosa and M. spinosum had a high proportion of dead fine fuel load and low bulk density. Dead fine tissues have low moisture content and burn quickly: the lower the moisture value, the lower the amount of energy required to trigger ignition. In addition, when dead tissues burn rapidly dries live tissues intermingled in the plant, increasing fire spread (Schwilk, 2003). In less compact plants, residence time of fire is low, and bud meristem tissue can survive and resprout even after wildfires of high intensity (Gonzalez et al., 2015a,b). Nassauvia axillaris had high heat released (i.e., flame height and temperature) and the comparatively highest proportion of coarse fuel load that allow long combustion, could develop severe wildfires if the high temperature damaged buds of nearby species (Pausas et al., 2012; Gonzalez et al., 2015b). Nassauvia axillaris itself could probably survive due to the position of bud, near of soil surface or slightly buried (Ghermandi, personal communication), however, little is known about the postfire strategy of shrubs that dominate the LBNP. Anarthrophyllum rigidum leaves presented low moisture content, short ignition time, and high flame height. However, the plants had a low amount of dead fuel and high bulk density, and both characteristics decrease species flammability. The flammability of a species is the result of the balance between the leaf and plant flammability and can be complex to interpret.
On the other hand, Mulinum spinosum contains terpenes (Damascos and Ghermandi, 2007), that are highly flammable secondary metabolites. Colliguaja integerrima leaves also contain terpenes (Bittner et al., 2001), which enable rapid ignition (second after P. speciosa), however, high leaf moisture content and low fine fuel load counteract the terpenes effect. More investigations should be carried out to explore the relationship between the secondary compounds and the flammability variables assessed by means of epiradiator tests.
Flammability at landscape-level responds to the presence of corridors that facilitate fire spread between different flammable areas and barriers that act as firebreaks (Littell and Gwozdz, 2011). In general, areas categorized as high fire hazard act as corridors between zones inside and outside of the protected areas. The vegetation unit N. axillaris-G. chiloensis-M. spinosum at the east of the Blanca lagoon is an example of a corridor because this high fire hazard can promote fire spread from P. speciosa-M. spinosum-N. axillaris vegetation units on basaltic plateaus to C. integerrima-N. axillaris (both of moderate fire hazard) on the base of volcano cones. In these areas are located the houses of the rangers and tourist-recreational activities are prevalent. On the other hand, the large Blanca lagoon and continuous rocky outcrops should act as barriers if winds (frequent from the west) pushed a wildfire in this direction.
Fire risk is related to the ignition probability and the land uses by settlers and tourists increase notably the occurrence of wildfires in the park (61% were anthropic). These uses are developed in high fire hazard vegetation units. The transhumance routes of livestock and camping areas involve fire pits that can be abandoned with some negligence. Several fires have occurred in the vicinity of the mentioned areas (rangers, personal communication).
There are several areas in the park of archeological and biodiversity value (Laguna Blanca National Park fire management plan, 2017). The vegetation unit P. speciosa-R. patagonica (high fire hazard), for example, is located in near the smaller lagoons of the park which are considered relevant for conservation due to their associated fauna and flora. Also in the volcanic cone of the southern Mellizo hill, there are large areas of mixed shrubland on the southern and southeastern slopes (Schinus spp., A. rigidum, C. integerrima, L. chilense, and Ephedra chilensis species) considered relevant for conservation. One of the intangible zones (i.e., areas where land use is prohibited) within the national reserve was also classified as high fire hazard.
Conclusion
Although species of fire-prone areas are adapted to a determined fire regime, particularly in WUI areas there are lives of people and building infrastructure to protect from fire damage. In this sense, our fire hazard map constitutes a valuable tool because identify the most vulnerable wildland urban-natural interface areas present in the LBNP. For example, if an initial fire were detected in a high fire hazard area, there should be a quick response to extinguish it immediately. Our results could be used by park fire managers to take preventive measures to reduce losses of lives, infrastructures, and biodiversity.
In general, wildfire management policies in National Parks of Argentina focus almost exclusively on fire suppression, while failing to address the problem from prevention. Currently, the discussion focuses on fighting fires or encouraging prescribed burning to reduce biomass where fire has been excluded for years. In the park prescribed fire is difficult to apply because of a lack of knowledge about the natural fire regime and the postfire strategies of plants, and because it could put in danger the infrastructure and the houses inside the park. To know the fire regime, it is necessary to complete fire records using satellite images and train the park staff for that. It will also be possible to forecast if fires are increasing in the current context of climate change.
The elaboration of fire hazard maps is only one of many fire-management strategies and other actions must be carried out to prevent and mitigate wildfires in protected areas. We suggest the following: early initial attack of all fires, especially of those present in vegetation units cataloged as a high fire hazard because they contain highly flammable species that spread the fire to other vegetation units very rapidly, because the initial attack is the most effective way to control fires; monitoring of daily lightning strikes (for example utilizing the World Wide Lightning Location Network [WWLLN], 2022) followed by real-time observation of eventual starting fires; regulation of anthropic activities that increase the fire risk (i.e., transhumance and tourism); organization of workshops with the local population (in the park and in Zapala schools) to increase the awareness about the vulnerability to fire.
Data availability statement
The original contributions presented in this study are included in the article/Supplementary material, further inquiries can be directed to the corresponding author.
Author contributions
SG and LG wrote the manuscript. SG and SA made data analysis and performed field monitoring. All authors contributed to the article and approved the submitted version.
Acknowledgments
We are very grateful to the rangers of the Laguna Blanca park for helping us with logistics, to Administración de Parques Nacionales who offered us GIS coverages of the park.
Conflict of interest
The authors declare that the research was conducted in the absence of any commercial or financial relationships that could be construed as a potential conflict of interest.
Publisher’s note
All claims expressed in this article are solely those of the authors and do not necessarily represent those of their affiliated organizations, or those of the publisher, the editors and the reviewers. Any product that may be evaluated in this article, or claim that may be made by its manufacturer, is not guaranteed or endorsed by the publisher.
Supplementary material
The Supplementary Material for this article can be found online at: https://www.frontiersin.org/articles/10.3389/ffgc.2022.855790/full#supplementary-material
References
Administration of National Parks (2022). National Parks. Available Online at: https://www.argentina.gob.ar/parquesnacionales (accessed Jan 15, 2022). doi: 10.3390/fire4030059
Aragoneses, E., and Chuvieco, E. (2021). Generation and mapping of fuel types for fire risk assessment. Fire 4:59. doi: 10.1071/WF13099
Bianchi, L., and Defossé, G. (2015). Live fuel moisture content and leaf ignition of forest species in Andean Patagonia, Argentina. Int. J. Wildland Fire 24, 340–348. doi: 10.1093/forsci/fxy054
Bianchi, L., Oddi, F. J., Muñoz, M., and Defossé, G. (2018). Comparison of leaf moisture content and ignition characteristics among native species and exotic conifers in Northwestern Patagonia, Argentina. For. Sci. 65, 375–386. doi: 10.4067/S0366-16442001000400006
Bittner, M., Alarcón, J., Aqueveque, P., Becerra, J., Hernández, V., Hoeneisen, M., et al. (2001). Estudio químico de especies de la familia Euphorbiaceae en Chile. Bol. Soc. Chil. Quím. 46, 419–431. doi: 10.1016/j.landurbplan.2019.04.002
Blackhall, M., and Raffaele, E. (2019). Flammability of Patagonian invaders and natives: When exotic plant species affect live fine fuel ignitability in wildland-urban interfaces. Landsc. Urban Plan. 189, 1–10.
Bridge, S. R. J., Miyanishi, K., and Johnson, E. A. (2005). A critical evaluation of fire suppression effects in the boreal forest of Ontario. For. Sci. 51, 41–50.
Cabrera, A. L. (1976). Regiones fitogeográficas argentinas. Enciclopedia Argentina de Agricultura y Jardinería. Buenos Aires, AR: ACME.
Damascos, M. A., and Ghermandi, L. (2007). Morfología del arbusto nativo Mulinum spinosum en sectores quemados de pastizales del NO de la Patagonia. Ecol. Aust. 17, 143–150. doi: 10.1007/s13595-019-0873-4
De Cáceres, M., Casals, M., Gabriel, E., and Castro, X. (2019). Scaling-up individual-level allometric equations to predict stand-level fuel loading in Mediterranean shrublands. Ann. For. Sci. 76:87. doi: 10.1007/s00267-012-9825-6
de Torres Curth, M. I., Biscayart, C., Ghermandi, L., and Pfister, G. (2012). Wildlande urban interface fires and socioeconomic conditions: A case study of a northwestern Patagonia city. Environ. Manag. 49, 876–91. doi: 10.1016/j.foreco.2010.11.021
Defossé, G. E., Loguercio, G., Oddi, F., Molina, J., and Kraus, P. D. (2011). Potential CO2 emissions mitigation through forest prescribed burning: A case study in Patagonia, Argentina. For. Ecol. Manag. 261, 2243–2254.
Dentoni, M. C., and Muñoz, M. M. (2013). “Evaluación del peligro de incendios,” in Glosario de términos relacionados con el manejo del fuego Report N°8, eds Programa Nacional de Evaluación de Peligro, de Incendios y Alerta Temprana Plan Nacional de Manejo del Fuego (Chubut: Secretaría de Ambiente y Desarrollo Sustentable). doi: 10.1175/JCLI-D-12-00001.1
Garreaud, R., Lopez, P., Mienvielle, M., and Rojas, M. (2013). Large scale control on the Patagonian climate. J. Clim. 26, 215–230. doi: 10.1016/j.jenvman.2016.09.051
Ghermandi, L., Beletzky, N. A., de Torres Curth, M. I., and Oddi, F. (2016). From leaves to landscape: A multiscale approach to assess fire hazard in wildland-urban interface areas. J. Environ. Manag. 183, 925–937.
Ghermandi, L., de Torres Curth, M., Gonzalez, S., and Lescano, N. (2005). Workshop de integración regional: Los incendios en la Patagonia. San Carlos de Bariloche: National University of Comahue. doi: 10.1071/WF18097
Godoy, M., Martinuzzi, S., and Kramer, A. (2019). Rapid WUI growth in a natural amenity-rich region in central-western Patagonia, Argentina. Int. J. Wildland Fire 28, 473–484. doi: 10.1007/s11284-014-1210-x
Gonzalez, S., Ghermandi, L., and Peláez, D. (2015a). Fire temperature effects on perennial grasses from northwestern Patagonian grasslands. Ecol. Res. 30, 67–74. doi: 10.1071/WF14134
Gonzalez, S., Ghermandi, L., and Peláez, D. (2015b). Growth and reproductive post-fire responses of two shrubs in semiarid Patagonian grasslands. Int. J. Wildland Fire 24, 809–818. doi: 10.1016/j.foreco.2005.01.029
Hardy, C. (2005). Wildland fire hazard and risk: Problems, definitions, and context. For. Ecol. Manag. 211, 73–82.
Hoermann, I. (2004). La vegetación del Parque Nacional Laguna Blanca. Report. Zapala: Administración Parques Nacionales. doi: 10.1073/pnas.1705168114
Holz, A., Paritsis, J., Mundo, I. A., Veblen, T. T., Kitzberger, T., Williamson, G. J., et al. (2017). Southern Annular Mode drives multicentury wildfire activity in southern South America. Proc. Natl. Acad. Sci. U.S.A. 114, 9552–9557. doi: 10.1186/s42408-020-00077-x
Huffman, D. W., Roccaforte, J. P., Springer, J. D., and Crous, J. E. (2020). Restoration applications of resource objective wildfires in western US forests: A status of knowledge revie. Fire Ecol. 16, 1–18.
Lebart, I., Morineau, A., and Pyron, M. (1995). Statistique Exploratoire Multidimensionnelle. Parıs: Dunod. doi: 10.1007/978-94-007-0301-8_5
Littell, J. S., and Gwozdz, R. (2011). “Climatic water balance and regional fire years in the Pacific Northwest, USA: Linking regional climate and fire at landscape scales,” in The Landscape Ecology of Fire, Ecological Studies 213, eds D. McKenzie, C. M. Miller, and D. A. Falk (Dordrecht: Springer), 117–139. doi: 10.1007/s10021-008-9201-9
Miller, J. D., Safford, H. D., Crimmins, M., and Thode, A. E. (2009). Quantitative evidence for increasing forest fire severity in the Sierra Nevada and southern Cascade Mountains. California and Nevada, USA. Ecosystems 12, 16–32.
Ministry of Environment and Sustainable Development (2018). Ministry of Environment and Sustainable Development. Available Online at: https://www.argentina.gob.ar/ambiente. (accessed Nov 15, 2018). doi: 10.1088/1748-9326/ab541e
Moreira, F., Ascoli, D., Safford, H., Adams, M., Moreno, J., Pereira, J., et al. (2020). Wildfire management in Mediterranean-type regions: Paradigm change needed. Environ. Res. Lett. 15:1001.
Mueller-Dombois, D., and Ellenberg, H. (1974). Aims and Methods of Vegetation ecology. Hoboken: Wiley and Sons. doi: 10.1111/j.1469-8137.2011.03945.x
Pausas, J. G., Alessio, G. A., Moreira, B., and Corcobado, G. (2012). Fires enhance flammability in Ulex parviflorus. New Phytol. 195, 18–23. doi: 10.1111/1365-2745.12691
Pausas, J. G., Keeley, J. E., and Schwilk, D. W. (2017). Flammability as an ecological and evolutionary driver. J. Ecol. 105, 289–297. doi: 10.1111/j.1469-8137.2012.04132.x
Pausas, J. G., and Moreira, B. (2012). Flammability as a biological concept. New Phytol. 194, 610–613. doi: 10.5755/j01.erem.59.1.856
Pereira, P., Mierauskas, P., Úbeda, X., Mataix-Solera, J., and Cerda, A. (2012). Fire in protected areas - the effect of protection and importance of fire management. Environ. Res. Eng. Manag. 59, 52–62. doi: 10.1111/nph.13372
Schwilk, D. W. (2003). Flammability is a niche construction trait: Canopy architecture affects fire intensity. Am. Nat. 162, 725–733.
United Nations Educational, Scientific, and Cultural Organization [UNESCO] (2022). United Nations Educational, Scientific, and Cultural Organization. Available Online at: https://whc.unesco.org/en/ramsar/ (accessed May 5, 2022).
Valette, J. C. (1992). “Inflammabilities of Mediterranean species,” in Forest Fire Risk and Management: European School of Climatology and Natural Hazard Course General Directorate for Science, eds P. Balabanis, G. Eftichidis, and R. Fantechi (Halkidiki: European Commission), 51–64.
Villamil, C. B., and Testoni, D. (2012). La Flora del Parque Nacional Laguna Blanca. Bahía Blanca: Universidad Nacional del Sur.
World Wide Lightning Location Network [WWLLN] (2022). World Wide Lightning Location Network. Avaialble Online at: http://wwlln.net/ (accessed Jan 15, 2022).
Keywords: fire hazard map, flammable species, multivariate analysis, protected areas, steppe, wildland urban interface fires
Citation: Gonzalez S, Aller SA and Ghermandi L (2022) Fire hazard assessment at different scales in the wildland-urban interface of semiarid areas of northern Patagonia. Front. For. Glob. Change 5:855790. doi: 10.3389/ffgc.2022.855790
Received: 16 January 2022; Accepted: 16 August 2022;
Published: 13 September 2022.
Edited by:
Peter Fule, Northern Arizona University, United StatesReviewed by:
Liliana Del Giudice, National Research Council of Italy, Institute of BioEconomy (CNR, IBE), ItalyAlan Ager, US Forest Service Research and Development, United States
Copyright © 2022 Gonzalez, Aller and Ghermandi. This is an open-access article distributed under the terms of the Creative Commons Attribution License (CC BY). The use, distribution or reproduction in other forums is permitted, provided the original author(s) and the copyright owner(s) are credited and that the original publication in this journal is cited, in accordance with accepted academic practice. No use, distribution or reproduction is permitted which does not comply with these terms.
*Correspondence: Sofía Gonzalez, c2dvbnphbGV6QGNvbWFodWUtY29uaWNldC5nb3YuYXI=