- 1Key Laboratory of Vegetation Restoration and Management of Degraded Ecosystems, South China Botanical Garden, Chinese Academy of Sciences, Guangzhou, China
- 2Hawkesbury Institute for the Environment, Western Sydney University, Richmond, NSW, Australia
- 3Global Centre for Land-Based Innovation, Western Sydney University, Richmond, NSW, Australia
- 4Guangdong Provincial Key Laboratory of Applied Botany, South China Botanical Garden, Chinese Academy of Sciences, Guangzhou, China
- 5University of Chinese Academy of Sciences, Beijing, China
Tree growth may be affected by rising temperature. We conducted two long-term, independent warming experiments in a subtropical forest; one experiment used translocation warming and one experiment used infra-red (IR) warming. Both warming techniques are designed to increase air and soil temperatures (Tair and Tsoil), but may also differentially affect other environmental variables, including soil volumetric water content (SVWC), air relative humidity (RH) and vapor pressure deficit (VPD). Hence, tree response ascribed to Tair and Tsoil may be dependent on the indirect effects of the warming techniques. We experimentally tested these ideas on three native tree species (Machilus breviflora, Syzygium rehderianum, and Schima superba), which occurred at all experimental sites, in subtropical China. We translocated trees from higher elevation sites to lower elevation sites in the coniferous and broadleaf mixed forest (Tair was 0.68 ± 0.05°C higher; 8 years) and mountain evergreen broadleaf forest (Tair was 0.95 ± 0.06°C and 1.63 ± 0.08°C higher; 8 years). IR warming was imposed at an experimental site in a monsoon evergreen broadleaf forest (Tair was 1.82 ± 0.03°C higher; 5 years). We found that both methods directly increased Tair and Tsoil (although to varying degrees), while translocation warming indirectly dried the soil (lower SVWC) and IR warming indirectly dried the air (lower RH and higher VPD). Machilus breviflora exposed to translocation warming exhibited lower photosynthesis due to higher Tsoil and lower SVWC, leading to declining growth. Higher Tair and Tsoil due to translocation warming increased photosynthesis and growth for S. superba. Trees exposed to IR warming exhibited reduced photosynthesis due to lower RH (M. breviflora) and to lower stomatal conductance (gs) as a function of higher Tair (S. rehderianum and S. superba). This study highlights the potential direct and indirect effects of different warming techniques on the physical environment of forest ecosystems, and subsequently their impacts on biological traits of trees. Hence, different warming techniques may provide different outcomes when assessing the impact of warming on trees in future climates.
Introduction
The global average air temperature is predicted to increase by 1.5°C during 2030-2052 (IPCC, 2018). Tropical forests play a vital role in the global carbon cycle, accounting for more than one-third of terrestrial net primary productivity, due to high metabolic activity and species diversity (Slot and Winter, 2017). Warming conditions put tropical forests at risk, since increasing temperatures could be approaching the high-temperature threshold associated with narrower temperature tolerances in tropical trees (Cunningham and Reed, 2002). Given our limited understanding of tropical forest response to warming conditions, field warming experiments will greatly improve our knowledge regarding the adaptability of tropical trees to higher temperatures (Cavaleri et al., 2015; Kimball et al., 2018).
The patterns of forest ecosystem response may vary based on different warming treatments (Natali et al., 2011). Numerous warming methods, including greenhouses, open top chambers, infra-red (IR) heaters, cables and studies along elevation gradients, have been employed to investigate warming effects on tree physiology in forest ecosystems (Booth, 1988; De Frenne et al., 2010; Wang et al., 2017; Kimball et al., 2018). Translocating plants and soils from cooler high elevation sites to warmer low elevation sites may achieve changes in environmental variables, containing air temperature (Tair), soil temperature (Tsoil) and soil volumetric water content (SVWC), anticipated in a warmer world, that more accurately reflect the potential effects of altered environmental variables on plant and soil processes (Luan et al., 2014; Nottingham et al., 2019; Wu et al., 2020b). Higher Tair and Tsoil under translocation warming may alleviate lower temperature limitations to stomatal conductance (gs) (Strand et al., 2002; Li et al., 2016) and photosynthetic capacity (Zhou et al., 2018), thereby facilitating plant growth. In contrast, some studies reported that warming temperatures reduced photosynthesis due to stomatal limitation induced by lower SVWC (Wertin et al., 2012; Reich et al., 2018). The reductions in nutrient availability and absorption, due to increasing Tsoil and declining SVWC, could decrease foliar nutrient concentrations, leading to non-stomatal limitations to photosynthesis and inhibition of plant growth (Leon-Sanchez et al., 2019).
Infra-red (IR) heaters are commonly used to directly increase Tair and Tsoil, but they may also indirectly increase leaf-to-air vapor pressure deficit (VPD) by reducing air relative humidity (RH) in the field (Kimball et al., 2008; de Boeck and Nijs, 2011). In these studies, photosynthetic capacity and rate may decline with increasing Tair, which may generate leaf temperatures that exceed the optimum temperature (Topt), reduce leaf chlorophyll (Dusenge et al., 2020) and Rubisco concentrations (Stinziano and Way, 2017). In addition, increased VPD due to IR warming, can directly reduce gs and photosynthesis independent of temperature, eventually limiting tree growth (Restaino et al., 2016; Grossiord et al., 2020). However, IR manipulation may have limited impact on Tsoil in some tropical forests, due to canopy interception of radiation by high vegetation cover (Rich et al., 2015); changes in Tsoil might also be buffered in soils with higher SVWC (McDaniel et al., 2013) and depth (Luo et al., 2010).
Previous studies have demonstrated that environmental variables other than Tair and Tsoil regulate plant physiological response to warming (Day, 2000; Reich et al., 2018), but few studies have assessed the impact of different warming techniques on the physical environment and subsequent biological responses in tropical forest ecosystems. Greater understanding of tree response to altered environmental variables in the field, and whether these responses change with warming technique, will increase our capacity to predict the responses of tropical forest ecosystems to warming (Shaver et al., 2000; Wan et al., 2002). In 2012, we initiated field warming experiments in the subtropical forest of China (Dinghushan) by translocation (initiated in 2012) and IR (initiated in 2015) warming techniques, which increased Tair and Tsoil by 1–2°C. Our previous results showed that biomass of S. superba was enhanced, while that of S. rehderianum and Machilus breviflora were not influenced by translocation warming in the coniferous and broadleaf mixed forest (CBMF) (Li et al., 2017). Translocation warming increased photosynthesis and growth of S. superba and S. rehderianum, but reduced that for M. breviflora in the mountain evergreen broadleaf forest (MEBF) (Wu et al., 2020a,b). Infra-red warming reduced photosynthesis of Schima superba, but not for Syzygium rehderianum in the monsoon evergreen broadleaf forest (MEBMF) (Wu et al., 2018).
In this study, we analyzed the impacts of two independent experiments that compared two warming techniques – translocation (CBMF and MEBF) and IR (MEBMF) – on the physical environment and subsequent impacts on plant physiology and growth for the three common tree species (M. breviflora, S. rehderianum, and S. superba). We recognize that it would have been ideal to conduct a single experiment that directly compared translocation and IR warming within the same biological community in identical soils, but that was not possible given restrictions on using IR warming within the biological reserve. However, the two experiments reported here provide insight into potential, differential indirect effects of these warming techniques on the physical environment. Here, we report physiological data (last 3 years) and growth data (last 5 years) from the two experimental warming treatments. Our objectives were to determine the direct and indirect effects of the warming techniques on the air and soil environment, and if they differed, to determine whether this had variable impacts on tree physiology and growth. Importantly, we need to know if the warming technique affects the outcome of studies on tree responses to future climate change scenarios.
Materials and Methods
Study Site
This study was conducted at the Dinghushan Biosphere Reserve (DBR, 23°09’N–23°11’N, 112°30’E–112°33’E) in southern China, which exhibits a typical tropical monsoon climate. The mean annual temperature is approximately 21°C, and the relative humidity averages 80% throughout the year. The mean annual precipitation is approximately 1900 mm; nearly 80% of the rainfall occurs during the wet season (April–September) and 20% during the dry season (October–March) (Liu et al., 2017; Wu et al., 2020a). There are three major tropical forest types in DBR, including the monsoon evergreen broadleaf forest (MEBMF, 30 m altitude above sea level), coniferous and broadleaf mixed forest (CBMF, 300 m altitude above sea level) and mountain evergreen broadleaf forest (MEBF, 600 m altitude above sea level). During 1954–2009, the mean annual temperature at the DBR has increased by approximately 1°C (Zhou et al., 2011).
Warming Experiment Design
Translocation Warming Experiment
Tair and Tsoil of CBMF and MEBF were increased by translocation warming. Coniferous and broadleaf mixed forest was translocated from the altitude of 300 m (current climate) to 30 m (Tair was 0.68 ± 0.05°C higher). The three plots of CBMF were constructed at 300 m elevation site and the other three plots of CBMF were constructed at 30 m elevation site. MEBF was translocated from the altitude of 600 m (current climate) to 300 (Tair was 0.95 ± 0.06°C higher) and 30 m (Tair was 1.63 ± 0.08°C higher). The three plots of MEBF were constructed at 600 m, 300 m, and 30 m elevation sites, respectively (Figure 1). The site was in an open area where they were exposed to full natural sunlight and rain. Each chamber had an edge length of 3 m, with a 0.8-m-deep belowground section. The belowground section was surrounded by a concrete brick wall covered with ceramic tiles to prevent lateral and vertical movement of water and nutrients from the surrounding soil. There was a hole at the top and another hole at the bottom of the wall. The holes (inner diameter: 2 cm) were connected to stainless-steel water collection boxes to collect surface and ground water samples. Both holes were capped by a 2-mm plastic net to prevent losses other than leachates.
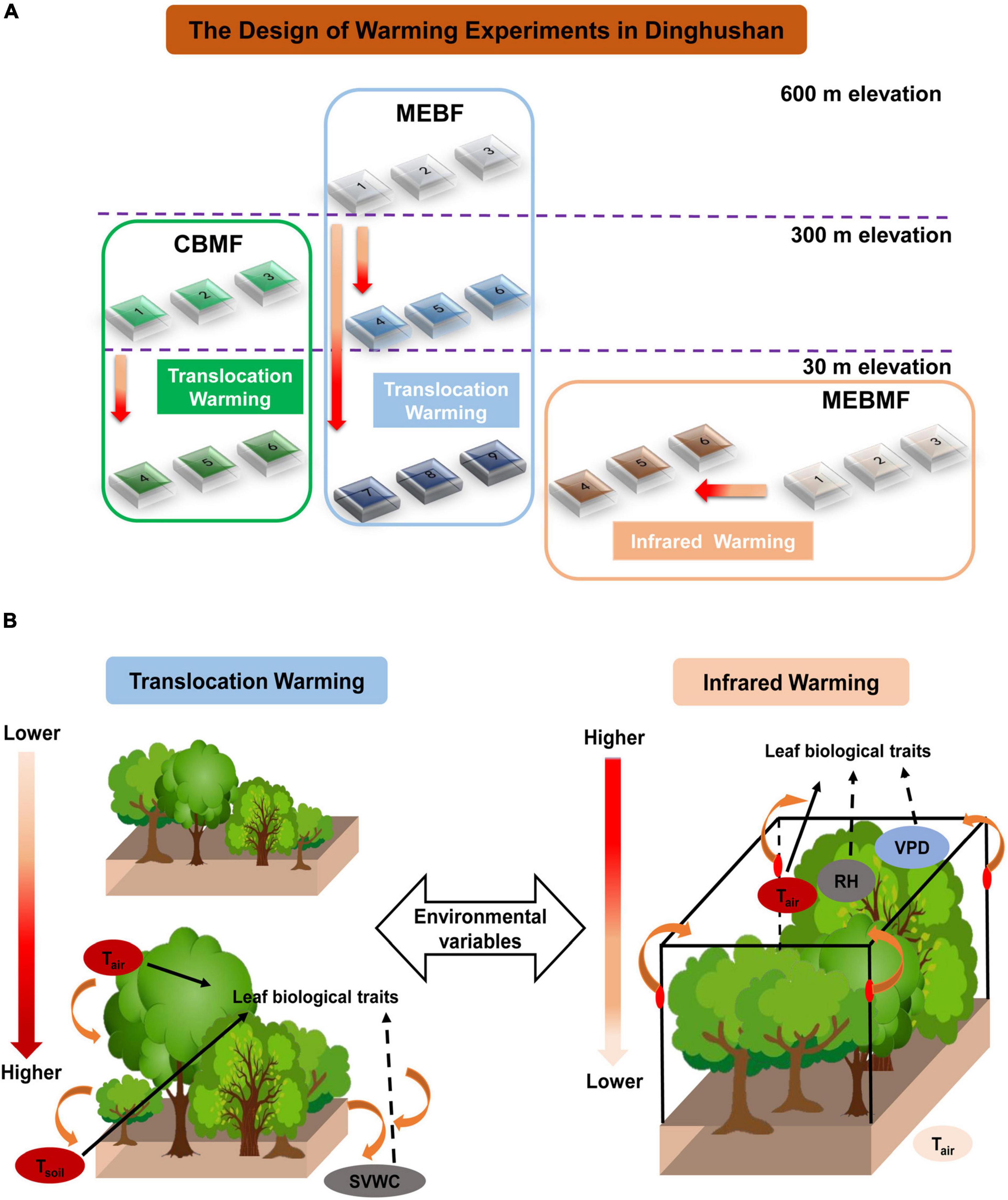
Figure 1. (A) Schematic of the long-term filed warming experiments in Dinghushan, China: higher temperatures for coniferous and broadleaf mixed forest (CBMF) and mountain evergreen broadleaf forest (MEBF) were achieved by translocation warming; while higher temperatures for monsoon evergreen broadleaf forest (MEBMF) was achieved by infrared (IR) warming. (B) the conceptual diagram for the effects of environmental variables caused by translocation and infrared warming on biological traits. Tranloscation warming increased Tair and Tsoil but decreased SVWC; IR warming enhanced Tair and VPD but reduced RH.
In April 2012, soils from three different layers (0–20, 20–40, and 40–70 cm) were collected from the CBMF at approximately 300 m altitude, homogenized separately, and then transplanted by individual layers into the macrocosm model CBMF (300 and 30 m), resulting in 6.3 m3 of soil in each chamber. Soils were allowed to settle and develop structure for one year before seedlings were added to the plots. Similarly, soils were collected from the MEBF at approximately 600 m altitude, and then translocated to model MEBF (600, 300 and 30 m). In May 2013, 1-year old seedlings of M. breviflora, S. rehderianum, and S. superba (similar size, stem basal diameter was approximately 4 mm and height was approximately 30 cm) were collected in the field, according to their occurrence and distribution in CBMF (300 m) and MEBF (600 m). Then, six individuals per species were randomly transplanted into model CBMF and MEBF at each elevation. Each individual plant was transplanted in a randomized block design so that all plants received approximately equal light. A litter trap (0.3 × 0.3 m) with a mesh size of 1 mm was randomly placed in each plot about 0.3 m above the soil surface to collect litter from June 2014. Further details of the experimental design can be found in Liu et al. (2017). Here, we present data on physiological traits (last 3 years) and growth (last 5 years) of the experiment.
Tair, RH and water vapor pressure (e) of CBMF and MEBF were monitored and recorded at each elevation, using a HMP155A temperature probe. Tsoil at 5-cm depth was automatically recorded in each chamber using Campbell 109 constantan-copper thermocouples (Campbell Scientific, Logan, UT, United States). SVWC at 5-cm depth was measured at the same depth using time domain reflectometer probes (CS616). Vapor pressure deficit (VPD) was calculated as: e/RH – e (e: water vapor pressure; RH: air relative humidity). Photosynthetically active radiation (PAR) and rainfall were recorded at each elevation. All data on microclimate were recorded every half hour using data loggers (CR1000, Cambell Scientific) and analyzed for the last 5 years.
Infra-Red Warming Experiment
Tair and Tsoil of MEBMF were increased by IR heaters. At an altitude of 30 m, three plots of MEBMF were sealed by transparent glass to form open top chambers (OTCs, 3 m in height, Tair was 1.82 ± 0.03°C higher) in March 2012, which were heated by forced air blown over closed-loop resistance wires surrounding the OTCs at 1.5 m in height to simulate a warming environment in 2014; the remaining three plots were used as controls (Wu et al., 2018) (Figure 1). Three different soil layers and seedlings of M. breviflora, S. rehderianum and S. superb were collected from the MEBMF at an altitude of 30 m. Soil filling and seedlings planting were taken in MEBMF as indicated in CBMF and MEBF (shown above). Here, we present data on physiological traits (last 3 years) and growth (last 5 years) of the experiment.
Tair, RH and e of MEBMF (controls) were monitored and recorded at 30 m elevation, and air environmental variables of MEBMF (+ 1.82°C, warming treatment) were monitored and recorded in each chamber. Tsoil and SVWC at 5-cm depth were automatically recorded in each chamber. Data were recorded hourly using data loggers (CR1000, Campbell Scientific) and analyzed for the last 5 years.
We were not allowed to use IR warming at the CBMF and MEBF sites, located within the core area of the Biosphere Reserve, due to potential fire risk from faulty IR heaters. Therefore, we translocated seedlings of M. breviflora, S. rehderianum and S. superb and soils from the CBMF (300 m) and MEBF (600 m) sites to lower elevations to achieve natural increases in temperatures. However, we were able to use IR warming at the MEBMF site because it was not located within the core of the Reserve. Although this was not an ideal experimental design, it still allowed us to assess the indirect effects of the two warming treatments on the physical environment and subsequent biological response.
Leaf Gas Exchange Measurements
The net assimilation rate-intercellular CO2 concentration (A-Ci) curves were generated on fully expanded leaves from three or four seedlings per chamber per species (n = 3–4) between 0900 and 1400 h on clear days in August 2019 and 2020 (last 2 years), using a portable open path gas exchange system (Licor-6400, Li-Cor, Lincoln, NE, United States) equipped with a leaf chamber fluorometer (6400-40). A-Ci curves were generated at saturating PAR of 1500 μmol m–2 s–1, leaf temperature (25°C) and ambient relative humidity inside the leaf chamber (∼60%). A-Ci curves were generated using leaf chamber CO2 values of (in order): 400, 200, 100, 50, 400, 400, 600, 800, 1200, 1400, 1600, and 1800 μmol mol–1. The maximum rate of photosynthetic Rubisco carboxylation (Vmax, μmol m–2 s–1) and apparent maximum rate of photosynthetic electron transport (Jmax, μmol m–2 s–1) were estimated from A-Ci curves.
Leaf light-saturated photosynthesis (Asat, μmol m–2 s–1), stomatal conductance (gs, mol m–2 s–1) and transpiration rate (E, mmol m–2 s–1) were measured in August 2018, 2019 and 2020 (last 3 years), at saturating PAR of 1500 μmol m–2 s–1, CO2 of 400 μmol mol–1 and leaf temperature (25 °C). Instantaneous water-use efficiency (iWUE, μmol m mol–1) was calculated as Asat/gs.
Measurements of Nutrient and Chlorophyll Concentrations
For gas exchange measurements, opposite leaves were harvested for chemical analyses in August 2019 and 2020 (last 2 years). Five leaf discs (6 mm diameter) from one individual per treatment per chamber were cut and dipped into 5 mL of 80% acetone to determine the chlorophyll concentrations [Chl(a+b), μg cm–2] (Arnon, 1949).
Some leaves were used to determine leaf area (LA, cm2), with petioles removed, using a leaf area meter (Li-3000A; Li-Cor, Lincoln, NE, United States). The leaves were oven-dried for one week at 65°C, and dry biomass (LD, g) was determined. Leaf mass area (LMA, g cm–2) was calculated as LD/LA. Oven-dried foliar samples were ground to fine powder in a ball mill. Leaf mass-based nitrogen (N) concentration (Nmass, g kg–1) was measured using the Kjeldahl method (Bremner and Mulvaney, 1982). Leaf mass-based phosphorus (P) concentration (Pmass, g kg–1) was measured photometrically after samples were digested with H2SO4-H2O2 (Anderson and Ingram, 1989). Leaf area-based N concentration (Na, mg cm–2) was calculated as Nmass × LMA, and leaf area-based P concentration (Pa, mg cm–2) was calculated as Pmass × LMA.
Measurement of Anatomical Traits
Three leaves of each species from randomly selected individuals per chamber were measured for stomatal anatomical traits in June 2019 and 2020 (last 2 years), using image analysis software (OPTPro 2012 4.0, Optec XTS20, Chongqing Optec Instrument, China). Stomatal density (SD), guard cell width (W, μm) and length (L, μm) were also measured using the image analysis software. Stomatal size (SS, μm2) was defined as L * 2 * W (Franks and Beerling, 2009; Wu et al., 2018).
Soil Properties
We collected soils (0–10 cm) in the wet season (June) from 2015 to 2019. Soil cores (inner diameter: 3.5 cm) from each layer of each chamber were combined, homogenized. There were three replicates per treatments for each forest type. A soil subsample was air-dried before determination of pH, total N (TN, g kg–1), total P (TP, g kg–1), soil available N concentration (AN, mg kg–1) and soil available P concentration (AP, mg kg–1). Soil pH was measured at a soil-to-water ratio of 1:2.5. After digestion with concentrated sulfuric acid, TN was determined by the Kjeldahl method (Bremner and Mulvaney, 1982) and TP by an ultraviolet spectrophotometer (Lambda650/250, PE, United States) (Anderson and Ingram, 1989). After soil was extracted with 1 M KCl solution, AN was determined colorimetrically (Liu et al., 2017). AP was extracted with 0.03 M NH4F and 0.025 M HCl and measured by inductively coupled plasma optical emission spectrometer (ICP-OES) (Optima 2000 DV, Perkin Elmer, United States) (Bray and Kurtz, 1945).
Growth Measurements
The stem basal diameter (D, mm) and height (H, cm) of all seedlings per species in each chamber were measured in June (wet season) and December (dry season) from 2015 to 2019. The stem volume (V, cm3) of all seedlings per species in each chamber was also calculated as 3.14 * (D/2) ^ 2 * H.
Statistical Analysis
Data were assessed using Kolmogorov-Smironv test for normality and Levene’s test for homogeneity of variance prior to statistical analysis. When the data did not conform to the assumption of normality and homogeneity of variances, they were logarithmically transformed. A one-way ANOVA was used to evaluate warming effect on Tair, Tsoil, RH, SVWC, VPD, PAR and rainfall in the wet and dry seasons in the three forest types. Significant differences in foliar morphological, physiological traits and soil properties among the treatments were analyzed using a one-way ANOVA, followed by Tukey’s multiple comparison test. A repeated measures general liner model was used to evaluate the effects of warming and time on D2H for the four tree species. Data were analyzed using SPSS 24.0 (SPSS Inc., Chicago, IL, United States). The mixed liner model was used to analyze the main effects of environmental variables on the responses of photosynthetic capacity (Jmax and Vmax), gas exchange (Asat, gs, E and iWUE), biochemistry (concentrations of Chl(a+b), Na and Pa) and stomatal morphology (SD and SS). Differences were considered to be statistically significant at P < 0.05. Data were analyzed using R (R 3.5.3). We calculated the response ratio (RR) of AN and AP with warming (mean value measured in the warmed environment divided by the mean value measured in the control environment).
The relative importance of stomatal traits (gs, SD and SS), biochemistry [concentrations of Chl(a+b), Na and Pa], concentrations of soil nutrient availability (AN and AP) and soil types (lateritic soil and yellow soil) in explaining variations in photosynthesis (Jmax, Vmax and Asat), was determined using variation partitioning analysis (VPA) and redundancy analysis (RDA) using the “varpart” function in the “Vegan” package in R (R 3.5.3).
Results
Environmental Variables
Under translocation warming, Tair in CBMF at 30 m was 0.86 ± 0.07°C (wet season), 0.52 ± 0.02°C (dry season) and 0.68 ± 0.05°C (annual) higher compared to temperatures at 300 m, but they were not significantly different (Figure 2A). Tsoil in CBMF at 30 m was 1.35 ± 0.01°C (wet season) higher compared to temperature at 300 m, and although it was higher (0.68 ± 0.01°C) in the dry season, it was not significantly different (Figure 2B). Tair in MEBF at 300 m was 1.01 ± 0.01°C (wet season) and 0.95 ± 0.06°C (annual) higher, and at 30 m was 1.87 ± 0.08°C (wet season) and 1.63 ± 0.08°C (annual) higher compared to temperatures at 600 m. Tair trended higher at 300 m (0.78 ± 0.10°C) and 30 m (1.3 ± 0.08°C) compared to temperature at 600 m in the dry season, but it was not significantly different (Figure 2C). Tsoil in MEBF at 300 m was 0.78 ± 0.01°C (wet season) and 1.46 ± 0.01°C (dry season) higher, and at 30 m was 2.22 ± 0.01°C (wet season) and 2.50 ± 0.01°C (dry season) higher compared to temperatures at 600 m (Figure 2D). In MEBF and CBMF, the average monthly soil SVWC was significantly lower at 30 m compared to 600 and 300 m, in the wet and dry seasons (P < 0.05, Table 1).
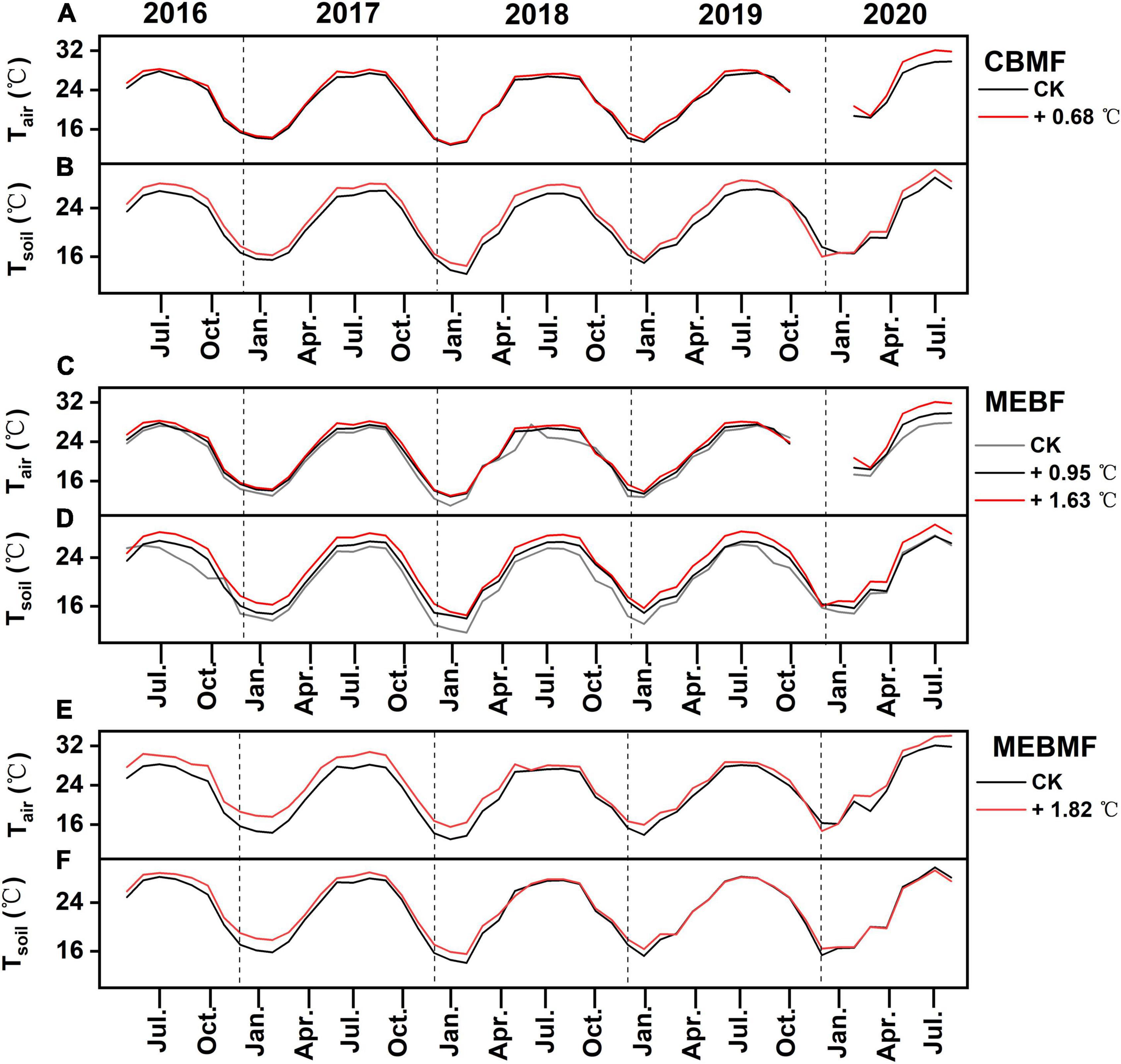
Figure 2. Monthly mean air temperature (Tair, °C) and monthly mean soil temperature (Tsoil, °C) at (A,B) coniferous and broadleaf mixed forest (CBMF), (C,D) mountain evergreen broadleaf forest (MEBF) and (E,F) monsoon evergreen broadleaf forest (MEBMF) from 2015 to 2020.
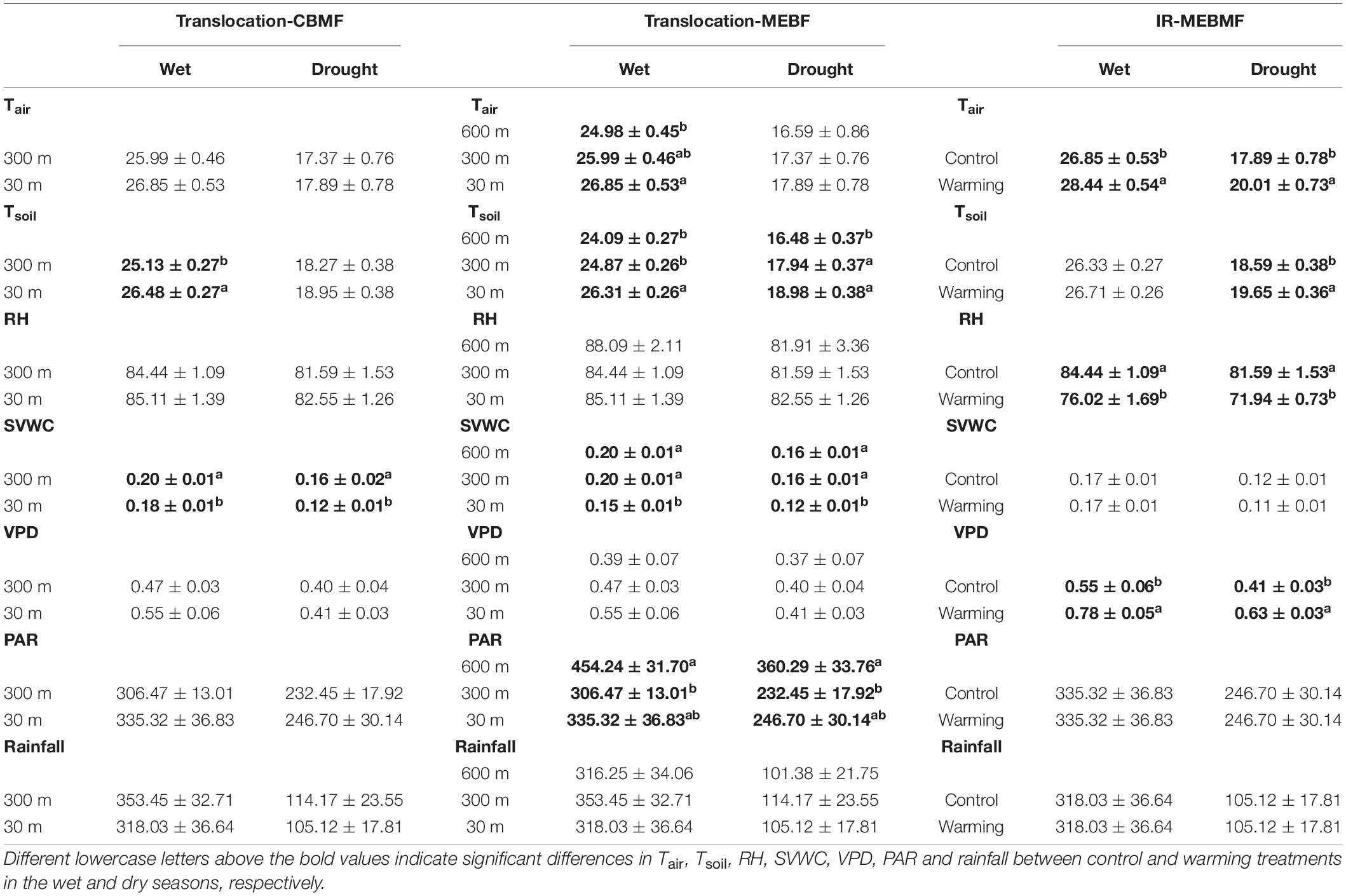
Table 1. The average monthly air temperature (Tair, °C, n = 24), soil temperature (Tsoil, °C, n = 72), air relative humidity (RH,%, n = 24), soil volumetric water content (SVWC, m3 m–3, n = 72), vapor pressure deficit (VPD, kPa, n = 24), photosynthetically active radiation (PAR, μmol m–2 s–1, n = 24) and rainfall (mm, n = 24) in the wet and dry seasons from 2016 to 2020 for coniferous and broadleaf forest (CBMF), mountain evergreen broadleaf forest (MEBF) and monsoon evergreen broadleaf forest (MEBMF).
During the last 5 years of the IR warming experiment, Tair in MEBMF in the warming treatment was 1.59 ± 0.01°C (wet season), 2.12 ± 0.05°C (dry season) and 1.82 ± 0.03°C (annual) higher compared to temperatures in the control plots (Figure 2E). Tsoil in the IR warming treatment was 1.06 ± 0.02°C (dry season) higher compared to temperature in the control plots, and although it trended higher (0.38 ± 0.01°C) in the wet season, it was not significantly different (Figure 2F). Infra-red warming treatments significantly reduced the average monthly air RH, and increased VPD, of MEBMF in the wet and dry seasons (P < 0.05, Table 1).
Physiological, Stomatal Anatomical and Plant Chemical Traits
In CBMF, 0.68°C of translocation warming induced higher gs for S. superba, but no effects on stomatal density and smaller stomatal size for M. breviflora and S. rehderianum (Figures 3A–C). The 0.68°C translocation warming treatment reduced transpiration of M. breviflora but enhanced that of S. superba (Figure 3D). Declining iWUE was observed in S. rehderianum and S. superba (Figure 3E). In MEBF, gs of M. breviflora was increased by 0.95°C of translocation warming, but that was reduced by 1.63°C of translocation warming. S. rehderianum and S. superba had higher gs under 0.95°C and 1.63°C of translocation warming (Figure 3F). Warming did not affect stomatal density for the three tree species (Figure 3G). Stomatal size of S. rehderianum was decreased by 1.63°C translocation warming (Figure 3H). Transpiration of M. breviflora was increased by 0.95°C translocation warming, but reduced by 1.63°C translocation warming. S. rehderianum and S. superba had higher transpiration under 0.95°C and 1.63°C translocation warming (Figure 3I). iWUE for the three tree species were not affected by warming (Figure 3J).
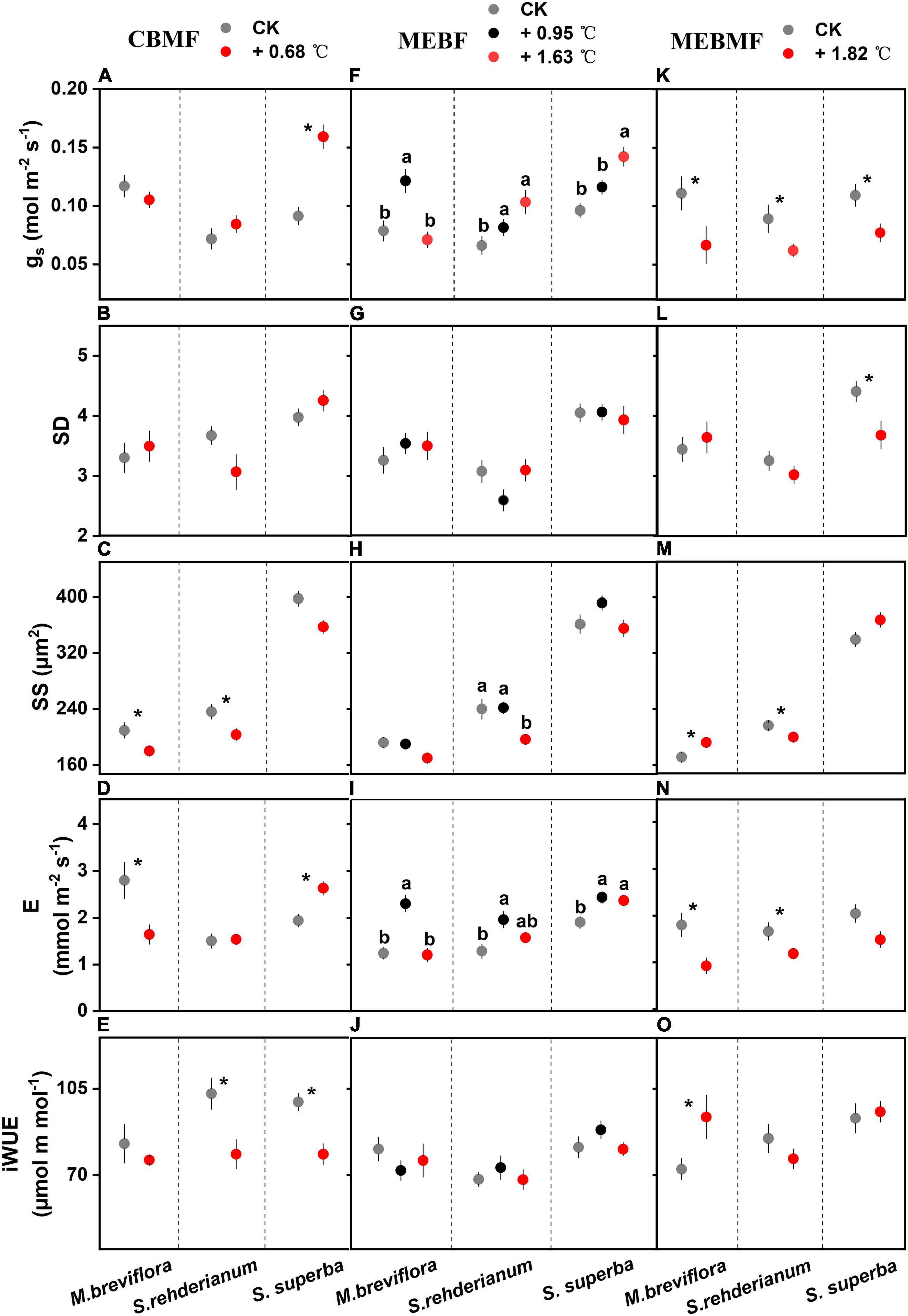
Figure 3. (A,F,K) Stomatal conductance (gs, mol m–2 s–1, n = 12), (B,G,L) stomatal density (SD, 10–4, n = 15), (C,H,M) stomatal size (SS, μm2, n = 50), (D,I,N) transpiration rate (E, mmol m–2 s–1, n = 12) and (E,J,O) instantaneous water use efficiency (iWUE, μmol m mol–1, n = 12) of Machilus breviflora, Syzygium rehderianum and Schima superba exposed to various warming treatments in coniferous and broadleaf mixed forest (CBMF), mountain evergreen broadleaf forest (MEBF) and monsoon evergreen broadleaf forest (MEBMF). Different lowercase letters above the error bars (standard errors) indicate significant differences in gs, SD, SS, E and iWUE from August 2018 or 2019 to August 2020.
In MEBMF, gs of M. breviflora, S. rehderianum and S. superba were reduced by the 1.82°C IR warming treatment (Figure 3K). Schima superba exhibited reduced stomatal density under 1.82°C of IR warming (Figure 3L). The 1.82°C IR warming treatment enhanced stomatal size for M. breviflora, but decreased that of S. rehderianum (Figure 3M). Decreasing transpiration had been observed in M. breviflora and S. rehderianum under the 1.82°C IR warming treatment (Figure 3N), but iWUE of M. breviflora was enhanced by 1.82°C of IR warming (Figure 3O).
In CBMF, S. rehderianum had higher Chl(a+b) concentration in the 0.68°C translocation warming treatment (Figure 4A). Na for the three tree species were not affected by translocation warming (Figure 4B). Pa of S. superba was enhanced by 0.68°C of translocation warming (Figure 4C). In MEBF, the concentrations in Chl(a+b), Na and Pa for M. breviflora, S. rehderianum and S. superba were not affected by warming (Figures 4D–F). In MEBMF, warming exerted no effects on the concentrations in Chl(a+b) and Na for the three tree species (Figures 4G,H). In MEBMF, Pa of S. superba was decreased by 1.82°C of IR warming (Figure 4I).
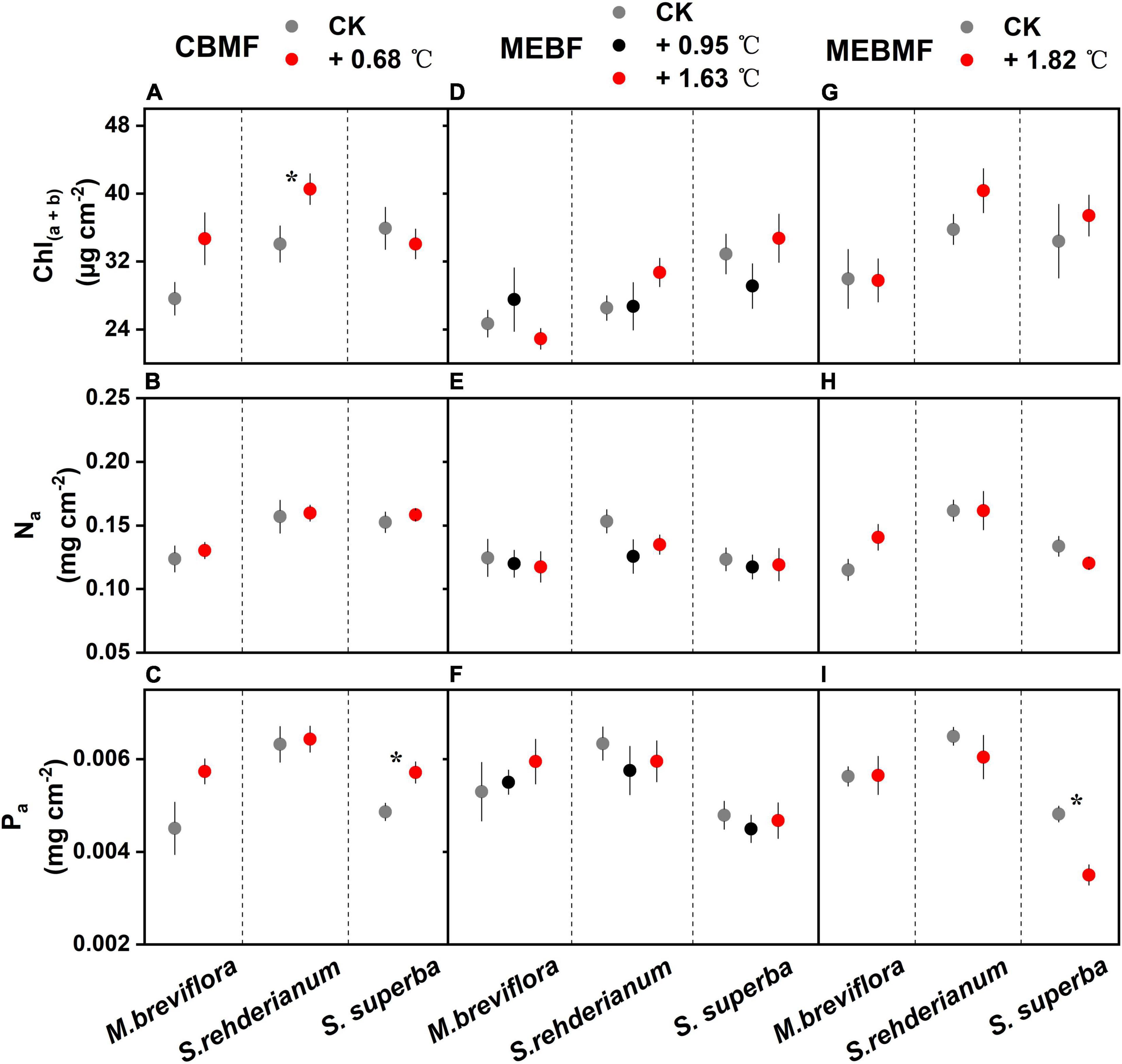
Figure 4. (A,D,G) The total concentrations of chlorophyll a and chlorophyll b [Chl(a+b), μg cm–2, n = 6], (B,E,H) the area-based nitrogen concentrations in the leaves (Na, mg cm–2, n = 6) and (C,F,I) area-based phosphorus concentrations in the leaves (Pa, mg cm–2, n = 6) of Machilus breviflora, Syzygium rehderianum and Schima superba exposed to various warming treatments in coniferous and broadleaf mixed forest (CBMF), mountain evergreen broadleaf forest (MEBF) and monsoon evergreen broadleaf forest (MEBMF). Different lowercase letters above the error bars (standard errors) indicate significant differences in Chl(a+b), Na and Pa from August 2018 or 2019 to August 2020.
Photosynthetic Capacity and Light-Saturated Rates
In CBMF, Jmax and Vmax of S. superba in the 0.68°C translocation warming treatment were significantly increased by 106.2% and 43.6%, respectively (Figures 5A,B). The 0.68 °C translocation warming treatment did not affect Jmax/Vmax for M. breviflora, S. rehderianum and S. superba (Figure 5C). The 0.68°C translocation warming treatment decreased Asat of M. breviflora by 26.9%, but increased that of S. superba by 33.4% (Figure 5D). In MEBF, Jmax for the three tree species were not influenced by warming (Figure 5E). Vmax of S. superba under the 1.63°C translocation warming treatment was enhanced by 56.1% (Figure 5F). The 1.63°C translocation warming treatment decreased Jmax/Vmax for S. superba (Figure 5G). The 0.95°C translocation warming treatment enhanced Asat of M. breviflora by 43.9%, but the 1.63°C translocation warming treatment decreased Asat by 17.4%. The 0.95°C and 1.63°C translocation warming treatments enhanced Asat of S. rehderianum and S. superba by approximately 40.0% (Figure 5H).
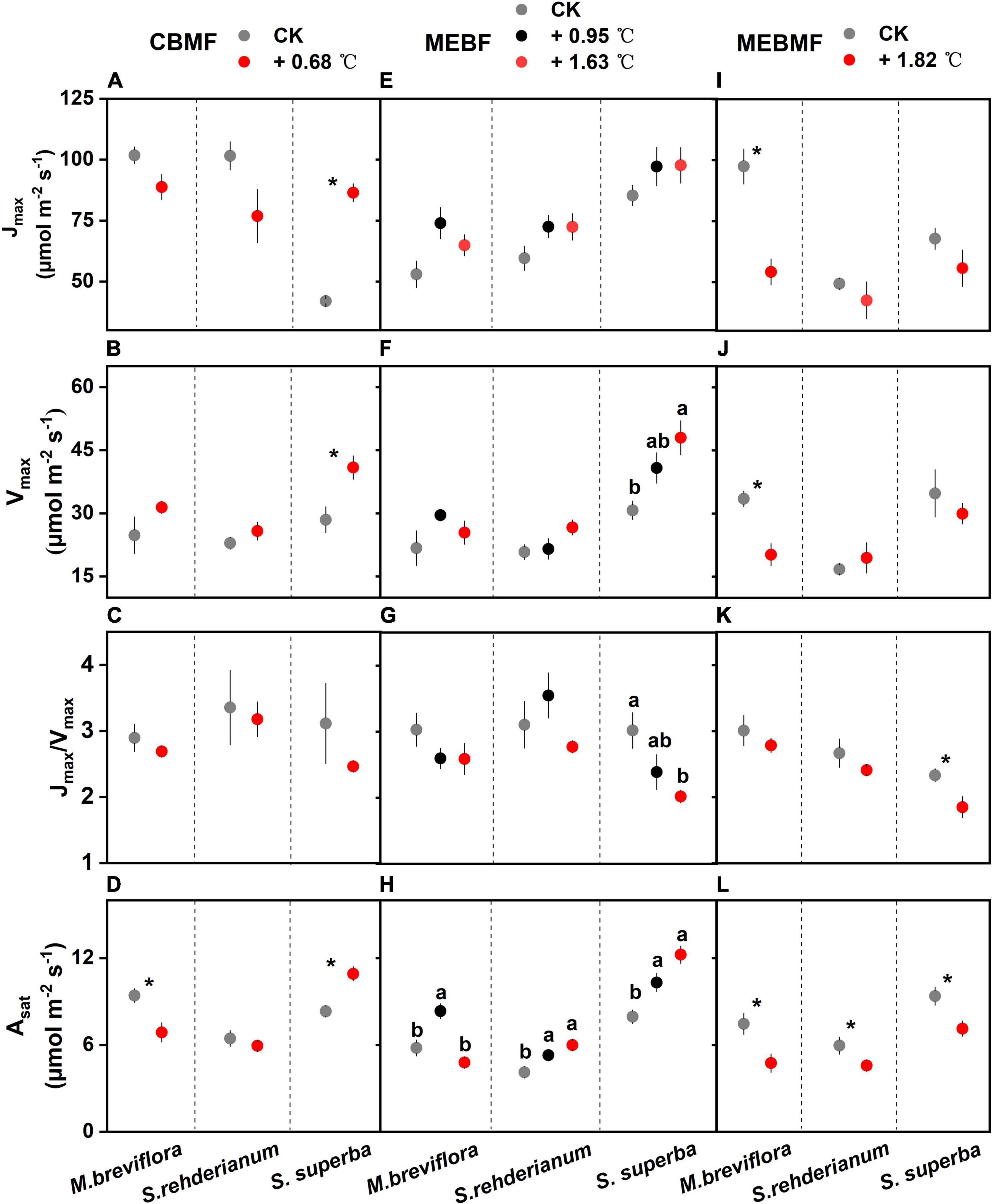
Figure 5. (A,E,I) The area-based maximum rate of photosynthetic electron transport (Jmax, μmol m–2 s–1, n = 6), (B,F,J) the area-based maximum rate of photosynthetic Rubisco carboxylation (Vmax, μmol m–2 s–1, n = 6), (C,G,K) the ratio of the area-based maximum rate of photosynthetic electron transport and area-based maximum rate of photosynthetic Rubisco carboxylation (Jmax/Vmax, n = 6) and (D,H,L) the light-saturated photosynthetic rate (Asat, μmol m–2 s–1, n = 12) of Machilus breviflora, Syzygium rehderianum and Schima superba exposed to various warming treatments in coniferous and broadleaf mixed forest (CBMF), mountain evergreen broadleaf forest (MEBF) and monsoon evergreen broadleaf forest (MEBMF). Different lowercase letters above the error bars (standard errors) indicate significant differences in Jmax, Vmax, Jmax/Vmax and Asat from August 2018 or 2019 to August 2020.
In MEBMF, 1.82°C of IR warming significantly reduced Jmax and Vmax of M. breviflora by 34.4% and 26.1%, respectively (Figures 5I,J). Lower Jmax/Vmax for S. superba was observed in MEBMF (Figure 5K). Asat of M. breviflora, S. rehderianum and S. superba in the 1.82°C IR warming treatment were decreased by 36.3%, 23.1%, and 23.8%, respectively (Figure 5L).
The Soil Types, Soil Chemistry and Nutrient Availability in the Three Forest Types
The soil type of CBMF and MEBMF was lateritic soil, and that of MEBF was yellow soil. The concentrations of TN and TP, and pH for CBMF, MEBF and MEBMF were not significantly influenced by warming (Table 2). In CBMF, AN was significantly increased by 0.68°C of translocation warming, while AP showed decreasing trends to warming (Supplementary Figure 1A). In contrast, the 0.95°C and 1.63°C translocation warming treatments in MEBF significantly decreased AN but had no significant effects on AP (Supplementary Figure 1B). In MEBMF, AN and AP were not significantly affected by IR warming (Supplementary Figure 1C).
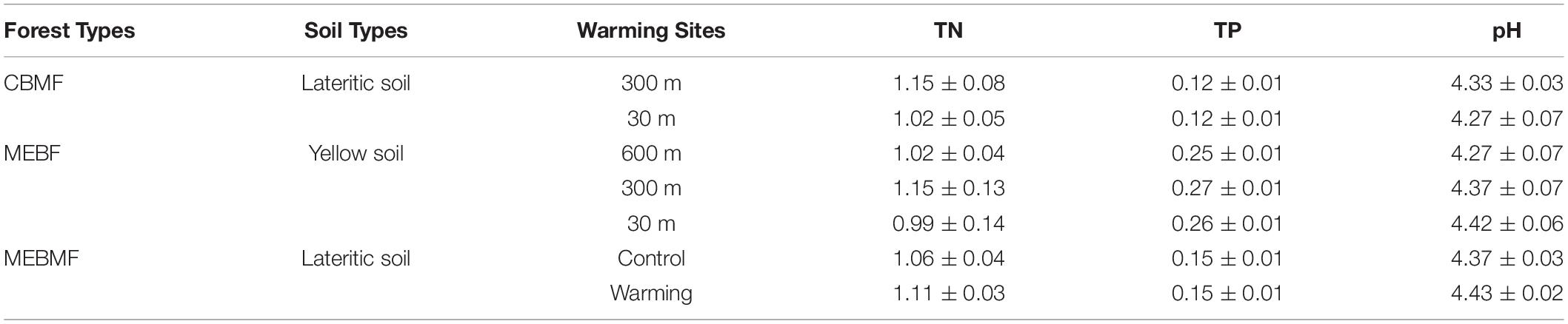
Table 2. The soil types, concentrations of total nitrogen (TN, g kg–1, n = 9) and phosphorus (TP, g kg–1, n = 9), and pH in the substrate soil (0–10 cm) in the wet season from 2017 to 2019 for coniferous and broadleaf forest (CBMF), mountain evergreen broadleaf forest (MEBF) and monsoon evergreen broadleaf forest (MEBMF).
Impacts of Environmental Variables on Physiology Under Translocation and Infra-Red Warming Treatments
In the translocation warming treatment, lower Asat for M. breviflora was mainly driven by higher Tsoil and lower SVWC. Stomatal size of M. breviflora was significantly decreased by higher Tair. Higher Tair significantly increased gs for S. rehderianum, but decreased its stomatal size. The enhancements in photosynthetic capacity and gas exchange for S. superba were significantly driven by higher Tair and Tsoil (Table 3, P < 0.05).
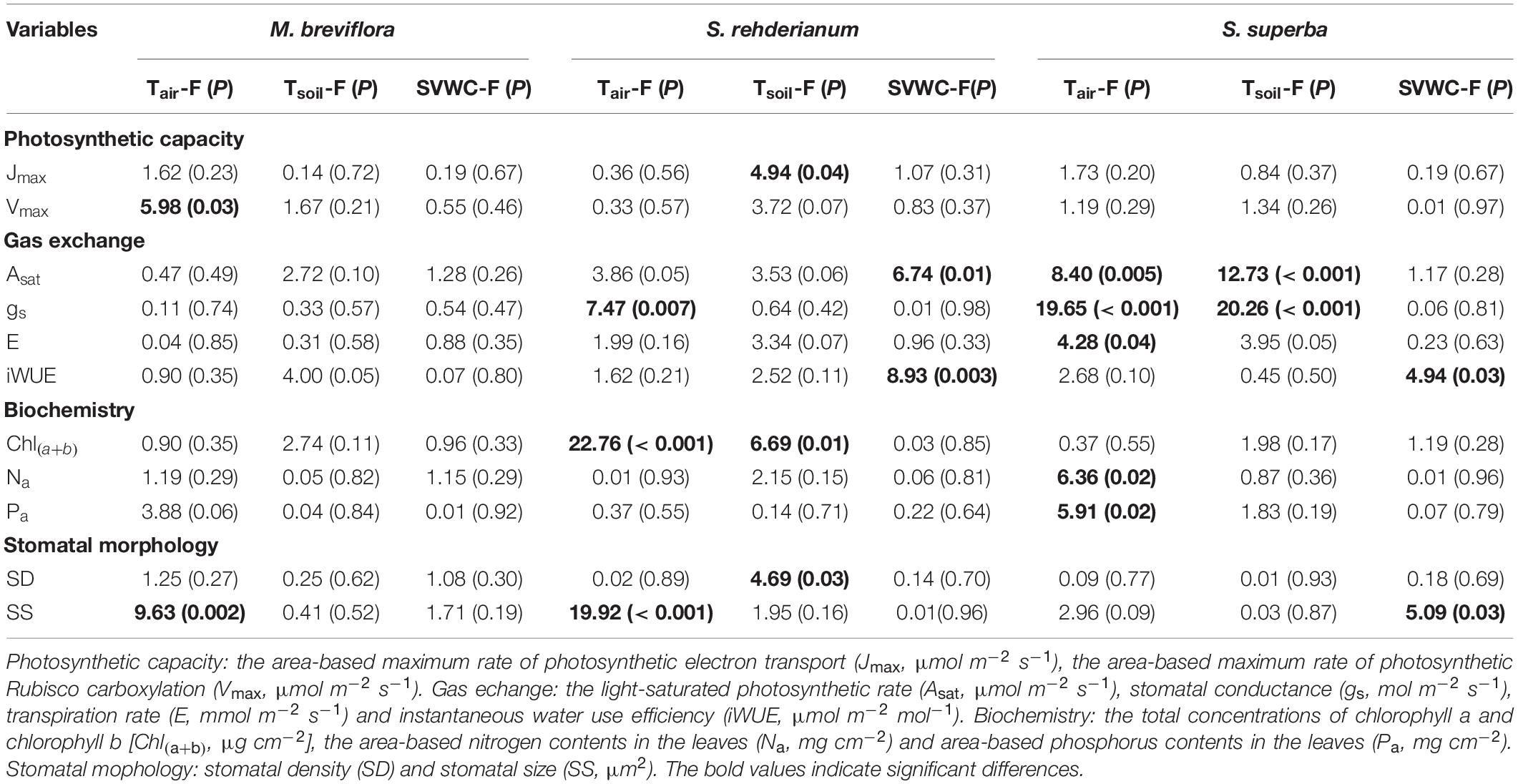
Table 3. Summary report of effects of air temperature (Tair, °C), soil temperature (Tsoil, °C) and soil volumetric water content (SVWC, m3 m–3) on photosynthetic capacity, gas exchange, biochemistry for Machilus breviflora, Syzygium rehderianum, and Schima superba in last 2 or 3 years under translocation warming by the mixed liner model.
In the 1.82°C IR warming treatment, lower RH significantly decreased gas exchange for M. breviflora. Stomatal size of M. breviflora was significantly increased by higher Tair and lower RH. gs of S. rehderianum and S. superba, and stomatal size of S. rehderianum were mainly reduced by increasing Tair. Transpiration of S. rehderianum was decreased by higher Tair and VPD. The reduction in Pa for S. superba was driven by Tair, RH and VPD. Stomatal density of S. superba was significantly decreased by declining RH and increasing VPD (Table 4, P < 0.05).
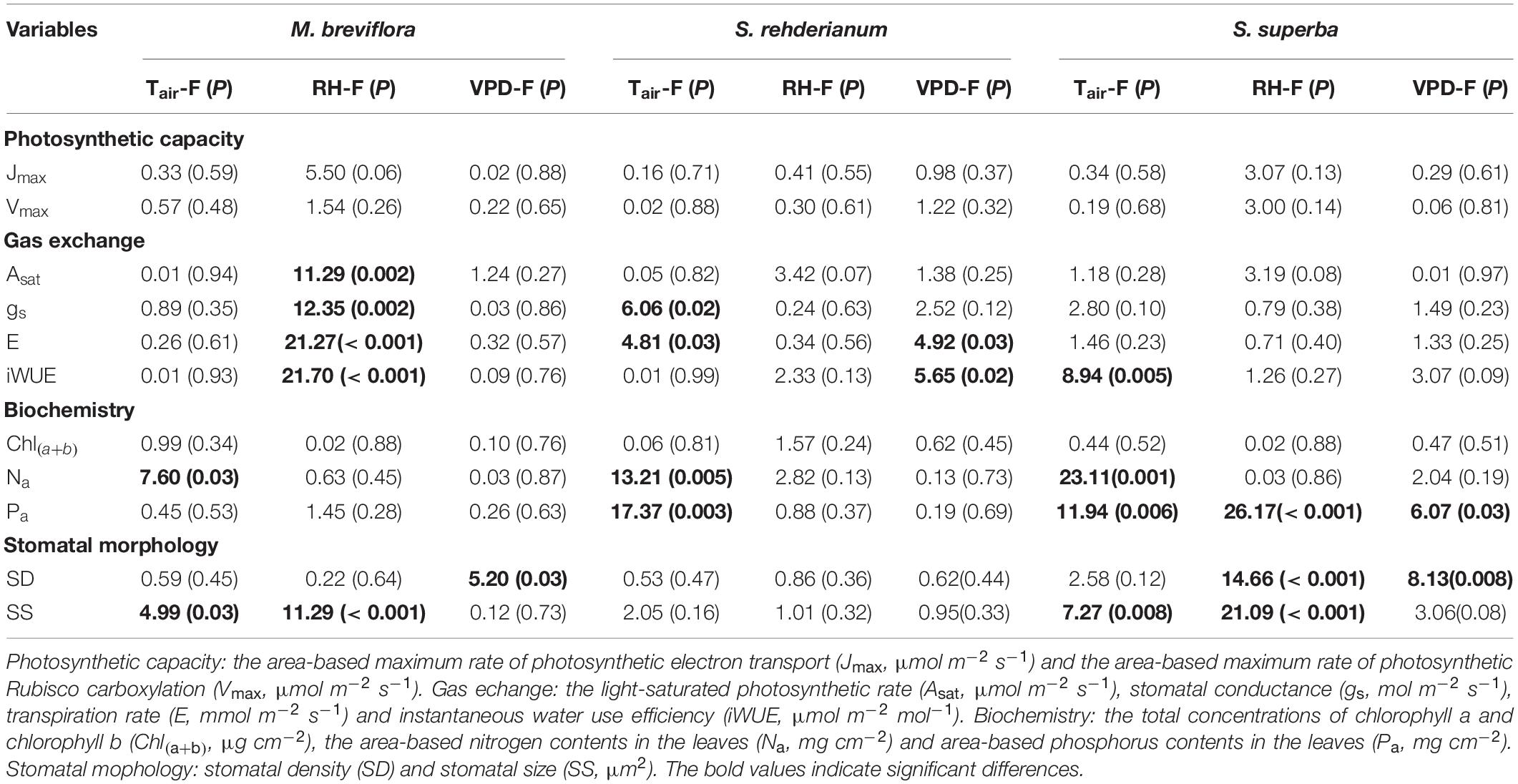
Table 4. Summary report of effects of air temperature (Tair, °C), air moisture (RH,%) and vapor pressure difference (VPD, kPa) on photosynthetic capacity, gas exchange, biochemistry and stomatal morphology for Machilus breviflora, Syzygium rehderianum, and Schima superba in last 2 or 3 years under infrared warming by the mixed liner model.
The Effects of Stomatal Traits, Biochemistry, Nutrient Availability and Soil Types on Photosynthesis Traits for the Three Tree Species Between Coniferous and Broadleaf Mixed Forest and Mountain Evergreen Broadleaf Forest
The change in photosynthesis trait for M. breviflora between CBMF and MEBF was mainly correlated with soil type, stomatal traits and nutrient availability, and their ratios were 43%, 26%, and 16%, respectively (Supplementary Figure 2A). The soil type explained the 10% changes in photosynthesis trait for S. rehderianum between CBMF and MEBF (Supplementary Figure 2B). Biochemistry was main factor inducing the shift in photosynthesis trait for S. superba between CBMF and MEBF (Supplementary Figure 2C).
Growth
In CBMF, the 0.68°C translocation warming treatment reduced stem volume of M. breviflora and S. rehderianum by 6.2% and 6.3%, respectively (Figures 6A,B). The stem volume of S. superba was increased by 117.8% (Figure 6C). In MEBF, the 1.63°C translocation warming treatment significantly decreased the stem volume of M. breviflora by 54.9% (Figure 6D). The 0.95°C and 1.63°C translocation warming treatments significantly increased the stem volume of S. rehderianum in MEBF by 26.5% and 106.5%, respectively (Figure 6E). The 0.95°C and 1.63°C translocation warming treatment significantly enhanced stem volume in MEBF by 33.5% and 119.7% (Figure 6F).
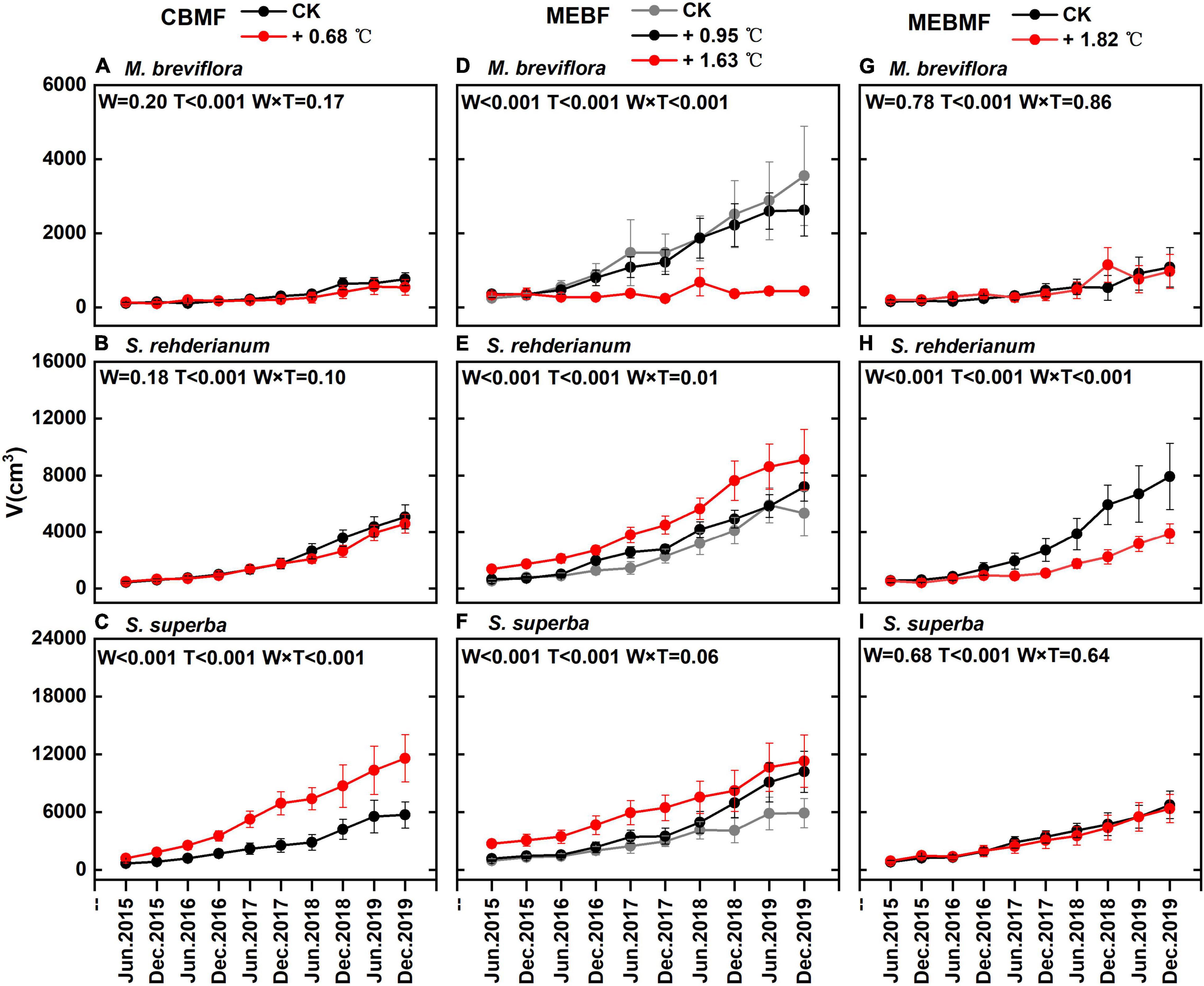
Figure 6. (A,D,G) The stem volume (V, cm3) of Machilas breviflora in coniferous and broadleaf mixed forest (CBMF), mountain evergreen broadleaf forest (MEBF) and monsoon evergreen broadleaf forest (MEBMF), from June 2015 to December 2019; (B,E,H) V of Syzygium rehderianum in CBMF, MEBF and MEBMF; (C,F,I) V of Schima superba in CBMF, MEBF and MEBMF.
In MEBMF, the 1.82°C IR warming treatment reduced stem volume of S. rehderianum by 42.8% (Figure 6H). The 1.82°C IR warming treatment had no significant effect on the stem volume of M. breviflora and S. superba (Figures 6G,I).
Discussion
We designed our experiment to increase Tair and Tsoil in a subtropical forest using two common techniques – translocation and IR warming– but which also differentially affected other environmental variables, including SVWC, RH and VPD. We found that the warming methods differed in their impacts on the physical environment. In general, both methods directly increased Tair and Tsoil (although to varying degrees), while translocation warming indirectly dried the soil (lower SVWC) and IR warming indirectly dried the air (lower RH and higher VPD). This study highlighted the direct and indirect effects of different warming techniques on the physical environment of forest ecosystems, and subsequently, the complex and variable biological impacts on trees. Hence, different warming techniques may provide different outcomes when assessing the impact of warming on trees in future climates.
The Impacts of Translocation and Infra-Red Warming on the Physical Environment
Translocation experiments utilize natural temperature gradients to increase Tair and Tsoil (Dunne et al., 2004; Salinas et al., 2011), but may indirectly reduce soil moisture, leading to lower SVWC (Hu et al., 2016; Fang et al., 2020). Although some studies have analyzed the impact of few environmental variables on biological traits in response to translocation treatments, few have investigated the full suite of physical environmental variables and subsequent impacts on biological traits (Budge et al., 2011; Li et al., 2016; Soderberg et al., 2020; Lie et al., 2021).
The IR heaters directly increased Tair and Tsoil in the dry (but not wet) season in MEBMF. The IR heaters also dried the air, leading to lower RH and higher VPD, as has been observed in other studies, with observations of some impacts on the plants (Amthor et al., 2010; de Boeck et al., 2012). However, most studies using IR heaters did not address the potential impacts of the underlying indirect effects of reduced RH and increased VPD, although it was biological relevant (Aronson and McNulty, 2009). Overall, the main difference between the two warming methods was that translocation warming indirectly dried the soil, while IR warming indirectly dried the air.
Direct Effects of Higher Tair and Tsoil on Plant Physiology and Growth
The 0.68°C (CBMF) and 1.63°C (MEBF) of translocation warming decreased Asat for M. breviflora in the wet season, inhibiting its growth. In contrast, translocation warming increased Asat for S. rehderianum in the wet season in MEBF, and increased Jmax, Vmax and Asat for S. superba in the wet season in CBMF and MEBF, leading to more rapid growth. M. breviflora in CBMF exhibited smaller stomatal size in response to 0.68°C of translocation warming, which may have contributed to lower Asat (Zhu et al., 2020). Asat for M. breviflora in CBMF and MEBF were mainly reduced by the direct effect of higher Tsoil, subsequently leading to lower water uptake from the soil, and lower gs. Higher Tair directly increased gs of S. rehderianum in MEBF, leading to increasing Asat and growth (Dai et al., 2021). However, growth of S. rehderianum in CBMF was lower due to translocation warming. The differential responses of photosynthesis for S. rehderianum in the CBMF and MEBF sites may be attributable to warming impacts on soil types and nutrient availability. S. rehderianum exhibited smaller stomatal size in response to increasing Tair caused by translocation and IR warming, which would be beneficial for lowering transpiration, as has been observed previously (Parkhurst, 1994; Pellizzari et al., 2017; Wu et al., 2020a). Jmax, Vmax, and Asat for S. superba were increased by higher Tair and Tsoil in both translocation warming sites. Tair at the translocation warming sites might be lower than Topt of S. superba, which enhanced its photosynthetic capacity and rate due to increasing gs (Way and Yamori, 2014; Slot and Winter, 2017). Higher Tsoil could increase water uptake to promote leaf water supply, thereby increasing gs for S. superba, resulting in increased photosynthetic capacity and rate (Wu et al., 2012; Harrison et al., 2020; Wu et al., 2020b). Increased Tair induced by translocation warming resulted in higher transpiration for S. superba, which might be used to cool leaves (Drake et al., 2018).
IR warming reduced Asat for S. rehderianum and S. superba in the wet season in MEBMF. gs of S. rehderianum and S. superba in the wet season in MEBMF was mainly reduced by the direct effect of higher Tair under 1.82°C of IR warming, when Tair might exceed its Topt (Taylor et al., 2019), which reduced Asat. Additionally, S. rehderianum and S. superba under 1.82°C of IR warming might have limited capacity to alter Topt to acclimate to higher Tair (Carter et al., 2021), resulting in declining gs and Asat. Thus, the 1.82°C IR warming treatment significantly decreased growth for S. rehderianum in MEBMF due to declining Asat.
Indirect Effects of Soil Volumetric Water Content, Relative Humidity, and Vapor Pressure Deficit on Plant Physiology and Growth
Translocation warming indirectly reduced SVWC due to higher Tsoil. Lower SVWC may decrease soil water availability, thereby increasing stomatal limitation to Asat for M. breviflora in the wet season, and inhibiting its growth (Zhang et al., 2020). Similar to our results, Reich et al. (2018) found that warming impacts on photosynthesis was dependent on SVWC. In contrast, growth of M. breviflora was enhanced by translocation warming in MEBF, when SVWC was higher, leading to higher Asat.
IR heaters indirectly reduced RH and increased VPD. The reductions in Jmax, Vmax and Asat for M. breviflora in the wet season in MEBMF were attributable to indirect effect of declining RH rather than increasing Tair under 1.82°C of IR warming, which was also related to decreased gs due to high stomatal sensitivity to dry air (Kitao et al., 2014). Xiao et al. (2020) also found that reduced gs was driven by declining RH instead of increasing Tair. Higher Tair and lower RH both enhanced stomatal size for M. breviflora, which might be beneficial for increasing transpirational cooling to reduce leaf temperature in warmer environments (Miserere et al., 2021). Stomatal density for S. superba was decreased by the indirect effects of higher RH and lower VPD, which could reduce Asat and water loss, similar to previous observations (Du et al., 2019; Fanourakis et al., 2020; Miserere et al., 2021). The IR warming treatment reduced leaf Pa concentration for S. superba due to lower transpiration, which further inhibited photosynthesis (Aliniaeifard and van Meeteren, 2013; Leon-Sanchez et al., 2019; Shrestha et al., 2021).
Conclusion
We found that biological responses to warming, in three tropical tree species, differed between translocation and IR warming due to differential impacts of the warming technique on the physical environment, including indirect effects on SVWC, RH, and VPD. Hence, different warming techniques may provide different outcomes when assessing the impact of warming on trees in future climates. Importantly, future experiments should directly compare the warming techniques at the same sites and in the same soils to test our findings. Subsequently, we should monitor a full suite of environmental variables in warming experiments to determine the mechanistic basis for the biological responses to warming, which will increase our predictive capacity regarding the impact of climate change on forests.
Data Availability Statement
Data used in this study are publicly available at Figshare (https://figshare.com/s/0269e551f98cb926450b).
Author Contributions
TW, DT, and JL wrote the draft. GZ, JY, YL, XT, SL, GC, and ZM helped design the warming experiment. MZ revised the draft. QY provided experimental equipment. XL and YS helped finished field experiment. All authors contributed to the article and approved the submitted version.
Funding
This work was supported by the Key Research and Development Program of Guangdong Province (2020B1111530004), National Natural Science Foundation of China (Grant Nos. 41991285, 41977287, and 41825020), China Postdoctoral Science Foundation (E11512BH01), and Guangdong provincial finance special for forestry project.
Conflict of Interest
The authors declare that the research was conducted in the absence of any commercial or financial relationships that could be construed as a potential conflict of interest.
Publisher’s Note
All claims expressed in this article are solely those of the authors and do not necessarily represent those of their affiliated organizations, or those of the publisher, the editors and the reviewers. Any product that may be evaluated in this article, or claim that may be made by its manufacturer, is not guaranteed or endorsed by the publisher.
Supplementary Material
The Supplementary Material for this article can be found online at: https://www.frontiersin.org/articles/10.3389/ffgc.2022.877025/full#supplementary-material
Supplementary Figure 1 | (A) The response ratio (RR) of available N (AN) and available P (AP) in the substrate soil (0–10 cm) in the wet season from 2015/2016 to 2019 for (A) coniferous and broadleaf forest (CBMF), (B) mountain evergreen broadleaf forest (MEBF) and (C) monsoon evergreen broadleaf forest (MEBMF).
Supplementary Figure 2 | Variation partitioning analysis (VPA) showing the effects of stomatal traits, biochemistry, nutrient availability and soil types on photosynthesis traits for Machilas breviflora (A), Syzygium rehderianum (B) and Schima superba (C) between coniferous and broadleaf mixed forest (CBMF) and mountain evergreen broadleaf forest (MEBF). Photosynthesis traits are represented by the area-based maximum rate of photosynthetic electron transport (Jmax), the area-based maximum rate of photosynthetic Rubisco carboxylation (Vmax), and the light-saturated photosynthetic rate (Asat). Stomatal traits contain stomatal conductance (gs), stomatal density (SD) and size (SS). Biochemistry includes the concentrations of chlorophyll a and b (Chl(a+b)), and the concentrations of nitrogen and phosphorus in leaves (Na and Pa). Nutrient availability contains nitrogen availability (AN) and phosphorus availability (AP). Soil types contain lateritic soil and yellow soil.
Supplementary Table 1 | Some data in this paper were previously published, but have been provided in this paper to support the comprehensive study of different warming techniques at different sites.
References
Aliniaeifard, S., and van Meeteren, U. (2013). Can prolonged exposure to low VPD disturb the ABA signalling in stomatal guard cells? J. Exp. Bot. 64, 3551–3566. doi: 10.1093/jxb/ert192
Amthor, J. S., Hanson, P. J., Norby, R. J., and Wullschleger, S. D. (2010). A comment on “appropriate experimental ecosystem warming methods by ecosystem, objective, and particality” by Aronson and McNulty. Agr. Forest. Meteorol 150, 497–498. doi: 10.1016/j.agrformet.2009.11.020
Anderson, J. M., and Ingram, J. (1989). Tropical Soil Biology And Fertility. Wallingford: CAB international.
Arnon, D. I. (1949). Copper enzymes in isolated chloroplasts-polyphenoloxidase in beta vulgaris. Plant Physiol. 24, 1–15. doi: 10.1104/pp.24.1.1
Aronson, E. L., and McNulty, S. G. (2009). Appropriate experimental ecosystem warming methods by ecosystem, objective, and practicality. Agr. Forest. Meteorol. 149, 1791–1799. doi: 10.1016/j.agrformet.2009.06.007
Booth, W. (1988). Johnny appleseed and the greenhouse. Science 242, 19–20. doi: 10.1126/science.242.4875.19
Bray, R. H., and Kurtz, L. T. (1945). Determination of total, organic, and available forms of phosphorus in soils. Soil Sci. 59, 39–45. doi: 10.1097/00010694-194501000-00006
Bremner, J., and Mulvaney, C. (1982). Nitrogen-total methods of soilanalysis, part 2, chemical and microbiological properties, 2ndEdn Edn. Madison: American Society of Agronomy. Inc.
Budge, K., Leifeld, J., Egli, M., and Fuhrer, J. (2011). Soil microbial communities in (sub)alpine grasslands indicate a moderate shift towards new environmental conditions 11 years after soil translocation. Soil Biol Biochem. 43, 1148–1154. doi: 10.1016/j.soilbio.2011.02.001
Carter, K. R., Wood, T. E., Reed, S. C., Butts, K. M., and Cavaleri, M. A. (2021). Experimental warming across a tropical forest canopy height gradient reveals minimal photosynthetic and respiratory acclimation. Plant Cell Env. 44, 2879–2897. doi: 10.1111/pce.14134
Cavaleri, M. A., Reed, S. C., Smith, W. K., and Wood, T. E. (2015). Urgent need for warming experiments in tropical forests. Global Change Biol. 21, 2111–2121. doi: 10.1111/gcb.12860
Cunningham, S. C., and Reed, J. (2002). Comparison of temperate and tropical rainforest tree species: photosynthetic responses to growth temperature. Oecologia 133, 112–119. doi: 10.1007/s00442-002-1034-1
Dai, L. L., Xu, Y. S., Harmens, H., Duan, H. L., Feng, Z. Z., Hayes, F., et al. (2021). Reduced photosynthetic thermal acclimation capacity under elevated ozone in poplar (Populus tremula) saplings. Global Change Biol. 27, 2159–2173. doi: 10.1111/gcb.15564
Day, M. E. (2000). Influence of temperature and leaf-to-air vapor pressure deficit on net photosynthesis and stomatal conductance in red spruce (Picea rubens). Tree Physiol. 20, 57–63. doi: 10.1093/treephys/20.1.57
de Boeck, H. J., and Nijs, I. (2011). An alternative approach for infrared heater control in warming and extreme event experiments in terrestrial ecosystems. J. Ecol. 99, 724–728. doi: 10.1111/j.1365-2745.2011.01799.x
de Boeck, H. J., Kimball, B. A., Miglietta, F., and Nijs, I. (2012). Quantification of excess water loss in plant canopies warmed with infrared heating. Global Change Biol. 18, 2860–2868. doi: 10.1111/j.1365-2486.2012.02734.x
De Frenne, P., De Schrijver, A., Graae, B. J., Gruwez, R., Tack, W., Vandelook, F., et al. (2010). The use of open-top chambers in forests for evaluating warming effects on herbaceous understorey plants. Ecol. Res. 25, 163–171. doi: 10.1007/s11284-009-0640-3
Drake, J. E., Tjoelker, M. G., Aspinwall, M. J., Reich, P. B., Pfautsch, S., and Barton, C. V. M. (2018). The partitioning of gross primary production for young Eucalyptus tereticornis trees under experimental warming and altered water availability. New Phytol. 222, 1298–1312. doi: 10.1111/nph.15629
Du, Q. J., Liu, T., Jiao, X. C., Song, X. M., Zhang, J. Y., and Li, J. M. (2019). Leaf anatomical adaptations have central roles in photosynthetic acclimation to humidity. J. Exp. Bot. 70, 4949–4961. doi: 10.1093/jxb/erz238
Dunne, J. A., Saleska, S. R., Fischer, M. L., and Harte, J. (2004). Integrating experimental and gradient methods in ecological climate change research. Ecology 85, 904–916. doi: 10.1890/03-8003
Dusenge, M. E., Madhavji, S., and Way, D. A. (2020). Contrasting acclimation responses to elevated CO2 and warming between an evergreen and a deciduous boreal conifer. Global Change Biol. 26, 3639–3657. doi: 10.1002/ecs2.2311
Fang, X., Zhou, G. Y., Qu, C., Huang, W. J., Zhang, D. Q., Li, Y. L., et al. (2020). Translocating subtropical forest soils to a warmer region alters microbial communities and increases the decomposition of mineral-associated organic carbon. Soil Biol Biochem 142:107707. doi: 10.1016/j.soilbio.2020.107707
Fanourakis, D., Aliniaeifard, S., Sellin, A., Giday, H., Korner, O., Nejad, A. R., et al. (2020). Stomatal behavior following mid- or long-term exposure to high relative air humidity: a review. Plant Physiol Bioch. 153, 92–105. doi: 10.1016/j.plaphy.2020.05.024
Franks, P. J., and Beerling, D. J. (2009). Maximum leaf conductance driven by CO2 effects on stomatal size and density over geologic time. Proc. Natl. Acad. Sci. U.S.A. 106, 10343–10347. doi: 10.1073/pnas.0904209106
Grossiord, C., Buckley, T. N., Cernusak, L. A., Novick, K. A., Poulter, B., Siegwolf, R. T. W., et al. (2020). Plant responses to rising vapor pressure deficit. New Phytol. 226, 1550–1566. doi: 10.1111/nph.16485
Harrison, J. L., Sanders-DeMott, R., Reinmann, A. B., Sorensen, P. O., Phillips, N. G., and Templer, P. H. (2020). Growing-season warming and winter soil freeze/thaw cycles increase transpiration in a northern hardwood forest. Ecology 101:e03173. doi: 10.1002/ecy.3173
Hu, Y. G., Wang, Q., Wang, S. P., Zhang, Z. H., Dijkstra, F. A., Zhang, Z. S., et al. (2016). Asymmetric responses of methane uptake to climate warming and cooling of a Tibetan alpine meadow assessed through a reciprocal translocation along an elevation gradient. Plant Soil. 402, 263–275.
Kimball, B. A., Alonso-Rodriguez, A. M., Cavaleri, M. A., Reed, S. C., Gonzalez, G., and Wood, T. E. (2018). Infrared heater system for warming tropical forest understory plants and soils. Ecol. Evol. 8, 1932–1944. doi: 10.1002/ece3.3780
Kimball, B. A., Conley, M. M., Wang, S., Lin, X., Luo, C., Morgan, J., et al. (2008). Infrared heater arrays for warming ecosystem field plots. Global Change Biol. 14, 309–320. doi: 10.1111/j.1365-2486.2007.01486.x
Kitao, M., Komatsu, M., Hoshika, Y., Yazaki, K., Yoshimura, K., Fujii, S., et al. (2014). Seasonal ozone uptake by a warm-temperate mixed deciduous and evergreen broadleaf forest in western Japan estimated by the penman-monteith approach combined with a photosynthesis-dependent stomatal model. Env. Pollut. 184, 457–463. doi: 10.1016/j.envpol.2013.09.023
Leon-Sanchez, L., Nicolas, E., Prieto, I., Nortes, P., Maestre, F. T., Querejeta, I., et al. (2019). Altered leaf elemental composition with climate change is linked to reductions in photosynthesis, growth and survival in a semi-arid shrubland. J. Ecol. 108, 47–60. doi: 10.1111/1365-2745.13259
Li, Y. Y., Liu, J. X., Zhou, G. Y., Huang, W. J., and Duan, H. L. (2016). Warming effects on photosynthesis of subtropical tree species: a translocation experiment along an altitudinal gradient. Sci. Rep. 6:24895. doi: 10.1038/srep24895
Li, Y. Y., Zhou, G. Y., and Liu, J. X. (2017). Different growth and physiological responses of six subtropical tree species to warming. Front. Plant. Sci. 8:1511. doi: 10.3389/fpls.2017.01511
Lie, Z. Y., Huang, W. J., Liu, X. J., Zhou, G. Y., Yan, J. H., Li, Y. L., et al. (2021). Warming leads to more closed nitrogen cycling in nitrogen-rich tropical forests. Global Change Biol. 27, 664–674. doi: 10.1111/gcb.15432
Liu, J. X., Liu, S. G., Li, Y. Y., Liu, S. Z., Yin, G. C., Huang, J., et al. (2017). Warming effects on the decomposition of two litter species in model subtropical forests. Plant Soil. 420, 277–287. doi: 10.1007/s11104-017-3392-9
Luan, J. W., Liu, S. R., Chang, S. X., Wang, J. X., Zhu, X. L., Liu, K., et al. (2014). Different effects of warming and cooling on the decomposition of soil organic matter in warm-temperate oak forests: a reciprocal translocation experiment. Biogeochemistry 121, 551–564. doi: 10.1007/s10533-014-0022-y
Luo, C. Y., Xu, G. P., Chao, Z. G., Wang, S. P., Lin, X. W., Hu, Y. G., et al. (2010). Effect of warming and grazing on litter mass loss and temperature sensitivity of litter and dung mass loss on the tibetan plateau. Global Change Biol. 16, 1606–1617. doi: 10.1111/j.1365-2486.2009.02026.x
McDaniel, M. D., Wagner, R. J., Rollinson, C. R., Kimball, B. A., Kaye, M. W., and Kaye, J. P. (2013). Microclimate and ecological threshold responses in a warming and wetting experiment following whole tree harvest. Theor. Appl. Climatol. 116, 287–299. doi: 10.1007/s00704-013-0942-9
Miserere, A., Rousseaux, M. C., Ploschuk, E. L., Brizuela, M. M., Curcio, M. H., Zabaleta, R., et al. (2021). Effects of prolonged elevated temperature on leaf gas exchange and other leaf traits in young olive trees. Tree Physiol. 41, 254–268. doi: 10.1093/treephys/tpaa118
Natali, S. M., Schuur, E. A. G., Trucco, C., Hicks Pries, C. E., Crummer, K. G., and Baron Lopez, A. F. (2011). Effects of experimental warming of air, soil and permafrost on carbon balance in alaskan tundra. Global Change Biol. 17, 1394–1407. doi: 10.1111/j.1365-2486.2010.02303.x
Nottingham, A. T., Whitaker, J., Ostle, N. J., Bardgett, R. D., McNamara, N. P., Fierer, N., et al. (2019). Microbial responses to warming enhance soil carbon loss following translocation across a tropical forest elevation gradient. Ecol. Lett. 22, 1889–1899. doi: 10.1111/ele.13379
Parkhurst, D. F. (1994). Diffusion of CO2 and other gases inside leaves. New Phytol. 126, 449–479. doi: 10.1111/j.1469-8137.1994.tb04244.x
Pellizzari, E., Camarero, J. J., Gazol, A., Granda, E., Shetti, R., Wilmking, M., et al. (2017). Diverging shrub and tree growth from the polar to the mediterranean biomes across the European continent. Global Change Biol. 23, 3169–3180. doi: 10.1111/gcb.13577
Reich, P. B., Sendall, K. M., Stefanski, A., Rich, R. L., Hobbie, S. E., and Montgomery, R. A. (2018). Effects of climate warming on photosynthesis in boreal tree species depend on soil moisture. Nature 562, 263–267. doi: 10.1038/s41586-018-0582-4
Restaino, C. M., Peterson, D. L., and Littell, J. (2016). Increased water deficit decreases Douglas fir growth throughout western US forests. Proc. Natl. Acad. Sci. U.S.A. 113, 9557–9562. doi: 10.1073/pnas.1602384113
Rich, R. L., Stefanski, A., Montgomery, R. A., Hobbie, S. E., Kimball, B. A., and Reich, P. B. (2015). Design and performance of combined infrared canopy and belowground warming in the B4WarmED (boreal forest warming at an ecotone in danger) experiment. Global Change Biol. 21, 2334–2348. doi: 10.1111/gcb.12855
Salinas, N., Malhi, Y., Meir, P., Silman, M., Roman Cuesta, R., Huaman, J., et al. (2011). The sensitivity of tropical leaf litter decomposition to temperature: results from a large-scale leaf translocation experiment along an elevation gradient in Peruvian forests. New Phytol. 189, 967–977. doi: 10.1111/j.1469-8137.2010.03521.x
Shaver, G. R., Canadell, J., Chapin, F. S., Gurevitch, J., Harte, J., Henry, G., et al. (2000). Global warming and terrestrial ecosystems: a conceptual framework for analysis. Bioscience 50, 871–882. doi: 10.1371/journal.pbio.1002281
Shrestha, R. K., Lei, P., Shi, D., Hashimi, M. H., Wang, S., Xie, D. T., et al. (2021). Response of maize (zea mays L.) towards vapor pressure deficit. Environ. Exp. Bot 181:104293. doi: 10.1016/j.envexpbot.2020.104293
Slot, M., and Winter, K. (2017). In situ temperature response of photosynthesis of 42 tree and liana species in the canopy of two Panamanian lowland tropical forests with contrasting rainfall regimes. New Phytol. 214, 1103–1117. doi: 10.1111/nph.14469
Soderberg, D. N., Mock, K. E., Hofstetter, R. W., and Bentz, B. J. (2020). Translocation experiment reveals capacity for mountain pine beetle persistence under climate warming. Ecol. Monogr. 91:e01437. doi: 10.1002/ecm.1437
Stinziano, J. R., and Way, D. A. (2017). Autumn photosynthetic decline and growth cessation in seedlings of white spruce are decoupled under warming and photoperiod manipulations. Plant Cell Environ. 40, 1296–1316. doi: 10.1111/pce.12917
Strand, M., Lundmark, T., Soderbergh, I., and Mellander, P. E. (2002). Impacts of seasonal air and soil temperatures on photosynthesis in Scots pine trees. Tree Physiol. 22, 839–847. doi: 10.1093/treephys/22.12.839
Taylor, T. C., Smith, M. N., Slot, M., and Feeley, K. J. (2019). The capacity to emit isoprene differentiates the photosynthetic temperature responses of tropical plant species. Plant Cell Env. 42, 2448–2457. doi: 10.1111/pce.13564
Wan, S., Luo, Y., and Wallace, L. L. (2002). Changes in microclimate induced by experimental warming and clipping in tallgrass prairie. Global Change Biol. 8, 754–768. doi: 10.1046/j.1365-2486.2002.00510.x
Wang, W. Z., Jia, M., Wang, G. X., Zhu, W. Z., and McDowell, N. G. (2017). Rapid warming forces contrasting growth trends of subalpine fir (Abies fabri) at higher- and lower-elevations in the eastern tibetan plateau. Forest Ecol. Manag. 402, 135–144. doi: 10.1016/j.foreco.2017.07.043
Way, D. A., and Yamori, W. (2014). Thermal acclimation of photosynthesis: on the importance of adjusting our definitions and accounting for thermal acclimation of respiration. Photosynth. Res. 119, 89–100. doi: 10.1007/s11120-013-9873-7
Wertin, T. M., McGuire, M. A., and Teskey, R. O. (2012). Effects of predicted future and current atmospheric temperature and CO2 and high and low soil moisture on gas exchange and growth of Pinus taeda seedlings at cool and warm sites in the species range. Tree Physiol. 32, 847–858. doi: 10.1093/treephys/tps051
Wu, G. L., Liu, H., Hua, L., Luo, Q., Lin, Y. X., He, P. C., et al. (2018). Differential responses of stomata and photosynthesis to elevated temperature in two co-occurring subtropical forest tree species. Front. Plant. Sci. 9:467. doi: 10.3389/fpls.2018.00467
Wu, S. H., Jansson, P. E., and Kolari, P. (2012). The role of air and soil temperature in the seasonality of photosynthesis and transpiration in a boreal Scots pine ecosystem. Agr. Forest. Meteorol. 156, 85–103. doi: 10.1016/j.agrformet.2012.01.006
Wu, T., Liu, S. Z., Lie, Z. Y., Zheng, M. H., Duan, H. L., Chu, G. W., et al. (2020a). Divergent effects of a 6-year warming experiment on the nutrient productivities of subtropical tree species. Forest. Ecol. Manag 461:117952. doi: 10.1016/j.foreco.2020.117952
Wu, T., Tissue, D. T., Li, X., Liu, S. Z., Chu, G. W., Zhou, G. Y., et al. (2020b). Long-term effects of 7-year warming experiment in the field on leaf hydraulic and economic traits of subtropical tree species. Global Change Biol. 26, 7144–7157. doi: 10.1111/gcb.15355
Xiao, M. Z., Yu, Z. B., Kong, D. D., Gu, X. H., Mammarelia, I., Montagnani, L., et al. (2020). Stomatal response to decreased relative humidity constrains the acceleration of terrestrial evapotranspiration. Env. Res. Lett. 15:094066. doi: 10.1088/1748-9326/ab9967
Zhang, Y., Parazoo, N. C., Williams, A. P., Zhou, S., and Gentine, P. (2020). Large and projected strengthening moisture limitation on end-of-season photosynthesis. Proc. Natl. Acad. Sci. U.S.A. 117, 9216–9222. doi: 10.1073/pnas.1914436117
Zhou, G. Y., Wei, X. H., Wu, Y. P., Liu, S. G., Huang, Y. H., Yan, J. H., et al. (2011). Quantifying the hydrological responses to climate change in an intact forested small watershed in Southern China. Global Change Biol. 17, 3736–3746. doi: 10.1111/j.1365-2486.2011.02499.x
Zhou, H. R., Xu, M., Hou, R. X., Zheng, Y. P., Chi, Y. G., and Ouyang, Z. (2018). Thermal acclimation of photosynthesis to experimental warming is season-dependent for winter wheat (Triticum aestivum L.). Env. Exp. Bot. 150, 249–259. doi: 10.1016/j.envexpbot.2018.04.001
Keywords: environmental variables, long-term field warming, infra-red warming, translocation warming, physiological plasticity, subtropical forest, tree growth
Citation: Wu T, Tissue DT, Zhou G, Yan J, Zheng M, Li X, Song Y, Li Y, Tang X, Liu S, Chu G, Meng Z, Ye Q and Liu J (2022) Direct and Indirect Effects of Long-Term Field Warming Methods on the Physical Environment and Biological Responses in a Subtropical Forest. Front. For. Glob. Change 5:877025. doi: 10.3389/ffgc.2022.877025
Received: 16 February 2022; Accepted: 06 May 2022;
Published: 09 June 2022.
Edited by:
Kuno Kasak, University of Tartu, EstoniaReviewed by:
Leonardo Montagnani, Free University of Bozen-Bolzano, ItalyJacqueline E. Mohan, University of Georgia, United States
Copyright © 2022 Wu, Tissue, Zhou, Yan, Zheng, Li, Song, Li, Tang, Liu, Chu, Meng, Ye and Liu. This is an open-access article distributed under the terms of the Creative Commons Attribution License (CC BY). The use, distribution or reproduction in other forums is permitted, provided the original author(s) and the copyright owner(s) are credited and that the original publication in this journal is cited, in accordance with accepted academic practice. No use, distribution or reproduction is permitted which does not comply with these terms.
*Correspondence: Juxiu Liu, ljxiu@scbg.ac.cn
†ORCID: Ting Wu, orcid.org/0000-0001-6045-036X; David Thomas Tissue, orcid.org/0000-0002-8497-2047; Guoyi Zhou, orcid.org/0000-0002-5667-7411; Juxiu Liu, orcid.org/0000-0001-7850-1006