Global Patterns and Drivers of Litter Decomposition Under Nitrogen Enrichment: A Meta-Analysis
- 1College of Grassland Science, Shanxi Agriculture University, Jinzhong, China
- 2Shanxi Key Laboratory of Grassland Ecological Protection and Native Grass Germplasm Innovation, Shanxi Agriculture University, Jinzhong, China
- 3State Key Laboratory of Desert and Oasis Ecology, Xinjiang Institute of Ecology and Geography, Chinese Academy of Sciences, Ürümqi, China
- 4Beijing Key Laboratory of Farmland Soil Pollution Prevention and Remediation, College of Resources and Environmental Sciences, China Agricultural University, Beijing, China
Nitrogen (N) enrichment has substantially altered patterns of terrestrial litter decomposition, with positive, neutral, and negative effects. However, the general response patterns and drivers of litter decomposition to N enrichment rates are poorly understood, and how litter decomposition has changed under the N enrichment rate, especially in different ecosystems, still requires further study. We reviewed 118 published papers dealing with litter mass remaining after N enrichment to assess the influences of various environmental and experimental factors on the relationships between N enrichment and litter decomposition in grasslands, forests, and wetland ecosystems. The results indicated that N enrichment had an insignificant effect on litter decomposition globally. However, the effects varied greatly among ecosystem types, with an increase in litter decomposition of 3.91% in grasslands and 1.82% in wetlands and a decrease of 1.23% in forests. When forests were subdivided into plantations, primary, and secondary forests, the results showed that N enrichment significantly slowed litter decomposition rate by 2.96% in plantations but had no significant influence in primary and secondary forests. However, litter decomposition was significantly influenced by the level of N addition in plantations and secondary forests, with an increase in litter mass loss at low N addition (50 kg N ha–1 year–1) and a decrease in litter mass loss at high N addition (>50 kg N ha–1 year–1). The magnitude and direction of the N effect are affected by experimental and environmental factors. Specifically, mixed N enrichment (for example, urea and glycine) exerted a stronger effect on litter decomposition compared with an N fertilizer alone. Our findings indicated the different effects of N on litter decomposition in forests and grasslands and knowledge which will greatly advance our ability to accurately evaluate and predict global C cycling under increased N deposition, which should improve future models of global biogeochemical cycling.
Introduction
Plant litter decomposition in terrestrial ecosystems contributes to substantial annual global carbon (C) fluxes and nutrient cycling (Ciais et al., 2013). It has been estimated that the release of CO2 due to litter decomposition accounts for 70% of the global annual C flux (Raich and Schlesinger, 1992). Ambient nitrogen (N) deposition, mainly resulting from fossil fuel combustion, urbanization, and N fertilizer input, is considered a crucial contributor to global climate change (Ciais et al., 2013). Global N deposition has increased 3–5-folds since the Industrial Revolution and is projected to increase continuously in the future (Lamarque et al., 2005). Enhanced atmospheric N deposition has been reported to significantly affect litter decomposition processes and disrupt the global C balance and nutrient cycling (Knorr et al., 2005; van Diepen et al., 2015). Even minor changes in soil N availability caused by N deposition will have tremendous impacts on global C fluxes and nutrient cycling due to the vast C stocks (70–150 Pg) in plant litter (Schimel, 1995). Therefore, a quantitative evaluation of the influence of N enrichment on global litter decomposition processes is crucial to better predict future ecosystem C and nutrient budgets (See et al., 2019).
Numerous studies have reported the effect of N enrichment on litter decomposition in recent years (Hobbie, 2000; Allison et al., 2009; van Diepen et al., 2015; Hou et al., 2020). However, these studies have reached conflicting conclusions, showing that N enrichment can either accelerate, inhibit, or have no detectable effect on litter decomposition processes (Hobbie, 2000; Song et al., 2019; Hou et al., 2020). These conflicting results may be due to differences in a variety of factors, including litter quality, N application rates and forms, ambient N deposition, experimental durations, and climate variables (Knorr et al., 2005). Chen et al. (2015) showed that although litter decomposition was stimulated by low N enrichment, it was inhibited when N enrichment was high. In contrast, Mo et al. (2006) and Fang et al. (2007) concluded that the litter decomposition rate was accelerated and inhibited during the early and late stages of N enrichment, respectively. The mechanisms explaining the influences of these various factors on the relationship between N enrichment and litter decomposition remain unclear. The numerous conflicting results have prevented a general understanding of global litter decomposition in response to N enrichment. A meta-analysis of previous studies presents an effective means of identifying the general responses and patterns of litter decomposition to N enrichment.
Numerous previous studies have found that litter quality can determine the effect of N enrichment on litter decomposition processes (Vivanco and Austin, 2011; Averill and Waring, 2018; Argiroff et al., 2019; See et al., 2019; Hou et al., 2020). The N enrichment has generally been found to increase the decomposition of high-quality litter, i.e., with high N and low lignin concentrations (Hobbie et al., 2012; Zhang and Wang, 2012; Hou et al., 2020), but decrease the decomposition of poorer quality litter with high lignin concentrations (Carreiro et al., 2000; Knorr et al., 2005). Knorr et al. (2005) estimated that the N enrichment significantly stimulated the decomposition of litter with a low lignin content (<10%), had insignificant effects on litter with a medium lignin content (10–20%), and significantly inhibited the decomposition of litter with high lignin content. These results are consistent with a previous report that the rapid decomposition of cellulose, but the accumulation of lignin, inhibited litter decomposition during the later stages of decomposition (Hobbie et al., 2012). Therefore, a better understanding of the interactive effects of litter quality and N enrichment on litter decomposition is crucial to the prediction of litter decomposition rates following N enrichment.
Grasslands, wetlands, and plantations play crucial roles in the global terrestrial C sink (Gray and Fierer, 2012; Wang et al., 2019a; Hong et al., 2020; Yu et al., 2020a). Plantations comprise ∼80% of the forest C sink in China (Hong et al., 2020). Changes in land use from plantations to mature forests have significantly affected soil moisture levels and plant diversity (Zhou et al., 2013; Wang et al., 2019a), decreased the C:N ratio and lignin content of litter, and accelerated litter decomposition (Huang et al., 2011). In addition, Yu et al. (2020b) reported that the increased area under primary forest and the insignificant decrease in plantation coverage have resulted in differential responses of soil C accumulation to N enrichment. The inconsistent results of these various studies have limited the accurate evaluation of C cycling processes in forest ecosystems, and a better understanding of litter decomposition responses to N enrichment in different ecosystems is extremely important to accurately assess and predict the global C balance.
Previously, only two meta-analyses have investigated litter decomposition under N enrichment, with both studies being limited in scope (Knorr et al., 2005; Zhang et al., 2018). Knorr et al. (2005) showed that N enrichment resulted in an insignificant decrease in litter decomposition in forests in Europe and the United States. In contrast, Zhang et al. (2018) showed that N enrichment affected litter decomposition differently in different biomes, with positive impacts on secondary forests, no impacts on primary forests, wetlands, and grasslands, and negative impacts on plantations. Results from Knorr et al. (2005) indicated that N enrichment had no significant effect on litter decay when averaging overall studies. But litter decomposition was significantly inhibited (−5%) at low levels of N enrichment (<75 kg N ha–1 year–1), significantly accelerated (+17%) under medium N enrichment (75–125 kg N ha–1 year–1), and was significantly and strongly inhibited (−9%) at high N enrichment (>125 kg N ha–1 year–1). Overall, Knorr et al. (2005) showed that litter decomposition followed an inverted U-shaped curve in response to increasing N enrichment, while, Zhang et al. (2018) found that litter decomposition followed a linear response to increasing N enrichment, with a greater inhibitory effect at high N enrichment levels. These contrasting results hinder our ability to understand the general patterns and drivers of litter decomposition under nitrogen enrichment on a global scale. We took a broader view compared with these previous analyses and considered N enrichment forms, ambient N deposition, mesh size of the litter decomposition sampling bags, litter lignin content, and ambient humidity. Furthermore, N enrichment rates were examined in grasslands, wetlands, and forests. Consequently, our study represents a critical and comprehensive evaluation of the impacts of N enrichment on global litter decomposition. In addition, our database was more extensive, and a global synthesis was performed to investigate the effects of a wider range of environmental and experimental factors on the effects of N enrichment on litter decomposition, including forest, wetland, and grassland ecosystems, to enable the most comprehensive evaluation of the impacts of experimental and environmental factors to date.
Our study particularly set out to (1) identify the global pattern of litter decomposition in response to N enrichment and (2) determine how experimental and environmental factors influenced litter decomposition as a result of N enrichment.
Materials and Methods
Data Collection
Data were obtained from peer-reviewed journals presented on the Web of Science and the China National Knowledge Infrastructure (CNKI). We selected publications containing the keywords “N enrichment,” “N fertilization,” “N application,” “N input,” or “N deposition” together with “litter decay,” “litter decomposition,” “litter mass remaining,” or “litter mass loss.” The following criteria were used to collate data from the studies detected: (1) data were collected only from N addition experiments; (2) data were collected under the same experimental conditions; (3) the litter decay rate (mean and standard error), as well as sample sizes, must have been reported; (4) in studies that investigated enrichment with other nutrients in addition to N, only the control and N enrichment group data were extracted; and (5) the type or rate of N enrichment were regarded as independent observations (Zhang et al., 2018; Deng et al., 2019). In total, 3,215 observations from 118 papers were included in our study.
We investigated the impacts of various factors on the response of litter mass decomposition to N enrichment, including ecosystem type (forests, grasslands, and wetlands), N treatments, and experimental period. Sufficient data on forests allowed the data to be subdivided according to community succession stage: plantations, primary forests, and secondary forests. The N application forms were grouped as follows: (1) CO(NH2)2; (2) NH4NO3; (3) NH4+; (4) NO3–; and (5) mixed N, for example, urea and glycine. The N addition rates were classified as either <50, 50–150, and >150 kg N ha–1 year–1. The data were further classified according to experimental duration (<6, 6–12, 13–24, >24 months); annual ambient N deposition in grasslands (<10, 10–20, 20–30, >30 kg N⋅ha–1 year–1); annual ambient N deposition in forests (<30, 30–40, 40 kg N⋅ha–1 year–1) and ratio of N enrichment rate:ambient N deposition in grasslands (<5, 5–10, >10) and ratio of N enrichment rate:ambient N deposition in forests (<5, 5–10, >10); and humidity index (HI) (≤30, 30–60, >60, where HI = MAP/(MAT+10) (mean annual precipitation and mean annual temperature, respectively). Litter types were grouped by lignin content, with <10, 10–20, and >20% corresponding to low, medium, and high litter-quality groups, respectively (Knorr et al., 2005). Exclusion methods include physical exclusion classified as coarse (≥1 mm mesh litterbag) and fine (<1 mm mesh litterbag). The data for N enrichment rates investigated in the current study ranged from 2.4 to 640 kg N⋅ha–1 year–1, and ambient N deposition ranged between 2 and 97.5 kg N⋅ha–1 year–1. The duration of litter decomposition ranged from 0.5 to 72 months. MAT ranged from −5.3 to 26.6°C, and MAP ranged from 150 to 5,100 mm.
The environmental factor data included in the current study, such as ambient N deposition, N enrichment rate, MAT, MAP, and geographical locations (Supplementary Figure 1), were obtained from the published data. All data were extracted from tables or figures and digitized into graph form using the GetData Graph Digitizer.1
Meta-Analysis
The response ratio (RR) of individual observations was calculated as treatment (Xt) divided by control (Xc) (Xia and Wan, 2008; Wang et al., 2019b; Sha et al., 2020):
In Equation (1), RR is the response ratio representing the percentage change; Xt represents the mean value of the litter mass remaining after N enrichment; and Xc represents the mean value of the litter mass remaining in control plots (without N enrichment).
The variance (v) was calculated using the following equation:
In Equation (2), nt and nc indicate the number of sample replications for treatments and controls, respectively. St and Sc represent standard deviations (SDs). When SDs were not stated in the publication, the SDs were calculated from the standard error (SE):
The weighting factor (w) for each RR was calculated as follows:
Since some data in the papers did not include variance, weighting by sample sizes was implemented, following previous studies (Peng et al., 2017; Mao et al., 2020; Sha et al., 2020):
The weighted effect size ln(RR′) was obtained by the following equation:
Finally, the mean variance-weighted effect size was applied to evaluate the total impacts of N enrichment on litter decay rate by the following equation:
where and wi are the lnRR’ and w of the ith observation, respectively.
Percentage change was obtained by the following equation:
All calculations were performed using MetaWin (version 2.1).2 The N enrichment was regarded to have significantly affected litter mass remaining after N enrichment if the 95% confidence intervals (CIs) did not overlap with zero. In addition, groups were considered to be significantly different from each other if the 95% CIs of each group did not overlap (Sha et al., 2020). A positive and negative percentage change in comparison with the control group represents deceleration and acceleration of litter decomposition due to N enrichment, respectively.
Statistical Analysis
The humidity index was taken as the climatic factor influencing the effects of N enrichment on litter mass remaining, following Deng et al. (2019), and was categorized as follows: (1) 0–30; (2) 30–60; and (3) >60. General linear regressions were used to evaluate the relationships between percentage change in litter mass remaining after N enrichment and the various factors, including the level of N enrichment, ambient N deposition, MAT, MAP, HI, and litter nutrients. All relevant analyses and graphs were performed using Origin (version 9.0).3
Results
The Effects of N Enrichment on Litter Mass Remaining
Averaging the data overall, studies indicated that N enrichment did not significantly affect litter mass loss after N enrichment. As shown in Figure 1, the effects of N enrichment on litter decomposition varied greatly among different ecosystem types. The N enrichment significantly accelerated the loss of litter mass by 3.91 and 1.82% in grasslands and wetlands, respectively, whereas, it significantly decelerated the loss of litter mass by 1.18% in forests. Furthermore, although N enrichment decelerated litter decay by 2.96% in plantations, there were no significant effects in primary and secondary forests. In addition, although N enrichment significantly altered litter decomposition, the magnitude and direction of the effects were regulated by litter quality, N enrichment rate, N enrichment form, experimental duration, ambient N deposition, litterbag mesh size, ecosystem type, and climate variables (Supplementary Figures 3–8).
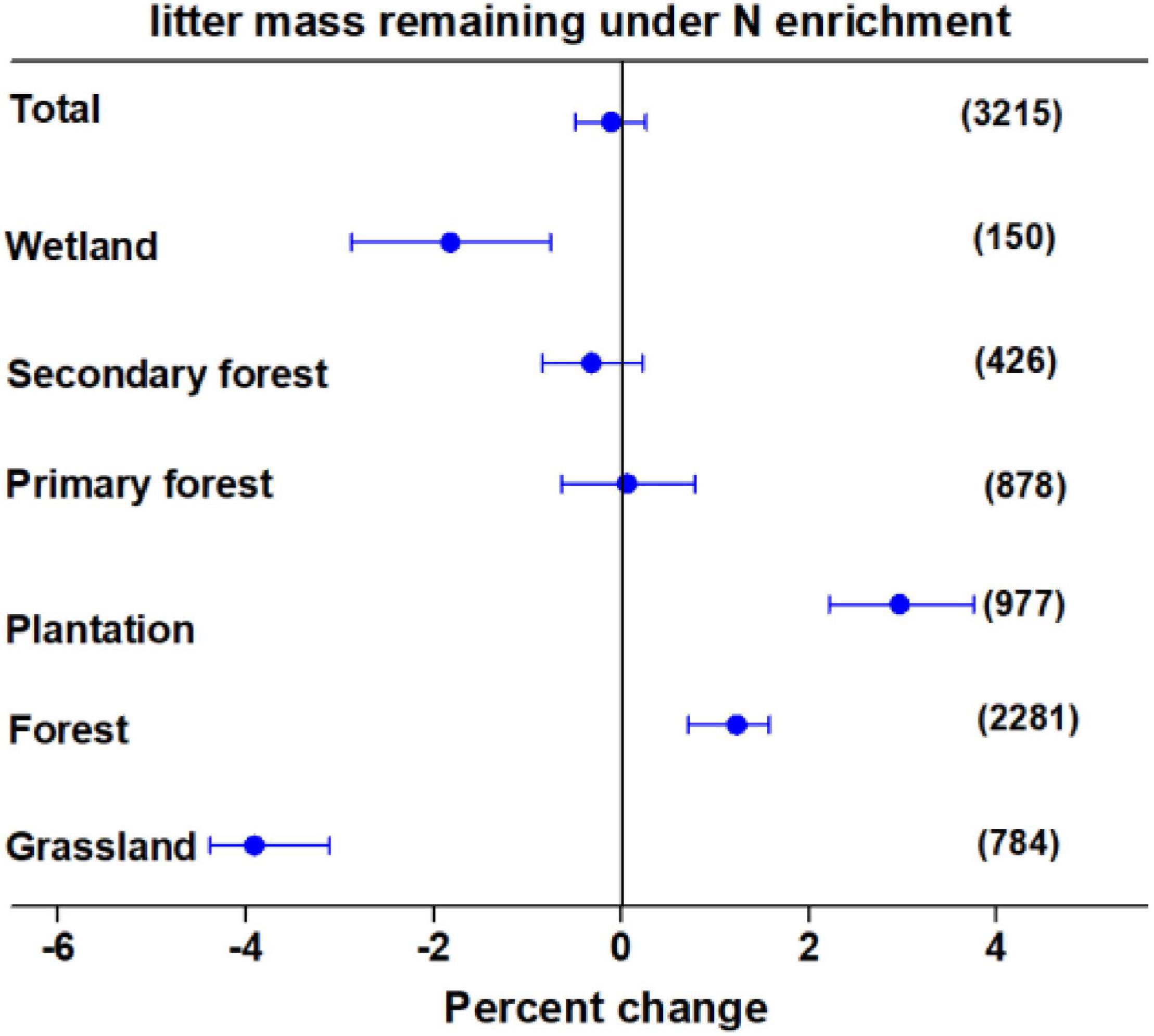
Figure 1. Mean percent change in litter mass remaining after nitrogen (N) enrichment among different ecosystem types. Differences in mean levels were regarded as significant if the 95% confidence intervals (CIs) did not overlap zero. Values in brackets represent the number of observations for each ecosystem type.
The Effect of N Enrichment on Litter Mass Remaining in Grasslands
The N addition rate and ambient N deposition significantly affected litter decay in grasslands, with the effect varying among different ranges of N deposition. The N deposition <50 kg N ha–1 year–1 had a small but significant effect in enhancing litter decay by 0.92%, whereas N deposition in the range of 50–150 kg N ha–1 year–1 stimulated litter decay by 5.69%, and N addition >150 kg N ha–1 year–1 stimulated litter decay by 3.34% (Figure 2). Low and medium levels of ambient N deposition of <10 and <10–20 kg N ha–1 year–1 significantly accelerated litter decay by 5.71 and 2.73%, respectively. The N enrichment showed no significant effects on litter decay at high levels of ambient N deposition. Expressing N addition rate as ambient N deposition indicated that N addition significantly stimulated litter decay by 1.40–4.66%, with a greater effect as N addition increased.
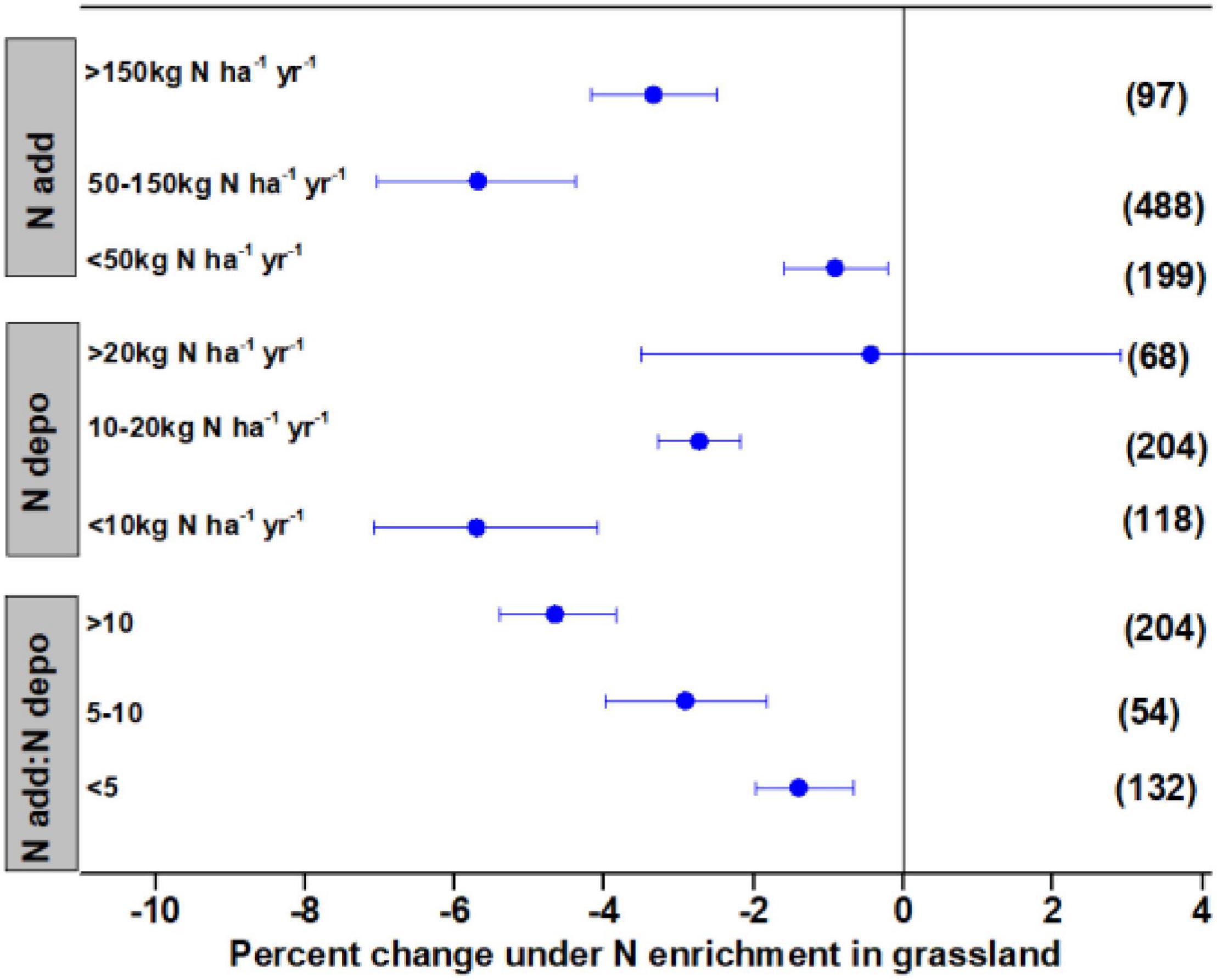
Figure 2. Mean percent change of litter mass remaining after N enrichment, ambient N deposition, and the ratio of N addition:ambient N deposition in grassland. Differences in mean levels were regarded as significant if the 95% CIs did not overlap zero. Values in brackets represent the number of observations for each ecosystem type.
The Effect of N Enrichment on Litter Mass Remaining in Forests
The N enrichment and ambient N deposition significantly affected litter decomposition in forests, with the effect varying among the different ranges of N deposition. The N deposition of <50, 50–150, and >150 kg N ha–1 year–1 stimulated litter decomposition by 0.62, 1.92, and 4.98%, respectively (Figure 2). The N enrichment significantly accelerated litter decay by 1.45% at low levels of ambient N deposition (<30 kg N ha–1 year–1), whereas there was no significant effect at medium levels of ambient N deposition (<10–20 kg N ha–1 year–1). High levels of ambient N deposition inhibited litter decay. Expressing the N application rate as ambient N deposition showed that N enrichment significantly slowed litter decay by 1.86% (a factor of 5–10) in plantations but had no significant effect in other forest types.
Data for forests were grouped according to community successional stages into plantations, primary forests, and secondary forests. These different groups showed different effects of N enrichment on litter decay. Low N enrichment levels (<50 kg N ha–1 year–1) accelerated litter decay in plantations and secondary forests by 4.48 and 1.47%, respectively, whereas, medium and high N enrichment inhibited litter decay by 3.85–5.63% in plantations and had no detectable impact on the litter decay in secondary forests (Figure 3). The N enrichment showed no detectable impact on litter decay in primary forests. The effects of N enrichment on litter decay in the different forest types differed under different levels of ambient N deposition. Expressing N enrichment as ambient N deposition showed that N enrichment significantly inhibited litter decay in plantations but showed variable effects on litter decay in primary and secondary forests.
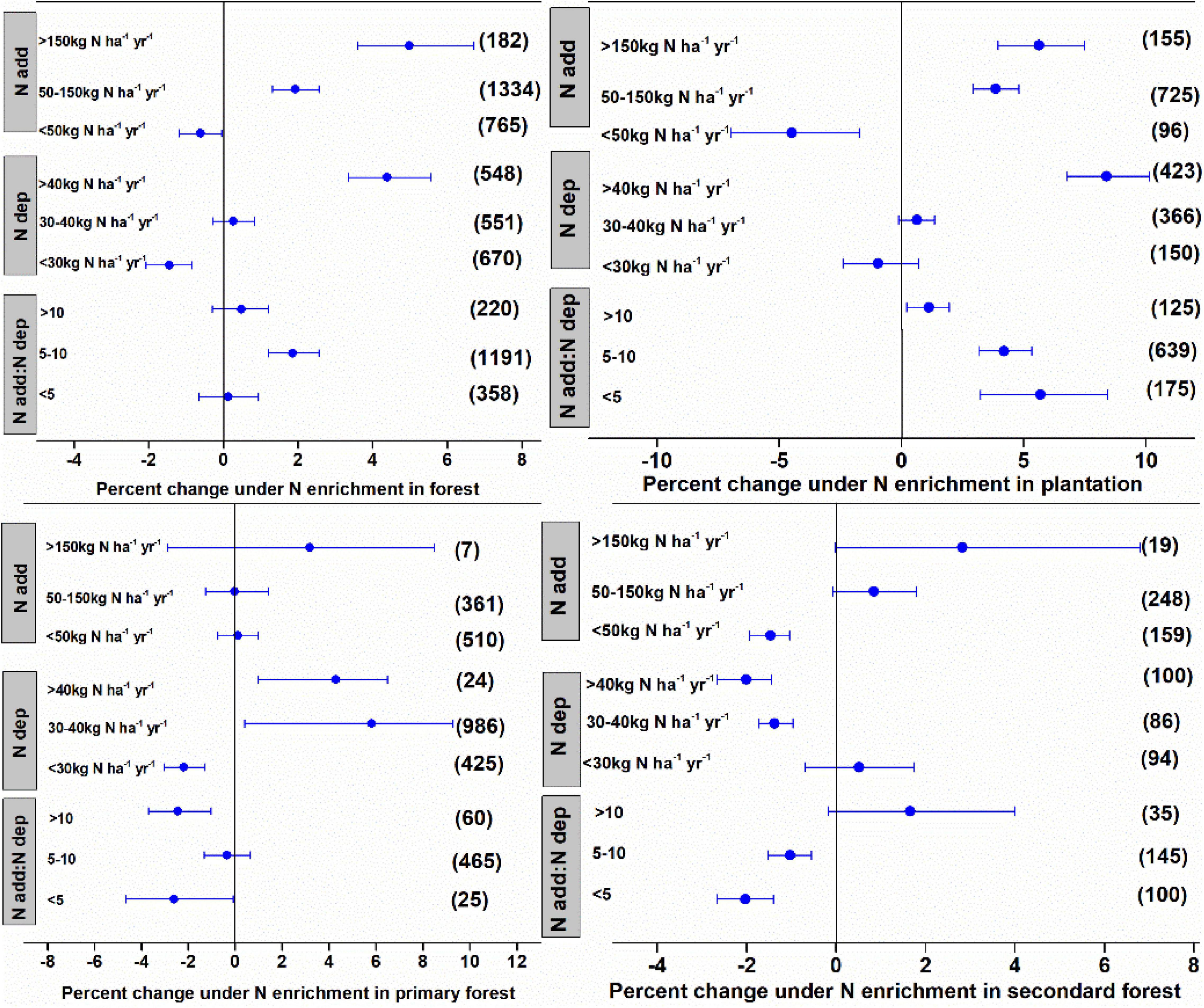
Figure 3. Mean percent change in litter mass remaining after N enrichment, ambient N deposition, and the ratio of N addition:ambient N deposition in the forest. The data were further grouped according to the community successional stages of the plantation, primary forest, and secondary forest. Differences in mean levels were regarded as significant if the 95% CIs did not overlap zero. Values in brackets represent the number of observations for each ecosystem type.
Environmental Factors Affecting the Responses of Litter Decomposition to N Enrichment in Grasslands and Forests
There is a negative relationship between the N addition rate and litter decay rate in the forest ecosystem, but this relationship is not significant in grassland (Figure 4). Ambient N deposition affected the RRs of litter mass remaining after N enrichment. Specifically, the RRs of litter mass remaining after N enrichment increased linearly as ambient N deposition increased.
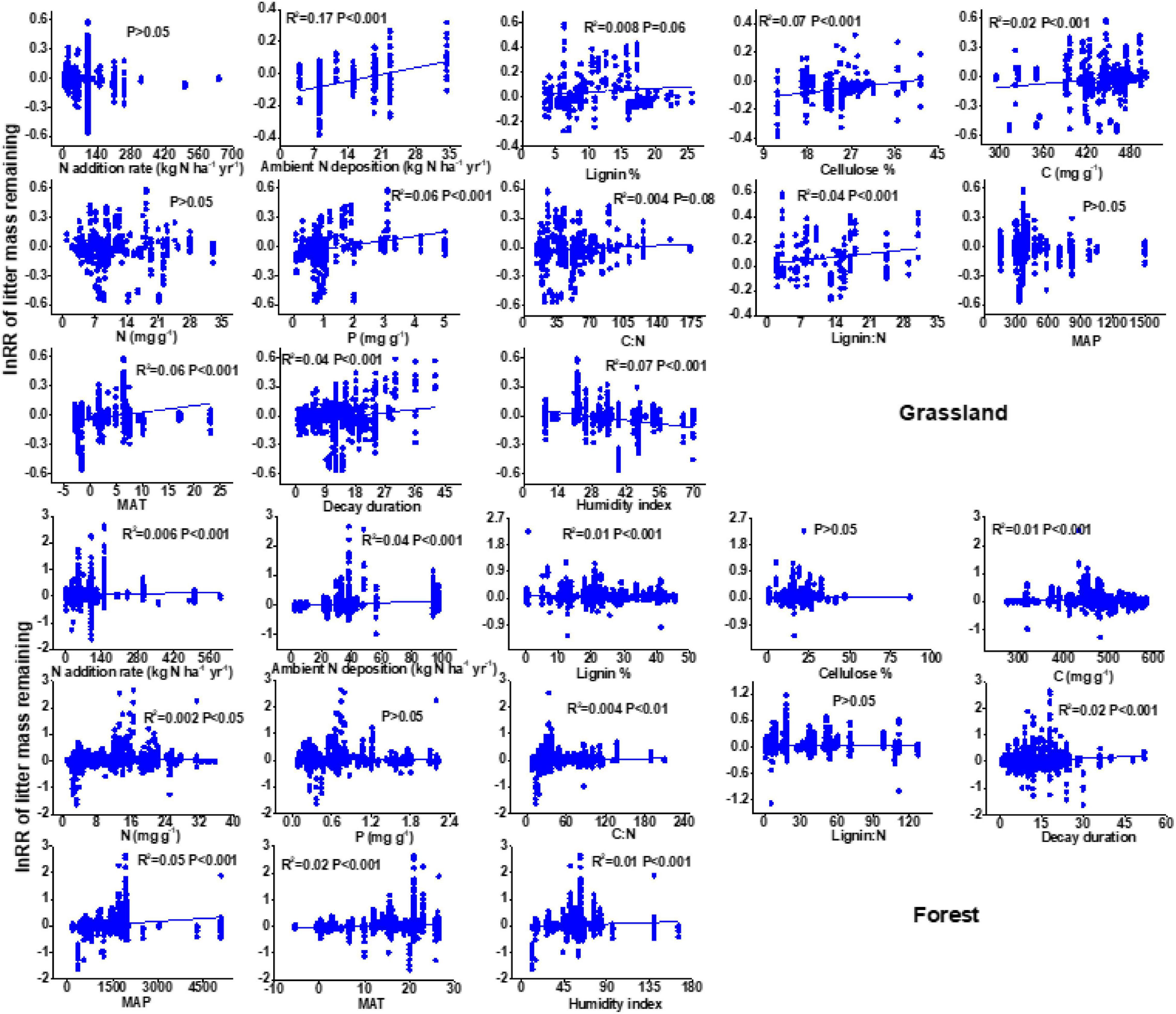
Figure 4. Relationships of the response ratio of litter mass remaining after N enrichment with N enrichment rate, ambient N deposition, lignin, cellulose, litter nutrient status, and climatic factors.
There were significant differences in lignin, cellulose, and litter nutrient status (C, N, and P), and each had a significant effect on litter decay (Figure 4 and Supplementary Figure 2). In particular, litter N content had no detectable impact on litter decay in grasslands but was significantly positively related to litter decay in forests. In addition, the response ratio of litter mass remaining after N enrichment increased with increasing MAP and MAT (Figure 4). HI showed a positive effect on the percentage change in litter mass remaining in grasslands, but a negative effect on forests.
Discussion
This meta-analysis provided a comprehensive quantitative evaluation of litter decomposition as a result of increased N enrichment in terrestrial ecosystems, especially in grasslands, wetlands, and forests. Results indicated that N enrichment had no notable impact on overall litter decomposition at the global scale, consistent with previous reports at the regional or global scales (Knorr et al., 2005; Zhang et al., 2018). However, the results provided solid evidence that globally increased N enrichment does have different effects on litter decomposition in different ecosystems. First, our results indicated that N enrichment accelerated litter decomposition in grasslands and wetlands, but significantly slowed it in forests. Second, the subdivision of the forest data according to land use difference showed that N enrichment significantly inhibited litter decomposition in plantations, but not in primary and secondary forests. This study challenges previous views that N enrichment did not affect litter decomposition in grasslands and wetlands and increases litter decomposition in secondary forests (Zhang et al., 2018). Third, our meta-analysis showed that N enrichment effects were dependent on experimental and environmental factors. Specifically, the influence of N enrichment on litter decay was accelerated at low N addition rates, but inhibiting effects increased significantly as the N addition rate changed in forests, plantations, and secondary forests. The litter decomposition rate exhibited an inverted U-shape response to increasing N addition rates, with the fastest decomposition at inputs of 50–150 kg N ha–1 year–1. Unfortunately, the limited data available on ambient N deposition in wetlands hampered the exploration of any differing effects of ambient N deposition and N enrichment on litter decomposition, and N enrichment effects on litter decomposition in wetlands remain largely unknown. Our results showed that a better understanding of the divergent responses of litter decomposition to N enrichment greatly improved our ability to accurately evaluate and predict the global C cycle and should be considered and applied in future global biogeochemical models.
The Positive Effect of N Enrichment on Litter Decomposition in Grasslands
Nitrogen is a key nutrient for plant growth in grassland ecosystems (Bai et al., 2010). The N enrichment significantly increases soil N availability, thereby influencing grassland productivity and its C cycle. Changes resulting from N enrichment have notable effects on litter decomposition in grasslands (Averill and Waring, 2018). This study found that N enrichment significantly accelerated grassland litter decomposition, with 784 observations showing that the effect was larger at medium or high N enrichment levels than at low levels. This result contradicted that of a previous meta-analysis of 251 observations, which concluded that N enrichment had no detectable impact on grassland litter decomposition (Zhang et al., 2018). This discrepancy could be attributed to the inclusion of more grassland samples in our meta-analysis, notably the inclusion of N enrichment studies in a variety of grassland types (meadow steppes, typical steppes, desert grasslands, and alpine grasslands) (Wang et al., 2021). Many of these studies indicated that N enrichment significantly accelerated grassland litter decomposition, e.g., evidence from meadow steppes (Dong et al., 2019), typical steppes (Gong et al., 2020; Hou et al., 2020), and desert grasslands (Zhang et al., 2020). The inclusion of this data improved our ability to identify the significant differences between grasslands more broadly. Several possible factors could enhance the positive effects of increased N enrichment on grassland litter decomposition. First, N enrichment significantly increased the litter N content and decreased the litter C:N ratio (Xia and Wan, 2008; Lu et al., 2011; Hou et al., 2018; You et al., 2018; Mao et al., 2020), thereby stimulating litter decomposition. Second, N enrichment significantly decreased soil pH, resulting in an increase in soil Mn content (Yang et al., 2012; Zeng et al., 2016; Hou et al., 2020), leading to the stimulation of litter decay (Sun et al., 2019; Hou et al., 2020). Third, N enrichment significantly changed the composition of the soil microbial community (Mooshammer et al., 2014; Zeng et al., 2016). The N enrichment significantly increased the bacteria:fungi ratio, suggesting that N enrichment could result in nutrient recycling in grassland systems being dominated by the bacterial community. Bacteria-dominated systems generally stimulate litter decomposition under relatively high nutrient availability since bacteria have higher nutrient demands and metabolic activities compared to fungi (Mooshammer et al., 2014; Zhou et al., 2017). Fourth, N enrichment significantly enhanced litter Ca and Mn contents (Hou et al., 2020), which have crucial functions in regulating litter decomposition (Davey et al., 2007; Berg et al., 2013; Whalen et al., 2018; Sun et al., 2019). For example, N enrichment consistently accelerated litter decay due to higher litter Mn and soil Mn contents, with a stronger effect of soil Mn relative to litter Mn (Whalen et al., 2018; Hou et al., 2020). Regarding Ca, Pichon et al. (2020) found that N enrichment significantly enhanced litter Ca content and improved litter quality, thus accelerating litter decomposition. While, some previous studies have demonstrated the negative effects of N enrichment on soil microbial activity and the consequent inhibition of litter decomposition (Lu et al., 2011; Zhou et al., 2017), this study showed that N enrichment increased grassland litter decomposition due to the positive effects of enhanced soil N availability overcoming any negative effects (Liu et al., 2010).
The Negative Effects of N Enrichment on Litter Decomposition in Forests
The present study showed that N enrichment significantly inhibited litter decay in forests. Subdividing the data according to forest successional type (plantations, primary forests, and secondary forests) showed that N enrichment significantly inhibited litter decomposition in plantations but had no detectable impact on it in primary and secondary forests. These results partly support previous meta-analyses, which suggested that N enrichment had mixed effects on forest litter decomposition (Zhang et al., 2018), or inhibited it (Knorr et al., 2005). The negative response of plantations was the strongest response to N enrichment among all the ecosystems examined, although primary and secondary forests showed insignificant and moderate responses to N enrichment, respectively.
A previous study also showed that N enrichment inhibited, had no detectable effect, or stimulated litter decomposition in plantations, primary forests, and secondary forests, respectively (Zhang et al., 2018). In particular, our study demonstrated the critical fact that low N enrichment levels (<50 kg N ha–1 year–1) significantly increased litter decomposition in plantations and secondary forests, while medium (50–150 kg N ha–1 year–1) or high (>150 kg N ha–1 year–1) levels of N enrichment inhibited it and that the inhibitory effect increased significantly with increasing N enrichment rate. Several possible factors may account for this difference. Earlier studies indicated that litter quality was tightly related to species diversity and forest successional stage (Berg and Laskowski, 2006; Deng and Janssens, 2006). The diversity and richness of plant species have been extensively used to clarify variations in litter decay mainly because the rate of litter decomposition is higher in more diverse forests than in less diverse ones (Perez et al., 1998; Huang et al., 2011). This may explain why low N enrichment levels significantly increased litter decomposition in secondary forests and plantations which are more species diverse and have different litter qualities (e.g., lower C:N ratios) than primary forests (Li et al., 2005; Yu et al., 2014). In addition, earlier studies have also provided evidence that land use differences significantly influenced soil moisture and plant diversity (Zhou et al., 2013; Wang et al., 2019a), which generally decreased the litter C:N ratios and litter lignin content leading to accelerated litter decomposition (Huang et al., 2011). However, medium or high N enrichment consistently decreased the rate of litter decomposition in secondary forests and plantations, perhaps because N enrichment increased both litter lignin content and litter C:N ratios (Cizungu et al., 2014). This view supports the results of a previous study showing that the accumulation of soil C in plantations is due to the very slow decomposition of organic matter (Yu et al., 2020a,b). Other studies have indicated that lowered soil pH induced by N addition significantly decreased soil microbial activity (Treseder, 2008; Hobbie et al., 2012) and that the negative effects of N enrichment significantly reduced the activity of lignin-degrading enzymes, resulting in the inhibition of litter decay (Carreiro et al., 2000). There is no denying that N enrichment also improves litter quality and increases N content (Mao et al., 2020), the negative effects of increased soil N on litter decay surpassing the positive effects of improved litter quality (Liu et al., 2010). Long-term, high N enrichment was shown to contribute to soil acidification in forest ecosystems (Yang et al., 2015) and to further decrease soil-based cations (K+, Na+, Mg2+, Ca2+). These changes in soil macronutrients can significantly affect litter decay rates (Vivanco and Austin, 2019; Zhou et al., 2020). Our study suggested that low or medium N enrichment did not affect litter decomposition in primary forests, while high N enrichment led to an insignificant decrease of 3.17%, based on seven observations. This was consistent with a previous study showing that N enrichment significantly inhibited litter decomposition due to significantly increased soil N availability, leading to soil N saturation (Mo et al., 2006). The insignificant results obtained when testing litter decomposition responses in primary forests may result from the underrepresentation of samples from high N enrichment studies, preventing us from identifying the significant differences within ecosystems more broadly.
The Positive Effect of N Enrichment on Litter Decomposition in Wetlands
Our results included 150 wetland observations, with N input gradients, indicating that wetland N enrichment consistently and significantly stimulated litter decomposition, a result which differed from that of Zhang et al. (2018), based on only 17 observations, in which N enrichment had no effect on wetland litter decomposition. Soil water saturation had direct positive effects on litter decomposition through increased N enrichment and also significantly influenced microbial activity (Song et al., 2011). Apparently, changes in the availability of soil or water N and soil water saturation may have interactive or complex effects on litter decomposition. The high variability in the responses of wetland litter decomposition to increased N enrichment presents great challenges to the prediction of litter-mediated global C cycling and nutrient budgets under future enhanced N deposition (Bardgett et al., 2014).
Factors Affecting the Responses of Litter Decomposition to N Enrichment
This study showed that the nutrient, cellulose, and lignin contents of litter were significantly different in the different ecosystems (Supplementary Figure 2). It should be noted that although N enrichment altered litter decomposition, the magnitude and direction of the effect were regulated by the nutrient and lignin contents of litter, N application dose, N enrichment form, experimental duration, litterbag mesh size, and climatic variables (Supplementary Figures 3–8), in accord with previous studies (Knorr et al., 2005; Zhang et al., 2018). The N addition increased litter decomposition under low N addition but inhibited decomposition under high N addition. This result could be attributed to the positive effect of improved litter quality at low N enrichment exceeding the negative effect on soil microbial activity (Fang et al., 2007; Liu and Greaver, 2010). A study by Chen et al. (2015) similarly found that low N enrichment significantly enhanced litter decay, whereas medium or high N enrichment notably inhibited it.
The magnitude and direction of the effect of N enrichment on litter decay varied greatly among the different forms of N addition (Supplementary Figures 3–8). The addition of mixed forms of N, for example, urea and glycine, showed stronger positive effects on litter decomposition compared to those of N alone. This result supported a previous meta-analysis (Knorr et al., 2005) and the study by Zhang et al. (2014). Most of the data for the addition of mixed N forms were derived from a single study on the meadow steppes of inner Mongolia, using five grassland species and community litter (Dong et al., 2020). Another study also showed that the addition of mixed forms of N in a field experiment significantly stimulated the decay of Metrosideros polymorpha litter (Hobbie, 2000). These studies indicated that the effects of the addition of mixed forms of N on litter decay differ from those of single forms of N, as seen in the present meta-analysis, indicating that global C emissions from litter decay may be underestimated (Gray and Fierer, 2012). Future studies should look further into the effects of the addition of mixed forms of N on litter decay.
Ambient N deposition, experimental duration, litterbag mesh size, and HI regulated the degree of the N enrichment effect on litter decay to some extent (Supplementary Figures 3–8). The N enrichment for a period of less than 6 months tended to stimulate litter decay, whereas longer periods of N enrichment either significantly inhibited or had no effect on litter decay. Previous studies have found that the duration of litter decay had significant effects on the litter decay rate, with an increased decay rate during the initial phase of litter decay, falling during the later phase (Zhang and Wang, 2012). This result was consistent with previous studies reporting the rapid decomposition of labile C fractions due to the high degrading activities of enzymes during the initial period, whereas the accumulation of lignin in litter later on reduced the activity of lignin-degrading enzymes (Zak et al., 2008; Hobbie et al., 2012; Zhang and Wang, 2012). Expressing the N enrichment rate as ambient N deposition showed that the effects of N enrichment on litter decay were regulated by the ambient N deposition and the N addition:ambient N deposition ratio. This demonstrated the noticeable impact of ambient N deposition on litter decomposition.
This study found a significant linear correlation between MAT and MAP (P < 0.001) (Supplementary Figure 8). Therefore, HI was used as a climatic indicator to quantify the relationship between litter mass remaining after N enrichment and climate. HI significantly influenced the effect of N enrichment on the litter decay rate (Supplementary Figures 3–8), whereas no significant difference among ecosystem groups was evident. Linear regression analysis showed that the RR of litter mass remaining increased significantly with increasing MAP and MAT, which partly supported the results of previous meta-analyses (Knorr et al., 2005; Zhang et al., 2018). Although linear regression analysis indicated that the RRs of litter mass remaining after N enrichment decreased significantly with increasing HI in grasslands, they increased with increasing HI in forests. This result indicated that climate could be a crucial factor regulating the effects of N enrichment on litter decomposition. Our meta-analysis of all field experimental results under N enrichment revealed a general pattern of the response of litter decomposition to N enrichment and the factors regulating this response. Therefore, the results of the present study are of value for accurately evaluating and predicting the impact of future global changes on litter decay.
Conclusion
Understanding the impacts of N enrichment on global plant litter decomposition is crucial for predicting the global C cycle and nutrient budgets of litter mediation in terrestrial ecosystems. Overall, our synthesis indicated that N enrichment significantly accelerated litter decomposition in grasslands and wetlands, but inhibited it in plantations and that the magnitude and direction of these effects are regulated by experimental and environmental variables. Litter decomposition rates were stimulated by low N enrichment but significantly inhibited by both medium and high N enrichment, with a greater inhibitory effect at high N enrichment in forests (plantations and secondary forests), suggesting that the impacts of N enrichment on litter decomposition are highly dependent on N enrichment rate. These results showed the importance of considering and incorporating these differential responses into global biogeochemical models to improve our ability to more accurately evaluate and predict the global ecosystem nutrient cycle of plant litter mediation under increasing N enrichment.
Data Availability Statement
The raw data supporting the conclusions of this article will be made available by the authors, without undue reservation.
Author Contributions
XL and KL contributed to the study conception and design. YS conducted data collection and analysis. XM, YG, and WH revised and improved the manuscript. All authors contributed to the article and approved the submitted version.
Funding
This study was supported financially by the Strategic Priority Research Program of the Chinese Academy of Sciences (XDA20050103), the National Natural Science Foundation of China (41425007, 41673079, and 32101345), and the Light of West China Program of the Chinese Academy of Sciences (WH).
Conflict of Interest
The authors declare that the research was conducted in the absence of any commercial or financial relationships that could be construed as a potential conflict of interest.
Publisher’s Note
All claims expressed in this article are solely those of the authors and do not necessarily represent those of their affiliated organizations, or those of the publisher, the editors and the reviewers. Any product that may be evaluated in this article, or claim that may be made by its manufacturer, is not guaranteed or endorsed by the publisher.
Acknowledgments
We appreciate all the researchers for their published manuscript used in our meta-analysis.
Supplementary Material
The Supplementary Material for this article can be found online at: https://www.frontiersin.org/articles/10.3389/ffgc.2022.895774/full#supplementary-material
Footnotes
- ^ http://www.getdata-graph-digitizer.com
- ^ https://metawin.software.informer.com/2.1/
- ^ https://www.originlab.com/
References
Allison, S. D., LeBauer, D. S., Ofrecio, M. R., Reyes, R., Ta, A. M., and Tran, T. M. (2009). Low levels of nitrogen addition stimulate decomposition by boreal forests fungi. Soil Biol. Biochem. 41, 293–302.
Argiroff, W. A., Zak, D. R., Upchurch, R. A., Salley, S. O., and Grandy, A. S. (2019). Anthropogenic N deposition alters soil organic matter biochemistry and microbial communities on decaying fine roots. Glob. Change Biol. 25, 4369–4382. doi: 10.1111/gcb.14770
Averill, C., and Waring, B. (2018). Nitrogen limitation of decomposition and decay: how can it occur? Glob. Change Biol. 24, 1417–1427. doi: 10.1111/gcb.13980
Bai, Y. F., Wu, J. G., Clark, C. M., Naeem, S., Pan, Q. M., Huang, J. H., et al. (2010). Tradeoffs and thresholds in the effects of nitrogen addition on biodiversity and ecosystem functioning: evidence from inner Mongolia Grasslands. Glob. Change Biol. 16, 358–372.
Bardgett, R. D., Mommer, L., and De Vries, F. T. (2014). Going underground: root traits as drivers of ecosystem processes. Trends Ecol. Evol. 29, 692–699. doi: 10.1016/j.tree.2014.10.006
Berg, B., Erhagen, B., Johansson, M. B., Vesterdal, L., Faituri, M., and Sanborn, P. (2013). Manganese dynamics in decomposing needle and leaf litter-a synthesis. Can. J. For. Res. 43, 1127–1136.
Berg, B., and Laskowski, R. (2006). Litter Decomposition: A Guide To Carbon And Nutrient Turnover. Amsterdam: Elsevier, 1–421.
Carreiro, M. M., Sinsabaugh, R. L., Repert, D. A., and Parkhurst, D. F. (2000). Microbial enzyme shifts explain litter decay responses to simulated nitrogen deposition. Ecology 81, 2359–2365.
Chen, H., Li, D. J., Gurmesa, G. A., Yu, G. R., Li, L. H., Zhang, W., et al. (2015). Effects of nitrogen deposition on carbon cycle in terrestrial ecosystems of China: a meta-analysis. Environ. Pollut. 206, 352–360. doi: 10.1016/j.envpol.2015.07.033
Ciais, P., Sabine, C., Bala, G., Bopp, L., Brovkin, V., Canadell, J., et al. (2013). “Carbon and other biogeochemical cycles,” in Climate Change 2013: The Physical Science Basis. Contribution Of Working Group I To The Fifth Assessment Report Of The Intergovernmental Panel On Climate Change, eds T. F. Stocker, D. Qin, G.-K. Plattner, M. Tignor, S. K. Allen, J. Boschung, et al. (Cambridge: Cambridge University Press), 465–570.
Cizungu, L., Staelens, J., Huygens, D., Walangululu, J., Muhindo, D., Cleemput, O. V., et al. (2014). Litterfall and leaf litter decomposition in a central African tropical mountain forest and Eucalyptus plantation. For. Ecol. Manage. 326, 109–116.
Davey, M. P., Berg, B., Emmett, B. A., and Rowland, P. (2007). Decomposition of oak leaf litter is related to initial litter Mn concentrations. Can. J. Bot. 85, 16–24.
Deng, L., Huang, C. B., Kim, D. G., Shangguan, Z. P., Wang, K. B., Song, X. Z., et al. (2019). Soil GHG fluxes are altered by n deposition: new data indicate lower N stimulation of the N2O flux and greater stimulation of the calculated C pools. Glob. Change Biol. 26, 2613–2629. doi: 10.1111/gcb.14970
Deng, Z., and Janssens, M. J. J. (2006). “Litter fall production in west-african forests and plantations,” in Proceedings of the Conference on International Agricultural Research for Development, (Bonn: University of Bonn), 1–5.
Dong, L. L., Berg, B., Sun, T., Wang, Z. W., and Han, X. G. (2020). Response of fine root decomposition to different forms of N deposition in a temperate grassland. Soil Biol. Biochem. 147:107845. doi: 10.1016/j.soilbio.2020.107845
Dong, L. L., Sun, T., Berg, B., Zhang, L. L., Zhang, Q. Q., and Wang, Z. W. (2019). Effects of different forms of N deposition on leaf litter decomposition and extracellular enzyme activities in a temperate grassland. Soil Biol. Biochem. 134, 78–80.
Fang, H., Mo, J., Peng, S., Li, Z., and Wang, H. (2007). Cumulative effects of nitrogen additions on litter decomposition in three tropical forests in southern China. Plant Soil 297, 233–242. doi: 10.1007/s11104-007-9339-9
Gong, J. R., Zhu, C. C., Yang, L. L., Yang, B., Wang, B., Baoyin, T. T., et al. (2020). Effects of nitrogen addition on above-and belowground litter decomposition and nutrient dynamics in the litter-soil continuum in the temperate steppe of Inner Mongolia, China. J. Arid Environ. 172:104036.
Gray, C. M., and Fierer, N. (2012). Impacts of nitrogen fertilization on volatile organic compound emissions from decomposing plant litter. Glob. Change Biol. 18, 739–748.
Hobbie, S. E. (2000). Interactions between litter lignin and nitrogenitter lignin and soil nitrogen availability during leaf litter decomposition in a Hawaiian Montane Forest. Ecosystems 3, 484–494. doi: 10.1007/s100210000042
Hobbie, S. E., Eddy, W. C., Buyarski, C. R., Adair, E. C., Ogdahl, M. L., and Weisenhorn, P. (2012). Response of decomposing litter and its microbial community to multiple forms of nitrogen enrichment. Ecol. Monogr. 82, 389–405.
Hong, S. B., Yin, G. D., Piao, S. L., Dybzinski, R., Cong, N., Li, X. Y., et al. (2020). Divergent responses of soil organic carbon to afforestation. Nat. Sustain. 3, 694–700. doi: 10.1111/gcb.15430
Hou, S. L., Freschet, G. T., Yang, J. J., Zhang, Y. H., Yin, J. X., Hu, Y. Y., et al. (2018). Quantifying the indirect effects of nitrogen deposition on grasslands litter chemical traits. Biogeochemistry 139, 261–273.
Hou, S. L., Hättenschwiler, S., Yang, J. J., Sistla, S., Wei, H. W., Zhang, Z. W., et al. (2020). Increasing rates of long-term nitrogen deposition consistently increased litter decomposition in a semi-arid grassland. New Phytol. 229, 296–307. doi: 10.1111/nph.16854
Huang, Y. H., Li, Y. L., Xiao, Y., Wenigmann, K. O., Zhou, G. Y., Zhang, D. Q., et al. (2011). Controls of litter quality on the carbon sink in soils through partitioning the products of decomposing litter in a forests succession series in south China. For. Ecol. Manage. 261, 1170–1177.
Knorr, M., Frey, S. D., and Curtis, P. S. (2005). Nitrogen additions and litter decomposition: a meta-analysis. Ecology 86, 3252–3257.
Lamarque, J. F., Kiehl, J. T., Brasseur, G. P., Butler, T., Cameron-Smith, P., Collins, W. D., et al. (2005). Assessing future nitrogen deposition and carbon cycle feedback using a multimodel approach: analysis of nitrogen deposition. J. Geophys. Res. 110. doi: 10.1029/2005JD005825
Li, Y. Q., Xu, M., Zou, X. M., Shi, P. J., and Zhang, Y. Q. (2005). Comparing soil organic carbon dynamics in plantation and secondary forest in wet tropics in Puerto Rico. Glob. Change Biol. 11, 239–248.
Liu, L. L., and Greaver, T. L. (2010). A global perspective on belowground carbon dynamics under nitrogen enrichment. Ecol. Lett. 13, 819–828. doi: 10.1111/j.1461-0248.2010.01482.x
Liu, P., Huang, J. H., Sun, O. J. X., and Han, X. G. (2010). Litter decomposition and nutrient release as affected by soil nitrogen availability and litter quality in a semiarid grassland ecosystem. Oecologia 162, 771–780. doi: 10.1007/s00442-009-1506-7
Lu, M., Yang, Y. H., Luo, Y. Q., Fang, C. M., Zhou, X. H., Chen, J. K., et al. (2011). Responses of ecosystem nitrogen cycle to nitrogen addition: a meta-analysis. New Phytol. 189, 1040–1050. doi: 10.1111/j.1469-8137.2010.03563.x
Mao, J. H., Mao, Q. G., Zheng, M. H., and Mo, J. M. (2020). Responses of foliar nutrient status and stoichiometry to nitrogen addition in different ecosystems: a meta-analysis. J. Geophys. Res. 125:e2019JG005347.
Mo, J. M., Brown, S., Xue, J. H., Fang, Y. T., and Li, Z. A. (2006). Response of litter decomposition to simulated N deposition in disturbed, rehabilitated and mature forests in Subtropical China. Plant Soil 282, 135–151.
Mooshammer, M., Wanek, W., Zechmeister-Boltenstern, S., and Richter, A. (2014). Stoichiometric imbalances between terrestrial decomposer communities and their resources: Mechanisms and implications of microbial adaptations to their resources. Front. Microbiol. 5:22. doi: 10.3389/fmicb.2014.00022
Peng, Y. F., Guo, D. L., and Yang, Y. H. (2017). Global patterns of root dynamics under nitrogen enrichment. Glob. Ecol. Biogeogr. 26, 102–114.
Perez, C. A., Hedin, L. O., and Armesto, J. J. (1998). Nitrogen mineralization in two unpolluted old-growth forests of contrasting biodiversity and dynamics. Ecosystems 1, 361–373.
Pichon, N. A., Cappelli, S. L., Soliveres, S., Hölzel, N., Klaus, V. H., Kleinebecker, T., et al. (2020). Decomposition disentangled: a test of the multiple mechanisms by which nitrogen enrichment alters litter decomposition. Funct. Ecol. 34, 1485–1496.
Raich, J. W., and Schlesinger, W. H. (1992). The global carbon dioxide flux in soil respiration and its relationship to vegetation and climate. Tellus B Chem. Phys. Meteorol. 44, 81–99.
See, C. R., McCormack, M., Hobbie, S. E., Flores-Moreno, H., Silver, W. L., and Kennedy, P. G. (2019). Global patterns in fine root decomposition: climate, chemistry, mycorrhizal association and woodiness. Ecol. Lett. 22, 946–953. doi: 10.1111/ele.13248
Sha, Z. P., Ma, X., Wang, J. X., Lv, T. T., Li, Q. Q., Misselbrook, T., et al. (2020). Effect of N stabilizers on fertilizer-N fate in the soil-crop system: a meta-analysis. Agric. Ecosyst. Environ. 290, 106763.
Song, C. C., Liu, D. Y., Yang, G. S., Song, Y. Y., and Mao, R. (2011). Effect of nitrogen addition on decomposition of Calamagrostis angustifolia litters from freshwater marshes of Northeast China. Ecol. Eng. 37, 1578–1582.
Song, Y. Y., Song, C. C., Ren, J. S., Zhang, X. H., and Jiang, L. (2019). Nitrogen input increases Deyeuxia angustifolia litter decomposition and enzyme activities in a marshland ecosystem in Sanjiang Plain, Northeast China. Wetlands 39, 549–557.
Sun, T., Cui, Y. L., Berg, B., Zhang, Q. Q., Dong, L. L., Wu, Z. J., et al. (2019). A test of manganese effects on decomposition in forest and cropland sites. Soil Biol. Biochem. 129, 178–183.
Treseder, K. K. (2008). Nitrogen additions and microbial biomass: a meta-analysis of ecosystem studies. Ecol. Lett. 11, 1111–1120. doi: 10.1111/j.1461-0248.2008.01230.x
van Diepen, L. T. A., Frey, S. D., Sthultz, C. M., Morrison, E. W., Minocha, R., and Pringle, A. (2015). Changes in litter quality caused by simulated nitrogen deposition reinforce the N-induced suppression of litter decay. Ecosphere 6:205.
Vivanco, L., and Austin, A. T. (2011). Nitrogen addition stimulates forest litter decomposition and disrupts species interactions in Patagonia, Argentina. Glob. Change Biol. 17, 1963–1974.
Vivanco, L., and Austin, A. T. (2019). The importance of macro- and micro-nutrients over climate for leaf litter decomposition and nutrient release in Patagonian temperate forests. For. Ecol. Manage. 441, 144–154.
Wang, G. S., Huang, W. J., Mayes, M. A., Liu, X. D., Zhang, D. Q., Zhang, Q. M., et al. (2019a). Soil moisture drives microbial controls on carbon decomposition in two subtropical forests. Soil Biol. Biochem. 130, 185–194.
Wang, N., Quesada, B., Xia, L. L., Butterbach-Bahl, K., Goodale, C. L., and Kiese, R. (2019b). Effects of climate warming on carbon fluxes in grasslands-A global meta-analysis. Glob. Change Biol. 25, 1839–1851. doi: 10.1111/gcb.14603
Wang, J. S., Defrenne, C., McCormack, M. L., Yang, L., Tian, D. S., Luo, Y. Q., et al. (2021). Fine-root functional trait responses to experimental warming: a global meta-analysis. New Phytol. 230, 1856–1867. doi: 10.1111/nph.17279
Whalen, E. D., Smith, R. G., Grandy, A. S., and Frey, S. D. (2018). Manganese limitation as a mechanism for reduced decomposition in soils under atmospheric nitrogen deposition. Soil Biol. Biochem. 127, 252–263.
Xia, J. Y., and Wan, S. Q. (2008). Global response patterns of terrestrial plant species to nitrogen addition. New Phytol. 179, 428–439. doi: 10.1111/j.1469-8137.2008.02488.x
Yang, Y. H., Ji, C. J., Ma, W. H., Wang, S. F., Wang, S. P., Han, W. X., et al. (2012). Significant soil acidification across northern China”s grasslands during 1980s-2000s. Glob. Change Biol. 18, 2292–2300.
Yang, Y. H., Li, P., He, H. L., Zhao, X., Datta, A., Ma, W. H., et al. (2015). Long-term changes in soil pH across major forest ecosystems in China. Geophys. Res. Lett. 42, 933–940.
You, C. M., Wu, F. Z., Yang, W. Q., Xu, Z. F., Tan, B., Yue, K., et al. (2018). Nutrient-limited conditions determine the responses of foliar nitrogen and phosphorus stoichiometry to nitrogen addition: a global meta-analysis. Environ. Pollut. 241, 740–749. doi: 10.1016/j.envpol.2018.06.018
Yu, M. X., Wang, Y. P., Baldock, J. A., Jiang, J., Mo, J. M., Zhou, G. Y., et al. (2020a). Divergent responses of soil organic carbon accumulation to 14 years of nitrogen addition in two typical subtropical forests. Sci. Total Environ. 707:136104. doi: 10.1016/j.scitotenv.2019.136104
Yu, Z., Zhou, G. Y., Liu, S. R., Sun, P. S., and Agathokleous, E. (2020b). Impacts of forest management intensity on carbon accumulation of China”s forest plantations. For. Ecol. Manage. 472:118252.
Yu, Y. F., Peng, W. X., Song, T. Q., Zeng, F. P., Wang, K. L., Wen, L., et al. (2014). Stoichiometric characteristics of plant and soil C, N and P in different forests types in depressions between karst hills, southwest China. Chinese J. Appl. Ecol. 25, 947–954.
Zak, D. R., Holmes, W. E., Burton, A. J., Pregitzer, K. S., and Talhelm, A. F. (2008). Simulated atmospheric NO3-deposition increases soil organic matter by slowing decomposition. Ecol. Appl. 18, 2016–2027. doi: 10.1890/07-1743.1
Zeng, J., Liu, X. J., Song, L., Lin, X. G., Zhang, H. Y., Shen, C. C., et al. (2016). Nitrogen fertilization directly affects soil bacterial diversity and indirectly affects bacterial community composition. Soil Biol. Biochem. 92, 41–49. doi: 10.1038/ismej.2011.159
Zhang, C. Y., Zhao, H. M., Liu, H., Zhang, W. T., Su, Y. G., Cheng, J. H., et al. (2020). Effects of exogenous nitrogen addition on surface litter decomposition and nutrient release in a temperate desert. Chinese J. Appl. Ecol. 31, 3631–3638. doi: 10.13287/j.1001-9332.202011.001
Zhang, T. A., Luo, Y. Q., Chen, H. Y. H., and Ruan, H. H. (2018). Responses of litter decomposition and nutrient release to N addition: a meta-analysis of terrestrial ecosystems. Appl. Soil Ecol. 128, 35–42.
Zhang, W. D., and Wang, S. L. (2012). Effects of NH4+ and NO3- on litter and soil organic carbon decomposition in a Chinese fir plantation forest in South China. Soil Biol. Biochem. 47, 116–122.
Zhang, W. D., Wang, X. F., and Wang, S. L. (2014). Fate of Chinese-fir litter during decomposition as a result of inorganic N additions. Appl. Soil Ecol. 74, 30–36.
Zhou, G. Y., Peng, C. H., Li, Y. L., Liu, S. Z., Zhang, Q. M., Tang, X. L., et al. (2013). A climate change-induced threat to the ecological resilience of a subtropical monsoon evergreen broad-leaved forest in Southern China. Glob. Change Bioly. 19, 1197–1210. doi: 10.1111/gcb.12128
Zhou, S. X., Butenschoen, O., Barantal, S., Handa, I. T., Makkonen, M., Vos, V., et al. (2020). Decomposition of leaf litter mixtures across biomes: the role of litter identity, diversity and soil fauna. J. Ecol. 108, 2283–2297.
Keywords: litter decomposition, nitrogen enrichment, ambient N deposition, meta-analysis, plantation, grassland
Citation: Su Y, Ma X, Gong Y, Ahmed Z, Han W, Li K and Liu X (2022) Global Patterns and Drivers of Litter Decomposition Under Nitrogen Enrichment: A Meta-Analysis. Front. For. Glob. Change 5:895774. doi: 10.3389/ffgc.2022.895774
Received: 22 March 2022; Accepted: 02 May 2022;
Published: 10 June 2022.
Edited by:
Yunting Fang, Institute of Applied Ecology (CAS), ChinaReviewed by:
Weidong Zhang, Institute of Applied Ecology (CAS), ChinaWeijun Shen, Guangxi University, China
Copyright © 2022 Su, Ma, Gong, Ahmed, Han, Li and Liu. This is an open-access article distributed under the terms of the Creative Commons Attribution License (CC BY). The use, distribution or reproduction in other forums is permitted, provided the original author(s) and the copyright owner(s) are credited and that the original publication in this journal is cited, in accordance with accepted academic practice. No use, distribution or reproduction is permitted which does not comply with these terms.
*Correspondence: Kaihui Li, likh@ms.xjb.ac.cn; Xuejun Liu, liu310@cau.edu.cn