- 1Institute of Environment and Water Resources of Bahia (INEMA), Salvador, Brazil
- 2Laboratory of Vegetation Studies (LEV), Graduate Program in Ecology, Theory Application, and Values, Biology Institute, Federal University of Bahia, Salvador, Brazil
Habitat loss is a significant threat to biodiversity in tropical forests, leading to changes in composition, structure, and function. In this study, we aimed to evaluate the influence of the forest amount at the landscape scale on the structure and the stored carbon of tree communities. Eleven landscape samples of 36 km2 (6 × 6 km), with different amounts of forest cover at the landscape scale (between 5 and 60%), were randomly selected within a wide latitudinal range of Atlantic Forests in Brazil. We also used a sample design to control and avoid some biases in landscape-scale studies during the landscape selection, such as the rescue effect from the surrounding landscapes, differences in matrix permeability in each landscape, and the control for the same vegetation type or successional stage. In each selected landscape, we surveyed all woody individuals with a diameter at breast height (DBH) ≥ 2.5 cm in eight plots of 250 m2, randomly placed in the landscape’s forested areas. The structural variables evaluated were: total abundance, the abundance of prominent families, the abundance of large trees (DBH ≥ 30 cm), mean DBH, and average height, besides carbon storage. From the set of 8,179 individuals, we observed a positive correlation between forest amount and the total abundance, the abundance of large trees, and carbon stock. The abundance of the prominent hardwood tree families were positively correlated with forest amount. When comparing the two landscapes with the lowest proportion of habitats (5–15%) and the highest proportion (55−60%), almost one thousand trees and more than 150 T of carbon per hectare are lost. This reduction in the overall abundance and carbon indicates no compensatory effects on the tree community due to a turnover in composition or substitution of large trees for smaller ones. The Atlantic Forest is undergoing a process of homogenization and functional collapse, derived directly or indirectly from habitat loss, leading to a possible regime shift.
1. Introduction
One of the notorious characteristics of a forest is its structure, evidenced mainly by the size of its individuals and the density of trees in woody plant communities (Pütz and Redford, 2010). In general, the structural characteristics of a forest emerge from metabolic and demographic aspects (Muller-Landau et al., 2006; Enquist et al., 2009; West et al., 2009).
Demographic aspects are associated with birth dynamics and tree mortality (Coomes et al., 2003) and may reflect environmental conditions at different spatial levels over reproduction, dispersion, establishment, and survival processes. However, the metabolic aspects tend to explain the individuals’ variations in size and shape in response to the physical-chemical constraints of the environment (Brown et al., 2004; Muller-Landau et al., 2006). The environment could affect individual growth rates, and variations in growth rates tend to change the proportion between size classes within a population (Condit et al., 1998; Wright et al., 2003).
Changes in tree communities may reflect natural and human-made disturbances (Sposito and Santos, 2001; Nagendra, 2012). Disorders caused by humans, such as the loss and fragmentation of natural habitats, have had profound impacts on the structure and functioning of forest tree communities (Millennium Ecosystem Assessment [MEA]., 2005; Magnago L. et al., 2015; Rocha-Santos et al., 2016).
With forest suppression, the reproductive success of different tree populations is also reduced, either by the loss of pollinators and dispersers, in the latter case mainly of medium and large size animals (Silva and Tabarelli, 2000; Jorge et al., 2013), or by Allee effect under critical densities (Stephens and Sutherland, 1999). Such changes directly impact the demographic rates of tree species and, consequently, the forest structure.
Forests reduction at the landscape scale increases mean distances between patches, the mean size of forest remnant patches, and the proportion of border areas (Fahrig, 2003) and, consequently, greater exposure to the edge effect (Murcia, 1995; Tabarelli et al., 2004; Rigueira et al., 2012). Many edge effects are associated with microclimatic changes in the forest fragments, such as humidity, luminosity, wind incidence, and other effects (Matlack, 1993; Murcia, 1995; Laurance and Curran, 2008; Magnago L. F. S. et al., 2015). Those can shape metabolic and demographic aspects, leading to structural changes in tree communities. Deforestation at the landscape scale reduces the overall abundance of all tree species in the landscape. It also influences a series of tree community and population aspects in the remaining tropical forest: increasing the mortality of large trees, reducing of mean size of its individuals and overall abundance of trees, and loss of important tree families species, for example (Laurance et al., 2000, 2006; Rocha-Santos et al., 2016).
Reducing the forest amount at the landscape scale can lead to abrupt changes in plant communities, i.e., threshold responses, as observed in the diversity loss in different plants groups (Rigueira et al., 2013; Lima and Mariano-Neto, 2014; Rocha-Santos et al., 2016; Benchimol et al., 2017b). These patterns were observed both for the entire community of woody plants (Rigueira and Mariano-Neto in prep.) and for specific families, usually composed of shade-tolerant species and denser wood (Rigueira et al., 2013; Lima and Mariano-Neto, 2014, Rocha-Santos et al., 2016, Benchimol et al., 2017b). Hunting or logging also tends to be accentuated in more fragmented landscapes, aggravating the synergistic effects of habitat loss in forested tropical landscapes (Echeverría et al., 2007; Laurance et al., 2011; Galetti and Dirzo, 2013; Liu and Slik, 2014).
As species diversity is a crucial attribute for ecological process maintenance in different ecosystems (Norberg et al., 2008), it is expected that these processes, such as the carbon stock in tropical forests, may be compromised by habitat loss. It is known that the fragmentation of tropical forests (Pütz et al., 2014; Magnago L. et al., 2015) and their defaunation (Bello et al., 2015) lead to the loss of large amounts of stored carbon, but not if the quantity of habitat amount at landscape scale influences such stocks. In addition, the differentiated loss of trees families capable of storing more carbon, such as Sapotaceae and Myrtaceae (Rigueira et al., 2013, Lima and Mariano-Neto, 2014), may have a more significant impact on ecological structure and processes, such as carbon sequestration and storage. Tropical forests stores about 40% of the carbon from terrestrial environments (Dixon et al., 1994), and deforestation contributes 7−14% of the global carbon emission (Harris et al., 2012). Therefore, it is crucial to understand carbon stock responses to habitat amount, given the vital role of carbon in climate regulation, both locally, regionally, and globally (Millennium Ecosystem Assessment [MEA]., 2005).
From the perspective of landscape management, information on changes in forest structure has been used to indicate the occurrence of local disturbances (Karna et al., 2010; Nagendra et al., 2010; Banks-Leite et al., 2011). Once the relationship between forest structuring and habitat loss at the landscape scale has been unrevealed, we need to investigate the mechanisms that affect or maintain essential ecological processes in human-altered landscapes (Melo et al., 2013; Banks-Leite et al., 2014).
This study of Tropical Forest fragmented landscapes (Atlantic Forest) aims to evaluate the influence of the forest habitat amount on the structuring of tree communities (size and abundance of trees) and, consequently, on the carbon stocking process. We also consider the possible variation in the abundance of particular plant families with the expected forest structure change, considering factors acting at different hierarchical levels. We will evaluate the following hypotheses: (i) the structure of tree communities, in their totality and prominent families, is determined by the habitat amount at the landscape; (ii) the habitat amount positively influences the carbon stock at the landscape.
2. Materials and methods
This study is part of a multi-taxa project developed by a researchers group from Institute of Biology of the Federal University of Bahia (UFBA) to evaluate the influence of habitat loss at the landscape scale on different taxonomic groups and ecological processes in the Atlantic Forest (CNPq/FAPESB research founding PNX0016_2009).
2.1. Study area and sample landscapes
The present study was developed in eleven landscapes, randomly selected from a large extent in the central and coastal portion of the Bahia Atlantic Forest in the northeast of Brazil (between latitudes 11° 80’ and 18° 49’ S and between the longitudes 49° 08’ and 21° 24’ W) (Figure 1). The Atlantic Forest harbors an unequaled amount of world biodiversity and represents one of the most threatened natural biomes of the planet (Myers et al., 2000; Laurance, 2009), remaining only 11.4−16% of its original 150 million hectares (Ribeiro et al., 2009).
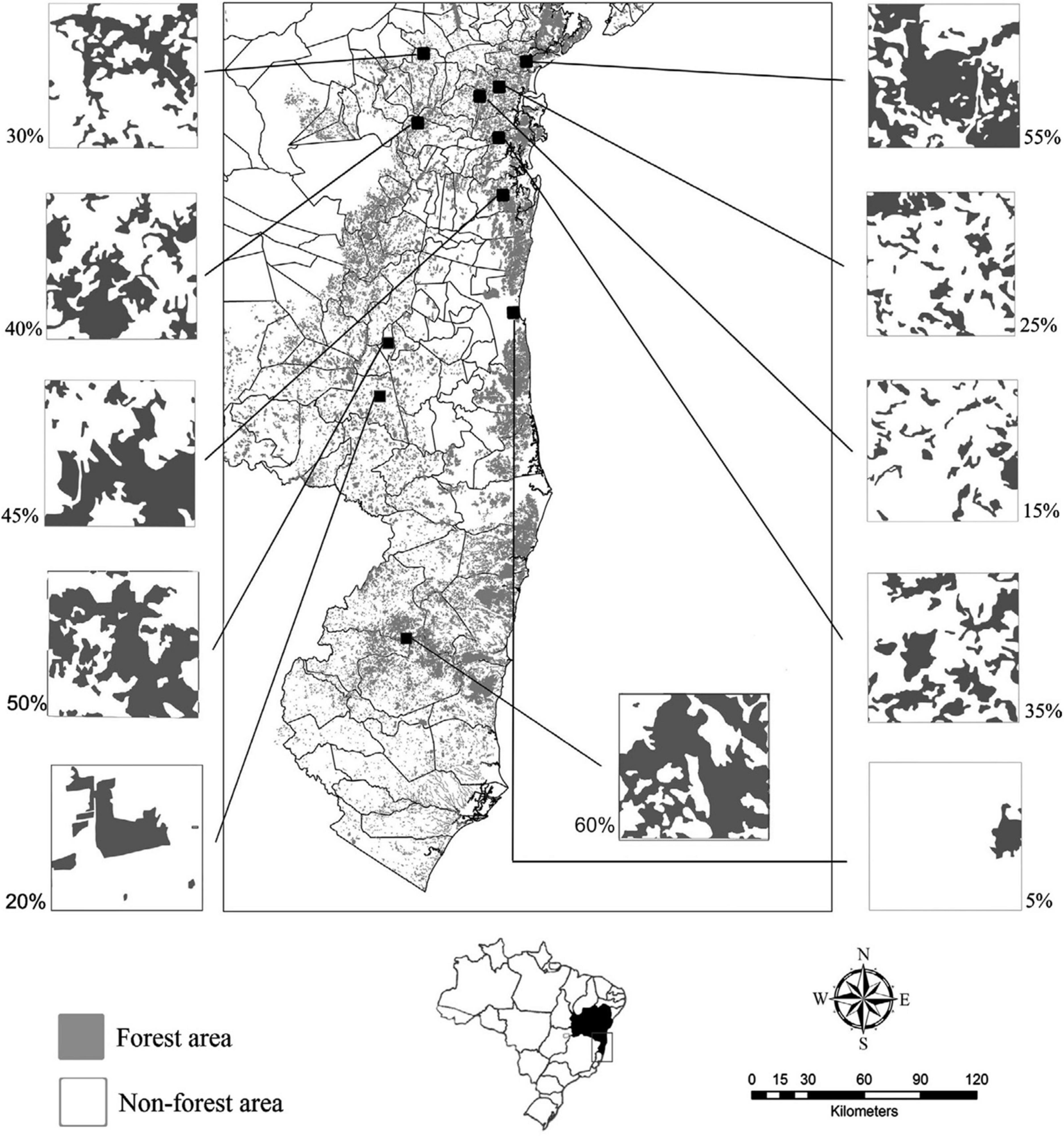
Figure 1. Study area in the state of Bahia, Brazil, Atlantic Forest remnants are in gray, approx. 93.500 km2, from lat 8° 32’ 00” S and long 39° 22’ 49” W to lat 18° 20’ 07” S and long 39° 39’ 48” W. The black squares represent the eleven sampled landscapes. On the side, each sampled landscape with its forest cover percentage at the landscape scale. Above right, the Brazilian map, with the state of Bahia limits.
This region is characterized by a relatively old use and occupation process dating from the early 16th century (Cavalcanti, 2006), and the most intense suppression of its native vegetation occurred only in the mid-twentieth century (Mendonça et al., 1994).
We select the landscapes from 1,500 squares of 6 × 6 km (36 km2) along the Atlantic Forest in Bahia, Brazil, using the forest cover map of the Atlas of the Atlantic Forest Remnants (SOS Mata Atlântica and Instituto Nacional de Pesquisas Espaciais, 2008).1 Through this map, we calculated the proportion of forest habitat in landscapes. Then, we classify, according to the proportion of forest habitat, into the classes 5, 15, 20, 25, 30, 35, 40, 45, 50, 55, and 60%. To be included in a class, the landscape should have forest cover between (X + 2)% and (X−2)%, where “X” represents the class value. The landscape choice represents a size that can include relevant ecological processes for tropical forest trees, such as the dispersion of their propagules and pollen, at the landscape scale (Condit et al., 1992). Only one landscape was chosen in each class of habitat amount.
A sampling of 11 landscapes with specific percentages of habitat coverage requires that the spatial scope of the design has regional proportions (hundreds of kilometers). The immense extension increases the variability of the response variables as an action of confounding factors associated with each locality’s specific conditions. We established selection criteria to minimize the effect of four relevant and controllable variables through design: two related to forest quality (successional stage) and matrix (permeability to typical forest species) and two related to quantitative indicators of the forested habitat in the landscape surroundings (habitat quantity and presence of potential source areas).
2.1.1. Successional stage
There are significant differences in richness and composition between forests with different successional stages (Liebsch et al., 2008; Santos et al., 2008). Thus, we chose to exclude from the sample universe landscapes with forests in the initial stage of succession, including only medium and advanced stages, based on the proposal of CONAMA Resolution 05/1994, corresponding to structural mature and rich forests (Conselho Nacional de Meio Ambiente [CONAMA], 1994).
2.1.2. Matrix permeability
It is also known that non-habitat matrices structurally similar to forests (e.g., silviculture) are more permeable to forest specialist organisms and can minimize the effects of habitat loss and fragmentation at the landscape scale (Ewers and Didham, 2006; Franklin and Lindenmayer, 2009). Therefore, we established in this study that the landscape matrix should comprise at least 80% of open physiognomies (e.g., pasture, grassland, or shrub), non-forested and non-urban.
2.1.3. Habitat amount and presence of source area in the surroundings
To avoid a possible source effect of the surrounding area on the sample landscape, derived from a larger quantity of forests or larger fragments, we established, for each landscape of 36 square kilometers, a larger square of 324 km2 (18 × 18 km) centered on the landscape to control these two variables (Figure 2). We used the larger square to compute the percentage of vegetation cover and the area occupied by the largest fragment (Largest Patch Index - LPI - Fragstats 3.3). We exclude landscapes where the percentage of habitat and LPI coverage in the larger square was greater than in the sample landscape.
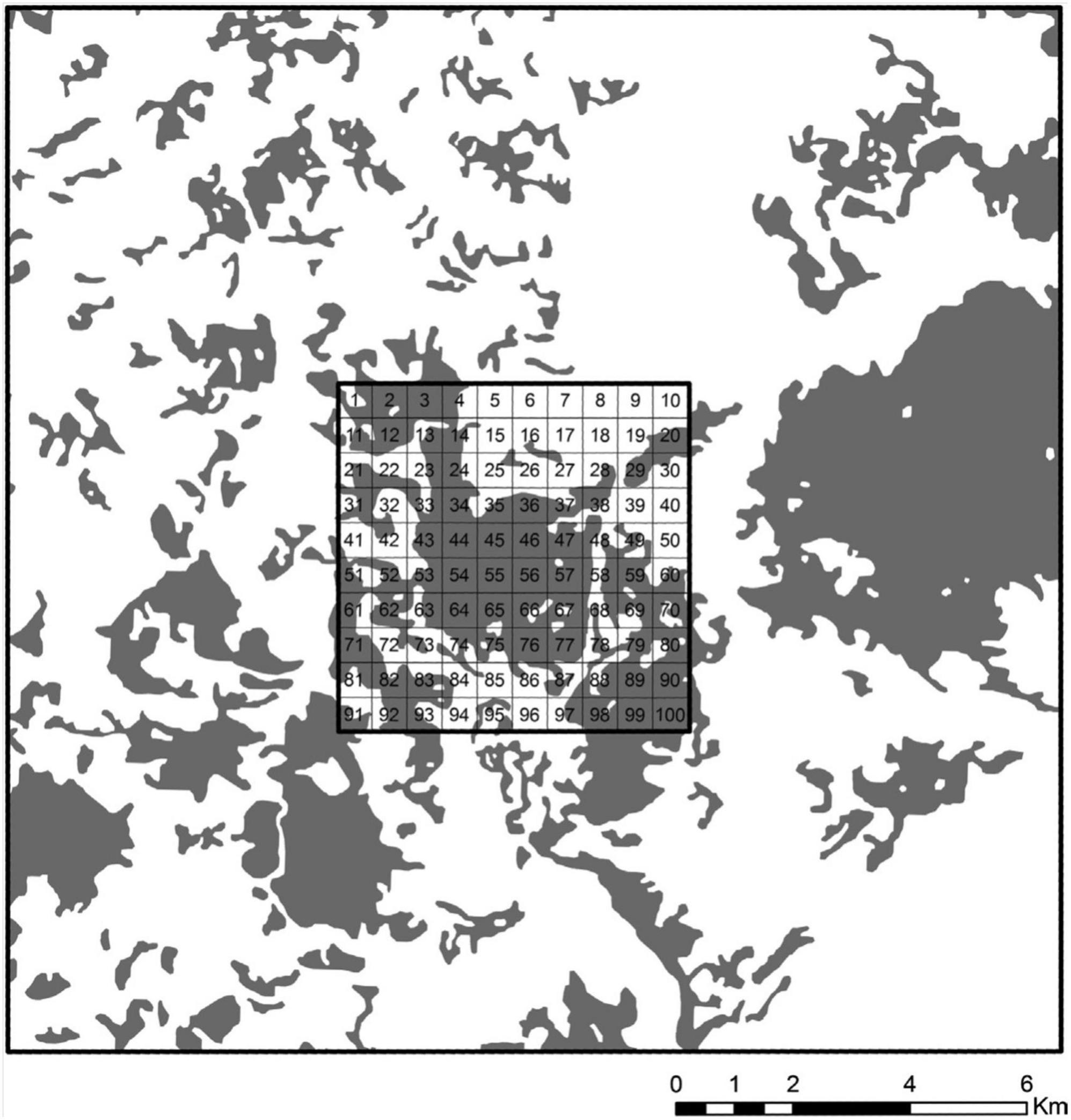
Figure 2. The landscape with 55% of forest cover at the landscape scale, centered on a large landscape of 18 × 18 km. The large landscape was used to control for habitat quality and the presence of source areas surrounding the target 6 × 6 km landscape. Each 6 × 6 km landscape was subdivided into 100 squares, from which eight, containing forests, were randomly chosen for the samplings. Dark areas represent forest cover.
In this study, we tested the strength of the habitat amount at the landscape scale, in a control design, as a predicted variable of forest structure features and carbon stocks. We did not consider fragment size in our analysis. Although fragment size and edge effects could influence our dependent variables locally, we cannot control them in our sampling design; otherwise, there will be no sufficient landscapes for this study.
Our premise that habitat amount at the landscape scale is a good predictor of changes in tree communities is based on simulated landscape studies (Andren, 1994; Fahrig, 2003) and predicts that populations will decrease and eventually become extinct due to reduced habitat amount at the landscape scale. In simulations and real data, several landscapes and patch features are dependent and correlated (linearly or non-linearly) to habitat amount at the landscape scale (Gustafson and Parker, 1992). Including the mean distance between patches, edge density metrics, and mean patch size. Pardini et al. (2010) also pointed out that the correlation between biodiversity metrics and fragment size depends on the habitat amount at the landscape scale. Size and distance are related to the persistence and immigration rates of a metapopulation. In landscapes with a larger amount of habitat, rescue effects could maintain species in small fragments, and in landscapes with lesser amounts of habitat, lack of immigration impedes restoring richness after local extinctions.
Although predictions of the effects of habitat amount at the landscape scale were built for processes at the metapopulation level, the effects have been tested in several community-level studies (Rigueira et al., 2013; Lima and Mariano-Neto, 2014; Morante-Filho et al., 2015; Benchimol et al., 2017a). In this study, we expected some ecological processes to be disrupted inside a landscape as the habitat amount decreases, influencing the community structure and the carbon stocks. We also expect to detect the effects with random samples chosen inside those landscapes, regardless of patch size.
2.2. Sample plots and biological data
To sort the sample plots, we superimposed a grid with 100 cells of 600 × 600 m on the landscape and considered cells that were totally or mostly occupied by forest remnants.
We randomly selected eight cells, and a sample plot was established consisting of a rectangle of 10 × 25 m (250 m2) located at a minimum distance of 50 m from the forest boundary. That avoids the more intense edge effects (Murcia, 1995; Rigueira et al., 2012). In each plot, the circumference at breast height (CBH) and height of all the trees with CBH ≥ 8 cm (diameter of 2.5 cm) were measured. The most abundant families were also evaluated separately, based on the classification proposed by APG III (2009).
The botanical material was collected following IBAMA/ICMBio collection authorization 12023-1 and subsequent authorizations.
2.3. Stored carbon quantification
Initially, we estimated the above-ground biomass (AGB) in trees and palms from allometric equations based on diameter at breast height (DBH). An equation was used for the set of trees, one specific for embaúbas (Cecropia spp.), plus a third equation for the palm trees. Thus, three (03) different allometric equations were used to evaluate the above-ground biomass (AGB), namely:
Atlantic Forest trees (Arevalo et al., 2002):
AGB = 0,1184*(DBH)2,53
Cecropia species of Tropical forests in Bolívia (Pearson et al., 2005):
AGB = 2,764 + 0,2588*(DBH)2.0515
Palm species of Tropical forests (Pearson et al., 2005):
AGB = 6,6666 + (12,826*((DBH)0,5)*ln(DBH))
At where:
AGB, Above-ground biomass.
DBH, Diameter at breast height.
Numeric values = Constants.
We quantified the above-ground stored carbon by multiplication the AGB by 0.5.
2.4. Statistical analyzes
We used a multimodel selection based on the Akaike Information Criterion (ΔAIC) to evaluate the relationship between the forest structure and the habitat amount at the landscape scale (Akaike, 1978). Three models commonly used in studies in fragmented tropical forest landscapes were tested: (1) - null (constant), (2)- linear, and (3)- logistic of four parameters. Parsimony and residual analysis were used as tie-breaking criteria in cases where concurrent models have similar AIC values. The selected models had their residuals analyzed to validate the model.
We assumed Poisson error distribution for counting data of individuals (woody plant abundance: all individuals with DBH ≥ 2.5 cm, and abundance of large trees: only individuals with DBH ≥ 30 cm). For height and DBH data, we used the Gaussian error distribution. Finally, given the residuals heteroscedasticity of the carbon data, a single linearized model (GLM) was made, assuming a negative binomial error distribution. This model was evaluated through its significance and analysis of parameters and residues.
All the analyzes were performed in the R 3.3.2 environment (R Development Core Team., 2017) using the packages “bbmle” (Bolker, 2016), “nlme” (Pinheiro et al., 2016), and “vegan” (Oksanen, 2013).
3. Results
3.1. Community structure of woody plants
We sampled 8,179 woody plants (DAP ≥ 2.5 cm) in the 11 landscapes. The landscape with 55% of habitat presented the highest abundance, with a total of 931 individuals (4,655 indiv./ha), followed by 40% forest cover with 932 individuals (4,615 indiv./ha). The 5% forest landscape had the lowest number of individuals per forest area, totaling 581 individuals sampled (2,905 indiv./ha), followed by the 15% landscape with 594 individuals (2,970 indiv./ha). A positive relationship was observed between habitat loss and the number of individuals of woody plants (Figure 3) through linear adjustment (Table 1).
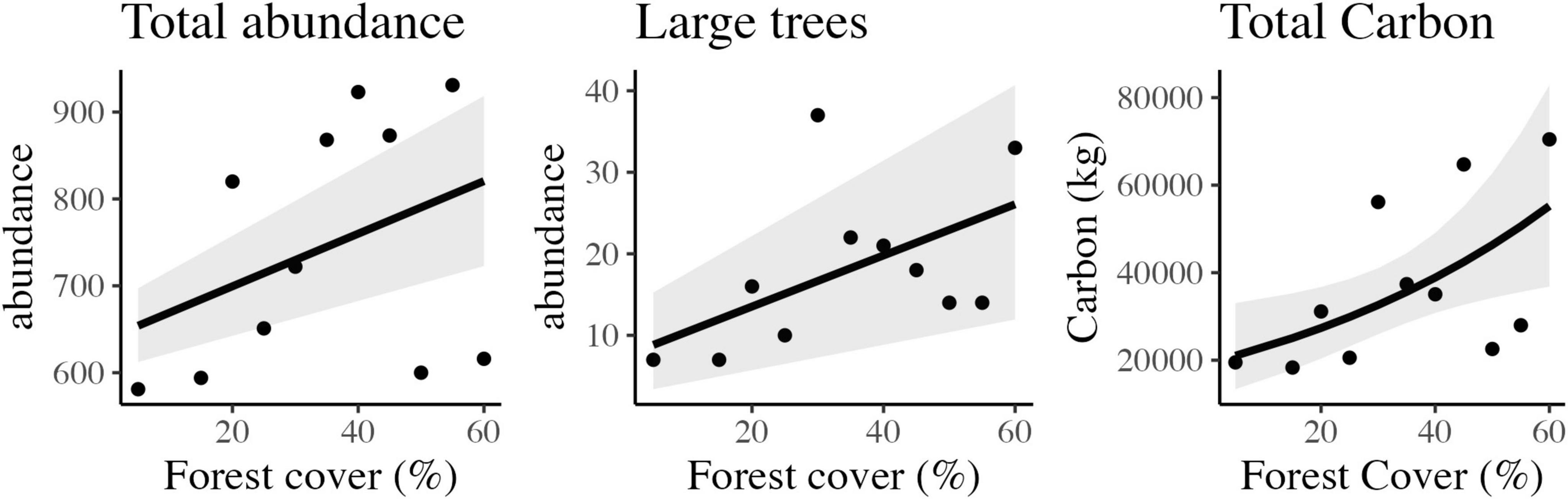
Figure 3. Scatter plots and the best-fitted models with confidence intervals of the observed relationship between forest cover and total tree abundance, large tree abundance, and total carbon stored in tropical rainforest landscapes in Northeast Brazil.
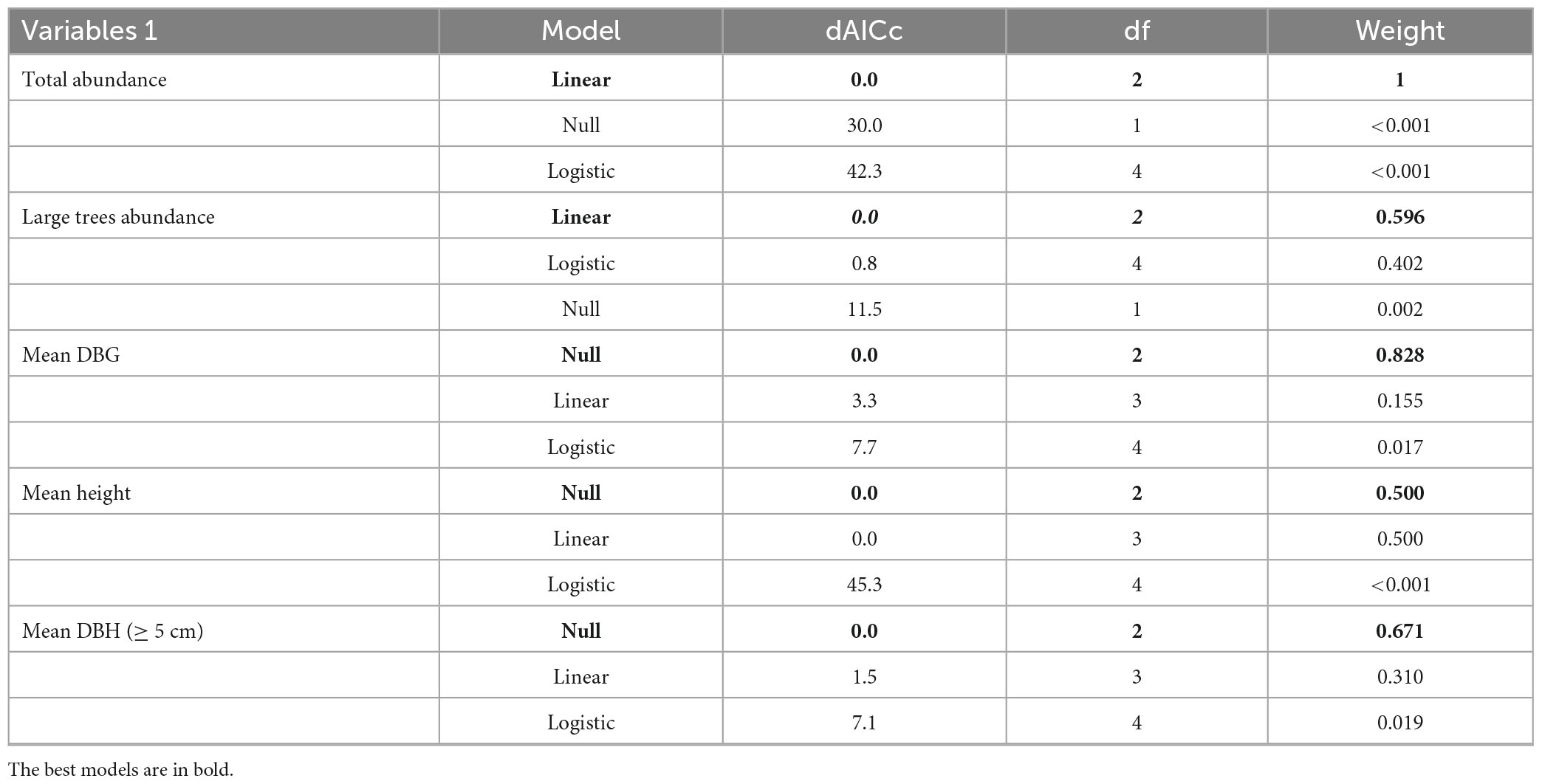
Table 1. Model selection between forest cover at the landscape scale and structural variables of a woody community in Atlantic Forest – Bahia Brazil.
Large tree abundance varied from seven (07), in landscapes of 5 and 15%, to 37 in the landscape of 30% of forest cover. A positive relationship between habitat loss and the number of large trees (DBH ≥ 30 cm) was also observed (Figure 4) with linear adjustment (Table 1).
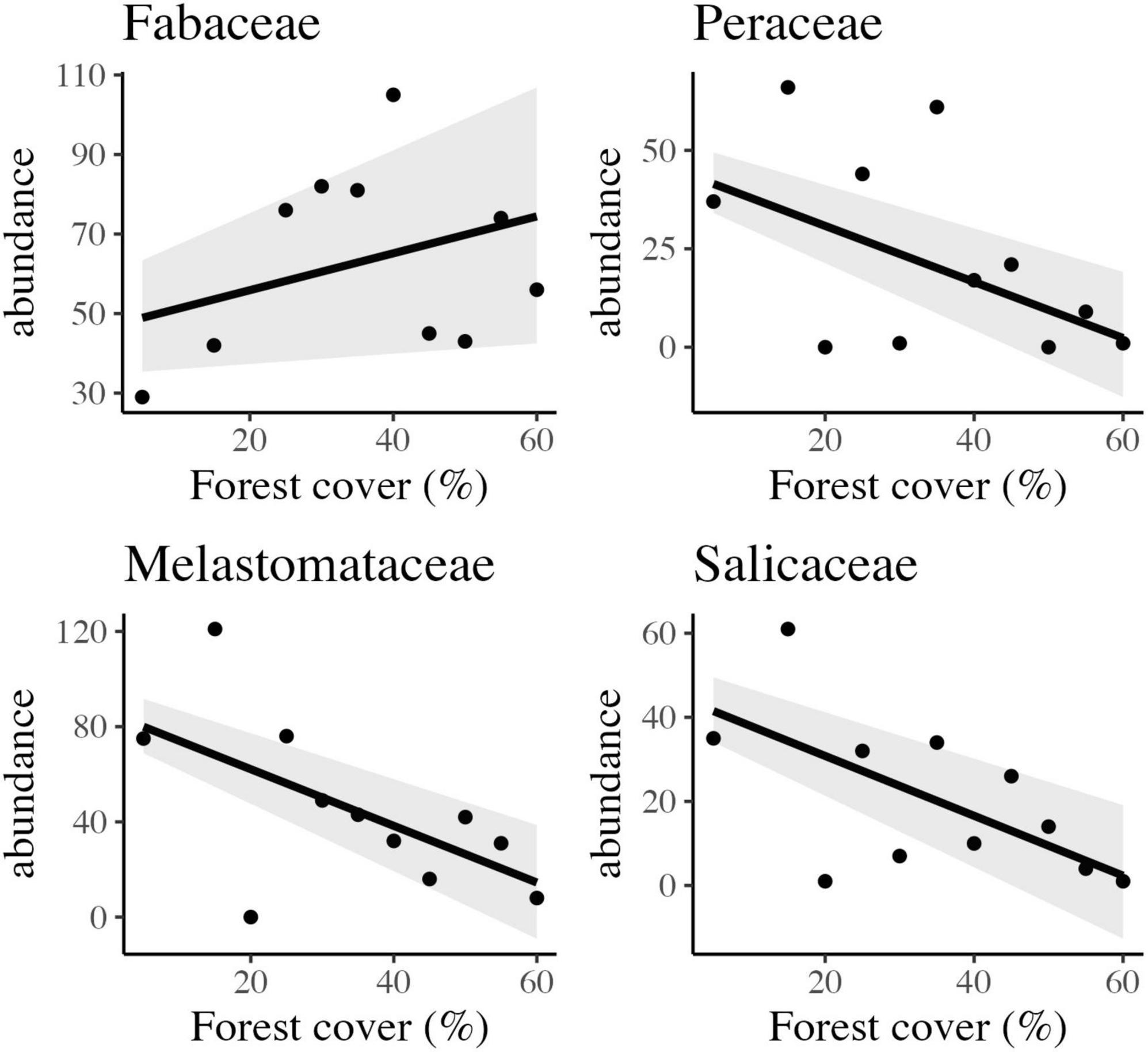
Figure 4. Scatter plots and the best-fitted models with confidence intervals of the observed relationship between forest cover and abundance of four different families of trees in tropical rainforest landscapes in Northeast Brazil.
The mean DBH ranged from 6.9 to 9.49 cm in the 20 and 60% forest landscapes. The average height varied from 7.33 m, in the landscape of 20%, to 10.97 m, in the landscape of 30%. In the case of height, both the linear and the null models were similar (Table 1). Mean DBH was best adjusted to the null model (Table 1), and the linear and null models were equivalent in plants with DBH ≥ 5 cm (Table 1).
3.2. Structural aspects in different woody plants families
Abundant woody families were strongly influenced by forest amount at the landscape scale. The six (06) most abundant families presented a negative or positive response, representing 39.5% of the total abundance sampled. Four responded negatively and linearly to habitat loss: Myrtaceae, Euphorbiaceae, Rubiaceae, and Arecaceae (Figure 5 and Table 2). However, Fabaceae and Melastomataceae presented a positive and linear response, with increased abundance in response to habitat loss.
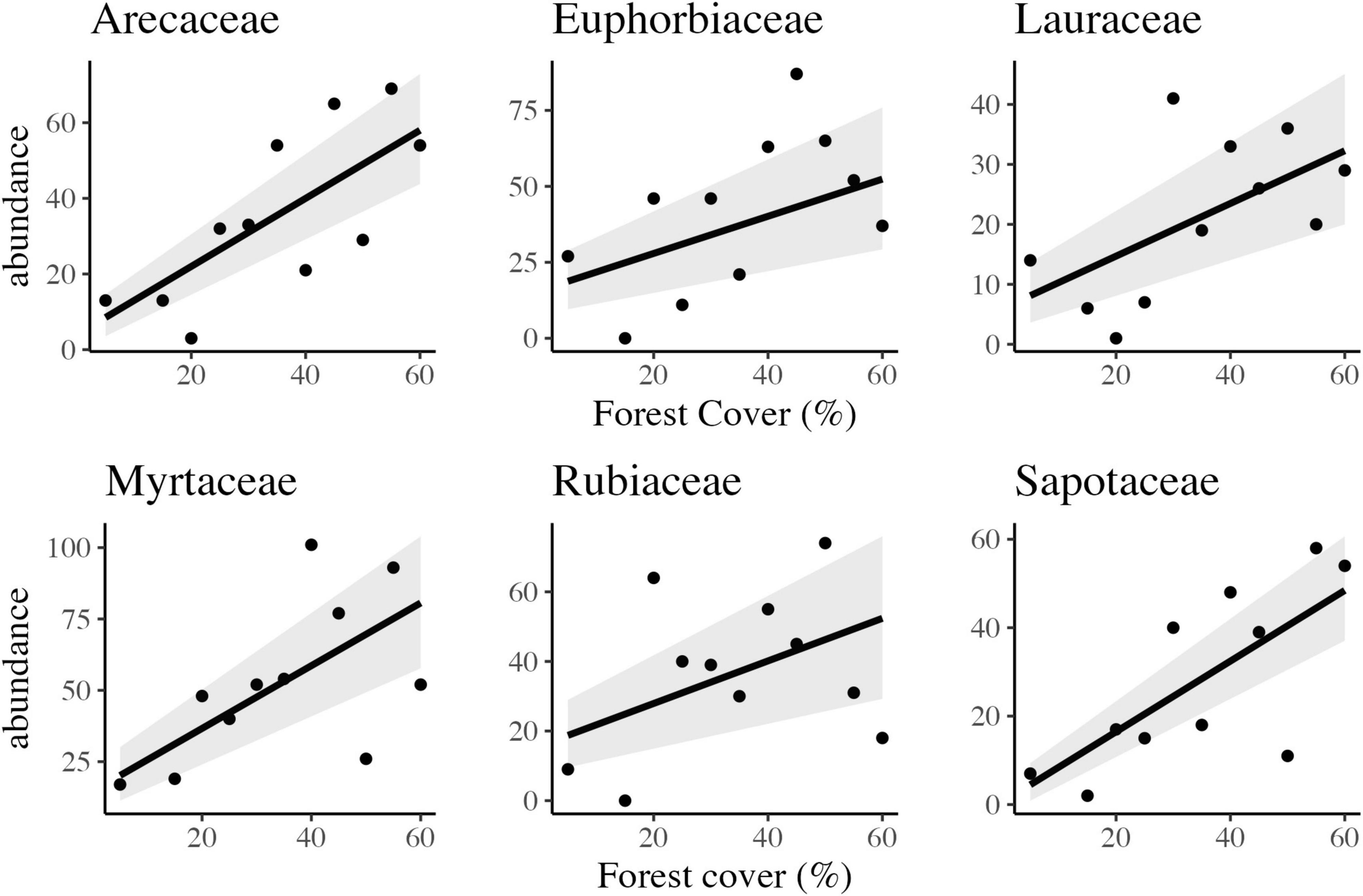
Figure 5. Scatter plots and the best-fitted models with confidence intervals of the observed relationship between forest cover and abundance of six different families of trees in tropical rainforest landscapes in Northeast Brazil.
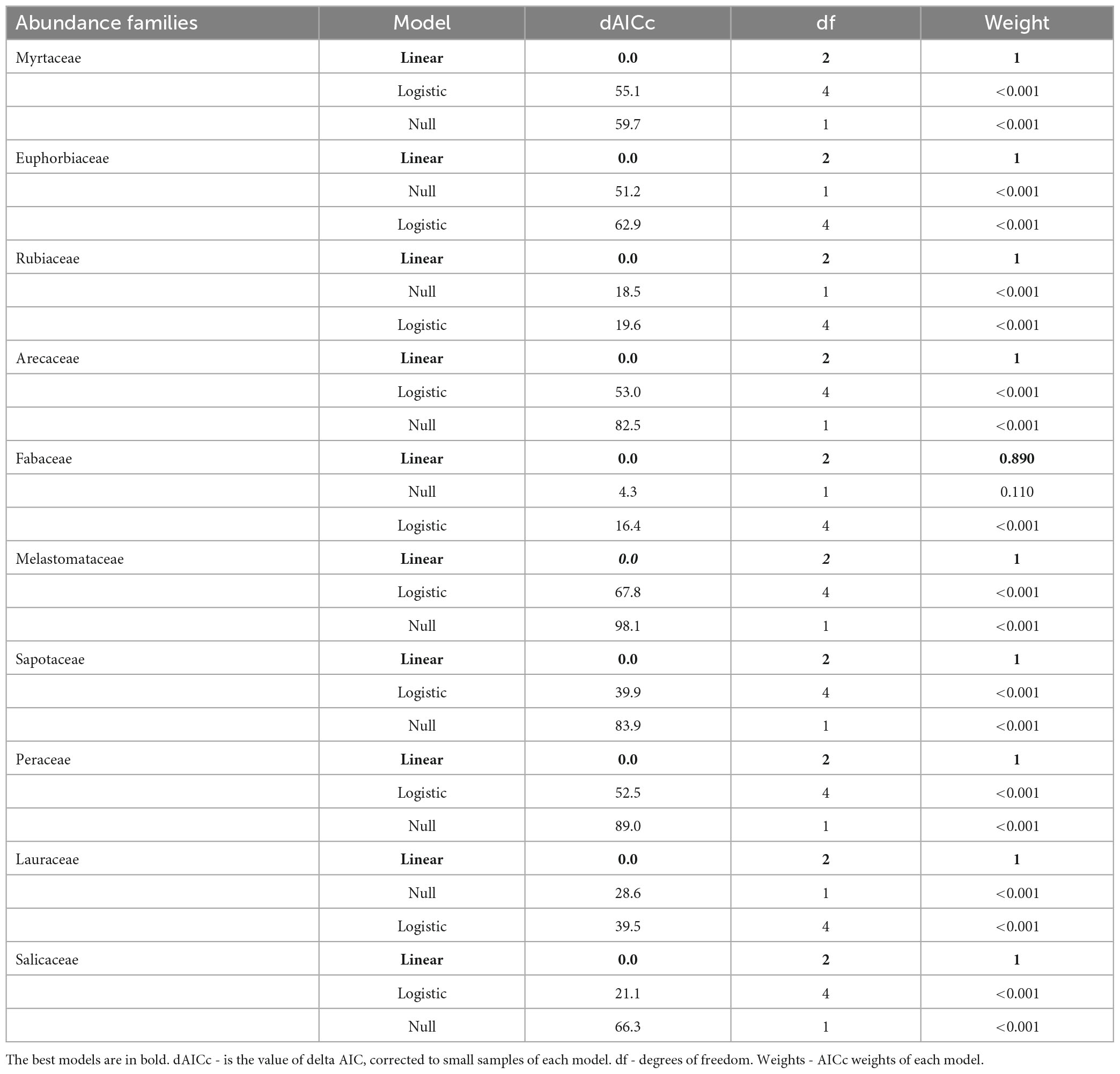
Table 2. Model selection between forest cover at the landscape scale and the abundance of the most important families of a woody community in Atlantic Forest – Bahia, Brazil.
Other important families, with significant saplings abundance, such as Sapotaceae, Peraceae, Lauraceae, and Salicaceae, with 309, 257, 232, and 225 individuals, were also influenced by the habitat amount at the landscape scale (Figure 5). Peraceae and Salicaceae showed a positive and linear response to habitat loss (Figure 5) section “3.3 Carbon stock assessment.”
3.3. Carbon stock assessment
A positive effect on the relationship between habitat loss and stored carbon (Figure 3) was observed with a generalized linear model (p = 0.009). The carbon stored per hectare varied from 18,327 kg (91.63 T/ha), in the landscape of 15%, to 70,466 kg (352.33 T/ha), in the 60% landscape. Considering all the landscapes, the average value of stored carbon was 184.84 T/ha.
4. Discussion
4.1. Habitat loss and changes in the woody plant community
The habitat amount at the landscape scale influences essential aspects of the physical structure of the tropical forest. Several studies have shown the negative influence of fragmentation on some structural elements of tropical forests patches (Laurance et al., 2000, 2002; Santos et al., 2008; Lopes et al., 2009; Magnago L. F. S. et al., 2015; Rocha-Santos et al., 2016). The results of the current study corroborate findings in another similar study on forested landscapes in Atlantic Forest, which indicates a structural alteration associated with habitat loss at the landscape scale (Rocha-Santos et al., 2016); But, in the present study, with linear response to habitat loss.
Regarding the abundance, the results observed in this study do not corroborate the compensatory effect in forest fragments exposed to the strong edge effect (Harper et al., 2005; Gonzalez and Loreau, 2009), which tends to favor pioneer species, leading to the substitution of species and a larger number of trees in small fragments. In the present study, despite the scattering of the data and large confidence intervals, there was an average decline of about 25% in the number of woody individuals per unit area in low forest cover landscapes (5−15%) compared to forest-predominant landscapes (45−60%), representing almost an average of thousand trees per hectare. Although landscapes with less habitat are generally subject to a more substantial edge effect (Fahrig, 2003), the abundance of woody plants in the Atlantic Forest often tends to decline with the habitat fragmentation at the landscape scale, as shown in similar studies (Santos et al., 2008; Rocha-Santos et al., 2016).
One possible explanation for abundance reduction would be the low recruitment of trees in landscapes with reduced amounts of forest. Organisms responsible for pollination and dispersal of different groups of plants also suffer a reduction with habitat loss (Silva and Tabarelli, 2000; Jorge et al., 2013; Poulsen et al., 2013), and the reproductive success and the establishment can get depressed. In addition, reducing the abundance of woody plants also compromises reproductive success as it exposes small populations to the Allee effect (Stephens and Sutherland, 1999).
Total abundance and large trees’ abundance (DBH ≥ 30 cm) reduced linearly with habitat loss. Besides, the large tree abundance of the two landscapes with the lowest proportions of forest (5 and 15%) was notably lower than those with the highest (55 and 60%) forest cover. Based on the data from eight points in each landscape, we estimate an average density of 35 indiv/ha in landscapes of 5 and 15% of forest cover compared to an average of 117 indiv/ha trees in the landscapes with 55 and 60%.
Probably, landscapes with low forest cover experience a reduction of large trees because of several factors, such as the edge effect (Laurance et al., 2000; Oliveira et al., 2008) and the loss of mediating fauna involved in the reproductive processes of large trees (Bello et al., 2015). Furthermore, that is more common and prominent in landscapes with lower proportions of natural habitat (Laurance et al., 2002; Fahrig, 2003). There is a remarkable reduction in the carbon stock retained by these less forested landscapes due to the reduction of the big trees; also, these landscapes presented smaller variances compared to landscapes with higher amounts of forests. Large trees store disproportional amounts of this element and are essential links in carbon cycling (Bello et al., 2015). In addition, the disappearance of large trees leads to microclimatic changes, such as increased light penetration, influencing vegetation regeneration dynamics, and favoring groups of pioneer plants (Laurance et al., 2000).
The forest cover reduction did not influence the average height and DBH of the individuals, probably due to the low recruitment of trees in these conditions. We analyzed mean values of height and thickness. Recruitment drops and small specimens can influence the overall height and DBH trend. However, we did not detect a significant change in these two metrics, even considering only individuals with DBH ≥ 15 cm. This lack of response to habitat loss can be explained by the action of other factors not controlled, such as the different types of soils, with potential influence on the sizes of the trees (Clark and Clark, 2000, Baldeck et al., 2012; Peña-Claros et al., 2012).
There could be a sum or synergy of different factors, such as low recruitment and mortality, especially of large trees, associated with microclimatic changes due to the edge effect in landscapes with low amounts of natural habitat. All those factors possibly cause the structural changes observed, mainly involving the abundance of woody plants and large trees. The process of habitat loss probably has a preponderant influence on the structure of tropical forests.
4.2. Habitat loss and changes in abundance of woody plant families
Different families responded differently to habitat loss. For important Atlantic Forest families, there is a linear reduction of abundance with habitat loss. Such as Myrtaceae, Sapotaceae, Arecaceae, Lauraceae, Rubiaceae, and Euphorbiaceae.
These six families are very expressive in terms of diversity throughout the biome (Giulietti et al., 2005; Souza and Lorenzi, 2005) and form a critical functional group, important as a fruit, pollen, and nectar source for several animals of this ecosystem (Poulin et al., 1999; Tabarelli et al., 1999; Castro and Oliveira, 2002; Tabarelli and Peres, 2002; Castro et al., 2004; Lopes and Buzato, 2005; Bello et al., 2017). For example, palm trees (Arecaceae) are essential for tropical frugivores, producing large quantities of fruits and seeds (Galetti et al., 2013). Some species of Sapotaceae can produce fruits during prolonged periods and represent an important fruit and nectar source throughout the year for a wide range of animals (Oliveira et al., 2010; Pessoa et al., 2011). Rubiaceae and Myrtaceae are among the main sources of fleshy fruits for animals in the Atlantic Forest (Tabarelli et al., 1999; Tabarelli and Peres, 2002; Bello et al., 2017). Thus, reducing the abundance of these families may influence the functioning of different mutualistic processes and may lead to cascading extinctions of various animal groups (Bennett et al., 2009; Kurten, 2013). In fact, Pinto et al. (2021) found a reduction in interactions between frugivorous birds and fleshy fruit plants and simplified bird-plant interaction networks in landscapes with small forest amounts in the same biome. Moreover, they assume that the impoverishment of birds and plant communities was responsible for that pattern.
On the other hand, other families composed mainly of fast-growing early secondary species that are mostly shade-intolerant, such as Melastomataceae, Peraceae, and Salicaceae, had a considerable increase in abundance. Frequently, these families have reproductive processes mediated by physical mechanisms or small animals (Souza and Lorenzi, 2005). Similar response patterns of these families were observed in other studies in the hyper-fragmented tropical forests of northeastern Brazil (Tabarelli and Peres, 2002; Tabarelli et al., 2004; Santos et al., 2008; Lopes et al., 2009).
According to the effect of compensation, this pattern of loss in the abundance of one set of families and the gain of others was expected (Harper et al., 2005; Gonzalez and Loreau, 2009). On the other hand, the overall community pattern was the linear abundance reduction (up to about 25%). Based on our data, we cannot say that the space left by the individuals of families more sensitive to the changes triggered by the habitat loss (e.g., more dependent on mutualistic animals) was occupied by the individuals favored by the edge effects (less tolerant to shade and less dependent on mutualistic animals). Our results indicate that these forests in low-habitat landscapes can become emptier, with less structural complexity, dominated by the secondary process and homogenization of the woody community (Santos et al., 2008; Lopes et al., 2009).
4.3. Habitat loss and carbon stock reduction
A relation between different landscape metrics and carbon stock was observed in some tropical forests (Groeneveld et al., 2009; Pütz et al., 2011, 2014; Magnago L. et al., 2015). However, our study reveals the influence of the habitat amount at the landscape scale on carbon stocks in one of the world’s most endangered rainforests. Also, we find that, compared to the landscapes with the highest forest cover (55−60%), there was an average decrease of 2.6 times in carbon stocks in less forested landscapes (5−15%), equivalent to a loss of 150 T/ha on average.
The average value of carbon stored that we found in the eleven landscapes was 184 T/ha, within the range observed in other tropical forests, such as mature Amazon forests, varying between 170 T/ha (Pan et al., 2011) and 320 T/ha (Houghton et al., 2000), and in other forest formations of the Atlantic Forest, between 125 T/ha (Groeneveld et al., 2009) and 360 T/ha (Magnago L. et al., 2015). However, it differed significantly from values observed in Atlantic Forest’s initial successional stages, around 19 T/ha (Ribeiro et al., 2010). Our landscapes with a low proportion of forest habitat (e.g., 5 and 15%) showed an average of 94.5 T/ha, and our sampling design aimed to avoid areas in early successional stages, even in the landscapes with low forest cover. This pattern indicates that landscapes with lower forest cover ratios have reduced stock capacity in the late secondary stages — a loss of a critical ecological process in the ecosystem’s functioning.
Recognizing such patterns for tropical forests is essential, given their importance to global carbon sequestration and stocking (Dixon et al., 1994; Millennium Ecosystem Assessment [MEA]., 2005; Pan et al., 2011). In tropical forests’ carbon cycling process, the sequestration and stocking by woody plants represent one of the primary services for climate regulation on a local, regional and global scale (Millennium Ecosystem Assessment [MEA]., 2005).
Some processes can explain the carbon stock loss in tropical forests, such as the reduction in the recruitment of trees with denser woods, mutualistic faunal loss (Bello et al., 2015), fragmentation, and an increase in the edge proportion (Laurance et al., 2000), in addition to the differential loss of large trees due to the edge effect (Groeneveld et al., 2009; Pütz et al., 2011, 2014; Magnago L. F. S. et al., 2015). However, such processes ultimately derive from habitat loss.
Our results are probably conservative since the values of stored carbon were obtained using three sets of plants (Cecropia trees, palm trees, and other trees). Thus, the category “other trees” encompasses a large set of trees of different ecological groups and, consequently, with different values of stored carbon (Groeneveld et al., 2009). The carbon stored in landscapes with low forest cover is probably lower than those estimated here since those communities present an increase in more softwood and fast-growing plants, such as in the Melastomataceae. By incorporating the different wood densities associated with functional groups, more disparate patterns among the landscapes with larger and smaller proportions could emerge.
4.4. Structural changes, homogenization, and regime shift
Structural variables of the woody vegetation reflect the biomass and abundance changes in response to the forest cover reduction and changes in functional groups. Other studies dealing with species richness in the Atlantic Forest have revealed non-linear loss patterns through thresholds. These thresholds, occurring around 30% of habitat on the landscape scale, were observed for the species richness of some plant families (Rigueira et al., 2013; Lima and Mariano-Neto, 2014; Andrade et al., 2015; Rueda et al., 2015; Benchimol et al., 2017b), and also for forest specialists animals (Pardini et al., 2010; Banks-Leite et al., 2011; Estavillo et al., 2013). In addition, another study in the Atlantic Forest reported thresholds associated with altered forest structure have also been reported (Rocha-Santos et al., 2016), indicating that abrupt responses could also occur with carbon stocks and other structural aspects. In the present study, some fast-growing families had a positive response to habitat loss, which may have masked a possible threshold, linearizing the pattern of a total loss, if the analysis were concentrated on shade-tolerant species and more reliant on mutualistic animals, for example.
Oliver et al. (2015) presented a model to understand the resilience of a complex system subjected to disturbances regarding different life strategies. A turnover between fast-growing and slowly-growing species is expected to occur locally after disturbances, the first group is responsible for the rapid recovery (adaptation), and the second is responsible for the long-term resistance to minor disturbances. The existence of these two groups is essential to the overall resilience of a system at the community level because they maintain positive feedbacks that keep the system. Regarding carbon storage, slow-growing species are responsible for a significant portion compared to fast-growing species. Both are important to maintaining the system functioning following Oliver et al. (2015) resilience model.
The loss of slow-growing species in landscapes with small amounts of forested areas means that those forests could lose their resilience (positive feedback mechanisms) and be subject to a regime shift, as Scheffer and Carpenter (2003) proposed: Forest reduction at the landscape scale can be viewed as a chronicle factor, and there is evidence of several non-linear responses at the community level (Banks-Leite et al., 2011; Estavillo et al., 2013; Rigueira et al., 2013; Lima and Mariano-Neto, 2014; Andrade et al., 2015; Rueda et al., 2015; Benchimol et al., 2017b), even if an emergent property, such as carbon storage presented a linear response to forest reduction.
A regime shift with a preponderance of fast-growing species could mean an unfriendly scenario for the Atlantic Forest landscapes with reduced proportions of forest cover. Less key forest species, structural alteration, compromise ecosystem services, and maintaining forest remnants in levels of early stages of succession, even in the long run (Santos et al., 2008; Tabarelli et al., 2008, 2012; Lopes et al., 2009; Rocha-Santos et al., 2016).
4.5. Subsidies for the management of forested tropical landscapes
Changes in tree abundance may indicate vegetation structure and composition derived from local environmental disturbances (Karna et al., 2010; Nagendra et al., 2010). Also, maintaining carbon stocks in tropical forests linked with maintaining biodiversity (Magnago L. et al., 2015). In the present study, verifying the positive influence of the habitat amount at the landscape scale on the abundance of woody plants and the carbon stored in these organisms was possible.
The processes that triggered such structural changes in the Atlantic Forest were identified in the range of forest cover variation between 5 and 60%. Woody plants are a biological model with fairly robust responses to habitat loss. Thus, these results provide evidence about the relationship between structural loss and resilience of Atlantic Forest landscapes, and could be used as a reference for managing forested tropical landscapes. As landscape metrics, such as habitat quantity, are easy to acquire (Banks-Leite et al., 2012; Nagendra, 2012), this information can aid decision-making, the definition of conservation strategies, and the standardization of appropriate management techniques in tropical forest landscapes.
The sampling procedures and results observed here have practical implications for the quantification of carbon stocks in fragmented landscapes of the Atlantic Forest and, consequently, may favor the establishment of policies aimed at the payment for this ecosystem service. One of the fastest-growing markets for ecosystem services is related to carbon sequestration (Millennium Ecosystem Assessment [MEA]., 2005). Only in 2012, this market handled more than € 100 billion. In 2015, around 6.2 gigatons of emission allowances and offsets were traded globally, amounting to € 48.4 billion (Thomson Reuters., 2016). Thus, conservation strategies based on payment for environmental services of sequestration and carbon stock in tropical forests, such as the Atlantic Forest, should consider the habitat amount at the landscape scale in that compute.
Data availability statement
The original contributions presented in this study are included in the article/supplementary material, further inquiries can be directed to the corresponding author.
Author contributions
DR and EM-N: idea, results, manuscript writing, analyses, and finally produce the manuscript. Both authors contributed to the article and approved the submitted version.
Funding
This work was supported by the Foundation for Research support of the State of Bahia (FAPESB) and the National Council for Scientific and Technological Development (CNPq) for the funding of this study (grant nos. APP0049/2009, PNX0016/2009, and PPP0004/2010), the Coordination for the Improvement of Higher Education Personnel (CAPES) for the scholarship granted, and to CNPq by the productivity grant of researcher EM-N (310901/2015-4).
Acknowledgments
We thank the Brazilian Institute for the Environment and Renewable Natural Resources (IBAMA) and the State Secretariat of the Environment of Bahia (SEMA) for logistical support and the granting of collection licenses, Rio Tinto and Odebrecht for the loan of part of the cars used in the study, to the municipal governments of the localities where the collections were made, for the logistical support; Charbel El-Hani, Pedro L. B. da Rocha and Nadia Roque, for the design of the Project Threshold and rich guidelines, Alessandra Nasser Caiafa, Deborah Faria, Mauro Ramalho, and Ricardo Dobrovolski for contributions and suggestions, the entire Threshold Project team for support in fieldwork, and the entire HALCB, Flora, and LEV team at UFBA for their support throughout the collection and sorting work.
Conflict of interest
The authors declare that the research was conducted in the absence of any commercial or financial relationships that could be construed as a potential conflict of interest.
Publisher’s note
All claims expressed in this article are solely those of the authors and do not necessarily represent those of their affiliated organizations, or those of the publisher, the editors and the reviewers. Any product that may be evaluated in this article, or claim that may be made by its manufacturer, is not guaranteed or endorsed by the publisher.
Footnotes
References
Akaike, H. (1978). A Bayesian analysis of the minimum AIC procedure. Ann. Inst. Statist. Math. 30, 9–14. doi: 10.1007/BF02480194
Andrade, E. R., Jardim, J. G., Santos, B. A., Melo, F. P. L., Talora, D. C., Faria, D., et al. (2015). Effects of habitat loss on taxonomic and phylogenetic diversity of understory Rubiaceae in Atlantic forest landscapes. For. Ecol. Manag. 349, 73–84. doi: 10.1016/j.foreco.2015.03.049
Andren, H. (1994). Effects of habitat fragmentation on birds and mammals in landscapes with different proportions of suitable habitat: a review. Oikos 71, 355–366. doi: 10.2307/3545823
Arevalo, L. A., Alegre, J. C., and Vilcahuaman, L. J. M. (2002). Metodologia para estimar o estoque de carbono em diferentes sistemas de uso da terra. Colombo: Embrapa Florestas.
Baldeck, C., Harms, K., Yavitt, J., John, R., Turner, B., Valencia, R., et al. (2012). Soil resources and topography shape local tree community structure in tropical forests. Proc Biol Sci. 280, 20122532. doi: 10.1098/rspb.2012.2532
Banks-Leite, C., Ewers, R. M., Kapos, V., Martensen, A. C., and Metzger, J. P. (2011). Comparing species and measures of landscape structure as indicators of conservation importance. J. Appl. Ecol. 48, 706–714. doi: 10.1111/j.1365-2664.2011.01966.x
Banks-Leite, C., Ewers, R. M., Pimentel, R., and Metzger, J. P. (2012). Decisions on temporal sampling Protocol Influence the Detection of Ecological Patterns. Biotropica 44, 378–385. doi: 10.1111/j.1744-7429.2011.00801.x
Banks-Leite, C., Pardini, R., Tambosi, L., Pearse, W., Bueno, A., Bruscagin, R., et al. (2014). Using ecological thresholds to evaluate the costs and benefits of set-asides in a biodiversity hotspot. Science 345, 1041–1045. doi: 10.1126/science.1255768
Bello, C., Galetti, M., Pizo, M., Magnago, L., Rocha, M., Lima, R., et al. (2015). Defaunation affects carbon storage in tropical forests. Sci Adv. 1, e1501105. doi: 10.1126/sciadv.1501105
Bello, C., Galetti, M., Montan, D., Pizo, M., Mariguela, T., Culot, L., et al. (2017). Atlantic frugivory: a plant-frugivore interaction data set for the Atlantic Forest. Ecology 98, 1729. doi: 10.1002/ecy.1818
Benchimol, M., Mariano-Neto, E., Faria, D., Rocha-Santos, L., Pessoa, M. S., Gomes, F. S., et al. (2017a). Translating plant community responses to habitat loss into conservation practices: Forest cover matters. Biological Conservation 209, 499–507. doi: 10.1016/j.biocon.2017.03.024
Benchimol, M., Talora, D. C., Mariano-Neto, E., Oliveira, T. L. S., Leal, A., Mielke, M. S., et al. (2017b). Losing our palms: The influence of landscape-scale deforestation on Arecaceae diversity in the Atlantic Forest. For. Ecol. Manag. 384, 314–322. doi: 10.1016/j.foreco.2016.11.014
Bennett, A. F., Haslem, A., Cheal, D., Clarke, M. F., Jones, R. N., Koehn, J., et al. (2009). Ecological processes: a key element in strategies for nature conservation. Ecol. Manage. Restor. 10, 192–199. doi: 10.1111/j.1442-8903.2009.00489.x
Bolker, B. M. (2016). bbmle: Tools for general maximum likelihood estimation. R package version 1.0.18.
Brown, J. H., Gillooly, J. F., Allen, A. P., Savage, V. M., and West, G. B. (2004). Toward a metabolic theory of ecology. Ecology 85, 1771–1789. doi: 10.1890/03-9000
Castro, C., Oliveira, P., and Alves, M. (2004). Breeding system and floral morphometry of distylous Psychotria L. species in the Atlantic rain forest, SE Brazil. Plant Biol 6, 755–760. doi: 10.1055/s-2004-830349
Castro, C. C., and Oliveira, P. E. (2002). Pollination biology of distylous Rubiaceae in the Atlantic rain forest, SE Brazil. Plant. Biol. 4, 640–646. doi: 10.1055/s-2002-35433
Cavalcanti, C. (2006). “Opulência vegetal, cobiça insaciável e a entropização da entropia: uma visão da história socioambiental da Mata Atlântica,” in Fragmentos da Mata Atlântica do Nordeste – biodiversidade, conservação e suas bromélias, eds J. A. Siqueira-Filho and E. Leme (Rio de Janeiro: Andrea Jakobsson Estúdio).
Clark, D. B., and Clark, D. A. (2000). Landscape-scale variation in forest structure and biomass in a tropical rain forest. For. Ecol. Manage. 137, 185–198. doi: 10.1016/S0378-1127(99)00327-8
Conselho Nacional de Meio Ambiente [CONAMA]. (1994). Resolução n°05 que define vegetação primária e secundária nos estágios inicial, médio e avançado de regeneração da Mata Atlântica, a fim de orientar os procedimentos de licenciamento de atividades florestais no Estado da Bahia. DOU 101:7912-7913.
Condit, R., Hubbell, S., and Foster, R. (1992). Recruitment near conspecific adults and the maintenance of tree and shrub diversity in a neotropical forest. Am Nat. 140, 261–286. doi: 10.1086/285412
Condit, R., Sukumar, R., Hubbell, S., and Foster, R. (1998). Predicting population trends from size distributions: a direct test in a tropical tree community. Am Nat. 152, 495–509. doi: 10.1086/286186
Coomes, D. A., Duncan, R. P., Allen, R. B., and Truscott, J. (2003). Disturbances prevent stem size-density distributions in natural forests from following scaling relationships. Ecol. Lett. 6, 980–989. doi: 10.1046/j.1461-0248.2003.00520.x
Dixon, R., Solomon, A., Brown, S., Houghton, R., Trexier, M., and Wisniewski, J. (1994). Carbon pools and flux of global forest ecosystems. Science 263, 185–190. doi: 10.1126/science.263.5144.185
Echeverría, C., Newton, A. C., Lara, A., Benayas, J. M. R., and Coomes, D. A. (2007). Impacts of forest fragmentation on species composition and forest structure in the temperate landscape of southern Chile. Glob. Ecol. Biogeogr. 16, 426–439. doi: 10.1111/j.1466-8238.2007.00311.x
Enquist, B., West, G., and Brown, J. (2009). Extensions and evaluations of a general quantitative theory of forest structure and dynamics. Proc Natl Acad Sci U S A 106, 7046–7051. doi: 10.1073/pnas.0812303106
Estavillo, C., Pardini, R., and da Rocha, P. (2013). Forest loss and the biodiversity threshold: an evaluation considering species habitat requirements and the use of matrix habitats. PLoS One 8:e82369. doi: 10.1371/journal.pone.0082369
Ewers, R., and Didham, R. (2006). Confounding factors in the detection of species responses to habitat fragmentation. Biol Rev Camb Philos Soc. 81, 117–142. doi: 10.1017/S1464793105006949
Fahrig, L. (2003). Effects of habitat fragmentation on biodiversity. Annu. Rev. Ecol. Evol. Syst. 34, 487–515. doi: 10.1146/annurev.ecolsys.34.011802.132419
Franklin, J., and Lindenmayer, D. (2009). Importance of matrix habitats in maintaining biological diversity. Proc Natl Acad Sci U S A 106, 349–350. doi: 10.1073/pnas.0812016105
Galetti, M., and Dirzo, R. (2013). Ecological and evolutionary consequences of living in a defaunated world. Biol. Conserv. 163, 1–6. doi: 10.1016/j.biocon.2013.04.020
Galetti, M., Guevara, R., Côrtes, M., Fadini, R., Von Matter, S., Leite, A., et al. (2013). Functional extinction of birds drives rapid evolutionary changes in seed size. Science 340, 1086–1090. doi: 10.1126/science.1233774
Giulietti, A. M., Harley, R. M., Queiroz, L. P., Wanderley, M. G. L., and van den Berg, C. (2005). Biodiversity and conservation of plants in Brazil. Conserv. Biol. 19, 632–639. doi: 10.1111/j.1523-1739.2005.00704.x
Gonzalez, A., and Loreau, M. (2009). The Causes and Consequences of Compensatory Dynamics in Ecological Communities Annu. Rev. Ecol. Evol. Syst. 40, 393–414. doi: 10.1146/annurev.ecolsys.39.110707.173349
Groeneveld, J., Alves, L. F., Bernacci, L. C., Catharino, E. L. M., Knogge, C., Metzger, J. P., et al. (2009). The impact of fragmentation and density regulation on forest succession in the Atlantic rain forest. Ecol. Modell. 220, 2450–2459. doi: 10.1016/j.ecolmodel.2009.06.015
Gustafson, E. J., and Parker, G. R. (1992). Relationships between landcover proportion and indices of landscape spatial pattern. Land. Ecol. 7, 101–110. doi: 10.1007/BF2418941
Harper, K. A., Macdonald, S. E., Burton, P. J., Chen, J., Brosofske, K. D., Saunders, S. C., et al. (2005). Edge influence on forest structure and composition in fragmented landscapes. Conserv. Biol. 19, 768–782. doi: 10.1111/j.1523-1739.2005.00045.x
Harris, N., Brown, S., Hagen, S., Saatchi, S., Petrova, S., Salas, W., et al. (2012). Baseline map of carbon emissions from deforestation in tropical regions. Science 336, 1573–1576. doi: 10.1126/science.1217962
Houghton, R., Skole, D., Nobre, C., Hackler, J., Lawrence, K., and Chomentowski, W. (2000). Annual fluxes of carbon from deforestation and regrowth in the Brazilian Amazon. Nature 403, 301–304. doi: 10.1038/35002062
Jorge, M. L. S. P., Galetti, M., Ribeiro, M. C., and Ferraz, K. M. P. M. B. (2013). Mammal defaunation as surrogate of trophic cascades in a biodiversity hotspot. Biol. Conserv. 163, 49–57. doi: 10.1016/j.biocon.2013.04.018
Karna, B. K., Shivakoti, G. P., and Webb, E. W. (2010). Resilience of community forestry under conditions of armed conflict in Nepal. Environ. Conserv. 37, 51–61. doi: 10.1017/S0376892910000263
Kurten, E. L. (2013). Cascading effects of contemporaneous defaunation on tropical forest communities. Biol. Conserv. 163, 22–32. doi: 10.1016/j.biocon.2013.04.025
Laurance, W. F. (2009). Conserving the hottest of the hotspots. Biol. Conser. 142, 1137. doi: 10.1016/j.biocon.2008.10.011
Laurance, W., Delamônica, P., Laurance, S., Vasconcelos, H., and Lovejoy, T. (2000). Rainforest fragmentation kills big trees. Nature 404, 836. doi: 10.1038/35009032
Laurance, W. F., Lovejoy, T. E., Vasconcelos, H. L., Gascon, C., Bierregaard, R. O., Laurance, S. G., et al. (2002). Ecosystem decay of Amazonian Forest fragments: a 22-year investigation. Conserv. Biol. 16, 605–618. doi: 10.1046/j.1523-1739.2002.01025.x
Laurance, W., Nascimento, H., Laurance, S., Andrade, A., Ribeiro, J., Giraldo, J., et al. (2006). Rapid decay of tree-community composition in Amazonian forest fragments. Proc Natl Acad Sci U S A 103, 19010–19014. doi: 10.1073/pnas.0609048103
Laurance, W. F., and Curran, T. J. (2008). Impacts of wind disturbance on fragmented tropical forests: A review and synthesis. Austral. Ecol. 33, 399–408. doi: 10.1111/j.1442-9993.2008.01895.x
Laurance, W. F., Camargo, J. L. C., Luizão, R. C. C., Laurance, S. G., Pimm, S. L., Bruna, E. M., et al. (2011). The fate of Amazonian forest fragments: a 32-year investigation. Biol. Conserv. 144, 56–67. doi: 10.1016/j.biocon.2010.09.021
Liebsch, D., Marques, M. C. M., and Goldenberg, R. (2008). How long does the Atlantic Rain Forest take to recover after a disturbance? Changes in species composition and ecological features during secondary succession. Biol. Conserv. 141, 1717–1725. doi: 10.1016/j.biocon.2008.04.013
Lima, M. M., and Mariano-Neto, E. (2014). Extinction thresholds for Sapotaceae due to forest cover in Atlantic Forest landscapes. For. Ecol. Manage. 312, 260–270. doi: 10.1016/j.foreco.2013.09.003
Liu, J. J., and Slik, J. W. F. (2014). Forest fragment spatial distribution matters for tropical tree conservation. Biol. Conserv. 171, 99–106. doi: 10.1016/j.biocon.2014.01.004
Lopes, L., and Buzato, S. (2005). Biologia reprodutiva de Psychotria suterella Muell. Arg. (Rubiaceae) e a abordagem de escalas ecológicas para a fenologia de floração e frutificação. Rev. Bras. Bot. 28, 785–795. doi: 10.1590/S0100-84042005000400013
Lopes, A. V., Girão, L. C., Santos, B. A., Peres, C. A., and Tabarelli, M. (2009). Long-term erosion of tree reproductive trait diversity in edge-dominated Atlantic forest fragments. Biol. Conserv. 142, 1154–1165. doi: 10.1016/j.biocon.2009.01.007
Magnago, L., Magrach, A., Laurance, W., Martins, S., Meira-Neto, J., Simonelli, M., et al. (2015). Would protecting tropical forest fragments provide carbon and biodiversity cobenefits under REDD+? Glob Chang Biol 21, 3455–3468. doi: 10.1111/gcb.12937
Magnago, L. F. S., Rocha, M. F., Meyer, L., Martins, S. V., and Meira-Neto, J. A. A. (2015). Microclimatic conditions at forest edges have significant impacts on vegetation structure in large Atlantic Forest fragments. Biodivers. Conserv. 24, 2305–2318. doi: 10.1007/s10531-015-0961-1
Matlack, G. R. (1993). Microenvironmental variation within and among deciduous forest edge sites in the eastern United States. Biol. Conserv. 66, 185–194. doi: 10.1016/0006-3207(93)90004-K
Millennium Ecosystem Assessment [MEA]. (2005). Ecosystems and Human Well-being: Biodiversity Synthesis. Washington, DC: World Resources Institute.
Mendonça, J. R., de Carvalho, A. M., Mattos-Silva, L. A., and Thomas, W. W. (1994). 45 anos de desmatamento no sul da Bahia, remanescentes da Mata Atlântica – 1945, 1960, 1974, 1990. Ilhéus: Projeto Mata Atlântica Nordeste/CEPEC.
Melo, F., Arroyo-Rodríguez, V., Fahrig, L., Martínez-Ramos, M., and Tabarelli, M. (2013). On the hope for biodiversity-friendly tropical landscapes. Trends Ecol Evol. 28, 462–468. doi: 10.1016/j.tree.2013.01.001
Morante-Filho, J. C., Faria, D., Mariano-Neto, E., and Rhodes, J. (2015). Birds in anthropogenic landscapes: The responses of ecological groups to forest loss in the Brazilian Atlantic forest. PLoS One 10:e0128923. doi: 10.1371/journal.pone.0128923
Muller-Landau, H., Condit, R., Harms, K., Marks, C., Thomas, S., Bunyavejchewin, S., et al. (2006). Comparing tropical forest tree size distributions with the predictions of metabolic ecology and equilibrium models. Ecol Lett. 9, 589–602. doi: 10.1111/j.1461-0248.2006.00915.x
Murcia, C. (1995). Edge effects in fragmented forests: implications for conservation. Trends Ecol Evol. 10, 58–62. doi: 10.1016/S0169-5347(00)88977-6
Myers, N., Mittermeier, R., Mittermeier, C., da Fonseca, G., and Kent, J. (2000). Biodiversity hotspots for conservation priorities. Nature 403, 853–858. doi: 10.1038/35002501
Nagendra, H., Rocchini, D., and Ghate, R. (2010). Beyond parks as monoliths: spatially differentiating park-people relationships in the Tadoba Andhari Tiger Reserve in India. Biol. Conserv. 143, 2900–2908. doi: 10.1016/j.biocon.2010.04.050
Nagendra, H. (2012). Assessing relatedness and redundancy of forest monitoring and change indicators. J Environ Manage 95, 108–113. doi: 10.1016/j.jenvman.2011.10.002
Norberg, J., Wilson, J., Walker, B., and Ostrom, E. (2008). “Diversity and resilience of socio-ecological systems,” in Complexity theory for a sustainable future, eds J. Norberg and G. S. Cumming (New York: Columbia University Press), 46–80.
Oliveira, M. A., Santos, A. M. M., and Tabarelli, M. (2008). Profound impoverishment of the large tree stand in a hyper-fragmented landscape of the Atlantic forest. For. Ecol. Manag. 256, 1910–1917. doi: 10.1016/j.foreco.2008.07.014
Oliveira, L. C., Hankerson, S. J., Dietz, J. M., and Raboy, B. E. (2010). Key tree species for the golden-headed lion tamarin and implications for shade-cocoa management in southern Bahia. Brazil. Anim Conserv 13, 60–70. doi: 10.1111/j.1469-1795.2009.00296.x
Oliver, T., Heard, M., Isaac, N., Roy, D., Procter, D., Eigenbrod, F., et al. (2015). Biodiversity and Resilience of Ecosystem Functions. Trends Ecol Evol. 30, 673–684. doi: 10.1016/j.tree.2015.08.009
Pan, Y., Birdsey, R., Fang, J., Houghton, R., Kauppi, P., Kurz, W., et al. (2011). A large and persistent carbon sink in the world’s forests. Science 333, 988–993. doi: 10.1126/science.1201609
Pardini, R., Bueno, A. A., Gardner, T., Prado, P., and Metzger, J. (2010). Beyond the fragmentation threshold hypothesis: regime shifts in biodiversity across fragmented landscapes. PLoS One 5:e13666. doi: 10.1371/journal.pone.0013666
Pearson, T., Walker, S., and Brown, S. (2005). Source book for LULUCF projects. Arlington, VA: Winrock International.
Peña-Claros, M., Poorter, L., Alarcon, A., Blate, G., Choque, U., Fredericksen, T. S., et al. (2012). Soil Effects on Forest Structure and Diversity in a Moist and a Dry Tropical Forest. Biotropica 44, 276–283. doi: 10.1111/j.1744-7429.2011.00813.x
Pessoa, M. S., Vleeschouwer, K. M., Amorim, A. M., and Talora, D. C. (2011). Calendário fenológico: uma ferramenta para auxiliar no cultivo de espécies arbóreas nativas da Floresta Atlântica no sul da Bahia. Ilhéus: Editus.
Pinheiro, J., Bates, D., DebRoy, S., and Sarkar, D. (2016). nlme: Linear and Nonlinear Mixed Effects Models. R package version 3.1-128.
Pinto, I. M., Emer, C., Cazetta, E., and Morante-Filho, J. C. (2021). Deforestation simplifies understory bird seed-dispersal networks in human modified landscapes. Front. Ecol. Evol. 9:640210. doi: 10.3389/fevo.2021.640210
Poulin, B., Wright, S. J., Lefebvre, G., and Calderón, O. (1999). Interspecific synchrony and asynchrony in the fruiting phenologies of congeneric bird-dispersed plants in Panama. J. Trop. Ecol. 15, 213–227. doi: 10.1017/S0266467499000760
Poulsen, J. R., Clark, C. J., and Palmer, T. M. (2013). Ecological erosion of an Afrotropical forest and potential consequences for tree recruitment and forest biomass. Biol. Conserv. 163, 122–130. doi: 10.1016/j.biocon.2013.03.021
Pütz, F. E., and Redford, K. H. (2010). The importance of defining ‘forest’: tropical forest degradation, deforestation, long-term phase shifts, and further transitions. Biotropica 42, 10–20. doi: 10.1111/j.1744-7429.2009.00567.x
Pütz, F. E., Groeneveld, J., Alves, L. F., and Metzger, J. P. (2011). Fragmentation drives tropical forest fragments to early successional states: A modelling study for Brazilian Atlantic forests. Ecol. Modell. 222, 1986–1997. doi: 10.1016/j.ecolmodel.2011.03.038
Pütz, S., Groeneveld, J., Henle, K., Knogge, C., Martensen, A., Metz, M., et al. (2014). Long-term carbon loss in fragmented Neotropical forests. Nat Commun. 5, 5037. doi: 10.1038/ncomms6037
R Development Core Team. (2017). R: a language and environment for statistical computing. Vienna: R Foundation for Statistical Computing.
Ribeiro, M. C., Metzger, J. P., Martensen, A. C., Ponzoni, F. J., and Hirota, M. M. (2009). The Brazilian Atlantic Forest: How much is left, and how is the remaining forest distributed? Implications for conservation. Biol. Conserv. 142, 1141–1153. doi: 10.1016/j.biocon.2009.02.021
Ribeiro, S. C., Jacovine, L. A. G., Soares, C. P. B., Martins, S. V., Nardelli, A. M. B., Lopes de Souza, A., et al. (2010). Quantificação de biomassa e estimativa de estoque de carbono em uma capoeira da zona da mata mineira. Rev. Árvore 34, 495–504. doi: 10.1590/S0100-67622010000300013
Rigueira, D. M. G., Molinari, A. L. M., Mariano, D. L. S., Reis, R. M., Portugal, A. B., Santana, N. S., et al. (2012). Influência da distância da borda e do adensamento foliar sobre a abundância de plantas pioneiras em um fragmento de floresta tropical submontana na Estação Ecológica de Wenceslau Guimarães (Bahia, Brasil). Acta. Bot. Bras. 26, 197–202. doi: 10.1590/S0102-33062012000100019
Rigueira, D. M. G., da Rocha, P. L. B., and Mariano-Neto, E. (2013). Forest cover, extinction thresholds and time lags in woody plants (Myrtaceae) in the Brazilian Atlantic Forest: resources for conservation. Biodivers. Conserv. 22, 3141–3163. doi: 10.1007/s10531-013-0575-4
Rocha-Santos, L., Pessoa, M. S., Cassano, C. R., Talora, D. C., Orihuela, R. L. L., Mariano-Neto, E., et al. (2016). The shrinkage of a forest: Landscape-scale deforestation leading tooverall changes in local forest structure. Biol. Conserv. 196, 1–9. doi: 10.1016/j.biocon.2016.01.028
Rueda, M., Moreno Saiz, J., Morales-Castilla, I., Albuquerque, F., Ferrero, M., and Rodríguez, M. (2015). Detecting fragmentation extinction thresholds for forest understory plant species in peninsular Spain. PLoS One 10:e0126424. doi: 10.1371/journal.pone.0126424
Santos, B. A., Peres, C. A., Oliveira, M. A., Grillo, A., Alves-Costa, C. P., and Tabarelli, M. (2008). Drastic erosion in functional attributes of tree assemblages in Atlantic Forest fragments of northeastern Brazil. Biol. Conserv. 141, 249–260. doi: 10.1016/j.biocon.2007.09.018
Scheffer, M., and Carpenter, S. R. (2003). Catastrophic regime shifts in ecosystems: linking theory to Observation. Trends Ecol Evol. 18, 648–656. doi: 10.1016/j.tree.2003.09.002
Silva, J., and Tabarelli, M. (2000). Tree species impoverishment and the future flora of the Atlantic forest of northeast Brazil. Nature 404, 72–74. doi: 10.1038/35003563
SOS Mata Atlântica and Instituto Nacional de Pesquisas Espaciais. (2008). Atlas dos remanescentes forestais da Mata Atlântica, period of 2000 at 2005. Available online at: http://www.sosmatatlantica.org.br/ (accessed August 8, 2010).
Souza, V. C., and Lorenzi, H. (2005). Botânica sistemática: guia ilustrado para identificação das famílias de Angiospermas da flora brasileira, baseado em APG II. São Paulo: Instituto Plantarum.
Sposito, T., and Santos, F. (2001). Scaling of stem and crown in eight Cecropia (Cecropiaceae) species of Brazil. Am J Bot. 88, 939–949. doi: 10.2307/2657047
Stephens, P. A., and Sutherland, W. J. (1999). What is the Allee effect? Oikos 87, 185–190. doi: 10.2307/3547011
Tabarelli, M., Mantovani, W., and Peres, C. A. (1999). Effects of habitat fragmentation on plant guild structure in the montane Atlantic forest of southeastern Brazil. Biol. Conserv. 91, 119–127. doi: 10.1016/S0006-3207(99)00085-3
Tabarelli, M., and Peres, C. A. (2002). Abiotic and vertebrate seed dispersal in the Brazilian Atlantic forest: implications for forest regenaration. Biol. Conserv. 106, 165–176. doi: 10.1016/S0006-3207(01)00243-9
Tabarelli, M., da Silva, J. M. C., and Gascon, C. (2004). Forest fragmentation, synergisms and the impoverishment of neotropical forests. Biodivers. Conserv. 13, 1419–1425. doi: 10.1023/B:BIOC.0000019398.36045.1b
Tabarelli, M., Lopes, A. V., and Peres, C. A. (2008). Edge-effects drive Tropical Forest fragments towards an early-successional system. Biotropica 40, 657–661. doi: 10.1111/j.1744-7429.2008.00454.x
Tabarelli, M., Peres, C. A., and Melo, F. P. L. (2012). The ‘few winners and many losers’ paradigm revisited: Emerging prospects for tropical forest biodiversity. Biol. Conserv. 155, 136–140. doi: 10.1016/j.biocon.2012.06.020
West, G., Enquist, B., and Brown, J. (2009). A general quantitative theory of forest structure and dynamics. Proc Natl Acad Sci U S A 106, 7040–7045.
Keywords: homogenization, habitat amount, regime shift, tree community, payment for environmental services, ecological process
Citation: Rigueira DMG and Mariano-Neto E (2023) Structural changes and carbon reduction due to habitat loss in Atlantic Forest. Front. For. Glob. Change 6:1041448. doi: 10.3389/ffgc.2023.1041448
Received: 10 September 2022; Accepted: 14 April 2023;
Published: 05 May 2023.
Edited by:
Rita De Cassia Quitete Portela, Universidade Federal do Rio de Janeiro, BrazilReviewed by:
Camila Barros, Federal University of Rio de Janeiro, BrazilPedro Paulo Da Silva Ferreira, Federal University of Rio de Janeiro, Brazil
Copyright © 2023 Rigueira and Mariano-Neto. This is an open-access article distributed under the terms of the Creative Commons Attribution License (CC BY). The use, distribution or reproduction in other forums is permitted, provided the original author(s) and the copyright owner(s) are credited and that the original publication in this journal is cited, in accordance with accepted academic practice. No use, distribution or reproduction is permitted which does not comply with these terms.
*Correspondence: Dary Moreira Gonçalves Rigueira, ZGFyeS5yaWd1ZWlyYUBpbmVtYS5iYS5nb3YuYnI=