- 1Faculté d’Agronomie, Université de Parakou, Parakou, Benin
- 2Department of Plant and Soil Sciences, University of Pretoria, Pretoria, South Africa
- 3Laboratory of Applied Ecology, University of Abomey-Calavi, Cotonou, Benin
Introduction: Managing the degradation and loss of Sudanian woodlands to ensure sustainable use for mainly firewood, poles, and timber is receiving increasing attention, and it requires tailored strategies to improve stand productivity. This study determined: (i) tree response (density, recruitment, and height growth) to selective stem thinning and branch pruning; and (ii) tree species that are most affected by selective stem thinning and branch pruning in the Sudanian woodland of Benin.
Methods: Three homogeneous vegetation units of 80 m × 80 m each were identified, representing the three stages of woodland development (WDS): early and young WDS of 3 and 3–5 years old, respectively, and the advanced to mature secondary WDS of 6–9 years old. Three random blocks of 20 m × 20 m each, subdivided into four 10 m × 10 m treatment plots, were delineated per vegetation unit. Each of these plots was randomly assigned a treatment (T): T1—no thinning and no pruning; T2—30% thinning; T3—60% thinning; T4—100% thinning. Branch pruning was applied to all remaining stems in T2 and T3. Tree species, stem abundance and height of individuals = 1 m were recorded every 6 months in 2015 and 2016.
Results and discussion: Thinning intensity significantly influence tree density both in WDS 2 and 3 with the 30% thinning showing the best tree density (3355.56 ± 250.19 stems/ha and 3255.56 ± 772.68 stems/ha, respectively). However, more stem recruitment was observed without thinning and pruning (883.33 ± 212.13 stems/ha), and 30% thinning and pruning (383.33 ± 164.99 stems/ha). There was a significant increase in tree height growth both in WDS 2 and 3 with an increasing thinning intensity. The two most affected tree species were Terminalia avicennioides and Pteleopsis suberosa with an average growth in height after 6 months of 105.5 ± 1.05 mm (for 30% thinning and pruning in stage 3), and 61.75 ± 0.67 mm (for 60% thinning and pruning in stage 3), respectively. Overall, moderate stem thinning (30%) at WDS 1 and more severe thinning at WDS 2 and 3 resulted in the best tree height growth and recruitment.
Introduction
The importance of non-timber forest products (NTFPs) to the livelihoods of rural communities in developing countries has been widely reported over the past decade (Shackleton et al., 2015). These NTFPs, including food, medicines, fuelwood, fiber, and some plant and fungal products, play an important role in livelihood security and poverty alleviation of rural communities in many tropical countries (Bruschi et al., 2014). In the Sudanian zone, NTFPs make up 39% of the yearly income (Heubach et al., 2011), and the major contributors are native woody tree species (Kabré et al., 2020). A recent study suggested that NTFPs harvesting can also contribute to biodiversity conservation (Tonga Ketchatang et al., 2017). Despite the importance of NTFPs in rural development and resource management, their unsustainable harvesting could cause economic and ecological challenges and compromise forest conservation (Stanley et al., 2012; Hernández-Barrios et al., 2015).
In accordance with the demand-offer and resource-advantage theories, the scarcity of the resource was identified as a key factor that influences the value assigned to the resource by local populations (Bell et al., 2012). The relationship between demand and supply underlies the forces behind the allocation of resources. Increased resource consumption especially harvested from natural stands can cause resource scarcities, and scarcities impose costs on natural resources (Ghermandi et al., 2010). In turn, the cost on natural resources determines the pressure on the resource. Woodland as a natural resource is a major component of biodiversity and ecosystem services, that provides economic services (by supporting the livelihoods of local communities) as well as ecological services such as maintaining soil quality, regulating water flow systems, sequestering carbon, and regulating microclimate (Kalema et al., 2015). Previous studies (Ouedraogo et al., 2010; Syampungani et al., 2016) showed that woodlands are under tremendous pressure in connection with the increasing demand for fuelwood and charcoal production. This pressure leads to the reduction of soil fertility, lower agricultural productivity ultimately causing food insecurity (Lawali et al., 2018). Fuelwood scarcity is both the cause and consequence of deforestation and forest degradation in the majority of developing countries (Tadele et al., 2020). Sustainable management of woodlands requires a balance between growth potential and removal rates (Manyanda et al., 2020). In a situation where removals exceed growth rates, sustainability is not assured (Manyanda et al., 2020). Furthermore, selective logging remains a widespread practice in most tropical forests, but the long-term effects of wood harvest on tree recruitment among hundreds of tree species are difficult to predict (Hogan et al., 2018). This uncertainty could potentially exacerbate threats to harvested tree species. In response to this situation, management of natural resources particularly woody species is of paramount importance. To ensure sustainable use, it is therefore necessary to develop management strategies to improve productivity and maintain the balance between growth rate and harvesting.
In the Sudanian zone, woodland management includes total protection and controlled use mainly for livestock and wildlife feeding. Woodland in this context is defined as the type of land cover characterized by trees and shrubs with a canopy of 5–10% of trees capable of reaching a height of 5 m at maturity and a canopy of more than 10% of trees not capable of reaching a height of 5 m at maturity, and a lower diversity of species (Gonzalez, 2005). The management strategies of the Sudanian woodland included keeping logged plots free from fire and livestock grazing for 3–5 years. Although an increase in biomass was observed in such a case, early fire following the 3-year protection from grazing has shown a detrimental effect on ligneous regeneration due to the accumulation of grass biomass that favors intense fires (Manaute, 1996). On the other hand, tree diversity and subsequently standing biomass were substantially improved in Sudanian forest fallow systems after 5 years (Assédé et al., 2012). However, in old fallows (>8 years), the woodland system is characterized by a depletion in tree species due to competition for soil nutrients and light (Assédé et al., 2012). Yet, concerns around the perceived degradation and loss of Sudanian woodlands in terms of tree species, wood production, and use value for diverse user needs, could be managed through selective stem thinning and branch pruning. As recently demonstrated in an experiment in Miombo and Sudanian woodland (Geldenhuys et al., 2018; Assèdé et al., 2021), selective thinning and pruning of woodland in different development stages could maintain and improve biomass production. The question is how long, and how different is tree species response to selective and thinning and pruning in terms of density, recruitment and height growth.
This study aimed at determining: (i) tree response (density, recruitment and height growth) to selective stem thinning and branch pruning; and (ii) tree species that are most affected by selective stem thinning and branch pruning in the Sudanian woodland of Benin. We predicted that: (1) tree response (density, recruitment and height growth) to selective stem thinning and branch pruning is species specific; (2) tree species response (density, recruitment, and height growth) is positively correlated with the intensity of selective stem thinning and branch pruning; (3) woodland development stage could significantly affect tree response (density, recruitment and height growth) to selective stem thinning and branch pruning thinning.
Materials and methods
Study area
The study was conducted in 2015 and 2016 around the Biosphere Reserve of Pendjari (BRP) located in the Sudanian woodlands area of Benin (10°30′ to 11°30′N, 0°50′ to 2°00′E). The reserve is comprised of a strictly protected core area and two adjacent hunting zones surrounded by a buffer zone (Assèdé et al., 2021). The experimentation took place in the controlled occupied area in the buffer zone, a Human influence zone of about 30510 km2 (Figure 1). This area is subject to anthropogenic activities with a vegetation composed of the different woodland development stages (Assédé et al., 2012). The BRP is characterized by one rainy season (April/May–October) and one dry season (November–March/April). The total annual rainfall averages 1000 mm with 60% falling in summer between July and September. The mean annual daily temperature is 27°C. The vegetation composed of more than 802 plant species is a mosaic of grassland, savanna and woodland, as well as gallery forest alongside water courses (Assédé et al., 2012). Livelihood activities of the local population around the reserve are dominated by crop cultivation and livestock farming. Cultivated crops include maize, yam, rice, and cotton (Djibril, 2002). The vegetation in the buffer zone of the reserve (a mixture of woodlands at different stages of development and fallow systems) is also used for intensive collection of timber, poles and fuel wood (the main source of energy for the local population), as well as livestock pasture.
Experimental design and data collection
The experimental design followed Assèdé et al. (2021) and consisted of three vegetation units of about 80 m × 80 m each representing the three main woodland development stages in the study area (Assédé et al., 2012): (i) Development stage 1: early woodland development stage of less than 3 years with stems <2 m tall; (ii) Development stage 2: young woodland development stage of 3–5 years old with stems 2–4 m tall; (iii) Development stage 3: advanced to mature secondary woodland of 6–9 years old with stems >4 m tall. Each vegetation unit was characterized by a relatively uniform floristic composition and stand structure (Geldenhuys et al., 2013; Assèdé et al., 2021). In each of these homogeneous vegetation units, three random blocks (repetition) of 20 m × 20 m each were demarcated, and each block was further subdivided into four treatment (T1 to T4) plots of 10 m × 10 m each (Figure 2). Each treatment plot had a buffer zone of 5 m wide and was randomly allocated to a treatment. Plots were not fenced, but under strict control without anthropogenic disturbance from the collaboration agreement with local people.
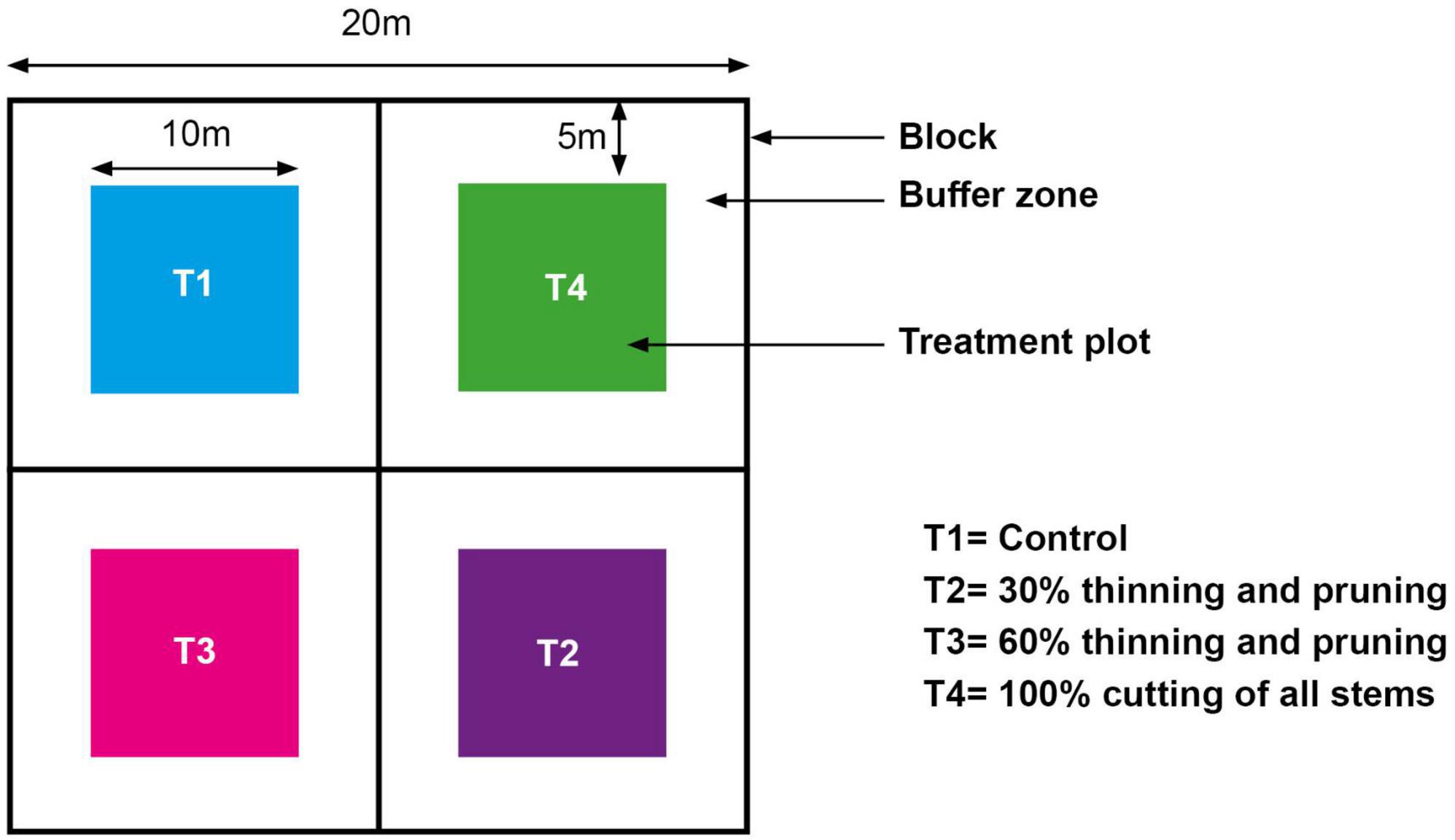
Figure 2. Experimental design and layout of treatment plots (Assèdé et al., 2021).
The treatments consisted of selective stem thinning and branch pruning of:
– T1: Control: No thinning and no pruning.
– T2: 30% thinning. The number of stems to be removed was 30% of the total stems ≥1 m height recorded.
– T3: 60% thinning: The number of stems to be removed was 60% of the total stems ≥1 m height recorded.
– T4: 100% thinning: Cutting of all recorded stems ≥1 m height.
The remaining stems in T2 and T3 were pruned with a suitable pruning saw, to improve the stem form. Only the poorest parts of forked stems and branches up to 50% of the stem height were removed. The branches were cut as close as possible to the main stem to ensure rapid recovery of the wound.
The height of all stems ≥1 m height was measured every 6 months from June 2015 through December 2016, thus at three time periods during the experimentation. At each measurement occasion, species richness, stem abundance and tree height were collected.
Data analysis
In each woodland development stage, stems were organized per species, per treatment, and per period of data collection. Similarly, tree density was calculated per treatment, period of data collection, and woodland development stage.
The analysis of variance (ANOVA) was performed using R 4.1 (R Core Team, 2022) software to test the effect of selective thinning and branch pruning, and measurement period on tree density. Density was calculated using the following formula:
Where n is the number of stems ≥1 m height per treatment or period.
ANOVA and a t-test were performed to assess the effects of selective thinning and branch pruning on tree recruitment. The tree recruitment is the difference in number of stems ≥1 m height per hectare between two periods of data collection.
A linear mixed effects model (Pinheiro and Bates, 2000) on repeated measures was used to test the influence of thinning intensity and period of data collection on tree height growth. In this model, woodland development stage, treatment (thinning intensity), and their interactions were included as fixed effects (Wang et al., 2017). A linear model (LM) m0, and three linear mixed effect models (LMM) m1 to m3 with different random components, were fitted with the nlme package of R (R Core Team, 2022) and compared to identify the most appropriate model. A logarithmic transformation was performed to improve the normality within the homoscedastic residuals.
The specifications of the models that were compared to identify the optimal random model structure were as follows:
– m0: linear model with no random effect (null model),
– m1: linear mixed-effects model with the period of measurement nested into the block as a random factor,
– m2: linear mixed effects model with the block as a random factor,
– m3: linear mixed effects model with the measurement period as a random factor.
The optimal model showing the lowest Akaike Information Criterion (AIC) was m3, a mixed-effects model with only the period of measurement as a random variable (Table 1). This optimal random model was then used to evaluate the effect of explanatory variables (i.e., woodland development stage and treatment) on tree height growth. Linear mixed effects (LMM) models (m3.1 to m3.4) were further fitted by including different combinations of explanatory variables and compared in R-4.1 (R Core Team, 2022). The specifications of the models derived from the selected m3 optimal random model were as follows:
– m3.1: The inclusion of Treatment, Stand Development Stage, and their interaction as explanatory variables,
– m3.2: The inclusion of Treatment and Stand Development Stage as explanatory variables, without interaction,
– m3.3: The inclusion of Treatment as the only explanatory variable,
– m3.4: The inclusion of stand development stage as the only explanatory variable.
Models’ comparison used the built-in ANOVA function to assess whether the potential models were significantly different based on the test probability “p.” When p ≤ 5%, the most complex model was selected, whereas for p > 5%, the simplest model was preferred. The criteria for model selection were also based on the AIC. The smaller their value, the better the fit (Zuur et al., 2009). The selected model was represented by the complex model m3.1 (Table 1).
Statistical analyses of the continuous variables were performed using the lme function in the nlme package of the software R-4.1. ANOVA of the linear mixed effect model was performed followed by the post-hoc test using the lsmeans function to identify the groups of models that are significantly different. The ggplot function of the ggplot2 package was used to produce the graph.
Tree height growth was calculated between two consecutive periods of data collection. An average stems’ growth per species and treatment was calculated to determine the most affected tree species by selective thinning and branch pruning for each woodland development stage. Only tree species with the three measures were considered.
Considering the short period of the experimentation, tree height growth for treatment 4 (100% clearing) was not significant to be considered in the analysis to assess treatment effect on tree growth in height.
Results
Influence of thinning intensity and branch pruning on tree density and recruitment
The Figure 3 showed the effect of thinning intensity along the three periods of measurement on the average tree density of stems ≥1 m height of the woodland development stages. Results showed no significant effect of thinning intensity and branch pruning on tree density in the woodland development stage 1 (F = 4.47; p = 0.096) (Table 2). However, a significantly higher tree density (F = 20.88; p = 7.64 × 10–3) (Table 2) was observed during the second (1977.78 ± 269.43 stems/ha) and third (2311.11 ± 167.77 stems/ha) period of measurement (Figures 3A, B).
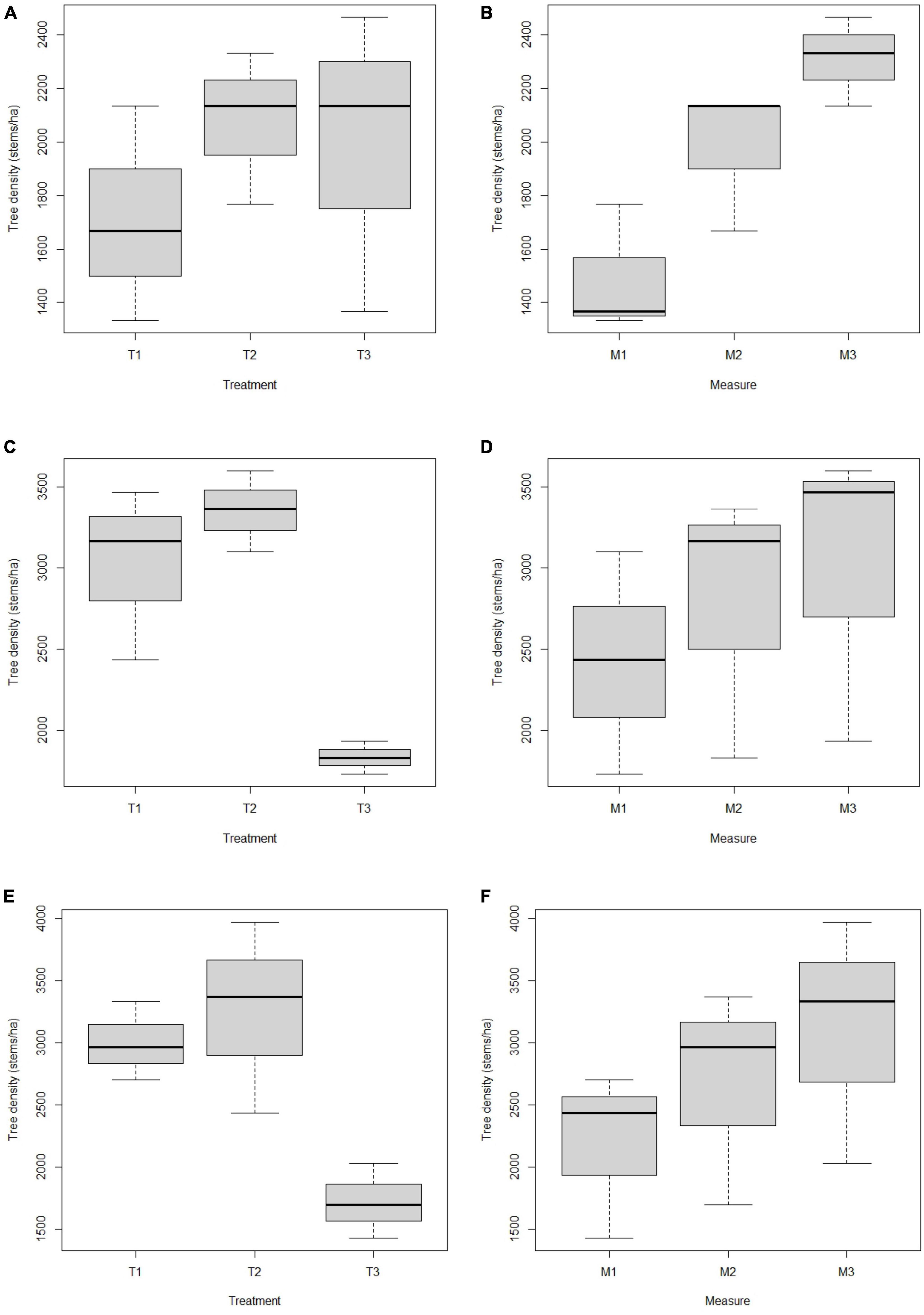
Figure 3. Boxplot showing relation between tree density (stems/ha), treatment and period of measurement of tree species with stems ≥1 m height in the woodland development stages. (A,B) Stage 1; (C,D) stage 2; (E,F) stage 3; T1: treatment 1 (no thinning and pruning); T2: treatment 2 (30% thinning and pruning); T3: treatment 3 (60% thinning and pruning); M: period of measurement of tree species. M1: first measurement; M2: second measurement; M3: third measurement.
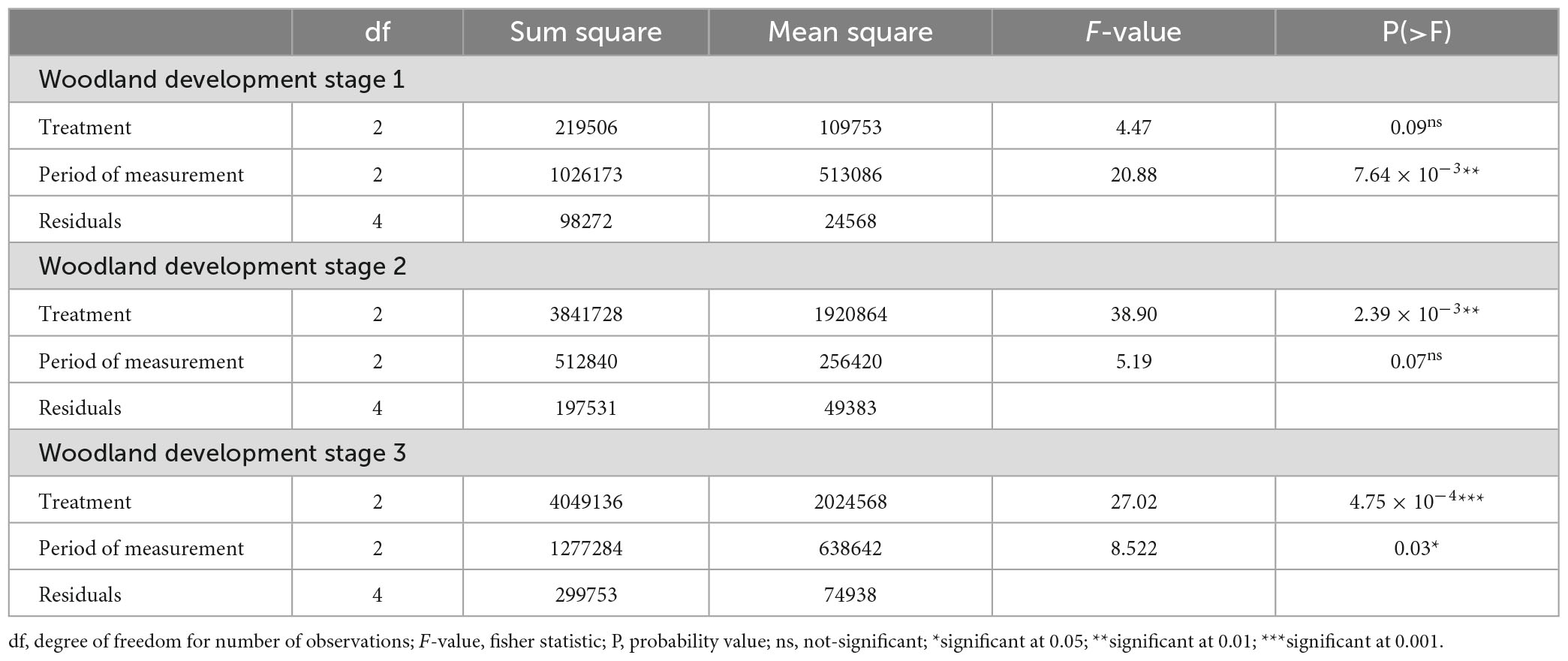
Table 2. ANOVA to compare tree density according to the treatments (T1 - no thinning and no pruning; T2 - 30% thinning; T3 - 60% thinning) and the period of measurement.
In the woodland development stage 2, thinning intensity significantly influenced trees density (F = 38.9; P = 2.39 × 10–3) (Table 2). The 30% thinning and branch pruning presented the best tree density (3355.56 ± 250.19 stems/ha) (Figure 3C). However, no significant difference of tree density was shown between the three periods of measurement (F = 5.19; p = 0.077) (Figure 3D and Table 2).
The thinning intensity (F = 27.02; P = 4.75 × 10–4) as well as the period of measurement (F = 8.52; P = 0.036) significantly influenced tree density in the woodland development stage 3 (Table 2). The 30% thinning and branch pruning presented the best tree density (3255.56 ± 772.68 stems/ha) (Figure 3E). The results showed that tree density was the highest during the third period of measurement (3111.11 ± 985.64 stems/ha) (Figure 3F).
While there was a significant decrease in tree recruitment (F = 10.92; P = 0.04) with an increasing thinning intensity in stage 2, no effect was observed in both stage 1 (F = 1.96; P = 0.28) and stage 3 (F = 4.14; P = 0.14) of the woodland development stages (Appendix 1). Tree recruitment is higher with no thinning and pruning (T1: 883.33 ± 212.13 stems/ha) and 30% thinning and pruning (T2: 383.33 ± 164.99 stems/ha) than 60% thinning and pruning (Figure 4 and Appendix 1).
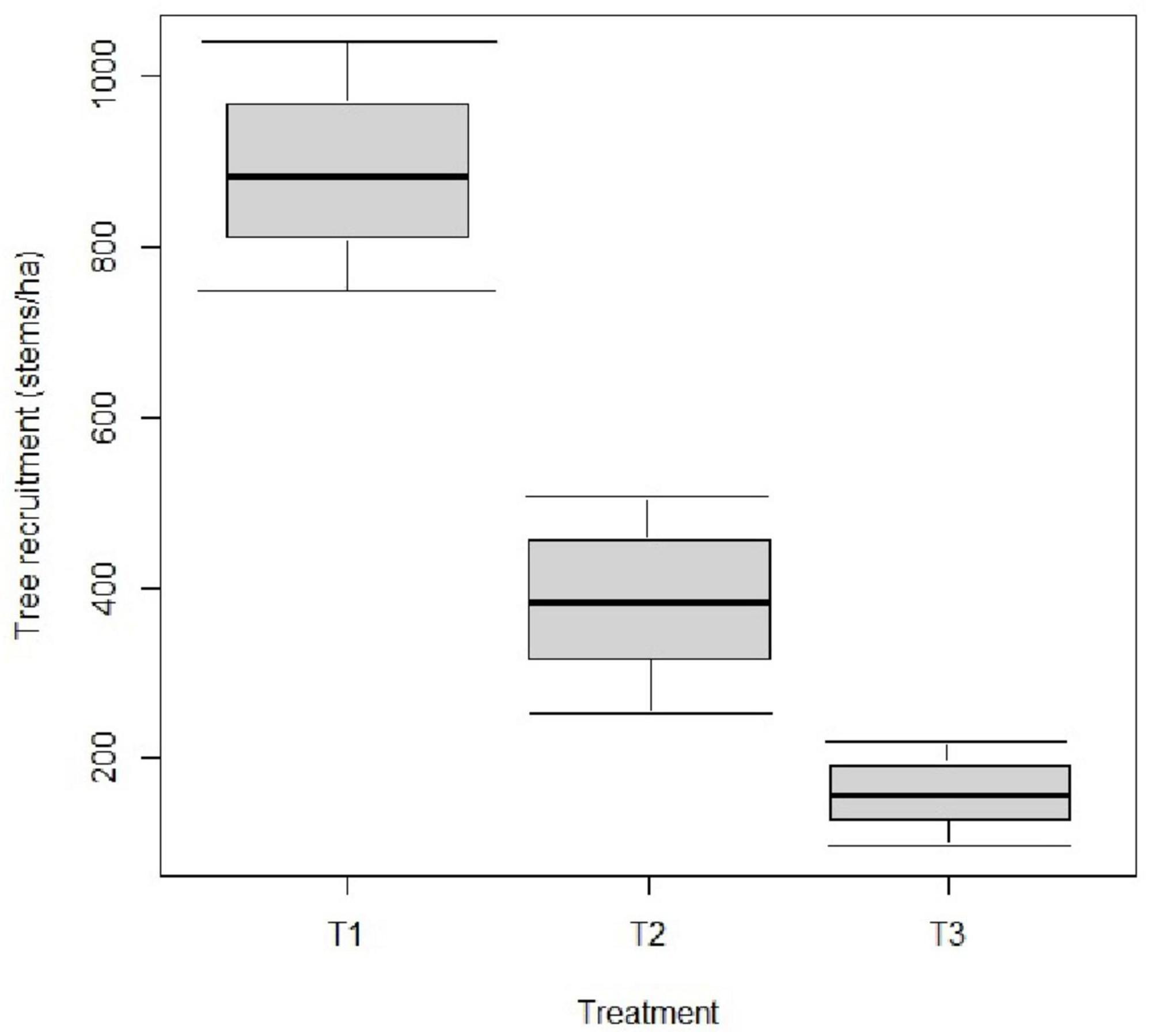
Figure 4. Boxplot showing effect of thinning intensity on tree recruitment (stems/ha). T1: treatment 1 (no thinning and no pruning); T2: treatment 2 (30% thinning and pruning); T3: treatment 3 (60% thinning and pruning).
Influence of thinning intensity and branch pruning, and woodland development stage on tree height growth
Tree height growth was significantly influenced by thinning intensity (F = 6.46; p = 0.0016), woodland development stage (F = 228.82; p < 0.0001), as well as the interaction between thinning intensity and woodland development stage (F = 3.21; P = 0.0124) (Table 3).
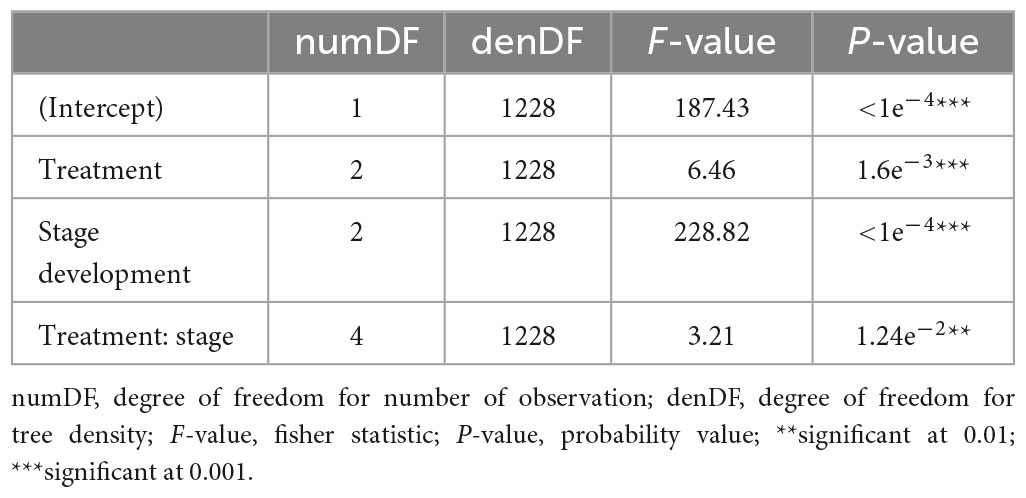
Table 3. ANOVA to compare the mixed-effect and fixed-effect candidate models for tree growth in height.
Table 4 presents the results of the post-hoc analysis of average woodland tree growth in height as a function of the thinning intensity. Groups with the same letters indicate no significant difference in tree growth in height inside the groups. Despite the absence of significant differences in average tree growth in height between thinning intensities (T1: no thinning and pruning, T2: 30% thinning and pruning and T3: 60% thinning and pruning) in woodland development stage 1 (Table 4), the results from pairwise analysis indicated the highest value was with treatment 2 (Figure 5). However, in woodland development stage 2, there was a significant increase in tree height growth with an increasing thinning intensity, with the highest value recorded for treatment 3. Similarly, in stage 3, treatment 3 presented significantly higher tree height growth compared to treatment 1 and 2 (Figure 5 and Table 4).
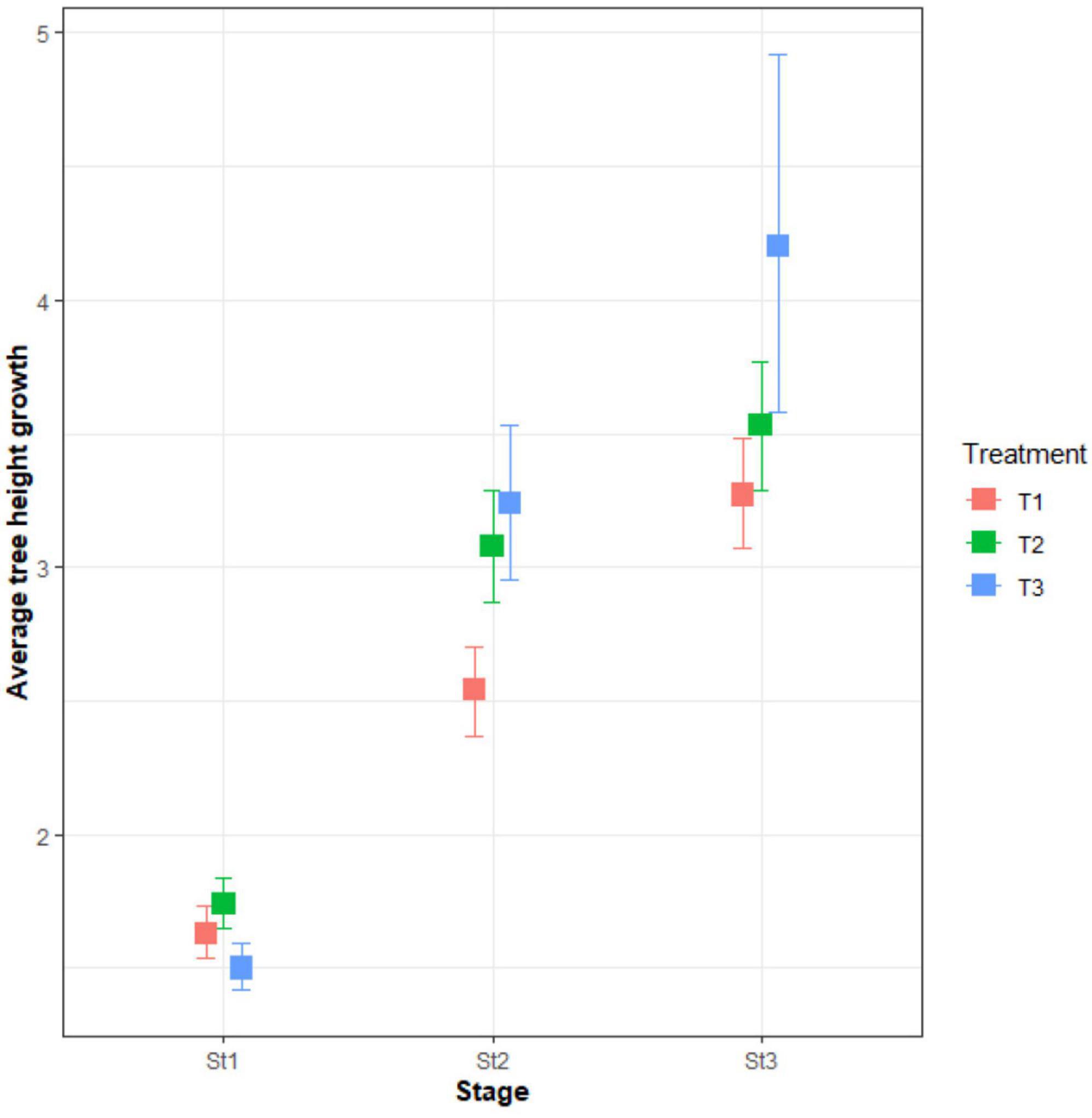
Figure 5. Boxplot of the relation between tree growth in height (mm), treatment and woodland development stages. St1: woodland development stage 1; St2: woodland development stage 2; St3: woodland development stage 3; T1: treatment 1 (no thinning and pruning); T2: treatment 2 (30% thinning and pruning); T3: treatment 3 (60% thinning and pruning).
Most affected tree species by thinning intensity and branch pruning in woodland development stages
Tree species are variably affected by thinning intensity and branch pruning along woodland development stages (Table 5).
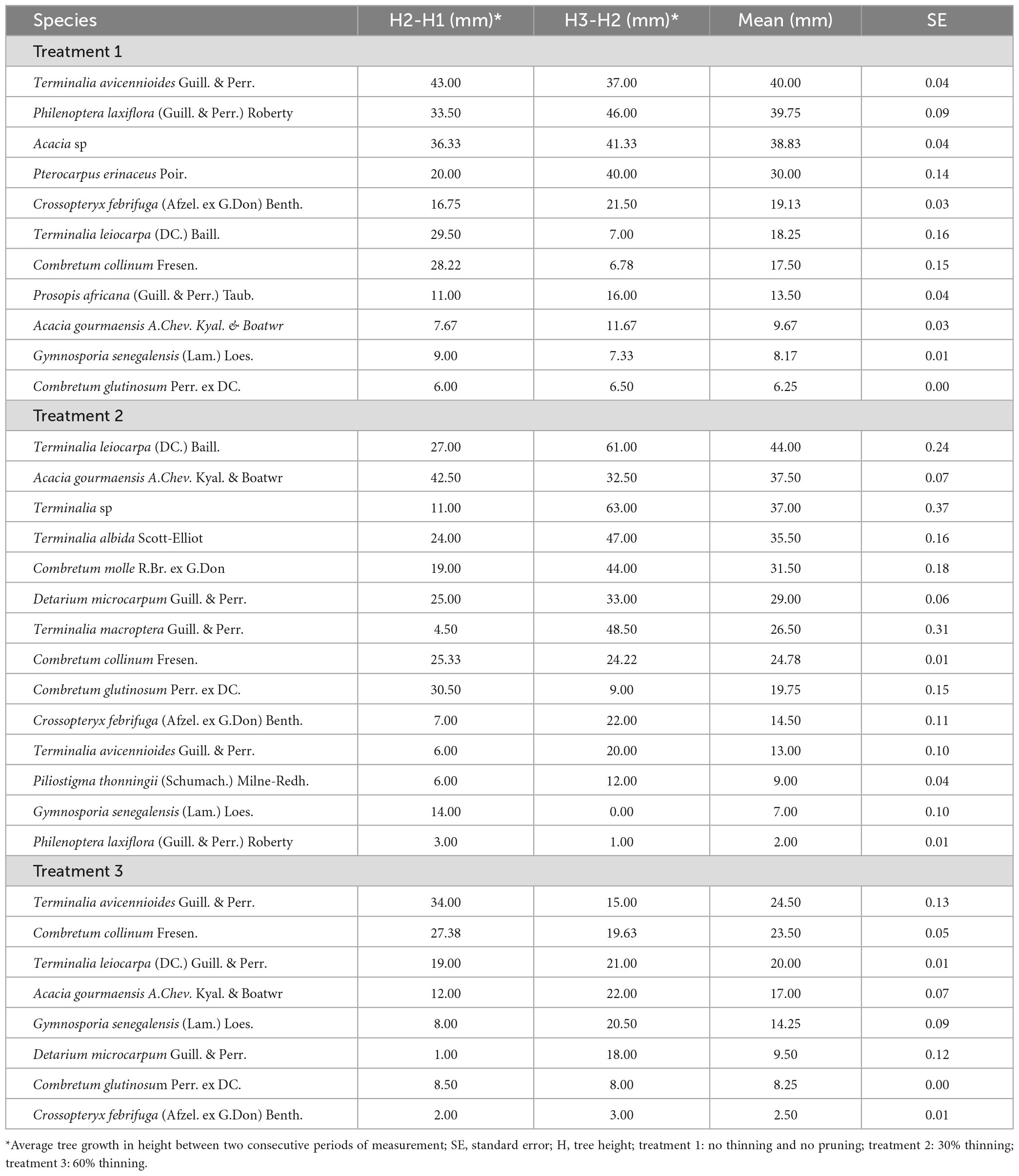
Table 5. Average tree growth in height in woodland development stage 1 by thinning intensities and branch pruning after 6 months.
– Stage 1
The best tree growth in height in woodland development stage 1 was observed with Terminalia leiocarpa (DC.) Baill. and T. avicennioides Guill. & Perr. The average growth in height for the two tree species after 6 months was, respectively 44 ± 0.24 mm (with 30% thinning and pruning) and 40 ± 0.04 mm (in the absence of thinning and pruning). However, in the absence of thinning and pruning (treatment 1) Philenoptera laxiflora (Guill. & Perr.) Roberty (39.7 ± 0.09 mm) and Acacia sp (38.8 ± 0.04 mm) presented the best average growth in height after T. avicennioides. After 30% thinning (treatment 2), Acacia gourmaensis (37.5 ± 0.07 mm) and Terminalia sp (37.8 ± 0.37 mm) presented the best average growth in height after T. leiocarpa. Average tree growth in height was globally low with 60% thinning. The three species with the best growth were T. avicennioides (24.5 ± 0.1 mm), Combretum collinum (23.5 ± 0.05 mm) and T. leiocarpa (20 ± 0.01 mm).
– Stage 2
The best tree growth in height in woodland development stage 2 was observed with Acacia sp and Daniellia oliveri (Rolfe) Hutch. & Dalziel (Table 6). The average stem growth in height for the two tree species after 6 months of experimentation was, respectively 44 ± 0.24 mm, observed with 60% thinning (treatment 3) and 57.38 ± 0.04 mm observed with no thinning (treatment 1). In the absence of thinning (treatment 1), the best tree growth in height in woodland development stage 2 was observed with Combretum collinum (47.5 ± 0.12 mm) and Pteleopsis suberosa Engl. & Diels (34.12 ± 0.23 mm) after D. oliveri (57.38 ± 0.13 mm). The average tree growth in height was globally low after 30% thinning (treatment 2). Crossopteryx febrifuga (Afzel. ex G.Don) Benth. (28.75 ± 0.20 mm), Gymnosporia senegalensis (Lam.) Loes. (28.19 ± 0.07 mm) and P. suberosa (27.88 ± 0.16 mm) expressed the best average growth in height. The average tree growth in height after 60% thinning was globally high. Tree species with the best growth were D. oliveri (43.55 ± 0.53 mm), C. collinum (43 ± 0.24 mm) after Acacia sp. (61.5 ± 0.59 mm).
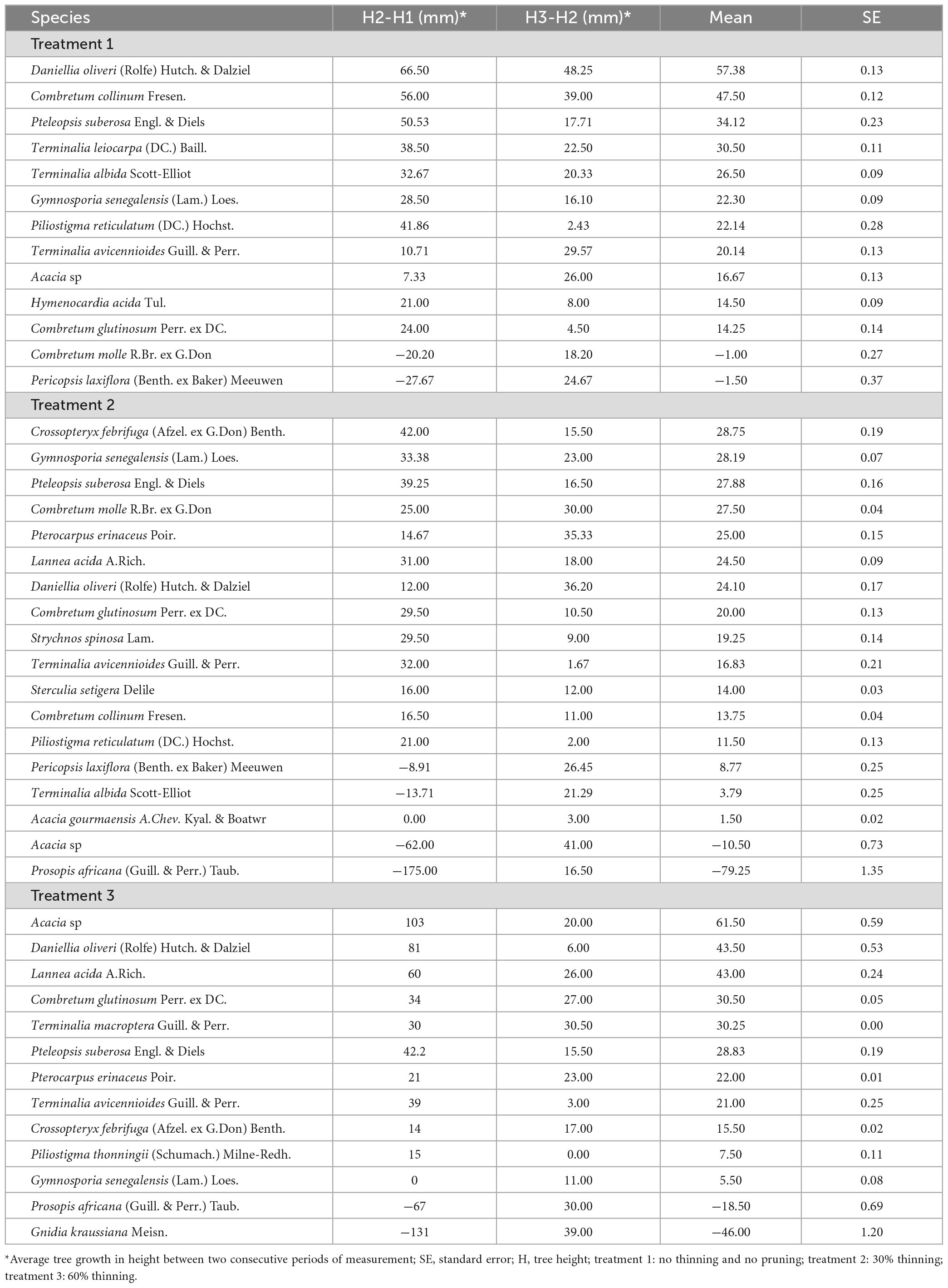
Table 6. Average tree growth in height in woodland development stage 2 by thinning intensities and branch pruning after each 6 months.
– Stage 3
The best tree growth in height in woodland development stage 3 was recorded with Terminalia avicennioides and Pteleopsis suberosa (Table 7). The average growth in height for the two tree species after 6 months was, respectively 105.5 ± 1.05 mm observed with 30% thinning and pruning (treatment 2) and 61.75 ± 0.67 mm with 60% thinning and pruning (treatment 3). In the absence of thinning (treatment 1), the best tree growth in height in woodland development stage 3 after 6 months was obtained with Combretum collinum (51 ± 0.13 mm), Pericopsis laxiflora (Benth. ex Baker) Meeuwen (49.25 ± 0.46 mm) and Terminalia albida (45.37 ± 0.16 mm). The average tree growth in height after 30 % thinning (treatment 2) was globally high. Daniellia oliveri (59.19 ± 0.59 mm), Gymnosporia senegalensis (49.5 ± 0.41 mm) expressed the best average growth in height after Terminalia avicennioides (105.5 ± 1.05 mm). After 60% thinning (treatment 3), tree species with the best growth were Pteleopsis suberosa (61.75 ± 0.67 mm), Daniellia oliveri (61 ± 0.47 mm) and Terminalia albida (59.50 ± 0.33 mm) presented the best growth in height.
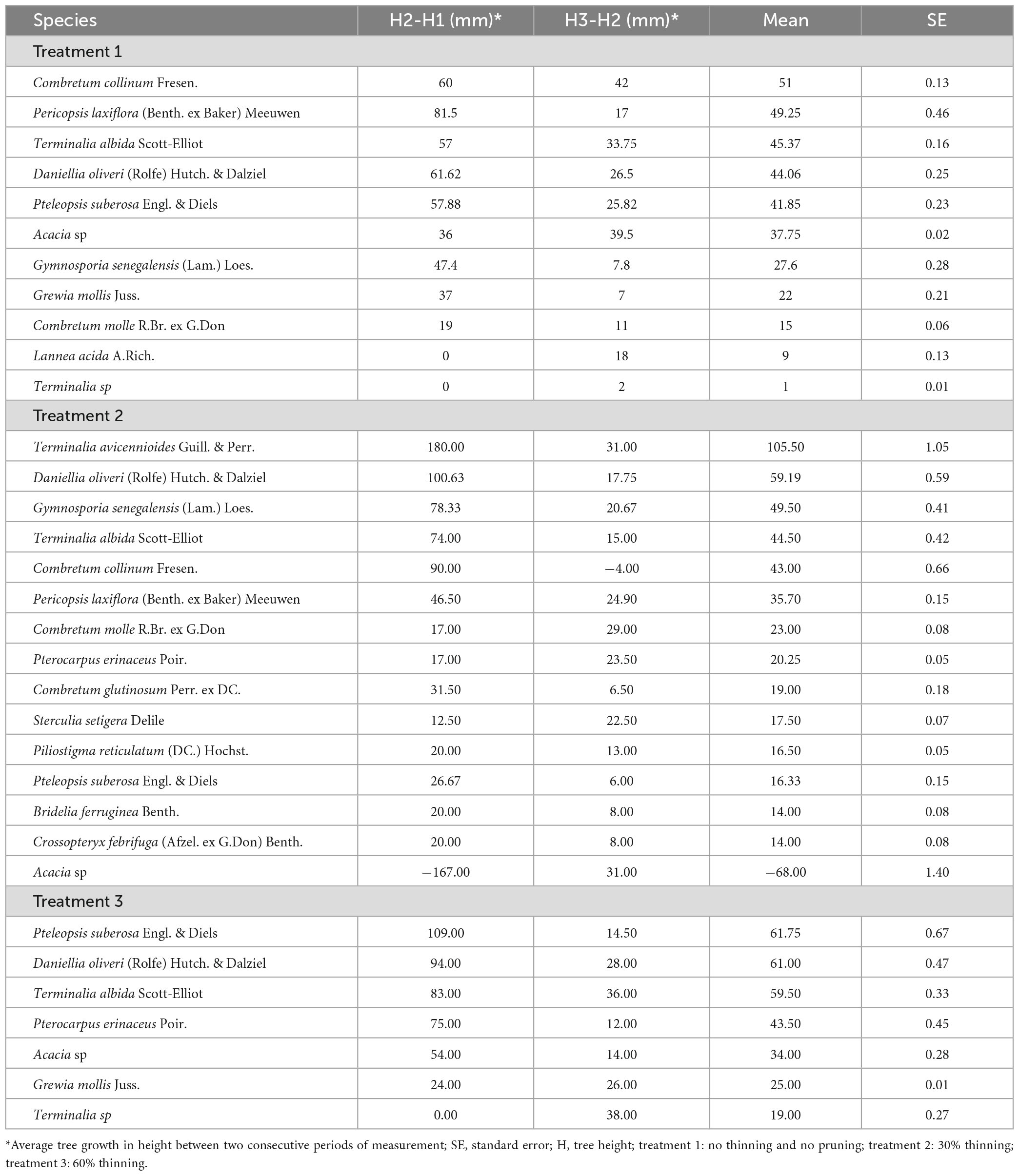
Table 7. Average tree growth in height in woodland development stage 1 along thinning and pruning intensities after 6 months.
Discussion
Influence of thinning and pruning intensity on tree density, height growth, and recruitment
In contrast to the woodland development stage 2 and 3, no thinning intensity and branch pruning effect was observed on tree density in stage 1. The question of what determines the distribution and abundance of woody plants in Sudanian woodlands has been widely investigated in plant ecology (Burrows et al., 1985; Archer, 1995). The opening of the canopy at stage 2 and 3 would have a significant impact on tree recruitment especially the 2 m > height ≥ 1 m. Tree density is driven by the process of stem recruitment (Comita et al., 2010). In the same way, the recruitment of new tree stems plays a key role in determining tree density in forest ecosystems and this process can be affected by a range of factors, including soil conditions, disturbance events, and competition with other plants (Garcia-Barrios et al., 2009; Valencia et al., 2014). After selective thinning and pruning, there would be the redistribution of growth resources in the soil allowing a better tree growth (both in height and diameter) (Assédé et al., 2015). This also results in increased tree recruitment, which translates into increased density. In stage 2 and 3, the 30% thinning and pruning significantly influenced the density of stems ≥1 m in height suggesting a better tree response with this treatment in these two woodland development stages. However, the observed tree density through the experimentation period would be predetermined by the initial tree density of each studied plot, which differ between plots of a woodland stage. During the experimentation, several stems were damaged by uncontrolled factors including the wind, rainfall, insects and small mammals directly, which may have affected tree density of the different plots. In order to improve accuracy, future studies should include all these factors. Whatever the disturbance on a vegetation unit, plant response will always reach an equilibrium depending on the intensity of the disturbance. The decrease in the tree density after a year during the third measurement could be attributed to the establishment of a new equilibrium between plant species. In general, tree density is driven by factors including soil characteristics and disturbances such as fire, browsing, tree harvesting and natural tree death (Comita et al., 2010), and how these factors affect tree density may differ with species and vegetation unit. In this context, tree recruitment may be a better variable to explain the effect of thinning and pruning intensity on woodland development stage as it refers to the increase in the number of stems ≥1 m height between two periods of data collection. A significantly higher stem recruitment at stage 2 with 30% thinning was not surprising because of an existing potential of stems ≤1 m height for the recruitment under this management regime. Several studies discussed the relationship between canopy openness, light penetration, and tree recruitment in forests (Ding and Zang, 2021; Barreto et al., 2022; Mahayani et al., 2022). They provided evidence that increase canopy openness can lead to greater light penetration and better recruitment of trees in forests. However, this may depend on the existing potential for recruitment, the vegetation structure and composition (de Avila et al., 2015, 2017; Barreto et al., 2022) explaining the decrease in stem recruitment at 60% thinning. In stage 1, the canopy cover is not as dense as in stage 2, while in stage 3 there would be few stems <1 m height with the close canopy. Therefore, in these two contexts, there is not a significant difference in the average tree recruitment regardless of the treatment regime. Tree recruitment linked to factors including height growth (Fischer et al., 2021). In the advanced to mature secondary forest, thinning at 60% results in better tree height growth. Both Hall (2011) and Yu et al. (2022) agreed that exposure of the tree crown to full sunlight through tree harvesting is critical for recruitment of remaining tree individuals in the forest understory (Yu et al., 2022). Clearance treatments and targeted gap openings provide and enhanced light environment for regeneration and recruitment (Hall, 2011), thus favor tree growth.
A general increase in tree growth for height is observed with thinning intensity and analyses revealed significant differences among treatments and woodland developmental stages. At high thinning intensity (60% thinning), tree density in the stand may decrease, allowing a better light penetration. However, because stem size is inherently included in thinning treatments, there is already variation in the absolute size of the remaining trees (Saarinen et al., 2020). With the increasing intensity of thinning, many trees were removed, which benefited the remaining trees for available resources, allowing them to secure their height growth (Saarinen et al., 2020). So, the increase in height growth with thinning intensity can be explained by the increase in light intensity in the stand after thinning for the benefit of the remaining tree community (Valinger et al., 2019). Studies developed by Akouehou et al. (2018) indicated that the basal area of tree species in the logged or thinned stand increased significantly compared to the control stand during the first 4 years following the silvicultural treatment (thinning or logging). Without a clear information on the intensity of thinning, the results of Akouehou et al. (2018) are consistent with the results found in our study. However, the absolute size and diameter of the trees were not considered in our study. In contrast, studies in Pinus populations found that thinning intensity reduces tree height growth to promote diameter growth (Nguyen et al., 2017; Deng et al., 2019).
However, most ecological theories have been applied to dense forest tree species (Wright, 2002) and their application to Sudanian woodland ecosystems is questionable. Under Sudanian forest conditions, canopy cover did not strongly affect light penetration, with the exception of stage 4 with a narrow canopy. Variation in recruitment limitation (Hubbell et al., 1999) and resource heterogeneity across a range of ecosystems have been highlighted as important factors determining tree growth and forest productivity. Although efforts were made to select homogeneous stands, in addition to the treatment effect, there was still soil or environmental heterogeneity at a finer scale which could need more attention for further studies.
Species the most affected by thinning and pruning intensity
We found that the height growth is highly diverse between species and may be correlated to the combined effect of treatment and uncontrolled disturbances like wind and rainfall. Terminalia avicennioides, T. leiocarpa, Pteleopsis suberosa, Daniellia oliveri and Combretum collinum, stand out with the best growth performance in the different woodland development stages. This explains their dominance in the vegetation landscape of the study area (Assèdé et al., 2021). The two most dominant tree genera in the Biosphere Reserve of Pendjari are Terminalia and Combretum with fourteen and six species, respectively (Assédé et al., 2012). The highest tree height growth was recorded with T. avicennioides (105.5 mm after 6 months) in stage 3 with 30% thinning. The physiognomy of the vegetation of the Biosphere Reserve of Pendjari is defined by T. avicennioides (Assédé et al., 2012). Several Terminalia tree species were known as fast growth species, especially T. leiocarpa recorded as one of the three highly productive tree species of Sudanian woodland (Hérault et al., 2020). In stage 3, the lowest tree recruitment favored a better growth in height for some species like T. avicennioides. However, there is paucity of relevant and updated information on growth performance of most tree species of the study area. Tree species in the same area may be differently affected by management treatment regimes. Understanding the inter and intra-species functional characteristics of tree species in the different woodland development stages, that contribute to the growth-survival trade-off is a prerequisite for sustainable woodland management (Fayolle et al., 2015). A deep root system may grant better access to subsoil water and great resistance to water stress (Ouédraogo-Koné et al., 2007) especially in dry season. As observed in previous studies in Sudanian woodland, local species showed remarkable productivity performance in mixed stand (Assèdé et al., 2021), a typical characteristic of the vegetation of the study area. The observed negative growth is mainly attributed to stem breaking either by the wind, rainfall and/or small rodents.
Conclusion
Tree response (density, recruitment and height growth) to selective stem thinning and branch pruning is species-specific and varied with thinning intensity and woodland development stages. Findings showed a significant increase in tree density after 30% thinning in woodland development stage 2 and 3. The best tree growth in height is recorded with 30% thinning in woodland development stage 1 and 60% thinning in woodland development stages 2 and 3. A remarkable recruitment of stems ≥1 m height is observed in the absence of thinning and with 30% thinning in woodland development stage 2, while no effect is observed in both stage 1 and 3. Terminalia avicennioides and Pteleopsis suberosa showed the highest average growth in height after 6 months with 30% and 60% thinning and pruning in the woodland development stage 3 respectively. Future long-term studies should test the combined response of trees in terms of diameter and height growth to selective stem thinning. A clear understanding of how climate, pedology of the area, and fauna affect tree growth will allow for a better planning of the management of the developmental stages of Sudanian woodland. This will inform future interventions to improve productivity, ecosystem services including especially provisioning to meet the needs of the local population, biodiversity conservation and ultimately ensuring sustainable resource use and management.
Data availability statement
The raw data supporting the conclusions of this article will be made available by the authors, without undue reservation.
Author contributions
EA, SB, AA, and CG: formulation of research idea and coordination of data collection. EA, SB, HO, and PC: data analyses, manuscript writing, and proof editing. All authors contributed to the article and approved the submitted version.
Funding
We are grateful to the International Foundation for Science (IFS) for financial support through the research grant (Reference: J/5730-1) to EA.
Acknowledgments
We thank the staff of the Biosphere Reserve of Pendjari, farmers and field guides, for their assistance and collaboration during the field work.
Conflict of interest
The authors declare that the research was conducted in the absence of any commercial or financial relationships that could be construed as a potential conflict of interest.
Publisher’s note
All claims expressed in this article are solely those of the authors and do not necessarily represent those of their affiliated organizations, or those of the publisher, the editors and the reviewers. Any product that may be evaluated in this article, or claim that may be made by its manufacturer, is not guaranteed or endorsed by the publisher.
References
Akouehou, G. S., Lokossou, R. S., Lègba, I. S., Bio, I. C., and Yaou, L. Z. (2018). Dynamique des peuplements des Parcelles d’Essais Sylvicoles (PES) de la forêt classée de la Sota au Nord-Est du Bénin. Int. J. Bio. Chem. Sci. 11:1994. doi: 10.4314/ijbcs.v11i5.5
Archer, S. (1995). Tree-grass dynamics in a Prosopis-thornscrub savanna parkland: reconstructing the past and predicting the future. Ecoscience 2, 83–99. doi: 10.1080/11956860.1995.11682272
Assédé, E. P., Adomou, A. C., and Sinsin, B. (2012). Magnoliophyta, biosphere reserve of Pendjari, atacora province, Benin. Check List. 8, 642–661. doi: 10.15560/8.4.642
Assèdé, E. S. P., Azihou, F. A., Biaou, S. S. H., Mariki, S. B., Geldenhuys, C. J., Sinsin, B. (2021). Managing woodland development stages in Sudanian dry woodlands to meet local demand in fuelwood. Ener. Sustain. Dev. 61, 129–138. doi: 10.1016/j.esd.2021.01.006
Assédé, E. S. P., Azihou, F. A., Oumarou, M., and Sinsin, B. (2015). Effet du relief sur la régénération des espèces ligneuses en zone soudanienne du Bénin. Bois For. Trop. 326, 15–24.
Barreto, T. E., Ivanauskas, N. M., Lima, R. A. F. D., Toniato, M. T. Z., Souza, F. M., and Rodrigues, R. R. (2022). Canopy openness and soil conditions explain community structure and diversity in a tropical seasonal forest in south-eastern Brazil. Acta Botan. Brasil. 35, 638–652.
Bell, J. E., Autry, C. W., Mollenkopf, D. A., and Thornton, L. M. (2012). A natural resource scarcity typology: theoretical foundations and strategic implications for supply chain management. J. Bus. Logist. 33, 158–166. doi: 10.1111/j.0000-0000.2012.01048.x
Bruschi, P., Mancini, M., Mattioli, E., Morganti, M., and Signorini, M. A. (2014). Traditional uses of plants in a rural community of Mozambique and possible links with Miombodegradation and harvesting sustainability. J. Ethnobiol. Ethnomed. 10:59. doi: 10.1186/1746-4269-10-59
Burrows, W. H., Beale, I. F., Silcock, R. G., and Pressland, A. J. (1985). “Prediction of tree and shrub population changes in a semi-arid woodland,” in Proceedings of the International Savanna Symposium, Brisbane.
Comita, L. S., Muller-Landau, H. C., Aguilar, S., and Hubbell, S. P. (2010). Asymmetric density dependence shapes species abundances in a tropical tree community. Science 329, 330–332. doi: 10.1126/science.1190772
de Avila, A. L., Ruschel, A. R., de Carvalho, J. O. P., Mazzei, L., Silva, J. N. M., do Carmo Lopes, J., et al. (2015). Medium-term dynamics of tree species composition in response to silvicultural intervention intensities in a tropical rain forest. Biol. Conserv. 191, 577–586. doi: 10.1016/j.biocon.2015.08.004
de Avila, A. L., Schwartz, G., Ruschel, A. R., do Carmo Lopes, J., Silva, J. N. M., de Carvalho, J. O. P., et al. (2017). Recruitment, growth and recovery of commercial tree species over 30 years following logging and thinning in a tropical rain forest. For. Ecol. Manage. 385, 225–235. doi: 10.1016/j.foreco.2016.11.039
Deng, C., Zhang, S., Lu, Y., Froese, R. E., Ming, A., and Li, Q. (2019). Thinning Effects on the Tree Height–Diameter Allometry of Masson Pine (Pinus massoniana Lamb.). Forests 10:1129. doi: 10.3390/f10121129
Ding, Y., and Zang, R. (2021). Determinants of aboveground biomass in forests across three climatic zones in China. For. Ecol. Manag. 482:118805. doi: 10.1016/j.jenvman.2020.111805
Djibril, B. R. (2002). Impacts de l’utilisation des engrais chimiques et des pesticides sur la qualité des eaux de surface dans la Réserve de Biosphère de la Pendjari.–Mémoire de fin de formation d’Ingénieurs de travaux en Techniques d’Aménagement et de Protection de l’Environnement. Cotonou: APE/CPU/UAC.
Fayolle, A., Ouédraogo, D.-Y., Ligot, G., Daïnou, K., Bourland, N., Tekam, P., et al. (2015). Differential performance between two timber species in forest logging gaps and in plantations in Central Africa. Forests 6, 380–394. doi: 10.3390/f6020380
Fischer, S., Greet, J., Walsh, C. J., and Catford, J. A. (2021). Restored river-floodplain connectivity promotes woody plant establishment. For. Ecol. Manage. 493:119264. doi: 10.1016/j.foreco.2021.119264
Garcia-Barrios, L., Galvan-Miyoshi, Y., Valdivieso-Perez, I., Masera, O., Bocco, G., and Van-dermeer, J. (2009). Neotropical forest conservation, agricultural intensification, and rural out-migration: The Mexican experience. BioScience 59, 863–873. doi: 10.1525/bio.2009.59.10.8
Geldenhuys, C. J., Prinsloo, A. J., and Antão, L. V. T. (2018). Monitoring the impact of selective thinning and pruning on recovery of condition, biodiversity and productivity in Miombo woodland development stages: Report 2: Wood harvested through different harvesting intensities, Vila Ulongwe and Nkantha areas, Tete Province, Mozambique. Report FW-07/18. Pretoria: Forest wood cc.
Geldenhuys, C. J., Sippel, W. E., and Sippel, E. (2013). Indigenous woodland management training manual, Universal Leaf Africa: forestry for small-scale farmers. Nelspruit: Working on Fire International and Universal Leaf Africa. Galela: WoFI International Holdings (Pty) Ltd.
Ghermandi, A., Van Den Bergh, J. C., Brander, L. M., De Groot, H. L., and Nunes, P. A. (2010). Values of natural and human-made wetlands: A meta-analysis. Water Resour. Res. 46, 1–12. doi: 10.1029/2010WR009071
Gonzalez, P. (2005). Forest and woodland systems. Millennium ecosystem assessment: ecosystems and human well-being: current state & trends assessment. Washington, DC: Island Press.
Hall, J. S. (2011). “Natural Forest Silviculture for Central African Meliaceae,” in Silviculture in the Tropics, eds S. Günter, M. Weber, B. Stimm, and R. Mosandl (Berlin: Springer Berlin Heidelberg).
Hérault, B., N’guessan, A. K., Ouattara, N., Ahoba, A., Bénédet, F., Coulibaly, B., et al. (2020). The long-term performance of 35 tree species of sudanian West Africa in pure and mixed plantings. For. Ecol. Manage. 468:118171.
Hernández-Barrios, J. C., Anten, N. P., and Martínez-Ramos, M. (2015). Sustainable harvesting of non-timber forest products based on ecological and economic criteria. J. Appl. Ecol. 52, 389–401. doi: 10.1111/1365-2664.12384
Heubach, K., Wittig, R., Nuppenau, E. A., and Hahn, K. (2011). The economic importance of non-timber forest products (NTFPs) for livelihood maintenance of rural west African communities: A case study from northern Benin. Ecol. Eco. 70, 1991–2001. doi: 10.1016/j.ecolecon.2011.05.015
Hogan, J. A., Hérault, B., Bachelot, B., Gorel, A., Jounieaux, M., and Baraloto, C. (2018). Understanding the recruitment response of juvenile Neotropical trees to logging intensity using functional traits. Ecol. Appl. 28, 1998–2010. doi: 10.1002/eap.1776
Hubbell, S. P., Foster, R. B., O’Brien, S. T., Harms, K. E., Condit, R., Wechsler, B., et al. (1999). Light-gap disturbances, recruitment limitation, and tree diversity in a neotropical forest. Science 283, 554–557. doi: 10.1126/science.283.5401.554
Kabré, B., Belem/Ouédraogo, M., Lankoandé, B., and Ouédraogo, A. (2020). Variabilité démographique de Saba senegalensis (A. DC.) Pichon suivant le gradient climatique au Burkina Faso. Bois For. Trop. 345, 75–85. doi: 10.19182/bft2020.345.a31930
Kalema, V. N., Witkowski, E. T. F., Erasmus, B. F. N., and Mwavu, E. N. (2015). The Impacts of Changes in Land Use on Woodlands in an Equatorial African Savanna. Land Degrad. Dev. 26, 632–641. doi: 10.1002/ldr.2279
Lawali, S., Diouf, A., Morou, B., Abdou Kona, K., Saidou, L., Guero, et al. (2018). Régénération Naturelle Assistée (RNA): outil d’adaptation et résilience des ménages ruraux d’Aguié au Niger. Int. J. Bio. Chem. Sci. 12:75. doi: 10.4314/ijbcs.v12i1.6
Mahayani, N. P. D., Slik, F. J., Webb, E. L., Savini, T., and Gale, G. A. (2022). Changes in tree functional composition and forest functioning ten years after logging and thinning interventions in Bornean tropical forests. For. Ecol. Manage. 506:119948. doi: 10.1016/j.foreco.2021.119948
Manaute, J. (1996). Etude de l’influence du feu et du pâturage sur la régénération par rejets de souche d’un peuplement naturel exploité en coupe sélective dans le centre ouest du Burkina Faso. Résultats préliminaires à trois ans de suivis. Paris: ENGREF.
Manyanda, B. J., Nzunda, E. F., Mugasha, W. A., and Malimbwi, R. E. (2020). Estimates of Volume and Carbon Stock Removals in Miombo Woodlands of Mainland Tanzania. Int. J. For. Res. 2020, 1–10. doi: 10.1155/2020/4043965
Nguyen, T. L., Koh, B. S., Lee, G., Lee, Y., Shin, W. T., Tran, S. N., et al. (2017). “Coastal ecosystem and water quality monitoring in Soc Trang Province, Vietnam,” in Proceedings of the 13th International MEDCOAST Congress on Coastal and Marine Sciences, Engineering, Management and Conservation, Ho Chi Minh City.
Ouedraogo, I., Tigabu, M., Savadogo, P., Compaoré, H., Odén, P. C., and Ouadba, J. M. (2010). Land cover change and its relation with population dynamics in Burkina Faso, West Africa: LAND COVER CHANGE IN BURKINA FASO. Land. Degrad. Dev. 21, 453–462. doi: 10.1002/ldr.981
Ouédraogo-Koné, S., Kaboré-Zoungrana, C. Y., and Ledin, I. (2007). Important characteristics of some browse species in an agrosilvopastoral system in West Africa. Agrofor. Syst. 74, 213–221. doi: 10.1007/s10457-007-9095-0
Pinheiro, J. C., and Bates, D. M. (2000). Mixed-effects models in S and S-PLUS, Statistics and computing. New York, NY: Springer, doi: 10.1007/978-1-4419-0318-1
R Core Team (2022). R: A Language and Environment for Statistical Computing. Vienna: R Foundation for Statistical Computing.
Saarinen, N., Kankare, V., Yrttimaa, T., Viljanen, N., Honkavaara, E., Holopainen, et al. (2020). Assessing the effects of thinning on stem growth allocation of individual Scots pine trees. For. Ecol. Manage. 474:118344. doi: 10.1016/j.foreco.2020.118344
Shackleton, C. M., Pandey, A. K., and Ticktin, T. (2015). Ecological sustainability for non-timber forest products. Milton Park: Taylor & Francis, doi: 10.4324/9781315851587
Stanley, D., Voeks, R., and Short, L. (2012). Is non-timber forest product harvest sustainable in the less developed world? A systematic review of the recent economic and ecological literature. Ethnobiol. Conserv. 1:9. doi: 10.15451/ec2012-8-1.9-1-39
Syampungani, S., Geldenhuys, C. J., and Chirwa, P. W. (2016). Regeneration dynamics of miombo woodland in response to different anthropogenic disturbances: forest characterisation for sustainable management. Agroforest. Syst. 90, 563–576. doi: 10.1007/s10457-015-9841-7
Tadele, M., Birhane, E., Kidu, G., G-Wahid, H., and Rannestad, M. M. (2020). Contribution of Parkland Agroforestry in Meeting Fuel Wood Demand in the Dry Lands of Tigray, Ethiopia. J. Sustain. For. 39, 841–853. doi: 10.1080/10549811.2020.1738946
Tonga Ketchatang, P., Zapfack, L., Kabelong Banoho, L. P. R., and Endamana, D. (2017). Disponibilité des produits forestiers non ligneux fondamentaux à la périphérie du Parc national de Lobeke. VertigO - la revue électronique en sciences de l’environnement. Paris: OpenEdition, doi: 10.4000/vertigo.18770
Valencia, V., García-Barrios, L., West, P., Sterling, E. J., and Naeem, S. (2014). The role of coffee agroforestry in the conservation of tree diversity and community composition of native forests in a Biosphere Reserve. Agric. Ecosyst. Environ. 189, 154–163. doi: 10.1016/j.agee.2014.03.024
Valinger, E., Sjögren, H., Nord, G., and Cedergren, J. (2019). Effects on stem growth of Scots pine 33 years after thinning and/or fertilization in northern Sweden. Scand. J. For. Res. 34, 33–38. doi: 10.1080/02827581.2018.1545920
Wang, H., Aragam, B., and Xing, E. P. (2017). “Variable selection in heterogeneous datasets: A truncated-rank sparse linear mixed model with applications to genome-wide association studies,” in Paper presented at the 2017 IEEE International Conference on Bioinformatics and Biomedicine (BIBM), Kansas City, MO.
Wright, J. S. (2002). Plant diversity in tropical forests: A review of mechanisms of species coexistence. Oecologia 130, 1–14. doi: 10.1007/s004420100809
Yu, J., Zhang, X., Xu, C., Hao, M., Choe, C., and He, H. (2022). Thinning can increase shrub diversity and decrease herb diversity by regulating light and soil environments. Front. Plant Sci. 13:948648. doi: 10.3389/fpls.2022.948648
Zuur, A. F., Ieno, E. N., Walker, N. J., Saveliev, A. A., and Smith, G. M. (2009). Mixed effects modelling for nested data, in: Mixed Effects Models and Extensions in Ecology with R. Berlin: Springer.
Appendix
Keywords: woodland, tree growth, stem thinning, branch pruning, Sudanian zone
Citation: Assèdé ESP, Biaou SSH, Azihou AF, Orou H, Geldenhuys CJ and Chirwa PW (2023) Trees response to selective thinning and pruning in Sudanian woodland zone. Front. For. Glob. Change 6:1108323. doi: 10.3389/ffgc.2023.1108323
Received: 25 November 2022; Accepted: 26 May 2023;
Published: 15 June 2023.
Edited by:
Robert Nofemela, Agricultural Research Council (ARC), South AfricaReviewed by:
Nyong Princely Awazi, The University of Bamenda, CameroonAbayneh Derero, Ethiopian Forestry Development, Ethiopia
Copyright © 2023 Assèdé, Biaou, Azihou, Orou, Geldenhuys and Chirwa. This is an open-access article distributed under the terms of the Creative Commons Attribution License (CC BY). The use, distribution or reproduction in other forums is permitted, provided the original author(s) and the copyright owner(s) are credited and that the original publication in this journal is cited, in accordance with accepted academic practice. No use, distribution or reproduction is permitted which does not comply with these terms.
*Correspondence: Eméline Sêssi Pélagie Assèdé, assedeemeline@gmail.com