- 1Climate Impacts Research Centre, Department of Ecology and Environmental Science, Umeå University, Umeå, Sweden
- 2Department of Physiological Diversity, Helmholtz Centre for Environmental Research – UFZ, Leipzig, Germany
- 3German Centre for Integrative Biodiversity Research (iDiv) Halle-Jena-Leipzig, Leipzig, Germany
- 4Department of Soil and Environment, Swedish University of Agricultural Sciences, Uppsala, Sweden
- 5Terrestrial Ecology Section, Department of Biology, University of Copenhagen, Copenhagen, Denmark
- 6Department of Forest Ecology and Management, Swedish University of Agricultural Sciences, Umeå, Sweden
- 7Department of Ecology and Environmental Sciences, Umeå University, Umeå, Sweden
Introduction: Survival and growth of tree seedlings are key processes of regeneration in forest ecosystems. However, little is known about how climate warming modulates seedling performance either directly or in interaction with understory vegetation and post-fire successional stages.
Methods: We measured survival (over 3 years) and growth of seedlings of three tree species (Betula pubescens, Pinus sylvestris, and Picea abies) in a full-factorial field experiment with passive warming and removal of two plant functional groups (feather moss and/or ericaceous shrubs) along a post-fire chronosequence in an unmanaged boreal forest.
Results: Warming had no effect on seedling survival over time or on relative biomass growth. Meanwhile, moss removal greatly increased seedling survival overall, while shrub removal canceled this effect for B. pubescens seedlings. In addition, B. pubescens and P. sylvestris survival benefitted most from moss removal in old forests (>260 years since last fire disturbance). In contrast to survival, seedling growth was promoted by shrub removal for two out of three species, i.e., P. sylvestris and P. abies, meaning that seedling survival and growth are governed by different understory functional groups affecting seedling performance through different mechanism and modes of action.
Discussion: Our findings highlight that understory vegetation and to a lesser extent post-fire successional stage are important drivers of seedling performance while the direct effect of climate warming is not. This suggests that tree regeneration in future forests may be more responsive to changes in understory vegetation or fire regime, e.g., indirectly caused by warming, than to direct or interactive effects of rising temperatures.
1. Introduction
The boreal forest is one of the world’s largest biomes and it provides important ecosystem services such as carbon storage, biodiversity refuges, and timber (Pan et al., 2011; Gauthier et al., 2015). Recurrent fires are a natural part of the boreal biome and a key driver of forest community dynamics (Bradshaw, 1993; Kuuluvainen and Aakala, 2011; Davis et al., 2018), because fire-induced disturbance can among others create recruitment opportunities for seedlings (Kuuluvainen and Rouvinen, 2000). The frequency and severity of fire events in large parts of the boreal region are expected to increase in response to climate change (de Groot et al., 2013; Flannigan et al., 2013), although this is counteracted by fire management and increasing precipitation in some regions (Pinto et al., 2020; Krikken et al., 2021). Changes in fire regimes coupled with rising temperatures, expected to be particularly amplified in high latitudes (Previdi et al., 2021), are likely to have large effects on boreal forest ecosystems, including on those processes that are involved in post-fire seedling recruitment (Walker et al., 2019; McDowell et al., 2020). Seedling recruitment is essential for forest regeneration and ultimately influences the composition and resilience of future forests. Warming and fire history are also likely to alter under- and overstory vegetation composition (Hedwall et al., 2019; Mekonnen et al., 2019), which in turn impacts on forest regeneration (Nilsson and Wardle, 2005; Johnstone et al., 2010). However, little is known about direct effects of warming, and combined effects of warming, understory vegetation and fire history, on seedling recruitment.
Seedling establishment is often temperature-limited (Körner and Paulsen, 2004) and higher temperatures might therefore increase seedling survival and growth in high latitude boreal systems. Indeed, a recent study showed that seedling survival increased in response to warming in greenhouse conditions, but in contrast decreased in the field (Hansen and Turner, 2019). Further, relaxation of cold-limitation can positively affect seedling growth by alleviating physiological constraints on bud break (Walck et al., 2011) and photosynthesis (Saxe et al., 2000; Danby and Hik, 2007; Ryan, 2013). However, seedling growth has been observed to increase (Kellomaki and Wang, 2001; Xu et al., 2012), remain unchanged (Lathi et al., 2005; Pumpanen et al., 2012) or decrease (Day et al., 2005; Okano and Bret-Harte, 2015) in response to higher temperatures. This suggests that both seedling survival and growth responses to warming could be mediated by a variety of biotic and abiotic conditions that vary considerably among unmanaged boreal forest ecosystems, but the role of these mediators in influencing seedlings in these forests is not well studied.
The boreal understory vegetation is dominated by ericaceous shrubs (e.g., Empetrum hermaphroditum, Vaccinium myrtillus, and Vaccinium vitis-idaea) and feather mosses (e.g., Pleurozium schreberi), which can suppress seedling establishment by competition for light (Montgomery et al., 2010; Thrippleton et al., 2016) and nutrients (Stuiver et al., 2014). In general, climate warming is expected to promote competitive rather than facilitative effects (Callaway et al., 2002). For example, climate warming can increase competition for resources (Zackrisson et al., 1998; Soudzilovskaia et al., 2011) and potentially exacerbate the negative effect of surrounding vegetation on seedling establishment. However, feather mosses can also retain water (Oleskog and Sahlen, 2000; Turetsky, 2003; Elumeeva et al., 2011), buffer soil temperatures (Blok et al., 2011; Wheeler et al., 2011) and fix nitrogen (N) via association with cyanobacteria (DeLuca T. H. et al., 2002; Turetsky, 2003; Sorensen et al., 2012), all of which could potentially enhance seedling performance (Davis et al., 2018). As such, seedlings growing in moss might be able to better withstand moisture limitation resulting from climate warming (Oleskog and Sahlen, 2000; Turetsky, 2003; Elumeeva et al., 2011) and soil temperature fluctuations (Blok et al., 2011; Wheeler et al., 2011). Seedlings growing among shrubs are likely to be exposed to resource competition (Montgomery et al., 2010), which could potentially be intensified by climate change. On the other hand, shrubs can benefit seedlings, for example by protecting them from herbivores or frost damage (Gomez-Aparicio et al., 2008; Barbosa et al., 2009; Jensen et al., 2012). Thus, shrubs and mosses could affect seedling responses to warming differently, but this has not yet been studied. Furthermore, the combined effects of mosses and shrubs could have either positive or negative interactive effects on seedling performance (Wardle et al., 2008), yet our understanding of such complex mechanisms is limited.
Boreal forest regeneration is governed by recurring fire events, which drive successional trajectories in the forest under- and overstory (Nilsson and Wardle, 2005). With increasing time since fire, ericaceous dwarf shrubs with higher litter quality (e.g., V. myrtillus) are gradually replaced by dwarf shrubs with lower litter quality (e.g., E. hermaphroditum) (Nilsson and Wardle, 2005). This succession leads to a decline in nutrient availability (DeLuca T. et al., 2002), which can reduce seedling establishment (Kuuluvainen and Rouvinen, 2000; Mallik, 2003). Simultaneously, moss cover increases in thickness in the understory during succession and as time since fire increases (DeLuca T. et al., 2002; Turetsky et al., 2010), thus potentially making it more competitive against tree seedlings (Stuiver et al., 2014) especially if water becomes a limiting factor (Lindo and Gonzalez, 2010; Soudzilovskaia et al., 2013). However, warmer temperatures might also reduce moss cover (Alatalo et al., 2020) and thus weaken potential moss-induced facilitative as well as competitive interaction with tree seedlings. The combined effects of warming, moss cover and shrub cover on tree seedling performance change across post-fire successions have not been studied, but understanding this is important for predicting how boreal forest ecosystems will respond to future changes in climate and fire regime.
The aim of our study is to investigate how climate warming both directly and in interaction with plant functional groups and post-fire successional stage can modify tree seedling performance in unmanaged boreal forests. To investigate seedling performance, we followed for 3 years the survival and growth of planted seedlings of the three most common Fennoscandian boreal tree species (Betula pubescens, Pinus sylvestris, and Picea abies) in response to climate warming, understory functional group removal (i.e., feather mosses and/or ericaceous shrubs) and post-fire successional stage. We hypothesized that (1) warming increases seedling performance, with the positive effect of warming on seedling performance being weakened by the presence of mosses and shrubs, and (2) that the positive effect of warming on seedling performance and the mediation of this effect by the presence of mosses and/or shrubs, depends on post-fire successional stage, with stronger positive effects in early- rather than in late-successional stages.
2. Materials and methods
2.1. Study site and experimental design
This experiment was carried out in northern Sweden on ten experimental sites in the vicinity of Arvidsjaur (65°35′–66°07′N, 17°15′–19°26′E). The sites are distributed along a natural fire-induced gradient of successional stages with time since the last fire disturbance ranging from 48 to 368 years (Zackrisson et al., 1996). Based on the time since last fire disturbance, the sites are classified as young (<100 years since the last fire; N = 3), intermediate (100–260 years since the last fire; N = 4), or old (>260 years since the last fire; N = 3) (Jackson et al., 2013; Supplementary Figure 1 and Supplementary Table 1). The most abundant tree species on all sites are B. pubescens, P. abies, and P. sylvestris (Jackson et al., 2013). The understory vegetation across the ten sites is characterized by ericaceous shrubs (E. hermaphroditum, V. myrtillus, and V. vitis-idaea) and the feather moss P. schreberi (Jackson et al., 2013). The relative abundance of the various over- and understory species is dependent on the time since the last fire: younger sites are characterized by shallow feather moss layer (mean: 29.2 mm), dominant biomass of V. myrtillus (mean: 102.3 g/m2) and high basal area of P. sylvestris (mean: 18.4 m2/ha), whereas older sites have a thick feather moss layer (mean: 51.4 mm), high biomass of E. hermaphroditum (mean: 90.5 g/m2) and dominant basal area of P. abies (mean: 8.9 m2/ha) (DeLuca T. et al., 2002; Jackson et al., 2013; De Long et al., 2015; Supplementary Table 1). The soils of all sites of the chronosequence are either Typic or Entic Haplocryods, which are acidic podzols typical of cold and wet environments (DeLuca T. et al., 2002). The mean annual air temperature across the sites is −2°C (average temperature in January: −14°C; average temperature in July: 12°C) and the mean annual precipitation is 600 mm (Jackson et al., 2013).
We established the experiment in 2010 with a full factorial combination of passive warming, shrub removal and moss removal at each of the ten sites. To apply the treatments, we established hexagonally shaped plots (diameter of 165 cm × 190 cm; total area 2.35 m2), which each contained a homogeneous vascular plant community composition and feather moss (Hylocomium splendens and P. schreberi) coverage specific for each site (De Long et al., 2015). Each of these plots was randomly assigned to one of four full factorial combinations of warming (ambient or increased temperature) and shrub removal (shrubs present or removed). These plots were then halved by a north-south middle line to create subplots randomly assigned to one level of the moss removal treatment (moss present or moss removed). This resulted in 8 different treatment combinations per site (Supplementary Figure 1) and 80 experimental subplots across the 10 sites.
To increase soil and air temperature of the plots assigned to warming we used transparent Perspex Open-Top Chambers (OTCs) (diameter: 165 cm × 190 cm, height: 47 cm, central exposed area: 0.95 m2, MakroLife, Arla Plast AB, Sweden); a widely used method to investigate warming effects in tundra (Henry and Molau, 1997; Marion et al., 1997) and forested systems (Munier et al., 2010; Kaarlejärvi et al., 2012). To simulate summer warming during the growing season, we placed the OTCs over each plot assigned to warming each year in June and removed them again in October. The OTCs increased the air temperature by 0.4°C and the soil temperature by 0.2°C during summer (De Long et al., 2015) which is comparable to other studies in forest ecosystems (De Frenne et al., 2009). To avoid interference due to continuous shading of the forest canopy with the warming treatment the experimental plots were placed under canopy gaps at each site. In addition, we severed all roots around the outer edge of each plot with a shovel (to a depth of approximately 25 cm or the nearest rock) to exclude interference from tree roots.
For the shrub and moss removal treatments, we manually removed the aboveground parts of the respective functional group at the beginning of the growing season, but left the belowground parts intact to avoid disturbance to the litter layer and other plants (Wardle and Zackrisson, 2005; De Long et al., 2015). The functional group removal treatment was done once per year.
2.2. Seedlings planting and harvest
In the summer of 2013, when the disturbance impact of the initial vegetation cover removal was considered to have largely ceased (Wardle and Zackrisson, 2005), we planted two cohorts of tree seedlings in each subplot, one in June and one in August (hereafter referred to as “first” and “second” seedlings, respectively). Prior to transplanting, we grew all seedlings from commercial seeds for northern Swedish and Finnish provenances (Svenska Skogsplantor AB): B. pubescens (Patana I SV-421), P. sylvestris (ZON 6800 200), and P. abies (Björkebo FP-19). Before transplantation to the field in the beginning of June, the “first” seedlings were grown in trays with sand; B. pubescens seedlings in warmed (initially 20°C, later 15°C) and lit growth chambers for two and a half weeks, and P. sylvestris and P. abies seedlings in a greenhouse for one and a half weeks. The “second” seedlings were all grown in individual small pots in a 1:1 (vol.) sand:potting-soil mixture in the greenhouse for approximately 2 months before transplantation to the field at the end of August 2013. The evening before transplantation, we gently washed all seedlings free of sand and soil and kept them between moist tissues at 5°C overnight. At the time of transplantation, all first seedlings had only developed their first cotyledons and a limited root system (tap root with beginning lateral roots), while the second seedlings had developed several leaves and a viable root system.
In June 2013, we planted the first cohort: 50 B. pubescens seedlings, 20 P. sylvestris seedlings, and 10 P. abies seedlings per subplot. To account for heterogeneity in soil and vegetation, individuals were planted in one of two locations for each species within each subplot, resulting in two groups of 25 B. pubescens seedlings, two groups of ten P. sylvestris seedlings, and two groups of five P. abies seedlings per subplot. The number of transplanted seedlings varied between species, due to interspecific differences in germination and pre-transplantation survival, but the distance between transplanted seedlings was kept constant across species (approximately 1.5 cm). As there was very little within-species size variation in this cohort of pre-grown first seedlings, we randomly distributed all these seedlings across sites and subplots.
In late August 2013, we planted a second cohort of larger seedlings than in the first cohort to make sure that there would be enough many seedlings survive throughout the years for the final biomass analysis. This was done because the seedlings of the first cohort were very small and thus had a high risk of dying before the end of the study. The second cohort consisted of five B. pubescens seedlings and four of each P. sylvestris and P. abies seedlings per subplot. Each of these individual seedlings was planted at its own location within each subplot, to account for within-plot heterogeneity, and these locations were different than the locations at which the first cohort seedlings were planted. Further, to account for within-species size variation in the pre-grown “second” seedlings, all these seedlings were grouped into 3–4 size classes and seedlings within each size class were then randomly distributed across all sites and subplots. In addition, we oven-dried (48 h at 60°C) and weighed multiple seedlings of each cohort and species to obtain an estimate of the initial species- and cohort-specific total dry biomass (see Supplementary Table 2).
We planted all seedlings at least 30 cm from the outer edge of the hexagonal plots and at least 10 cm from the border between the subplots, as in Lett et al. (2020). The seedlings were planted at a depth that is comparable to that at which naturally germinated seedlings are positioned, i.e., in the feather moss layer with their stem base a few cm below the tips of the mosses, or in the organic soil with their stem base a few mm below the soil surface (Lett et al., 2017). After transplantation, all living seedlings from the “first” cohort were counted twice per year, in early (June/July) and late (August/September) summer, through 2013 until 2016, to assess their survival.
To obtain the biomass growth dataset in spite of a high seedling mortality rate, we combined biomass data from both cohorts of seedlings (see below). In August 2016, we thus harvested the above- and belowground biomass of the largest of the surviving seedlings of each species per subplot for both the first and second seedling cohorts, in all cases in which at least one seedling was still remaining. We used the largest seedlings because the size of the largest seedling more accurately reflects treatment-induced plot conditions on seedling biomass growth than the average biomass of all surviving seedlings per plot, which is biased by seedling mortality (Wardle et al., 2008). All harvested seedlings were washed in water to remove soil particles and any attached plant material. The seedlings were oven-dried for 48 h at 60°C and weighed. To combine the biomass data for the two cohorts, which differed due to age differences and planting date, we fitted linear regression models for each species describing the relation between first and second seedling biomass (Supplementary Figure 2). We then used these relations to convert the biomass values of second seedlings into that of first seedlings. For subplots with both second and first seedlings, we averaged the biomass of the first and converted second seedling to be consistent with the approach used for subplots with seedlings of only one cohort. In total, we thus harvested 276 seedlings for biomass analysis and obtained growth data for 94, 90, and 92 individuals of B. pubescens, P. sylvestris, and P. abies, respectively. We then calculated total relative biomass increase for each individual seedling (hereafter called “growth”) as: (individual seedling total dry mass at harvest − initial species-specific × cohort-specific total dry mass) / initial species-specific × cohort-specific total dry mass.
2.3. Statistical analysis
To assess the main and interactive effects of warming, moss removal, shrub removal and successional stage on seedling survival from 2013 to 2016, we applied a Cox mixed effects model for each tree species separately, using only the survival data from the first cohort of seedlings. Cox models evaluate the survival probability for each individual subject (here tree seedling) at a given time and allow for integration of random factors. Here we used subplot nested within plot nested within site as a random factor to account for the nested experimental design. We assessed the significance of the effects of the treatments and their interactions using Chi-square tests (Therneau, 2020).
To analyze the main and interactive effects of warming, moss removal, shrub removal, and successional stage on seedling growth, we applied linear mixed effect models separately for each specie, using the combined data from both seedling cohorts. We used subplot nested within plot nested within site as random factor to account for the nested experimental design. Due to 100% mortality in some of the treatment combinations (i.e., no seedling was available for biomass harvest) we excluded four-way interactions from the models. The significance of treatment effects was assessed with F-tests. We used model diagnostic plots to check the homogeneity of variances and the normality of errors (Crawley, 2007) and the data were log- or square-root-transformed whenever necessary (Tables 1, 2).
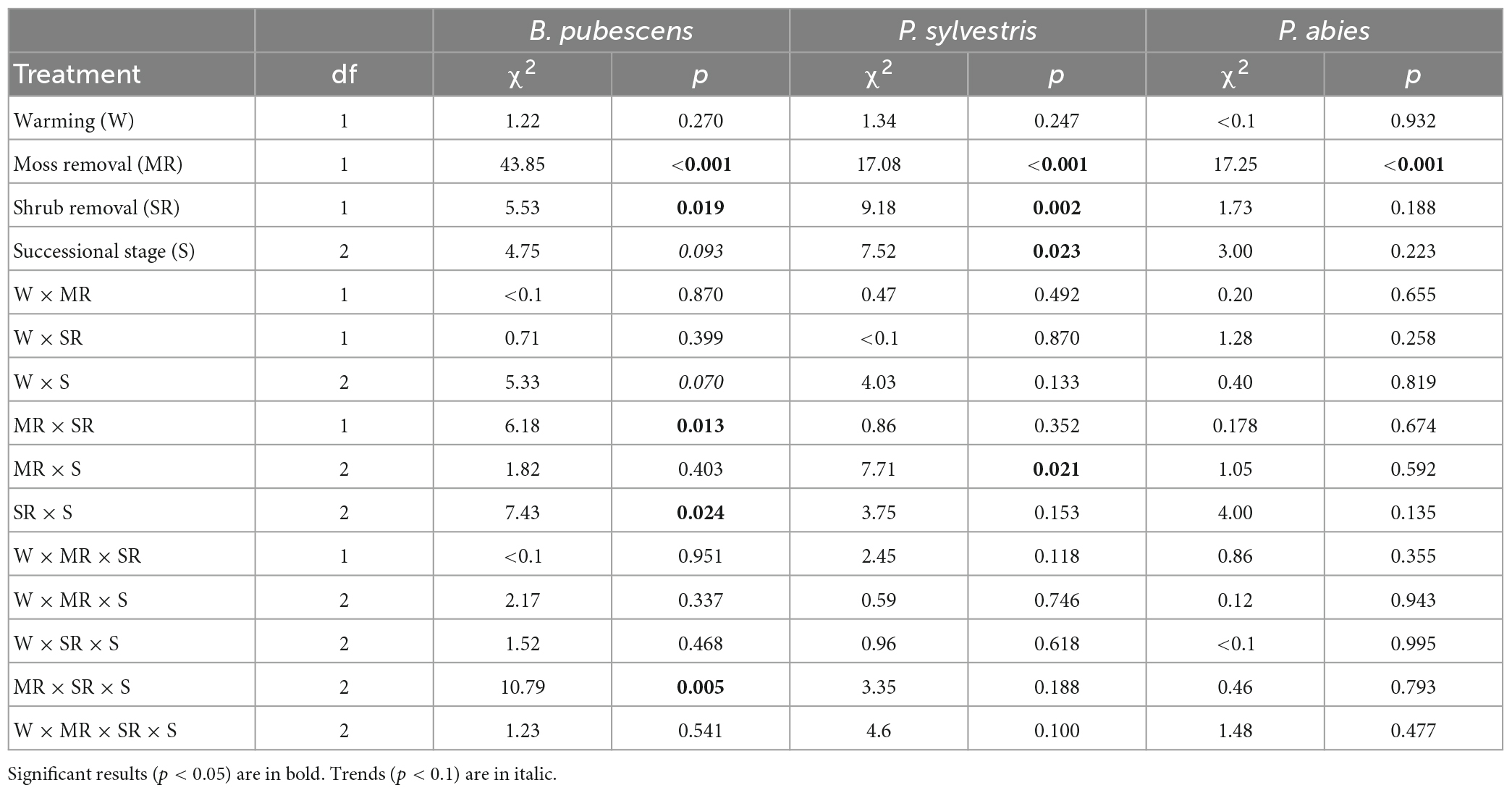
Table 1. Results of Cox mixed effect models testing the effects of warming, moss removal, shrub removal, successional stage, and their interactions on seedling survival from 2013 to 2016 for B. pubescens, P. sylvestris, and P. abies.
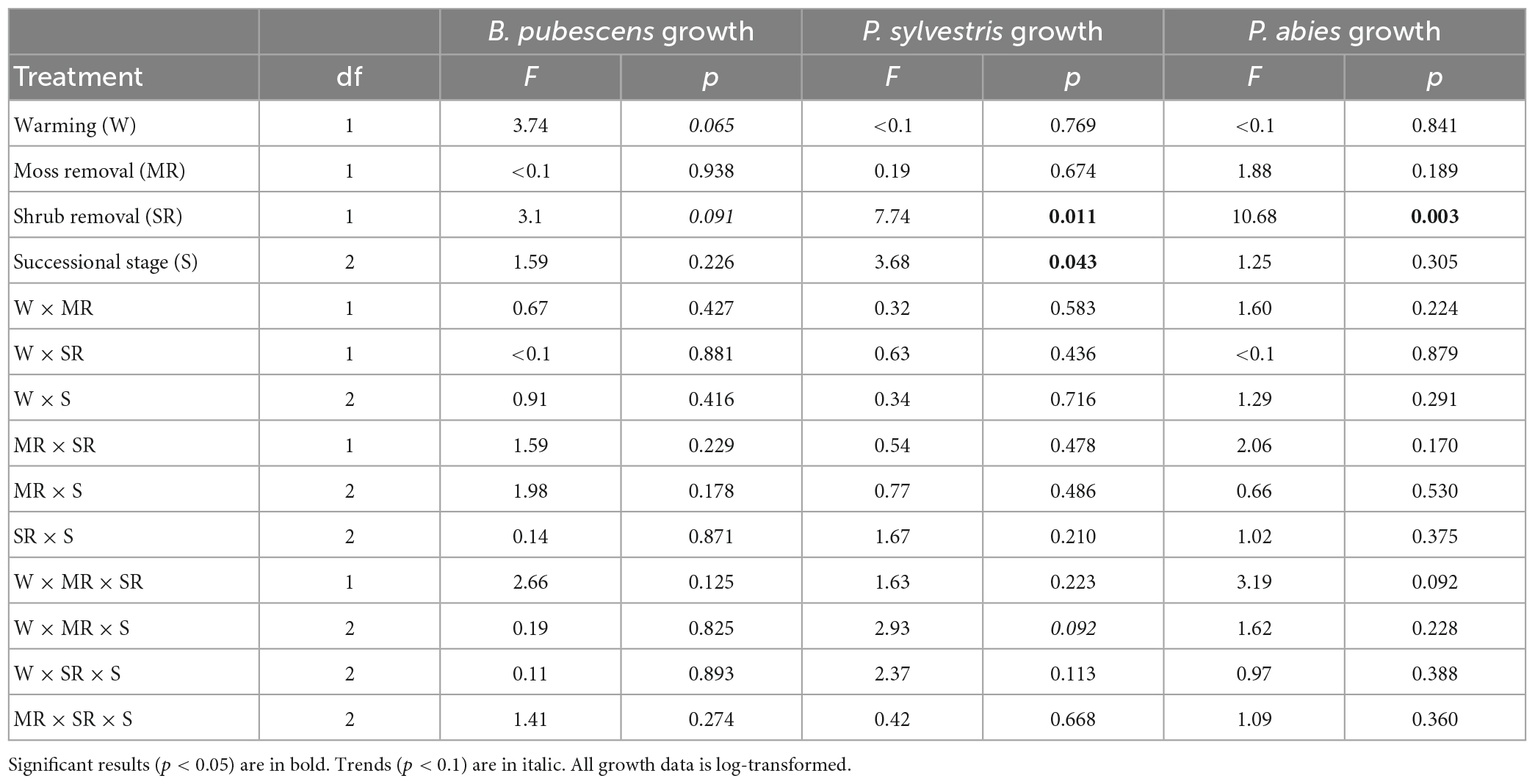
Table 2. Results of the linear mixed effect models testing the effects of warming, moss removal, shrub removal, successional stage, and their interactions on B. pubescens, P. sylvestris, and P. abies growth.
We used the “coxme” package (Therneau, 2020) to run Cox mix effect models, the “lme4” package (Bates et al., 2015) to run linear mixed effect models, the “tidyverse” and the “reshape2” package (Wickham, 2007; Wickham et al., 2019) to arrange data and the “ggplot2” package (Wickham, 2016) for plotting in R statistical software (R Core Team, 2020 version 4.0.2).
3. Results
3.1. Tree seedling survival
At the last survival counting occasion after 3 years 361 (9%) out of 4,000 planted B. pubescens, 357 (22.3%) out of 1,600 planted P. sylvestris and 105 (13.1%) out of 800 planted P. abies seedlings had survived. Throughout the duration of the experiment, warming had no significant effects on survival of any of the three tree seedling species either by itself or in combination with any other factor (Table 1), although there was a trend (p < 0.1) for a weak positive warming effect in the younger successional stages changing into a negative effect in the older stages for B. pubescens (Figure 1A, warming × successional stage interaction, Table 1).
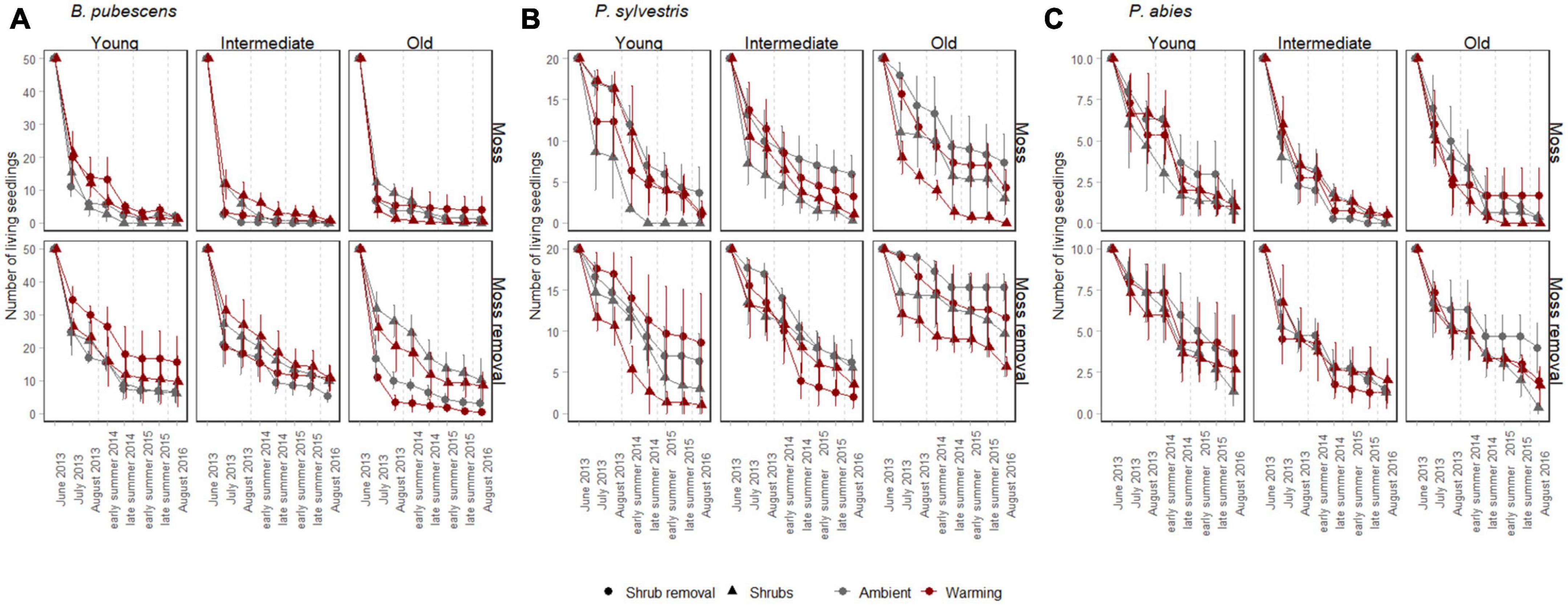
Figure 1. Mean survival (± SE) of B. pubescens seedlings (A, 50 initially planted individuals per subplot), P. sylvestris seedlings (B, 20 initially planted individuals per subplot), and P. abies seedlings (C, 10 initially planted individuals per subplot) from 2013 to 2016. The seedlings were surveyed in warmed (red) and ambient (gray) conditions, with shrubs present (triangle) or with shrubs removed (point), with mosses present (upper panel) or with mosses removed (lower panel) along a post-fire chronosequence with young, intermediate and old sites. Seedling survival was assessed twice per year during early (June/July) and late (August/September) summer.
Moss removal, shrub removal and post-fire successional stage all exhibited some significant species-specific main and interactive effects on seedling survival. As such, moss removal increased survival of all three species, with B. pubescens receiving the greatest benefit (Table 1 and Figures 1A–C). For this species, moss removal moreover increased survival more when shrubs were present compared to when shrubs were removed (moss removal × shrub removal interaction, Table 1) and this effect was more pronounced in the late post-fire successional stage (moss removal × shrub removal × successional stage interaction, Figure 1A and Table 1). For P. sylvestris seedlings, the positive effect of moss removal on survival was also highest in old successional stages (moss removal × successional stage interaction, Figure 1B and Table 1) but not dependent on shrub removal. The positive effect of moss removal on P. abies seedlings was independent of shrub removal and post fire successional stage (Figure 1C and Table 1).
Shrub removal reduced survival overall for B. pubescens seedlings, increased survival overall for P. sylvestris seedlings and had no effect on P. abies seedlings (Figures 1A–C and Table 1). The effect of shrub removal on B. pubescens survival changed from slightly positive to negative with increasing time since last fire (shrub removal × successional stage interaction, Figure 1A and Table 1), which was primarily due to a strong negative effect of shrub removal on B. pubescens seedling survival when combined with moss removal in the older successional stages (shrub removal × moss removal × successional stage interaction, Figure 1A and Table 1). There was no interactive effect of shrub removal with any other factor on seedling survival of either P. sylvestris or P. abies.
There was a significant main effect of post-fire successional stage on P. sylvestris seedling survival, as more seedlings survived in old compared to young and intermediate sites (Figure 1C and Table 1). There was no significant main effect of successional stage on seedling survival for P. abies, but a weak trend (p < 0.1) of higher survival in young compared to intermediate and old sites for B. pubescens (Figure 1A and Table 1).
3.2. Tree seedling growth
Warming did not significantly affect total seedling growth for any of the three tree seedling species, although there was a positive trend (p < 0.1) for B. pubescens (Table 2). Interestingly, despite its strong effect on survival, moss removal did not have a significant effect on growth of the surviving seedlings in any of the species (Table 2).
Shrub removal increased growth for P. abies and P. sylvestris and there was a weak positive trend (p < 0.1) for B. pubescens as well (Figures 2A–C and Table 2). Furthermore, growth of P. sylvestris seedlings exhibited weaker growth in intermediate and old successional sites compared to young sites, which effect seemed (p < 0.1) primarily to occur in combination with moss removal and warming (Figure 2B and Table 2). However, growth of B. pubescens and P. abies seedlings was not affected by post-fire successional stage (Table 2). There were also no significant interactive effects among factors for any of the three species (Table 2).
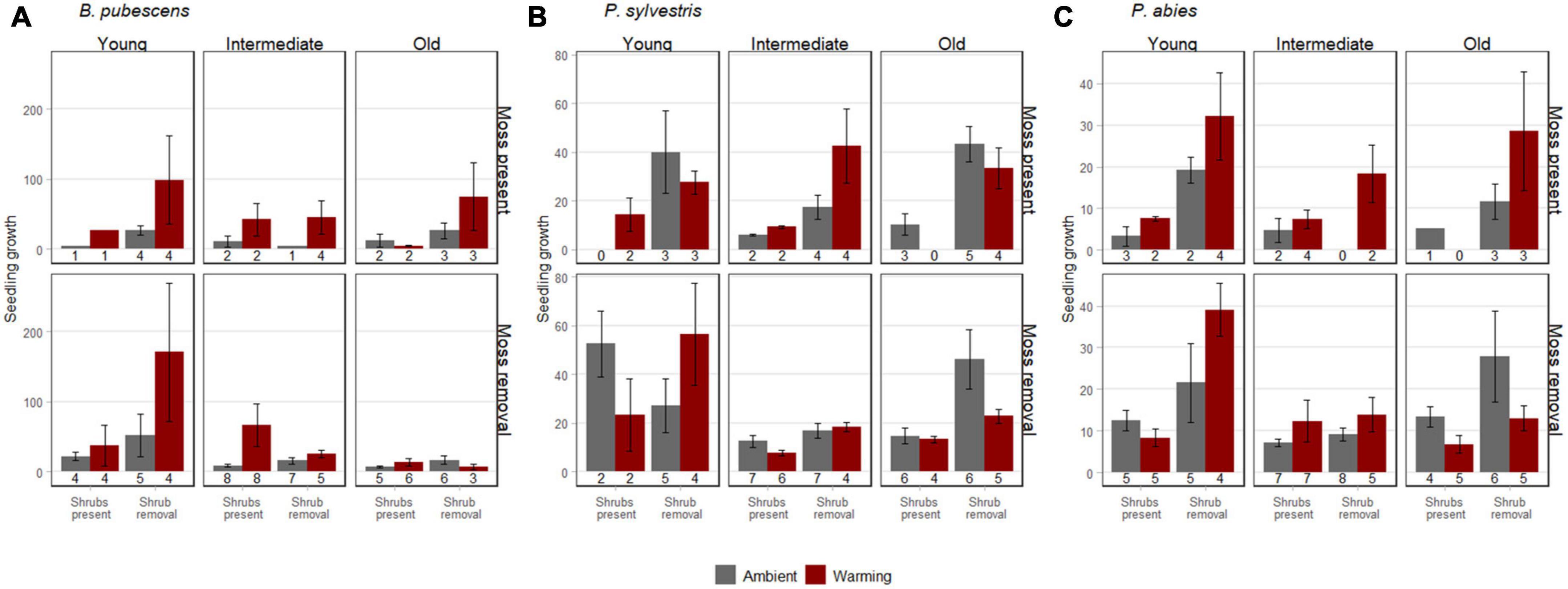
Figure 2. Growth (± SE) of B. pubescens seedlings (A), P. sylvestris seedlings (B), and P. abies seedlings (C) at the point of harvest in 2016. The numbers below the histogram bars represent the number of replicates with surviving seedlings (maximum number of replicates possible is 8 including first and second seedlings). The seedlings were harvested in warmed (red) and ambient (gray) conditions, with shrubs present or with shrubs removed, and with mosses present (upper panel) or with mosses removed (lower panel) along a post-fire chronosequence with young (<100 years since fire), intermediate (100–260 years since fire), and old (>100 years since fire) sites. Seedling growth was defined as total biomass at the point of harvest after 3 years, relative to biomass shortly after transplantation.
4. Discussion
Warming of air and soil temperature, induced by the OTCs, during the vegetation period had no significant effects on the survival and growth of B. pubescens, P. sylvestris, or P. abies seedlings in an unmanaged boreal forest system that includes different successional stages. Instead, for all three tree species, the overall seedling survival benefited from absence of mosses, and this effect was sometimes reduced by shrub removal or mediated by post-fire successional stage depending on the species. In contrast, seedling growth was not affected by absence of mosses for any of the tree species, but instead primarily promoted by shrub removal for P. sylvestris and P. abies. Taken together, our results highlight the importance of understory vegetation and fire history for tree seedling establishment, and show that seedling survival and growth can be affected by different understory functional groups regardless of temperatures.
4.1. Lack of seedling response to warming
In contrast to the prediction of our first hypothesis, experimental warming of air and soil temperature did not significantly increase seedling survival or growth. This is consistent with the results of Marty et al. (2020) who found no effect of experimental warming on seedling growth, but is in contrast to Nissinen et al. (2020) and Okano et al. (2021) who observed a positive effect on growth, and Wright et al. (2018) who found a negative effect on survival. These divergent responses can origin from different magnitudes of warming in different experiments, ranging from 0.4°C in our experiment, over 2°C in the experiment of Marty et al. (2020) to 3.4°C in the experiment of Wright et al. (2018). Boreal forests are expected to experience a 1.5–4°C increase of temperature (Gauthier et al., 2015; IPCC, 2021) suggesting that the magnitude of warming in our study was comparatively small and therefore may not have triggered a seedling response. It is also possible that other essential factors such as nutrient, water and light availability could act as limiting factor for seedling responses to warming (Luo et al., 2019). We observed weak trends of positive warming responses on B. pubescens survival and growth and P. sylvestris growth that were limited to the young sites. This may be related to higher nutrient availability, because an earlier study conducted in the same experiment measured higher ammonium amounts in warmed and young successional stage plots (De Long et al., 2015). Besides nutrient availability, light availability, soil moisture, and/or surrounding vegetation might be other factors more strongly affecting seedling performance than warming (Lett and Dorrepaal, 2018) especially when they are a limiting factor and not alleviated by warming.
4.2. Seedling performance and understory functional groups
We did not find support for the second part of our first hypothesis that warming responses in seedlings would depend on the presence of mosses and shrubs. Still, the main and some interactive effects of moss and/or shrub removals strongly impacted seedling performance. Interestingly, survival and growth were strongest affected by different understory functional groups: seedling survival of all three species responded positively to moss removal and seedling growth of P. sylvestris and P. abies responded positively to shrub removal. This may indicate different mechanisms for seedling survival and growth, as well as different modes of action for mosses and shrubs, such as facilitation or competition. Earlier findings have shown that the presence of mosses can be a great barrier for seedling germination and survival in the boreal forest (Valkonen and Maguire, 2005; Hypponen et al., 2013; Kokkonen et al., 2018) and relate that to changes in moisture (Lindo and Gonzalez, 2010; Soudzilovskaia et al., 2013) and competition for space as mosses can also suffocate tree seedlings (Stuiver et al., 2014). Shrubs have also been shown to suppress seedling growth (Wardle et al., 2008), e.g., E. hermaphroditum is known to produce poor quality litter with allelopathic compounds hampering seedling establishment (Nilsson and Zackrisson, 1992). This assumption is supported by positive effects of shrub removal on P. sylvestris root and shoot biomass and P. abies shoot biomass (Supplementary Table 3). Furthermore, interspecific competition, for nutrients and light, could be another mechanism, explaining why shrubs have a negative effect on seedling growth because established shrubs have a better developed root system and higher photosynthetic biomass than young tree seedlings (Kuuluvainen and Rouvinen, 2000). Seedling survival might thus be governed by competition for water and space while seedling growth might be more affected by competition for light and nutrients, overall highlighting the importance of different understory functional groups and their influences on resource availability as drivers of tree seedling performance.
We also found species-specific effects of shrub removal on seedling survival. B. pubescens seedlings generally survived better with shrubs, while P. sylvestris seedlings survived better without them. This difference in response between these two species could be due to a trade-off between avoiding competition and being eaten. Notably, B. pubescens seedlings are more palatable for small mammalian herbivores than conifer seedlings (Lyly et al., 2014) and potentially benefit from being surrounded by shrubs of low palatability as this will lower their accessibility to herbivores and thus reduce their mortality (Callaway et al., 2005; Barbosa et al., 2009; Jensen et al., 2012). This can also explain why the positive effect of moss removal on B. pubescens survival was more pronounced when shrubs were present than when they were absent. In addition, the presence of shrubs could have increased moisture in understory vegetation (Angell and Kielland, 2009), promoting slug herbivory particularly targeting P. sylvestris seedlings (Nystrand and Granstrom, 1997). Overall, this further implies the importance of understory functional groups for forest regeneration in terms of seedling survival and illustrates potential facilitating effects which are, however, species-specific.
4.3. Seedling performance, understory functional groups, and fire history
We also found no support for our second hypothesis that the effect of warming on seedling survival and growth, and how this is governed by understory functional groups, is mediated by the post-fire successional stage. However, we found stronger positive effects of moss removal on seedling survival in late compared to early post-fire successional stages for B. pubescens and P. sylvestris. Old post-fire sites in our chronosequence have higher moss biomass than do intermediate and young sites (DeLuca T. et al., 2002; Jackson et al., 2013), therefore the removal of mosses likely relaxed the competition from mosses for space and resources more in the older sites (Nilsson and Wardle, 2005). Furthermore, B. pubescens survival also benefitted from the combined effect of moss removal and shrub presence in old post-fire successional stages. These older successional stages support a higher biomass of E. hermaphroditum relative to that of V. myrtillus (DeLuca T. et al., 2002; Jackson et al., 2013; De Long et al., 2015), and it could therefore be expected that seedling survival in these older forests would be impaired because of the ability of E. hermaphroditum to produce phytotoxic allelochemicals (Nilsson et al., 2000). However, as the effects of shrub presence on B. pubescens seedlings was positive and not negative, a different mechanism must have been involved. For example, a high abundance of E. hermaphroditum, which is a species of low nutritional quality, might have protected B. pubescens seedlings from consumption by small mammalian herbivores like hare, vole and/or lemmings (Callaway et al., 2005; Barbosa et al., 2009; Jensen et al., 2012; Lyly et al., 2014).
4.4. Implications for future forests
In general, our results show that understory functional groups are stronger drivers of tree seedling performance than direct effects of experimentally generated warmer temperatures ranging at the lower end of other experimental warming studies (Rustad et al., 2001). However, warmer temperatures at the level expected in boreal forests (1.5–4°C) and an increase in precipitation (Gauthier et al., 2015; IPCC, 2021) can also affect the cover and abundance of understory functional groups (Hedwall et al., 2015, 2019) and thus indirectly influence seedling responses. Overall, climate change is expected to decrease the cover of shrubs (Hedwall et al., 2015; Jonsson et al., 2021), while climate change effects on moss cover are less clear; potentially leading to an increase (Jonsson et al., 2021), but also a reduction (Alatalo et al., 2020). A decrease in shrub cover in future forests may in particular favor P. abies and P. sylvestris regeneration, because these species profited from shrub removal in terms of growth and P. sylvestris additionally benefitted from shrub removal in terms of survival.
In addition, climate change is also expected to promote fire events due to warmer temperatures (de Groot et al., 2013; Flannigan et al., 2013), resulting in post fire understory vegetation change following successional trajectories (Nilsson and Wardle, 2005). However, fire suppression (Pinto et al., 2020) and likely increasing precipitation in the boreal forests of northern Europe might also dampen the risk of forest fires (Krikken et al., 2021), leading to a higher number of old forest sites (>260 years since fire disturbance). In our study, time since last fire disturbance modified B. pubescens and P. sylvestris responses to moss and shrub removal such that performance was always worse at old sites. However, this effect was clearly weaker than the effect of functional group removal leading to the conclusion potential climate change effects on future forest regeneration may primarily act through changes in understory functional groups.
Data availability statement
Publicly available datasets were analyzed in this study. This data can be found here: https://doi.org/10.6084/m9.figshare.22717678.v1.
Author contributions
ED, M-CN, and DW designed the study. ED, EK, and LT performed the seedling planting and counting. ED and M-TJ performed the seedling harvest. M-TJ analyzed the data with support from SL. M-TJ wrote the manuscript with contributions from all other authors. All authors contributed to the article and approved the submitted version.
Funding
This study was funded by Kempe Foundation (JCK-1112), Swedish Research Council VR (Dnr 621-2011-5444), and Knut and Alice Wallenberg Foundation (Wallenberg Academy Fellowship KAW 2012.0152) to ED.
Acknowledgments
We thank Katharina Krumpholz, Johannes Moeser, and Luise Pabst for valuable help in the field and lab and Adam Clark for helpful discussions on the statistics. We also thank the Swedish Polar Research Secretariat and SITES for support, accommodation and laboratory facilities at Abisko Scientific Research Station.
Conflict of interest
The authors declare that the research was conducted in the absence of any commercial or financial relationships that could be construed as a potential conflict of interest.
Publisher’s note
All claims expressed in this article are solely those of the authors and do not necessarily represent those of their affiliated organizations, or those of the publisher, the editors and the reviewers. Any product that may be evaluated in this article, or claim that may be made by its manufacturer, is not guaranteed or endorsed by the publisher.
Supplementary material
The Supplementary Material for this article can be found online at: https://www.frontiersin.org/articles/10.3389/ffgc.2023.1130532/full#supplementary-material
References
Alatalo, J. M., Jagerbrand, A. K., Erfanian, M. B., Chen, S., Sun, S. Q., and Molau, U. (2020). Bryophyte cover and richness decline after 18 years of experimental warming in alpine sweden. AoB Plants 12:laa061. doi: 10.1093/aobpla/plaa061
Angell, A. C., and Kielland, K. (2009). Establishment and growth of white spruce on a boreal forest floodplain: interactions between microclimate and mammalian herbivory. For. Ecol. Manag. 258, 2475–2480. doi: 10.1016/j.foreco.2009.08.030
Barbosa, P., Hines, J., Kaplan, I., Martinson, H., Szczepaniec, A., and Szendrei, Z. (2009). Associational resistance and associational susceptibility: having right or wrong neighbors. Annu. Rev. Ecol. Evol. Syst. 40, 1–20. doi: 10.1146/annurev.ecolsys.110308.120242
Bates, D., Maechler, M., Bolker, B., and Walker, S. (2015). Fitting linear mixed-effects models using lme4. J. Stat. Softw. 67, 1–48. doi: 10.18637/jss.v067.i01
Blok, D., Heijmans, M. M. P. D., Schaepman-Strub, G., van Ruijven, J., Parmentier, F. J. W., Maximov, T. C., et al. (2011). The cooling capacity of mosses: controls on water and energy fluxes in a siberian tundra site. Ecosystem 14, 1055–1065. doi: 10.1007/s10021-011-9463-5
Bradshaw, R. H. W. (1993). Tree species dynamics and disturbance in three swedish boreal forest stands during the last two thousand years. J. Veg. Sci. 4, 759–764. doi: 10.2307/3235612
Callaway, R., Brooker, R. W., Choler, P., Kikvidze, Z., Lortie, C. J., Michalet, R., et al. (2002). Positive interactions among alpine plants increase with stress. Nature 417, 844–848. doi: 10.1038/nature00812
Callaway, R. M., Kikodze, D., Chiboshvili, M., and Khetsuriani, L. (2005). Unpalatable plants protect neighbors from grazing and increase plant community diversity. Ecology 86, 1856–1862. doi: 10.1890/04-0784
Danby, R. K., and Hik, D. S. (2007). Responses of white spruce (picea glauca) to experimental warming at a subarctic alpine treeline. Glob. Change Biol. 13, 437–451. doi: 10.1111/j.1365-2486.2006.01302.x
Davis, K. T., Higuera, P. E., Sala, A., and Fox, C. (2018). Anticipating fire-mediated impacts of climate change using a demographic framework. Funct. Ecol. 32, 1729–1745. doi: 10.1111/1365-2435.13132
Day, M. E., Schedlbauer, J. L., Livingston, W. H., Greenwood, M. S., White, A. S., and Brissette, J. C. (2005). Influence of seedbed, light environment, and elevated night temperature on growth and carbon allocation in pitch pine (pinus rigida) and jack pine (pinus banksiana) seedlings. For. Ecol. Manag. 205, 59–71. doi: 10.1016/j.foreco.2004.10.004
De Frenne, P., De Schrijver, A., Graae, B. J., Gruwez, R., Tack, W., Vandelook, F., et al. (2009). The use of open-top chambers in forests for evaluating warming effects on herbaceous understorey plants. Ecol. Res. 25, 163–171. doi: 10.1007/s11284-009-0640-3
de Groot, W. J., Flannigan, M. D., and Cantin, A. S. (2013). Climate change impacts on future boreal fire regimes. For. Ecol. Manag. 294, 35–44. doi: 10.1016/j.foreco.2012.09.027
De Long, J. R., Dorrepaal, E., Kardol, P., Nilsson, M.-C., Teuber, L. M., and Wardle, D. A. (2015). Contrasting responses of soil microbial and nematode communities to warming and plant functional group removal across a post-fire boreal forest successional gradient. Ecosystem 19, 339–355. doi: 10.1007/s10021-015-9935-0
DeLuca, T., Nilsson, M. C., and Zackrisson, O. (2002). Nitrogen mineralization and phenol accumulation along a fire chronosequence in northern Sweden. Oecologia 133, 206–214. doi: 10.1007/s00442-002-1025-2
DeLuca, T. H., Zackrisson, O., Nilsson, M. C., and Sellsted, A. (2002). Quantifying nitrogen-fixation in feather moss carpets of boreal forests. Nature 419, 917–920. doi: 10.1038/nature01051
Elumeeva, T. G., Soudzilovskaia, N. A., During, H. J., and Cornelissen, J. H. C. (2011). The importance of colony structure versus shoot morphology for the water balance of 22 subarctic bryophyte species. J. Veg. Sci. 22, 152–164. doi: 10.1111/j.1654-1103.2010.01237.x
Flannigan, M., Cantin, A. S., de Groot, W. J., Wotton, M., Newbery, A., and Gowman, L. M. (2013). Global wildland fire season severity in the 21st century. For. Ecol. Manag. 294, 54–61. doi: 10.1016/j.foreco.2012.10.022
Gauthier, S., Bernier, P., Kuuluvainen, T., Shvidenko, A. Z., and Schepaschenko, D. G. (2015). Boreal forest health and global change. Science 349, 819–822. doi: 10.1126/science.aaa9092
Gomez-Aparicio, L., Zamora, R., Castro, J., and Hodar, J. A. (2008). Facilitation of tree saplings by nurse plants: microhabitat amelioration or protection against herbivores? J. Veg. Sci. 19, 161–172. doi: 10.3170/2008-8-18347
Hansen, W. D., and Turner, M. G. (2019). Origins of abrupt change? postfire subalpine conifer regeneration declines nonlinearly with warming and drying. Ecol. Monogr. 89:e01340. doi: 10.1002/ecm.1340
Hedwall, P. O., Gustafsson, L., Brunet, J., Lindbladh, M., Axelsson, A. L., and Strengbom, J. (2019). Half a century of multiple anthropogenic stressors has altered northern forest understory plant communities. Ecol. Appl. 29:e01874. doi: 10.1002/eap.1874
Hedwall, P. O., Skoglund, J., and Linder, S. (2015). Interactions with successional stage and nutrient status determines the life-form-specific effects of increased soil temperature on boreal forest floor vegetation. Ecol. Evol. 5, 948–960. doi: 10.1002/ece3.1412
Henry, G. H. R., and Molau, U. (1997). Tundra plants and climate change: the international tundra experiment (itex). Glob. Chang. Biol. 3, 1–9. doi: 10.1111/j.1365-2486.1997.gcb132.x
Hypponen, M., Hallikainen, V., Niemela, J., and Rautio, P. (2013). The contradictory role of understory vegetation on the success of scots pine regeneration. Silva Fennica 47, 1–19. doi: 10.14214/sf.903
IPCC (2021). Climate Change 2021: the Physical Science Basis. Contribution of Working Group i to the Sixth Assessment Report of the Intergovernmental Panel on Climate Change, eds V. Masson-Delmotte, P. Zhai, A. Pirani, S. L. Connors, C. Péan, S. Berger, et al. (Geneva: IPCC).
Jackson, B. G., Nilsson, M.-C., and Wardle, D. A. (2013). The effects of the moss layer on the decomposition of intercepted vascular plant litter across a post-fire boreal forest chronosequence. Plant Soil 367, 199–214. doi: 10.1007/s11104-012-1549-0
Jensen, A. M., Götmark, F., and Löf, M. (2012). Shrubs protect oak seedlings against ungulate browsing in temperate broadleaved forests of conservation interest: a field experiment. For. Ecol. Manag. 266, 187–193. doi: 10.1016/j.foreco.2011.11.022
Johnstone, J. F., Hollingsworth, T. N., Chapin, F. S., and Mack, M. C. (2010). Changes in fire regime break the legacy lock on successional trajectories in alaskan boreal forest. Glob. Change Biol. 16, 1281–1295. doi: 10.1111/j.1365-2486.2009.02051.x
Jonsson, B. G., Dahlgren, J., Ekström, M., Esseen, P.-A., Grafström, A., Ståhl, G., et al. (2021). Rapid changes in ground vegetation of mature boreal forests—an analysis of swedish national forest inventory data. Forests 12:475. doi: 10.3390/f12040475
Kaarlejärvi, E., Baxter, R., Hofgaard, A., Hytteborn, H., Khitun, O., Molau, U., et al. (2012). Effects of warming on shrub abundance and chemistry drive ecosystem-level changes in a forest–tundra ecotone. Ecosystem 15, 1219–1233. doi: 10.1007/s10021-012-9580-9
Kellomaki, S., and Wang, K. Y. (2001). Growth and resource use of birch seedlings under elevated carbon dioxide and temperature. Ann. Bot. 87, 669–682. doi: 10.1006/anbo.2001.1393
Kokkonen, N. A., Macdonald, S. E., Curran, I., Landhäusser, S. M., and Lieffers, V. J. (2018). Effects of substrate availability and competing vegetation on natural regeneration of white spruce on logged boreal mixedwood sites. Can. J. For. Res. 48, 324–332. doi: 10.1139/cjfr-2017-0307
Körner, C., and Paulsen, J. (2004). A world-wide study of high altitude treeline temperatures. J. Biogeogr. 31, 713–732. doi: 10.1111/j.1365-2699.2003.01043.x
Krikken, F., Lehner, F., Haustein, K., Drobyshev, I., and van Oldenborgh, G. J. (2021). Attribution of the role of climate change in the forest fires in sweden 2018. Nat. Hazards Earth Syst. Sci. 21, 2169–2179. doi: 10.5194/nhess-21-2169-2021
Kuuluvainen, T., and Aakala, T. (2011). Natural forest dynamics in boreal fennoscandia: a review and classification. Silva Fenn. 45, 823–841. doi: 10.14214/sf.73
Kuuluvainen, T., and Rouvinen, S. (2000). Post-fire understorey regeneration in boreal pinus sylvestris forest sites with different fire histories. J. Veg. Sci. 11, 801–812. doi: 10.2307/3236550
Lathi, M., Aphalo, P. J., Finér, L., Ryyppö, A., Lehto, T., and Mannerkoski, H. (2005). Effects of soil temperature on shoot and root growth and nutrient uptake of 5-year-old norway spruce seedlings. Tree Physiol. 25, 115–122. doi: 10.1093/treephys/25.1.115
Lett, S., and Dorrepaal, E. (2018). Global drivers of tree seedling establishment at alpine treelines in a changing climate. Funct. Ecol. 32, 1666–1680. doi: 10.1111/1365-2435.13137
Lett, S., Nilsson, M.-C., Wardle, D. A., Dorrepaal, E., and Canham, C. (2017). Bryophyte traits explain climate-warming effects on tree seedling establishment. J. Ecol. 105, 496–506. doi: 10.1111/1365-2745.12688
Lett, S., Teuber, L. M., Krab, E. J., Michelsen, A., Olofsson, J., Nilsson, M. C., et al. (2020). Mosses modify effects of warmer and wetter conditions on tree seedlings at the alpine treeline. Glob. Change Biol. 26, 5754–5766. doi: 10.1111/gcb.15256
Lindo, Z., and Gonzalez, A. (2010). The bryosphere: an integral and influential component of the earth’s biosphere. Ecosystem 13, 612–627. doi: 10.1007/s10021-010-9336-3
Luo, Y., McIntire, E. J. B., Boisvenue, C., Nikiema, P. P., Chen, H. Y. H., and Bellingham, P. (2019). Climatic change only stimulated growth for trees under weak competition in central boreal forests. J. Ecol. 108, 36–46. doi: 10.1111/1365-2745.13228
Lyly, M., Klemola, T., Koivisto, E., Huitu, O., Oksanen, L., and Korpimaki, E. (2014). Varying impacts of cervid, hare and vole browsing on growth and survival of boreal tree seedlings. Oecologia 174, 271–281. doi: 10.1007/s00442-013-2761-1
Mallik, A. U. (2003). Conifer regeneration problems in boreal and temperate forests with ericaceous understory: role of disturbance, seedbed limitation, and keytsone species change. Crit. Rev. Plant Sci. 22, 341–366. doi: 10.1080/713610860
Marion, G. M., Henry, G. H. R., Freckman, D. W., Johnstone, J., Jones, G., Jones, M. H., et al. (1997). Open-top designs for manipulating field temperature in high-latitude ecosystems. Glob. Change Biol. 3, 20–32. doi: 10.1111/j.1365-2486.1997.gcb136.x
Marty, C., Piquette, J., Dussault-Chouinard, É, Morin, H., Thiffault, N., Houle, D., et al. (2020). Canopy nitrogen addition and soil warming affect conifer seedlings’ phenology but have limited impact on growth and soil n mineralization in boreal forests of eastern canada. Front. For. Glob. Change 3:581363. doi: 10.3389/ffgc.2020.581363
McDowell, N. G., Allen, C. D., Anderson-Teixeira, K., Aukema, B. H., Bond-Lamberty, B., and Chini, L. (2020). Pervasive shifts in forest dynamics in a changing world. Science 368:eaaz9463. doi: 10.1126/science.aaz9463
Mekonnen, Z. A., Riley, W. J., Randerson, J. T., Grant, R. F., and Rogers, B. M. (2019). Expansion of high-latitude deciduous forests driven by interactions between climate warming and fire. Nat. Plants 5, 952–958. doi: 10.1038/s41477-019-0495-8
Montgomery, R. A., Reich, P. B., and Palik, B. J. (2010). Untangling positive and negative biotic interactions: views from above and below ground in a forest ecosystem. Ecology 91, 3641–3655. doi: 10.1890/09-1663.1
Munier, A., Hermanutz, L., Jacobs, J. D., and Lewis, K. (2010). The interacting effects of temperature, ground disturbance, and herbivory on seedling establishment: Implications for treeline advance with climate warming. Plant Ecol. 210, 19–30. doi: 10.1007/s11258-010-9724-y
Nilsson, M. C., and Wardle, D. A. (2005). Understory vegetation as a forest ecosystem driver: evidence from the northern swedish boreal forest. Front. Ecol. Environ. 3, 421–428. doi: 10.1890/1540-9295(2005)003[0421:UVAAFE]2.0.CO;2
Nilsson, M. C., and Zackrisson, O. (1992). Inhibition of scots pine seedling establishment by Empetrum hermaphroditum. J. Chem. Ecol. 18, 1857–1870. doi: 10.1007/BF02751109
Nilsson, M. C., Zackrisson, O., Sterner, O., and Wallstedt, A. (2000). Characterisation of the differential interference effects of two boreal dwarf shrub species. Oecologia 123, 122–128. doi: 10.1007/s004420050997
Nissinen, K., Virjamo, V., Kilpeläinen, A., Ikonen, V.-P., Pikkarainen, L., Ärväs, I.-L., et al. (2020). Growth responses of boreal scots pine, norway spruce and silver birch seedlings to simulated climate warming over three growing seasons in a controlled field experiment. Forests 11:943. doi: 10.3390/f11090943
Nystrand, O., and Granstrom, A. (1997). Forest floor moisture controls predator activity on juvenile seedlings of pinus sylvestris. Can. J. Forest Res. 27, 1746–1752. doi: 10.1139/x97-148
Okano, K., and Bret-Harte, M. S. (2015). Warming and neighbor removal affect white spruce seedling growth differently above and below treeline. Springerplus 4:79. doi: 10.1186/s40064-015-0833-x
Okano, K., Bret-Harte, M. S., Mulder, C. P. H., and Juday, G. P. (2021). Resource availability drives plant–plant interactions of conifer seedlings across elevations under warming in alaska. Ecosphere 12:e03508. doi: 10.1002/ecs2.3508
Oleskog, G., and Sahlen, K. (2000). Effects of seedbed substrate on moisture conditions and germination of scots pine (pinus sylvestris) seeds in a mixed conifer stand. New For. 20, 119–133. doi: 10.1023/A:1006783900412
Pan, Y., Birdsey, R. A., Fang, J., Houghton, R., Kauppi, P. E., Kurz, W. A., et al. (2011). A large and persistent carbon sink in the world’s forests. Science 333, 988–993. doi: 10.1126/science.1201609
Pinto, G. A. S. J., Rousseu, F., Niklasson, M., and Drobyshev, I. (2020). Effects of human-related and biotic landscape features on the occurrence and size of modern forest fires in sweden. Agric. For. Meteorol. 291:108084. doi: 10.1016/j.agrformet.2020.108084
Previdi, M., Smith, K. L., and Polvani, L. M. (2021). Arctic amplification of climate change: a review of underlying mechanisms. Environ. Res. Lett. 16:093003. doi: 10.1088/1748-9326/ac1c29
Pumpanen, J., Heinonsalo, J., Rasilo, T., Villemot, J., and Ilvesniemi, H. (2012). The effects of soil and air temperature on co2 exchange and net biomass accumulation in norway spruce, scots pine and silver birch seedlings. Tree Physiol. 32, 724–736. doi: 10.1093/treephys/tps007
R Core Team (2020). R: a Language and Environment for Statistical Computing. 4.0.2 ed. Vienna: R Foundation for Statistical Computing.
Rustad, L., Campbell, J., Marion, G., Norby, R., Mitchell, M., Hartley, A., et al. (2001). A meta-analysis of the response of soil respiration, net nitrogen mineralization, and aboveground plant growth to experimental ecosystem warming. Oecologia 126, 543–562. doi: 10.1007/s004420000544
Ryan, M. G. (2013). Three decades of research at flakaliden advancing whole-tree physiology, forest ecosystem and global change research. Tree Physiol. 33, 1123–1131. doi: 10.1093/treephys/tpt100
Saxe, H., Canell, M. G. R., Johnsen, O., Ryan, M. G., and Vourlitis, G. (2000). Tree and forest functioning in response to global warming. New Phytol. 149, 369–399. doi: 10.1046/j.1469-8137.2001.00057.x
Sorensen, P. L., Lett, S., and Michelsen, A. (2012). Moss-specific changes in nitrogen fixation following two decades of warming, shading, and fertilizer addition. Plant Ecol. 213, 695–706. doi: 10.1007/s11258-012-0034-4
Soudzilovskaia, N. A., Graae, B. J., Douma, J. C., Grau, O., Milbau, A., Shevtsova, A., et al. (2011). How do bryophytes govern generative recruitment of vascular plants? New Phytol. 190, 1019–1031. doi: 10.1111/j.1469-8137.2011.03644.x
Soudzilovskaia, N. A., van Bodegom, P. M., Cornelissen, J. H. C., and Schweitzer, J. (2013). Dominant bryophyte control over high-latitude soil temperature fluctuations predicted by heat transfer traits, field moisture regime and laws of thermal insulation. Funct. Ecol. 27, 1442–1454. doi: 10.1111/1365-2435.12127
Stuiver, B. M., Wardle, D. A., Gundale, M. J., and Nilsson, M. C. (2014). The impact of moss species and biomass on the growth of pinus sylvestris tree seedlings at different precipitation frequencies. Forests 5, 1931–1951. doi: 10.3390/f5081931
Therneau, T. M. (2020). Coxme: Mixed Effects Cox Models. 2.2-16 ed. Available online at: https://CRAN.R-project.org/package=coxme (accessed September 23, 2022).
Thrippleton, T., Bugmann, H., Kramer-Priewasser, K., and Snell, R. S. (2016). Herbaceous understorey: an overlooked player in forest landscape dynamics? Ecosystem 19, 1240–1254. doi: 10.1007/s10021-016-9999-5
Turetsky, M. R. (2003). The role of bryophytes in carbon and nitrogen cycling. Bryologist 106, 395–409. doi: 10.1639/05
Turetsky, M. R., Mack, M. C., Hollingsworth, T. N., and Harden, J. W. (2010). The role of mosses in ecosystem succession and function in alaska’s boreal forest. Can. J. Forest Res. 40, 1237–1264. doi: 10.1139/X10-072
Valkonen, S., and Maguire, D. A. (2005). Relationship between seedbed properties and the emergence of spruce germinants in recently cut norway spruce selection stands in southern finland. For. Ecol. Manag. 210, 255–266. doi: 10.1016/j.foreco.2005.02.039
Walck, J. L., Hidayati, S. N., Dixon, K. W., Thompson, K. E. N., and Poschlod, P. (2011). Climate change and plant regeneration from seed. Glob. Chang. Biol. 17, 2145–2161. doi: 10.1111/j.1365-2486.2010.02368.x
Walker, X. J., Baltzer, J. L., Cumming, S. G., Day, N. J., Ebert, C., Goetz, S., et al. (2019). Increasing wildfires threaten historic carbon sink of boreal forest soils. Nature 572, 520–523. doi: 10.1038/s41586-019-1474-y
Wardle, D. A., Lagerström, A., and Nilsson, M.-C. (2008). Context dependent effects of plant species and functional group loss on vegetation invasibility across an island area gradient. J. Ecol. 96, 1174–1186. doi: 10.1111/j.1365-2745.2008.01437.x
Wardle, D. A., and Zackrisson, O. (2005). Effects of species and functional group loss on island ecosystem properties. Nature 435, 806–810. doi: 10.1038/nature03611
Wheeler, J. A., Hermanutz, L., and Marino, P. M. (2011). Feathermoss seedbeds facilitate black spruce seedling recruitment in the forest-tundra ecotone (labrador, canada). Oikos 120, 1263–1271. doi: 10.1111/j.1600-0706.2010.18966.x
Wickham, H. (2007). Reshaping data with the reshape package. J. Stat. Softw. 21:78. doi: 10.18637/jss.v021.i12
Wickham, H. (2016). Ggplot2: Elegant Graphics for Data Analyis. New York: Springer-Verlag. doi: 10.1007/978-3-319-24277-4
Wickham, H., Averick, M., Bryan, J., Chang, W., McGowan, L., and François, R. (2019). Welcome to the tidyverse. J. Open source Softw. 4:1686. doi: 10.21105/joss.01686
Wright, A. J., Fisichelli, N. A., Buschena, C., Rice, K., Rich, R., Stefanski, A., et al. (2018). Biodiversity bottleneck: Seedling establishment under changing climatic conditions at the boreal–temperate ecotone. Plant Ecol. 219, 691–704. doi: 10.1007/s11258-018-0827-1
Xu, Z., Yin, H., Xiong, P., Wan, C., and Liu, Q. (2012). Short-term responses of picea asperata seedlings of different ages grown in two contrasting forest ecosystems to experimental warming. Environ. Exp. Bot. 77, 1–11. doi: 10.1016/j.envexpbot.2011.10.011
Zackrisson, O., Dahlberg, A., Norberg, G., Nilsson, M.-C., and Jäderlund, A. (1998). Experiments on the effects of water availability and exclusion of fungal hyphae on nutrient uptake and establishment of pinus sylvestris seedlings in carpets of the moss pleurozium schreberi. Ecoscience 5, 77–85. doi: 10.1080/11956860.1998.11682444
Keywords: climate change, forest fire, moss, plant functional group removal, shrubs, survival, forest regeneration
Citation: Jessen M-T, Krab EJ, Lett S, Nilsson M-C, Teuber L, Wardle DA and Dorrepaal E (2023) Understory functional groups and fire history but not experimental warming drive tree seedling performance in unmanaged boreal forests. Front. For. Glob. Change 6:1130532. doi: 10.3389/ffgc.2023.1130532
Received: 23 December 2022; Accepted: 24 April 2023;
Published: 11 May 2023.
Edited by:
Zuoqiang Yuan, Northwestern Polytechnical University, ChinaReviewed by:
Charles Marty, Université du Québec à Chicoutimi, CanadaMark Bloomberg, University of Canterbury, New Zealand
Copyright © 2023 Jessen, Krab, Lett, Nilsson, Teuber, Wardle and Dorrepaal. This is an open-access article distributed under the terms of the Creative Commons Attribution License (CC BY). The use, distribution or reproduction in other forums is permitted, provided the original author(s) and the copyright owner(s) are credited and that the original publication in this journal is cited, in accordance with accepted academic practice. No use, distribution or reproduction is permitted which does not comply with these terms.
*Correspondence: Maria-Theresa Jessen, bWFyaWEtdGhlcmVzYS5qZXNzZW5AaWRpdi5kZQ==