- 1Laboratorio de Ecología Geográfica, Facultad de Ciencias, Universidad de Chile, Santiago, Chile
- 2Instituto de Ecología y Biodiversidad (IEB), Santiago, Chile
- 3Facultad de Ciencias Biológicas, Pontificia Universidad Católica de Chile, Santiago, Chile
- 4Projeto Origem, São João del Rei, Brazil
- 5Cape Horn Investigation Center (CHIC), Isla Navarino, Chile
Introduction: Biotic interactions are a crucial component of the plant regeneration process, which has been traditionally studied at more local scales, providing the tools for planning and decision-making. Studies showing the signature of species interactions at coarser spatial scales contrasting with animal-plant interactions at fine scales have been scarce. This study aimed at integrating an approach, over both biogeographic and local scales, by testing two endemic species of Mediterranean central Chile: the relict and southernmost threatened Chilean palm Jubaea chilensis (Chilean palm; Molina; Baillón) and the caviomorph scatter-hoarding rodent Octodon degus (Degu; Molina), on which this palm currently relies for seed dispersal.
Methods: Integrating Geographic Information Systems and Ecological Niche Modeling, the intensity of seed-rodent interactions from a territorial perspective was evaluated in the range of the palm, at a biogeographic scale, identifying areas with greater or lesser potential for seed-rodent interactions; and in local populations, incorporating a variety of environmental factors that might affect palm regeneration.
Results: The present results show that the rodent (Octodon degus) may play a role in Chilean palm (Jubaea chilensis) seed dispersal and seed establishment, since; Chilean palm regeneration is higher in areas where both species co-occur. At a local scale, a prominent overlap between palm seedlings and degu burrows was also found, which, allied with other abiotic variables such as altitude and topographic humidity, are crucial for successful palm regeneration.
Discussion: Understanding the full extent of animal-plant interactions and how they are affected by habitat perturbation in a wide range will provide essential information for the design of effective conservation and management strategies, such as rewilding based on plant species.
Introduction
Global change has caused drastic declines in species populations worldwide (Dirzo et al., 2014), impoverishing ecosystems and ecological interactions (Valiente-Banuet et al., 2015; Genes et al., 2017). The dispersal of plant seeds by frugivores is a key biotic interaction (BI), and the disruption of this mutualistic interaction can impede ecosystem functioning. This matter is especially important for large-seeded species that rely on animals for the dispersal of their seeds (Galetti et al., 2006; Kurten, 2013).
Frugivory is a seed dispersal process of plant-animal interaction that links the reproductive cycle of mature plants with the establishment of their offspring (Blanco et al., 2018) and with their animal dispersers through seed consumption, increasing the chance of seeds to reach safe sites for seedling survival (Schupp, 1993; Nathan and Muller-Landau, 2000; Muscarella and Fleming, 2007; Schupp et al., 2010). Plant-animal interactions are a key process in ecosystem conservation because they promote ecosystem functionality (Svenning et al., 2016); they also enhance ecosystem functions (Dirzo et al., 2014; Peres et al., 2016), trophic interactions, complement other approaches like habitat rewilding (Genes and Dirzo, 2022) and enhance corridor restoration in fragmented landscapes (Schooler et al., 2020) or reduce defaunation (Young et al., 2016).
Recent studies suggest that BI signatures can be expressed geographically (Dormann et al., 2018; Poggiato et al., 2021); however, empirical studies to test these assertions are scarce and deserve further analysis. Some attempts to consider BIs in a spatially explicit way include: (i) the use of correlation matrices to assess positive, neutral, or negative spatial patterns of co-occurrence (Carvajal et al., 2018; Poggiato et al., 2021); (ii) the use of surrogates of BIs such as productivity gradients (Pottier et al., 2013), and (iii) the linkage of dynamic thermodynamics equations obtained from macro-ecology with spatial grids (Violle et al., 2014). However, there is a need to establish more accessible and parsimonious methods, such as the use of Species Distribution Models (SDMs), which correlate large-scale abiotic factors (climate) with the realized presence points of species in a certain region, supporting ecological interactions for decision-makers who must make urgent decisions for conservation, reintroduction, and rewilding policies. SDMs provide a map showing suitable and unsuitable habitats in the geographic space (Guisan and Zimmermann, 2000; Guisan and Thuiller, 2005; Elith, 2016; Carvajal et al., 2018; Alaniz et al., 2020). However, the inclusion of BIs in SDMs is regarded as a real challenge both in theory and in practice because the change of scales does not necessarily imply that the interaction between species will be maintained (Araújo and Luoto, 2007; Heikkinen et al., 2007; Schweiger et al., 2008; Broennimann et al., 2012). Here, SDMs was employed to explore BIs at a broader spatial scale, intersecting the resulting models obtained from interacting species (see Lany et al., 2017; Alaniz et al., 2020) and thus capturing the geographic co-occurrence zones of the species and their potential spatial interaction.
Jubaea chilensis [Chilean palm, (Molina) Baillón; Arecaceae] is a monotypic palm endemic to Central Chile. It has been regarded as a biogeographic relict whose ancestor originated in Tertiary subtropical forests (Parsons, 2007; González et al., 2009). The current palm population is estimated to be only 2.5% of the original abundance of palms prior to the arrival of the Spaniards (∼5 million individuals), which is why nowadays this palm is considered as an endangered species (Michea, 1988; Cabello, 2006; González et al., 2017; CONAF, 2020). Long-distance seed dispersal is usually the most important mechanism in plant communities (Wills et al., 1997; Harms et al., 2000). The fruit of this palm is a drupe 4 cm in length with one spherical seed (the “coquito”) with a diameter of 2–2.5 cm. This large and fleshy-pulped fruit species of this Chilean palm is considered a paleo tropical fruit, which was originally dispersed by megafauna nowadays geographically extinct (mammals >103 kg; Guimarães et al., 2008). Extinct dispersers (camelids) have been replaced by abiotic factors (i.e., gravity, water, and runoff), by introduced horses and cattle that consume the fallen fruits pericarp (phase one), and are then secondarily dispersed by rodents, which act as substitute dispersers (phase two; Hunter, 1989; Vander Wall and Longland, 2004; Jansen et al., 2012).
Although seeds are consumed by livestock and horses, these animals do not ingest the Chilean palm seeds. They consume the fruit pericarp and regurgitate seeds on the surface of the soil. These seed are then prone to rapid desiccation or consumption by exotic rats, if they are not buried in the soil by the two Chilean food-hoarding endemic species of Central Chile, Octodon degus [Degu; (Molina)], and to a lesser extent, Spalacopus cyanus [Coururo; (Molina)] (Vander Wall and Longland, 2004; Quispe et al., 2009; González et al., 2017). Several tropical palm species have proved to be strongly dependent on scatter hoarding rodents, such as squirrels (Sciurus), spiny rats (Trinomys, Proechimys, Heteromys), acouchies (Myoprocta), and agoutis (Dasyprocta) (Vander Wall, 1990). The Degu rodents largely consume the Chilean palm seeds where the species coexist (Zunino et al., 1992; Yates et al., 1994); however, evidence for their potential role as secondary seed dispersers is still lacking.
Octodon degus is a seed predator that also acts as disperser because of their collection of non-consumed cached seeds may be “forgotten” and can subsequently germinate (i.e., safe sites; Harper, 1977), allowing the seeds to succeed to the seedling stage. The abundance of cached seeds is abundant in late summer when degu populations are in their peak of hoarding activities (Zunino et al., 1992).
In this study, the interactions between Octodon degus and Jubaea chilensis over two spatial scales at (a) biogeographic scale (∼1 km), using Species distribution models (SDMs), and (b) at a local, or site-scale (∼12 m) was examined. To this aim the effects of Octodon degus on Jubaea chilensis seedling density in conjunction with other local environmental factors, like topographic exposure, elevation, or vegetation cover, were assessed in several known natural populations of the palm, in locations where both species co-occur.
Materials and methods
Biogeographic scale analysis
To estimate the potential spatial distribution for Octodon degus and Jubaea chilensis, species distribution models (SDMs) based on the maximum entropy approach applied in MaxEnt version 3.4 (Phillips et al., 2006, 2017; Elith, 2016; Bosso et al., 2017) were used. These SDMs require information from two data sources: (i) species occurrence points and (ii) environmental variables. Our databases for both Jubaea chilensis and Octodon degus consisted of georeferenced records from the Global Biodiversity Information Facility (GBIF, 2018) and datasets from literature sources (Fulk, 1976; Fuentes et al., 1983; Meserve et al., 1993; Ebensperger and Bozinovic, 2000; Ebensperger and Wallem, 2002; Vasquez, 2002; Saavedra and Simonetti, 2003; Bozinovic et al., 2009; Díaz-Calderón, 2009; Pozo, 2009; Quispe et al., 2009; Medina, 2011; Correa et al., 2015; Davis et al., 2016; Miranda et al., 2016; Youlton et al., 2016). For environmental variables, the 19 bioclimatic variables obtained from Pliscoff et al. (2014) at the spatial resolution of one kilometer (Supplementary Table 1) were used.
We applied a distance filter (Brown et al., 2017) to identify and remove agglomerated and autocorrelated occurrence points, reducing their numbers from 217 to 141 for Octodon degus and 671 to 108 for Jubaea chilensis (Supplementary Table 2). Exploratory SDMs were performed for each species to find and assess spatial collinearity among environmental variables, employing all 19 bioclimatic variables, adding one replication model per ∼20 occurrence points (see Wisz et al., 2008; van Proosdij et al., 2016), for a total of nine and five replicates with k-fold cross-validation technique for Octodon degus and Jubaea chilensis models, respectively. In order to reduce the required number of variables for each model, Two statistics to assess model overfitting related to model complexity driven by excess of variables, Permutation Importance (PI) and Percent Contribution (PC) (Phillips et al., 2006) were used. In addition, the Shapiro–Wilk test to check normality (Royston, 1983, 1992) and Spearman correlation (Myers and Sirois, 2014) to test for correlation among bioclimatic variables were employed. These statistical analyses were performed for each species’ point-occurrence dataset.
The final SDMs for each species were built with the retained variables with low correlation (r < | 0.7|) and the highest PI and PC (retained variables, including correlation matrices available on S3). The rest of the model parameters used to configure SDMs were maintained from preliminary models (see Supplementary Tables 4A, B). The area under the receiver operating characteristic (ROC) curve (AUC) was applied to assess the accuracy (sensitivity and specificity) of SDMs (Phillips et al., 2006).
We generated the Potential Interaction Distribution Model (PIDM), employing the two previously generated SDMs. First, the continuous suitability raster grids into new rasters with discrete suitability values ranging from low to high (1 = low; 2 = medium; 3 = high), leaving out suitability values below percentile ten, which were classified as null (0 = null), were reclassified. Then the new rasters were multiplied pixel-wise in the GIS environment (sensu Alaniz et al., 2020), resulting in a single model for both species as a surrogate of the intensity of BIs, which ranged from 1 (no interaction) to 9 (maximum interaction).
To seek the biogeographic signature of species interactions, our PIDM model categories were contrasted with palm seedling densities measured in the field; we expected the highest seedling density values to correspond to zones with the highest values of PIDM (raster values). The statistical analysis was performed using a non-parametric Wilcox significance test in four study sites, where we assessed palm seedling density: Ocoa (32°58′ S–71° 5′ W; ID = 1), Cocalán (34°13′ S–71° 8′ W; ID = 2), Petorca (32° 9′ S–71°13′ W; ID = 7), and Hualañe (approx 34°56′ S–71°48′ W; ID = 12; Figure 1). These localities were grouped into two sampling groups by their PIDM-scores as follows: Ocoa + Cocalan = Very High; Petorca + Hualañe = Medium-High. These four localities encompass almost 90% of the entire species’ distribution range (González et al., 2009, 2017) (sampling procedure details in Table 1).
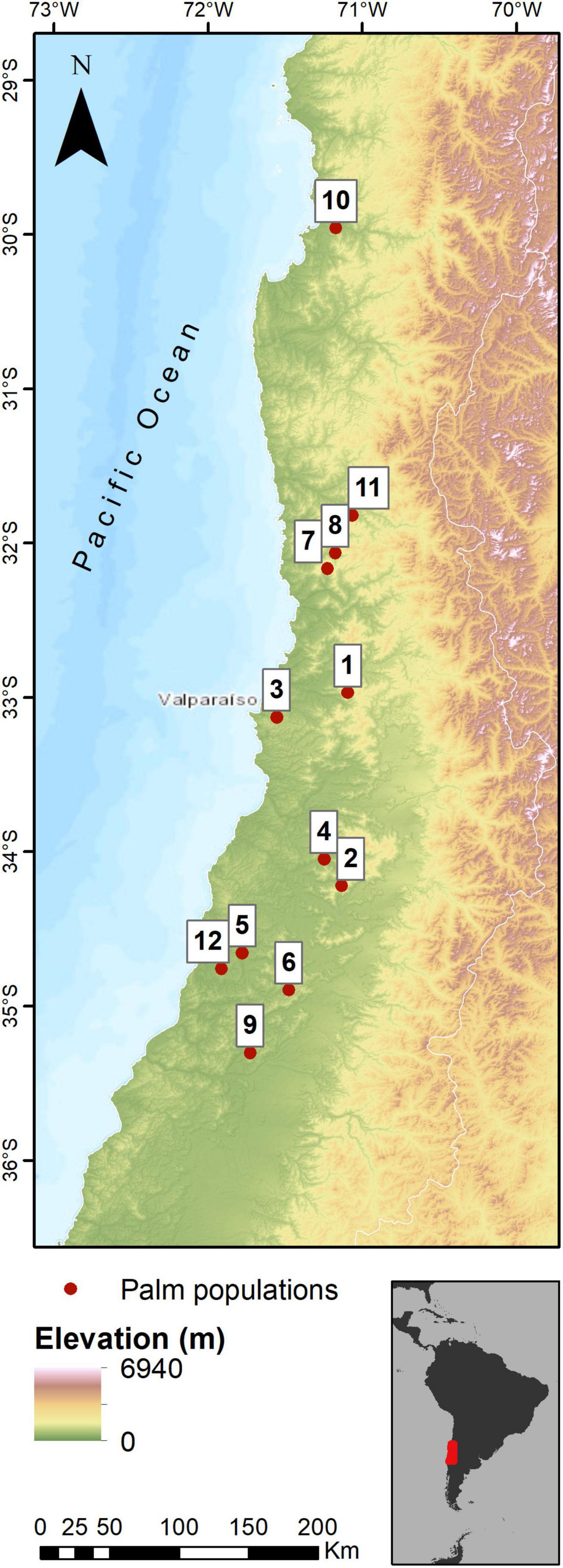
Figure 1. Natural palm populations identified by González et al. (2009). Population ID list is presented as follows: 1 Ocoa area; 2 Cocalán area; 3 Viña–valparaíso area; 4 Cuesta los guindos–alhué; 5 San miguel de las palmas; 6 La candelaria; 7 Túnel las palmas–petorca; 8 Tilama–pichidangui; 9 Tapihue–pencahue; 10 La serena; 11 Limahuida–los vilos; 12 Paredones–el asiento–talami–and others. Other study areas correspond to places in which natural populations of Jubaea chilensis have been identified, but in which the population size is so small (compared to other documented sites) that it does not allow the site to be identified as a population. The latter includes our “Hualañé” population. Please refer to Table 1 for more details on the populations chosen to perform analyzes at the local scale in this study.
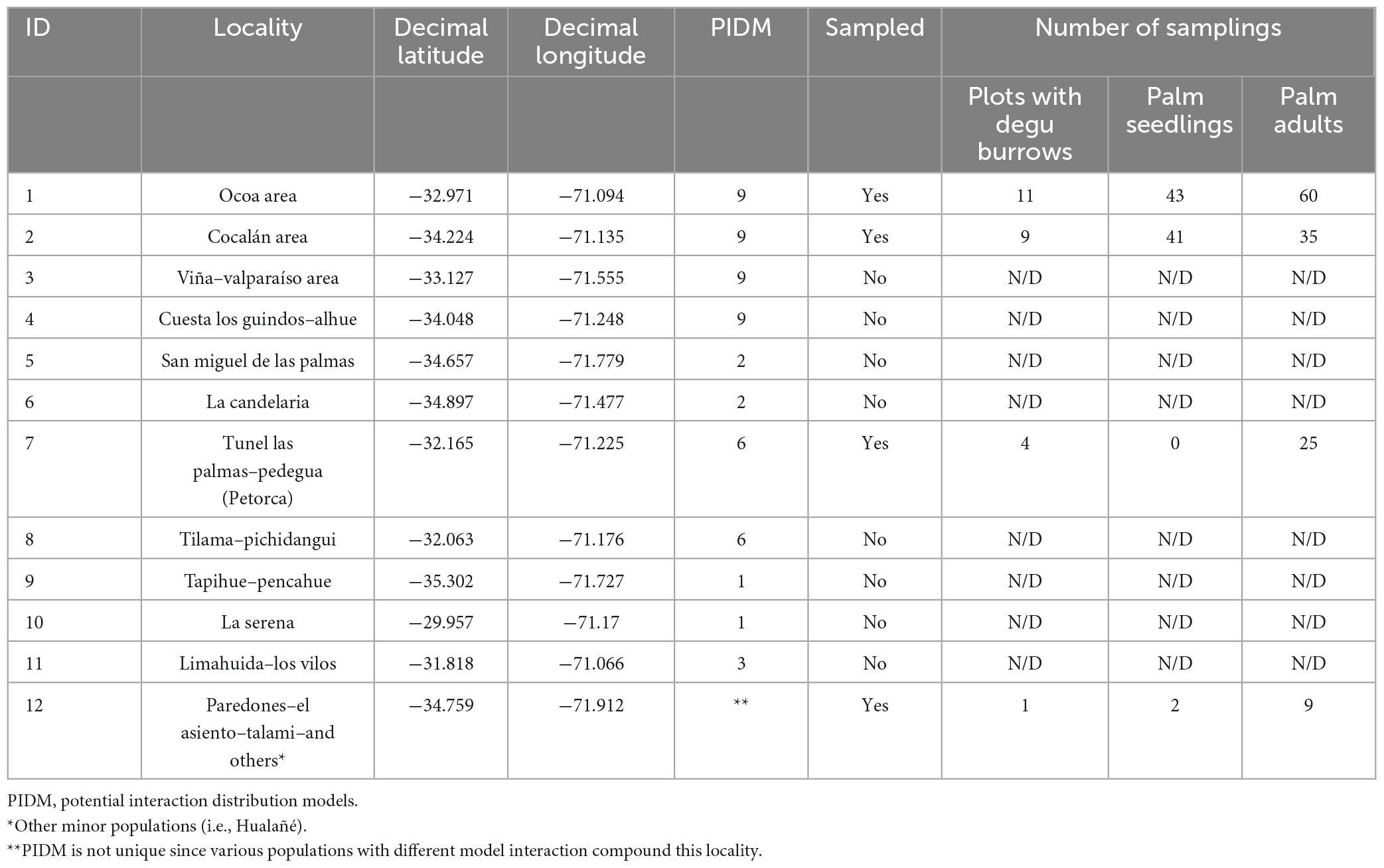
Table 1. Palm populations described in González et al. (2009) and palm–degu burrow sampling.
Local scale analysis
Local analysis was conducted in the same localities used to assess seedling density (Ocoa, Cocalán, Petorca, and Hualae; Figure 1). A proportional stratified random sampling design was adopted to establish fifteen sampling plots of 50 × 20 m per population, arranged 150 m from each other to assure spatial independence (Hurlbert, 1984). This distance exceeds more than ten times the normal range of aggregation observed in adult palm individuals (Miranda et al., 2016). Due to variation in palm density distribution over ecological gradients, sampling plots at (i) basin heads, (ii) water ravines, and (iii) bottom valleys were set up, with five plots per sampling location, totaling 1.5 ha in total for each locality.
All Jubaea chilensis seedlings, adult trees, and Octodon degus burrows in each sampling plot were recorded using a GPS. Burrows were considered suitable microhabitats for this rodent species. A degu burrow consists of an underground gallery that supports a family group of degus, which are aboveground connected with others by several runways (Vasquez et al., 2002; Ebensperger et al., 2004; Quispe et al., 2009). Pictures of burrows with the presence of eaten “coquitos” (picture in Figure 2), which had been hollowed out when eaten, were taken to discern between active and inactive burrows, these pictures were sent to specialists to ensure that burrows correspond to Degu and not to other fossorial species, such as the Coururo (Spalacopus cyanus), which coexists with Degu.
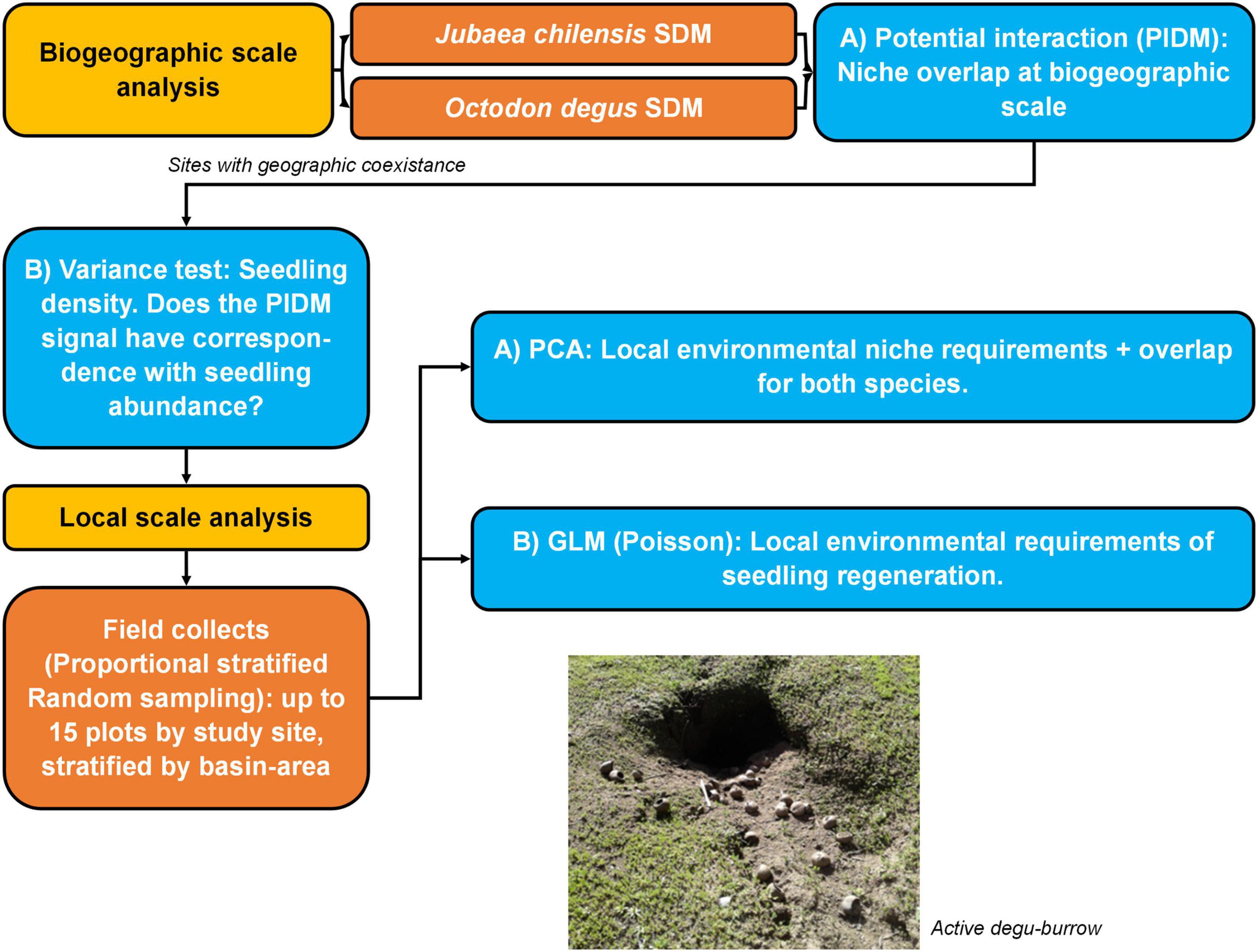
Figure 2. Methodology description. Boxes in yellow correspond to biogeographic and local scale analysis. Boxes in orange correspond to methodological procedures and boxes in blue correspond to statistical analyses. All samples were collected over the year 2017.
Principal Components Analysis (PCA) was performed to build both species local niches and their overlap based on local environmental requirements. The PCA analysis was made with the “ecospat” v3.3 R package (with the functions dudi.pca, suprow, ecospat.grid.clim.dyn and, ecospat.niche.overlap). The resulting overlaps were plotted employing the NiceOverPlot function (Fernández-López, 2017; Moreno-Contreras et al., 2020; Wan et al., 2020; Yap et al., 2022). The Schoener’s D test was used to measure niche overlap (function name = ecospat.plot.overlap.test), a metric that varies between 0 for no overlap between niches to 1 for complete overlap. This metric is a proxy of niche similarity (Broennimann et al., 2012; Cola et al., 2017). Separately, we tested the effect of the presence of Degu burrows on palm abundance, plus the effect of other local environmental variables over seedling abundance, conducting a General Linear regressive Model (GLM) with Poisson distribution and log link function, in a single model.
Both models (PCA and GLM) were based on local environmental variables (∼12 m spatial resolution), with the distinction that GLMs were measured from the plot central coordinate (in perspective of field plots) and PCAs from each individual within every plot (which is equal to each burrow/palm seedling) coordinate.
Results
Biogeographic distribution models (SDMs) and potential interaction models (PIDM)
The performance of the SDMs measured by the AUC values for Jubaea chilensis were 0.961 (SD ± 0.014), and were 0.996 (SD ± 0.001) for Octodon degus. Climatic preferences for both species have fitted for Mediterranean climates, where precipitation accumulates in the cold season, followed by a drier, warmer season. The precipitation of the warmest quarter (bio18) was the most important climatic variable for Octodon degus [Permutation Importance (PI) = 57.8%; Percent Contribution (PC) = 81%], followed by precipitation of the coldest quarter (bio19; PI = 13.2%; PC = 14), accounting for more than 94% of the total variation of the Degu model. Octodon degus seem to be less tolerant to mild, wet winters, where suitability values in curves dropped from near 90 to 0% (>0 mm). Under the coldest quarter, the model suggests a monotonic curve between 0 and 600 mm, with maximum suitability of 90% near 200–400 mm (see Supplementary Figure 6A).
In the Jubaea chilensis model, precipitation of the driest month (bio14, PI = 40.5%; PC = 30.1%) and the coldest quarter (bio19; PI = 26.9%; PC = 19.4%) explained more than 65% of the model (see Supplementary Figure 6B). For the driest month, palm habitat suitability values in curves are up to 4 mm, indicating adult individuals’ toleration to dry climates, while in the coldest quarter, palm-suitable habitats ranged from 200 to 1,200 mm, as the suitability curves suggest.
In the PIDM (interaction model), higher values of interactions were obtained toward the center of the distribution of Jubaea chilensis (between 32 and 34°S), specifically between the Coast and Andes ranges of Central Chile (or between Ocoa and Cantillana localities; 71–72’W). Lower values were obtained toward the northern and southern distribution ranges, i.e., above 31’S and below 34’S (Figures 3A–C).
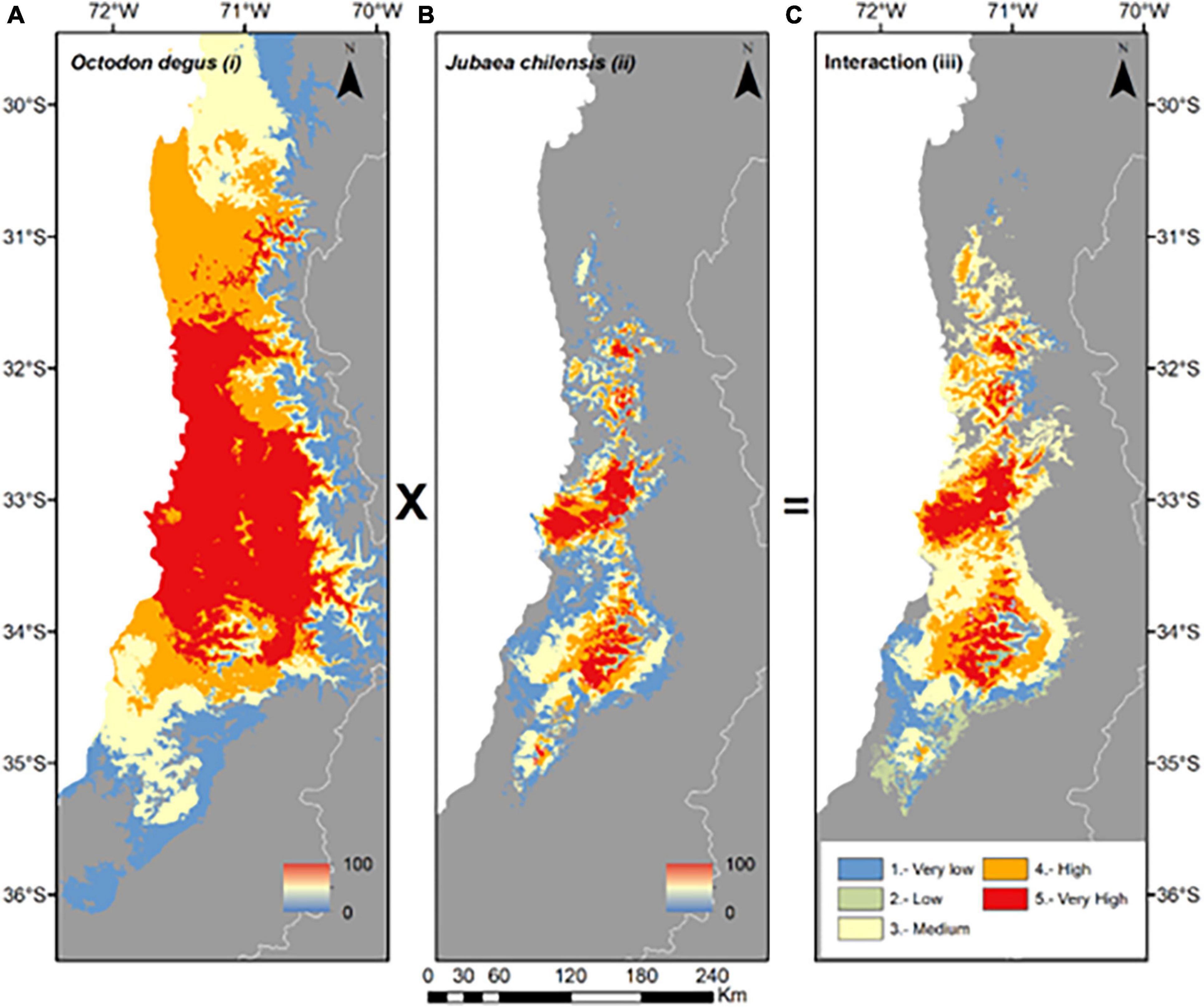
Figure 3. Spatial distribution models and potential spatial interactions (PSI) between Octodon degus and Jubaea chilensis. (A) SDM for Octodon degus; (B) SDM for Jubaea chilensis; (C) PIDM model between both species, which resulted from the product of (A) and (B), weighted in five values (1, Very low; 2, Low; 3, Medium; 4, High; and 5, Very high).
Several variables for PCA, GLM, or both, were used as follows: (A) both models: (i) elevation (DEM), (ii) Topographic exposure (Aspect), (iii) Land Surface Temperature (LST), (iv) Enhanced Vegetation Index (EVI). (B) Only PCAs: (vi) Topographic Roughness Index (TRI), (vii) Topographic Wetness Index (TWI), (viii) Normalized Salinity Index (NDSI), (ix) Euclidean distance to watercourses (Disthidro), and (x) Euclidean distance to palm adults (Distad). (C) Only GLMs: (v) presence or absence of Degu burrows inside each plot (IsMadr; as the most parsimonious approach); (vi) Number of Jubaea chilensis adults within each plot (n_adult). Variables were obtained from satellite images from Sentinel-2A annual composites (“COPERNICUS/S2_SR_HARMONIZED”; median composite; year = 2018; which have a ∼12 m spatial resolution at Central Chile) obtained from Google Earth Engine, a cloud-based supercomputer for geoscientific spatial analyses (from which the clouds before conducting the image composites were masked out; Gorelick et al., 2017; see S5 for each variable details). All R analyses were made with version 4.2 (For a graphic description of the methodology, please refer to Figure 2).
Jubaea chilensis survey
We recorded 215 individuals of Jubaea chilensis in the four sites analyzed: 86 seedlings and 129 adults. Higher abundances were recorded in Ocoa (43 seedlings; 60 adults) and Cocalán (41 seedlings; 35 adults), which are the geographic zones with higher interaction intensity (PIDM). In contrast, seedling densities in Petorca and Hualañé were lower (0 seedlings; 25 adults; 2 seedlings; 9 adults, respectively) and in the predicted zones with lower PIDM (Wilcoxon, P = 0.0158; Figure 4 and Table 1).
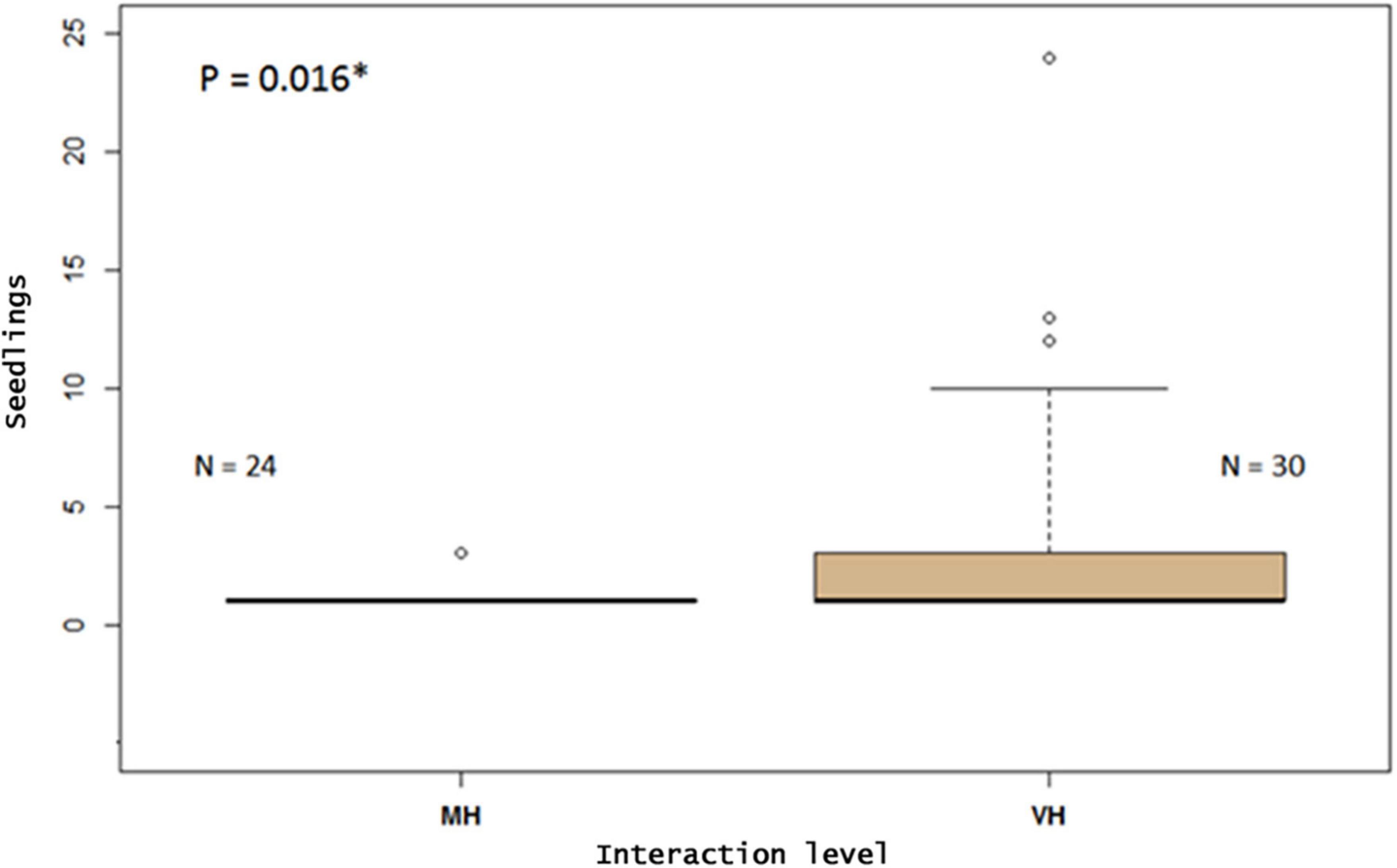
Figure 4. Seedling field measure for Jubaea chilensis vs. sampling group plots (N plots = 54). MH, medium-high (Petorca + Hualañe). VH, very-high (Ocoa + Cocalan). Wilcox non-parametric test was used (P = 0.016). The symbol * indicates that the p value is significant.
Local spatial analysis
The two axes of the PCA explained 66.20% of the variance, strongly characterized by specific environmental requirements for Jubaea chilensis seedling establishment as well as Octodon degus burrows. The Jubaea chilensis and Octodon degus requirements overlapped by 83.24%, much more than expected by chance (P-value = 0.001; Figure 5). Non-overlapping areas corresponded to exclusive environmental areas for palm seedlings and Degu burrows, which were even smaller for seedlings (Figure 5). PC1 is characterized by specific NDSI, NDVI, EVI, NDWI, LST, and TWI, while for PC2 the most contributing variables to each component were Slope, TRI, DEM, and TWI (for further details, see Figure 6). Following the latter argument, PC1 is more weighted by salinity, vegetation, wetness, and temperature variables than PC2, which is more influenced by topographic variables. Nevertheless, TWI, an index related to watercourses, influenced both components.
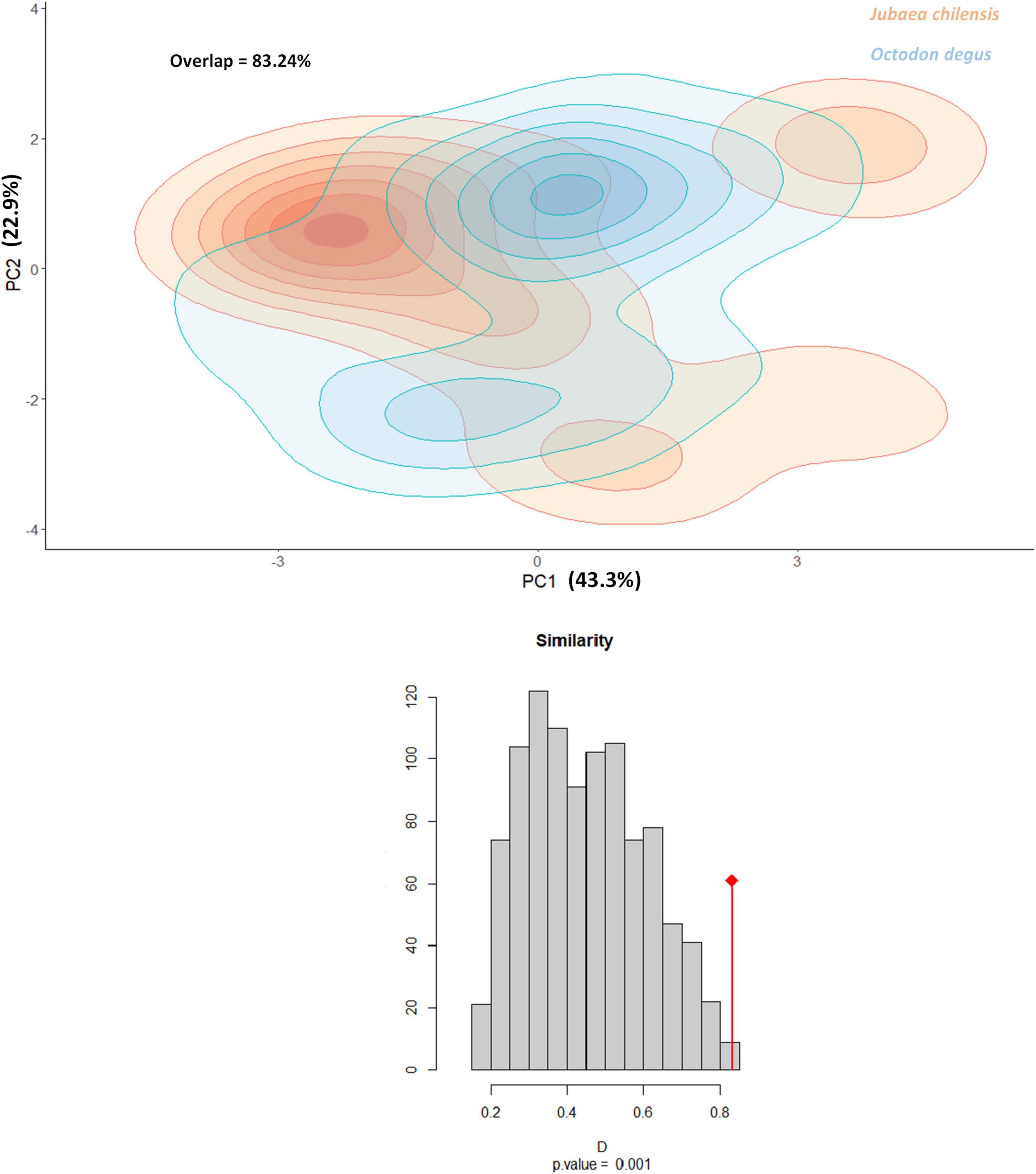
Figure 5. Representation of ecological niche models for Octodon degus (blue) and Jubaea chilensis (orange) overlapping distributions. PC1 contribution = 43.44%. PC2 contribution = 23.69%. Spatial overlap = 83.24%. Similarity test = 0.77 (P-value = 0.001).
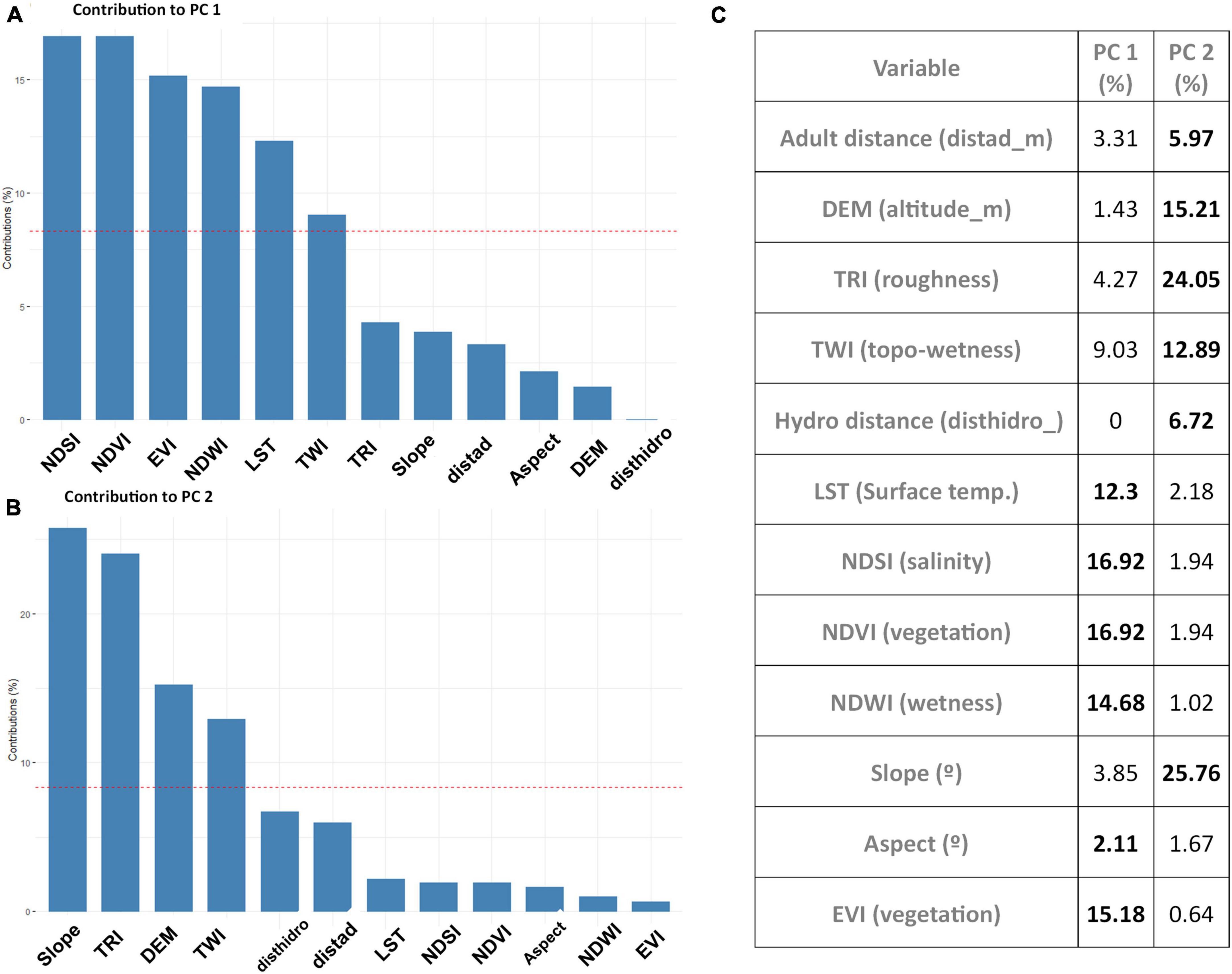
Figure 6. Principal components analysis (PCA) contributions. The latter corresponds to the environmental variables from local niche overlap between Octodon degus and Jubaea chilensis. (A) Principal component 1 variable contribution. (B) Principal component 2 variable contribution. (C) Both components expanded variable contribution. All values are expressed in percentages. Red dashed lines correspond to the average contribution of all variables within each component, so the variables above each dashed line represent the most contributing variables.
In our GLM for Jubaea chilensis, several variables were found to be significant (P-value < = 0.05), listed here in decreasing order of contribution to the model’s intercept: the EVI index (vegetation), the NDWI index (wetness), the number of adult Jubaea chilensis trees, DEM (altitude), and LST (surface temperature). Both DEM and LST had negative estimates, whereas the other listed variables had positive estimates (further details on Table 2 and Figure 7). Interestingly we observed that the presence-absence of degu burrows had a greater impact on the model (estimate = 0.410) than the number of adult Jubaea chilensis trees (estimate = 0.335); however, the variable effect was not significant (P-value = 0.1246). Our analysis also revealed that the wetness index positively correlated with seedling density, suggesting that sites with dryer conditions are associated with lower seedling densities when contrasted with wetter conditions sites (refer to Table 2 and Supplementary Figure 5 for more details).
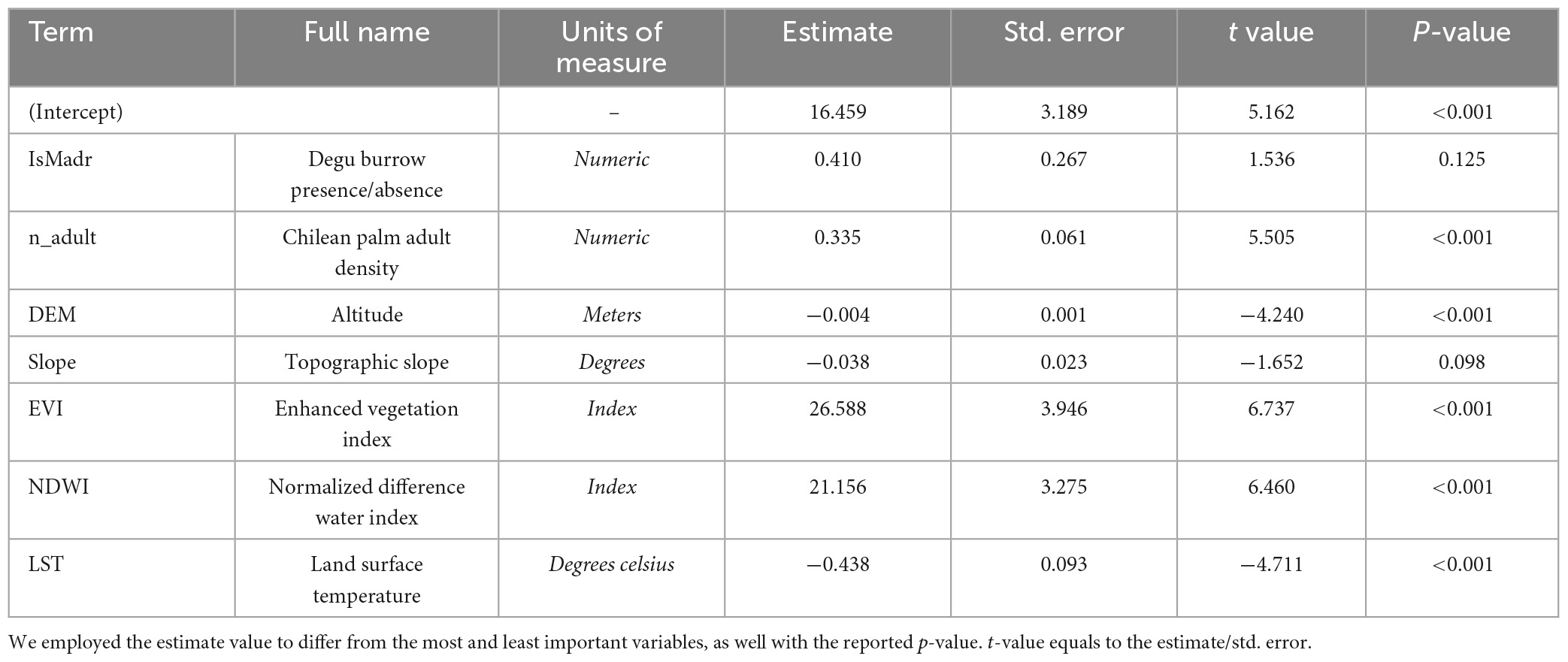
Table 2. General linear model results of the effects of variables explaining seedling densities of Jubaea chilensis in our four study areas and at local scale (∼12 m).
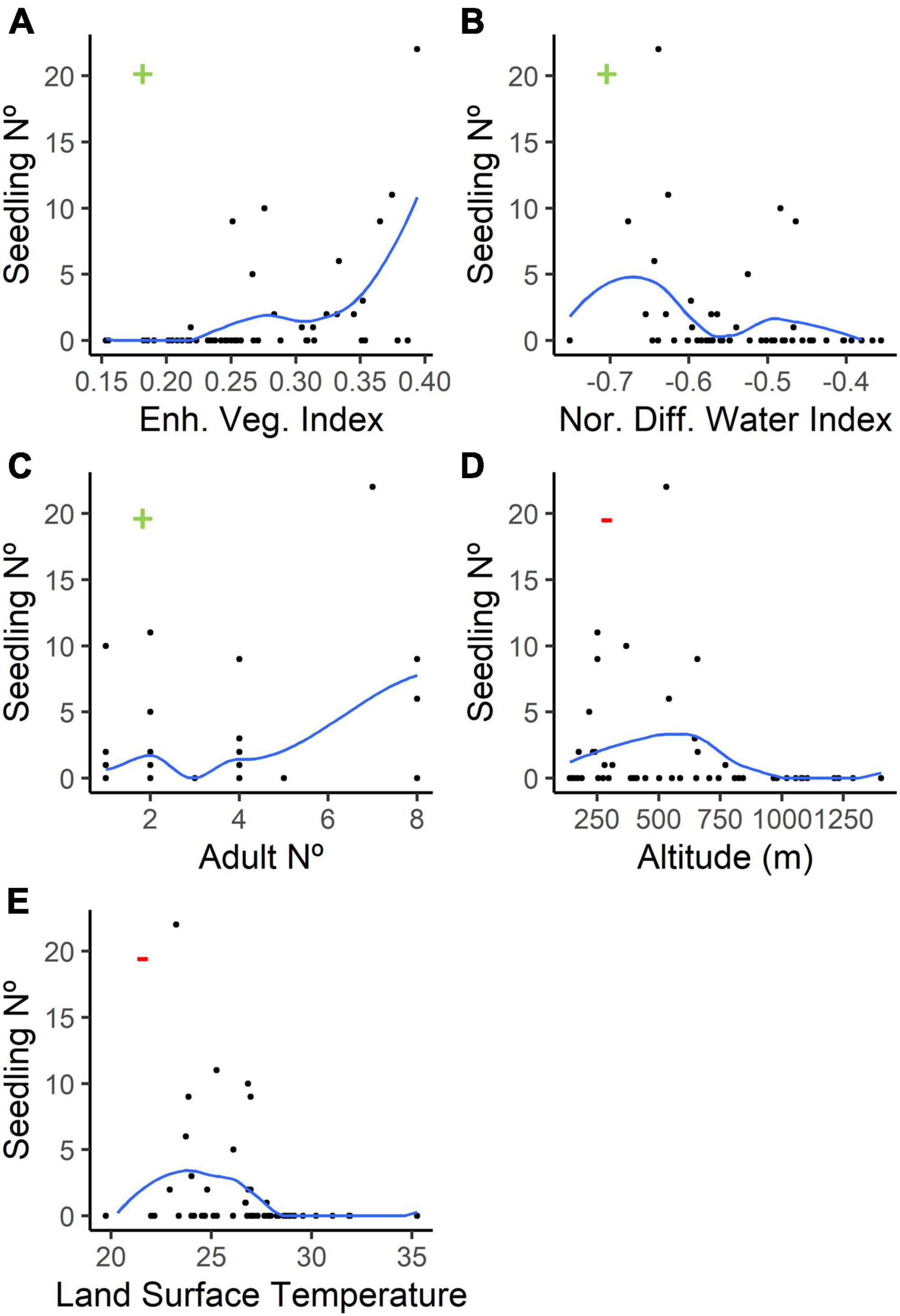
Figure 7. This figure shows the effect of the significant local environmental variables (p-value < = 0.05) on Jubaea chilensis seedling density, as predicted by our GLM model. On each graph, black dots correspond to different densities of Chilean palm seedlings within sampled plots. The following variables were found to be significant: (A) EVI (enhanced vegetation index), (B) NDWI (normalized difference water index), (C) the number of adult Jubaea chilensis individuals (Adult N°), (D) DEM (altitude above sea level), and (E) LST (land surface temperature). EVI and NDWI are normalized indexes that range from −1 to 1, with negative values indicating bare soils and dry areas, respectively. Altitude is measured in meters, and LST is measured in degrees Celsius. Each plot is marked with a + (green) or–(red) sign, indicating whether the interaction is positive or negative, as given by the “estimate” parameter. The Loess curve represents the best fit.
Discussion
In this study, we examined whether the mutualistic interactions between Jubaea chilensis and Octodon degus are expressed at two spatial scales: (a) at a biogeographic scale, using SDMs for palms and Degu and exploring co-occurrence zones as indirect evidence of mutualism (Araújo and Rozenfeld, 2014); (b) at a local scale, firstly exploring niche overlap between both species using local environmental variables, and secondly examining whether palm seedling density responds to the presence/absence of degu burrows as well as to local environmental variables.
Mutualistic effects of degus on palms
The present results support the existence of mutualistic interactions between Jubaea chilensis and Octodon degus, which may be perceptible at both evaluated spatial scales. There was indeed a high seedling density in localities with the highest potential interactions (predicted from SDMs) relative to those localities with lower potential interactions, reinforcing the idea of a biogeographic signal of biotic interactions (BI). These findings oppose previous reports in the literature that suggests that animal-plant BI can be detected only at local scales (Wisz et al., 2013; Cabra-Rivas et al., 2016). This large-spatial scale effect is a consequence of a long-term coevolutionary history of more than six million years between the Chilean palm and the Degu (Valladares, 2009; Rivera et al., 2016).
Regarding the local scale analysis, significant overlap between the palm and degu niches was detected, suggesting a positive association between both species; however, the presence of Degu burrows did not increase significantly seedling density. This issue deserves to be analyzed with caution. The positive effect of seed dispersers can occur at earlier life cycle stages, such as the seed stage (Bustamante and Canals, 1995), which we did not analyze in our study. Moreover, (i) by digging seeds, Degus provides refuge for seeds against other seed predators such as native and exotic rodents; (ii) the soil affected by rodent activity increases fertility as a consequence of digging, urine, and feces, thus increasing germination and seedling growth; (iii) as seedlings become older, the positive effects of Degu (i.e., fertility) may be diluted by the preeminence of other local factors, such as those evaluated in our GLM.
Influence of local scale factors over Jubaea chilensis seedling abundance
We found that NDWI, EVI/NDVI, and Slope were important local variables for the Chilean palm seedling density. Most of these factors can be a proxy of water availability and vegetation cover, which have been regarded as determinant elements for plant survival along ecological gradients (Pettorelli et al., 2017). Water availability and vegetation cover are essential in Mediterranean ecosystems as limiting factors for native plant abundance and distribution (Matías et al., 2011), explicitly affecting seedling survival (McDowell et al., 2008). We have evidence that palm seedlings grow preferentially beneath the canopy of Mediterranean vegetation (Fleury et al., 2015; Flores et al., 2016; Miranda et al., 2016) because native shrubs provide refuge against herbivores (cattle, rabbits) and are as well fertile nutrient patches, which in turn favor seedling growth (Maestre et al., 2009).
Spatial scale of studies
We argue that the study of BI at different spatial scales is a complementary approach. SDMs provide clues about the locations where mutualistic interactions should be more intense; those locations (local scale) should be used to address the underlying mechanisms that explain the fitness responses of interacting species. Using SDMs, we detected in the present study the zones where mutualistic interactions should be more intense, and also examined this interaction at a local scale, assessing the effect of Degus and other factors on palm regeneration. In sum, SDMs constitute the first step to examine the hypothetical sites within the geographic mosaic (Thompson, 2005), where mutualistic interactions are more probable (Araújo and Luoto, 2007).
The conservation of Jubaea chilensis: current threats, restoration, and rewilding
Seed harvesting by people constitutes a significant threat to the future of palm populations (Youlton et al., 2016), due to massive seed removal since colonials time (González et al., 2017). Given that seed abundance is regarded as a modulator of the intensity of seed predation (predator satiation hypothesis; sensu Janzen, 1971; Xiao et al., 2013), we hypothesize that human overharvest can be detrimental to Chilean palm regeneration because the available seeds could be not enough to satiate the Degu, which will tend to burrow more seeds. Further research should be conducted to test this prediction because if this mechanism occurs in our case, assessing the seed density threshold (available after harvesting) will be mandatory to define whether Degu is a net seed predator or a net seed disperser.
The current conservation status of the Chilean palm is endangered (CONAF, 2020). Consequently, it is mandatory to conduct conservation actions to enhance the persistence of this singular component of biodiversity in Central Chile. However, it is challenging to conduct efficient conservation actions, as the Chilean palms are part of a subtle and complex network of BI that includes degus, humans, exotic species, and the environment.
Habitat rewilding (see Mittelman et al., 2022) appears to be a viable alternative since it focuses on restoring lost interactions through different actions. In the case of the Chilean palm, habitat rewilding could be addressed by reintroducing the Andean camelids (Lama guanicoe and Lama glama) to restore lost trophic interactions that were predominant before the colonial times, because Andean camelids are considered the original seed dispersers for many plant species of southern South America (Hubbe et al., 2013; Root-Bernstein and Svenning, 2016). Furthermore, we argue that rewilding with camelids could significantly increase the chance of the Chilean palm expanding its geographic range as these mammals can disperse seeds at larger distances, sometimes encompassing several kilometers (Root-Bernstein and Svenning, 2016).
Mediterranean shrublands were one of the native habitats for camelids and palms; they should also be rewilded to provide them with conditions to maintain viable and healthy populations over time (Benayas et al., 2009; Jones et al., 2018). However, rewilding must include overhunting regulations, since their lack explains why there are no natural populations of camelids in central Chile nowadays (see Westreicher et al., 2007). Alongside habitat rewilding, there is a need to control exotic rodents (Rattus rattus and Rattus norvegicus), as they are active seed consumers (Towns et al., 1997; Dukes and Mooney, 2004; Gosse et al., 2011; Cordero et al., 2021).
How can SDMs guide restoration and rewilding?
Given our current SDMs results, we hypothesize two scenarios. A first scenario characterized by places where Octodon degus is unsuitable and in which Jubaea chilensis is suitable (but not present). In this case, we can dispose of palm seedlings under the native vegetation canopy as they are micro-habitats for Mediterranean seed survival. Therefore, the reintroduction with camelids could be conducted to restore the ancient mutualistic interactions. A second scenario, in which there are places suitable for both Octodon degus and Jubaea chilensis, but in which the rodent is not present (but the palm is). In this case, Octodon degus could be trained to capture, cache, and consume the palm seeds (Tokimoto and Okanoya, 2004; Okanoya et al., 2008; Nakajima et al., 2016) and reintroduce them in the wild. This last strategy, however, must be guided in the first phase because Degus do not recognize palms as a resource, as they are not part of their habitat. However, Octodon degus is a species with learning skills (Vasquez et al., 2002), which can learn to consume palm seedlings if given enough time.
The use of Joint SDMs (JSDMs) to disentangle species co-occurrence patterns in the geographic space is becoming a novel approach to examining BI in the geographic space (Poggiato et al., 2021). Our attempt to examine mutualisms between two species using SDMs for only two species is the first stage to better comprehending BI. Future work may involve the development of more complex models that consider multi-species interactions, such as the possible interactions among palms, rats, rabbits, camelids, cattle, or even vegetation and humidity, to find zones that will allow to identify competition, herbivory, seed predation, or mutualism. The latter is now possible extracting the residual correlation matrix from JSDMs (Poggiato et al., 2021); the best regions as potential candidates to begin rewilding efforts should be those which include the majority of biotic interactions among native species.
Concluding remarks
In this study, we report that the interactions between Jubaea chilensis and Octodon degus are expressed at both biogeographic and local scales. The high seedling density in localities with high potential interactions reinforces the idea of a biogeographic signal of biotic interactions. Moreover, we also detected a significant overlap between the palm and degu niches at the local scale, suggesting a positive association between both species. However, the presence of Degu burrows did not increase significantly seedling density (if this variable is contrasted with other local variables), an issue which deserves further analysis. Seed harvesting by humans constitutes a significant threat to the future of palm populations, and habitat rewilding appears to be a viable alternative to enhance their conservation. Finally, we argue that the study of biotic interactions at different spatial scales is a complementary approach, with SDMs providing clues about locations where mutualistic interactions are more intense, and local studies addressing the underlying mechanisms.
Data availability statement
The raw data supporting the conclusions of this article will be made available by the authors, without undue reservation.
Author contributions
IN-H and RB conceived the idea and the study design. IN-H collected and analyzed the data and was responsible for manuscript writing. IN-H, MF, and RB helped with the methodology and revised the manuscript. All authors contributed to the final draft and gave their final approval for publication.
Funding
IN-H was funded by the National Agency for Research and Development (ANID)/Scholarship Program/CONICYT-PFCHA/Doctorado Nacional/2020–21201096. MF was funded by the Conselho Nacional de Pesquisa (CNPq, PDE248592/2013-0). RB was funded by Grants ANID/BASAL FB210006 and CHIC-AND/BASAL PFB210018.
Acknowledgments
We are grateful to Estefany Goncalves, Paula Formas, Nicolás Saez, and Ronny Zuñiga for their help with field work; and to “Corporación Nacional Forestal”, “Oasis La Campana”, “Hacienda Las Palmas de Cocalán”, and “Forestal Arauco Zona Norte, Unidad de Agua y Biodiversidad” for field support, allowing our access and data collecting permissions for “La Campana”, “Cocalán”, and “Hualañé”, respectively. We also thank Drs. Rodrigo Vasquez and Luis González for their valuable comments and discussions on the manuscript.
Conflict of interest
The authors declare that the research was conducted in the absence of any commercial or financial relationships that could be construed as a potential conflict of interest.
Publisher’s note
All claims expressed in this article are solely those of the authors and do not necessarily represent those of their affiliated organizations, or those of the publisher, the editors and the reviewers. Any product that may be evaluated in this article, or claim that may be made by its manufacturer, is not guaranteed or endorsed by the publisher.
Supplementary material
The Supplementary Material for this article can be found online at: https://www.frontiersin.org/articles/10.3389/ffgc.2023.1143208/full#supplementary-material
References
Alaniz, A., Núñez-Hidalgo, I., Carvajal, M., Alvarenga, T., Gómez-Cantillana, P., and Vergara, P. (2020). Current and future spatial assessment of biological control as a mechanism to reduce economic losses and carbon emissions: The case of Solanum sisymbriifolium in Africa. Pest Manag. Sci. 76, 2395–2405. doi: 10.1002/ps.5776
Araújo, M., and Luoto, M. (2007). The importance of biotic interactions for modelling species distributions under climate change. Global Ecol. Biogeogr. 16, 743–753. doi: 10.1111/j.1466-8238.2007.00359.x
Araújo, M. B., and Rozenfeld, A. (2014). The geographic scaling of biotic interactions. Ecography 37, 406–415. doi: 10.1111/j.1600-0587.2013.00643.x
Benayas, J. M. R., Newton, A. C., Diaz, A., and Bullock, J. M. (2009). Enhancement of biodiversity and ecosystem services by ecological restoration: A meta-analysis. Science 325, 1121–1124. doi: 10.1126/science.1172460
Blanco, G., Hiraldo, F., and Tella, J. (2018). Ecological functions of parrots: An integrative perspective from plant life cycle to ecosystem functioning. Emu Aust. Ornithol. 118, 36–49. doi: 10.1080/01584197.2017.1387031
Bosso, L., De Conno, C., and Russo, D. (2017). Modelling the risk posed by the zebra mussel Dreissena polymorpha: Italy as a case study. Environ. Manage 60, 304–313. doi: 10.1007/s00267-017-0882-8
Bozinovic, F., Rojas, J., Broitman, B., and Vásquez, R. (2009). Basal metabolism is correlated with habitat productivity among populations of degus (Octodon degus). Comp. Biochem. Physiol. A Mol. Integr. Physiol. 152, 560–564. doi: 10.1016/j.cbpa.2008.12.015
Broennimann, O., Fitzpatrick, M., Pearman, P., Petitpierre, B., Pellissier, L., Yoccoz, N., et al. (2012). Measuring ecological niche overlap from occurrence and spatial environmental data: Measuring niche overlap. Global Ecol. Biogeogr. 21, 481–497. doi: 10.1111/j.1466-8238.2011.00698.x
Brown, J., Bennett, J., and French, C. (2017). SDMtoolbox 2.0: The next generation Python-based GIS toolkit for landscape genetic, biogeographic and species distribution model analyses. PeerJ 5:e4095. doi: 10.7717/peerj.4095
Bustamante, R. O., and Canals, M. (1995). Dispersal quality in Plants: How to measure efficiency and effectiveness of a seed disperser. Oikos 73:133. doi: 10.2307/3545736
Cabello. (2006). “Jubaea chilensis (Mol.) Baillon,” in Las especies arbóreas de los bosques templados de chile y Argentina autoecología, ed. C. Donoso (Valdivia: Marisa Cúneo Ediciones), 678.
Cabra-Rivas, I., Saldaña, A., Castro-Díez, P., and Gallien, L. (2016). A multi-scale approach to identify invasion drivers and invaders’ future dynamics. Biol. Invas. 18, 411–426. doi: 10.1007/s10530-015-1015-z
Carvajal, M., Alaniz, A., Smith-Ramírez, C., and Sieving, K. (2018). Assessing habitat loss and fragmentation and their effects on population viability of forest specialist birds: Linking biogeographical and population approaches. Divers. Distr. 24, 820–830. doi: 10.1111/ddi.12730
Cola, V., Broennimann, O., Petitpierre, B., Breiner, F., D’Amen, M., Randin, C., et al. (2017). Ecospat: An R package to support spatial analyses and modeling of species niches and distributions. Ecography 40, 774–787. doi: 10.1111/ecog.02671
CONAF (2020). Palma chilena es recategorizada “En Peligro” y se aumentan medidas para su recuperación. Available online at: https://www.conaf.cl/palma-chilena-es-recategorizada-en-peligro-y-se-aumentan-medidas-para-su-recuperacion/. (accessed on 24 Oct, 2022).
Cordero, S., Gálvez, F., and Fontúrbel, F. (2021). Multiple anthropogenic pressures lead to seed dispersal collapse of the southernmost palm Jubaea chilensis. Front. Ecol. Evol. 9:719566. doi: 10.3389/fevo.2021.719566
Correa, J., Bacigalupo, A., Fontúrbel, F., Oda, E., Cattan, P., Solari, A., et al. (2015). Spatial distribution of an infectious disease in a small mammal community. Naturwissenschaften 102:51. doi: 10.1007/s00114-015-1304-5
Davis, G. T., Vásquez, R. A., Poulin, E., Oda, E., Bazán-León, E. A., Ebensperger, L. A., et al. (2016). Octodon degus kin and social structure. J. Mammal. 97, 361–372. doi: 10.1093/jmammal/gyv182
Díaz-Calderón, E. (2009). Estructura de poblaciones naturales de palma chilena, Jubaea chilensis (Mol.) Baillon. Ph.D thesis. Santiago: Universidad de Chile.
Dirzo, R., Young, H., Galetti, M., Ceballos, G., Isaac, N., and Collen, B. (2014). Defaunation in the Anthropocene. Science 345, 401–406. doi: 10.1126/science.1251817
Dormann, C., Bobrowski, M., Dehling, D., Harris, D. J., Hartig, F., Lischke, H., et al. (2018). Biotic interactions in species distribution modelling: 10 questions to guide interpretation and avoid false conclusions. Global Ecol. Biogeogr. 27, 1004–1016. doi: 10.1111/geb.12759
Dukes, J., and Mooney, H. (2004). Disruption of ecosystem processes in western North America by invasive species. Rev. Chil. Hist. Nat. 77, 411–437. doi: 10.4067/S0716-078X2004000300003
Ebensperger, L., and Bozinovic, F. (2000). Communal burrowing in the hystricognath rodent, Octodon degus?: A benefit of sociality? Behav. Ecol. Sociobiol. 47, 365–369. doi: 10.1007/s002650050678
Ebensperger, L., and Wallem, P. (2002). Grouping increases the ability of the social rodent, Octodon degus, to detect predators when using exposed microhabitats. Oikos 98, 491–497. doi: 10.1034/j.1600-0706.2002.980313.x
Ebensperger, L., Hurtado, M., Soto-Gamboa, M., Lacey, E., and Chang, A. (2004). Communal nesting and kinship in degus (Octodon degus). Naturwissenschaften 91, 391–395. doi: 10.1007/s00114-004-0545-5
Elith, J. (2016). Predicting distributions of invasive species. Arxiv [Preprint]. doi: 10.48550/arXiv.1312.0851
Fernández-López, J. (2017). NiceOverPlot, or when the number of dimensions does matter(R script). Available online at: https://allthiswasfield.blogspot.com/2017/05/niceoverplot-or-when-number-of.html (accessed on 15 Nov, 2021).
Fleury, M., Marcelo, W., Vásquez, R., González, L., and Bustamante, R. (2015). Recruitment dynamics of the relict palm, Jubaea chilensis: Intricate and pervasive effects of invasive herbivores and nurse shrubs in central chile. PLoS One 10:e0133559. doi: 10.1371/journal.pone.0133559
Flores, S., Promis, A., and Faúndez, L. (2016). Caracterización florística y estructural de la población natural de Jubaea chilensis (Molina) Baill. más austral de Chile. Phyton 85, 324–332.
Fuentes, E., Jaksiæ, F., and Simonetti, J. (1983). European rabbits versus native rodents in Central Chile: Effects on shrub seedlings. Oecologia 58, 411–414. doi: 10.1007/BF00385244
Fulk, G. (1976). Notes on the activity, reproduction, and social behavior of Octodon degus. J. Mammal. 57, 495–505. doi: 10.2307/1379298
Galetti, M., Donatti, C., Pires, A., Guimarães, P., and Jordano, P. (2006). Seed survival and dispersal of an endemic Atlantic forest palm: The combined effects of defaunation and forest fragmentation. Bot. J. Linn. Soc. 151, 141–149. doi: 10.1111/j.1095-8339.2006.00529.x
Genes, L., and Dirzo, R. (2022). Restoration of plant-animal interactions in terrestrial ecosystems. Biol. Conserv. 265:109393. doi: 10.1016/j.biocon.2021.109393
Genes, L., Cid, B., Fernandez, F., and Pires, A. (2017). Credit of ecological interactions: A new conceptual framework to support conservation in a defaunated world. Ecol. Evol. 7, 1892–1897. doi: 10.1002/ece3.2746
González, L., Ibáñez, M., and Cerrillo, N. (2017). Palma Chilena: Una especie emblemática que Chile necesita recuperar. Dublin: Origo Ediciones.
González, L., Navarro, C., Rafael, M., Miguel, A., and Manuel, T. (2009). Ecology and management of the Chilean Palm (Jubaea chilensis): History, current situation and perspectives. Palms 53, 68–74.
Gorelick, N., Hancher, M., Dixon, M., Ilyushchenko, S., Thau, D., Moore, R., et al. (2017). Google earth engine: Planetary-scale geospatial analysis for everyone. Remote Sens. Environ. 202, 18–27. doi: 10.1016/j.rse.2017.06.031
Gosse, J., Hermanutz, L., and McLaren, B. (2011). Degradation of boreal forests by nonnative herbivores in newfoundland’s national parks: Recommendations for ecosystem restoration. Nat. Areas J. 31, 331–339. doi: 10.3375/043.031.0403
Guimarães, P., Galetti, M., and Jordano, P. (2008). Seed dispersal anachronisms: Rethinking the fruits extinct megafauna ate. PLoS One 3:e1745. doi: 10.1371/journal.pone.0001745
Guisan, A., and Thuiller, W. (2005). Predicting species distribution: Offering more than simple habitat models. Ecol. Lett. 8, 993–1009. doi: 10.1111/j.1461-0248.2005.00792.x
Guisan, A., and Zimmermann, N. (2000). Predictive habitat distribution models in ecology. Ecol. Model. 135, 147–186.
Harms, K. E., Wright, S. J., Calderón, O., Hernández, A., and Allen Herre, E. (2000). Pervasive density-dependent recruitment enhances seedling diversity in a tropical forest. Nature 404, 493–495. doi: 10.1038/35006630
Heikkinen, R., Luoto, M., Virkkala, R., Pearson, R., and Körber, J. (2007). Biotic interactions improve prediction of boreal bird distributions at macro-scales. Global Ecol. Biogeogr. 16, 754–763. doi: 10.1111/j.1466-8238.2007.00345.x
Hubbe, A., Hubbe, M., and Neves, W. A. (2013). The Brazilian megamastofauna of the Pleistocene/Holocene transition and its relationship with the early human settlement of the continent. Earth Sci. Rev. 118:1e10.
Hunter, J. R. (1989). Seed dispersal and germination of Enterolobium cyclocarpum (Jacq.) Griseb. (Leguminosae: Mimosoideae): Are megafauna necessary? J. Biogeogr. 16, 369–378.
Hurlbert, S. (1984). Pseudoreplication and the design of ecological field experiments. Ecol. Monogr. 54, 187–211. doi: 10.2307/1942661
Jansen, P., Hirsch, B., Emsens, W., Zamora-Gutierrez, V., Wikelski, M., and Kays, R. (2012). Thieving rodents as substitute dispersers of megafaunal seeds. Proc. Natl. Acad. Sci. U. S. A. 109, 12610–12615. doi: 10.1073/pnas.1205184109
Janzen, D. (1971). Seed predation by animals. Annu. Rev. Ecol. Syst. 2, 465–492. doi: 10.1146/annurev.es.02.110171.002341
Jones, H. P., Jones, P. C., Barbier, E. B., Blackburn, R. C., Benayas, J. M., Holl, K. D., et al. (2018). Restoration and repair of Earth’s damaged ecosystems. Proc. R. Soc. B 285:20172577. doi: 10.1098/rspb.2017.2577
Kurten, E. (2013). Cascading effects of contemporaneous defaunation on tropical forest communities. Biol. Conserv. 163, 22–32. doi: 10.1016/j.biocon.2013.04.025
Lany, N., Zarnetske, P., and Gouhier, T. (2017). Incorporating the effects of climate change on species interactions into species distribution models. Integr. Comp. Biol. 57, E320–E320.
Maestre, F., Callaway, R., Valladares, F., and Lortie, C. (2009). Refining the stress-gradient hypothesis for competition and facilitation in plant communities. J. Ecol. 97, 199–205.
Matías, L., Gómez-Aparicio, L., Zamora, R., and Castro, J. (2011). Effects of resource availability on plant recruitment at the community level in a Mediterranean mountain ecosystem. Persp. Plant Ecol. Evol. Syst. 13, 277–285. doi: 10.1016/j.ppees.2011.04.005
McDowell, N., Pockman, W., Allen, C., Breshears, D., Cobb, N., Kolb, T., et al. (2008). Mechanisms of plant survival and mortality during drought: Why do some plants survive while others succumb to drought? New Phytol. 178, 719–739. doi: 10.1111/j.1469-8137.2008.02436.x
Medina, D. (2011). Variación en la coloración del pelaje en poblaciones de Octodon degus a lo largo de su distribución geográfica. Ph.D thesis. Santiago: Universidad de Chile.
Meserve, P., Gutiérrez, J., and Jaksic, F. (1993). Effects of vertebrate predation on a caviomorph rodent, the degu (Octodon degus), in a semiarid thorn scrub community in Chile. Oecologia 94, 153–158. doi: 10.1007/BF00341311
Michea, G. (1988). Estudio poblacional de la palma chilena (Jubaea chilensis) en el sector de Ocoa, parque nacional la campana. Revista 9, 124–130.
Miranda, A., Hernández, H., Bustamante, R., Díaz, E., González, L., and Altamirano, A. (2016). Regeneración natural y patrones de distribución espacial de la palma chilena Jubaea chilensis (Molina) Baillon en los bosques mediterráneos de Chile central. Gayana Bot. 73, 54–63.
Mittelman, P., Landim, A., Genes, L., Assis, A., Starling-Manne, C., Leonardo, P., et al. (2022). Trophic rewilding benefits a tropical community through direct and indirect network effects. Ecography 2022:ecog.05838. doi: 10.1111/ecog.05838
Moreno-Contreras, I., Sánchez-González, L., Arizmendi, M., Prieto-Torres, D., and Navarro-Sigüenza, A. (2020). Climatic niche evolution in the Arremon brunneinucha complex (Aves: Passerellidae) in a Mesoamerican Landscape. Evol. Biol. 47, 123–132. doi: 10.1007/s11692-020-09498-7
Muscarella, R., and Fleming, T. (2007). The role of frugivorous bats in tropical forest succession. Biol. Rev. 82, 573–590. doi: 10.1111/j.1469-185X.2007.00026.x
Myers, L., and Sirois, M. (2014). “Spearman Correlation Coefficients, Differences between,” in Wiley statsref: Statistics reference online, (Atlanta, GA: American Cancer Society).
Nakajima, S., Kuramoto, Y., and Nagaishi, T. (2016). Position Learning of Degus (Octodon degus) in a Semi-natural “Counting” Task. Kwansei Gakuin University Humanities Review 20:1–14.
Nathan, R., and Muller-Landau, H. C. (2000). Spatial patterns of seed dispersal, their determinants and consequences for recruitment. Trends Ecol. Evol. 15, 278–285.
Okanoya, K., Tokimoto, N., Kumazawa, N., Hihara, S., and Iriki, A. (2008). Tool-use training in a species of rodent: The emergence of an optimal motor strategy and functional understanding. PLoS One 3:e1860
Parsons, R. (2007). The southernmost limits for palms. New Zeal. J. Bot. 45, 477–478. doi: 10.1080/00288250709509730
Peres, C., Emilio, T., Schietti, J., Desmoulière, S., and Levi, T. (2016). Dispersal limitation induces long-term biomass collapse in overhunted Amazonian forests. Proc. Natl. Acad. Sci. U. S. A. 113, 892–897. doi: 10.1073/pnas.1516525113
Pettorelli, N., Bühne, H., Tulloch, A., Dubois, G., Macinnis-Ng, C., Queirós, A., et al. (2017). Satellite remote sensing of ecosystem functions: Opportunities, challenges and way forward. Remote Sens. Ecol. Conserv. 4, 71–93. doi: 10.1002/rse2.59
Phillips, S., Anderson, R., and Schapire, R. (2006). Maximum entropy modeling of species geographic distributions. Ecol. Model. 190, 231–259. doi: 10.1016/j.ecolmodel.2005.03.026
Phillips, S., Anderson, R., DudÍk, M., Schapire, R., and Blair, M. (2017). Opening the black box: An open-source release of Maxent. Ecography 40, 887–893.
Pliscoff, P., Luebert, F., Hilger, H., and Guisan, A. (2014). Effects of alternative sets of climatic predictors on species distribution models and associated estimates of extinction risk: A test with plants in an arid environment. Ecol. Model. 288, 166–177. doi: 10.1016/j.ecolmodel.2014.06.003
Poggiato, G., Münkemüller, T., Bystrova, D., Arbel, J., Clark, J., and Thuiller, W. (2021). On the interpretations of joint modeling in community ecology. Trends Ecol. Evol. 36, 391–401. doi: 10.1016/j.tree.2021.01.002
Pottier, J., Dubuis, A., Pellissier, L., Maiorano, L., Rossier, L., Randin, C., et al. (2013). The accuracy of plant assemblage prediction from species distribution models varies along environmental gradients. Global Ecol. Biogeogr. 22, 52–63.
Pozo, R. (2009). Selección de pareja en Octodon degus provenientes de dos poblaciones contrastantes de diferente distribución geográfica. Ph.D thesis. Santiago: Universidad de Chile.
Quispe, R., Villavicencio, C., Cortés, A., and Vásquez, R. (2009). Inter-population variation in hoarding behaviour in degus, Octodon degus. Ethology 115, 465–474. doi: 10.1111/j.1439-0310.2009.01621.x
Rivera, D., Vianna, J., Ebensperger, L., and Eduardo Palma, R. (2016). Phylogeography and demographic history of the andean degu, Octodontomys gliroides (Rodentia: Octodontidae). Zool. J. Linn. Soc. 178, 410–430. doi: 10.1111/zoj.12412
Root-Bernstein, M., and Svenning, J. (2016). Prospects for rewilding with camelids. J. Arid Environ. 130, 54–61. doi: 10.1016/j.jaridenv.2016.03.011
Royston, J. (1983). A simple method for evaluating the shapiro-francia W’ Test of non-normality. Statistician 32:297. doi: 10.2307/2987935
Royston, P. (1992). Approximating the Shapiro-Wilk W-test for non-normality. Stat. Comput. 2, 117–119. doi: 10.1007/BF01891203
Saavedra, B., and Simonetti, J. (2003). Holocene distribution of Octodontid rodents in central Chile. Rev. Chil. Hist. Nat. 76, 383–389. doi: 10.4067/S0716-078X2003000300004
Schooler, S. L, Johnson, M. D., Njoroge, P., and Bean, W. T. (2020). Shade trees preserve avian insectivore biodiversity on coffee farms in a warming climate. Ecol. Evol. 10, 12960–12972. doi: 10.1002/ece3.6879
Schupp, E. (1993). “Quantity, quality and the effectiveness of seed dispersal by animals,” in Frugivory and seed dispersal: Ecological and evolutionary aspects, eds T. H. Fleming and A. Estrada (Dordrecht: Springer), 15–29.
Schupp, E., Jordano, P., and Gómez, J. (2010). Seed dispersal effectiveness revisited: A conceptual review. New Phytol. 188, 333–353. doi: 10.1111/j.1469-8137.2010.03402.x
Schweiger, O., Settele, J., Kudrna, O., Klotz, S., and Kühn, I. (2008). Climate change can cause spatial mismatch of trophically interacting species. Ecology 89, 3472–3479. doi: 10.1890/07-1748.1
Svenning, J., Pedersen, P., Donlan, C., Ejrnæs, R., Faurby, S., Galetti, M., et al. (2016). Science for a wilder Anthropocene: Synthesis and future directions for trophic rewilding research. Proc. Natl. Acad. Sci. U. S. A. 113, 898–906. doi: 10.1073/pnas.1502556112
Thompson, J. N. (2005). The Geographic mosaic of coevolution. Chicago, IL: University of Chicago Press.
Tokimoto, N., and Okanoya, K. (2004). Spontaneous construction of “Chinese boxes” by Degus (Octodon degu): A rudiment of recursive intelligence? Jpn. Psychol. Res. 46, 255–261.
Towns, D., Simberloff, D. A., and Atkinson, I. (1997). Restoration of New Zealand islands: Redressing the effects of introduced species. Pac. Conserv. Biol. 3:99. doi: 10.1071/PC970099
Valiente-Banuet, A., Aizen, M., Alcántara, J., Arroyo, J., Cocucci, A., Galetti, M., et al. (2015). Beyond species loss: The extinction of ecological interactions in a changing world. Funct. Ecol. 29, 299–307. doi: 10.1111/1365-2435.12356
Valladares, J. (2009). Variación geográfica de la conducta antidepredatoria del Octodon degus (molina 1782) bajo un contexto filogeografico. Ph.D thesis. Santiago: Universidad de Chile.
van Proosdij, A. S. J., Sosef, M., Wieringa, J., and Raes, N. (2016). Minimum required number of specimen records to develop accurate species distribution models. Ecography 39, 542–552. doi: 10.1111/ecog.01509
Vander Wall, S., and Longland, W. (2004). Diplochory: Are two seed dispersers better than one? Trends Ecol. Evol. 19, 155–161. doi: 10.1016/j.tree.2003.12.004
Vasquez, R. (2002). Vigilance and social foraging in Octodon degus (Rodentia: Octodontidae) in central Chile. Rev. Chil. Hist. Nat. 70, 557–563.
Vasquez, R. A., Ebensperger, L. A., and Bozinovic, F. (2002). The influence of habitat on travel speed, intermittent locomotion, and vigilance in a diurnal rodent. Behav. Ecol. 13, 182–187.
Violle, C., Reich, P. B., Pacala, S. W., Enquist, B. J., and Kattge, J. (2014). The emergence and promise of functional biogeography. Proc. Natl. Acad. Sci. U. S. A. 111, 13690–13696.
Wan, J., Oduor, A., Pouteau, R., Wang, B., Chen, L., Yang, B., et al. (2020). Can polyploidy confer invasive plants with a wider climatic tolerance? A test using Solidago canadensis. Ecol. Evol. 10, 5617–5630. doi: 10.1002/ece3.6303
Westreicher, C. A., Merega, J. L., and Palmili, G. (2007). The economics of pastoralism: Study on current practices in South America. Nomadic Peoples 11:87e105.
Wills, C., Condit, R., Foster, R., and Hubbell, S. (1997). Strong density- and diversity-related effects help to maintain tree species diversity in a neotropical forest. Proc. Natl. Acad. Sci. U. S. A. 94, 1252–1257. doi: 10.1073/pnas.94.4.1252
Wisz, M., Hijmans, R., Li, J., Peterson, A., Graham, C., and Guisan, A. (2008). Effects of sample size on the performance of species distribution models. Divers. Distr. 14, 763–773. doi: 10.1111/j.1472-4642.2008.00482.x
Wisz, M., Pottier, J., Kissling, W., Pellissier, L., Lenoir, J., Damgaard, C., et al. (2013). The role of biotic interactions in shaping distributions and realised assemblages of species: Implications for species distribution modelling. Biol. Rev. Camb. Philos. Soc. 88, 15–30. doi: 10.1111/j.1469-185X.2012.00235.x
Xiao, Z., Zhang, Z., and Krebs, C. (2013). Long-term seed survival and dispersal dynamics in a rodent-dispersed tree: Testing the predator satiation hypothesis and the predator dispersal hypothesis. J. Ecol. 101, 1256–1264.
Yap, J., Rossetto, M., Das, S., Wilson, P., Beaumont, L., and Henry, R. (2022). Tracking habitat or testing its suitability? Similar distributional patterns can hide very different histories of persistence versus nonequilibrium dynamics. Evolution 76, 1209–1228. doi: 10.1111/evo.14460
Yates, L., Saiz, F., and Zunino, S. (1994). Octodon degus: Valor nutricional y preferencia del recurso trófico en el palmar de Ocoa, Parque Nacional La Campana, Chile. Rev. Chil. Hist. Nat. 67, 89–99.
Youlton, C., Hormazabal, C., Schiappacasse, I., Contreras, P., and Poblete-Echeverría, C. (2016). Distribución, tamaño y estructura poblacional de Jubaea chilensis en “Las Palmas”, comuna de Petorca, región de Valparaíso - Chile. Bosque 37, 501–507. doi: 10.4067/S0717-92002016000300007
Young, H., McCauley, D., Galetti, M., and Dirzo, R. (2016). Patterns, causes, and consequences of Anthropocene Defaunation. Annu. Rev. Ecol. Evol. Syst. 47, 333–358. doi: 10.1146/annurev-ecolsys-112414-054142
Keywords: Jubaea chilensis, biological interactions, species distribution models (SDMs), multi-scale approach, effective disperser, ecological conservation
Citation: Núñez-Hidalgo I, Fleury M and Bustamante RO (2023) Niche models as a tool to inform restoration and conservation strategies: the case of Jubaea chilensis (palm, Arecaceae) and its seed disperser Octodon degus (rodent, Octodontidae). Front. For. Glob. Change 6:1143208. doi: 10.3389/ffgc.2023.1143208
Received: 12 January 2023; Accepted: 31 March 2023;
Published: 18 April 2023.
Edited by:
Meredith Root-Bernstein, CNRS UMR 7204, FranceReviewed by:
Sarah Sapsford, Murdoch University, AustraliaRajendra Mohan Panda, Mississippi State University, United States
Copyright © 2023 Núñez-Hidalgo, Fleury and Bustamante. This is an open-access article distributed under the terms of the Creative Commons Attribution License (CC BY). The use, distribution or reproduction in other forums is permitted, provided the original author(s) and the copyright owner(s) are credited and that the original publication in this journal is cited, in accordance with accepted academic practice. No use, distribution or reproduction is permitted which does not comply with these terms.
*Correspondence: Ramiro O. Bustamante, cmJ1c3RhbWFAdWNoaWxlLmNs