- 1Department for Innovation in Biological, Agro-Food and Forest Systems, University of Tuscia, Viterbo, Italy
- 2Department of Agricultural, Food, Environmental and Animal Sciences, University of Udine, Udine, Italy
- 3Council for Agricultural Research and Economics, Research Centre for Forestry and Wood, Arezzo, Italy
In some geographical areas of North America and Southeast Asia cities are frequently hit by heavy windstorms capable of knocking down hundreds of urban trees and large branches in a few minutes. Falling trees generate a broad array of disservices that vary over time and space. In Europe and worldwide the frequency of these extreme weather events has increased in recent decades and climate change could intensify these windstorms while the effects of urban land expansion could increase the extent of damage. However, Europe’s urban populations are unprepared for extreme weather events and are unable to limit the effects that the widespread loss of trees over limited space and time can have on people, buildings and city road networks. Preparing for rare, extreme future events that could strongly affect urban green infrastructures is a demanding challenge for city dwellers and for those who should ensure the continuity of the ecosystem services provided by urban trees. In fact, the damage caused by fallen trees is combined with the loss of the benefits provided by the trees themselves. Therefore the aim of this paper is to: (a) investigate the disruptions that a windstorm can cause in an urban area full of trees using a conceptual model; (b) conduct a literature review to determine how high the risk of these disservices occurring in Europe really is, which is definitely more likely than commonly perceived and could increase as a result of climate change and; (c) indicate what kind of measures can be taken in European cities to prevent or at least reduce the risks from falling urban trees during a strong windstorm, starting from the experience gained in the geographical areas most frequently and intensely affected by this type of weather event.
1. Introduction
In contemporary cities, trees are fundamental components of the urban landscape and they provide many ecosystem services to urban residents’ wellbeing (Gómez-Baggethun and Barton, 2013; Haase et al., 2014; Escobedo et al., 2015; Luederitz et al., 2015; Livesley et al., 2016; Dickinson and Hobbs, 2017; Nesbitt et al., 2017; Evans et al., 2022; Pinto et al., 2022). Urban tree-planting rates are likely to rise in the coming years in order to create more sustainable cities (Anguluri and Narayanan, 2017; Nitoslawski et al., 2019). Among the initiatives for adapting cities to climate change much attention is given to urban green infrastructures, especially to trees, since they provide shade to mitigate the health impacts of heat waves, improve rainwater infiltration and reduce flood risk during extreme rainfall events, absorb pollutants thus improving urban air quality (Nowak et al., 2006; Celikuilmaz-Ademir, 2010; Govindarajulu, 2014; Escobedo et al., 2015; Janhäll, 2015; Calfapietra et al., 2016; Kabisch et al., 2016; European Economic Area [EEA], 2020; Ramyar et al., 2021).
However, urban trees can also provide ecosystem disservices (Lyytimäki and Sipilä, 2009; von Döhren and Haase, 2015; Roman et al., 2021) such as damage caused by falling trees or large branches due to the wind. The most dangerous urban trees are those adjacent to buildings, roads, vehicular and pedestrian circulation facilities and other infrastructures that are essential for the functioning of the urban system and the life of its inhabitants. Furthermore, trees growing in these locations are more vulnerable to stress that make them more susceptible to windthrow (Referowska-Chodak, 2019) which can have serious consequences for people, vehicles and buildings.
Windthrow is both a natural phenomenon of forest ecosystems and a major disturbance agent. The time required for forests to recover differs depending on the magnitude of the phenomenon (Johnson and Miyanishi, 2020). However, the falling of a tree in the city is a traumatic event that nullifies the benefits that the tree can bring and can cause damage to property and injury to passersby. Therefore, windthrow should not occur in urban areas and must be prevented by felling and/or replacing trees before they become dangerous, which is one of the basic principles of risk management in urban green spaces (Pokorny, 1992; Tomao et al., 2015; Meunpong et al., 2019; Linhares et al., 2021).
Fortunately, serious accidents triggered by urban windstorms are rare. The annual mortality rate caused by the falling of a single tree or a large branch is relatively low when compared to the mortality rates of other types of accidents. In Australia mortality from windthrow per million inhabitants was estimated at 0.19 and at only 0.06 in urban areas (Walsh and Ryan, 2017; Way and Balogh, 2022), while in the United Kingdom mortality from windthrow is equal to 0.1 in both rural and urban areas, thus the risk of being killed by a falling tree is extremely low and not considered to be a social risk (Ball and Watt, 2013).
The situation changes on stormy days with very strong winds. In the United States 407 people died between 1995 and 2007 from trees that fell during heavy windstorms, tornadoes and cyclones mainly in urban environments (Schmidlin, 2009). There were between 2.26 and over 5 deaths per million inhabitants depending on the state. Other deaths and serious injuries can be caused by the obstacles rescue teams face due to blocked roads cut off by windthrown trees, and storm damage caused by falling trees or branches as well as by trees weakened during high winds, that collapse in the following weeks (Schmidlin, 2009). In Great Britain, 64% deaths or serious injuries caused by falling trees or parts of them during the decade 1999–2008 occurred in densely populated areas in a few days with wind gusts above 75 km/h (Ball and Watt, 2013). Several hundred or thousands of urban trees can be windfallen during violent windstorms or tornadoes (Jim and Liu, 1997; Duryea et al., 2007; Kane, 2008; Hallett et al., 2018; Lin et al., 2020).
In the countries more vulnerable to windstorm events like the United States, disaster risk reduction initiatives have been in place for some time to enhance disaster preparedness for response and anticipate extreme weather events with the aim of mitigating and managing their impact on urban communities (Doswell, 2003). This is not the case in Europe despite the fact that several important urban areas have been hit by windstorms and tornadoes which have caused severe damage to trees (Bocheva et al., 2018; Gross, 2018; Pipinato, 2018). Windstorm frequency and intensity has increased in recent decades (Spinoni et al., 2020) and the severity of these events is underestimated according to some authors (Doswell, 2015; Antonescu et al., 2017). A longitudinal study carried out in the Netherlands has shown how the annual incidence of accidents caused by falling trees in urban areas and the amount of compensation paid are increasing (van Haaften et al., 2016). Windstorms are included among the climate-related impacts to be considered when developing urban climate change adaptation plans for European cities, also due to the impacts they can have on urban forest (European Economic Area [EEA], 2020).
Constant tree care is essential for the health and stability of urban trees and along with replacing or restoring dead or decaying trees reduces the risk of them falling during a windstorm. However, wind speeds during extreme windstorm events are often so high that it is impossible to prevent urban trees from falling. Therefore, citizens and public decision-makers need to carefully evaluate windthrow and its consequences.
The aim of the research reported in this paper was to: (a) investigate the disservices that a windstorm can cause in an urban area with numerous trees, using a conceptual model; (b) conduct a literature review to determine how high the risk of these disservices occurring in Europe really is, as they are definitely more likely than commonly perceived and could increase as a result of climate change and the growing landscape urbanization and; (c) indicate what type of measures can be taken to prevent or reduce the risk of trees falling during high winds starting from the experience gained in the geographical areas most frequently and intensely affected by windstorms.
It is not the authors’ intention to bring into question the benefits of urban trees, especially those located near roads and buildings. However, it is important to note that even in European cities the decision makers responsible for the design and maintenance of green areas with trees must take due account of the problems that they can pose during extreme weather events such as windstorms and address these issues in order to maximize the benefits and minimize the possible, unavoidable disservices from a perspective in which risk prevention and risk management complement each other (Lyytimäki, 2015; Speak et al., 2018).
2. Development of the conceptual model
The consequences of urban tree falls can be defined as disservices as they have actual or perceived negative impacts on the residents’ wellbeing (Lyytimäki and Sipilä, 2009; Shackleton et al., 2016; Lyytimäki, 2017; Roman et al., 2021).
On closer inspection, the disservices caused by urban tree fall due to the wind are potentially numerous and varied and increase when many trees fall over short periods of time which may occur during violent windstorms. In a climate change scenario in which the frequency or intensity of extreme weather events can increase (European Economic Area [EEA], 2017; Spinoni et al., 2020), defining the set of disservices resulting from numerous simultaneously falling urban trees appears useful and appropriate in order to support European policymakers who will be repeatedly requested to: (i) raise public awareness of the risks associated with windthrown trees; (ii) establish priorities and urgency of intervention for the expansion and management of urban green areas in order to find a trade-off between the services and disservices provided by urban trees and; (iii) evaluate the possibility of including trees in the incident and emergency response plans developed to cope with extreme weather events. These initiatives are essential for Europe given the increased awareness of the real risks posed by tornadoes and other extreme wind phenomena which will be addressed in chapter 3.
Some authors have suggested using conceptual models to facilitate the incorporation of ecosystem services in public sector decision-making processes. Conceptual models link changes in biophysical environments caused by external factors to positive changes in peoples’ wellbeing who benefit from the system modification, and represent ecosystem services (Kelble et al., 2013; Potschin-Young et al., 2018; Olander et al., 2021). Using indicators capable of measuring social value, it is possible to evaluate the socio-economic effects of the changes that have taken place (Wainger and Mazzotta, 2011; Olander et al., 2017, 2018). To this aim, conceptual modeling was applied to map and measure the ecosystem disservices caused by numerous falling trees or large branches in an urban context during a windstorm.
In the general model shown in Figure 1, the biophysical system is composed of the trees present in an urban green infrastructure, in particular those located along roadways and footpaths or near buildings. The external disturbance event affecting the system is a windstorm with wind gusts above 80–90 km/h which can cause the fall of many trees and large branches regardless of their condition (Cullen, 2002). However, tree health may have already been compromised due to poor management or lack of management, root damage caused by road works and trenching or deterioration due to pollution and parasites. Therefore, trees mismanagement is treated in the model as a sort of wind multiplier to highlight the fact that some fallen trees could have survived if they had been well managed and cared for.

Figure 1. General conceptual model of the process that leads to the generation of ecosystem disservices due to the fall of trees in an urban system hit by a violent windstorm (see text for more details).
The detailed model (Figure 2) shows that the effects of urban tree fall are related to the ecosystem disservices generated, distinguishing the effects that occur when the trees fall and those that occur over time. The effects of disservices on the urban socio-economic system, which generate monetary costs for the community or private economic sectors, are also included in the model.
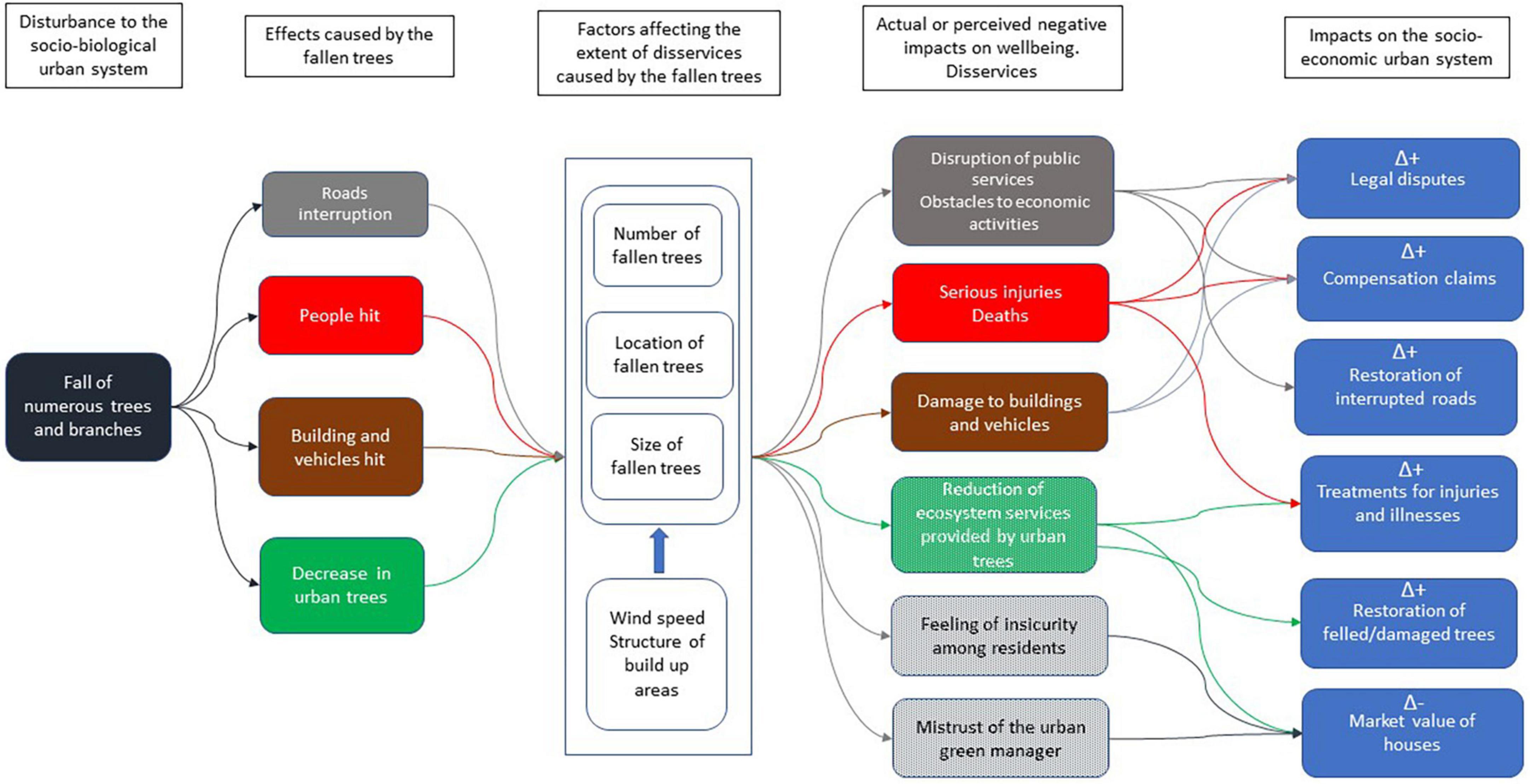
Figure 2. Detailed conceptual model of ecosystem disservices caused by the fall of numerous urban trees. The colors connect the different effects of falling trees with the generated disservices. In light gray boxes are the disservices attributable to all four effects and the extent of which will depend on the response of the urban green manager. The finely dotted background indicates that the disservice will manifest over the time following the disturbance event (see text for more details).
2.1. Immediate disservices
The model takes into account four main effects, represented in the graph in Figure 2 by different colors. Three of these effects have immediate consequences, namely, injuries to passersby, damages to physical assets such as buildings and vehicles and interruption of road sections (Figure 3). Fallen trees are one of the most common causes of death, injury and road network disruptions during hurricanes (Ashley and Black, 2008; Schmidlin, 2009; Rappaport, 2014; Vajda et al., 2014; Demiroluk and Ozbay, 2015; Choy et al., 2020; Ghorbanzadeh et al., 2020; Kakareko et al., 2020). If several roadblocks occur simultaneously in various stretches of the road network, especially along important traffic arteries, they can seriously hamper rescue operations during a storm as well as the resumption of social and economic activities in the following days, because of the time required to remove fallen trees and debris (Kocatepe et al., 2019). It is important to note that serious accidents can also occur when removing fallen trees (Marshall et al., 2018).
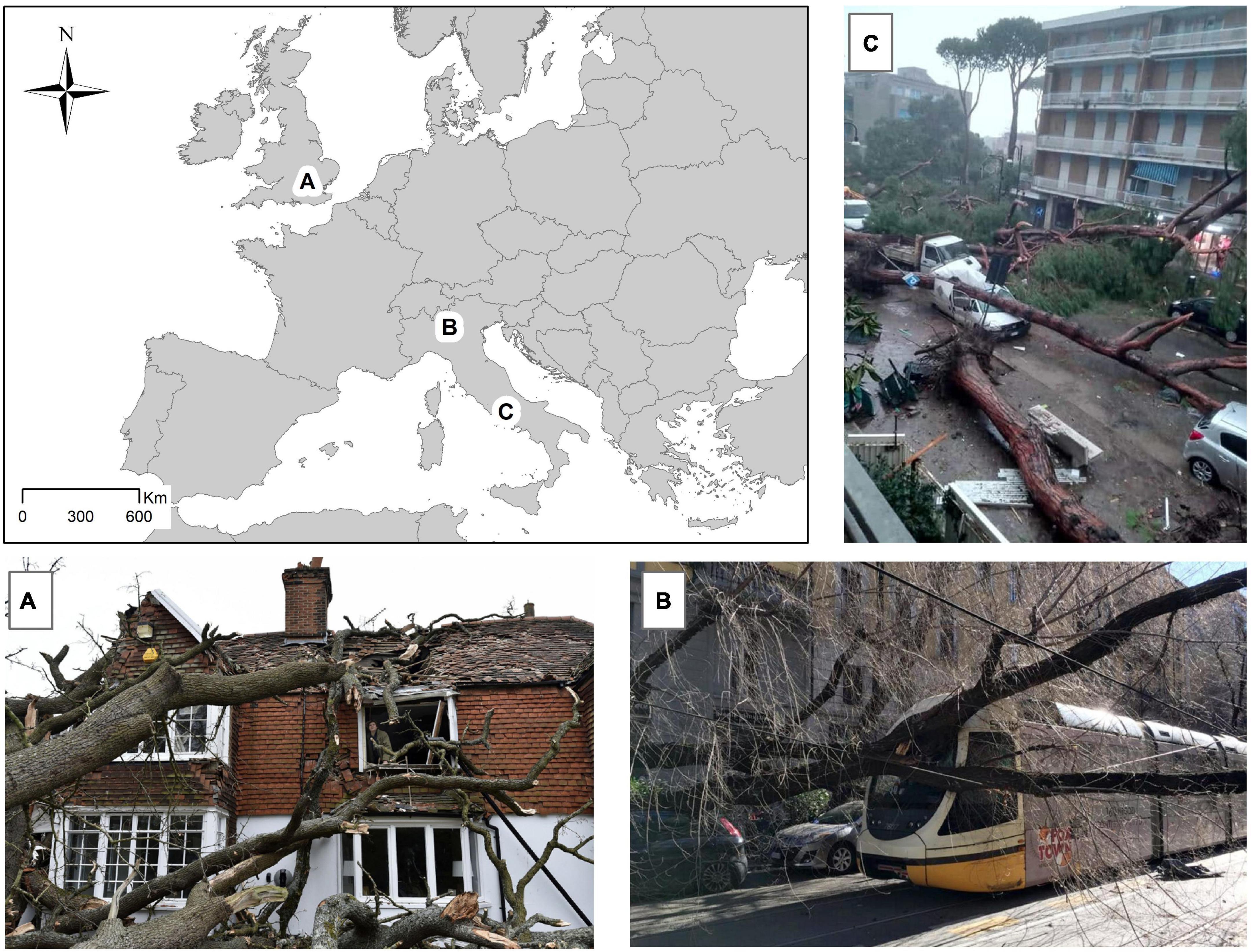
Figure 3. Different types of disservices caused by falling trees during heavy storms that hit European cities in the last years. (A) Extensive damage caused by a large oak that struck a building after being torn down by storm Eunice on 18 February 2022 in Great Britain (source: https://www.gulftoday.ae/news/2022/02/19/14-dead-as-storm-eunice-hits-power-and-transport-in-europe). (B) Public transport service disruption due to a collapsed tree on a tram during the storm of 7 February 2022 in Milan (source: https://tg24.sky.it/cronaca/2022/02/07/vento-milano-danni#23). (C) A road was interrupted and some cars crushed by several pines uprooted by the Adrian cyclone that landed on the Tyrrhenian coastal town of Terracina (Central Italy) on 29 October 2018 (source: https://www.latina24ore.it/provincia-2/149944/foto-tromba-aria-terracina-2018/).
2.2. Long-term disservices
There is a category of disservices that can cause indirect negative effects which only manifest themselves to their full extent after the event (shown by squares with fine gray and green dotted backgrounds in Figure 2). Fallen trees can no longer provide the ecosystem services they used to and a long time is required before new trees can provide the same benefits, especially those provided by large trees. A windstorm can seriously damage a city’s arboreal heritage and can consequently significantly reduce the amount of ecosystem services it provides. This reduction in the benefits provided by urban trees can be regarded as a disservice generated by the effects of the extreme weather event, which last for as long as it takes to replace the fallen trees and get them to reach the size of those felled by high wind.
Furthermore, a fallen tree is an event that can change the positive perception that residents have of urban trees and negatively affect their will to keep trees within the private green spaces near buildings (Conway and Yip, 2016). Residents’ perceptions of urban trees as providers of benefits are generally positive despite the issues they can cause and their ordinary maintenance costs (Schroeder et al., 2006; Camacho-Cervantes et al., 2014). However, peoples’ perceptions of urban trees may change if many people are affected by the disservices caused by numerous falling trees in a single day when compared to when the same number of trees fall over the course of several decades due to sporadic events affecting single plants (Conway and Yip, 2016).
The weight of this type of inefficiency depends on the response of the urban vegetation management, both immediately after the event and in the following period. The existence of an extreme weather prevention and response plan that includes trees as potential hazards and the ability to quickly remove and replace fallen trees and branches can mitigate people’s negative perceptions (Koeser et al., 2016; Hartel, 2019).
2.3. Intensity of disservices
The extent of damage and public nuisance strongly depends on the number of fallen trees, their size and location in the urban system, which in turn depends on the strength of the wind gusts (Francis and Gillespie, 1993). The structure of the built-up area can play an important role. The interactions between buildings and wind are complex and they can lead to barrier effects, channeling along surrounding roads and turbulence (Cole et al., 2006; Tamura et al., 2019; Tominaga and Shirzadi, 2021), thus protecting the trees that are downwind, or directing the wind toward specific roads or squares and ending up favoring the felling of the trees growing there (Gross, 2018; Giachetti et al., 2021).
The fall of a single tree is an event which may have serious consequences but only affects a certain small urban area, therefore the spatial extent of the disruptions caused to the urban system is minimal. It is similar to a minor trauma that affects a non-vital organ of an organism, which may be painful to experience but the injury is localized and will heal quickly. Contrastingly, when numerous trees fall in the same urban neighborhood simultaneously due to an extreme weather event, there are much greater long-lasting negative outcomes and issues.
Large fallen trees generally do the most damage as they have thicker stems and broader crowns. Medium-sized trees (20–40 cm in diameter) are those most likely to fall during hurricanes even to a greater extent than the weight this size class generally has in the urban forest; however, wind-thrown trees over 80 cm in diameter have also been recorded (Jim and Liu, 1997; Kane, 2008). Large trees are likely to lose important parts of the canopy therefore their health and stability are structurally compromised even if they survive the storm (Duryea et al., 2007).
As regards their position within the urban fabric, the trees that can create serious problems by falling during a windstorm are those located close to public buildings, such as schools and hospitals and along roads (Wu et al., 2020), while trees growing inside urban parks are much less risky, especially on stormy days when people are less likely to frequent green areas or are denied access as a precautionary measure.
2.4. Economic impact of disservices
In addition to the great inconvenience to people, windstorms that hit cities cause very large economic loss or financial damage that amount to billions of euros in the most serious cases (Klawa and Ulbrich, 2003; Bocheva et al., 2018; Choy et al., 2020). Fallen trees cause damage to people, buildings, vehicles and infrastructures, however, there is a lack of in-depth studies that measure their impact in money terms.
Furthermore, the simultaneous fall of hundreds of trees can have a significant economic impact on the urban system even after the windstorm. Firstly, councils have to cover the costs of clearing fallen trees, trunks, branches and foliage as well as the pruning or felling of remaining damaged trees and are therefore a threat to public safety. The amount of debris to be removed after a hurricane has been estimated at between 10 and 30 cubic meters per 100 m considering both sides of a tree-lined road, however, it can also reach 60 cubic meters per 100 m of road depending on tree cover density (Bloniarz et al., 2001; Escobedo et al., 2009). A study carried out in Houston County, Texas, following Hurricane Ike which affected areas with both urban and rural land use zones, led to an estimate of 28.9 m3 ha–1 of tree residues caused by the hurricane and between 5 and 6 million cubic meters of woody debris to be removed (Thompson et al., 2011). All of the studies examined agree that the characteristics of the tree cover (density, size, location of trees) exert a greater influence on the amount of debris than the storm that generated it.
In many countries the tree owner is held responsible for the damage that a fallen tree causes unless he can prove that he has routinely performed constant preventive maintenance or that the tree fell due to totally unpredictable weather events (Mortimer and Kane, 2004). For this reason, there has been an increase in legal disputes and compensation claims associated with urban trees falling during windstorm events in recent decades (van Haaften et al., 2016).
It is well-known the contribution of green infrastructures to ecosystem services provision: green areas in cities boost the physical health and mental wellbeing of the citizens by releasing oxygen into the air, removing pollutants (Gaglio et al., 2022; Muresan et al., 2022), favoring local cooling through shading and evapotranspiration (Speak et al., 2020; Pace et al., 2021), providing space for outdoor exercise, reducing stress and softening the urban landscape (Carrus et al., 2015, 2017; Tomao et al., 2016, 2018; Kondo et al., 2018; Ma et al., 2019). The sudden depletion of an urban forest caused by a windstorm can cause an increase in diseases and healthcare costs over time. It is also important to note that urban green spaces have a significant positive effect on house sale and rental prices (Donovan and Butry, 2011; Saphores and Li, 2012; Escobedo et al., 2015; Mei et al., 2018; Donovan et al., 2019). Therefore, the significant reduction of a city’s tree stock can have negative effects on the value of the houses located in the neighborhoods most affected by strong windstorms.
3. Windstorms in Europe
Convective storms accompanied by large hail, damaging wind gusts, tornadoes, flash flooding and non-convective high winds occur across Europe causing fatal injuries and considerable damage (Browning, 2004; Taszarek et al., 2020). The levels of storminess in Europe have increased over the last century, albeit with decadal-scale variability (Donat et al., 2011). However, there have also been contrasting scientific conclusions regarding extreme weather phenomena during the same period. Thanks to the studies of Alfred Wegener, a pan-European climatology of tornadoes had already been outlined in the first half of the twentieth century (Antonescu et al., 2019), while in the second half of the century, scientific interest was noticeably waning as tornadoes were deemed to be extremely rare phenomena, only capable of causing serious damage in other geographical areas of the planet such as the United States and the Far East (Antonescu et al., 2016, 2017; Yang et al., 2017).
A more realistic perception of the force and danger that high-impact meteorological phenomena can cause in Europe only occurred following the foundation of the ESSL (European Severe Storms Laboratory) in 2002 and the development of the ESWD (European Severe Weather Database) in which all of the extreme weather events reported by the media or by severe-weather spotter networks that have occurred in Europe since 1950 have been archived (Dotzek et al., 2009). These data showed that the number of extreme weather events reported annually is constantly increasing and there were over 12,000 in 2015 (Groenemeijer et al., 2017; Figure 4). From 2010 to 2018, 2625 weather events with wind speeds over 25 m/s (90 km/h), 1208 hailstorms with hailstone diameters of >2 cm and 232 tornadoes (excluding less intense watersprouts) were reported on average each year (Taszarek et al., 2020).
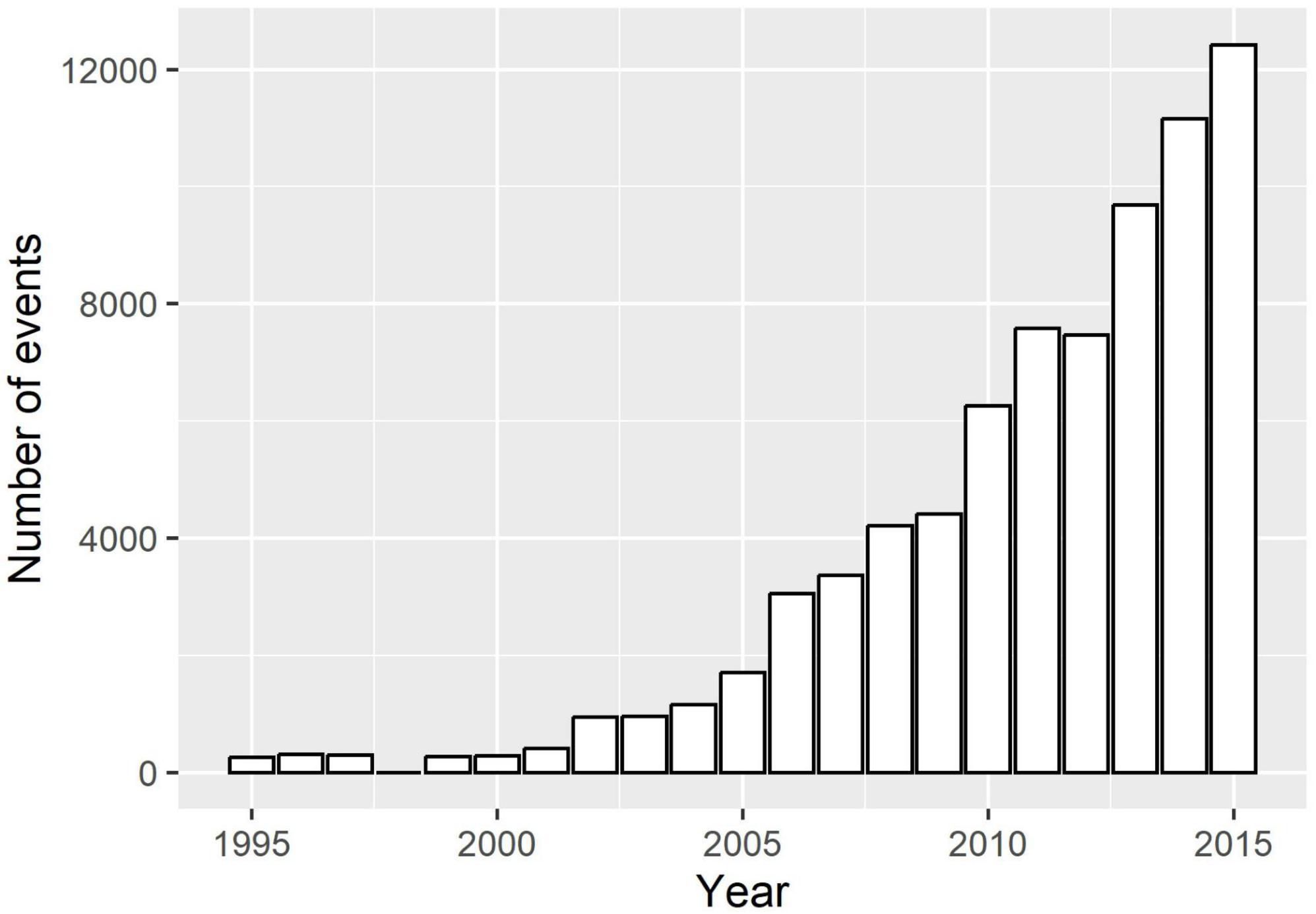
Figure 4. Number of severe convective storms (tornado, watersprout, severe wind, flash-flood and hail) reports in ESWD from 1995 to 2015 (adapted from Groenemeijer et al., 2017).
The most hazardous extreme weather events in Europe are undoubtedly tornadoes, which caused more than 4,400 injuries, 316 deaths and damage estimated at one billion euros between 1950 and 2015 (Antonescu et al., 2017). Most of these tornadoes were low intensity phenomena, classified as F0 according to the Fujita Tornado Scale (which includes six categories from F0 to F5; Cullen, 2002; see Supplementary Table 1), however, when only considering tornadoes that form on the mainland, the most harmful (classified from F2 to F5) represent 10% of the total number, which is the same percentage as in the United States (Dotzek et al., 2009). The ESWD data shows that no European region is completely unaffected by windstorms even by the most severe, although their frequency varies from country to country (Groenemeijer and Kühne, 2014; Antonescu et al., 2017).
In Europe most storms occur along the Mediterranean coasts and mountainous regions especially across the Italian peninsula, the eastern Adriatic coast and the southern side of the Alps (40–60 stormy days per year on average). Other regions with more than 35 stormy days per year are the foothills of the Pyrenees, the Atlas, the Carpathians, the Alps and central Europe. Damaging windstorms are less frequent in northern Europe (less than 10 stormy days per year). Similar geographical distribution shows that Mediterranean countries experience on average 15 to 30 particularly stormy days per year. Annual peak of thunderstorm activity occurs at different times of the year: during the spring-summer season in Central, Western and Eastern Europe and in the autumn-winter season in the Mediterranean area (Taszarek et al., 2019).
In the Mediterranean region tornadoes are often similar to tropical cyclones. According to some studies, climate change-induced rising sea temperatures could help to reduce the frequency of these events but significantly increase their intensity (Cavicchia et al., 2014). In addition, Dale et al. (2016) suggest that local, regional or global changes in temperatures and precipitation will probably affect the occurrence, timing, frequency, duration, extent, and intensity of disturbances, including localized “pulse events” such as extreme windstorms.
However, there is still a lot of uncertainty about the effect that global warming may have on the frequency and intensity of severe windstorms (Spinoni et al., 2020). It is difficult to define a historical data trend, as these are infrequent events. Models tend to extend the number of days in which favorable environmental conditions can be created to simulate an extreme weather event but doubts remain about how often these conditions will actually produce stormy weather and which type of storm event (tornadoes, windstorms, strong hail) will occur (Donat et al., 2011; Brooks, 2013; Diffenbaugh et al., 2013; Trapp and Hoogewind, 2016; Púčik et al., 2017; Allen, 2018; Rädler et al., 2019; Trapp et al., 2019; Spinoni et al., 2020; Taszarek et al., 2021). The main limitation of the models currently available is the low spatial and temporal resolution, which is unsuitable for predicting extreme weather events at a local scale. More information will be obtained from models created from more detailed datasets (in terms of spatio-temporal resolution) like the one obtained for Italy by dynamically downscaling the ERA5 reanalysis, originally available at ′31 km horizontal resolution, to ′2.2 km of resolution for the period 1989–2020 (Raffa et al., 2021).
Ultimately, extreme weather events certainly occur much more frequently in the United States than in Europe. However, severe weather phenomena such as hailstorms with hailstones up to 15 cm in diameter, wind speeds of 50 m/s or F4–F5 tornadoes occur in Europe too. These extreme weather events are relatively rare but what makes them particularly dangerous is the high level of social vulnerability in Europe considering the high population density, the high landscape urbanization level, the public’s lack of awareness that extreme weather events can occur and the lack of multi-hazard early warning systems like those operating in the United States (Ashley and Strader, 2016; Miglietta and Rotunno, 2016; Antonescu et al., 2018; Taszarek et al., 2020), eventually using web-GIS applications (Environmental Systems Research Institute [ESRI], 2014).
Even if the frequency and intensity of windstorms do not increase, economic growth will lead to an increase in urban density and the expansion of built-up areas and will consequently result in an increase in storm damages which are estimated to reach 11 billion euros per year throughout the 21st century (Hoeppe, 2016; Spinoni et al., 2020). In particular, an increase in vulnerability to hazards can be found along the urban fringe of development, where low-density settlements are established with the largest population changes (Hall and Ashley, 2008). These “sprawl areas” are very common in Europe and have increased especially around the Mediterranean basin (Salvati et al., 2013), making this area particularly vulnerable to potential damages. The relative population stability that currently characterizes European society could keep the number of people exposed to windstorms constant and therefore the number of victims, unless measures are adopted that make the territory and urban systems more resilient to extreme weather events (European Economic Area [EEA], 2020; Spinoni et al., 2020), which is a significant challenge for urban forest planners and stakeholders.
4. Implications for urban forestry in European cities
Strong European windstorms also affect cities, although less frequently than other geographic areas. Therefore, most European citizens believe that in their lifetime the city in which they live will not be affected by an extreme weather event that causes the fall of tens or hundreds of urban trees with the consequences highlighted in chapter 2 (this attitude is named normalcy bias; Doswell, 2015). This low risk perception leads us to underestimate hazards and makes us less prepared to act quickly and efficiently in emergencies.
However, urban trees provide numerous health benefits, so they cannot be felled simply to avoid the disruptions they can cause during rare severe weather events. We also don’t underestimate the benefits provided by trees when they are located along streets and near public and private buildings.
The increasing availability of information on the frequency, intensity and geographical location of the various types of European windstorms is a starting point for reducing the risk of urban trees causing injury to people and damage to property during extreme weather events. If we are aware of what can happen, appropriate measures can be taken to reduce the impact of potential consequences and be prepared (Table 1).
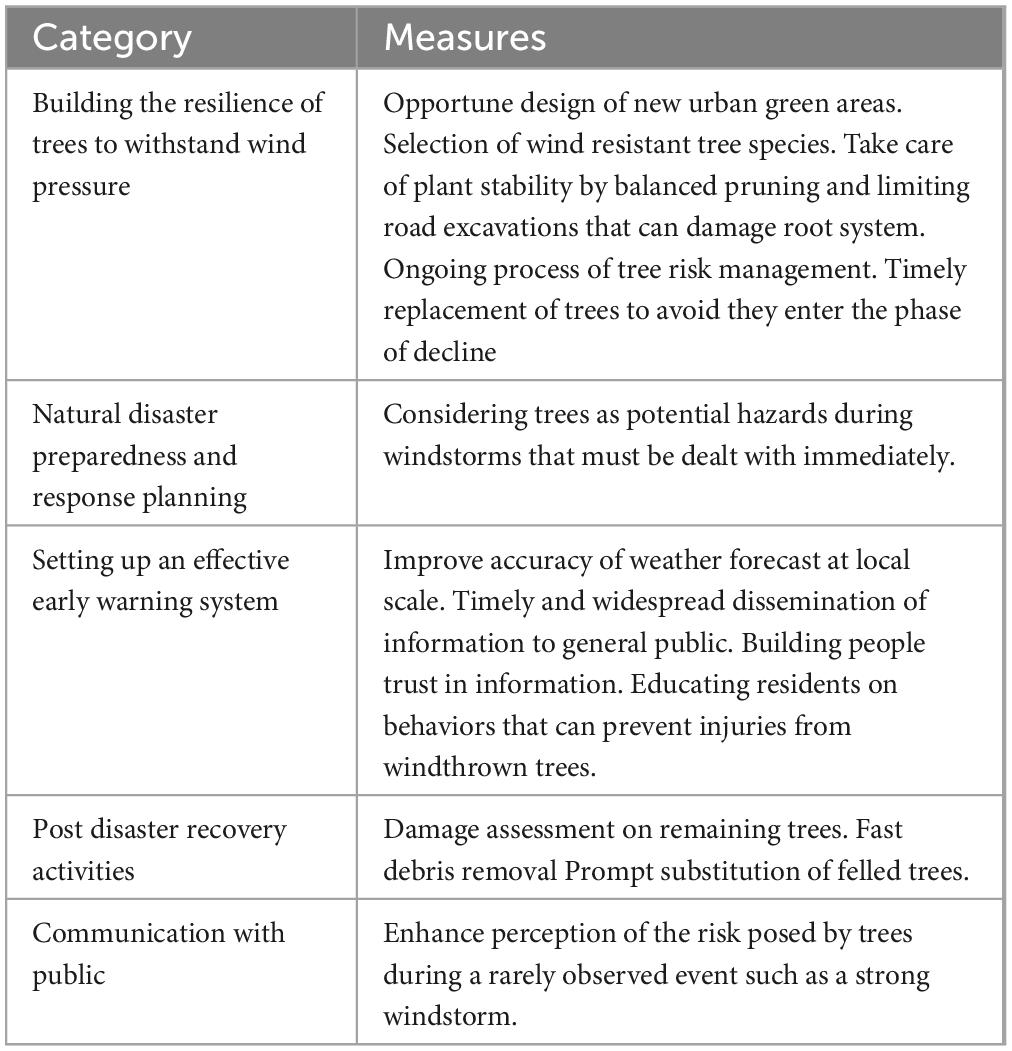
Table 1. Measures that can prevent or limit disservices created by the fall of urban trees during a severe windstorm in European cities.
North America and Southeast Asia have long been experiencing tropical storms and extreme weather, and mitigation strategies have been developed to reduce the effects of heavy windstorms. These strategies include urban forest management measures aimed at building the resilience of trees to withstand wind pressure and response plans for severe weather events, which include trees among the essential urban landscape elements that require emergency interventions.
Research conducted in the United States have shown that wind-related tree failure during hurricanes depends on various factors. In addition to what can be defined as triggering factors (wind speed, intensity and duration of rainfall), there are also biological factors (tree species, age and size, state of health), site specific conditions (soil quality, space available for the roots), structural and management factors of the urban forest (arrangement of trees in groups or isolated, species diversity, pruning, root damage due to excavations) that can play certain roles (Moore, 2014). Similarly, a study on the damage caused to an urban forest by typhoons that hit the cities of the Pearl River Delta in Southeast China showed that wind speed, tree species, tree health conditions and the characteristics of the urban space where the trees grow, are all contributing factors (Lin et al., 2020; Xu et al., 2021).
It is essential to take the above-mentioned factors into consideration when designing or maintaining a new urban green area in order to improve the urban forest’s resilience to high winds (Jim and Liu, 1997). In particular, wind-resistant tree species should be selected when designing and planting trees in urban green spaces or when choosing replacement species. Studies have long been carried out in Florida to identify the most appropriate species by analyzing the damage caused by windstorms (Duryea et al., 1996, 2007; Duryea and Kampf, 2007). In North America, information on the tree species most susceptible to windthrow in the most temperate climates has also been provided (Foran et al., 2015), while similar conclusions cannot be drawn for cities with boreal climate due to the rarity of violent windstorms (Wiersma et al., 2012). This research should also be carried out for the most exposed European urban contexts similarly to studies previously conducted in Great Britain and Ireland for the rural and forest context (Cutler et al., 1990; Everham and Brokaw, 1996). It would also be useful to gain a better understanding of methods for predicting the growth rate and longevity of trees in an European urban environment (see e.g., Sanders et al., 2013 for the USA), which differ from those growing in natural forest. This would provide arborists and green area managers with suitable parameters to determine the moment in which an urban tree, based on the tree species and the type of site where it has grown, enters the phase of decline (sensu Sinclair and Hudler, 1998 in Sanders et al., 2013) and must be removed before it becomes a threat to human life and property during a windstorm.
Proper urban green area management that promotes tree health is essential to make urban forests better prepared to deal with natural disasters (Koeser et al., 2020). However, regardless of how they are managed, urban trees remain exposed to damage from intense windstorms. For this reason, when preparing tornado preparedness and response plans, many cities in the United States, especially medium and large sized municipalities, consider trees as potential hazards that must be dealt with immediately (Koeser et al., 2016).
The effectiveness of a response plan can be enhanced by early warning systems. However, the experience acquired by the USA since the 1950s shows that the effectiveness of early warning systems does not only depend on weather forecast accuracy and their timely and widespread dissemination to the general public. Trust in public health officials and the information they provide as well as disaster preparedness initiatives are essential to protect the public from the risks posed by falling or windthrown trees (Doswell, 2015). The implementation of these initiatives is often problematic and poses many challenges to numerous European and non-European National Meteorological and Hydrological Services and Civil Protection services who must ensure that people living in areas affected by extreme weather events have a heightened awareness of the potential risks of the upcoming event (Rauhala and Schultz, 2009). A positive aspect is that many European countries have gradually transitioned toward using early warning systems based on the potential impact of the event, i.e., on the damage that an upcoming storm is capable of causing and on the behaviors to adopt, rather than the exceeding of climate thresholds (Kaltenberger et al., 2020).
When a windstorm has occurred a visual damage assessment of remaining trees must be promptly conducted to ensure that they are safe and that overall risk to the public from falling trees is negligible, the debris must be removed and tree maintenance must be carried out to save the recoverable plants in order to ensure the continuity of urban forest-based ecosystem services. To this end, Urban Forests Strike Teams, composed of state forestry agency certified arborists and urban foresters, are trained to provide tree damage and risk assessments following natural disasters and operate in the southwestern United States (Hartel, 2019).
As well as routine urban tree maintenance, preventing and mitigating the impacts of a catastrophic windstorm in a city also requires communication. It is important that an unstable tree is perceived as such by administrators and citizens not only when it is deemed unhealthy by an arborist but also in terms of the disservices that trees cause when they fall due to high winds during extreme weather events (Hauer, 2014; Koeser et al., 2016). Using standardized procedures such as QTRA, it is possible to quantify the extent of damage associated with the fall of a tree not only on the basis of its state of health but also considering the value of what it can hit by falling and the potential impact (Ellison, 2005; Stewart et al., 2013). These quantitative assessments strike a more accurate balance between the benefits that cutting down a tree bring in terms of risk and damage reduction and the costs for achieving it, not only the economic ones but also in terms of reduction of the services provided by the tree.
According to Starr (1969), community risk perceptions associated with unfrequently observed events tend to be low. Effective communication and information sharing is essential between experts, decision makers and the general public regarding the potential risks associated with the presence of trees in certain locations, especially if they belong to a vulnerable species and are large in size. Transparency and public engagement are required to avoid triggering negative reactions when a tree is cut down for safety reasons in the context of preventive maintenance.
5. Conclusions
One of the emerging issue on the urban policy agenda in Europe is how to make cities more resilient to climate change. A specific question is mitigating the effect of extreme weather events as heatwaves and floodings are expected to be more frequent and intense in the next decades. Green infrastructure, particularly urban trees, are considered part of the solution. However, even severe windstorms should become much less exceptional occurrence than perceived by European citizens today due to global warming. In fact, despite there is still uncertainty about the effect that global warming may have on severe windstorms, climate extremes are expected to intensify, thus increasing intensity and frequency of threats to inhabitants and property. In addition, urbanization expansion will likely increase potential targets of extreme events and consequently storm-related disservices.
When winds reach speeds in excess of 90 km/h and affect the trees along roads and near buildings in urban and peri-urban areas they may cause the multiple ecosystem disservices highlighted by the conceptual model used in this research, which may have serious social and economic impacts on the wellbeing of urban residents. This issue will need to be taken into account when undertaking EU policy initiatives such as the preparation of climate change adaptation plans for the urban environment which should include the measures shown in Table 1.
There are no geographic areas in Europe that are not potentially affected by windstorms. If the process of urbanization of the landscape continues as currently envisaged, the value of the damage caused by severe storms is also destined to increase as well as the number of victims. For this reason, greater cooperation among all European countries and common investments in research and development on the topics covered in this article are desirable. The experience gained in the non-European geographical areas that have been dealing with these issues for some time should also be a vital reference source for European cities. Likewise, responsible business managers of companies operating in areas affected by high-impact, low-frequency natural disasters can benefit from the experiences of others to better prepare for disasters that can have significant effects on their companies (Oetzel and Oh, 2021).
Preparing for future events that may never happen is a major challenge for policymakers, urban planners, and managers. However, the continuity of the ecosystem services provided by urban trees will depend on the city’s ability to cope with emergencies which are rare but can cause injury and damage. The consequences in terms of loss of tree benefits can be substantial and have long-term adverse effects.
It is impossible to eliminate the risks of windstorms but being aware of them and mitigating the damage they cause by making responsible decisions is the best way to make urban forests safer.
Author contributions
LP: conceptualization, data curation, resources, writing—original draft, and project administration. EM: methodology, resources, and writing—review and editing. AT: conceptualization, resources, writing—review and editing, and supervision. MA: conceptualization, supervision, writing—original draft, project administration, and funding acquisition. All authors contributed to the article and approved the submitted version.
Funding
This research was funded by the MIUR project “EUFORICC” – Establishing Urban Forest Based Solutions in Changing Cities (PRIN 20173RRN2S: “Projects of National Interest”).
Conflict of interest
The authors declare that the research was conducted in the absence of any commercial or financial relationships that could be construed as a potential conflict of interest.
Publisher’s note
All claims expressed in this article are solely those of the authors and do not necessarily represent those of their affiliated organizations, or those of the publisher, the editors and the reviewers. Any product that may be evaluated in this article, or claim that may be made by its manufacturer, is not guaranteed or endorsed by the publisher.
Supplementary material
The Supplementary Material for this article can be found online at: https://www.frontiersin.org/articles/10.3389/ffgc.2023.1155016/full#supplementary-material
References
Allen, J. T. (2018). Climate change and severe thunderstorms. Oxford: Oxford University Press, doi: 10.1093/acrefore/9780190228620.013.62
Anguluri, R., and Narayanan, P. (2017). Role of green space in urban planning: Outlook towards smart cities. Urban For. Urban Green. 25, 58–65. doi: 10.1016/j.ufug.2017.04.007
Antonescu, B., Fairman, J. G., and Schultz, D. M. (2018). What is the worst that could happen? Reexamining the 24-25 June 1967 tornado outbreak over western Europe. Weather Clim. Soc. 10, 323–340. doi: 10.1175/WCAS-D-17-0076.1
Antonescu, B., Ricketts, H. M. A. M., and Schultz, D. M. (2019). 100 years later: Reflecting on Alfred Wegener’s contributions to tornado research in Europe. Bull. Am. Meteorol. Soc. 100, 567–578. doi: 10.1175/BAMS-D-17-0316.1
Antonescu, B., Schultz, D. M., Holzer, A., and Groenemeijer, P. (2017). Tornadoes in Europe: An underestimated threat. Bull. Am. Meteorol. Soc. 98, 713–728. doi: 10.1175/BAMS-D-16-0171.1
Antonescu, B., Schultz, D. M., Lomas, F., and Kühne, T. (2016). Tornadoes in Europe: Synthesis of the observational datasets. Mon. Weather Rev. 144, 2445–2480. doi: 10.1175/MWR-D-15-0298.1
Ashley, W. S., and Black, A. W. (2008). Fatalities associated with nonconvective high-wind events in the United States. J. Appl. Meteorol. Climatol. 47, 717–725. doi: 10.1175/2007JAMC1689.1
Ashley, W. S., and Strader, S. M. (2016). Recipe for disaster how the dynamic ingredients of risk and exposure are changing the tornado disaster landscape. Bull. Am. Meteorol. Soc. 97, 817–820. doi: 10.1175/BAMS-D-15-00150.1
Ball, D. J., and Watt, J. (2013). The risk to the public of tree fall. J. Risk Res. 16, 261–269. doi: 10.1080/13669877.2012.737827
Bloniarz, D. V., Ryan, H. D. P. III., Luley, C. J., Bond, J., and Hawkins, D. C. (2001). An initial storm damage assessment protocol for urban and community forests. Amherst, MA: University of Massachusetts.
Bocheva, L., Dimitrova, T., Penchev, R., Gospodinov, I., and Simeonov, P. (2018). Severe convective supercell outbreak over western Bulgaria on July 8, 2014. Idojaras 122, 177–203. doi: 10.28974/idojaras.2018.2.5
Brooks, H. E. (2013). Severe thunderstorms and climate change. Atmos. Res. 123, 129–138. doi: 10.1016/j.atmosres.2012.04.002
Browning, K. A. (2004). The sting at the end of the tail: Damaging winds associated with extratropical cyclones. Q. J. R. Meteorol. Soc. 130, 375–399. doi: 10.1256/qj.02.143
Calfapietra, C., Morani, A., Sgrigna, G., Di Giovanni, S., Muzzini, V., Pallozzi, E., et al. (2016). Removal of ozone by urban and peri-urban forests: Evidence from laboratory, field, and modeling approaches. J. Environ. Qual. 45, 224–233. doi: 10.2134/jeq2015.01.0061
Camacho-Cervantes, M., Schondube, J. E., Castillo, A., and MacGregor-Fors, I. (2014). How do people perceive urban trees? Assessing likes and dislikes in relation to the trees of a city. Urban Ecosyst. 17, 761–773. doi: 10.1007/s11252-014-0343-6
Carrus, G., Scopelliti, M., Lafortezza, R., Colangelo, G., Ferrini, F., Salbitano, F., et al. (2015). Go greener, feel better? The positive effects of biodiversity on the well-being of individuals visiting urban and peri-urban green areas. Landsc. Urban Plan. 134, 221–228. doi: 10.1016/j.landurbplan.2014.10.022
Carrus, G., Scopelliti, M., Panno, A., Lafortezza, R., Colangelo, G., Pirchio, S., et al. (2017). A different way to stay in touch with “Urban Nature”: The perceived restorative qualities of botanical gardens. Front. Psychol. 8:914. doi: 10.3389/fpsyg.2017.00914
Cavicchia, L., Von Storch, H., and Gualdi, S. (2014). Mediterranean tropical-like cyclones in present and future climate. J. Clim. 27, 7493–7501. doi: 10.1175/JCLI-D-14-00339.1
Celikuilmaz-Ademir, G. (2010). “Cities and urban regions facing storms,” in Urban regions: Vulnerabilities, vulnerability assessments by indicators and adaptation options for climate change impacts. Scoping study. ETC/ACC Technical paper 2010/2012, I. Schauser ed.
Choy, C., Wu, M., and Lee, T. (2020). Assessment of the damages and direct economic loss in Hong Kong due to Super Typhoon Mangkhut in 2018. Trop. Cyclone Res. Rev. 9, 193–205. doi: 10.1016/j.tcrr.2020.11.001
Cole, T., Xiangyi, L., Eising, C., and Princevac, M. (2006). “Turbulence and channelling in a simple urban environment,” in 17th symposium on boundary layers an turbolence (San Diego, CA: American Meteorological Society).
Conway, T. M., and Yip, V. (2016). Assessing residents’ reactions to urban forest disservices: A case study of a major storm event. Landsc. Urban Plan. 153, 1–10. doi: 10.1016/j.landurbplan.2016.04.016
Cullen, S. (2002). Trees and wind: Wind scales and speeds. Arboric. Urban For. 28, 237–242. doi: 10.48044/jauf.2002.036
Cutler, D. F., Gasson, P. E., and Farmer, M. C. (1990). The wind blown tree survey: Analysis of results. Arboric. J. 14, 265–286. doi: 10.1080/03071375.1990.9746846
Dale, V. H., Hughes, M. J., and Hayes, D. J. (2016). “Climate change and the future of natural disturbances in the central hardwood region,” in Natural disturbances and historic range of variation: Type, frequency, severity, and post-disturbance structure in central hardwood forests USA, eds C. H. Greenberg and B. S. Collins (Cham: Springer International Publishing), 355–369. doi: 10.1007/978-3-319-21527-3_13
Demiroluk, S., and Ozbay, K. (2015). “Bayesian spatial modeling and risk mapping of downed trees along the roadways using data from hurricanes irene and sandy,” in Proceedings of the transportation research board 94th annual meeting, Washington, DC, 1–23.
Dickinson, D. C., and Hobbs, R. J. (2017). Cultural ecosystem services: Characteristics, challenges and lessons for urban green space research. Ecosyst. Serv. 25, 179–194. doi: 10.1016/j.ecoser.2017.04.014
Diffenbaugh, N. S., Scherer, M., and Trapp, R. J. (2013). Robust increases in severe thunderstorm environments in response to greenhouse forcing. Proc. Natl. Acad. Sci. U.S.A. 110, 16361–16366. doi: 10.1073/pnas.1307758110
Donat, M. G., Renggli, D., Wild, S., Alexander, L. V., Leckebusch, G. C., and Ulbrich, U. (2011). Reanalysis suggests long-term upward trends in European storminess since 1871. Geophys. Res. Lett. 38:L14703. doi: 10.1029/2011GL047995
Donovan, G. H., and Butry, D. T. (2011). The effect of urban trees on the rental price of single-family homes in Portland, Oregon. Urban For. Urban Green. 10, 163–168. doi: 10.1016/j.ufug.2011.05.007
Donovan, G. H., Landry, S., and Winter, C. (2019). Urban trees, house price, and redevelopment pressure in Tampa, Florida. Urban For. Urban Green. 38, 330–336. doi: 10.1016/j.ufug.2019.01.014
Doswell, C. A. (2003). Societal impacts of severe thunderstorms and tornadoes: Lessons learned and implications for Europe. Atmos. Res. 67–68, 135–152. doi: 10.1016/S0169-8095(03)00048-6
Doswell, C. A. (2015). Severe convective storms in the European societal context. Atmos. Res. 158–159, 210–215. doi: 10.1016/j.atmosres.2014.08.007
Dotzek, N., Groenemeijer, P., Feuerstein, B., and Holzer, A. M. (2009). Overview of ESSL’s severe convective storms research using the European severe weather database ESWD. Atmos. Res. 93, 575–586. doi: 10.1016/j.atmosres.2008.10.020
Duryea, M. L., and Kampf, E. (2007). Wind and trees: Lessons learned from hurricanes. EDIS 2007, 1–16. doi: 10.32473/edis-fr173-2007
Duryea, M. L., Blakeslee, G. M., Hubbard, W. G., and Vasquez, R. A. (1996). Wind and trees: A survey of homeowners after hurricane Andrew. J. Arboric. 22, 44–49. doi: 10.48044/jauf.1996.006
Duryea, M. L., Kampf, E., Littell, R. C., and Rodríguez-Pedraza, C. D. (2007). Hurricanes and the urban forest: II. Effects on tropical and subtropical tree species. Arboric. Urban For. 33, 98–112. doi: 10.48044/jauf.2007.011
Ellison, M. J. (2005). Quantified tree risk assessment used in the management of amenity trees. J. Arboric. 31, 57–65. doi: 10.48044/jauf.2005.007
Environmental Systems Research Institute [ESRI] (2014). Urban forest app makes cities storm ready. Available online at: https://www.esri.com/about/newsroom/arcwatch/urban-forest-app-makes-cities-storm-ready/ (accessed March 4, 2023).
Escobedo, F. J., Adams, D. C., and Timilsina, N. (2015). Urban forest structure effects on property value. Ecosyst. Serv. 12, 209–217. doi: 10.1016/j.ecoser.2014.05.002
Escobedo, F. J., Luley, C. J., Bond, J., Staudhammer, C., and Bartel, C. (2009). Hurricane debris and damage assessment for Florida urban forests. Arboric. Urban For. 35, 100–106. doi: 10.48044/jauf.2009.018
European Economic Area [EEA] (2017). Climate change adaptation and disaster risk reduction in Europe. Enhancing coherence of the knowledge base, policies and practices. Brussels: European Economic Area.
European Economic Area [EEA] (2020). Urban adaptation in Europe: How cities and towns respond to climate change. Brussels: European Economic Area.
Evans, D. L., Falagán, N., Hardman, C. A., Kourmpetli, S., Liu, L., Mead, B. R., et al. (2022). Ecosystem service delivery by urban agriculture and green infrastructure – a systematic review. Ecosyst. Serv. 54:101405. doi: 10.1016/j.ecoser.2022.101405
Everham, E. M., and Brokaw, N. V. L. (1996). Forest damage and recovery from catastrophic wind. Bot. Rev. 62, 113–185. doi: 10.1007/BF02857920
Foran, C. M., Baker, K. M., Narcisi, M. J., and Linkov, I. (2015). Susceptibility assessment of urban tree species in Cambridge, MA, from future climatic extremes. Environ. Syst. Decis. 35, 389–400. doi: 10.1007/s10669-015-9563-4
Francis, J., and Gillespie, A. (1993). Relating gust speed to tree damage in hurricane hugo, 1989. Arboric. Urban For. 19, 368–373. doi: 10.48044/jauf.1993.057
Gaglio, M., Pace, R., Muresan, A. N., Grote, R., Castaldelli, G., Calfapietra, C., et al. (2022). Species-specific efficiency in PM2.5 removal by urban trees: From leaf measurements to improved modeling estimates. Sci. Total Environ. 844:157131. doi: 10.1016/j.scitotenv.2022.157131
Ghorbanzadeh, M., Koloushani, M., Ulak, M. B., Ozguven, E. E., and Arghandeh, R. (2020). Statistical and spatial analysis of Hurricane-induced roadway closures and power outages. Energies 13:1098. doi: 10.3390/en13051098
Giachetti, A., Ferrini, F., and Bartoli, G. (2021). A risk analysis procedure for urban trees subjected to wind- or rainstorm. Urban For. Urban Green. 58:126941. doi: 10.1016/j.ufug.2020.126941
Gómez-Baggethun, E., and Barton, D. N. (2013). Classifying and valuing ecosystem services for urban planning. Ecol. Econ. 86, 235–245. doi: 10.1016/j.ecolecon.2012.08.019
Govindarajulu, D. (2014). Urban green space planning for climate adaptation in Indian cities. Urban Clim. 10, 35–41. doi: 10.1016/j.uclim.2014.09.006
Groenemeijer, P., and Kühne, T. (2014). A Climatology of tornadoes in Europe: Results from the European severe weather database. Mon. Weather Rev. 142, 4775–4790. doi: 10.1175/MWR-D-14-00107.1
Groenemeijer, P., Púcik, T., Holzer, A. M., Antonescu, B., Riemann-Campe, K., Schultz, D. M., et al. (2017). Severe convective storms in Europe: Ten years of research and education at the European severe storms laboratory. Bull. Am. Meteorol. Soc. 98, 2641–2651. doi: 10.1175/BAMS-D-16-0067.1
Gross, G. (2018). A windthrow model for urban trees with application to storm “Xavier.”. Meteorol. Zeitschrift 27, 299–308. doi: 10.1127/metz/2018/0905
Haase, D., Larondelle, N., Andersson, E., Artmann, M., Borgström, S., Breuste, J., et al. (2014). A quantitative review of urban ecosystem service assessments: Concepts, models, and implementation. Ambio 43, 413–433. doi: 10.1007/s13280-014-0504-0
Hall, S. G., and Ashley, W. S. (2008). Effects of urban sprawl on the vulnerability to a significant tornado impact in northeastern Illinois. Nat. Hazards Rev. 9, 209–219. doi: 10.1061/(ASCE)1527-698820089:4(209)
Hallett, R., Johnson, M. L., and Sonti, N. F. (2018). Assessing the tree health impacts of salt water flooding in coastal cities: A case study in New York City. Landsc. Urban Plan. 177, 171–177. doi: 10.1016/j.landurbplan.2018.05.004
Hartel, D. R. (2019). “Disaster recovery steps to maintain and improve urban forest resilience,” in Green readiness, response, recover: A collaborative synthesis, eds L. K. Campbell, E. Svendsen, N. F. Sonti, S. J. Hines, and D. Maddox (Newtown Square, PA: U.S. Department of Agriculture, Forest Service), 220–233.
Hauer, R. (2014). Trees and ice storms: Developing storm-resistant urban tree populations. Arborist News 23, 32–35.
Hoeppe, P. (2016). Trends in weather related disasters - Consequences for insurers and society. Weather Clim. Extrem. 11, 70–79. doi: 10.1016/j.wace.2015.10.002
Janhäll, S. (2015). Review on urban vegetation and particle air pollution - Deposition and dispersion. Atmos. Environ. 105, 130–137. doi: 10.1016/j.atmosenv.2015.01.052
Jim, C. Y., and Liu, H. H. T. (1997). Storm damage on urban trees in Guangzhou, China. Landsc. Urban Plan. 38, 45–59. doi: 10.1016/S0169-2046(97)00018-2
Johnson, E. A., and Miyanishi, K. (2020). Disturbance and succession. Plant Disturb. Ecol. 10, 1–15. doi: 10.1016/B978-0-12-818813-2.00001-0
Kabisch, N., Frantzeskaki, N., Pauleit, S., Naumann, S., Davis, M., Artmann, M., et al. (2016). Nature-based solutions to climate change mitigation and adaptation in urban areas: Perspectives on indicators, knowledge gaps, barriers, and opportunities for action. Ecol. Soc. 21:39. doi: 10.5751/ES-08373-210239
Kakareko, G., Jung, S., and Ozguven, E. E. (2020). Estimation of tree failure consequences due to high winds using convolutional neural networks. Int. J. Remote Sens. 41, 9039–9063. doi: 10.1080/01431161.2020.1797219
Kaltenberger, R., Schaffhauser, A., and Staudinger, M. (2020). What the weather will do-results of a survey on impact-oriented and impact-based warnings in European NMHSs. Adv. Sci. Res. 17, 29–38. doi: 10.5194/asr-17-29-2020
Kane, B. (2008). Tree failure following a windstorm in Brewster, Massachusetts, USA. Urban For. Urban Green. 7, 15–23. doi: 10.1016/j.ufug.2007.11.001
Kelble, C. R., Loomis, D. K., Lovelace, S., Nuttle, W. K., Ortner, P. B., Fletcher, P., et al. (2013). The EBM-DPSER conceptual model: Integrating ecosystem services into the DPSIR framework. PLoS One 8:e70766. doi: 10.1371/journal.pone.0070766
Klawa, M., and Ulbrich, U. (2003). A model for the estimation of storm losses and the identification of severe winter storms in Germany. Nat. Hazards Earth Syst. Sci. 3, 725–732. doi: 10.5194/nhess-3-725-2003
Kocatepe, A., Ulak, M. B., Kakareko, G., Ozguven, E. E., Jung, S., and Arghandeh, R. (2019). Measuring the accessibility of critical facilities in the presence of hurricane-related roadway closures and an approach for predicting future roadway disruptions. Nat. Hazards 95, 615–635. doi: 10.1007/s11069-018-3507-5
Koeser, A. K., Hauer, R. J., Hillman, A., and Peterson, W. (2016). Risk and storm management operations in the United States: How does your city compare? Arborist News 25, 20–23.
Koeser, A. K., Thomas Smiley, E., Hauer, R. J., Kane, B., Klein, R. W., Landry, S. M., et al. (2020). Can professionals gauge likelihood of failure? – Insights from tropical storm Matthew. Urban For. Urban Green. 52:126701. doi: 10.1016/j.ufug.2020.126701
Kondo, M. C., Fluehr, J. M., McKeon, T., and Branas, C. C. (2018). Urban green space and its impact on human health. Int. J. Environ. Res. Public Health 15:445. doi: 10.3390/ijerph15030445
Lin, H., Huang, S., Gu, Y., Chen, Z., Lu, Y., Yin, S., et al. (2020). Risk assessments of trees and urban spaces under attacks of typhoons: A survey and statistical study in Guangzhou, China. IOP Conf. Ser. Earth Environ. Sci. 588:032055. doi: 10.1088/1755-1315/588/3/032055
Linhares, C. S. F., Gonçalves, R., Martins, L. M., and Knapic, S. (2021). Structural stability of urban trees using visual and instrumental techniques: A review. Forests 12:1752. doi: 10.3390/f12121752
Livesley, S. J., McPherson, E. G., and Calfapietra, C. (2016). The urban forest and ecosystem services: Impacts on urban water, heat, and pollution cycles at the tree, street, and city scale. J. Environ. Qual. 45, 119–124. doi: 10.2134/jeq2015.11.0567
Luederitz, C., Brink, E., Gralla, F., Hermelingmeier, V., Meyer, M., Niven, L., et al. (2015). A review of urban ecosystem services: Six key challenges for future research. Ecosyst. Serv. 14, 98–112. doi: 10.1016/j.ecoser.2015.05.001
Lyytimäki, J. (2015). Ecosystem disservices: Embrace the catchword. Ecosyst. Serv. 12:136. doi: 10.1016/j.ecoser.2014.11.008
Lyytimäki, J. (2017). “Disservices of urban trees,” in Routledge handbook urban forestry, eds F. Francesco, K. van den Bosch Cecil, and F. Alessio (Oxford: Routledge), 164–176. doi: 10.4324/9781315627106-12
Lyytimäki, J., and Sipilä, M. (2009). Hopping on one leg - The challenge of ecosystem disservices for urban green management. Urban For. Urban Green. 8, 309–315. doi: 10.1016/j.ufug.2009.09.003
Ma, B., Zhou, T., Lei, S., Wen, Y., and Htun, T. T. (2019). Effects of urban green spaces on residents’ well-being. Environ. Dev. Sustain. 21, 2793–2809. doi: 10.1007/s10668-018-0161-8
Marshall, E. G., Lu, S. E., Williams, A. O., Lefkowitz, D., and Borjan, M. (2018). Tree-related injuries associated with response and recovery from hurricane sandy, New Jersey, 2011-2014. Public Health Rep. 133, 266–273. doi: 10.1177/0033354918766871
Mei, Y., Hite, D., and Sohngen, B. (2018). Estimation of house price differential of urban tree cover: An application of sample selection approach. Appl. Econ. 50, 2804–2811. doi: 10.1080/00036846.2017.1409419
Meunpong, P., Buathong, S., and Kaewgrajang, T. (2019). Google Street View virtual survey and in-person field surveys: An exploratory comparison of urban tree risk assessment. Arboric. J. 41, 226–236.
Miglietta, M. M., and Rotunno, R. (2016). An EF3 multivortex tornado over the ionian region: Is it time for a dedicated warning system over Italy? Bull. Am. Meteorol. Soc. 97, 337–344. doi: 10.1175/BAMS-D-14-00227.1
Moore, G. M. (2014). Wind-thrown trees: Storms or management? Arboric. Urban For. 40, 53–69. doi: 10.48044/jauf.2014.007
Mortimer, M. J., and Kane, B. (2004). Hazard tree liability in the United States: Uncertain risks for owners and professionals. Urban For. Urban Green. 2, 159–165. doi: 10.1078/1618-8667-00032
Muresan, A. N., Sebastiani, A., Gaglio, M., Fano, E. A., and Manes, F. (2022). Assessment of air pollutants removal by green infrastructure and urban and peri-urban forests management for a greening plan in the Municipality of Ferrara (Po river plain, Italy). Ecol. Indic. 135:108554. doi: 10.1016/j.ecolind.2022.108554
Nesbitt, L., Hotte, N., Barron, S., Cowan, J., and Sheppard, S. R. J. (2017). The social and economic value of cultural ecosystem services provided by urban forests in North America: A review and suggestions for future research. Urban For. Urban Green. 25, 103–111. doi: 10.1016/j.ufug.2017.05.005
Nitoslawski, S. A., Galle, N. J., van den Bosc, C. K., and Steenberg, J. W. N. (2019). Smarter ecosystems for smarter cities? A review of trends, technologies, and turning points for smart urban forestry. Sustain. Cities Soc. 51:101770. doi: 10.1016/j.scs.2019.101770
Nowak, D. J., Crane, D. E., and Stevens, J. C. (2006). Air pollution removal by urban trees and shrubs in the United States. Urban For. Urban Green. 4, 115–123. doi: 10.1016/j.ufug.2006.01.007
Oetzel, J., and Oh, C. H. (2021). A storm is brewing: Antecedents of disaster preparation in risk prone locations. Strateg. Manag. J. 42, 1545–1570. doi: 10.1002/smj.3272
Olander, L. P., Johnston, R. J., Tallis, H., Kagan, J., Maguire, L. A., Polasky, S., et al. (2018). Benefit relevant indicators: Ecosystem services measures that link ecological and social outcomes. Ecol. Indic. 85, 1262–1272. doi: 10.1016/j.ecolind.2017.12.001
Olander, L., Polasky, S., Kagan, J. S., Johnston, R. J., Wainger, L., Saah, D., et al. (2017). So you want your research to be relevant? Building the bridge between ecosystem services research and practice. Ecosyst. Serv. 26, 170–182. doi: 10.1016/j.ecoser.2017.06.003
Olander, L., Warnell, K., Warziniack, T., Ghali, Z., Miller, C., and Neelan, C. (2021). Exploring the use of ecosystem services conceptual models to account for the benefits of public lands: An example from national forest planning in the United States. Forests 12:267. doi: 10.3390/f12030267
Pace, R., De Fino, F., Rahman, M. A., Pauleit, S., Nowak, D. J., and Grote, R. (2021). A single tree model to consistently simulate cooling, shading, and pollution uptake of urban trees. Int. J. Biometeorol. 65, 277–289. doi: 10.1007/s00484-020-02030-8
Pinto, L. V., Inácio, M., Ferreira, C. S. S., Ferreira, A. D., and Pereira, P. (2022). Ecosystem services and well-being dimensions related to urban green spaces – A systematic review. Sustain. Cities Soc. 85:104072. doi: 10.1016/j.scs.2022.104072
Pipinato, A. (2018). Recent northeast italian tornado events: Lesson learned for improving structures. Nat. Hazards 2018:40. doi: 10.1007/s11069-018-3380-2
Pokorny, J. D. (1992). Urban tree risk management: A community guide to program design and implementation. St Paul, MN: USDA Forest Service Northeastern Area.
Potschin-Young, M., Haines-Young, R., Görg, C., Heink, U., Jax, K., and Schleyer, C. (2018). Understanding the role of conceptual frameworks: Reading the ecosystem service cascade. Ecosyst. Serv. 29, 428–440. doi: 10.1016/j.ecoser.2017.05.015
Púčik, T., Groenemeijer, P., Rädler, A. T., Tijssen, L., Nikulin, G., Prein, A. F., et al. (2017). Future changes in European severe convection environments in a regional climate model ensemble. J. Clim. 30, 6771–6794. doi: 10.1175/JCLI-D-16-0777.1
Rädler, A. T., Groenemeijer, P. H., Faust, E., Sausen, R., and Púčik, T. (2019). Frequency of severe thunderstorms across Europe expected to increase in the 21st century due to rising instability. npj Clim. Atmos. Sci. 2:30. doi: 10.1038/s41612-019-0083-7
Raffa, M., Reder, A., Marras, G. F., Mancini, M., Scipione, G., Santini, M., et al. (2021). VHR-REA_IT dataset: Very high resolution dynamical downscaling of ERA5 reanalysis over Italy by COSMO-CLM. Data 6:88. doi: 10.3390/data6080088
Ramyar, R., Ackerman, A., and Johnston, D. M. (2021). Adapting cities for climate change through urban green infrastructure planning. Cities 117:103316. doi: 10.1016/j.cities.2021.103316
Rappaport, E. N. (2014). Fatalities in the united states from atlantic tropical cyclones: New data and interpretation. Bull. Am. Meteorol. Soc. 95, 341–346. doi: 10.1175/BAMS-D-12-00074.1
Rauhala, J., and Schultz, D. M. (2009). Severe thunderstorm and tornado warnings in Europe. Atmos. Res. 93, 369–380. doi: 10.1016/j.atmosres.2008.09.026
Referowska-Chodak, E. (2019). Pressures and threats to nature related to human activities in European urban and suburban forests. Forests 10:765. doi: 10.3390/f10090765
Roman, L. A., Conway, T. M., Eisenman, T. S., Koeser, A. K., Ordóñez Barona, C., Locke, D. H., et al. (2021). Beyond “trees are good”: Disservices, management costs, and tradeoffs in urban forestry. Ambio 50, 615–620. doi: 10.1007/s13280-020-01396-8
Salvati, L., Sateriano, A., and Bajocco, S. (2013). To grow or to sprawl? Land cover relationships in a Mediterranean City region and implications for land use management. Cities 30, 113–121. doi: 10.1016/j.cities.2012.01.007
Sanders, J., Grabosky, J., and Cowie, P. (2013). Establishing maximum size expectations for urban trees with regard to designed space. Arboric. Urban For. 39, 68–73.
Saphores, J. D., and Li, W. (2012). Estimating the value of urban green areas: A hedonic pricing analysis of the single family housing market in Los Angeles, CA. Landsc. Urban Plan. 104, 373–387. doi: 10.1016/j.landurbplan.2011.11.012
Schmidlin, T. W. (2009). Human fatalities from wind-related tree failures in the United States, 1995-2007. Nat. Hazards 50, 13–25. doi: 10.1007/s11069-008-9314-7
Schroeder, H., Flannigan, J., and Coles, R. (2006). Resident’s attitudes toward street trees in the UK and U.S. communities. Arboric. Urban For. 32, 236–246. doi: 10.48044/jauf.2006.030
Shackleton, C. M., Ruwanza, S., Sinasson Sanni, G. K., Bennett, S., De Lacy, P., Modipa, R., et al. (2016). Unpacking pandora’s box: Understanding and categorising ecosystem disservices for environmental management and human wellbeing. Ecosystems 19, 587–600. doi: 10.1007/s10021-015-9952-z
Sinclair, W. A., and Hudler, G. W. (1988). Tree declines: Four concept causality. J. Arboricult. 14, 29–35.
Speak, A., Escobedo, F. J., Russo, A., and Zerbe, S. (2018). An ecosystem service-disservice ratio: Using composite indicators to assess the net benefits of urban trees. Ecol. Indic. 95, 544–553. doi: 10.1016/j.ecolind.2018.07.048
Speak, A., Montagnani, L., Wellstein, C., and Zerbe, S. (2020). The influence of tree traits on urban ground surface shade cooling. Landsc. Urban Plan. 197:103748. doi: 10.1016/j.landurbplan.2020.103748
Spinoni, J., Formetta, G., Mentaschi, L., Forzieri, G., and Feyen, L. (2020). Global warming and windstorm impacts in the EU. Luxembourg: Publications Office of the European Union, doi: 10.2760/039014
Starr, C. (1969). Social benefit versus technological risk. Science 165, 1232–1238. doi: 10.1126/science.165.3899.1232
Stewart, M. G., O’Callaghan, D., and Hartley, M. (2013). Review of QTRA and risk-based cost-benefit assessment of tree management. Arboric. Urban For. 39, 165–172. doi: 10.48044/jauf.2014.035
Tamura, Y., Xu, X., and Yang, Q. (2019). Characteristics of pedestrian-level mean wind speed around square buildings: Effects of height, width, size and approaching flow profile. J. Wind Eng. Ind. Aerodyn. 192, 74–87. doi: 10.1016/j.jweia.2019.06.017
Taszarek, M., Allen, J. T., and Brooks, H. E. (2021). Environments in a warming climate. Bull. Am. Meteorol. Soc. 102, 296–322. doi: 10.1126/sciadv.1700866
Taszarek, M., Allen, J. T., Groenemeijer, P., Edwards, R., Brooks, H. E., Chmielewski, V., et al. (2020). Severe convective storms across Europe and the United States. Part I: Climatology of lightning, large hail, severe wind, and tornadoes. J. Clim. 33, 10239–10261. doi: 10.1175/JCLI-D-20-0345.1
Taszarek, M., Allen, J., Púčik, T., Groenemeijer, P., Czernecki, B., Kolendowicz, L., et al. (2019). A climatology of thunderstorms across Europe from a synthesis of multiple data sources. J. Clim. 32, 1813–1837. doi: 10.1175/JCLI-D-18-0372.1
Thompson, B. K., Escobedo, F. J., Staudhammer, C. L., Matyas, C. J., and Qiu, Y. (2011). Modeling hurricane-caused urban forest debris in Houston, Texas. Landsc. Urban Plan. 101, 286–297. doi: 10.1016/j.landurbplan.2011.02.034
Tomao, A., Secondi, L., Carrus, G., Corona, P., Portoghesi, L., and Agrimi, M. (2018). Restorative urban forests: Exploring the relationships between forest stand structure, perceived restorativeness and benefits gained by visitors to coastal Pinus pinea forests. Ecol. Indic. 90, 594–605. doi: 10.1016/j.ecolind.2018.03.051
Tomao, A., Secondi, L., Corona, P., Carrus, G., and Agrimi, M. (2016). Exploring Individuals’ well-being visiting urban and peri-urban green areas: A quantile regression approach. Agric. Agric. Sci. Proc. 8, 115–122. doi: 10.1016/j.aaspro.2016.02.015
Tomao, A., Secondi, L., Corona, P., Giuliarelli, D., Quatrini, V., and Agrimi, M. (2015). Can composite indices explain multidimensionality of tree risk assessment? A case study in an historical monumental complex. Urban For. Urban Green. 14, 456–465. doi: 10.1016/j.ufug.2015.04.009
Tominaga, Y., and Shirzadi, M. (2021). Wind tunnel measurement of three-dimensional turbulent flow structures around a building group: Impact of high-rise buildings on pedestrian wind environment. Build. Environ. 206:108389. doi: 10.1016/j.buildenv.2021.108389
Trapp, R. J., and Hoogewind, K. A. (2016). The realization of extreme tornadic storm events under future anthropogenic climate change. J. Clim. 29, 5251–5265. doi: 10.1175/JCLI-D-15-0623.1
Trapp, R. J., Hoogewind, K. A., and Lasher-Trapp, S. (2019). Future changes in hail occurrence in the United States determined through convection-permitting dynamical downscaling. J. Clim. 32, 5493–5509. doi: 10.1175/JCLI-D-18-0740.1
Vajda, A., Tuomenvirta, H., Juga, I., Nurmi, P., Jokinen, P., and Rauhala, J. (2014). Severe weather affecting European transport systems: The identification, classification and frequencies of events. Nat. Hazards 72, 169–188. doi: 10.1007/s11069-013-0895-4
van Haaften, M. A., Meuwissen, M. P. M., Gardebroek, C., and Kopinga, J. (2016). Trends in financial damage related to urban tree failure in the Netherlands. Urban For. Urban Green. 15, 15–21. doi: 10.1016/j.ufug.2015.11.002
von Döhren, P., and Haase, D. (2015). Ecosystem disservices research: A review of the state of the art with a focus on cities. Ecol. Indic. 52, 490–497. doi: 10.1016/j.ecolind.2014.12.027
Wainger, L., and Mazzotta, M. (2011). Realizing the potential of ecosystem services: A framework for relating ecological changes to economic benefits. Environ. Manage. 48, 710–733. doi: 10.1007/s00267-011-9726-0
Walsh, R. A., and Ryan, L. (2017). Hospital admissions in the Hunter Region from trees and other falling objects, 2008–2012. Aust. N. Z. J. Public Health 41, 121–124. doi: 10.1111/1753-6405.12614
Way, T. L., and Balogh, Z. J. (2022). The epidemiology of injuries related to falling trees and tree branches. ANZ J. Surg. 92, 477–480. doi: 10.1111/ans.17481
Wiersma, Y. F., Davis, T. L., Eberendu, E. C., Gidge, I., Jewison, M., Martin, H. C., et al. (2012). Hurricane igor impacts at northern latitudes: Factors influencing tree fall in an urban setting. Arboric. Urban For. 38, 92–98. doi: 10.48044/jauf.2012.015
Wu, K. S., He, Y., Chen, Q., and Zheng, Y. (2020). Analysis on the damage and recovery of typhoon disaster based on UAV orthograph. Microelectron. Reliab. 107:113337. doi: 10.1016/j.microrel.2019.06.029
Xu, S., Zhu, X., Helmer, E. H., Tan, X., Tian, J., and Chen, X. (2021). The damage of urban vegetation from super typhoon is associated with landscape factors: Evidence from sentinel-2 imagery. Int. J. Appl. Earth Obs. Geoinf. 104:102536. doi: 10.1016/j.jag.2021.102536
Keywords: urban forest, disservices, severe windstorms, mitigation measures, Europe
Citation: Portoghesi L, Masini E, Tomao A and Agrimi M (2023) Could climate change and urban growth make Europeans regard urban trees as an additional source of danger? Front. For. Glob. Change 6:1155016. doi: 10.3389/ffgc.2023.1155016
Received: 31 January 2023; Accepted: 24 March 2023;
Published: 13 April 2023.
Edited by:
Mehebub Sahana, The University of Manchester, United StatesReviewed by:
Md. Omar Sarif, Lovely Professional University, IndiaKashif Imdad, Chhatrapati Shahu Ji Maharaj University, India
Copyright © 2023 Portoghesi, Masini, Tomao and Agrimi. This is an open-access article distributed under the terms of the Creative Commons Attribution License (CC BY). The use, distribution or reproduction in other forums is permitted, provided the original author(s) and the copyright owner(s) are credited and that the original publication in this journal is cited, in accordance with accepted academic practice. No use, distribution or reproduction is permitted which does not comply with these terms.
*Correspondence: Antonio Tomao, YW50b25pby50b21hb0B1bml1ZC5pdA==