- 1Institute of Lowland Forestry and Environment (ILFE), University of Novi Sad, Novi Sad, Serbia
- 2Austrian Research Centre for Forests (BFW), Vienna, Austria
- 3Slovenian Forestry Institute, Ljubljana, Slovenia
The Mura-Drava-Danube transboundary UNESCO Biosphere Reserve represents one of the best-preserved wetlands in Europe. The Reserve’s riparian forests play a significant role in ecosystem functioning and pedunculate oak (Quercus robur) is one of the keystone species of these forests. In recent years, pedunculate oak trees in the Reserve displayed symptoms of necrotic lesions on their leaves. The lesions varied in size, from small, circular to irregular reddish brown to grayish spots to larger necrotic areas that resembled leaf anthracnose and extended along the leaf nerves. In 2021, symptomatic leaves were collected in three countries of the Reserve, i.e. Austria, Slovenia, and Serbia to identify the causative agents of these diseases. Fungal cultures were obtained from symptoms and identified using morphology and multilocus phylogenetic analyses of the ITS rDNA, partial LSU rDNA, tef 1-α, BT2, CAL, ACT, and RPB2 genes. The fungi were identified as Tubakia dryina, Tubakia sp. (Tubakia dryinoides sensu lato), Didymella macrostoma, and Apiognomonia errabunda. Pathogenicity tests done by inoculating the leaves of one-year old pedunculate oak plants revealed that the isolated fungi caused symptoms as those seen in the forest. To our knowledge, this study represents the first report of D. macrostoma as the cause of pedunculate oak leaf spot disease in Serbia and worldwide. It is also the first finding of Tubakia leaf spot disease of pedunculate oak caused by T. dryina in Austria and Serbia. Moreover, Tubakia sp. was proven to be another causative agent of Tubakia leaf spot disease. Additionally, oak anthracnose caused by A. errabunda was found for the first time on pedunculate oak leaves in Austria and Slovenia. During the past decade, pedunculate oak trees have been facing increasing threats from multiple abiotic and biotic factors which has resulted in decline and absence of natural regeneration of these trees. The results of this study add to the understanding of the contributing factors to the decline of pedunculate oak in riparian forests and are important for the development of management strategies to counteract this decline.
1 Introduction
The transboundary UNESCO Mura-Drava-Danube Biosphere Reserve represents one of the best-preserved European wetlands in the border area between Austria, Slovenia, Hungary, Croatia, and Serbia. An almost 700 km long “green belt” formed by the Danube, Mura and Drava is the world’s first biosphere reserve spanning five countries, and the largest riverine protected area in Europe; hence it was named the “Amazon of Europe” (UNESCO, 2023). The Reserve consists of four wetland habitats protected by the Ramsar (2023) convention and fulfills many ecosystem services that contribute to environmental and human well-being (Oettel et al., 2022; Ramsar, 2023). It is a major carbon storage and drinking water reservoir and acts as a natural buffer by providing bank protection and climate regulation (Dybala et al., 2019; Riis et al., 2020). Moreover, its unique river landscape offers a range of recreational and nature-based tourism opportunities, and it represents a biodiversity hotspot that provides food and water resources for many rare and endangered plant, fish, insect, and animal species possibly acting as a future refugia against impacts of climate warming (Köck et al., 2022; Trišić et al., 2022; Zhang et al., 2023).
Despite their breathtaking natural features, in the past decades, floodplain ecosystems have faced numerous challenges that have resulted in their degradation (Xu et al., 2019; Tadić et al., 2022). The riverine area of the Reserve has suffered joint impacts from climate change and human intervention such as river regulation, water abstraction and dam constructions for the extension of agricultural land, protection of settlements from floods and industry needs. These impacts have resulted in changing of the hydrological regime and overall ecological conditions which have had a negative impact on the unique floodplain biotopes including the riparian forests (Skiadaresis et al., 2019; Tadić et al., 2022). Riparian forests of the Reserve are dominated by forest tree species, such as Quercus robur L., Alnus glutinosa L. (Gaertn.), Fraxinus angustifolia Vahl., Fraxinus excelsior L., Populus nigra L., Ulmus laevis Pall., and Ulmus minor Mill. Which depend on appropriate soil moisture content and water table level, as well as periodical floodings (Galić et al., 2019; Kostić et al., 2021). Therefore, the latest tree species distribution models predict a possible loss of these habitats and because of the above-mentioned perturbations, loss of biodiversity and ecosystem function (Sallmannshofer et al., 2021).
Pedunculate oak (Q.robur) is a long-lived deciduous tree species native to most of Europe and western Asia with major cultural, ecological, and economic importance (Eaton et al., 2016; Mölder et al., 2019). It is widespread in lowlands and often found close to rivers as it prefers moist soils with higher belowground water (gleysols: hydromorphic soils) and intermittent floodings (Eaton et al., 2016; Kostić et al., 2021, 2022; Kesić et al., 2023). It is a keystone species in many countries, and a major feature of internationally important habitats such as floodplain riparian forests of the Mura-Drava-Danube Biosphere Reserve (Sallmannshofer et al., 2021). Across Europe, pedunculate oak is experiencing decline of health, growth, and regeneration due to the synergistic stressors which include frequent and long-lasting droughts, lowering of the water table coupled with the absence of occasional flooding, or water table rise caused by river regulations like damming, as well as pests and diseases (Csóka et al., 2020; Stojanović et al., 2021; Kesić et al., 2023). Among the pests, the oak lace bug Corythucha arcuata (Say, 1832), spongy moth Lymantria dispar (Linnaeus, 1758), gall wasps (Cynipidae), leaf miners like Tischeria ekebladella (Bjerkander, 1795) and early spring defoliators (e.g. winter, tortrix and owlet moths, Lepidoptera: Geometridae, Tortricidae, Noctuidae) are the most notable (Csóka et al., 2020; Rađević et al., 2020; Hoch et al., 2023). Moreover, stem and root diseases caused by fungi, such as Diplodia seriata De Not., Fusarium sporotrichioides Sherb.; bacteria, such as Brenneria spp., Gibbsiella quercinecans Brady et al., 2010; Rahnella victoriana Brady et al., 2017, and pseudo fungi such as Phytophthora spp. are becoming increasingly problematic in European pedunculate oak forests (Zlatković et al., 2018; Milanović et al., 2020; Ruffner et al., 2020; Jankowiak et al., 2022).
Leaf diseases of pedunculate oak are numerous and some, for example powdery mildew can be symptom-specific (Demeter et al., 2021). Oak powdery mildew is caused by several cryptic species among which Erysiphe alphitoides (Griffon & Maubl.) U. Braun & S. Takam most commonly occurs on pedunculate oak (Bradshaw et al., 2022; Kebert et al., 2022). The disease symptoms are white powdery patches on the leaf surface with round, closed fruit bodies named chasmothecia (Demeter et al., 2021; Bradshaw et al., 2022). Other diseases, like Tubakia leaf spot and Apiognomonia anthracnose can be distinguished if fungal reproductive structures (i.e., fruiting bodies) are present on the leaf lesions (Kowalski, 2006; Boroń and Grad, 2017). Leaf spot disease caused by Tubakia dryina (Sacc.) Sutton (1973) is characterized by necrotic spots on the leaves and sometimes on the petioles. The spots can enlarge and merge to form leaf blotch, whereas petiole necrosis can cause premature defoliation (Kowalski, 2006). Tubakia species produce unique umbrella-like pycnothyrial conidiomata that consist of convex scutella made from pigmented setae-like cells fixed to the leaf surface by a central columnella. Underneath scutella mostly globose to elliptical, hyaline, subhyaline to pigmented conidia and sometimes microconidia are born on conidiogenous cells (Braun et al., 2018). Oak leaf anthracnose caused by Apiognomonia errabunda (Roberge ex Desm.) causes necrosis that develops along leaf veins with apothecia formed on the necrotic lesions (Kowalski, 2006). On the other hand, Taphrina caerulescens (Desm. & Mont.) Tul. produces small yellowish round spots on the leaves that swell upwards resembling blisters (Kowalski, 2006). However, there are leaf diseases with nonspecific symptoms such as small leaf spots of varying shape and color caused by e.g., Septoria spp., Mycosphaerella spp. or members of the Didymelaceae including Phoma-like species like Ascochyta spp., Phoma spp. and Didymella spp. (Butin, 1996; Kowalski, 2006) which challenges identification. The taxonomy of the species rich Didymellaceae has been demanding as the species identification relied on morphology and host association, but with the application of molecular phylogenetics substantial progress in species delimitation has been made (Chen et al., 2015, 2017; Hou et al., 2020a).
The synergistic effect of abiotic and biotic threats of pedunculate oak relates to “acute oak decline”, a phenomenon that has been known to occur throughout Europe, including the Mura-Drava-Danube Biosphere Reserve (Denman et al., 2014; Zlatković et al., 2018). In the Reserve, the pedunculate oak is threatened by the abiotic challenges, insect pests as well as fungal and pseudo fungal stem and root pathogens, namely D. seriata and Phytopthora spp., respectively (Zlatković et al., 2018; Milanović et al., 2020; Kostić et al., 2021). However, until 2019 nothing was known about the leaf diseases of this tree species in the Reserve. Thus, in the summer of 2019, disease symptoms such as small necrotic spots and larger necrotic areas along the leaf veins resembling leaf anthracnose were observed on the leaves of pedunculate oak in the Mura-Drava-Danube Biosphere Reserve. The presence of necrotic lesions was roughly assessed using binoculars or a branch was cut off and leaves were examined for the presence of fungal fruit bodies using hand lenses in the forest (de Groot et al., 2022). Symptoms and signs (lesions that extended along the leaf nerve and brownish cushion-like fruit bodies) resembled those of an Apiognomonia leaf anthracnose. On the other hand, certain lesions had blackish, round fungal fruit bodies resembling pycnidia/perithecia and were non-specific. Thus, these lesions were of unknown etiology and suspected to be caused by either Tubakia spp., Phoma species complex, Septoria spp., Mycosphaerella spp. and/or other leaf pathogens. Nonetheless, the true identity of the pathogens causing disease symptoms remained unknown. Therefore, in 2021 we collected leaves of pedunculate oak with symptoms such as leaf spots and anthracnose in three countries of the Reserve, i.e. Austria, Slovenia, and Serbia intending to identify the causal agents of these symptoms. We approached the diagnosis by conducting isolations from symptomatic leaves, followed by multigene phylogenetic and morphological analyses of the isolated fungi. Finally, we performed the pathogenicity tests to fulfil Koch’s postulates and determine whether the isolated fungi were the causative agents of the leaf spot and anthracnose observed in the forest.
2 Materials and methods
2.1 Sample collection and fungal isolations
In total, 33 randomly selected mature trees (average diameter at breast height 100 cm, average tree heigh 35 m) were sampled in 11 previously designed transects in Austria, Slovenia, and Serbia in the Mura-Drava-Danube Biosphere Reserve (Supplementary Table 1, three trees per transect, de Groot et al., 2022). Three leaves per tree displaying necrotic lesions were collected in paper envelopes and transferred to the laboratory for examination. Within the same day leaf spots were examined for the presence of fungal fruit bodies using Olympus SZX10 stereo microscope (Olympus Co., Tokyo, Japan). When found, fruit bodies were sectioned by hand, mounted in distilled water, and examined using Olympus BX53F light microscope with differential interference contrast (DIC) illumination equipped with Olympus SC50 digital camera and accompanying software. Leaves with symptoms were then separated into groups according to lesion dimensions, lesion position in relation to the leaf nerve, type of fungal fruit bodies found within lesions (i.e., pycnidia, pycnothyria or apothecia), color and dimensions of spores (Supplementary Table 2). Thereafter, two leaves per group per country were chosen for fungal isolation. Isolations were done immediately after leaf examinations.
To isolate fungi from the leaf spots small pieces (approximately 1 × 1mm) were cut at the margin of diseased and apparently healthy tissue of symptomatic leaves. The leaf pieces were shortly washed in tap water, then surface sterilized using 70% ethanol (1 min.), followed by 10% bleach (1 min.), washed in sterile distilled water, and blotted dry with sterile paper towels before they were put on AMEA (MEA Neogen, UK supplemented with lactic acid) to suppress bacterial growth. Fungi were also isolated from fruit bodies. Lesions were surface sterilized by spraying with 70% ethanol followed by spraying with sterile water and a fruit body was removed from the leaf using a sterile hypodermic needle and plated on AMEA. Petri dishes were kept in the dark in a cooled microbiological incubator (VWR international, Darmstadt, Germany) at 21°C for 1 week and checked daily. The mycelium was then hyphal tipped to obtain pure cultures. Isolates were separated into morphologically similar groups and depending on the number of available isolates, at least one representative isolate per country from each morphological group was chosen for further molecular phylogenetic identification and morphological characterization (Supplementary Table 2). Isolates used in the phylogenetic analyses (Supplementary Table 2) were stored in water or under mineral oil on +4°C and in 40% (v/v) glycerol on −80°C in Culture collection of the Laboratory of Forest Protection at the Slovenian Forestry Institute (ZLVG) as well as Collection of microorganisms of the Institute of Lowland Forestry and Environment (ILFE). The exception was four isolates with the morphology of Penicillium spp., Biscogniauxia sp., and Aureobasidium sp., respectively, which are well recognized as endophytes and biocontrol agents in the leaves of forest tree species (Terhonen et al., 2018). These isolates were therefore not used for further analyses.
2.2 DNA extractions, PCR, and sequencing
Since the oak leaves were collected in different countries, fungal isolations, DNA extractions, and PCR reactions of different fungal isolates were done in the country where the leaf was collected to avoid the transfer of potentially pathogenic isolates across international borders. The only exception was Phoma-like isolates (symptom group 2) which were solely obtained from leaves collected in Serbia and thus all the molecular work for these isolates was done at the Institute of Lowland Forestry and Environment (ILFE). At ILFE, to extract the DNA, fungal cultures were grown for 2 weeks on 2% MEA overlaid with sterile polypropylene discs with micro perforation (Macropack, Ledinci) to allow the fungus to reach MEA. The mycelium was then scraped from the disk surface using sterile scalpel or inoculation loop. The genomic DNA was extracted using Prepman Ultra Sample Preparation Reagent (Applied Biosystems, Foster City, USA) following manufacturer’s instructions with the following modifications: instead of vortexing, the mycelium was grinded using sterile micro pestles (Carl Roth, Germany) and spinning for two minutes was replaced with up to three ten minutes long centrifugation steps. Alternatively, at Austrian Research Center for Forests (BFW) and Slovenian Forestry Institute (SFI) the DNA was extracted using a NucleoSpin Plant II (Macherey Nagel, Düren, Germany) according to the manufacturer’s instructions, after homogenizing the fungal material with a Lysing Matrix A tube (MP Biomedicals, Solon, USA) using a Precellys Evolution device (Bertin Technologies, Montigny-le-Bretonneux, France). At ILFE, the DNA quality and concentrations were assessed using BioSpec-nano spectrophotometer (Shimadzu-Biotech, Japan), whereas at BFW and SFI the DNA was checked using biophotometer plus (Eppendorf, Germany). The isolates were initially screened and identified up to the genus level using universal barcode marker for fungi, i.e., ITS rDNA and primers ITS1F/ITS4 (White et al., 1990; Gardes and Bruns, 1993). Thereafter, the combination of other genes and primer sets used for amplification depended on the fungal genus. Thus, tef 1-α and BT2 genes were additionally amplified for Tubakia spp. isolates; LSU, RPB2 and BT2 were amplified for Didymella sp., whereas ACT and CAL genes were amplified for Apiognomonia sp. isolates (e.g., Sogonov et al., 2007; Braun et al., 2018; Boroń et al., 2019). The tef 1-α, BT2, LSU rDNA, RPB2, ACT, and CAL gene were amplified using the EF1/EF2 (O’Donnell et al., 1998), Bt-2a/Bt-2b (Glass and Donaldson, 1995), LR0R/LR6 (Vilgalys and Hester, 1990), RPB2-5F2/7CR (Liu et al., 1999; Sung et al., 2007), ACT-512-F/783-R (Carbone and Kohn, 1999; Udayanga et al., 2014), and CAL-228F/737R (Carbone and Kohn, 1999) primer sets, respectively.
PCR reactions for Phoma-like isolates (symptom group 2) were done at ILFE in a total volume of 25 μl, with the following components: 2 μl of previously diluted DNA template (20 ng/μl), 2.5 μl of 10 × Taq buffer with KCl (Thermo Scientific, Vilnius Lithuania), 3.5–5.5 μl of 25 mM MgCl2 (3.5 μl: BT2; 4.5 μl: ITS, LSU; 5.5 μl: RPB2), Thermo Scientific, Vilnius, Lithuania), 1μl of 100 mM of each dNTPs (Thermo Scientific, Vilnius, Lithuania), 0.5 μl of 100 mM of each primer (Invitrogen, Thermo Fisher Scientific, Paisley, UK), 0.3 μl of 5 U/μl Taq DNA polymerase (recombinant, Thermo Scientific, Vilnius, Lithuania) and sterile deionized filtered ultra-pure water. The PCR conditions were as described in Kovač et al., 2021 with annealing temperatures 55°C (ITS, BT2) and 60°C (LSU). The exception was the RPB2 gene which was amplified using a touchdown protocol at annealing temperatures of 65–55°C (Kovač et al., 2021). The PCR amplifications were done in MiniAmp Plus thermal cycler (Applied Biosystems, Thermo Fisher Scientific, Waltham, USA). The size of the amplified PCR products was estimated visually using O’RangeRuler 100bp DNA ladder (Thermo Scientific, Vilnius, Lithuania) after electrophoresis on 1.75% agarose gels stained with GelRed (Biotium, Hayward, USA). PCR products were cleaned using QIAquick PCR Purification Kit (Qiagen, Hilden, Germany) and sent to Macrogen Europe laboratories in the Netherlands for sequencing. While PCR reactions for Tubakia-like isolates (symptom group 1) were conducted at BFW, SFI and ILFE, PCR reactions for Apiognomonia-like isolates (symptom group 3) were done at BFW and SFI. Since chemicals and equipment differed in the three laboratories, the PCR mixtures and amplification conditions for these fungi are presented in Supplementary Table 3. The strands of all isolated fungi were sequenced in both directions with the primers as in PCR reactions.
2.3 Phylogenetic analyses
Consensus sequences were made using CLC Main Workbench 23 (Quiagen, Hilden, Germany), aligned using MAFFT v.7 online service with G-INS-1 strategy (Katoh et al., 2019), and manually improved where necessary in MEGA 11 (Tamura et al., 2021). Sequences were compared to those present in NCBI GenBank using BLASTn search, and sequences from this study were aligned with closely related reference sequences obtained from GenBank. Phylogenetic analyses including Maximum Likelihood (ML), Maximum Parsimony (MP) and Bayesian Inference (BI) were done first for the single gene sequence datasets and then also for the combined datasets (ITS-tef 1-α-BT2 for Tubakia; ITS-LSU-RPB2-BT2 for Didymella; ITS-ACT-CAL for Apiognomonia). ML analyses were done using PhyML online v.3.0 (Guindon et al., 2010) by employing an automatically selected substitution model and AKAIKE information criterion. The aligned sequences were analyzed for MP with PAUP v.40b10 and a partition homogeneity test (PHT) was performed to determine if the different gene datasets could be combined (Swafford, 2002). Posterior probability (BI) estimates were determined using Mrbayes v.3.2.7a and using the substitution model previously selected in ML analyses (Ronquist et al., 2012). MP and BI analyses were done as explained in Zlatković et al., 2016. Bootstrap analyses were conducted with 1000 bootstrap replications. Phylogenetic trees were viewed with Mega v.11 (Tamura et al., 2021) and further processed by Corel Photo Paint 2018, Microsoft Paint and Microsoft Paint3D. Nucleotide sequences from this study were deposited in GenBank, and the GenBank accession numbers of all sequences used in the analyses are provided in Supplementary Tables 4–6.
2.4 Morphological characterization
For the descriptions of colony morphology fungi were grown on 2% MEA for 2 weeks at 21°C in the dark. Colony colors were determined using the color charts of Rayner (1970). To promote fruit body production Tubakia-like isolates (symptom group 1) and Apiognomonia-like isolates (symptom group 3) were inoculated onto 2% MEA overlaid with triple autoclaved Pinus nigra J.F. Arnold needles and kept under room temperature (20 ± 2 °C) for up to two months under near UVA light (12h dark/12h light regime). Morphological characteristics of the fruit bodies and spores were checked by means of light microscopy using stereo and light microscopes described above and measurements of up to 20 conidia, microconidia and fruit bodies were made. Sections of pycnidia were made using rotary microtome cryostat MEV (SLEE medical GmbH, Mainz, Germany). Morphological characteristics of the isolated fungi were compared to those described in recent literature, e.g., Braun et al., 2018; Zhang et al., 2021; Zhu et al., 2022 (Tubakia); de Gruyter et al., 2002; Chen et al., 2015; (Didymella), Sogonov et al., 2007; Bensaci et al., 2021 (Apognomonia).
2.5 Pathogenicity test
To confirm that the isolated fungi were the cause of the disease symptoms observed in the forest, a pathogenicity test was conducted using one-year old pedunculate oak plants grown from acorns. Acorns were collected in pure pedunculate oak stand on alluvial soil (fluvisol, hydromorphic soil) in Apatin district (Serbian part of the Mura-Drava-Danube Biosphere Reserve; 45.62162°N 18.94562°E) in October 2021. To ensure that just one genotype of pedunculate oak was selected for the experiment, acorns were collected within a meter of the stem beneath the crown of a single, mature, dominant tree. The other mature dominant trees that produced acorns were spaced 23, 26, and 19 meters away in the east, south-west, and north, respectively, so their crowns did not overlap or touch the selected tree. Also, there were no acorns on the nearby codominant, smaller trees. Soil was taken from the same location, mixed with peat moss (3:1, Pešterski treset Production, Tutin), sterilized (by autoclaving at 121°C for 35 min.) and used to saw acorns in 3l pots. To remove damaged acorns before sowing, the acorns were soaked in tap water for 24 h and all floating acorns were discarded. Pots were kept in the greenhouse (20 ± 2°C day temperature, 17 ± 2°C night temperature, 70 ± 3% relative humidity, photoperiod of 16 h light/day) and watered as needed until the pathogenicity test was performed in April 2022.
One representative isolate of each of the previously identified T. dryina, Tubakia sp. (Tubakia dryinoides C. Nakash. sensu lato), Didymella macrostoma (Mont.) Qian Chen & L. Cai and A. errabunda was used to complete Koch’s postulates and confirm pathogenicity of these fungi on pedunculate oak leaves (Supplementary Table 2). This is because, aside from isolates of A. errabunda, the isolates of T. dryina, Tubakia sp. and D. macrostoma represented single haplotypes. For inoculation isolates were grown for 2 weeks at 21°C on MEA in a microbiological incubator (INCU-Line, VWR International, USA) in the dark. Leaves were first sterilized using 70% ethanol and then ruptured between major veins using a sterile needle and mycelial discs of 6 mm diameter, taken from the edges of fungal cultures were placed on the upper leaf surface, with the mycelium faced downwards. Mycelial plugs were used for inoculation instead of spore suspension since it was a more convenient method to test pathogenicity of fungi from three different genera with varying sporulation ease and times (Bhunjun et al., 2021). A total of ten plants per isolate was used for inoculations and on each plant two fully expanded leaves were inoculated giving a total of 20 leaves per isolate. Twenty leaves on ten plants served as mock-inoculated controls and these plants were inoculated using sterile MEA plugs. For the next 48h, to ensure sufficient humidity, plants were covered with transparent polyethylene bags that had tiny needle-punched ventilation holes. The plants were grown in the greenhouse with temperature ranging from 19 ± 2 °C (night) to 21 ± 2 °C (day), air humidity 80 ± 2%, and photoperiod of 16 h light/day. The plants were arranged in a completely randomized design and watered twice a week to field capacity. Leaves were monitored for disease symptoms every day until lesions emerged, then once a week. The experiment lasted for 4 weeks as by that time all the inoculated fungi developed lesions and produced fruit bodies on the leaves.
3 Results
3.1 Disease symptoms and isolations
Based on disease symptoms and signs, collected leaves were separated into three groups. The first group represented leaves with leaf spots ranging from 1 to 2.5 cm in diameter. Lesions were purplish brown, reddish brown to brown with indefinite margin or darker border. The older lesions were often with cracks, and superficial fungal fruit bodies resembling pycnothyria with scutella of radiating, pigmented cells and ellipsoid, hyaline to brown Tubakia-like spores (Figures 1, 2; Braun et al., 2018). Based on the presence of pycnothyria on necrotic areas, leaf lesions of this group were classified as Tubakia-like leaf spots. This type of lesions was found on leaves from each sampled country. Apart from three isolates of Biscogniauxia sp., Aureobasidium sp. and Penicillium sp., twelve isolates resembling Tubakia-like morphology (cultures initially white, fluffy and with rosette-like appearance turning dark with age) were obtained from these symptoms (eight from Austria, one from Slovenia, and two from Serbia). The Tubakia-like isolates were further separated into two subgroups with cultures that turned gray with age belonging to the first subgroup (seven from Austria and one from Serbia) and isolates turning blackish with age as the second subgroup (one from Austria, one from Slovenia, one from Serbia). Six representative isolates (three isolates from each subgroup with representatives from each country) were chosen for further molecular phylogenetic identification and morphological characterization (Supplementary Table 2).
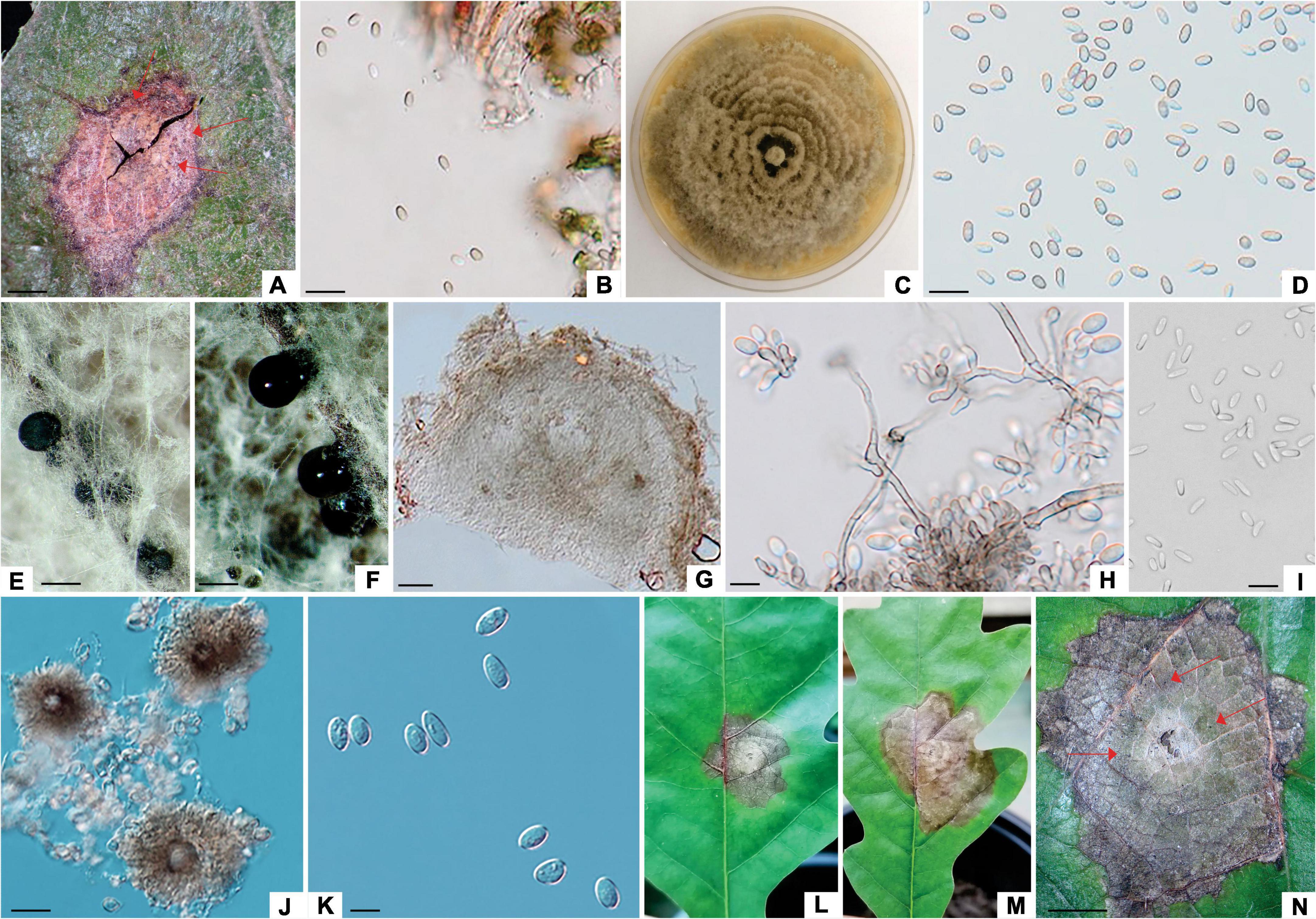
Figure 1. Tubakia dryina (ILFE 6). (A): Leaf lesion with conidiomata (pycnothyria, arrows) on leaves of Q. robur in Serbian part of the Mura-Drava-Danube Biosphere Reserve. (B, D): Conidia from pycnothyria on leaf lesions in the field. (C): Colony morphology of ILFE 6 on MEA after 2 weeks at 21°C in the dark. (E): Pycnidia formed on needles. (F): Sporodochial conidiomata formed on needles with blackish conidial masses. (G): Cross section of pycnidium formed on pine needles. (H): Conidiogenous cells giving rise to conidia. (I): Microconidia. (J): Pycnothyria with scutella. (K): Conidia. (L–N): Lesions formed during pathogenicity test with superficial pycnothyria (arrows). Scale bars: (A), (N) = 1 mm; (B), (D) = 30 μm; (E,F) = 0.5 mm; (G–I), (K) = 10μm; (J) = 20μm.
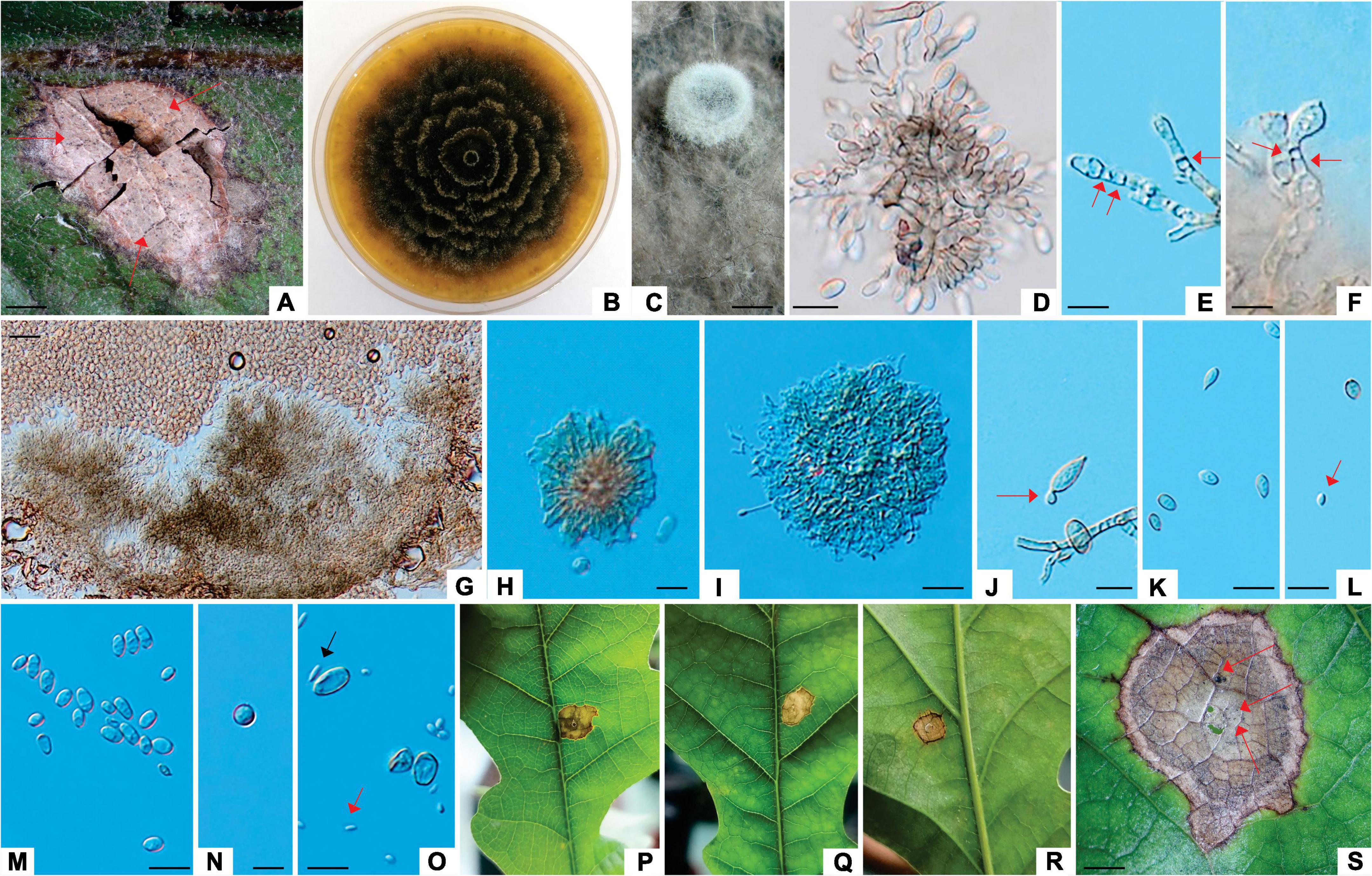
Figure 2. Tubakia dryinoides (ZLVG 972). (A): Leaf lesion with conidiomata (pycnothyria, arrows) on leaves of Q. robur in Slovenian part of the Mura-Drava-Danube Biosphere Reserve. (B): Colony morphology of ZLVG 972 on MEA after 2 weeks at 21°C in the dark. (C): A pycnidium formed on needles. (D–F): Conidiogenous cells giving rise to conidia (arrows indicate annelations). (G): Cross section of pycnidium formed on needles. (H,I): Underdeveloped pycnothyrium. (J): Microcycle conidiation (arrow). (K,M,N): Conidia. (L,O): Conidia and microconidia (arrows). (P–S): Lesions formed on inoculated leaves (pycnidia are denoted by arrows). Scale bar: (A,S) = 1 mm; (C) = 0.5 mm; (G) = 50 μm; (E,F,H,J,O) = 10 μm; (K, M,D) = 20μm.
The lesions of the second group were reddish brown to grayish brown, ranging from 0.2 to 0.7 cm in diameter containing pycnidial fruit bodies with conidia like those of the Phoma-like species (Figure 3), thus lesions of this group were classified as Phoma-like lesions. This type of lesions was present only on leaves collected in Serbia. One isolate with Penicillium-like and two isolates with Phoma-like morphology were obtained from these lesions, and Phoma-like isolates were used in subsequent phylogenetic and morphological analyses (Supplementary Table 2).
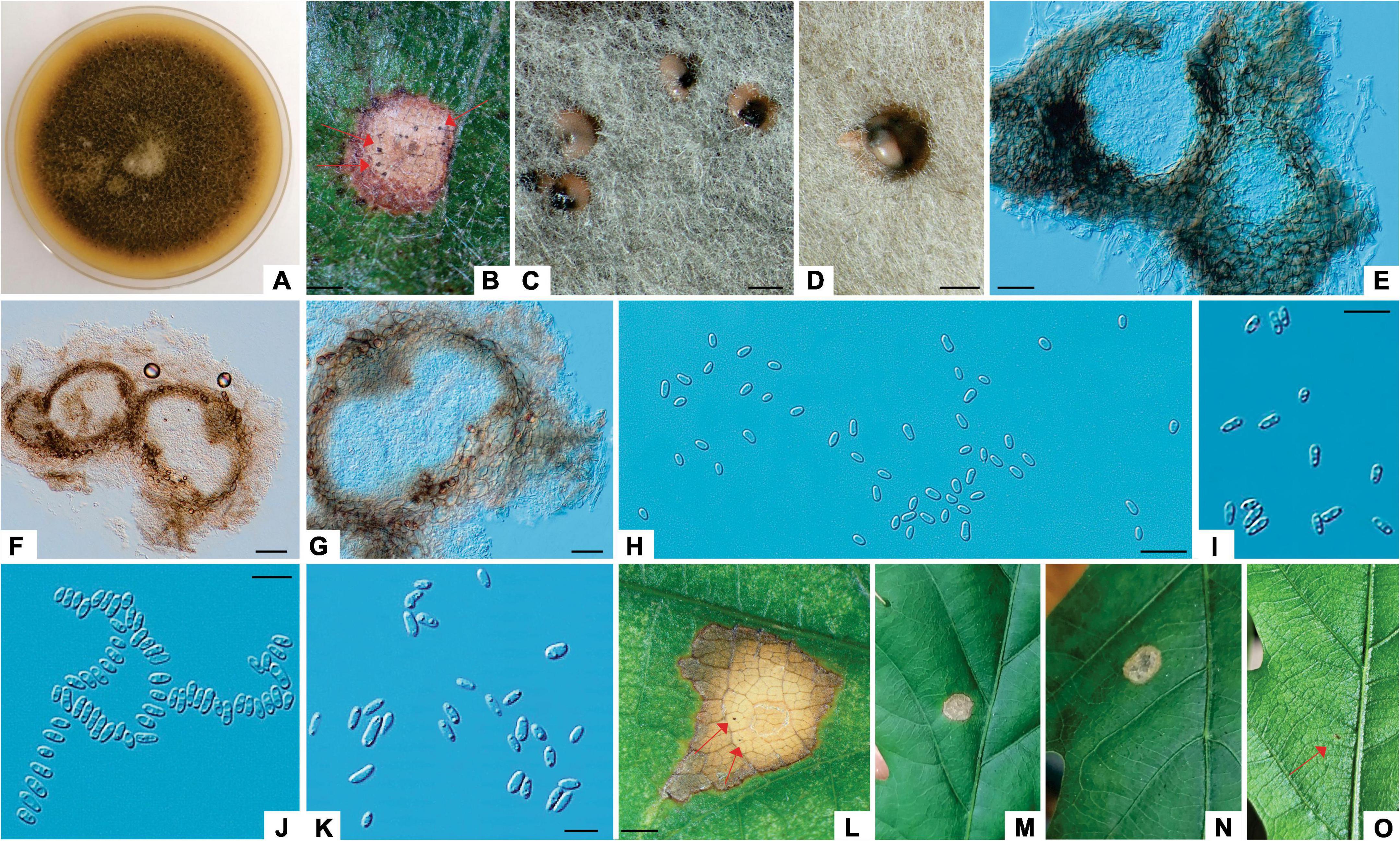
Figure 3. Didymella macrostoma (ILFE 8). (A): Colony morphology of ILFE 8 on MEA after 2 weeks of growth at 21°C in the dark. (B): Leaf lesion with conidiomata (pycnidia, arrows) on leaves of Q. robur in the Serbian part of the Mura-Drava-Danube Biosphere Reserve. (C,D): Pycnidia formed on MEA extruding spores in creamy colored spore masses. (E,G). Cross section of pycnidia. (H–K). Spores. (L–N). Lesions formed on inoculated leaves (pycnidia are denoted by arrows). (O): Control plant without disease symptoms showing only a small discoloration as a result of wounding (arrow). Scale bar: (B–D,L) = 1 mm; (F) = 50 μm; (E) = 25 μm; (H–J) = 20 μm; (K) = 10 μm.
The lesions of the third group were reddish brown to grayish extending along leaf veins, measuring 1.5–3 cm in diameter. These lesions contained brownish, cushion-shaped acervuli with conidia like those of the Apiognomonia species and they were characterized as Apiognomonia-like lesions. Four Apiognomonia-like isolates were isolated from these lesions (two from Austria and two from Slovenia) and these were further used in the analyses (Supplementary Table 2).
3.2 Molecular phylogenetic identification
A combined dataset (ITS-tef 1-α-BT2) of Tubakia species contained 42 sequences including two outgroup sequences of Paratubakia subglobosa (T. Yokoyama & Tubaki) U. Braun & C. Nakash. (CBS 193.71, CBS 124733). The matrix consisted of 1736 characters (ITS: 609, tef 1-α: 603, BT2: 524) of which 451 were parsimony-informative. The TN93 substitution model was automatically selected in the ML analyses and used in the BI analysis. There were 16 most parsimonious trees with TL = 726, CI = 0.78, RI = 0.94. The PHT value indicated that the three datasets could be combined (PHT = 0.01). The topologies of MP, BI and ML trees were substantially congruent, and the best ML tree is presented (Figure 4).
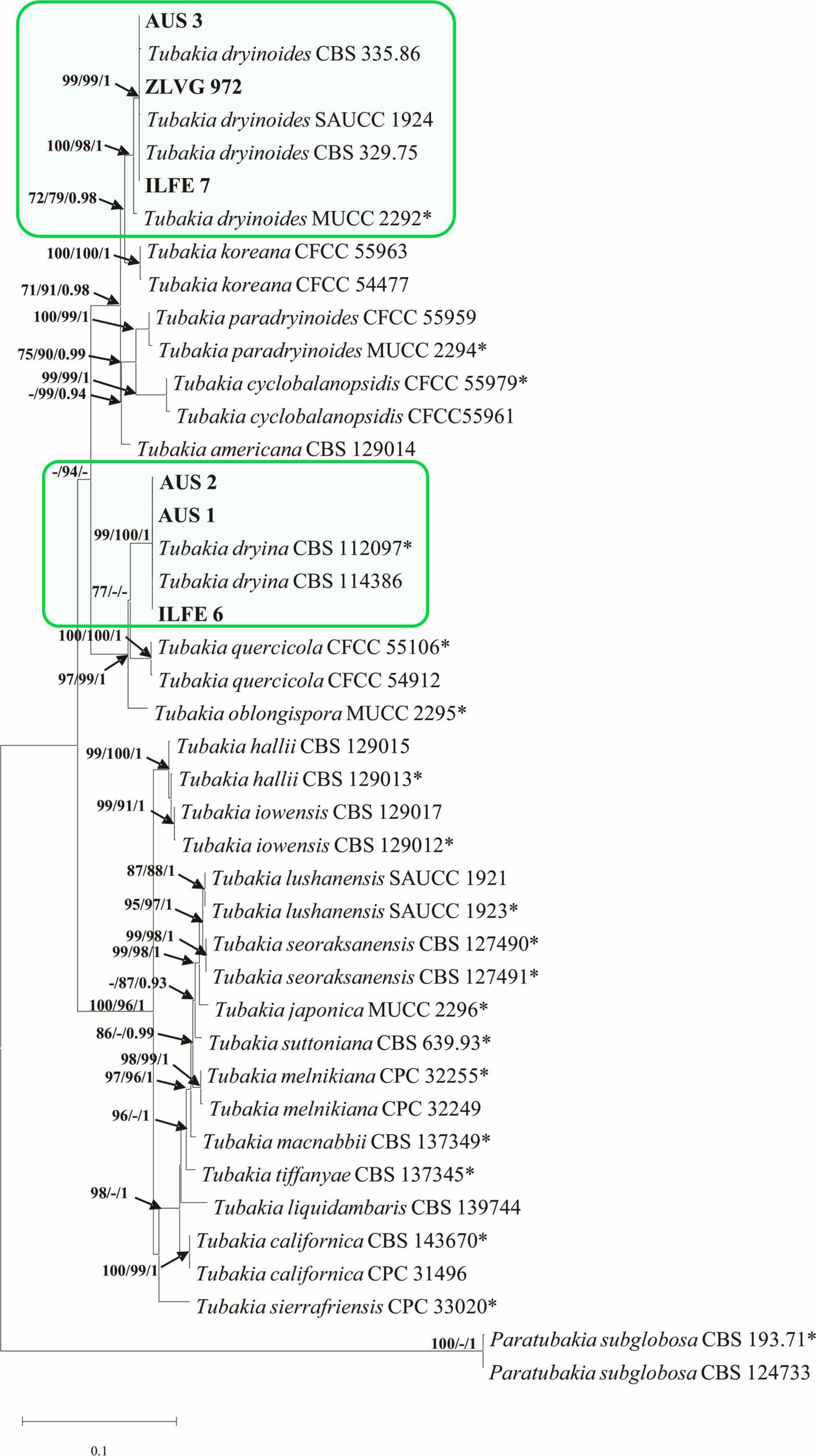
Figure 4. Phylogram of Tubakia species based on combined dataset of ITS, tef 1-α and BT2. Maximum likelihood (ML), maximum parsimony (MP) bootstrap support values (ML, MP > 70%), and Bayesian Posterior Probabilities (BPP ≥ 0.9) are shown at the tree nodes. The tree is rooted with Paratubakia subglobosa (CBS 193.71, CBS 124733). Ex-type strains are marked with an asterix, whereas strains from this study are indicated in bold type. The scale bar represents the number of changes per site. Species identified in this study are indicated with color blocks.
The multigene phylogenetic tree grouped isolates from the first subgroup of Tubakia-like leaf spots (symptom group 1, subgroup 1) in a highly supported single clade with T. dryina (99/100/1, ML, MP, BI bootstrap/posterior probability support) (Figure 4). In individual gene trees, these isolates also resided within T. dryina (Supplementary Figure 1).
The multigene phylogenetic analyses grouped isolates from the second subgroup of Tubakia-like leaf spots in a highly supported clade with the ex-type strain of T. dryinoides (T. dryinoides sensu stricto) and strains of T. dryinoides sensu lato (100/98/1, ML, MP, BI bootstrap/posterior probability support, Figure 4). Within this clade, our isolates clustered with isolates of T. dryinoides sensu lato (s.l.) in a highly supported sub clade (99/99/1 bootstrap/posterior probability support, Figure 4). In the ITS phylogeny isolates from this study clustered with T. dryinoides s.l., T. dryinoides sensu stricto (s.s.), Tubakia koreana H.Y.Yun and Tubakia paradryinoides C. Nakash. In the tef 1-α and BT2 phylogenies these isolates resided in a clade with T. dryinoides s.s. and T. dryinoides s.l. and within this clade in a subclade with strains of T. dryinoides s.l. (Supplementary Figure 1). Shared polymorphisms between Tubakia sp. isolates from this study, T. dryinoides s.s and T. dryinoides s.l. are shown in Supplementary Table 7. Molecular phylogenetic analyses identified Tubakia-like isolates as T. dryina and Tubakia sp. (T. dryinoides s.l.) (Figure 4).
A combined dataset (ITS-LSU-RPB2-BT2) of Didymella species contained 27 sequences including an outgroup sequence of Epicoccum nigrum Link (CBS 173.73). The matrix consisted of 2386 characters (ITS: 493; LSU: 964; RPB2: 596; BT2: 333) of which 295 were parsimony informative. The TN93+G+I substitution model was automatically selected in the ML analyses and used in the BI analysis. There were two most parsimonious trees with TL = 730, CI = 0.56, RI = 0.79. The PHT value was low but still acceptable and indicated that the datasets could be combined (PHT = 0.01). The topology of MP and BI trees was like that of the ML tree, and the best ML tree is presented (Figure 5).
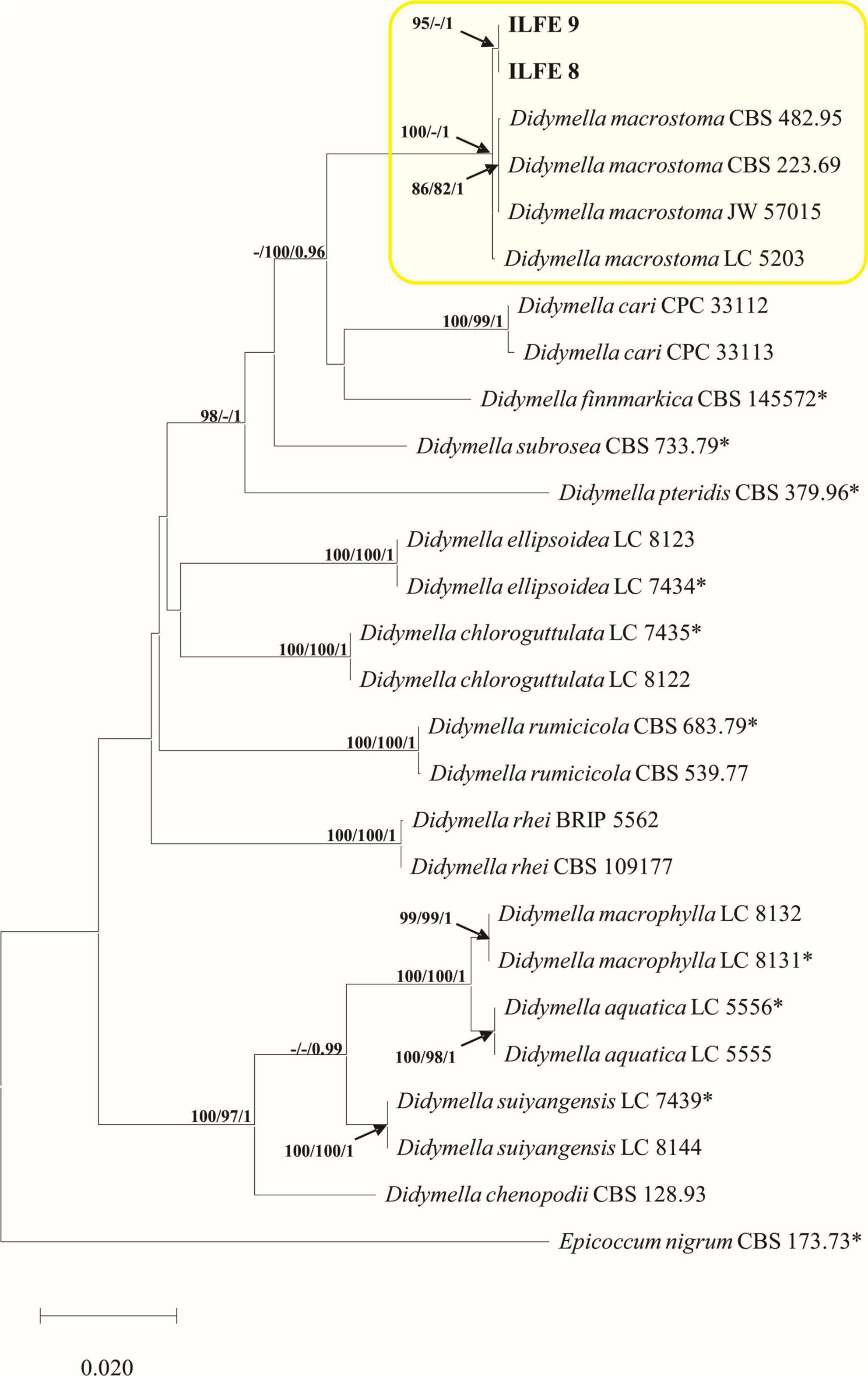
Figure 5. Phylogram of Didymellaceae based on combined ITS, BT2, RPB2 and LSU dataset. Maximum likelihood (ML), maximum parsimony (MP) bootstrap support values (ML, MP > 70%), and Bayesian Posterior Probabilities (BPP ≥ 0.9) are shown at the tree nodes. The tree is rooted with Epicoccum nigrum (CBS 173.73). Ex-type strains are marked with an asterix, whereas strains from this study are indicated in bold type. The scale bar represents the number of changes per site. Species identified in this study are indicated with color blocks.
The multigene phylogenetic analyses grouped isolates from the second group of Phoma-like leaf spots in a clade strongly supported in ML and BI analyses with D. macrostoma (100/1, ML, BI bootstrap/posterior probability support) (Figure 5). In the single gene analyses of RPB2 and BT2 isolates from the Phoma-like leaf spots grouped with isolates of D. macrostoma. In the ITS analyses isolates from this study are grouped with D. macrostoma, Didymella subrosea L.W. Hou, L. Cai & Crous, Didymella finnmarkica Crous & Rämä and Didymella pteridis L.W. Hou, Crous & L. Cai, whereas in the LSU analyses isolates from this study grouped with D. macrostoma, D. subrosea, Didymella aquatica Q. Chen, Crous & L. Cai, Didymella rumicicola (Boerema & Loerakker) Qian Chen & L. Cai, and D. finnmarkica (Supplementary Figure 2). Fixed polymorphisms unique for isolates of D. macrostoma from this study are shown in Supplementary Table 8. Molecular phylogenetic analyses identified Phoma-like isolates as D. macrostoma (Figure 5).
A combined dataset (ITS-ACT-CAL) of Apiognomonia species (third group of symptoms), had 14 taxa including an outgroup sequence of Plagiostoma aesculi (Fuckel) Sogonov (AR 3640). The matrix contained 1223 characters (ITS: 525, ACT: 260, CAL: 438) of which 64 were parsimony informative. The model GTR+I was selected in the ML analyses and used in the following BI analyses. The MP analyses gave 14 most parsimonious trees with TL = 72, CI = 1, RI = 1 and the PHT test showed that the three datasets could be combined (PHT = 1). The topologies of MP, BI and ML trees were concordant, and the best ML tree is presented (Figure 6).
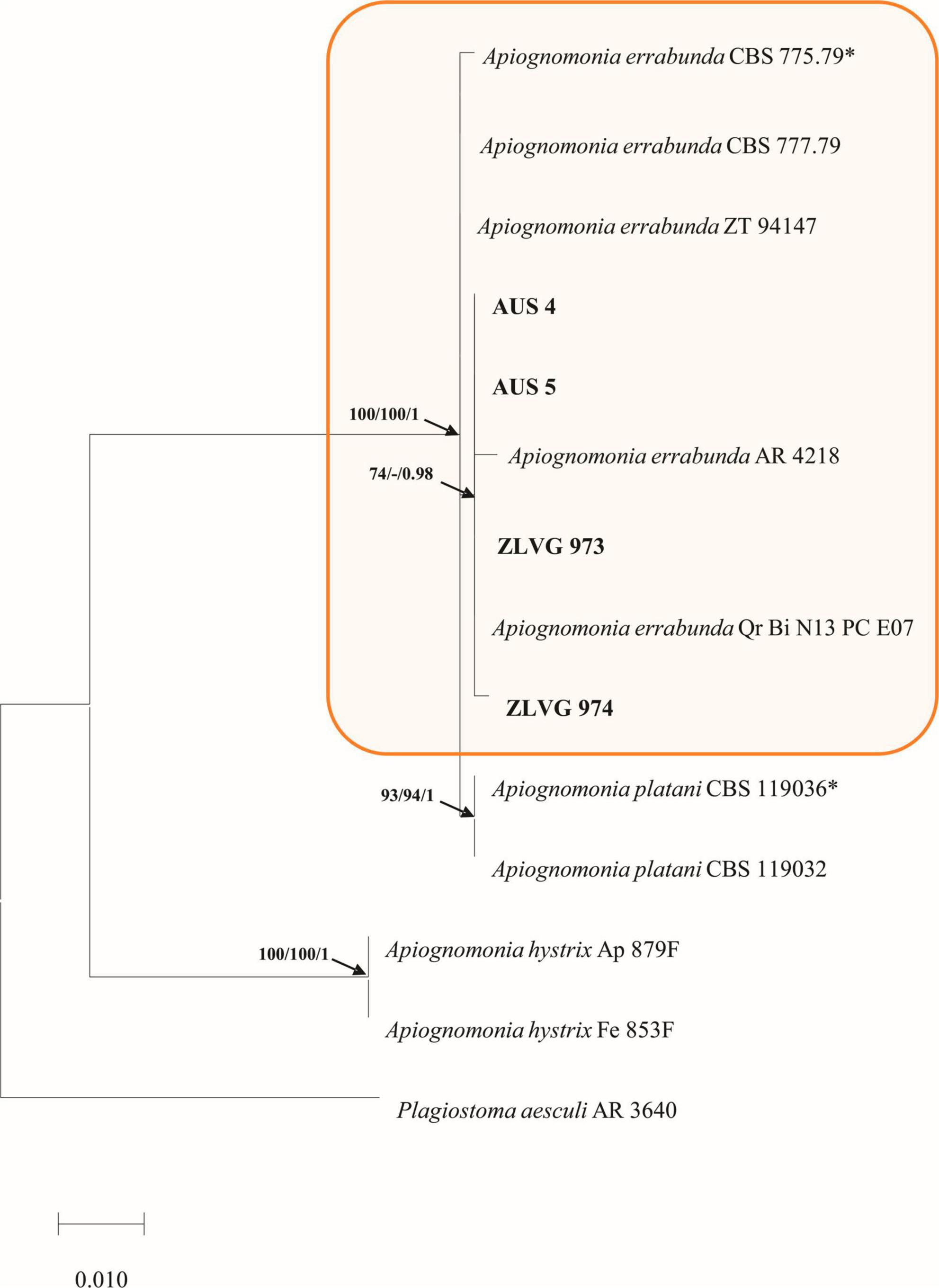
Figure 6. Phylogram of Apiognomoniaceae based on concatenated ITS, CAL and ACT sequence alignment. The maximum likelihood (ML) and maximum parsimony (MP) bootstrap support values (> 70%) as well as Bayesian Posterior Probabilities (BPP ≥ 0.9) are shown at the tree nodes. The tree is rooted to Plagiostoma aesculi AR 3640. Strains from the current study are in bold, whereas ex-type strains are marked with an asterix. The scale bar represents the number of changes per site. Species identified in this study are indicated with color blocks.
In the multigene phylogenetic analyses, isolates from this study grouped with isolates of A. errabunda forming a fully supported clade (100/100/1, ML, MP, BI bootstrap/posterior probability support) (Figure 6). In the single gene phylogenies isolates from this study grouped with A. errabunda in the ACT and CAL analyses, whereas in the ITS analyses they clustered with A. errabunda and Apiognomonia platani (Lév.) L. Lombard (Supplementary Figure 3). Molecular phylogenetic analyses identified Apiognomonia-like isolates as A. errabunda (Figure 6).
3.3 Morphological characterization
Based on phylogenetic analyses and morphology, this study identified three known species, i.e. T. dryina (symptom group 1, subgroup 1), D. macrostoma (symptom group 2) and A. errabunda (symptom group 3), while Tubakia sp. (T. dryinoides s.l., symptom group 1, subgroup 2) represented an undescribed cryptic species that is closely related to T. dryinoides. This species has been tentatively maintained in T. dryinoides by Braun et al., 2018. who preferred not to describe it as a new species due to the insufficient sampling. In this study, we also prefer not to name it for the same reason as Braun et al., 2018. However, to facilitate future studies, we have provided and illustrated its unique morphological characteristics. Moreover, since there are not many recent collections of T. dryina, D. macrotoma and A. errabunda from Europe, this section also contains morphological characteristics of these fungi, especially those that differ from what has been described in other studies.
3.3.1 Taxonomy
Tubakia dryina (Sacc.) Sutton (1973), Trans. Brit. Mycol. Soc. 60 (1): 165 (1973), Figures 1, 4, Supplementary Figure 1 and Supplementary Tables 1–4.
Colonies of T. dryina (symptom group 1, subgroup 1) initially white, fluffy and with a rosette-like appearance turning grayish with age, reverse grayish. Conidiomata (pycnothyria) on leaves black, scutellate, up to 200 μm diameter; conidiomata on previously autoclaved pine needles black, pycnidial, up to 500 μm diameter, often sporodochial extruding whitish or blackish conidial masses. Conidiophores reduced to hyaline to pale brown conidiogenous cells that proliferate percurrently to form periclinal thickenings or rarely annelations. Conidia 1-celled, broadly ellipsoidal, ellipsoidal or obovoid, pyriform with round or rarely truncate base, rarely globose, initially hyaline, turning pale brown with age, with rough outer wall, rarely smooth, often thick-walled, 9.82–15.20 μm × 5.26–7.93 μm (av. 11.32 × 6.25 μm, length/width ratio: 1.8, conidia in pycnothyria on leaves, n = 20); 12.76–15.66 μm × 8.01–8.46 μm (av. 13.9 × 8.46 μm, length/width ratio: 1.6, conidia in pycnidia on autoclaved pine needles, n = 20). Microconidia in pycnothyria on leaves 1-celled, fusiform, hyaline, smooth, 6.05–8.13 μm × 1.32–2.21 μm (av. 7.1 × 1.7 μm, length/width ratio: 0.6, n = 20).
Isolates examined: Serbia, Mura-Drava-Danube Biosphere Reserve, on necrotic lesion of a leaf of Q. robur, June 2021, M. Zlatković/M. Sallmannshofer (living isolate ILFE 6).
Notes: The morphology of an isolate of T. dryina from this study was in accordance with that described by Braun et al., 2018, with an exception that conidia were mostly with rough outer wall, and conidiogenous cells were hyaline, but also pale brown similarly to what was observed by Lee et al., 2018. Moreover, in this study, the fungus formed pycnidia and sporodochia instead of scutelloid pycnothyria on autoclaved pine needles. Similarly, in the work of Holdenrieder and Kowalski, 1989 pycnidia instead of pycnothyria had formed on previously autoclaved pedunculate oak twigs. Thus, it appears that pycnothyria may serve as a useful diagnostic character for T. dryina only when the fungus is found in nature on a necrotic lesion of a living plant that serves as its host.
Tubakia sp. (Tubakia dryinoides Nakash (2018). sensu lato), Fungal Systematics and Evolution 1: 80 (2018), Figures 2, 4, Supplementary Figure 1 and Supplementary Tables 1–7.
Colonies of Tubakia sp. (symptom group 1, subgroup 2) initially white and with rosette-like appearance, becoming blackish with time, reverse blackish. Conidiomata (pycnothyria) on leaves black, scutelloid, up to 200 μm diameter; conidiomata on previously autoclaved pine needles pycnothyrial or pycnidial covered with whitish or grayish hairs, up to 700 μm diameter. Conidiophores reduced to hyaline to pale brown conidiogenous cells, forming indistinct periclinal thickenings or annelations. Rarely, conidia produced by macrocycle conidiation. Conidia 1-celled, broadly ellipsoidal, ellipsoidal or obovoid, sometimes spindle shaped with one round and one tapered end, initially hyaline, turning pale brown with age, with rough outer wall, rarely smooth, sometimes thick-walled, 8–13.10 μm × 5.56–7.93 μm (av. 9.22 × 6.62 μm, length/width ratio: 1.4, conidia in pycnothyria on leaves, n = 20); 7.16–12.39 μm × 5.63–7.71 μm (av. 9.39 × 6.52 μm, length/width ratio: 1.4, conidia in pycnidia on autoclaved needles, n = 20); 8.10–13 μm × 5.6–8 μm (av. 9.10 × 6, length/width ratio: 1.5, conidia in pycnothyria on autoclaved needles, n = 20). Microconidia in pycnidia on autoclaved needles, 1-celled, hyaline, smooth, fusiform, 6–7.9 μm × 1.46–2 μm (av. 7 × 1.8 μm, n = 10) or pyriform to ellipsoid, 2.5–3.3 × 1.7–2.3 (av. 2.9 × 2.6 μm, n = 20).
Isolates examined: Serbia, Mura-Drava-Danube Biosphere Reserve, necrotic lesion on the leaf of Q. robur, June 2021, M. Zlatković/M. Sallmannshofer (living isolate ILFE 7); Slovenia, necrotic lesion on the leaf of Q. robur, June 2021, N. Ogris/B. Piškur (living isolate ZLVG 972).
Notes: Braun et al., 2018 noticed that the ex-type strain of T. dryinoides MUCC 2292, isolated from Quercus phillyreoides A. Grey in Japan, differs from the European collections of T. dryinoides. However, the authors decided to maintain European isolates in T. dryinoides due to the lack of morphological and ecological data and the insufficient number of isolates. Phylogenetic analyses from this study showed that isolates from this study AUS3, ZLVG 972 and ILFE 7, other European isolates of T. dryinoides (T. dryinoides s.l.) CBS 329.75 and CBS 335.86 and an isolate SAUCC 1924 from China represent cryptic species closely related to T. dryinoides. Nonetheless, since there are only two living isolates of Tubakia sp. available from this study, we prefer not to name the species. Future studies should morphologically and phylogenetically analyze all available living isolates of this cryptic taxon.
Isolates of Tubakia sp. from this study were morphologically similar to the type strain of T. dryinoides described by Braun et al., 2018 (T. dryinoides s.s.), but conidia of Tubakia sp. from this study were hyaline and dark like those of T. dryina and apart from a study of Zhu et al., 2022 differed from Asian collections of T. dryinoides. Moreover, our isolates were characterized by microcycle conidiation and conidiogenous cells were often pale brown, similar to isolates of T. dryina from this work. Furthermore, T. dryinoides has been reported to produce pycnothyrhia and sporodochial conidiomata (Braun et al., 2018; Zhang et al., 2021; Zhu et al., 2022), but Tubakia sp. from this study also produced pycnidia covered with whitish or grayish hairs.
Didymella macrostoma (Mont.) Chen and Cai (2015), Stud. Mycol. 82: 177 (2015). Figures 3, 5, Supplementary Figure 2 and Supplementary Tables 1, 2, 5, 8.
Colonies of D. macrostoma (symptom group 2) initially white, becoming dark reddish gray with age, reverse dark gray. Conidiomata pycnidial, up to 180 μm diameter, ostiolate, single or often aggregated. Conidia aseptate, variable in shape, ellipsoidal to oblong or allantoid, eguttulate or with one or two polar guttules, hyaline, smooth walled, extruding from pycnidia in buff colored spore masses, 3–9.6 μm × 2.5–4.3 μm (av. 6.6 × 3.4 μm, length/width ratio: 1.9, conidia in pycnidia on leaves, n = 20), 3.13–10.50 μm × 2.68–4.54 μm (av. 7.05 × 3.41 μm, length/width ratio: 2.1, conidia in pycnidia on MEA, n = 20).
Isolates examined: Serbia, Mura-Drava-Danube Biosphere Reserve, necrotic lesion on the leaf of Q. robur, June 2021, M. Zlatković/M. Sallmannshofer (living isolates ILFE 8, ILFE 9).
Notes: The D. macrostoma isolates obtained in this study exhibited similar morphological features as those reported by de Gruyter et al., 2002 and Jayasiri et al., 2017, with the difference that conidia were aseptate and pycnidia were frequently aggregated. Moreover, conidia were often eguttulate and some pycnidia contained only this type of conidia. Thus, it is important to look at multiple pycnidia because guttulate conidia may not always be the best diagnostic trait. Moreover, it appears that D. macrostoma is uncommon in Europe, or at the very least, the current sampling is poor. This is likely the cause of the name’s lack of stabilization through epitypification, which is crucial for the taxonomic and phylogenetic analysis of the taxon.
Apiognomonia errabunda (Roberge (1918) ex Desm.) Höhn., Ann. Mycol. 16 (1–2): 51 (1918). Figures 6, 7, Supplementary Figure 3 and Supplementary Tables 2, 3, 6.
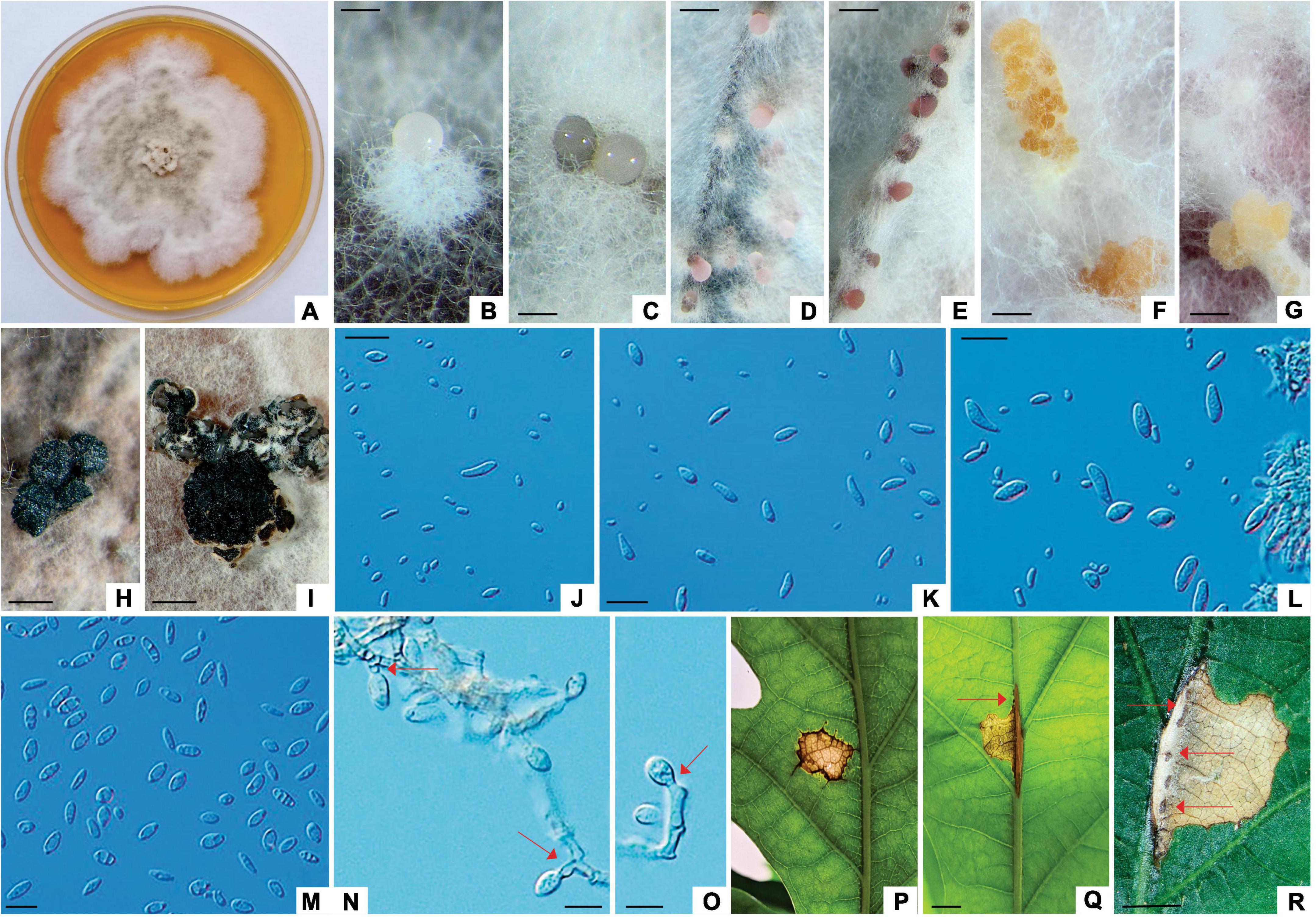
Figure 7. Apiognomonia errabunda (ZLVG 973). (A): Colony morphology of ZLVG 973 on MEA after 2 weeks at 21°C in the dark. (B–D): Pycnidial conidiomata formed on pine needles extruding spores in creamy white, greyish, pink or purpule spore masses. (F,G): Amber colored sporodochia formed on MEA with pine needles. (H,I): Pycnidium formed on MEA. (J,K,M): Conidia. (L); Conidia and conidiogenous cells. (N,O): Conidia formed on conidiogenous cells (annelations and periclinal thickenings are denoted by arrows). (P–R): Lesions formed on inoculated leaves (cushion-shaped brown acervulae are denoted by arrows). Scale bar: (B,C) = 200 μm; (D–F,H,I) = 0.5 mm; (G) = 1 mm, (J–O) = 20 μm; (Q,R) = 2mm.
Colonies of A. errabunda (symptom group 3) white becoming whitish gray at the center with age, with dense aerial hyphae, reverse grayish. Conidiomata on leaves brownish, cushion-like, mostly ellipsoid acervuli, up to 0.8 mm in length. Conidiomata on MEA with pine needles pycnidial, multilocular, aggregated in the black stroma, mostly ellipsoidal, up to 1mm in length, extruding spores in whitish or grayish spore masses or amber colored, sporodochial, gregarious, up to 2 mm in length. Conidiomata on autoclaved pine needles black with white to gray hairs, extruding spores in white, buff, pink or purple spore masses, up to 0.8 mm in diameter. Conidiophores hyaline, septate. Conidiogenous cells proliferating percurrently to form annelations or periclinal thickenings. Conidia variable in shape, broadly fusiform, oval to obovoid, ellipsoidal, occasionally obpyriform or oblong, usually tapered at one end, often slightly curved, with rough outer wall, often with 1–5 guttules, aseptate, older conidia prior to germination rarely 1-septate, 7.29–14.10 μm (av. 10.58 × 4.8 μm, length/width ratio: 2.2, n = 20). Microconidia oval, oblong to cylindrical, sometimes obpyriform, hyaline, occasionally with 1–2 guttules, in pycnidia on autoclaved pine needles and sporodochia on MEA with pine needles 3.85–7.08 μm (av. 5.39 × 2.74 μm, length/width ratio: 1.97, n = 20).
Isolates examined: Slovenia, Mura-Drava-Danube Biosphere Reserve, necrotic lesion on the leaf of Q. robur, June 2021, N. Ogris/B. Piškur (living isolates ZLVG 973, ZLVG 974).
Notes: The morphology of A. errabunda isolates from this study was similar to that reported by Sogonov et al., 2007 and Li et al., 2020. However, pycnidia from this study were also amber colored, sporodochial and conidia were variable in shape, with rough outer wall, sometimes 1-septate.
3.4 Pathogenicity test
One week after inoculation, small lesions started to appear on plants inoculated with each fungal isolate used in the pathogenicity test and 4 weeks later fungal fruit bodieswere found on leaves of the inoculated plants. Small reddish-brown lesions were observed on each leaf inoculated with T. dryina and on leaves inoculated with T. dryinoides. The lesions gradually enlarged in size; 4 weeks after inoculation the lesions were irregular in shape and all inoculated leaves were with pycnothyrial conidiomata containing Tubakia-like spores. The lesions of plants inoculated with T. dryina resembled leaf blight, covered large portions of the leaf and measured 1.5–2 × 1.7–2.2 cm. Lesions caused by Tubakia sp. were with a distinctive margin and measured 0.5–0.7 × 0.6–0.9 cm. Pycnothyria formed on ten leaves inoculated with T. dryinoides. Small grayish lesions were observed on plants inoculated with D. macrostoma. These remained grayish and small and measured 0.4–0.6 × 0.3–0.5 cm. Pycnidial conidiomata with Phoma-like spores formed on one leaf. Reddish-brown irregular lesions that measured 1.5–2 × 1.4–2.6 cm were present on the leaves of plants infected with A. errabunda. Brownish apothecia with Apiognomonia-like spores formed along and across leaf veins of two leaves.
On the control plants that were mock-inoculated no disease symptoms were observed. Inoculated leaves with necrotic lesions were collected and the fungi were re-isolated as described previously. Re-isolations resulted in cultures with morphology and ITS sequences of fungi used for inoculations, thus fulfilling Koch’s postulates.
4 Discussion
The current study represents the first attempt to identify the causative agents of the leaf spot and anthracnose diseases of pedunculate oak in the Mura-Drava-Danube Biosphere Reserve. Using the molecular phylogeny of multiple genes (ITS rDNA, partial LSU rDNA, tef 1-α, BT2, CAL, ACT and RPB2) the isolated fungi were identified as T. dryina, Tubakia sp. (T. dryinoides s.l.), D. macrostoma and A. errabunda. The pathogenicity of the fungi was confirmed by inoculating pedunculate oak leaves, which resulted in symptoms similar to those found in the forest. The study has identified T. dryina for the first time in Austria and Serbia; A. errabunda in Austria and Slovenia, D. macrostoma in Serbia, and an undescribed species of Tubakia in all three countries. Didymella macrostoma and Tubakia sp. (T. dryinoides s.l.) have been described for the first time as causes of a leaf spot disease in pedunculate oak.
The well-known pathogen of oaks, T. dryina (Holdenrieder and Kowalski, 1989; Kowalski, 2006) was isolated from lesions on pedunculate oak leaves collected in this study. Tubakia dryina is a causative agent of Tubakia leaf spot disease (Holdenrieder and Kowalski, 1989; Munkvold and Neely, 1990; Kowalski, 2006; Lee et al., 2018). The species is widespread in Europe (Germany, Italy, Poland, Romania, Russia, UK, Netherlands), and it has been speculated that T. dryina has been introduced in other parts of the world like New Zealand and USA with pedunculate oak which is its main host (Kowalski, 2006; Braun et al., 2018; Jankowiak et al., 2022). In this work, T. dryina was isolated for the first time from pedunculate oak in Austria and Serbia and this work adds to the knowledge on its global distribution. The pathogenicity test conducted in this study confirmed that T. dryina is a causative agent of Tubakia leaf spot disease of pedunculate oak in the Mura-Drava-Danube Biosphere Reserve.
A cryptic Tubakia species, Tubakia sp. (T. dryinoides s.l.) was isolated for the first time from lesions found on pedunculate oak leaves collected in Austria, Slovenia, and Serbia. The Tubakia leaf spot was initially described from pedunculate oak in Poland in 2006, but molecular phylogenetic analyses later revealed that T. dryina represents species complex comprising several cryptic species, including T. dryinoides s.s. (Kowalski, 2006; Braun et al., 2018; Zhang et al., 2021; Zhu et al., 2022). However, T. dryinoides s.s. was described based on Japanese isolate from Q. phillyraeoides and phylogenetic analyses of Braun et al., 2018 showed that European isolates of T. dryinoides (T. dryinoides s.l.) might represent another cryptic species closely related to T. dryinoides. This study confirmed this suggestion as our isolates were morphologically and phylogenetically different from what has been described as T. dryinoides in Braun et al., 2018; Zhang et al., 2021; Zhu et al., 2022. Host range and distribution of T. dryinoides s.l. are still largely unraveled and most reports of the Tubakia leaf spot disease were based on collections of leaf spots with pycnothyria and without living cultures and molecular phylogenetic identification which is necessary to identify cryptic species of T. dryina and T. dryinoides species complexes (Braun et al., 2018). Therefore, further sampling and re-examination of all isolates of T. dryinoides s.l. are urgently needed to unravel the identity, distribution, and ecology of members of the T. dryinoides species complex.
By conducting pathogenicity tests in this study and fulfilling Koch’s postulates, Tubakia sp. (T. dryinoides s.l.) was shown to represent a pathogen of pedunculate oak. Since its discovery, T. dryinoides s.s. has been known to live as an endophyte in its host leaves (Braun et al., 2018). However, Zhang et al., 2021 isolated T. dryinoides from lesions on Q. palustris leaves in China, but a test of pathogenicity was not done. Moreover, the fungus was isolated from the margins between apparently healthy and diseased tissues and thus could also represent an endophyte or saprophyte feeding on leaves already killed by another pathogen as has been shown by Taylor and Clark, 1996 for T. dryina s.l. Furthermore, it may be possible that T. dryina s.l. isolates used for pathogenicity tests done before molecular taxonomy in the past on pedunculate oak leaves (e.g., Holdenrieder and Kowalski, 1989; Munkvold and Neely, 1990; Kowalski, 2006) represented T. dryinoides s.s. or s.l. but this can only remain a matter of speculation. The current study confirmed that Tubakia sp. (T. dryinoides s.l.) represents an additional causative agent of Tubakia leaf spot disease of pedunculate oak.
Interestingly, D. macrostoma was found to cause a leaf spot disease of pedunculate oak in this study. The fungus was isolated from spots found on leaves in the Serbian part of the Mura-Drava-Danube Biosphere Reserve. To our knowledge, this is the first report of D. macrostoma causing leaf spot disease of pedunculate oak in Serbia, and anywhere worldwide. Didymella macrostoma is known as an endophyte, saprophyte and weak parasite commonly found on woody members of the Rosaceae, Ailanthus altissima (Mill.) Swingle, Larix decidua Mill., Acer pseudoplatanus L., P. nigra J.F. Arnold and F. excelsior (de Gruyter et al., 2002; Chen et al., 2015; Jayasiri et al., 2017; Barta et al., 2022). It is often found in lesions caused by other pathogens, in soil, and it has also been described as human pathogen causing onychomycosis (de Gruyter et al., 2002; Chen et al., 2015; Hou et al., 2020b; Kukhar et al., 2020). However, in this work we showed that D. macrostoma is a pathogen of pedunculate oak, capable of causing leaf spot disease. Inoculated leaves from this study were previously wounded, which is in accordance with the description of the fungus provided by de Gruyter et al., 2002 who considered it to be a wound pathogen.
Apiognomonia errabunda was isolated from lesions formed on pedunculate oak leaves in Austria and Slovenia in this work. Apiognomonia errabunda has been known as a leaf parasite, endophyte, and saprophyte of various broadleaved trees including those from Fagaceae, Salicaceae and Tiliaceae as well as herbaceous plants (Sogonov et al., 2007; Vainio et al., 2017; Li et al., 2020; Bensaci et al., 2021). For example, in Austria, A. errabunda has been isolated as an endophyte from sessile oak leaves as well as from necrotic lesions of European beech leaves (Halmschlager et al., 1993; Cech, 2010). Moreover, A. errabunda was described as the cause of the Tilia cordata Mill. leaf petiole necrosis that has led to defoliation of T. cordata trees in urban areas of Finland in 2016 (Vainio et al., 2017). In addition, it is a well-known cause of oak anthracnose disease in temperate regions of Europe, Russia, USA, and Canada (Hepting, 1971; Sinclair et al., 1987; Bensaci et al., 2021). Apiognomonia errabunda has been isolated from pedunculate oak in Switzerland, Germany, Poland, and Russia (Sogonov et al., 2007; Boroń et al., 2019). However, numerous isolates identified using morphology only and before molecular phylogenetics were found to represent closely related A. platani which causes anthracnose of plane trees or Apiognomonia hystrix (Tode) Sogonov which causes necrosis of leaf petioles of sycamore maple, whereas some isolates were characterized as intraspecific hybrids between A. errabunda and A. hystrix (Sogonov et al., 2007; Boroń et al., 2019; Kowalski et al., 2021). In this study A. errabunda was described for the first time as a pathogen of pedunculate oak in Slovenia, Austria, and the Mura-Drava-Danube Biosphere Reserve.
This research identified new leaf diseases (Tubakia and Didymella leaf spot, Apiognomonia anthracnose) of pedunculate oak in riparian forests of the Mura-Drava-Danube Biosphere Reserve. As recently demonstrated for the T. koreana infection of Quercus acutissima Carruth., leaf diseases can reduce photosynthetic capacity even if they usually do not kill the trees (Park et al., 2021). The Tubakia leaf spot disease is especially important as the fungus can cause stem necrosis of pedunculate oak seedlings (Jankowiak et al., 2022). Moreover, lesions of Tubakia leaf spot disease can coalesce in large necrotic areas leading to leaf blotch and if petioles are necrotized the tree may experience premature defoliation (Taylor and Clark, 1996; Kowalski, 2006). Similarly, T. dryina produced extensive lesions that covered a significant section of the leaves in our inoculation experiments and resembled leaf blight. Reduced photosynthetic activity caused by leaf pathogens can be particularly harmful to young seedlings and can change the host’s biochemical defenses against subsequent biotic attack from different pathogens and pests (Oliva et al., 2014; Pap et al., 2014; Hossain et al., 2019; Amaral et al., 2022). Moreover, T. dryina caused larger lesions in this study than did Tubakia sp. (T. dryinoides s.l.), D. macrostoma and A. errabunda. Given that this is the first study to look at the pathogenic potential of D. macrostoma and Tubakia sp. (T. dryinoides s.l.) it seems likely that these pathogens have less of an impact on the oak decline phenomenon than T. dryina. Furthermore, during our 4-week experiment, lesions caused by A. errabunda developed apothecia that followed a leaf nerve, like what has previously been reported for this pathogen (Kowalski, 2006). However, these lesions did not proceed towards leaf petioles. Therefore, the pedunculate oak genotype from this study may have been less susceptible to the disease since acorns originated from the Serbian portion of the Reserve, where A. errabunda has not yet been detected. Nevertheless, the pedunculate oak seedlings were not genotyped using molecular markers and other factors, such as temperature, might not have been optimal for A. errabunda infection and growth. However, since the isolates from this study were not examined for their temperature requirements, this may only be speculated. Forest management options in the Mura-Drava-Danube Biosphere Reserve are rather limited (Vastag et al., 2020; Zlatković et al., 2021). Therefore, ecological requirements of the fungi isolated in this study as well as susceptibility of different genotypes of pedunculate oak towards Tubakia, Didymella and Apiognomonia diseases should be a matter of further research.
Pedunculate oak trees sampled in this study might have been under stress. In the past decade, health, and growth of pedunculate oak in the Reserve has been affected by various abiotic and biotic stress factors (Rađević et al., 2020; Kostić et al., 2021). The most significant stress is related to changes in the hydrological regime due to climate change and human-generated river alterations (Kostić et al., 2021; Stojanović et al., 2021; Tadić et al., 2022). This has been negatively affecting pedunculate oak because due to its ecological traits, like deep root system and preference to moist soils with occasional flooding, pedunculate oak is much more sensitive to soil moisture fluctuations compared to other oak species (Eaton et al., 2016; Kostić et al., 2022). Moreover, pedunculate oak trees suffer from continuous infestations by oak lace bug, gall wasps, spongy moth, early oak defoliators, leaf miners and leaf aphids (Nikolić et al., 2019; Csóka et al., 2020; Rađević et al., 2020; Hoch et al., 2023). However, we can only speculate that various abiotic and biotic stresses to which pedunculate oak trees have been exposed in the Reserve might have increased susceptibility of oak trees to pathogens, including those that feed and grow in the leaves. In our pathogenicity test all the fungi isolated in this study induced disease symptoms. During the experiment plants were watered regularly and kept in controlled conditions because the aim of the study was not to subject the plants to water stress. However, since it is well known that stress conditions can enhance fungal diseases of plants (Desprez-Loustau et al., 2006; Hossain et al., 2019; Zolfaghari et al., 2022) the possible influence of predisposing stress to the diseases found in this study should be further investigated in the future.
Because of competing ground vegetation (mostly invasive plants), we were unable to find any seedlings beneath the crowns of pedunculate oak trees during sample collection for this study. As a result, we were unable to determine the occurrence and severity of leaf spot and anthracnose diseases on seedlings. However, we successfully completed Koch’s postulates and demonstrated the susceptibility of seedlings to the disease by conducting pathogenicity tests on the leaves of pedunculate oak seedlings. This is important since, in the Mura-Drava-Danube Biosphere Reserve, pedunculate oak regeneration is seriously endangered (Rađević et al., 2020). As was previously mentioned, several stresses that mature trees experience result in physiological weakening of the trees which in turn reduces the number of acorns produced (Nikolić et al., 2019; Pilipović et al., 2020). Moreover, the development of acorns is inhibited by the galls produced on female catkins by the gall wasp Andricus querquscalicis (Burgsdorf, 1783; Rađević et al., 2020). Also, the feeding habits of acorn weevils Curculio spp. cause acorns to drop off early, whereas the fungus Ciboria pseudotuberosa (Cooke) Rehm infects ripe acorns after they fall to the ground and cause serious problems during acorn storage (Drekić, 2006; Rađević et al., 2020). Furthermore, there isn’t enough periodic flooding which damages Curculio spp. and provides pedunculate oak trees root system with water (Rađević et al., 2020; Kostić et al., 2022; Kesić et al., 2023). In addition, extreme high temperatures, competition from ground vegetation (i.e., Rubus spp.), invasive alien species (i.e., Amorpha fruticosa L. and Ambrosia artemisiifolia L.) and woody species (i.e., F. excelsior, Carpinus betulus L.), game browsing, E. alphitoides and D. seriata infections and C. arcuata infestation are some of the additional stressors that these seedlings face (Pap et al., 2014; Zlatković et al., 2018; Rađević et al., 2020; Drekić et al., 2023). Therefore, leaf spot and anthracnose diseases from this work may pose a further risk to the regeneration of pedunculate oak. This is especially important in the core zones of the Mura-Drava-Danube Biosphere Reserve, where it is prohibited to use common human assistance techniques including sawing acorns from local seed sources and removing competitive vegetation, to assist in regeneration of pedunculate oaks. Pedunculate oak is one of the keystone species of the Reserve (SUPERB, 2023). Therefore, follow up studies are urgently needed to investigate the occurrence, severity and distribution of leaf spot and anthracnose diseases from this study on pedunculate oak seedlings in riparian forests of the Mura-Drava-Danube Biosphere Reserve.
Located along rivers, riparian forests are of major importance to overall biodiversity and floodplain ecosystem functioning (Dybala et al., 2019; Riis et al., 2020; Zhang et al., 2023). However, riparian forests of the Mura-Drava-Danube Biosphere Reserve are threatened by multiple abiotic and biotic stressors (Lapin et al., 2021; Zlatković et al., 2021; de Groot et al., 2022), including Tubakia, Didymella and Apiognomonia leaf diseases of pedunculate oak identified in this study. Future directions for studying leaf diseases in the Reserve should include sampling of other valuable riparian tree species like poplars and willows, and more thorough surveys with greater number of examined leaves and fungal isolates. Moreover, Penicillium spp., Biscogniauxia sp., and Aureobasidium sp. isolated in this research should be tested for biological control potential against Tubakia, Didymella and Apiognomonia leaf diseases of pedunculate oak.
Data availability statement
The datasets presented in this study can be found in online repositories. The names of the repository/repositories and accession number(s) can be found in the article/Supplementary material.
Author contributions
MZ: Writing−review and editing, Writing−original draft, Visualization, Validation, Software, Project administration, Methodology, Investigation, Formal analysis, Data curation, Conceptualization. MS: Writing−review and editing, Visualization, Project administration, Investigation. SSc: Writing−review and editing. TC: Writing−review and editing, Resources, Investigation. MD: Writing−review and editing, Visualization, Software, Formal analysis, Data curation. GH: Writing−review and editing. KL: Writing−review and editing. NO: Writing−review and editing, Validation, Investigation, Data curation. BP: Writing−review and editing, Software, Resources, Formal analysis, Data curation. KS: Writing−review and editing, Formal analysis, Data curation. SSt: Writing−review and editing, Methodology. MW: Writing−review and editing, Funding acquisition. SO: Writing−review and editing, Supervision, Resources, Methodology, Funding acquisition.
Funding
The author(s) declare financial support was received for the research, authorship, and/or publication of the article. This research was funded by the Resilient riparian forests as ecological corridors in the Mura-Drava-Danube Biosphere Reserve (DTP2-044-2.3-REFOCuS, Interreg-Danube Transnational Programme, co-funded by European Union); Ministry of Science, Technological development, and Innovations of the Republic of Serbia (contract no. 451-03-47/2023-01/200197), Ministry of Agriculture, Forestry and Fisheries-Forest Service (Monitoring and Forecasting Service-PIS, contract no. 401-00-58/2020-10) and Centre of Excellence Agro-U-For (contract no. 451-03-1524/2023-04/17). BP, NO, and MW acknowledge the research core group “Forest biology, ecology, and technology” (P4-0107) financed by the Slovenian Research and Innovation Agency.
Acknowledgments
We are grateful to colleagues at the public enterprise “Vojvodina Forests” and Dr. Leopold Poljaković-Pajnik (ILFE) for logistical support. We also thank MSc Sreten Vasić (ILFE), Dr. Lazar Kesić (ILFE), and Zina Devetak (SFI) for laboratory assistance; Simon Zidar (SFI) and Dr. Andreja Kavčič (SFI) for field work assistance and the two reviewers for suggestions that led to significant improvements of the manuscript.
Conflict of interest
The authors declare that the research was conducted in the absence of any commercial or financial relationships that could be construed as a potential conflict of interest.
Publisher’s note
All claims expressed in this article are solely those of the authors and do not necessarily represent those of their affiliated organizations, or those of the publisher, the editors and the reviewers. Any product that may be evaluated in this article, or claim that may be made by its manufacturer, is not guaranteed or endorsed by the publisher.
Supplementary material
The Supplementary Material for this article can be found online at: https://www.frontiersin.org/articles/10.3389/ffgc.2024.1363141/full#supplementary-material
References
Amaral, J., Valledor, L., Alves, A., Martín-García, J., and Pinto, G. (2022). Studying tree response to biotic stress using a multi-disciplinary approach: The pine pitch canker case study. Front. Plant Sci. 13:916138. doi: 10.3389/fpls.2022.916138
Barta, M., Pastirčáková, K., Ostrovský, R., Kobza, M., and Kádasi Horáková, M. (2022). Culturable endophytic fungi in Fraxinus excelsior and their interactions with Hymenoscyphus fraxineus. Forests 13:1098. doi: 10.3390/f13071098
Bensaci, O. A., Beghami, R., and Gouaref, K. (2021). First report of Apiognomonia errabunda on Quercus ilex in Algeria. Folia For. Pol. 63, 10–20. doi: 10.2478/ffp-2021-0002
Bhunjun, C. S., Phillips, A. J., Jayawardena, R. S., Promputtha, I., and Hyde, K. D. (2021). Importance of molecular data to identify fungal plant pathogens and guidelines for pathogenicity testing based on Koch’s postulates. Pathogens 10:1096. doi: 10.3390/pathogens10091096
Bjerkander, C. (1795): Phalæna Ekebladella En ny Nattfjäril beskrifven. — Kongl. Vetenskaps academiens nya Handlingar 16, 58–63.
Boroń, P., and Grad, B. (2017). The occurrence of Tubakia dryina in Poland–new hosts and ITS variation. For. Pathol. 47:e12294. doi: 10.1111/efp.12294
Boroń, P., Grad, B., Nawrot-Chorabik, K., and Kowalski, T. (2019). The genetic relationships within Apiognomonia errabunda and related species. Mycologia 111, 541–550. doi: 10.1080/00275514.2019.1631063
Bradshaw, M., Braun, U., and Pfister, D. H. (2022). Powdery mildews on Quercus: A worldwide distribution and rediscovered holotype provide insights into the spread of these ecologically important pathogens. For. Pathol. 52:e12742. doi: 10.1111/efp.12742
Brady, C., Arnold, D., McDonald, J., and Denman, S. (2017). Taxonomy and identification of bacteria associated with acute oak decline. World J. Microbiol. Biotechnol. 33, 1–11.
Brady, C., Denman, S., Kirk, S., Venter, S. Rodríguez-Palenzuela, P., and Coutinho, T. (2010). Description of Gibbsiella quercinecans gen. nov., sp. nov., associated with Acute Oak Decline. Syst. Appl. Microbiol. 33, 444–450. doi: 10.1016/j.syapm.2010.08.006
Braun, U., Nakashima, C., Crous, P. W., Groenewald, J. Z., Moreno-Rico, O., Rooney-Latham, S., et al. (2018). Phylogeny and taxonomy of the genus Tubakia s. lat. Fungal Syst. Evol. 1, 41–99. doi: 10.3114/fuse.2018.01.04
Burgsdorf (1783) in Raper C (2023) Andricus quercuscalicis f. agamic. United Kingdom Species Inventory (UKSI). Version 37.9. Natural History Museum. Checklist dataset https://doi.org/10.15468/rm6pm4 accessed via GBIF.org on 2024-03-29.
Carbone, I., and Kohn, L. (1999). A method for designing primer sets for speciation studies in filamentous ascomycetes. Mycologia 91, 553–556.
Chen, Q., Hou, L. W., Duan, W. J., Crous, P. W., and Cai, L. (2017). Didymellaceae revisited. Stud. Mycol. 87, 105–159.
Chen, Q., Jiang, J. R., Zhang, G. Z., Cai, L., and Crous, P. W. (2015). Resolving the Phoma enigma. Stud. Mycol. 82, 137–217.
Csóka, G., Hirka, A., Mutun, S., Glavendekić, M., Mikó, Á, Szõcs, L., et al. (2020). Spread and potential host range of the invasive oak lace bug [Corythucha arcuata (Say, 1832)–Heteroptera: Tingidae] in Eurasia. Agric. For. Entomol. 22, 61–74.
de Groot, M., Schueler, S., Sallmannshofer, M., Virgillito, C., Kovacs, G., Cech, T., et al. (2022). Forest management, site characteristics and climate change affect multiple biotic threats in riparian forests. For. Ecol. Manag. 508:120041. doi: 10.1016/j.foreco.2022.120041
de Gruyter, J., Boerema, G. H., and van der Aa, H. (2002). Contributions towards a monograph of Phoma (Coelomycetes) VI-2. Section Phyllostictoides: Outline of its taxa. Pers. Mol. Phylogeny Evol. Fungi 18, 1–52.
Demeter, L., Molnár, ÁP., Öllerer, K., Csóka, G., Kiš, A., Vadász, C., et al. (2021). Rethinking the natural regeneration failure of pedunculate oak: The pathogen mildew hypothesis. Biol. Conserv. 253:108928. doi: 10.1016/j.biocon.2020.108928
Denman, S., Brown, N., Kirk, S., Jeger, M., and Webber, J. (2014). A description of the symptoms of Acute Oak Decline in Britain and a comparative review on causes of similar disorders on oak in Europe. For. Int. J. For. Res. 87, 535–551.
Desprez-Loustau, M. L., Marçais, B., Nageleisen, L. M., Piou, D., and Vannini, A. (2006). Interactive effects of drought and pathogens in forest trees. Ann. For. Sci. 63, 597–612.
Drekić, M. (2006). Proučavanje štetnih insekata žira hrasta lužnjaka u semenskoj plantaži u Banovom Brodu (A study of insect pests of the pedunculate oak acorns in the seed plantation in Banovo Brod, M.A. thesis. Novi Sad: University of Novi Sad.
Drekić, M., Kovačević, B., Poljaković Pajnik, L., Pilipović, A., Milović, M., and Rađević, V. (2023). Impact of oak lace bug Corythucha arcuata on the height of pedunculate oak plants depending on the way of regeneration. Šumarski List 147, 457–463.
Dybala, K. E., Matzek, V., Gardali, T., and Seavy, N. E. (2019). Carbon sequestration in riparian forests: A global synthesis and meta-analysis. Glob. Change Biol. 25, 57–67. doi: 10.1111/gcb.14475
Eaton, E., Caudullo, G., Oliveira, S., and de Rigo, D. (2016). “Quercus robur and Quercus petraea in Europe: Distribution, habitat, usage and threats,” in European atlas of forest tree species, eds J. San-Miguel-Ayanz, D. de Rigo, G. Caudullo, T. Houston Durrant, and A. Mauri (Luxembourg: Publications Office of the European Union).
Galić, Z., Ponjarac, R., Samardžić, M., Novčić, Z., and Kiš, A. (2019). Spatial distribution and production characteristics of soil in MU “Kolut Kozara”. Topola 204, 71–78.
Gardes, M., and Bruns, T. D. (1993). ITS primers with enhanced specificity for basidiomycetes-application to the identification of mycorrhizae and rusts. Mol. Ecol. 2, 113–118. doi: 10.1111/j.1365-294x.1993.tb00005.x
Glass, N. L., and Donaldson, G. C. (1995). Development of primer sets designed for use with the PCR to amplify conserved genes from filamentous ascomycetes. Appl. Environ. Microbiol. 61, 1323–1330.
Guindon, S., Dufayard, J. F., Lefort, V., Anisimova, M., Hordijk, W., and Gascuel, O. (2010). New algorithms and methods to estimate maximum-likelihood phylogenies: Assessing the performance of PhyML 3.0. Syst. Biol. 59, 307–321. doi: 10.1093/sysbio/syq010
Halmschlager, E., Butin, H., and Donaubauer, E. (1993). Endophytic fungi in leaves and twigs of Quercus petraea. Eur. J. For. Pathol. 23, 51–63.
Hepting, G. H. (1971). Diseases of forest and shade trees of the United States. Washington, DC: US Department of Agriculture, Forest Service.
Hoch, G., Stemmelen, A., Eötvös, C. B., Hinterstoisser, W., Lanšćak, M., Stojnić, S., et al. (2023). Infestation intensity by the invasive oak lace bug, Corythucha arcuata (Say) in mixed and pure oak stands. J. Appl. Entomol. 148, 26–33. doi: 10.1111/jen.13205
Holdenrieder, O., and Kowalski, T. (1989). Pycnidial formation and pathogenicity in Tubakia dryina. Mycol. Res. 92, 166–169.
Hossain, M., Veneklaas, E. J., Hardy, G. E. S. J., and Poot, P. (2019). Tree host-pathogen interactions as influenced by drought timing: Linking physiological performance, biochemical defense and disease severity. Tree Physiol. 39, 6–18. doi: 10.1093/treephys/tpy113
Hou, L., Groenewald, J. Z., Pfenning, L. H., Yarden, O., Crous, P. W., and Cai, L. (2020a). The phoma-like dilemma. Stud. Mycol. 96, 309–396. doi: 10.1016/j.simyco.2020.05.001
Hou, L., Hernández-Restrepo, M., Groenewald, J. Z., Cai, L., and Crous, P. W. (2020b). Citizen science project reveals high diversity in Didymellaceae (Pleosporales. Dothideomycetes). MycoKeys 65:49. doi: 10.3897/mycokeys.65.47704
Jankowiak, R., Stępniewska, H., Bilański, P., and Taerum, S. J. (2022). Fungi as potential factors limiting natural regeneration of pedunculate oak (Quercus robur) in mixed-species forest stands in Poland. Plant Pathol. 71, 805–817.
Jayasiri, S. C., Hyde, K. D., Jones, E. B. G., Jeewon, R., Ariyawansa, H. A., Bhat, J. D., et al. (2017). Taxonomy and multigene phylogenetic evaluation of novel species in Boeremia and Epicoccum with new records of Ascochyta and Didymella (Didymellaceae). Mycosphere 8, 1080–1101.
Katoh, K., Rozewicki, J., and Yamada, K. D. (2019). MAFFT online service: Multiple sequence alignment, interactive sequence choice and visualization. Brief. Bioinform. 20, 1160–1166. doi: 10.1093/bib/bbx108
Kebert, M., Kostić, S., Zlatković, M., Stojnic, S., Čapelja, E., Zorić, M., et al. (2022). Ectomycorrhizal fungi modulate biochemical response against powdery mildew disease in Quercus robur L. Forests 13:1491. doi: 10.3390/f13091491
Kesić, L., Čater, M., Orlović, S., Matović, B., Stojanović, M., and Bojović, M. (2023). Proximity to riverbed influences physiological response of adult pedunculate oak trees. Topola 211, 21–28.
Köck, G., Schwach, G., and Mohl, A. (2022). Editorial Mura-Drava-Danube biosphere reserve: A long way from the original idea to the designation of the world’s first 5-country biosphere reserve. Int. J. Environ. Sustain. Dev. 21, 253–269.
Kostić, S., Kesić, L., Matović, B., Orlović, S., Stojnić, S., and Stojanović, D. B. (2021). Soil properties are significant modifiers of pedunculate oak (Quercus robur L.) radial increment variations and their sensitivity to drought. Dendrochronologia 67:125838. doi: 10.1016/j.dendro.2021.125838
Kostić, S., Levanič, T., Orlović, S., Matović, B., and Stojanović, D. B. (2022). Turkey oak (Quercus cerris L.) is more drought tolerant and better reflects climate variations compared to pedunculate oak (Quercus robur L.) in lowland mixed forests in northwestern Serbia: A stable carbon isotope ratio (δ13C) and radial growth approach. Ecol. Indic. 142:109242. doi: 10.1016/j.ecolind.2022.109242
Kovač, M., Diminić, D., Orlović, S., and Zlatković, M. (2021). Botryosphaeria dothidea and Neofusicoccum yunnanense causing canker and die-back of Sequoiadendron giganteum in Croatia. Forests 12:695. doi: 10.3390/f12060695
Kowalski, T. (2006). Tubakia dryina symptoms and pathogenicity to Quercus robur. Acta Mycol. 41, 299–304.
Kowalski, T., Bilański, P., and Grad, B. (2021). The occurrence of Apiognomonia hystrix and its pathogenicity towards Acer pseudoplatanus and Fraxinus excelsior under field conditions. Forests 13:35. doi: 10.3390/f13010035
Kukhar, E., Smagulova, A., and Kiyan, V. (2020). Biological properties of Phoma macrostoma related to non-dermatophyte onychomycosis. Med. Mycol. Case Rep. 27, 55–58. doi: 10.1016/j.mmcr.2020.01.005
Lapin, K., Bacher, S., Cech, T., Essl, F., Georges, F. I., Hoch, G., et al. (2021). Comparing environmental impacts of alien plants, insects and pathogens in protected riparian forests. NeoBiota 69, 1–28.
Lee, D. H., Seo, S. T., Lee, S. K., and Lee, S. K. (2018). Leaf spot disease on seedlings of Quercus acutissima caused by Tubakia dryina in Korea. Australas. Plant Dis. Notes 13, 1–3.
Li, W. J., McKenzie, E. H., Liu, J. K., Bhat, D. J., Dai, D. Q., Camporesi, E., et al. (2020). Taxonomy and phylogeny of hyaline-spored coelomycetes. Fungal Divers. 100, 279–801.
Linnaeus, C. (1758). Systema naturae per regna tria naturae, secundum classes, ordines, genera, species, cum characteribus, differentiis, synonymis, locis. Laurentii Salvii, Holmiae, 10th ed. Vol. 1. Available online at https://biodiversitylibrary.org/page/726886
Liu, Y. J., Whelen, S., and Hall, B. D. (1999). Phylogenetic relationships among ascomycetes: Evidence from an RNA polymerase II subunit. Mol. Biol. Evol. 16, 1799–1808.
Milanović, S., Milenković, I., Dobrosavljević, J., Popović, M., Solla, A., Tomšovský, M., et al. (2020). Growth rates of Lymantria dispar larvae and Quercus robur seedlings at elevated CO2 concentration and Phytophthora plurivora infection. Forests 11:1059. doi: 10.3390/f11101059
Mölder, A., Meyer, P., and Nagel, R. V. (2019). Integrative management to sustain biodiversity and ecological continuity in Central European temperate oak (Quercus robur, Q. petraea) forests: An overview. For. Ecol. Manag. 437, 324–339.
Nikolić, N., Pilipović, A., Drekić, M., Kojić, D., Poljaković-Pajnik, L., Orlović, S., et al. (2019). Physiological responses of pedunculate oak (Quercus robur L.) to Corythucha arcuata (Say, 1832) attack. Arch. Biol. Sci. 71, 167–176.
O’Donnell, K., Kistler, H. C., Cigelnik, E., and Ploetz, R. C. (1998). Multiple evolutionary origins of the fungus causing Panama disease of banana: Concordant evidence from nuclear and mitochondrial gene genealogies. Proc. Natl. Acad. Sci. U.S.A. 95, 2044–2049.
Oettel, J., Braun, M., Sallmannshofer, M., De Groot, M., Schueler, S., Virgillito, C., et al. (2022). River distance, stand basal area, and climatic conditions are the main drivers influencing lying deadwood in riparian forests. For. Ecol. Manag. 520:120415. doi: 10.1016/j.foreco.2022.120415
Oliva, J., Stenlid, J., and Martinez-Vilalta, J. (2014). The effect of fungal pathogens on the water and carbon economy of trees: Implications for drought-induced mortality. New Phytol. 203, 1028–1035. doi: 10.1111/nph.12857
Pap, P., Stojnić, S., Nikolić, N., Orlović, S., Marković, M., Vasić, V., et al. (2014). Impact of Erysiphe alphitoides (Griffon & Maubl.) U. Braun & S. takam. on leaf physiological parameters in pedunculate oak (Quercus robur L.) saplings. Balt. For. 20, 2–9.
Park, M., Yun, H. Y., Kim, Y. H., Kim, K., and Kim, H. S. (2021). Non-linear reduction of photosynthetic ability in oak trees infected with Tubakia koreana causing Quercus leaf blight. For. Pathol. 51:12665. doi: 10.1111/efp.12665
Pilipović, A., Drekić, M., Stojnić, S., Nikolić, N., Trudić, B., Milović, M., et al. (2020). Physiological responses of two pedunculate oak (Quercus robur L.) families to combined stress conditions–drought and herbivore attack. Šumarski List 144, 573–582.
Rađević, V., Pap, P., and Vasić, V. (2020). Management of the common oak forests in Ravni Srem: Yesterday, today, tomorrow. Topola 206, 41–52.
Ramsar (2023). The convention on wetlands. Available online at: https://www.ramsar.org/ (accessed November 12, 2023).
Riis, T., Kelly-Quinn, M., Aguiar, F. C., Manolaki, P., Bruno, D., Bejarano, M. D., et al. (2020). Global overview of ecosystem services provided by riparian vegetation. Bioscience 70, 501–514.
Ronquist, F., Teslenko, M., Van Der Mark, P., Ayres, D. L., Darling, A., Höhna, S., et al. (2012). MrBayes 3.2: Efficient Bayesian phylogenetic inference and model choice across a large model space. Syst. Biol. 61, 539–542. doi: 10.1093/sysbio/sys029
Ruffner, B., Schneider, S., Meyer, J., Queloz, V., and Rigling, D. (2020). First report of acute oak decline disease of native and non-native oaks in Switzerland. New Dis. Rep. 41:18. doi: 10.5197/j.2044-0588.2020.041.018
Sallmannshofer, M., Chakraborty, D., Vacik, H., Illés, G., Löw, M., Rechenmacher, A., et al. (2021). ). Continent-Wide tree species distribution models may mislead regional management decisions: A case study in the transboundary biosphere reserve Mura-Drava-Danube. Forests 12:330. doi: 10.3390/f12030330
Say, T. (1832). Descriptions of new species of Heteropterous Hemiptera of North America 5–39. New Harmony, IN. Available online at: https://catpalhet.linnaeus.naturalis.nl/linnaeus_ng/app/views/literature2/reference.php?id=6731
Sinclair, W. A., Lyon, H. H., and Johnson, W. T. (1987). Diseases of trees and shrubs. Ithaca, NY: Comstock Publishng Associates.
Skiadaresis, G., Schwarz, J. A., and Bauhus, J. (2019). Groundwater extraction in floodplain forests reduces radial growth and increases summer drought sensitivity of pedunculate oak trees (Quercus robur L.). Front. For. Glob. Change 2:5. doi: 10.3389/ffgc.2019.00005
Sogonov, M. V., Castlebury, L. A., Rossman, A. Y., and White, J. F. (2007). The type species of Apiognomonia, A. veneta, with its Discula anamorph is distinct from A. errabunda. Mycol. Res. 111, 693–709.
Stojanović, D. B., Orlović, S., Zlatković, M., Kostić, S., Vasić, V., Miletić, B., et al. (2021). Climate change within Serbian forests: Current state and future perspectives. Topola 208, 39–56.
Sung, G. H., Sung, J. M., Hywel-Jones, N. L., and Spatafora, J. W. (2007). A multi-gene phylogeny of Clavicipitaceae (Ascomycota, fungi): Identification of localized incongruence using a combinational bootstrap. Mol. Phylogenet. Evol. 44, 1204–1223. doi: 10.1016/j.ympev.2007.03.011
SUPERB (2023). SUPERB: Upscaling forest restoration. Available online at: https://forest-restoration.eu/ (accessed November 9, 2023).
Swafford, D. L. (2002). PAUP*. Phylogenetic analysis using parsimony (* and other methods). Sunderland, MA: Sinauer Associates.
Tadić, L., Tamás, E. A., Mihaljević, M., and Janjić, J. (2022). Potential climate impacts of hydrological alterations and discharge variabilities of the Mura, Drava, and Danube rivers on the natural resources of the MDD UNESCO biosphere reserve. Climate 10:139. doi: 10.3390/cli10100139
Tamura, K., Stecher, G., and Kumar, S. (2021). MEGA11: Molecular evolutionary genetics analysis version 11. Mol. Biol. Evol. 38, 3022–3027. doi: 10.1093/molbev/msab120
Taylor, J., and Clark, S. (1996). Infection and fungal development of Tubakia dryina on sweet gum (Liquidambar styraciflua). Mycologia 88, 613–618.
Terhonen, E., Kovalchuk, A., Zarsav, A., and Asiegbu, F. O. (2018). “Biocontrol potential of forest tree endophytes,” in The endophytes of forest trees: Biology and applications, 2nd Edn, eds A. M. Pirttila and A. C. Frank (Cham: Springer), 283–318.
Trišić, I., Privitera, D., Štetić, S., Petrović, M. D., Radovanović, M. M., Maksin, M., et al. (2022). Sustainable tourism to the part of transboundary UNESCO biosphere reserve “Mura-Drava-Danube”. A case of Serbia, Croatia and Hungary. Sustainability 14:6006. doi: 10.3390/su14106006
Udayanga, D., Castlebury, L. A., Rossman, A. Y., and Hyde, K. D. (2014). Species limits in Diaporthe: Molecular re-assessment of D. citri, D. cytosporella, D. foeniculina and D. rudis. Pers. Mol. Phylogeny Evol. 32, 83–101.
Vainio, E. J., Velmala, S. M., Salo, P., Huhtinen, S., and Müller, M. M. (2017). Defoliation of Tilia cordata trees associated with Apiognomonia errabunda infection in Finland. Silva Fennica 51:7749. doi: 10.14214/sf.7749
Vastag, E., Cocozza, C., Orlović, S., Kesić, L., Kresoja, M., and Stojnić, S. (2020). Half-sib lines of pedunculate oak (Quercus robur L.) respond differently to drought through biometrical, anatomical and physiological traits. Forests 11:153. doi: 10.3390/f11020153
Vilgalys, R., and Hester, M. (1990). Rapid genetic identification and mapping of enzymatically amplified ribosomal DNA from several Cryptococcus species. J. Bacteriol. 172, 4238–4246. doi: 10.1128/jb.172.8.4238-4246.1990
White, T. J., Bruns, T., Lee, S., Taylor, J., Innis, M. A., Gelfand, D. H., et al. (1990). “Amplification and direct sequencing of fungal ribosomal RNA genes for phylogenetics,” in PCR protocols: A guide to methods and applications, eds M. A. Innis, D. H. Gelfand, J. J. Sninsky, and T. J. White (New York, NY: Academic Press), 315–322.
Xu, T., Weng, B., Yan, D., Wang, K., Li, X., Bi, W., et al. (2019). Wetlands of international importance: Status, threats, and future protection. Int. J. Environ. Res. Public Health 16:1818. doi: 10.3390/ijerph16101818
Zhang, X., Ci, X., Hu, J., Bai, Y., Thornhill, A. H., Conran, J. G., et al. (2023). Riparian areas as a conservation priority under climate change. Sci. Total Environ. 858:159879. doi: 10.1016/j.scitotenv.2022.159879
Zhang, Z., Mu, T., Liu, S., Liu, R., Zhang, X., and Xia, J. (2021). Morphological and phylogenetic analyses reveal a new genus and two new species of Tubakiaceae from China. MycoKeys 84:185. doi: 10.3897/mycokeys.84.73940
Zhu, Y. Q., Jiang, N., Dou, Z. P., Xue, H., Piao, C. G., and Li, Y. (2022). Additions to the knowledge of Tubakia (Tubakiaceae, Diaporthales) in China. J. Fungi 8:1143. doi: 10.3390/jof8111143
Zlatković, M., Keča, N., Wingfield, M. J., Jami, F., and Slippers, B. (2016). Botryosphaeriaceae associated with the die-back of ornamental trees in the Western Balkans. Anton. Leeuw. 109, 543–564. doi: 10.1007/s10482-016-0659-8
Zlatković, M., Pap, P., Tenorio-Baigorria, I., Koltay, A., Ogris, N., and Cech, T. (2021). “Diseases of poplars and their hybrids with an emphasis on disease management recommendations,” in Perspectives for forest and conservation management in riparian forests, eds M. Sallmannshofer, S. Schuller, and M. Westergren (Ljubljana: Slovenian Forestry Institute, Silva Slovenica Publishing Centre), 126–132.
Zlatković, M., Wingfield, M. J., Jami, F., and Slippers, B. (2018). Host specificity of co-infecting Botryosphaeriaceae on ornamental and forest trees in the Western Balkans. For. Pathol. 48:e12410. doi: 10.1111/efp.12410
Keywords: Mura-Drava-Danube Biosphere Reserve, riparian forests, leaf spot diseases, Tubakia leaf spot, Didymella macrostoma, oak anthracnose, pedunculate oak
Citation: Zlatković M, Sallmannshofer M, Schueler S, Cech TL, Djilas M, Hoch G, Lapin K, Ogris N, Piškur B, Schwanda K, Stojnić S, Westergren M and Orlović S (2024) Tubakia spp., Didymella macrostoma and Apiognomonia errabunda causing leaf spot and anthracnose of Quercus robur in the Mura-Drava-Danube Biosphere Reserve. Front. For. Glob. Change 7:1363141. doi: 10.3389/ffgc.2024.1363141
Received: 29 December 2023; Accepted: 11 March 2024;
Published: 17 April 2024.
Edited by:
Christopher Asaro, Forest Health Protection, Forest Service (USDA), United StatesReviewed by:
Carmen Romeralo, Spanish National Research Council (CSIC), SpainCaterina Villari, University of Georgia, United States
Copyright © 2024 Zlatković, Sallmannshofer, Schueler, Cech, Djilas, Hoch, Lapin, Ogris, Piškur, Schwanda, Stojnić, Westergren and Orlović. This is an open-access article distributed under the terms of the Creative Commons Attribution License (CC BY). The use, distribution or reproduction in other forums is permitted, provided the original author(s) and the copyright owner(s) are credited and that the original publication in this journal is cited, in accordance with accepted academic practice. No use, distribution or reproduction is permitted which does not comply with these terms.
*Correspondence: Milica Zlatković, bWlsaWNhLnpsYXRrb3ZpY0B1bnMuYWMucnM=