- Department of Economic Theories, Faculty of Economics and Management, Czech University of Life Sciences Prague, Prague, Czechia
Woody trees are invaluable to ecosystems and economies, providing essential resources such as timber, fuel, food, and medicine. Trees also play a critical role in carbon sequestration, biodiversity conservation, and soil stabilization, making their health and productivity vital in the face of global environmental challenges. However, the changing climate and escalating threats from pests, diseases, and abiotic stresses pose significant risks to woody tree species, necessitating the development of sustainable approaches to enhance their growth and resilience. One promising strategy is artificial polyploidization, a biotechnological method that induces multiple complete sets of chromosomes, which has been successfully used in agriculture and horticulture to improve plant traits like growth and stress resistance. Despite its proven benefits in herbaceous and crop plants, polyploid induction remains underutilized in woody trees. It holds potential for enhancing both the ecological roles of woody trees, as well as their economically valuable characteristics. This mini-review explores the potential of polyploidy as a sustainable tool to boost growth and stress resilience in woody species. The mini-review examines the potential challenges associated with polyploid induction in woody trees, including technical difficulties and knowledge gaps, while also providing future directions for research and application.
1 Introduction
Polyploidy, the condition of possessing more than two complete sets of chromosomes, is a widespread phenomenon in the plant kingdom that has played a pivotal role in plant evolution (Madlung, 2013). Trees have gained increased genetic diversity through polyploidy, leading to enhanced adaptability, novel traits, and speciation. In nature, polyploidy occurs spontaneously, often resulting in new plant lineages that exhibit unique characteristics advantageous for survival in diverse environments (Heslop-Harrison et al., 2023). Artificial polyploidy, in contrast, is induced by human intervention through techniques that double or multiply chromosome sets. Unlike natural polyploidy, which develops over evolutionary timescales, artificial polyploidy is a rapid and controllable process widely used in experimental and agricultural settings (Niazian and Nalousi, 2020). Artificial polyploidy has been used to induce enhanced growth, and increased resistance to biotic and abiotic stresses in economically important plants (Wu et al., 2012; Sabooni and Gharaghani, 2022; Wen et al., 2022; Bharati et al., 2023a).
Despite its success in herbaceous crops, medicinal plants, and ornamentals, the application of artificial polyploidization in woody tree species remains comparatively underexplored. Woody trees are vital components of ecosystems, playing a pivotal role in maintaining environmental stability and ecological balance. They contribute significantly to global climate regulation through carbon sequestration, moderate the hydrological cycle, reduce soil erosion (Grassi et al., 2017; Liu et al., 2018). In forest ecosystems, trees act as long-term carbon sinks and serve as natural barriers against the adverse effects of climate change, making them indispensable to global environmental health. In addition to their ecological importance, woody trees hold substantial economic value. They serve as primary sources of timber, fuelwood, fiber, paper, food, essential oils, and medicinal compounds (Kebebew and Ozanne, 2024). However, their long lifespans and slow growth rates pose significant challenges for genetic improvement, limiting the pace of breeding programs aimed at enhancing traits like stress resilience, wood quality, or productivity through conventional approaches.
Traditional plant breeding methods, which primarily relies on selective breeding and hybridization to enhance desirable traits, have been the cornerstone of crop and forestry improvement. However, these approaches often face limitations such as long generation times, low genetic variability, and susceptibility to environmental influences, challenges that are particularly pronounced in woody trees. On contrary, induced polyploidy offers a promising approach to accelerate genetic improvement of woody tree species that would otherwise take decades to achieve through other traditional breeding methods (Touchell et al., 2020).
Artificial polyploidy holds potential for improving both the ecological functions of woody trees, such as maintaining environmental stability and promoting ecological balance, and their economically valuable traits. While still a genetic modification approach, artificial polyploidization is broadly accepted as one of the traditional methods and does not face as much scrutiny as transgenic approaches, that involves introduction of foreign gene or targeted modification of genomic structure, and are generally exempted from GMO regulations (Martínez-Fortún et al., 2022; Winter, 2024). This regulatory flexibility enhances its appeal as a strategy for improving traits in woody trees, especially in response to environmental and climate pressures. However, ecological considerations should be carefully considered depending on the specific research objectives.
By exploring recent research, methodologies, and applications, this mini-review aims to highlight how artificially induced polyploidy can offer effective approach as a tool to enhance growth performance and stress resistance in woody trees. The mini-review also aims to address the potential challenges associated with induced polyploidy in improving economically valuable woody tree species.
2 Effects of artificial polyploidy on growth traits and stress resistance in woody trees
In hardwood trees, several key traits are particularly valuable, including enhanced growth characteristics, improved photosynthetic efficiency, enhanced resistance to abiotic and biotic stress, and increased biomass production. Polyploid-mediated trait improvement in hardwood trees can address multiple challenges simultaneously, from supporting the timber industry to enhancing ecosystem services and combating climate change, while potentially increasing forest resilience and adaptability to future environmental conditions.
Polyploidy in hardwoods often leads to rapid growth, with triploid poplars growing up to 2–3 times faster in volume than diploid controls (Kang and Wei, 2022). This accelerated growth can result in higher timber yields and improved wood quality. However, rapid growth is not always a guaranteed outcome of polyploidy, as instances of reduced growth rates following polyploid induction have also been documented in several hardwood species (Fernando et al., 2019; Wu et al., 2022).
Examination of polyploidy effects on hardwood trees reveals other range of beneficial changes across various species, particularly in leaf characteristics. For example, tetraploid Eucalyptus urophylla exhibited significantly larger leaves with increased cell size compared to their progenitors. The leaf length increased by 78.26% and leaf width by 101.15% in tetraploids (Liu et al., 2022). This trend is also observed in some Populus species, where induced tetraploids often resulted in larger and thicker leaves with modified morphology. In Populus davidiana and Populus hopeiensis tetraploids leaf area increased by about 10% (Wu et al., 2023; Wang et al., 2025). Leaf morphology is a crucial aspect affected by polyploidy in hardwood trees. These leaf modifications can significantly contribute to enhanced photosynthetic capacity, potential biomass production and net carbon assimilation. Moreover, such alterations might have additional practical applications. For instance, Eucalyptus spp. leaves are known to possess several medicinal properties, including anti-diabetic, anti-bacterial, and anti-inflammatory activities (Salvatori et al., 2023). The morphological changes associated with polyploidy may amplify the concentration or efficacy of bioactive compounds, thereby enhancing these medicinal properties. However, these aspects of induced polyploids remain largely unexplored and warrant further investigation in future studies.
With larger and darker leaves, polyploid hardwood trees often demonstrate improved photosynthetic capabilities. In Acer buergerianum, the tetraploid variety ‘Xingwang’ contained 38.66% more total chlorophyll and demonstrated 7.79% higher maximal photochemical efficiency of PSII (Fv/Fm) and 43.48% higher potential photochemical efficiency of PSII (Fv/Fo) compared to its diploid counterpart (Wang et al., 2021). Eucalyptus urophylla tetraploids exhibited a 15.8% higher net photosynthetic rate (Pn) than diploids (Liu et al., 2022). Populus davidiana tetraploids showed remarkable improvements in chlorophyll fluorescence parameters, with Fv/Fm, ΦPSII, qP, and ETR being 1.08, 2.5, 2.3, and 2.68 times higher than those of diploids, respectively (Wang et al., 2025). Similarly, Liriodendron sino-americanum tetraploids showed significantly higher values for key photosynthetic parameters, including net photosynthetic rate (1.6-fold increase), stomatal conductance (2.2-fold increase), and transpiration rate (1.6-fold increase) compared to diploids (Chen et al., 2021). These significant improvements in photosynthetic parameters across various hardwood species suggest that an increase in ploidy levels enhances photosynthetic capacity, which may potentially lead to higher growth rates and increased biomass production.
Stomatal characteristics represent another critical trait influenced by polyploidy and are among the most extensively studied features in induced polyploids of hardwood trees. Many polyploid hardwood trees exhibit larger stomata in length and width but lower in density. This trend is consistently observed across various species. For instance, in Populus hopeiensis, octoploid plants displayed a 82.24% increase in stomatal length and a 65.57% increase in stomatal width, while stomatal density decreased by 50.27% compared to diploids (Wu et al., 2022). In Poplar 84K (Populus alba × Populus glandulosa), tetraploids exhibited a 124% increase in stomatal length and a 100% increase in stomatal width, while stomatal density decreased by 50% (Ren et al., 2021). Decreases in stomatal density are a consistent feature, with reductions of 22.67% in Eucalyptus urophylla tetraploids and 37.8% in Eriobotrya japonica tetraploids compared to their diploid counterparts (Blasco et al., 2015; Liu et al., 2022). These alterations in stomatal traits can significantly influence plant water use efficiency, gas exchange, and environmental adaptability, potentially conferring polyploid trees with enhanced resilience to environmental stresses, particularly under water-limited conditions.
Polyploid induction in hardwood species shows promising potential for improving growth parameters resulting in better wood quality and timber-related traits. A study on 20-year-old Eucalyptus grandis and Eucalyptus grandis × Eucalyptus urophylla hybrids revealed that triploid and tetraploid individuals exhibited superior growth characteristics compared to diploids (Longui et al., 2021). Specifically, triploid and tetraploid trees had wider diameter at breast height (DBH) (41.1 cm and 40.3 cm DBH, respectively, vs. 34.2 cm for diploids), were taller (46.8 m and 45.1 m vs. 40.1 m), and had longer stems (32.8 m and 32.1 m vs. 28.7 m). Notably, wood density in polyploid trees demonstrated greater homogeneity across the trunk, whereas diploids showed significant radial variation. Triploid and tetraploid Eucalyptus trees produced higher density wood (727 and 771 kg/m3, respectively) than their diploid counterparts (717 kg/m3), suggesting improved timber quality (Longui et al., 2021). In terms of anatomical features, polyploid trees did not display a clear radial pattern, indicating potential alterations in wood structure. Tetraploids exhibited wider vessels than diploids, which may contribute to increased hydraulic capacitance and efficiency, whereas triploids had narrower vessels (Longui et al., 2021). Fiber length is another important trait that tends to increase with polyploidy. In another study on Eucalyptus grandis × E. urophylla hybrids, Da Silva Souza et al. (2021) found that the mean fiber length in polyploid clones was 18% higher than in diploid clones. This increase in fiber length can significantly enhance wood quality and paper production, as longer fibers generally contribute to improved paper strength and durability. Overall, these findings highlight the potential of polyploidy to enhance timber production and quality in hardwood species, though specific quantitative improvements varied between studies and species.
Artificial polyploid induction impacts several physicochemical parameters and has significant potential as a tool for enhancing abiotic and biotic stress resilience in hardwood trees. While growth traits have been well-documented, stress assessment of induced polyploid hardwood trees is significantly lacking. Studies on some tree species demonstrate that induced polyploids often exhibit superior stress tolerance compared to their diploid counterparts. In Populus davidiana, artificially induced tetraploids displayed enhanced drought and salt tolerance, with higher proline content and antioxidant enzyme activities (Wang et al., 2025). Similarly, tetraploid Robinia pseudoacacia (black locust) showed increased salt tolerance, maintaining lower levels of reactive oxygen species and membrane damage under saline conditions (Meng et al., 2012). The enhanced stress resilience in polyploids is often attributed to physiological and morphological changes, such as larger leaf area, increased stomatal size, and altered gene expression patterns (Tossi et al., 2022). In Betula platyphylla (birch), autotetraploids exhibited an upregulation of genes associated with stress responses and plant-pathogen interactions, suggesting a potential role in enhancing resilience to biotic and abiotic stresses (Mu et al., 2012). However, a systematic investigation to assess the stress tolerance of these induced tetraploids remains an important avenue for future research. Understanding stress resilience in polyploid hardwoods is crucial, especially in the face of climate change and the increasing demand for sustainable timber production. Future research should focus on developing long-term stress assessment protocols specifically tailored for polyploid woody trees. This could involve a combination of controlled environment studies, field trials, and advanced genomic analyses to unravel the mechanisms behind enhanced stress tolerance in polyploid trees.
3 Methods for inducing artificial polyploidy
The methods for inducing polyploidy in hardwood trees can be broadly categorized into chemical and physical approaches, each with distinct advantages and applications in research and breeding programs.
3.1 Chemical methods
Chemical methods are the most widely used for inducing polyploidy and involve the application of mitotic inhibitors that disrupt spindle fiber formation during cell division, leading to chromosome doubling. Among these, colchicine, derived from the autumn crocus (Colchicum autumnale), remains the most extensively employed agent due to its effectiveness, thermostability, and broad applicability across diverse plant species (Dhooghe et al., 2011). In hardwood trees, colchicine has been successfully used to induce polyploidy in several species, demonstrating its versatility. For instance, treating transected leaf explants of Populus hopeiensis with a 40 mg/L colchicine solution for 4 days resulted in a polyploid induction rate of 21.2% (Wu et al., 2023). Similarly, the application of 0.05% colchicine in Paulownia tomentosa achieved a 40% polyploid induction rate (Tang et al., 2010), highlighting its potential for breeding programs aimed at developing improved hardwood trees.
While colchicine remains a popular choice, alternative chemical agents such as oryzalin and trifluralin have gained traction in other plant species due to their lower toxicity and higher specificity in targeting tree tubulin proteins resulting in better induction rates (Bharati et al., 2024). However, their use in hardwood trees remains limited, requiring further experimental evidence to validate their effectiveness over colchicine in these species.
The choice of chemical agent, concentration, and exposure time is highly species-specific and depends on the tissue being treated. Optimization of these parameters is critical to ensure successful polyploidy induction while minimizing phytotoxicity. Researchers often conduct preliminary experiments to determine the most effective treatment protocol for each species or genotype, which may involve testing different concentrations, exposure times, and developmental stages of the tree.
3.2 Physical methods
Physical methods, though less commonly employed, have been successfully used in specific cases to induce chromosomal doubling in hardwood trees. These approaches, including radiation (X-rays or gamma rays) and temperature shock treatments, are generally less precise and efficient than chemical methods but remain valuable in certain scenarios. For instance, high-temperature treatments have been employed to induce polyploidy in multiple woody tree species. Subjecting Eucalyptus urophylla to a temperature of 44°C for 6 h on the 25th day after pollination resulted in a polyploid induction rate of 6.45% (Liu et al., 2022). Similarly, in Populus simonii Carr. and its hybrid (P. simonii × P. nigra var. Italica), triploids were induced by exposing developing seeds to 38°C for 2–4 h at 66–72 h post-pollination, while tetraploids were obtained by applying a 41°C treatment for 2 h at 168 h after pollination, achieving polyploid induction rates of 11.76 and 5.95%, respectively (Guo et al., 2017). In another case, Populus adenopoda Maxim was subjected to a 44°C treatment for 2 h, 7 days after pollination, leading to a 14.12% polyploid induction rate (Lu et al., 2014) (Table 1).
3.3 Application approaches
Polyploidy induction in hardwood trees can be achieved through either in vivo or in vitro approaches, each with distinct advantages and limitations. However, the in vitro approach is increasingly recognized as the superior method due to its precision, higher success rates, and ability to overcome the limitations associated with the in vivo approach.
The in vivo approach involves applying mitotic inhibitors directly to meristematic tissues such as seeds, buds, or seedlings in a natural growing environment. While this method is favored for its simplicity and ease of application, particularly in field-grown trees or species lacking established in vitro protocols, it faces significant challenges. For instance, this method is often hindered by difficulties in controlling environmental factors, ensuring uniform exposure of target tissues to the antimitotic agent, and minimizing phytotoxicity. Additionally, the variability in tissue response and the inability to precisely regulate treatment conditions often result in inconsistent outcomes, making in vivo approaches less reliable for large-scale breeding programs. For instance, in Eucalyptus urophylla, the in vivo application of colchicine at 0.25% for 6 h resulted in a polyploidy induction rate of only 6.25% (Yang et al., 2018). Similarly, in Acacia mangium, the highest reported polyploid induction rate achieved through in vivo treatment was just 9% (Harbard et al., 2012). These relatively low success rates highlight the limitations of in vivo methods, reinforcing the need for more controlled and efficient polyploidy induction techniques.
In contrast, the in vitro approach, conducted under controlled laboratory conditions, offers greater precision, higher success rates, and the ability to manipulate critical factors influencing polyploidy induction (Bharati et al., 2023a). This method involves treating callus cultures, shoot tips, or other extrees with chemical agents, allowing for better control over dosage, exposure time, and environmental conditions. For example, in a study where in vitro polyploidy induction in some Eucalyptus species was attempted polyploid induction of up to 66.67% percentage was achieved (Suliman and Asander, 2020). A higher polyploid induction rate in hardwood tree species has been consistently reported. Some more examples include 40% induction in Paulownia tomentosa (Tang et al., 2010), 37% in Populus alba × P. glandulosa (Ren et al., 2021), and 21.2% in Populus hopeiensis (Wu et al., 2023) (Table 1).
Moreover, the in vitro approach allows for the treatment of a wide range of explants, including shoot tips, nodal segments, and callus cultures, which can be easily monitored and manipulated. This flexibility is particularly advantageous for hardwood species, which are often recalcitrant to genetic manipulation. The controlled environment of tissue culture systems enables researchers to optimize nutrient composition, growth regulators, and light conditions, which are critical for successful polyploidy induction and subsequent plant regeneration. Additionally, the regenerated shoots can be subjected to ploidy analysis using techniques such as flow cytometry or chromosome counting simultaneously, ensuring the accurate identification of polyploid individuals before further cultivation.
In light of these advancements, the in vitro approach is increasingly regarded as the preferred method for polyploidy induction in hardwood trees. Its ability to provide controlled conditions, higher induction rates, and compatibility with modern biotechnological tools makes it a more reliable and efficient option for researchers and breeders aiming to develop improved hardwood varieties. A simplified workflow illustrating the in vitro polyploidization approach in woody tree species is shown in Figure 1.
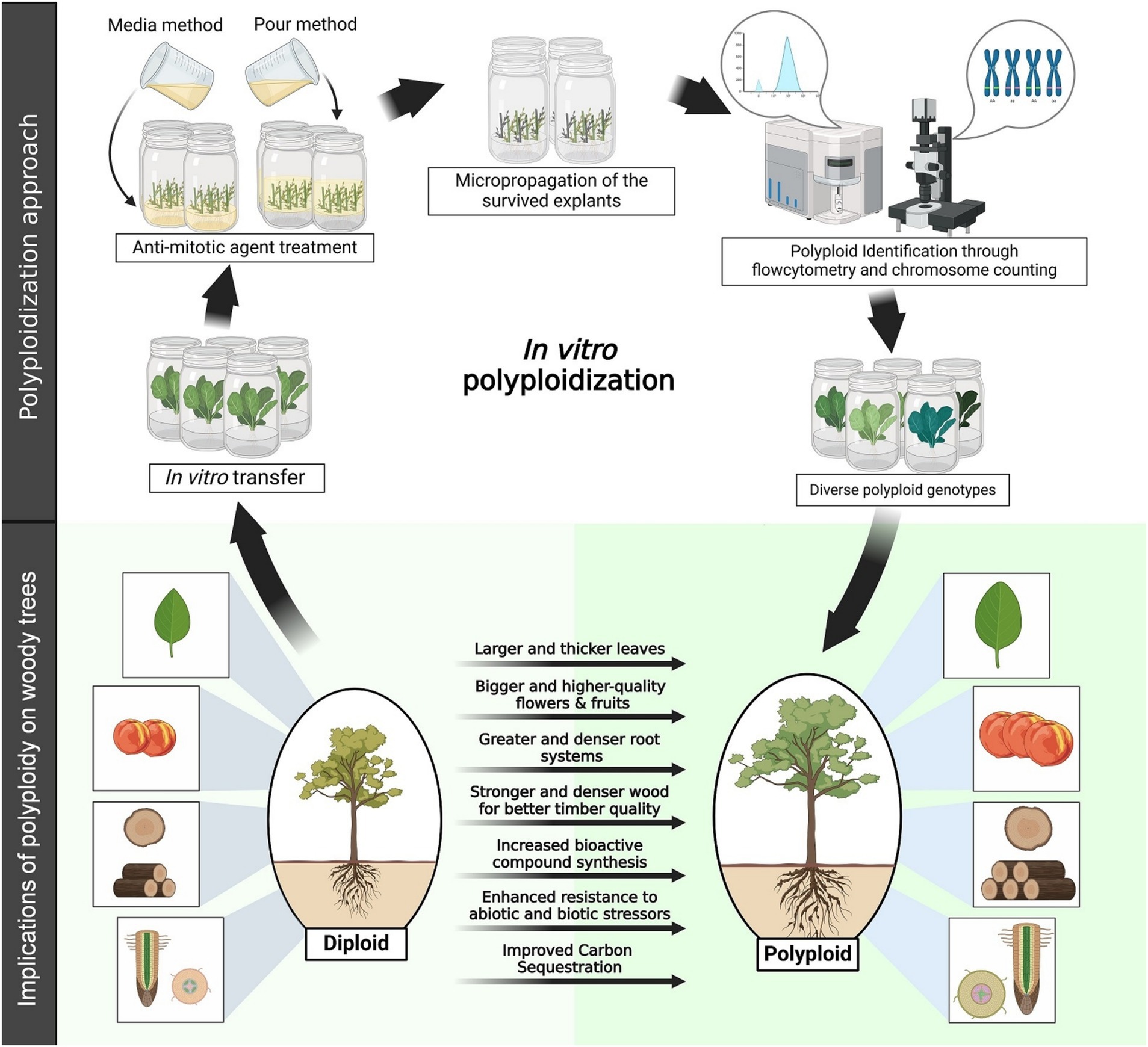
Figure 1. Schematic of in vitro polyploidization in woody trees and its trait enhancements. The upper panel outlines the polyploidization process, from anti-mitotic treatment to ploidy verification, leading to diverse polyploid genotypes. The lower panel contrasts diploid and polyploid traits, highlighting several potential key improvements, including larger, thicker leaves, enhanced flower and fruit quality, extensive roots, denser wood, higher bioactive compounds, greater stress tolerance, and increased carbon sequestration. This figure integrates laboratory methodologies with resultant phenotypic modifications, offering a comprehensive overview of artificial polyploidy induction and its benefits in woody tree species. The figure was created in BioRender.
4 Challenges and future perspectives
Woody trees are foundational to ecosystem health, biodiversity, carbon sequestration, and soil integrity. While artificial polyploidy holds immense promise for enhancing growth and stress resilience in hardwood species, several challenges must be addressed to unlock its full potential.
One of the key challenges in applying artificial polyploidization to woody species is the inherent unpredictability of outcomes. While the approach can enhance certain traits, such as stress tolerance or biomass production, it often leads to unintended consequences. Polyploid individuals may suffer from ploidy instability, where chromosomal aberrations or reversion to original ploidy levels occur over time—an especially serious issue in long-lived tree species, where negative effects may not emerge until years or even decades later. Additionally, induced polyploids can exhibit undesirable phenotypes, including reduced mechanical strength, altered secondary metabolite profiles, or abnormal reproductive structures that compromise both commercial value and ecological function. For instance, polyploid Eucalyptus polybractea has shown reduced growth, lower biomass accumulation, and decreased essential oil yield (Fernando et al., 2019). Similarly, tetraploid Gossypium arboreum displayed larger stomata and increased chloroplast content, but also produced malformed pollen, raising concerns about fertility and long-term viability (Yang et al., 2015). Reduced fertility is a common trait in polyploids, limiting their utility in breeding programs and hindering natural regeneration in forest ecosystems (Herben et al., 2017). These challenges are further compounded by the extended life cycles of hardwood trees, which make it difficult to quickly assess the long-term stability and ecological impact of induced polyploids. Critically, the absence of standardized in vitro protocols for many economically and ecologically important hardwood species severely restricts the broader application of polyploidization. While protocols exist for model species like Populus or Eucalyptus, they are lacking for high-value hardwoods and keystone forest trees, limiting scalability and reproducibility. Additionally, existing studies on polyploid woody trees disproportionately focus on morphological traits (e.g., leaf size, stomatal density), while wood-specific characteristics such as fiber length, lignin content, density, and hydraulic conductivity are scanty explored. These traits are vital for determining the commercial utility of polyploids in timber, pulp, or bioenergy industries, yet their variability in induced polyploids is poorly documented.
To address these challenges, emerging technologies must converge with traditional breeding strategies. Genomic selection can accelerate the identification of elite polyploid candidates by screening for desirable traits (e.g., stress resilience, growth vigor) early in development, minimizing reliance on lengthy field trials. Further integrating CRISPR-based genome editing with omics-driven marker systems (e.g., transcriptomics, metabolomics) could refine polyploid screening while mitigating off-target effects (Bharati et al., 2023b).
Equally important is the development of robust, species-specific in vitro protocols for polyploid induction. These systems are not only crucial for improving the efficiency and reproducibility of chromosome doubling but also serve as platforms for early-stage screening of induced genotypes under controlled stress conditions (e.g., drought, salinity, or pathogens). However, the creation of standardized protocols across species is inherently difficult due to the wide variability in physiological and developmental responses among woody trees.
Establishing a comprehensive, open-access protocols repository could significantly enhance progress in polyploid research for woody species. By compiling both successful and unsuccessful attempts at polyploid induction across diverse taxa, such a resource would provide valuable methodological insights that account for species-specific variability. This collective knowledge base would facilitate protocol refinement, reduce redundant experimentation, and accelerate the development of effective, adaptable methods. Moreover, it would support broader application of artificial polyploidy in hardwood species by enabling researchers to tailor approaches based on accumulated empirical evidences.
Author contributions
RB: Conceptualization, Data curation, Formal analysis, Investigation, Project administration, Software, Visualization, Writing – original draft, Writing – review & editing. LS: Formal analysis, Funding acquisition, Project administration, Supervision, Writing – review & editing.
Funding
The author(s) declare that financial support was received for the research and/or publication of this article. This work was funded by the Faculty of Economics and Management, Czech University of Life Sciences in Prague, grant number 2022B0004.
Acknowledgments
The authors acknowledge the use of the BioRender software (https://biorender.com) for generating the figures for this article. The authors would also like to thank Dr. Madhab Kumar Sen for figure licensing.
Conflict of interest
The authors declare that the research was conducted in the absence of any commercial or financial relationships that could be construed as a potential conflict of interest.
Generative AI statement
The authors declare that no Gen AI was used in the creation of this manuscript.
Publisher’s note
All claims expressed in this article are solely those of the authors and do not necessarily represent those of their affiliated organizations, or those of the publisher, the editors and the reviewers. Any product that may be evaluated in this article, or claim that may be made by its manufacturer, is not guaranteed or endorsed by the publisher.
References
Bharati, R., Gupta, A., Novy, P., Severová, L., Šrédl, K., Žiarovská, J., et al. (2023a). Synthetic polyploid induction influences morphological, physiological, and photosynthetic characteristics in Melissa officinalis L. Front. Plant Sci. 14:1332428. doi: 10.3389/fpls.2023.1332428
Bharati, R., Sen, M. K., Severová, L., Svoboda, R., and Cusimamani, E. F. (2023b). Polyploidization and genomic selection integration for grapevine breeding: a perspective. Front. Plant Sci. 14:1248978. doi: 10.3389/fpls.2023.1248978
Bharati, R., Shmeit, Y. H., Šedivá, J. H., Cong, T. T. N., Kundu, J. K., Severová, L., et al. (2024). Comparative assessment of morphological, cytological, and photosynthetic characteristics of the induced octoploid and its tetraploid counterpart of Celosia argentea L. BMC Plant Biol. 24:1227. doi: 10.1186/s12870-024-05973-x
Blasco, M., Badenes, M. L., and Naval, M. D. M. (2015). Colchicine-induced polyploidy in loquat (Eriobotrya japonica (Thunb.) Lindl.). Plant Cell Tissue Organ Cult. 120, 453–461. doi: 10.1007/s11240-014-0612-3
Cai, X., and Kang, X.-Y. (2011). In vitro tetraploid induction from leaf explants of Populus pseudo-simonii Kitag. Plant Cell Rep. 30, 1771–1778. doi: 10.1007/s00299-011-1085-z
Chen, T., Sheng, Y., Hao, Z., Long, X., Fu, F., Liu, Y., et al. (2021). Transcriptome and proteome analysis suggest enhanced photosynthesis in tetraploid Liriodendron sino-americanum. Tree Physiol. 41, 1953–1971. doi: 10.1093/treephys/tpab039
Da Silva Souza, T., Daolio, M. F., Mori, F. A., Ramalho, M. A. P., Mingossi, F. B., Missiaggia, A. A., et al. (2021). Polyploidy as a strategy to improve the industrial quality of eucalypt wood. Wood Sci. Technol. 55, 181–193. doi: 10.1007/s00226-020-01236-8
Dhooghe, E., Van Laere, K., Eeckhaut, T., Leus, L., and Van Huylenbroeck, J. (2011). Mitotic chromosome doubling of plant tissues in vitro. Plant Cell Tissue Organ Cult. 104, 359–373. doi: 10.1007/s11240-010-9786-5
Eng, W. H., Ho, W. S., and Ling, K. H. (2021). In vitro induction and identification of polyploid Neolamarckia cadamba plants by colchicine treatment. PeerJ 9:e12399. doi: 10.7717/peerj.12399
Fernando, S. C., Goodger, J. Q. D., Chew, B. L., Cohen, T. J., and Woodrow, I. E. (2019). Induction and characterisation of tetraploidy in Eucalyptus polybractea R.T. Baker. Ind. Crops Prod. 140:111633. doi: 10.1016/j.indcrop.2019.111633
Grassi, G., House, J., Dentener, F., Federici, S., den Elzen, M., and Penman, J. (2017). The key role of forests in meeting climate targets requires science for credible mitigation. Nat. Clim. Chang. 7, 220–226. doi: 10.1038/nclimate3227
Guo, L., Xu, W., Zhang, Y., Zhang, J., and Wei, Z. (2017). Inducing triploids and tetraploids with high temperatures in Populus sect. Tacamahaca. Plant Cell Rep. 36, 313–326. doi: 10.1007/s00299-016-2081-0
Harbard, J. L., Griffin, A. R., Foster, S., Brooker, C., Kha, L. D., and Koutoulis, A. (2012). Production of colchicine-induced autotetraploids as a basis for sterility breeding in Acacia mangium Willd. Forestry 85, 427–436. doi: 10.1093/forestry/cps041
Herben, T., Suda, J., and Klimešová, J. (2017). Polyploid species rely on vegetative reproduction more than diploids: a re-examination of the old hypothesis. Ann. Bot. 120, 341–349. doi: 10.1093/aob/mcx0009
Heslop-Harrison, J. S., Schwarzacher, T., and Liu, Q. (2023). Polyploidy: its consequences and enabling role in plant diversification and evolution. Ann. Bot. 131, 1–10. doi: 10.1093/aob/mcac132
Kang, X., and Wei, H. (2022). Breeding polyploid Populus: progress and perspective. For. Res. 2:4. doi: 10.48130/FR-2022-0004
Kebebew, Z., and Ozanne, C. (2024). Woody plants diversity and the associated provisioning ecosystem services across three contrasting forest management regimes in Southwest Ethiopia. Front. Conserv. Sci. 5:1382843. doi: 10.3389/fcosc.2024.1382843
Lam, H., Harbard, J., and Koutoulis, A. (2014). Tetraploid induction of Acacia crassicarpa using colchicine and oryzalin. J. Trop. For. Sci. 26, 347–354.
Lin, J., Zhang, B., Zou, J., Luo, Z., Yang, H., Zhou, P., et al. (2023). Induction of tetraploids in paper mulberry (Broussonetia papyrifera (L.) L'Hér. Ex vent.) by colchicine. BMC Plant Biol. 23:574. doi: 10.1186/s12870-023-04487-2
Liu, X., Trogisch, S., He, J.-S., Niklaus, P. A., Bruelheide, H., Tang, Z., et al. (2018). Tree species richness increases ecosystem carbon storage in subtropical forests. Proc. R. Soc. B 285:20181240. doi: 10.1098/rspb.2018.1240
Liu, Z., Wang, J., Qiu, B., Ma, Z., Lu, T., Kang, X., et al. (2022). Induction and characterization of tetraploid through zygotic chromosome doubling in Eucalyptus urophylla. Front. Plant Sci. 13:870698. doi: 10.3389/fpls.2022.870698
Longui, E. L., Custódio, G. H., Amorim, E. P., Silva Júnior, F. G. D., Oda, S., and Souza, I. C. G. (2021). Differences in wood properties among Eucalyptus grandis and Eucalyptus grandis x Eucalyptus urophylla with different degrees of ploidy. RSD 10:e395101624035. doi: 10.33448/rsd-v10i16.24035
Lu, M., Zhang, P., Wang, J., Kang, X., Wu, J., Wang, X., et al. (2014). Induction of tetraploidy using high temperature exposure during the first zygote division in Populus adenopoda maxim. Plant Growth Regul. 72, 279–287. doi: 10.1007/s10725-013-9859-7
Madlung, A. (2013). Polyploidy and its effect on evolutionary success: old questions revisited with new tools. Heredity 110, 99–104. doi: 10.1038/hdy.2012.79
Martínez-Fortún, J., Phillips, D. W., and Jones, H. D. (2022). Natural and artificial sources of genetic variation used in crop breeding: a baseline comparator for genome editing. Front. Genome Ed. 4:937853. doi: 10.3389/fgeed.2022.937853
Meng, F., Pang, H., Huang, F., Liu, L., and Wang, Y. (2012). Tetraploid black locust (Robinia pseudoacacia L.) increased salt tolerance by activation of the antioxidant system. Biotechnol. Biotechnol. Equip. 26, 3351–3358. doi: 10.5504/BBEQ.2012.0110
Mu, H.-Z., Liu, Z.-J., Lin, L., Li, H.-Y., Jiang, J., and Liu, G.-F. (2012). Transcriptomic analysis of phenotypic changes in birch (Betula platyphylla) autotetraploids. Int. J. Mol. Sci. 13, 13012–13029. doi: 10.3390/ijms131013012
Niazian, M., and Nalousi, A. M. (2020). Artificial polyploidy induction for improvement of ornamental and medicinal plants. Plant Cell Tissue Organ Cult. 142, 447–469. doi: 10.1007/s11240-020-01888-1
Ren, Y., Jing, Y., and Kang, X. (2021). In vitro induction of tetraploid and resulting trait variation in Populus alba × Populus glandulosa clone 84 K. Plant Cell Tissue Organ Cult. 146, 285–296. doi: 10.1007/s11240-021-02068-5
Riastiwi, I., Witjaksono, W., Juniarti Siregar, U., and Ratnadewi, D. (2024). Induction of polyploidy in Dalbergia latifolia Roxb. Using Oryzalin. J. Sains Malays. 53, 1889–1900. doi: 10.17576/jsm-2024-5308-13
Sabooni, N., and Gharaghani, A. (2022). Induced polyploidy deeply influences reproductive life cycles, related phytochemical features, and phytohormonal activities in blackberry species. Front. Plant Sci. 13:938284. doi: 10.3389/fpls.2022.938284
Salvatori, E. S., Morgan, L. V., Ferrarini, S., Zilli, G. A. L., Rosina, A., Almeida, M. O. P., et al. (2023). Anti-inflammatory and antimicrobial effects of Eucalyptus spp. essential oils: a potential valuable use for an industry byproduct. Evid. Based Complement. Alternat. Med. 2023:2582698. doi: 10.1155/2023/2582698
Silva, A. J., Carvalho, C. R., and Clarindo, W. R. (2019). Chromosome set doubling and ploidy stability in synthetic auto- and allotetraploid of Eucalyptus: from in vitro condition to the field. Plant Cell Tissue Organ Cult. 138, 387–394. doi: 10.1007/s11240-019-01627-1
Suliman, S., and Asander, S. (2020). Polyploidy induced by colchicine in Robinia pseudoacacia L. and its effects on morphological, physiological and anatomical seedling traits. Iraqi J. Agric. Sci. 51, 829–847. doi: 10.36103/ijas.v51i3.1038
Tang, Z.-Q., Chen, D.-L., Song, Z.-J., He, Y.-C., and Cai, D.-T. (2010). In vitro induction and identification of tetraploid plants of Paulownia tomentosa. Plant Cell Tissue Organ Cult. 102, 213–220. doi: 10.1007/s11240-010-9724-6
Tossi, V. E., Martínez Tosar, L. J., Laino, L. E., Iannicelli, J., Regalado, J. J., Escandón, A. S., et al. (2022). Impact of polyploidy on plant tolerance to abiotic and biotic stresses. Front. Plant Sci. 13:869423. doi: 10.3389/fpls.2022.869423
Touchell, D. H., Palmer, I. E., and Ranney, T. G. (2020). In vitro ploidy manipulation for crop improvement. Front. Plant Sci. 11:722. doi: 10.3389/fpls.2020.00722
Wang, Y., Jia, B., Ren, H., and Feng, Z. (2021). Ploidy level enhances the photosynthetic capacity of a tetraploid variety of Acer buergerianum Miq. PeerJ 9:e12620. doi: 10.7717/peerj.12620
Wang, X., Lu, M., Liu, Y., Li, S., Yu, Y., Chen, S., et al. (2025). Generation and characterization of a tetraploid Populus davidiana. Plant Cell Tissue Organ Cult. 160:17. doi: 10.1007/s11240-024-02953-9
Wen, Y., Liu, H., Meng, H., Qiao, L., Zhang, G., and Cheng, Z. (2022). In vitro induction and phenotypic variations of autotetraploid garlic (Allium sativum L.) with dwarfism. Front. Plant Sci. 13:917910. doi: 10.3389/fpls.2022.917910
Windarsih, G., Handayani, T., Riastiwi, I., Leksonowati, A., Praptosuwiryo, T. N., Ahmad, F., et al. (2024). Induction and characterization of induced tetraploid, mixoploid and control diploid teak seedlings (Tectona grandis L.f.) from shoot cultures treated with oryzalin. For. Sci. Technol. 20, 142–154. doi: 10.1080/21580103.2024.2323721
Winter, G. (2024). The European Union's deregulation of plants obtained from new genomic techniques: a critique and an alternative option. Environ. Sci. Eur. 36:47. doi: 10.1186/s12302-024-00867-z
Wu, J., Cheng, X., Kong, B., Zhou, Q., Sang, Y., and Zhang, P. (2022). In vitro octaploid induction of Populus hopeiensis with colchicine. BMC Plant Biol. 22:176. doi: 10.1186/s12870-022-03571-3
Wu, J.-H., Ferguson, A. R., Murray, B. G., Jia, Y., Datson, P. M., and Zhang, J. (2012). Induced polyploidy dramatically increases the size and alters the shape of fruit in Actinidia chinensis. Ann. Bot. 109, 169–179. doi: 10.1093/aob/mcr256
Wu, J., Zhou, Q., Sang, Y., Zhao, Y., Kong, B., Li, L., et al. (2023). In vitro induction of tetraploidy and its effects on phenotypic variations in Populus hopeiensis. BMC Plant Biol. 23:557. doi: 10.1186/s12870-023-04578-0
Xu, C., Zhang, Y., Huang, Z., Yao, P., Li, Y., and Kang, X. (2018). Impact of the leaf cut callus development stages of Populus on the tetraploid production rate by colchicine treatment. J. Plant Growth Regul. 37, 635–644. doi: 10.1007/s00344-017-9763-x
Yan, X., Zhang, J., and Zhang, H. (2021). Induction and characterization of tetraploids in poplar. Plant Cell Tissue Organ Cult. 146, 185–189. doi: 10.1007/s11240-021-02043-0
Yang, N., Rong, E., Li, Q., Dong, J., Du, T., Zhao, X., et al. (2015). Tetraploid induction and identification of Gossypium arboreum. Agric. Sci. 6, 436–444. doi: 10.4236/as.2015.64043
Yang, J., Wang, J., Liu, Z., Xiong, T., Lan, J., Han, Q., et al. (2018). Megaspore chromosome doubling in Eucalyptus urophylla S.T. Blake induced by colchicine treatment to produce triploids. Forests 9:728. doi: 10.3390/f9110728
Zeng, Q., Liu, Z., Du, K., and Kang, X. (2019). Oryzalin-induced chromosome doubling in triploid Populus and its effect on plant morphology and anatomy. Plant Cell Tissue Organ Cult. 138, 571–581. doi: 10.1007/s11240-019-01654-y
Keywords: anti-mitotic agents, chromosome doubling, in vitro , polyploid induction, tree breeding, tree improvement, woody species
Citation: Bharati R and Severová L (2025) Artificial polyploidy as a tool for improving growth and stress resilience in tree species. Front. For. Glob. Change. 8:1569384. doi: 10.3389/ffgc.2025.1569384
Edited by:
Carmen Arena, University of Naples Federico II, ItalyReviewed by:
Diah Ratnadewi, IPB University, IndonesiaCopyright © 2025 Bharati and Severová. This is an open-access article distributed under the terms of the Creative Commons Attribution License (CC BY). The use, distribution or reproduction in other forums is permitted, provided the original author(s) and the copyright owner(s) are credited and that the original publication in this journal is cited, in accordance with accepted academic practice. No use, distribution or reproduction is permitted which does not comply with these terms.
*Correspondence: Rohit Bharati, YmhhcmF0aUBmdHouY3p1LmN6