- 1Division of Pathology, IEO, European Institute of Oncology IRCCS, Milan, Italy
- 2Department of Oncology and Hemato-Oncology, University of Milan, Milan, Italy
- 3Department of Public Health, University Federico II, Naples, Italy
- 4Department of Pathology, Padua University Hospital, Padua, Italy
- 5Department of Medicine (DIMED), University of Padua, Padua, Italy
- 6Division of Pathology, Candiolo Cancer Institute FPO-IRCCS, Candiolo, Italy
- 7Department of Medical Sciences, University of Turin, Turin, Italy
- 8Division of Pathology and Cytopathology, Regina Elena National Cancer Institute IRCCS, Rome, Italy
- 9Department of Pathology, IRCCS Ospedale Policlinico San Martino, Genova, Italy
- 10Division of Early Drug Development for Innovative Therapies, IEO, European Institute of Oncology IRCCS, Milan, Italy
- 11Experimental Oncology and Hematology Center, A.O.U. Policlinico “G. Rodolico - S. Marco”, Catania, Italy
- 12Department of Clinical and Experimental Medicine, University of Catania, Catania, Italy
- 13Department of Emergency and Organ Transplantation, University of Bari Aldo Moro, Bari, Italy
Despite the significant achievements in the diagnosis and treatment of metastatic breast cancer (MBC), this condition remains substantially an incurable disease. In recent years, several clinical studies have aimed to identify novel molecular targets, therapeutic strategies, and predictive biomarkers to improve the outcome of women with MBC. Overall, ~40% of hormone receptor (HR)+/HER2− MBC cases harbor alterations affecting the (PI3K)/Akt/mammalian target of rapamycin (mTOR) pathway. This pathway is a major target in oncogenesis, as it regulates growth, proliferation, cell survival, and angiogenesis. Lately, the pharmacologic targeting of PIK3CA in HR+/HER2− MBC has shown significant benefits after the occurrence of endocrine therapy resistance. The orally available α-selective PIK3CA inhibitor, alpelisib, has been approved in this setting. To perform an optimal patients' selection for this drug, it is crucial to adopt a tailored methodology. Clinically relevant PIK3CA alterations may be detected in several biospecimens (e.g. tissue samples and liquid biopsy) using different techniques (e.g. real-time PCR and next-generation sequencing). In this study, we provide an overview of the role of PIK3CA in breast cancer and of the characterization of its mutational status for appropriate clinical management.
Background
Clinical Scenario
Breast cancer is the most common form of tumor and is the leading cause of cancer-related deaths in the female population worldwide, with a continuous rise in numbers (1). Despite advancements in the treatment of these patients, metastatic breast cancer (MBC) substantially remains an incurable disease (2). Difficulties related to the clinical management of these patients include prolongation of overall survival (OS), control of increasingly severe symptoms, and preservation of the health-related quality of life (3, 4). The currently available therapeutic options for MBC are chemotherapy, endocrine therapy (ET), targeted therapies, and immunotherapy (5). Regrettably, the median OS of MBC has steadily been ~3 years, with a 5-year survival rate of <26% (6).
Two-thirds of breast cancer cases express hormone receptors (HR) and lack HER2 overexpression and/or amplification (7, 8). For these patients, ET is the foremost medical treatment (9). The combination of ET with cyclin-dependent kinases (CDK)4/6 inhibitors (i.e., abemaciclib, palbociclib, and ribociclib) demonstrated significant survival benefits and is now considered the gold standard for HR+/HER2− MBC (10, 11). A significant percentage of patients, however, eventually develop ET resistance due to several mechanisms, including the dysregulation of phosphoinositide 3 kinase (PI3K)/Akt/mammalian target of rapamycin (mTOR) signaling (12). Approximately 40% of HR+/HER2− MBC cases show hyperactivation of this pathway, which has now become a therapeutic target for the treatment of breast cancer (13).
Targeting the PI3K Pathway in HR+/HER2– Advanced Breast Cancer
In the past decade, several translational research studies and clinical trials aimed to define the efficacy of targeting the PI3K pathway in breast cancer. However, the development of PI3K inhibitors has been historically troubled by toxicity, suboptimal activity, and the lack of reliable diagnostic strategies for an accurate selection of candidate patients (14). In the BOLERO-2 landmark study, the combination of ET with the mTOR inhibitor, everolimus, has been evaluated in postmenopausal women with ET-refractory HR+/HER2− MBC (15). In phase III clinical trial, 724 patients who have progressed after ET with aromatase inhibitors (AIs) were randomized to receive either exemestane plus everolimus or placebo plus exemestane. The study met its primary endpoint by showing a significant progression-free survival (PFS) benefit for the everolimus arm (HR 0.43; 95% CI: 0.35–0.54; p < 0.001), with an assessment from a local investigator. The blinded independent central review revealed a median PFS of 10.6 months in the experimental arm against a median PFS of 4.1 months in the control arm (HR 0.36; 95% CI: 0.27–0.47; p < 0.001). The OS was similar in both groups (median of 31 months in the everolimus group vs. 26.6 months in the placebo group, HR 0.89; 95% CI: 0.73–1.10; p = 0.14) (16). Given the substantial benefits observed, everolimus is now approved for use in HR+/HER2− MBC upon progression after AI therapy; however, no predictive biomarkers are available to date.
Buparlisib is a pan-PI3K inhibitor that has been evaluated in the BELLE-2 study as monotherapy or has been used in combination with ET and/or anti-HER2 therapy or cytotoxics in PIK3CA-mutant breast cancer cases (17). Despite the very low activity/toxicity ratio of this combination for the integration of buparlisib in clinical practice, the results of the BELLE-2 trial suggested that PI3K inhibition along with ET might provide clinically meaningful benefits to postmenopausal women with ET-resistant, HR+/HER2− MBC harboring PIK3CA mutations (18). Thereafter, targeting the PI3K/Akt/mTOR axis with more specific inhibitors has become the subject of great scientific efforts (19).
The orally available α-selective PIK3CA inhibitor, alpelisib, was the first PI3Kα inhibitor to demonstrate improvement in PFS in HR+/HER2− MBC cases with activating PIK3CA mutations (20). The SOLAR-1 trial included patients who were resistant to ET, with disease recurrence/progression on or after prior AI therapy (21). That study had two cohorts based on the PIK3CA mutational status. In each cohort, patients were randomized to receive alpelisib plus fulvestrant or placebo plus fulvestrant at the ratio of 1:1. The primary endpoint of the trial was PFS in the PIK3CA-mutated cohort. With a median follow-up of 20 months, the median PFS for the PIK3CA-mutated cohort was almost double with the addition of alpelisib (11.0 vs. 5.7 months, HR 0.65; 95% CI: 0.50–0.85; p < 0.001). Significant benefits were also demonstrated in terms of the overall response rate (26.6% [95% CI: 20.1–34.0] vs. 12.8% [95% CI: 8.2–18.7]) and the clinical benefit rate (61.5% [95% CI: 53.8–68.9] vs. 45.3% [95% CI: 37.8–53.1]). The OS in the PIK3CA-mutant cohort was a key secondary endpoint. In the final OS analysis for the PIK3CA-mutated cohort, OS did not cross the pre-specified O'Brien-Fleming efficacy boundary (one-sided p ≤ 0.0161), although median OS was prolonged by a clinically relevant figure of 7.9 months for patients in the alpelisib plus fulvestrant arm; no benefit was seen in the PIK3CA non-mutant cohort (22). Additionally, α-selective mutant-degrading PI3K inhibitors (e.g., GDC-0077) are currently under investigation in breast cancer (NCT03006172, NCT04191499).
Biology and Actionability of PIK3CA Mutations
PI3K/Akt/mTOR Pathway Signaling in Breast Cancer
Phosphatidylinositol-3 kinases are heterodimeric lipid kinases characterized by regulatory (p85) and catalytic (p110) subunits, with the former subunit inhibiting the latter in quiescent cells (23). PI3Ks are involved in several cellular processes, such as protein synthesis, cell proliferation and survival, glucose homeostasis, and DNA repair (24, 25). The interaction of the catalytic subunit with the phosphotyrosine residues of activated growth factor receptors or adaptor proteins (e.g., RAS proteins) is critical to elicit PI3K activation (26). As a consequence, the membrane lipid phosphatidylinositol-4,5-bisphosphate (PIP2) is converted to phosphatidylinositol-3,4,5-trisphosphate (PIP3) (27). PIP3 directly activates Akt and other proteins harboring the PIP3-binding pleckstrin-homology (PH) domains (12, 28). Upon complete activation, Akt induces multiple downstream cytosolic and nuclear effectors. This mechanism, considered as the “core” of cell survival and cell cycle progression, is turned off by several phosphatases (PTEN, TSC1, TSC2, and LKB1) that dephosphorylate mTORC1 and PIP3 (29–31) (Figure 1).
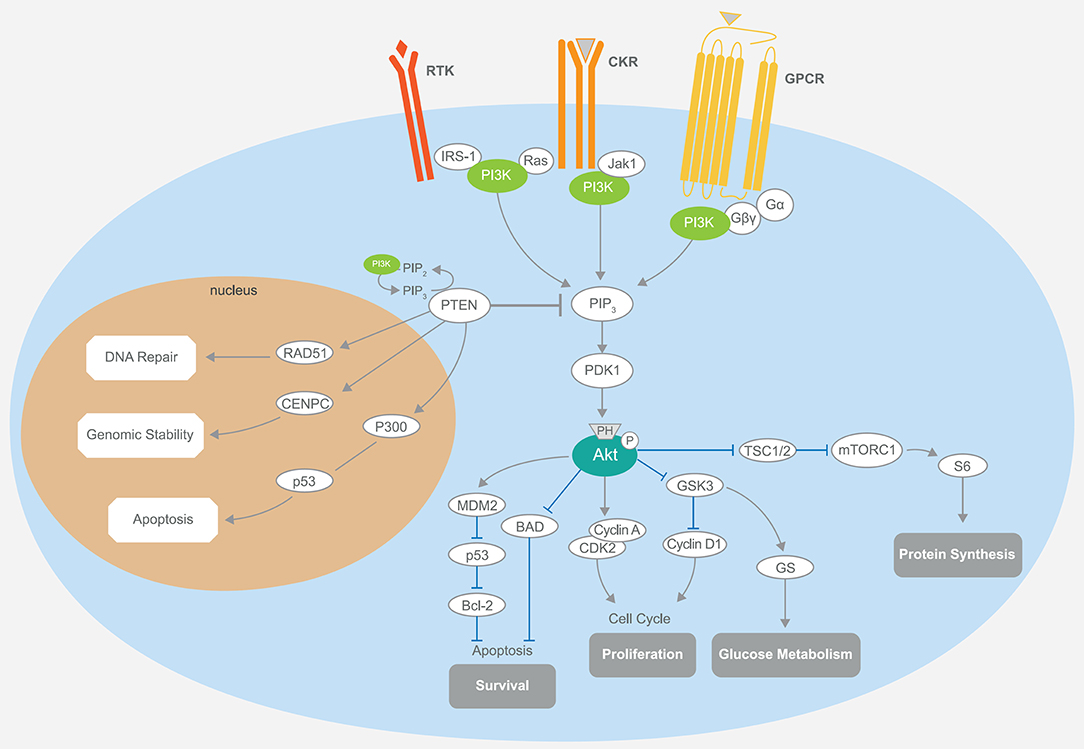
Figure 1. Schematic representation of the PI3K/Akt/mTOR signaling and its main components. The PI3K pathway regulates diverse cellular processes, including protein synthesis, cell survival, proliferation, glucose metabolism, apoptosis, DNA repair, and genome stability. Akt-mediated phosphorylation inhibits the activity of the TSC1–TSC2 complex, also known as hamartin-tuberin. This is a critical step for the negative regulation of mTORC1, whose activity controls anabolic processes. Another important downregulation of Akt phosphorylation is toward BAD, while MDM2 activity is enhanced, promoting the degradation of the tumor-suppressor p53, which also plays a part in the P300-mediated cell apoptosis. Cell cycle regulation occurs through the stimulation of cyclins A and D and the inhibition of GSK3. The latter event is also responsible for increased glucose metabolism. PTEN is intimately involved in the regulation of these mechanisms through its substrate PIP3. Notably, the activity of PTEN in the cell nucleus that leads to cell survival control is related to the upregulation of key mediators, such as RAD51, CDNPC, and P300. RTK, receptor tyrosine kinase; CKR, chemokine receptor; GPCR, G protein-coupled receptor; IRS-1, insulin receptor substrate 1; PI3K, phosphatidylinositol-3 kinase; JAK1, Janus kinase 1; PIP3, phosphatidylinositol-3,4,5-trisphosphate; PDK1, pyruvate dehydrogenase lipoamide kinase isozyme 1; TSC, tuberous sclerosis complex; mTORC1, mammalian target of rapamycin complex 1; MDM2, mouse double minute 2 homolog; BAD, BCL2 associated agonist of cell death; GSK3, glycogen synthase kinase-3; CDK2, cyclin-dependent kinase 2; CDNPC, centromere protein C.
Three classes of PI3Ks enzyme isoforms can be distinguished based on their coding genes, chemical structures, and substrate specificity/preferences (23). Mutations involving class IA genes, namely the PIK3CA alpha isoform which encodes p110α, are frequently associated with cancer development, progression, and drug resistance in many types of solid tumors, including HR+/HER2− breast cancer (32–36). Thus, in May 2019, the U.S. Food and Drug Administration (FDA) approved a α-selective PIK3CA inhibitor, alpelisib (BYL719; Novartis Pharma AG), in combination with fulvestrant for the treatment of postmenopausal patients diagnosed with HR+/HER2− PIK3CA-mutated, advanced breast cancer or MBC, following the progression on or after an endocrine-based regimen (37, 38). Several other compounds with selective activity against different PI3K isoforms (α, β, γ, and δ) or acting as pan-PI3K inhibitors (on all class I isoforms) have also been investigated. However, their clinical value in terms of effectiveness and toxicity profile remains controversial (39). Of note are the mutations in the PI3K regulatory subunit 1 (PIK3R1), which inhibits the α subunit, that can be observed in ~3% of patients with breast cancer (39, 40).
Different aberrations in the PI3K signaling pathway, such as PI3K mutation/amplification, loss/mutation of the phosphatase and tensin homolog, Akt overexpression/overactivation, and modulation of tuberous sclerosis protein 1 and 2 tumor suppressors, can be often observed in HR+ breast cancers. Different PIK3CA mutations affecting multiple domains of the protein have been shown in Figure 2 and Supplementary Table 1. In patients with breast cancer, the most common alterations clustered in the helical (exon 9 p. E545K and p.E542K) or the kinase (exon 20 p.H1047R) domains (41). Martínez-Sáez et al. confirmed the clustering, reporting that the most common PIK3CA alterations (69% of the total identified) affect exon 20 (p.H1047R in 35% and p.H1047L in 4% of patients) and exon 9 (p.E545K in 17% and p.E542K in 11% of patients) (42). All these alterations respond to alpelisib (20, 38, 39, 42). The fifth most frequently identified alteration was in exon 4 (p.N345K). Despite its likely pathogenicity, as reported in the COSMIC and OncoKB databases (43, 44), only pre-clinical studies have so far demonstrated the sensitivity of this mutation to PI3K inhibitors (45, 46). Another relevant PIK3CA alteration is the p.E726K substitution in exon 13, accounting for about 2.5% of mutated cases (42). Despite a low oncogenic activity per se, this mutation is frequently associated with other pathogenic PIK3CA sequence alterations, amplifying PI3K activity (47). Notably, although rare (0.7% of the analyzed samples), the PIK3CA exon 20 p.G1049R mutation is likely pathogenic, and pre-clinical models showed that this mutation, similar to p.E542K, leads to an increased alpelisib sensitivity (46). Similar sensitivity results, in pre-clinical studies, have been obtained for the exon 9 p.Q546K (0.8%) alteration (48). Finally, among other less frequent PIK3CA mutations, exon 7 p.C420R (1.9%), exon 9 p.Q546R (1.1%), p.E545A (0.5%), and p.E545G (0.5%) demonstrated responsiveness to alpelisib (21). It is worth mentioning that there is recent evidence to demonstrate that double cis-regulatory PIK3CA mutations are related to increased activation of the PI3K signaling compared to single mutants (47). One of the possible biological mechanisms underpinning this hyperactivation could be due to increased membrane binding and p85α disinhibition. Interestingly, tumors showing multiple PIK3CA mutations are markedly sensitive to PI3Kα inhibitors (47).
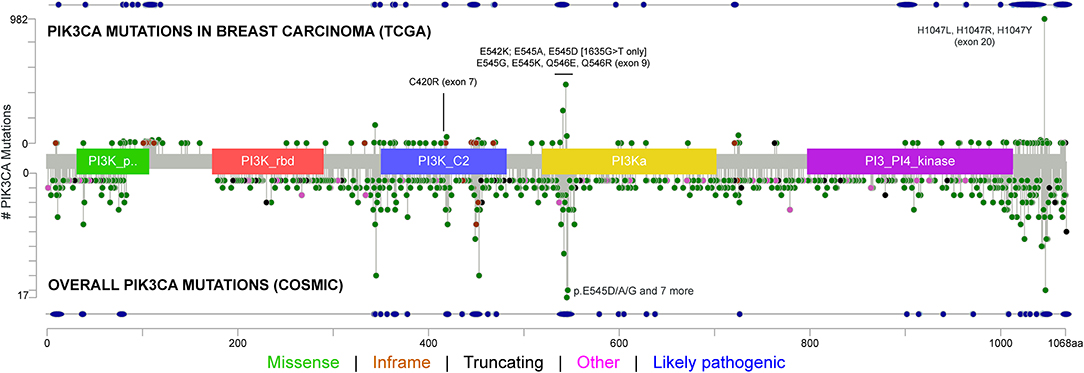
Figure 2. Type of mutations, frequency, and affected PIK3CA domains across breast cancers from The Cancer Genome Atlas (TCGA) Network and selected solid tumors from the Catalog Of Somatic Mutations In Cancer (COSMIC) datasets, including the mutations and exons covered by the FDA-approved the RT-PCR test. The tumor types included in this analysis are non-small cell lung cancer (i.e., squamous cell and adenocarcinoma), esophageal cancer, stomach cancer, colorectal cancer, cholangiocarcinoma, pancreatic cancer, liver cancer, bladder cancer, prostate adenocarcinoma, uterine cancer (i.e., endometrioid, serous, and carcinosarcoma), ovarian cancer, and invasive breast cancer. The types of mutations and their likely pathogenicity are color-coded based on the legend at the bottom.
Molecular Biology Techniques to Detect PIK3CA Mutations
Direct Sequencing
Direct or Sanger sequencing is the gold standard for the mutational assessment of different biomarkers due to its reliability, availability, reagent affordability, and relatively low costs. Despite these advantages, low sensitivity remains its main limitation (49). Arsenic and colleagues compared the results obtained by Sanger sequencing and next-generation sequencing (NGS) for PIK3CA hotspot mutations in exons 9 and 20 in 184 breast cancer samples, reporting a concordance rate of 98.4% (n = 52 mutated cases) (50). The three mutated tumors identified by NGS and missed by Sanger sequencing displayed a low (i.e., < 10%) allelic frequency. In another study, Sanger sequencing showed a lower performance rate in the detection of PIK3CA-mutated cases compared to multiplex PCR-mass spectroscopy (concordance rate 69%, 35/51 detected cases) and locked nucleic acid (LNA)-PCR (concordance rate 64%, 36/55 detected cases) (51).
Real-Time PCR
Real-time PCR (RT-PCR) is widely used to identify mutations in known genomic regions (52). Alvarez-Garcia et al. (53) proposed a simple, fast, and inexpensive diagnostic tool to determine the PIK3CA mutational status in patients with breast cancer based on the standard SYBR Green RT-PCR approach. Their findings support the use of RT-PCR to detect PIK3CA exon 20 p.H1047R and exon 9 p.E545K hotspot mutations with sensitivities of 5 and 10%, respectively. In the study performing 46 formalin-fixed and paraffin-embedded breast carcinoma specimens, Lambert et al. (54) compared three different RT-PCR approaches, namely the cobas® PIK3CA Mutation Test (Roche Diagnostics, Meylan, France), the PCR amplification-refractory mutation system (ARMS) Scorpion, and the High-Resolution Melting (HRM) PCR assay. Overall, 38% (n = 17), 28% (n = 13), and 41% (n = 19) of the tested samples showed a PIK3CA mutation with cobas®, ARMS, and HRM assays, respectively. The highest concordance rate was observed between cobas® and HRM [k = 0.95 (0.86; 1)], followed by cobas® and ARMS [k = 0.75 (0.55; 0.95)] and HRM and ARMS [k = 0.72 (0.51; 0.92)] (54). In a retrospective series, Harlè et al. (55) analyzed 102 breast cancer samples using both HRM and ARMS-PCR for PIK3CA exon 9 and exon 20 mutations. Taken together, 27.5 and 22.5% of PIK3CA substitutions were reported with PCR-HRM and PCR-ARMS, respectively. Based on these results, we proposed a combined approach with PCR-HRM and PCR-ARMS to better cope with the cost of routine PIK3CA mutation identification for invasive breast cancer. In line with these findings, a PIK3CA mutation rate of 33.4% has been reported by Cizkova et al. (56) using RT-PCR for exons 9 and 20. In a large, unselected cohort of 1,281 female patients with HR+ MBC, Chan et al. (57) adopted the FDA-cleared RT-PCR test (therascreen® PIK3CA RGQ PCR Kit [QIAGEN GmbH, Hilden, Germany]) that covers eleven mutations (exon 7 p.C420R; exon 9 p.E542K, p.E545A, p.E545D, p.E545G, p.E545K, p.Q546E, p.Q546R; and exon 20 p.H1047L, p.H1047R, p.H1047Y) in the PIK3CA gene, reporting a 37.5% mutation rate. The same technical approach was also employed in a large analysis by Martínez-Sáez et al. (42) that correctly detected the majority (72%) of the PIK3CA mutations of the analyzed samples.
Next-Generation Sequencing
The massively parallel sequencing technology, also referred to as NGS, has revolutionized the clinical management of patients with cancer (58). Despite different platforms being commercially available, the NGS workflow follows the same four sequential phases (i.e., library generation, clonal amplification, massively parallel sequencing, and data analysis) (59). Hempel et al. (13) adopted a broad NGS panel to analyze 41 MBC samples and detected PIK3CA mutations in 34% (n = 14) of them. In another study including patients with ET-resistant (n = 15) and ET-sensitive (n = 9) breast cancer cases, the same authors were able to identify PIK3CA alterations in 55% (n = 8) and 33% (n = 3) of the cases, respectively (60). Specifically, ET-resistant tumors displayed three pathogenic variants in the kinase domain, three pathogenic variants in the helical domain, and two variants of unknown significance, whereas ET-sensitive tumors presented two pathogenic variants in the kinase domain and one pathogenic variant in the helical domain. In a recent SAFIR02 study, 22% of the total population and 28% of patients with HR+ breast cancer featured a PIK3CA mutation (61). Using a broad NGS panel targeting 1,021 genes on 193 MBC samples, Tang et al. (62) detected 36 (18.7%) mutations in the kinase domain and 26 (13.5%) substitutions in the helical domain with 10 (5.2%) additional alterations distributed in the remaining PIK3CA sequence. One of the main advantages of NGS is that it allows for the identification of multiple mutations simultaneously, avoiding the need to perform sequential individual tests.
Analysis of Liquid Biopsy Samples
Liquid biopsy using blood components to assess PIK3CA mutations in circulating tumor DNA (ctDNA) of patients with breast cancer has been reported by different studies (39). Multiple technologies have been employed to assess breast cancer ctDNA with high sensitivity and specificity, leading to assays that have been useful in clinical trials and are entering clinical practice (63). Using an ARMS allele-specific PCR and Scorpion probes, Board et al. (64) were able to detect PIK3CA mutations in the vast majority (80%) of ctDNA samples from PIK3CA-mutated MBC but not in early breast cancer. Digital droplet PCR has been proposed as a more sensitive approach in the non-metastatic setting (65). The secondary endpoint in the SOLAR-1 study was to assess PFS according to the level of ctDNA (21). This trial confirmed that treatment with alpelisib and fulvestrant provided an extension of PFS for patients with PIK3CA-mutated disease based on ctDNA analysis. In particular, there was a 45% reduction of risk in PFS for patients with ctDNA PIK3CA mutations (HR 0.55; 95% CI: 0.39–0.79; n = 186) and a 20% reduction of risk in PFS for those without the mutations (HR 0.80; 95% CI: 0.60–1.06; n = 363). The increased magnitude of the PFS benefit for the alpelisib plus fulvestrant arm compared to the placebo plus fulvestrant arm was maintained at subgroup analyses, further highlighting the clinical value of liquid biopsy (66). Comparative analysis of PIK3CA mutations from tissue and plasma obtained from three phase III clinical trials, each utilizing different testing methods, showed concordance rates of 70–83%, while in the BOLERO2 study, a higher concordance was observed for metastatic lesions (82%). PIK3CA mutations were more frequently detected in tissue samples than in liquid biopsies in both studies. Circulating tumor cells (CTC) have been also investigated as a possible source of biologic materials for the detection of PIK3CA mutations. An ultra-sensitive (0.05%) combination of allele-specific, asymmetric rapid PCR and melting analysis to detect hotspot mutations (exons 9 and 20) has been applied on the CTC of patients with breast cancer (67). In the present study, we were able to detect PIK3CA mutations in 35.1% (20/57) and 19.5% (23/118) of MBC and early breast cancers, respectively. Notably, no false-positive results were reported in healthy donors. Unsurprisingly, CTCs were isolated with a higher frequency in the metastatic setting, where the presence of PIK3CA mutations correlated with a worse outcome (67). A more recent study showed a comparison between ctDNA and CTC analysis for PIK3CA molecular assessment (68). By using the same ultra-sensitive approach, they observed a superior concordance rate among the two methodologies in MBC.
Taken together, these data show that the technology of liquid biopsy for ctDNA analysis has been successfully used in patients with breast cancer to identify PIK3CA alterations without additional invasive testing. The main advantages of this procedure compared to conventional tissue biopsy are the non-invasive nature and repeatability. These allow for a timely follow-up, potentially overcoming spatial and temporal heterogeneity of tumor (63). On the other hand, a major limitation of this approach is the lower concentration of tumor-derived DNA compared to traditional tissue specimens. For this reason, careful attention should be paid to the technology chosen for liquid biopsy molecular analyses (69).
Conclusion
PIK3CA mutations are highly represented in ER+/HER2− breast cancer and are of clinical interest due to the availability of targeted therapy with alpelisib in the metastatic setting (Figure 3). From the diagnostic perspective, it is crucial to adopt a tailored methodology to cover all of the clinically relevant gene alterations in the different clinical settings (Figure 4). The versatility and efficiency of RT-PCR, coupled with its cost-effectiveness, sensitivity, and specificity, facilitate the adoption of this technology in virtually all molecular pathology laboratory settings. Furthermore, this approach provides results in ultra-fast time, as the average duration of the reaction is between 30 min and 2 h. Although RT-PCR has several advantages over direct sequencing, the technology has also intrinsic limitations, including its limited multiplexing capability. Another important limitation of RT-PCR is the lack of kits and standardized protocols to detect all possible PIK3CA alterations (Figure 2). Furthermore, the vast majority of commercially available kits, including the FDA-approved RT-PCR test for PIK3CA mutational analysis, do not provide quantitative information about mutant allele frequency. On the other hand, NGS panels allow covering several different alterations simultaneously, even starting from low input of DNA, and provide information on the fraction of alleles carrying the mutation. Although the approximate costs of this technology, which requires specialized centers with highly trained personnel, are relatively high, the optimization of the laboratory workflow and volumes allows for a favorable cost-benefit ratio. Although tissue samples remain the most suitable material to be tested, other sources of DNA (i.e., liquid biopsies) may be used to analyze clinically relevant gene alterations. The latter may be particularly valuable whenever the tissue material is not available or is inaccessible. On the other hand, clinicians should be fully aware of the indications and limitations of this type of analysis in breast cancer. It is important to remark that in case of a negative result in the ctDNA analysis, a second liquid biopsy or, if feasible, a tissue biopsy should be analyzed to avoid false-negative results. Finally, though tissue samples from initial diagnosis are a reliable source for PIK3CA testing, it is generally preferable (if clinically feasible) to run the test on a biopsy of the metastatic site, as this may best reflect the actual genomic profile of the disease.
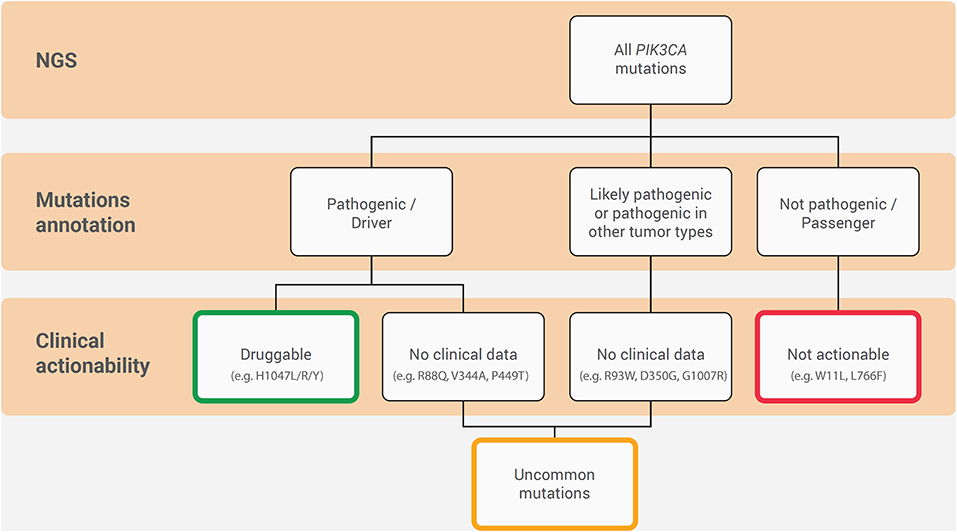
Figure 3. Hierarchy diagram portraying the annotation and clinical actionability of PIK3CA mutations in ER+/HER2− metastatic breast cancer. The connecting lines indicate the relationship between them.
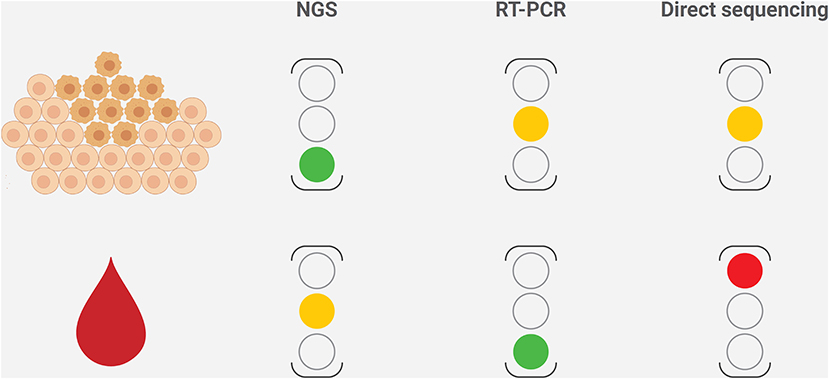
Figure 4. Strengths and limitations of the different methods to characterize PIK3CA mutational status in breast cancer, according to the sample type. The level of caution for each method is reported on the right, where the green light represents to proceed, the amber light represents warning on possible fails or false-negative results, and the red light represents discouraging the use of the technology in that setting. NGS, next-generation sequencing.
Author Contributions
GV, EM, and AD: supervision. NF and UM: manuscript writing (first draft) and iconography. MF, CM, SB, SZ, CC, and PV: first draft revision. All authors: study design, revision, and approval of the final draft.
Funding
The authors declare that this study received funding from Novartis Farma SpA. The funder was not involved in the study design, collection, analysis, interpretation of data, the writing of this article or the decision to submit it for publication.
Conflict of Interest
NF has received honoraria for consulting, advisory role, and/or speaker bureau from Merck Sharp & Dohme (MSD), Boehringer Ingelheim, and Novartis. UM received personal fees (for service in the speaker bureau and as an advisor) from Boehringer Ingelheim, AstraZeneca, Roche, MSD, Amgen, Merck, Eli Lilly, Thermofisher, and Diaceutics. MF received honoraria for consulting, advisory role, speaker bureau, and/or research funding from Astellas Pharma, QED Therapeutics, Diaceutics, Tesaro, Roche, Eli Lilly, and Novartis. CM received honoraria for consulting from Roche, Bayer, AstraZeneca, and Daiichi Sankyo. SB received honoraria for consulting/advisory role/speaker bureau from AstraZeneca, Novartis, Bayer, and Diaceutics. SZ received research funding from AstraZeneca. CC received honoraria for consulting/advisory role/speaker bureau from Novartis, Eli-Lilly, Pfizer, and Roche. PV received honoraria from AstraZeneca, BMS, Celgene, Eli-Lilly, GSK, Incyte, MSD, Novartis, Pfizer, and Teva and research funds from Novartis and Pfizer. AD received honoraria for advisory board roles from Bayer, Roche, Merck, PharmaMar, and Novartis Oncology. EM received honoraria for consulting, advisory role, and/or speaker bureau from Novartis, Roche, and Shire. GV received honoraria for consulting, advisory role, speaker bureau, travel, accommodation, expenses, and/or research funding from MSD Oncology, Pfizer, Dako, Roche/Genetech, Astellas Pharma, Novartis, Bayer, Daiichi Sankyo, Menarini, Ventana Medical Systems Dako/Agilent Technologies, Cepheid, and Celgene.
The handling editor declared a shared affiliation, though no other collaboration, with one of the authors UM.
Acknowledgments
We thank Forum Service s.r.l. for their medical editorial assistance with this manuscript.
Supplementary Material
The Supplementary Material for this article can be found online at: https://www.frontiersin.org/articles/10.3389/fonc.2021.644737/full#supplementary-material
References
1. Heer E, Harper A, Escandor N, Sung H, McCormack V, Fidler-Benaoudia MM. Global burden and trends in premenopausal and postmenopausal breast cancer: a population-based study. Lancet Glob Health. (2020) 8:e1027–37. doi: 10.1016/S2214-109X(20)30215-1
2. Thill M, Jackisch C, Janni W, Müller V, Albert US, Bauerfeind I, et al. AGO recommendations for the diagnosis and treatment of patients with locally advanced and metastatic breast cancer: update 2019. Breast Care. (2019) 14:247–55. doi: 10.1159/000500999
3. Invernizzi M, Kim J, Fusco N. Editorial: quality of life in breast cancer patients and survivors. Front Oncol. (2020) 10:620574. doi: 10.3389/fonc.2020.620574
4. Nardin S, Mora E, Varughese FM, D'Avanzo F, Vachanaram AR, Rossi V, et al. Breast cancer survivorship, quality of life, and late toxicities. Front Oncol. (2020) 10:864. doi: 10.3389/fonc.2020.00864
5. Grizzi G, Ghidini M, Botticelli A, Tomasello G, Ghidini A, Grossi F, et al. Strategies for increasing the effectiveness of aromatase inhibitors in locally advanced breast cancer: an evidence-based review on current options. Cancer Manag Res Volume. (2020) 12:675–86. doi: 10.2147/CMAR.S202965
6. Cardoso F, Spence D, Mertz S, Corneliussen-James D, Sabelko K, Gralow J, et al. Global analysis of advanced/metastatic breast cancer: decade report (2005–2015). Breast. (2018) 39:131–8. doi: 10.1016/j.breast.2018.03.002
7. Pereira B, Chin SF, Rueda OM, Vollan HK, Provenzano E, Bardwell HA, et al. The somatic mutation profiles of 2,433 breast cancers refines their genomic and transcriptomic landscapes. Nat Commun. (2016) 7:11479. doi: 10.1038/ncomms11479
8. Lopez G, Costanza J, Colleoni M, Fontana L, Ferrero S, Miozzo M, et al. Molecular insights into the classification of luminal breast cancers: the genomic heterogeneity of progesterone-negative tumors. Int J Mol Sci. (2019) 20:30510. doi: 10.3390/ijms20030510
9. Lei JT, Anurag M, Haricharan S, Gou X, Ellis MJ. Endocrine therapy resistance: new insights. Breast. (2019) 48(Suppl.1):S26–30. doi: 10.1016/S0960-9776(19)31118-X
10. Cardoso F, Paluch-Shimon S, Senkus E, Curigliano G, Aapro MS, André F, et al. 5th ESO-ESMO international consensus guidelines for advanced breast cancer (ABC 5). Ann Oncol. (2020) 31:1623–49. doi: 10.1016/j.annonc.2020.09.010
11. Piezzo M, Chiodini P, Riemma M, Cocco S, Caputo R, Cianniello D, et al. Progression-free survival and overall survival of CDK 4/6 inhibitors plus endocrine therapy in metastatic breast cancer: a systematic review and meta-analysis. Int J Mol Sci. (2020) 21:176400. doi: 10.3390/ijms21176400
12. Vasan N, Toska E, Scaltriti M. Overview of the relevance of PI3K pathway in HR-positive breast cancer. Ann Oncol. (2019) 30(Suppl.10):x3–11. doi: 10.1093/annonc/mdz281
13. Hempel D, Ebner F, Garg A, Trepotec Z, Both A, Stein W, et al. Real world data analysis of next generation sequencing and protein expression in metastatic breast cancer patients. Sci Rep. (2020) 10:10459. doi: 10.1038/s41598-020-67393-9
14. Esposito A, Viale G, Curigliano G. Safety, tolerability, and management of toxic effects of phosphatidylinositol 3-kinase inhibitor treatment in patients with cancer: a review. JAMA Oncol. (2019) 5:1347–54. doi: 10.1001/jamaoncol.2019.0034
15. Baselga J, Campone M, Piccart M, Burris HA, Rugo HS, Sahmoud T, et al. Everolimus in postmenopausal hormone-receptor–positive advanced breast cancer. N Engl J Med. (2011) 366:520–9. doi: 10.1056/NEJMoa1109653
16. Piccart M, Hortobagyi GN, Campone M, Pritchard KI, Lebrun F, Ito Y, et al. Everolimus plus exemestane for hormone-receptor-positive, human epidermal growth factor receptor-2-negative advanced breast cancer: overall survival results from BOLERO-2dagger. Ann Oncol. (2014) 25:2357–62. doi: 10.1093/annonc/mdu456
17. Baselga J, Im S-A, Iwata H, Cortés J, De Laurentiis M, Jiang Z, et al. Buparlisib plus fulvestrant versus placebo plus fulvestrant in postmenopausal, hormone receptor-positive, HER2-negative, advanced breast cancer (BELLE-2): a randomised, double-blind, placebo-controlled, phase 3 trial. Lancet Oncol. (2017) 18:904–16. doi: 10.1016/S1470-2045(17)30376-5
18. Criscitiello C, Viale G, Curigliano G, Goldhirsch A. Profile of buparlisib and its potential in the treatment of breast cancer: evidence to date. Breast Cancer. (2018) 10:23–9. doi: 10.2147/BCTT.S134641
19. Jazieh K, Bell R, Agarwal N, Abraham J. Novel targeted therapies for metastatic breast cancer. Ann Transl Med. (2020) 8:907. doi: 10.21037/atm.2020.03.43
20. Verret B, Cortes J, Bachelot T, Andre F, Arnedos M. Efficacy of PI3K inhibitors in advanced breast cancer. Ann Oncol. (2019) 30(Suppl.10):x12-−20. doi: 10.1093/annonc/mdz381
21. André F, Ciruelos E, Rubovszky G, Campone M, Loibl S, Rugo HS, et al. Alpelisib for PIK3CA-mutated, hormone receptor–positive advanced breast cancer. N Engl J Med. (2019) 380:1929–40. doi: 10.1056/NEJMoa1813904
22. André F, Ciruelos EM, Juric D, Loibl S, Campone M, Mayer I, et al. LBA18 Overall survival (os) results from SOLAR-1, a phase III study of alpelisib (ALP) + fulvestrant (FUL) for hormone receptor-positive (HR+), human epidermal growth factor receptor 2-negative (HER2–) advanced breast cancer (ABC). Ann Oncol. (2020) 31:S1150–1. doi: 10.1016/j.annonc.2020.08.2246
23. Vivanco I, Sawyers CL. The phosphatidylinositol 3-Kinase AKT pathway in human cancer. Nat Rev Cancer. (2002) 2:489–501. doi: 10.1038/nrc839
24. Thorpe LM, Yuzugullu H, Zhao JJ. PI3K in cancer: divergent roles of isoforms, modes of activation and therapeutic targeting. Nat Rev Cancer. (2015) 15:7–24. doi: 10.1038/nrc3860
25. Venetis K, Sajjadi E, Haricharan S, Fusco N. Mismatch repair testing in breast cancer: the path to tumor-specific immuno-oncology biomarkers. Transl Cancer Res. (2020) 9:1852. doi: 10.21037/tcr-20-1852
26. Fruman DA, Chiu H, Hopkins BD, Bagrodia S, Cantley LC, Abraham RT. The PI3K pathway in human disease. Cell. (2017) 170:605–35. doi: 10.1016/j.cell.2017.07.029
27. Carrera AC, Anderson R. The cell biology behind the oncogenic PIP3 lipids. J Cell Sci. (2019) 132:228395. doi: 10.1242/jcs.228395
28. Karakas B, Bachman KE, Park BH. Mutation of the PIK3CA oncogene in human cancers. Br J Cancer. (2006) 94:455–9. doi: 10.1038/sj.bjc.6602970
29. Fusco N, Sajjadi E, Venetis K, Gaudioso G, Lopez G, Corti C, et al. PTEN alterations and their role in cancer management: are we making headway on precision medicine? Genes. (2020) 11:719. doi: 10.3390/genes11070719
30. Hoxhaj G, Manning BD. The PI3K-AKT network at the interface of oncogenic signalling and cancer metabolism. Nat Rev Cancer. (2020) 20:74–88. doi: 10.1038/s41568-019-0216-7
31. Ortega MA, Fraile-Martinez O, Asunsolo A, Bujan J, Garcia-Honduvilla N, Coca S. Signal transduction pathways in breast cancer: the important role of PI3K/Akt/mTOR. J Oncol. (2020) 2020:9258396. doi: 10.1155/2020/9258396
32. Huang CH, Mandelker D, Schmidt-Kittler O, Samuels Y, Velculescu VE, Kinzler KW, et al. The structure of a human p110alpha/p85alpha complex elucidates the effects of oncogenic PI3Kalpha mutations. Science. (2007) 318:1744–8. doi: 10.1126/science.1150799
33. Burke JE, Perisic O, Masson GR, Vadas O, Williams RL. Oncogenic mutations mimic and enhance dynamic events in the natural activation of phosphoinositide 3-kinase p110alpha (PIK3CA). Proc Natl Acad Sci USA. (2012) 109:15259–64. doi: 10.1073/pnas.1205508109
34. Klempner SJ, Myers AP, Cantley LC. What a tangled web we weave: emerging resistance mechanisms to inhibition of the phosphoinositide 3-kinase pathway. Cancer Discov. (2013) 3:1345–54. doi: 10.1158/2159-8290.CD-13-0063
35. Porta C, Paglino C, Mosca A. Targeting PI3K/Akt/mTOR signaling in cancer. Front Oncol. (2014) 4:64. doi: 10.3389/fonc.2014.00064
36. Marchiò C, De Filippo MR, Ng CK, Piscuoglio S, Soslow RA, Reis-Filho JS, et al. PIKing the type and pattern of PI3K pathway mutations in endometrioid endometrial carcinomas. Gynecol Oncol. (2015) 137:321–8. doi: 10.1016/j.ygyno.2015.02.010
37. Markham A. Alpelisib: first global approval. Drugs. (2019) 79:1249–53. doi: 10.1007/s40265-019-01161-6
38. Copur MS. Alpelisib to treat breast cancer. Drugs Today. (2020) 56:357–63. doi: 10.1358/dot.2020.56.6.3137526
39. Anderson EJ, Mollon LE, Dean JL, Warholak TL, Aizer A, Platt EA, et al. A systematic review of the prevalence and diagnostic workup of PIK3CA mutations in HR+/HER2- metastatic breast cancer. Int J Breast Cancer. (2020) 2020:3759179. doi: 10.1155/2020/3759179
40. Cheung LW, Yu S, Zhang D, Li J, Ng PK, Panupinthu N, et al. Naturally occurring neomorphic PIK3R1 mutations activate the MAPK pathway, dictating therapeutic response to MAPK pathway inhibitors. Cancer Cell. (2014) 26:479–94. doi: 10.1016/j.ccell.2014.08.017
41. Samuels Y, Wang Z, Bardelli A, Silliman N, Ptak J, Szabo S, et al. High frequency of mutations of the PIK3CA gene in human cancers. Science. (2004) 304:554. doi: 10.1126/science.1096502
42. Martínez-Sáez O, Chic N, Pascual T, Adamo B, Vidal M, González-Farré B, et al. Frequency and spectrum of PIK3CA somatic mutations in breast cancer. Breast Cancer Res. (2020) 22:45. doi: 10.1186/s13058-020-01284-9
43. Chakravarty D, Gao J, Phillips SM, Kundra R, Zhang H, Wang J, et al. OncoKB: a precision oncology knowledge base. JCO Precis Oncol. (2017) 2017:11. doi: 10.1200/PO.17.00011
44. Tate JG, Bamford S, Jubb HC, Sondka Z, Beare DM, Bindal N, et al. COSMIC: the catalogue of somatic mutations in cancer. Nucl Acids Res. (2019) 47:D941–7. doi: 10.1093/nar/gky1015
45. Gymnopoulos M, Elsliger MA, Vogt PK. Rare cancer-specific mutations in PIK3CA show gain of function. Proc Natl Acad Sci USA. (2007) 104:5569–74. doi: 10.1073/pnas.0701005104
46. Dogruluk T, Tsang YH, Espitia M, Chen F, Chen T, Chong Z, et al. Identification of variant-specific functions of PIK3CA by rapid phenotyping of rare mutations. Cancer Res. (2015) 75:5341–54. doi: 10.1158/0008-5472.CAN-15-1654
47. Vasan N, Razavi P, Johnson JL, Shao H, Shah H, Antoine A, et al. Double PIK3CA mutations in cis increase oncogenicity and sensitivity to PI3Kalpha inhibitors. Science. (2019) 366:714–23. doi: 10.1126/science.aaw9032
48. Mayer IA, Abramson VG, Formisano L, Balko JM, Estrada MV, Sanders ME, et al. A phase Ib study of Alpelisib (BYL719), a PI3Kalpha-specific inhibitor, with letrozole in ER+/HER2- metastatic breast cancer. Clin Cancer Res. (2017) 23:26–34. doi: 10.1158/1078-0432.CCR-16-0134
49. Bakker E. Is the DNA sequence the gold standard in genetic testing? Quality Mol Genetic Tests Assessed Clin Chem. (2006) 52:557–8. doi: 10.1373/clinchem.2005.066068
50. Arsenic R, Treue D, Lehmann A, Hummel M, Dietel M, Denkert C, et al. Comparison of targeted next-generation sequencing and Sanger sequencing for the detection of PIK3CA mutations in breast cancer. BMC Clin Pathol. (2015) 15:20. doi: 10.1186/s12907-015-0020-6
51. Ang D, O'Gara R, Schilling A, Beadling C, Warrick A, Troxell ML, et al. Novel method for PIK3CA mutation analysis: locked nucleic acid–PCR sequencing. J Mol Diagn. (2013) 15:312–8. doi: 10.1016/j.jmoldx.2012.12.005
52. Nollau P, Wagener C. Methods for detection of point mutations: performance and quality assessment. The IFCC scientific division, committee on molecular biology techniques. J Int Fed Clin Chem. (1997) 9:162–70.
53. Alvarez-Garcia V, Bartos C, Keraite I, Trivedi U, Brennan PM, Kersaudy-Kerhoas M, et al. A simple and robust real-time qPCR method for the detection of PIK3CA mutations. Sci Rep. (2018) 8:4290. doi: 10.1038/s41598-018-22473-9
54. Lambert A, Salleron J, Lion M, Rouyer M, Lozano N, Leroux A, et al. Comparison of three real-time PCR assays for the detection of PIK3CA somatic mutations in formalin-fixed paraffin embedded tissues of patients with breast carcinomas. Pathol Oncol Res. (2019) 25:1117–23. doi: 10.1007/s12253-018-0538-x
55. Harle A, Lion M, Lozano N, Husson M, Harter V, Genin P, et al. Analysis of PIK3CA exon 9 and 20 mutations in breast cancers using PCR-HRM and PCR-ARMS: correlation with clinicopathological criteria. Oncol Rep. (2013) 29:1043–52. doi: 10.3892/or.2013.2229
56. Cizkova M, Susini A, Vacher S, Cizeron-Clairac G, Andrieu C, Driouch K, et al. PIK3CA mutation impact on survival in breast cancer patients and in ERalpha, PR and ERBB2-based subgroups. Breast Cancer Res. (2012) 14:R28. doi: 10.1186/bcr3113
57. Chan AC, Weiss LM, Bender R. Abstract P4-09-15: a large study of PIK3CA mutations in the community setting identifies varying degree of mutation positivity rates across age groups in advanced HR+, HER2- breast cancer patients using an FDA RT-PCR cleared test. Cancer Res. (2020) 80(4Suppl.):P4-09-15–P04-09-15. doi: 10.1158/1538-7445.SABCS19-P4-09-15
58. Rothberg JM, Hinz W, Rearick TM, Schultz J, Mileski W, Davey M, et al. An integrated semiconductor device enabling non-optical genome sequencing. Nature. (2011) 475:348–52. doi: 10.1038/nature10242
59. Vigliar E, Malapelle U, de Luca C, Bellevicine C, Troncone G. Challenges and opportunities of next-generation sequencing: a cytopathologist's perspective. Cytopathology. (2015) 26:271–83. doi: 10.1111/cyt.12265
60. Li R, Tang T, Hui T, Song Z, Li F, Li J, et al. Impact of next-generation sequencing (NGS) for primary endocrine resistance in breast cancer patients. Int J Clin Exp Pathol. (2018) 11:5450–8.
61. Mosele F, Stefanovska B, Lusque A, Tran Dien A, Garberis I, Droin N, et al. Outcome and molecular landscape of patients with PIK3CA-mutated metastatic breast cancer. Ann Oncol. (2020) 31:377–86. doi: 10.1016/j.annonc.2019.11.006
62. Tang Y, Li J, Xie N, Yang X, Liu L, Wu H, et al. PIK3CA gene mutations in the helical domain correlate with high tumor mutation burden and poor prognosis in metastatic breast carcinomas with late-line therapies. Aging. (2020) 12:1577–90. doi: 10.18632/aging.102701
63. Heidrich I, Ačkar L, Mohammadi PM, Pantel K. Liquid biopsies: potential and challenges. Int J Cancer. (2020) 148:528–45. doi: 10.1002/ijc.33217
64. Board RE, Wardley AM, Dixon JM, Armstrong AC, Howell S, Renshaw L, et al. Detection of PIK3CA mutations in circulating free DNA in patients with breast cancer. Breast Cancer Res Treat. (2010) 120:461–7. doi: 10.1007/s10549-010-0747-9
65. Beaver JA, Jelovac D, Balukrishna S, Cochran R, Croessmann S, Zabransky DJ, et al. Detection of cancer DNA in plasma of patients with early-stage breast cancer. Clin Cancer Res. (2014) 20:2643–50. doi: 10.1158/1078-0432.CCR-13-2933
66. Juric D, Ciruelos E, Rubovszky G, Campone M, Loibl S, Rugo H, et al. Abstract GS3-08: Alpelisib + fulvestrant for advanced breast cancer: Subgroup analyses from the phase III SOLAR-1 trial. Cancer Res. (2019) 79(4Suppl.):GS3-08–GS03-08. doi: 10.1158/1538-7445.SABCS18-GS3-08
67. Markou A, Farkona S, Schiza C, Efstathiou T, Kounelis S, Malamos N, et al. PIK3CA mutational status in circulating tumor cells can change during disease recurrence or progression in patients with breast cancer. Clin Cancer Res. (2014) 20:5823–34. doi: 10.1158/1078-0432.CCR-14-0149
68. Tzanikou E, Markou A, Politaki E, Koutsopoulos A, Psyrri A, Mavroudis D, et al. PIK3CA hotspot mutations in circulating tumor cells and paired circulating tumor DNA in breast cancer: a direct comparison study. Mol Oncol. (2019) 13:2515–30. doi: 10.1002/1878-0261.12540
Keywords: breast cancer, biomarkers, PIK3CA, targeted therapy, HR+/HER2-, RT-PCR, next-generation sequencing, liquid biopsy
Citation: Fusco N, Malapelle U, Fassan M, Marchiò C, Buglioni S, Zupo S, Criscitiello C, Vigneri P, Dei Tos AP, Maiorano E and Viale G (2021) PIK3CA Mutations as a Molecular Target for Hormone Receptor-Positive, HER2-Negative Metastatic Breast Cancer. Front. Oncol. 11:644737. doi: 10.3389/fonc.2021.644737
Received: 21 December 2020; Accepted: 10 February 2021;
Published: 25 March 2021.
Edited by:
Carmine De Angelis, University of Naples Federico II, ItalyReviewed by:
Lorenzo Gerratana, University of Udine, ItalyCarlos Martinez-Perez, Medical Research Council Institute of Genetics and Molecular Medicine (MRC), United Kingdom
Copyright © 2021 Fusco, Malapelle, Fassan, Marchiò, Buglioni, Zupo, Criscitiello, Vigneri, Dei Tos, Maiorano and Viale. This is an open-access article distributed under the terms of the Creative Commons Attribution License (CC BY). The use, distribution or reproduction in other forums is permitted, provided the original author(s) and the copyright owner(s) are credited and that the original publication in this journal is cited, in accordance with accepted academic practice. No use, distribution or reproduction is permitted which does not comply with these terms.
*Correspondence: Giuseppe Viale, giuseppe.viale@ieo.it
†These authors have contributed equally to this work