- 1Center of Hematology, The First Affiliated Hospital of Nanchang University, Nanchang, China
- 2Institute of Hematology, Academy of Clinical Medicine of Jiangxi Province, Nanchang, China
- 3Clinical Research Center for Hematologic Disease of Jiangxi Province, Nanchang, China
- 4Institute of Lymphoma and Myeloma, Nanchang University, Nanchang, China
Epstein Barr virus (EBV) can affect 90% of the human population. It can invade B lymphocytes, T lymphocytes and natural killer cells of the host and remain in the host for life. The long latency and reactivation of EBV can cause malignant transformation, leading to various lymphoproliferative diseases (LPDs), including EBV-related B-cell lymphoproliferative diseases (EBV-B-LPDs) (for example, Burkitt lymphoma (BL), classic Hodgkin’s lymphoma (cHL), and posttransplantation and HIV-related lymphoproliferative diseases) and EBV-related T-cell lymphoproliferative diseases (EBV-T/NK-LPDs) (for example, extranodal nasal type natural killer/T-cell lymphoma (ENKTCL), aggressive NK cell leukaemia (ANKL), and peripheral T-cell lymphoma, not otherwise specified (PTCL-NOS). EBV-LPDs are heterogeneous with different clinical features and prognoses. The treatment of EBV-LPDs is usually similar to that of EBV-negative lymphoma with the same histology and can include chemotherapy, radiotherapy, and hematopoietic stem cell transplant (HSCT). However, problems such as serious toxicity and drug resistance worsen the survival prognosis of patients. EBV expresses a variety of viral and lytic proteins that regulate cell cycle and death processes and promote the survival of tumour cells. Based on these characteristics, a series of treatment strategies for EBV in related malignant tumours have been developed, such as monoclonal antibodies, immune checkpoint inhibitors, cytotoxic T lymphocytes (CTLs) and epigenetic therapy. These new individualized therapies can produce highly specific killing effects on tumour cells, and nontumour cells can be protected from toxicity. This paper will focus on the latest progress in the treatment of EBV-LPDs based on pathological mechanisms.
Background
Epstein Barr virus (EBV) is classified as a gamma-1 herpesvirus, and people are generally susceptible to this virus. Patients with EBV infection are usually asymptomatic, and the development of symptomatic disease is associated with delayed primary infection, leading to infectious mononucleosis in young people. In addition, EBV infection is associated with various EBV-related malignancies, such as nasopharyngeal carcinoma (NPC), gastric cancer subtypes, and several lymphoproliferative diseases (LPDs), especially B-cell and T-cell lymphomas (1, 2). It causes about 200000 new cancer cases worldwide every year. In cancer cells, EBV usually remains latent to escape human immune surveillance but can switch from a latent to a lytic cycle to infect new cells in response to physiological stimuli (3, 4). There are three groups of viral proteins expressed by EBV during a latent infection: (1) the Epstein Barr virus nuclear antigen (EBNA) family, which includes EBNA1–EBNA4, within which EBNA3 encompasses EBNA3a, EBNA3b and EBNA3c; (2) the late membrane protein (LMP) group, which includes LMP1 and LMP2; and (3) EBV-encoded RNA (EBER). These viral proteins follow four latent expression patterns, and different host cells express different viral proteins (3) (Figure 1). In cells infected with latent EBV, the immediate early (IE) proteins BZLF1 (Zta) and BRLF1 (Rta) are key for mediating transformation, but the two promoters that control Zta and Rta gene transcription are inactive (4). Upon induction by stimulants that activate B cells, such as calcium ionophores, phorbol esters and histone deacetylase (HDAC) inhibitors, these promoters are activated, resulting in the expression of immediate early lytic proteins, followed by the production of early lytic proteins (BMRF1, BALF1, BHRF1, BSLF1, etc.) and the initiation of viral DNA replication (9, 10). At the same time, early lytic proteins and DNA replication trigger the expression of late lytic proteins (VCA-p18, gp350/220, MCP, gH/gL, etc.), which eventually leads to the production of infectious virus particles such as BLLF1 and BFRF3 (4, 9). More than 70 viral proteins can be produced in the entire process (11) (Figure 2).
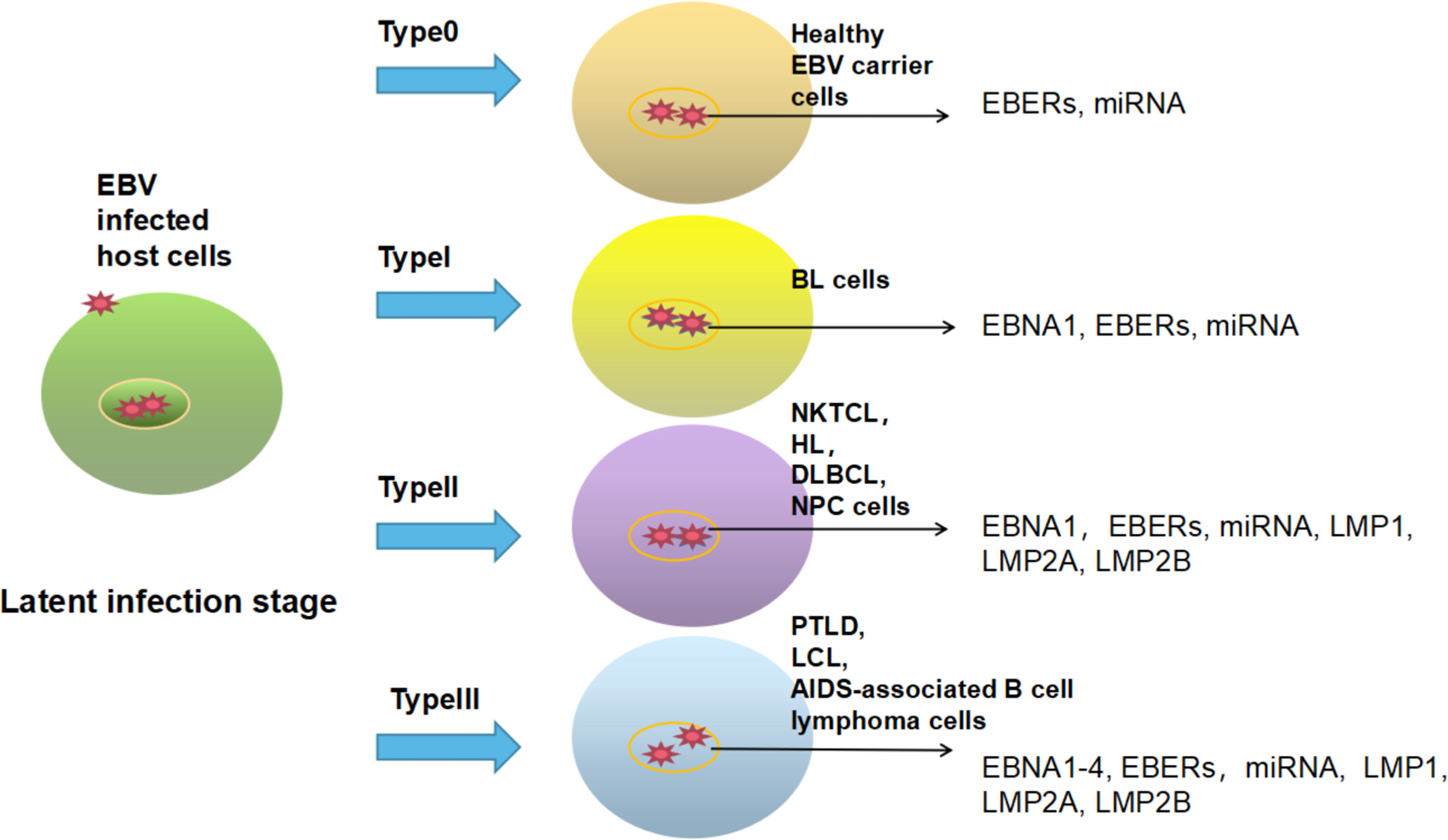
Figure 1 EBV expresses different viral proteins in different host cells during a latent infection. Latent type 0: EBER and miRNA are expressed in the nucleus of host cells, mainly in EBV carriers in good health. Latent type I: EBNA1, EBER and miRNA are commonly expressed in patients with Burkitt lymphoma (BL) (5). Latent type II: EBNA1, EBER, miRNA, LMP1, LMP2A, and LMP2B are generally expressed in patients with HL, nasal NK/T cell lymphoma (NKTCL), NPC and diffuse large B-cell lymphoma (DLBCL) (6, 7). Latent type III: EBNA1–4, EBER, miRNA, LMP1, LMP2A and LMP2B can be expressed in posttransplant lymphoproliferative disease (PTLD), lymphoblastic cell line (LCL) and AIDS-associated B-cell lymphoma (8).
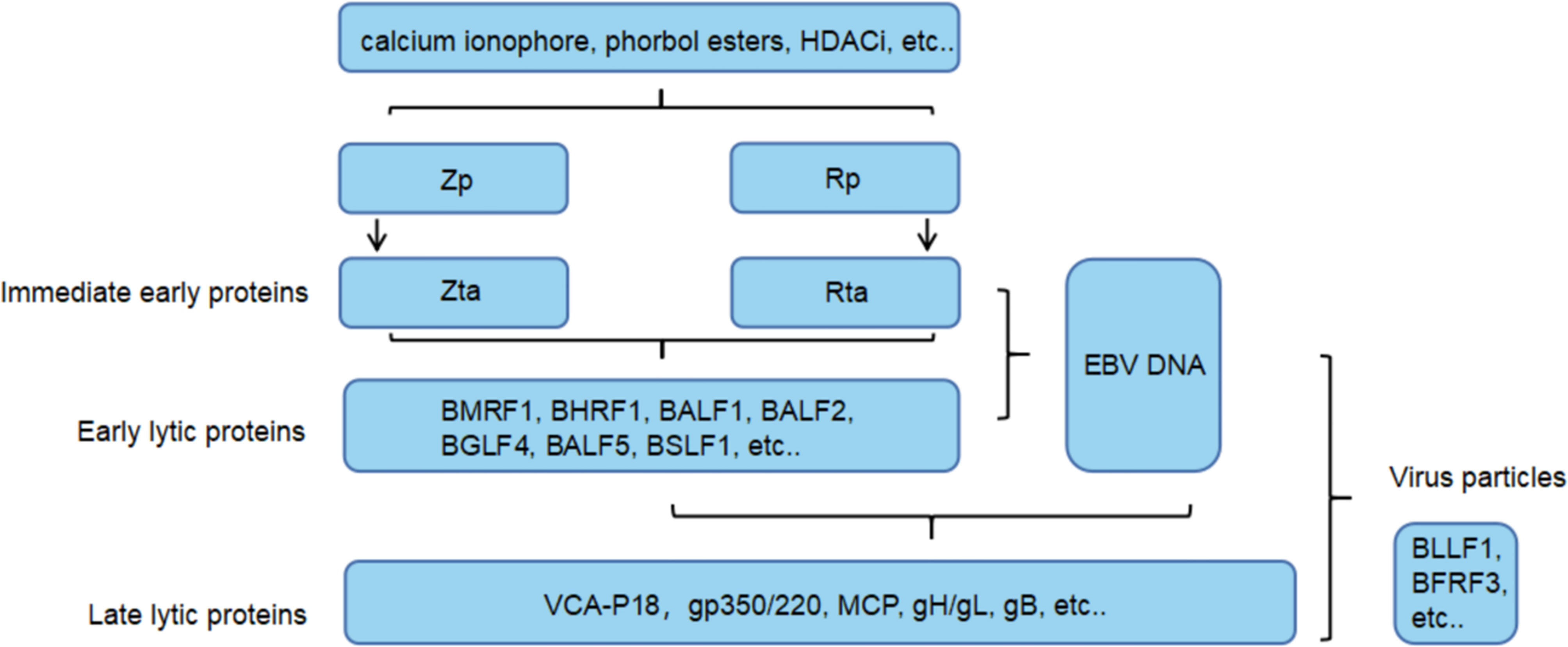
Figure 2 EBV lytic cycle reactivation. After a variety of stimulants activate the EBV Z/R promoters (Zp and Rp), the two immediate early proteins reciprocally activate expression and drive the EBV lytic cycle. In this process, a series of lytic proteins are produced to regulate gene expression and induce DNA replication. Finally, the virus particles are packaged by structural proteins and released through exocytosis.
There is increasing evidence that virus latent and lytic proteins can maintain the proliferation and survival of EBV-positive cancer cells by influencing cellular mechanisms regulating the cell cycle, apoptosis and immune recognition of host cells. Research to identify therapeutics for EBV has produced many surprising results. The specific killing effect not only improves the curative effect but also greatly reduces toxicity and side effects. Therefore, in addition to chemotherapy, regulatory immunosuppressive therapy and allogeneic hematopoietic stem cell transplant (allo-HSCT), different EBV-based treatment strategies, such as immunotherapy, gene therapy and epigenetic therapy, are research hotspots. This paper will summarize EBV-LPD treatment methods and discuss research advances regarding these new schemes.
EBV-Associated B-cell Lymphoproliferative Disorders
Chemotherapy
EBV was initially detected by Epstein MA et al. in isolated and cultured cells from a BL child in Uganda (12). BL is a highly proliferative B-cell non-Hodgkin lymphoma (NHL) that can be divided into three different variants: endemic (African) BL, sporadic BL and immunodeficiency-related BL (13). EBV can be detected in almost all cases of endemic BL and up to 40% of cases of immunodeficiency-related BL (14). Various intensive treatment schemes have shown great activity in patients with BL. Previous research found that CODOX-M/IVAC +/- R (cyclophosphamide, vincristine, doxorubicin, methotrexate, ifosfamide, etoposide, and cytarabine with or without rituximab), CALGB (Cancer and Leukemia Group B), Hyper-CVAD +/- R (cyclophosphamide, vincristine, doxorubicin, and dexamethasone with or without rituximab), and dose-adjusted (DA) R-EPOCH (rituximab, etoposide, prednisone, vincristine, cyclophosphamide, and doxorubicin) achieved significant results. The best results showed that patients can achieve 95% event-free survival (EFS) and 100% overall survival (OS) (15–20) (Table 1). The low-dose adjusted R-EPOCH regimen represents major progress, allowing the treatment of elderly and human immunodeficiency virus (HIV)-positive patients and solving the problem of limited application of a high-intensity regimen due to severe toxicity in these patient groups. However, for patients with endemic diseases, treatment schemes are usually limited by cost, and different low-income and middle-income countries approve different schemes. For example, the OS rate of patients in sub-Saharan Africa is between 51% and 67%, which is much worse than that in resource rich countries (38). Therefore, those successful studies cannot represent the heterogeneous population. Meanwhile, chemotherapy is not sufficient to block disease activity and eradicate infected cells. There is a lack of further salvage treatment for patients with recurrent or refractory BL, and there is an urgent need to develop more effective treatments.
Immunotherapy
Monoclonal antibodies
EBV is detected in 25–50% of Hodgkin’s lymphoma (HL) cases in the United States and Europe (39, 40). In immunosuppressed individuals, EBV-encoded LMP-1 contributes to NF-κB pathway activation and induces an antiapoptotic phenotype in Reed–Sternberg (RS) cells in HL (41). CD30, a transmembrane protein belonging to the tumour necrosis factor receptor family, is specifically expressed in normally activated (rather than static) B and T cells and NK cells. The CD30-targeted antibody brentuximab vedotin (BV) showed significant antitumour activity in recurrent or refractory (R/R) HL and anaplastic large cell lymphoma (ALCL), leading to its accelerated approval by the FDA (42). The results of a preliminary phase II study showed moderate efficacy of SGN-30 in HL patients with all levels of CD30 expression (43). In addition, continuous treatment with MDX-060, a human anti-CD30 immunoglobulin G1 monoclonal antibody, was well tolerated in phase I and II clinical trials. However, there has been no study on the effect of CD30 mAbs on EBV load.
In 2003, EBV+ diffuse large B cell lymphoma (DLBCL) was first described as a unique entity in elderly patients, and in situ hybridization showed that this disease was related to EBV (44). In 2008, the World Health Organization (WHO) defined the temporary entity of DLBCL based on a large number of studies conducted in Asian populations and named it ‘‘EBV positive DLBCL of the elderly”. After that, several groups studied the association between EBV and DLBCL in children and young people, and proved that EBV positive was also detected in these age groups (45–50), and some of which showing a larger morphological spectrum and better survival rate (51). Therefore, the term “elderly” was replaced with “not otherwise specified” (EBV + DLBCL, NOS) in the 2016 classification and is no longer considered provisional (52). Sarah Park et al. evaluated EBER expression by in situ hybridization in 380 samples from DLBCL patients to evaluate the significance of EBV positivity on the survival and prognosis of DLBCL patients. These researchers found that patients with EBER-positive DLBCL showed more rapid clinical deterioration and worse survival rates and treatment responses (53). CD20 is a nonglycosylated pan B-cell transmembrane phosphoprotein with a molecular weight of 35 KD that is expressed on the surface of most mature B cells. At present, rituximab, which targets CD20, combined with chemotherapy is the first-line treatment for EBV+ DLBCL. Different reactions to R-CHOP (rituximab, etoposide, prednisone, vincristine, cyclophosphamide, and doxorubicin) have been reported worldwide, but no prospective comparative study has been conducted (54). CD30 is expressed in some posttransplant lymphoproliferative diseases (PTLDs) induced by EBV infection, including DLBCL. Brentuximab vedotin (BV), a CD30-directed antibody–toxin conjugate, represents an attractive treatment. Thomas Mika et al. reported the first case of long-term control/cure of highly invasive EBV-DLBCL by combining BV and adoptive EBV-specific T cell therapy. The patient had no disease progression 3.5 years after PTLD. However, the long-term efficacy of BV monotherapy in the treatment of CD30+ DLBCL caused by PTLD has not been determined (22). Clinical trials of BV combined with other therapies are ongoing (Table 3). Other therapies were mainly studied in the background of PTLD, which has extensive effects on EBV+ DLBCL.
Reactivation of EBV after bone marrow transplantation usually leads to a LPD that does not respond well to standard treatment and is usually fatal. As early as 1969, the incidence rate of LPDs in solid organ and bone marrow transplant recipients was very high, between 0.5% and 17% (79, 80). EBV infection affects approximately 60–80% of patients with PTLD, including 100% of patients with early-onset PTLD (81). Associated EBV infection and CD20+ B cell proliferation were observed in 90% of cases. Therefore, in addition to stopping immunosuppressive drugs, monoclonal anti-CD20 antibodies (such as rituximab) can be used in standard treatment (82, 83). W J F M van der Velden et al. noted that pre-emptive treatment, which is defined as rituximab administration to patients with a symptomatic EBV infection, is related to the elimination of PTLD-related mortality (21). At present, there is no standardized rescue treatment. If there is no response to anti-CD20 treatment, patient prognosis is very poor. Patrick-Pascal Strunz et al. reported the first case of combined treatment with a CD38 antibody (daratumumab) and EBV-specific cytotoxic T lymphocytes for EBV+ rituximab-refractory PTLD. The flow cytometry results of a sample from the 55-year-old male patient showed the loss of CD20 and continuously high CD38 expression in the homogeneous B cell population. Therefore, after the administration of EBV-specific cytotoxic T lymphocytes to this patient, daratumumab was added, and a rapid decrease in EBV viral load was observed. However, the examination results showed early recurrence of PTLD after 2 weeks (23). The role of CD38-targeted immunotherapy in the treatment of rituximab-refractory CD38+ PTLD needs to be further explored. In addition, the CD30 antibody mentioned above is also used to treat PTLD.
Checkpoint Inhibitors
Programmed death ligand 1 (PD-L1) is an immunomodulatory molecule expressed by antigen-presenting cells that selectively binds to the PD-1 receptor on T cells to inhibit T cell immune function. Both RS cells of cHL and malignant B cells of PTLD express PD-L1, and this expression is promoted by EBV. Therefore, these diseases share this immune escape mechanism (35, 36, 84) (Figure 3). Nivolumab and pembrolizumab, humanized anti-PD-1 monoclonal antibodies, have been proven to be effective in the treatment of patients with R/R cHL. In 2016, Anas Younes and his team found that the overall response rate (ORR) for nivolumab was 66% with acceptable safety in cHL patients who failed autologous stem cell transplant (ASCT) (24). In 2017, a phase II study involving 221 R/R HL patients showed that the ORR for pembrolizumab was 69%, and the complete remission (CR) rate was 22.4% (26). Subsequent extended follow-up reported that the median PFS was 14 months (85). Nivolumab and pembrolizumab were approved by the FDA in 2016 and began to be used in the treatment of cHL in R/R patients in 2017. In addition, pembrolizumab can be included in consolidation treatment after ASCT. Philippe Armand et al. conducted the first immune checkpoint blocking study for consolidation therapy in patients with R/R cHL and proved that PD-1 blockade with pembrolizumab after ASCT had acceptable safety and a significant progression-free survival (PFS) benefit (86). A large number of combined therapy studies including PD-1 have emerged (Table 1). The combined use of BV-nivolumab is a promising scheme. In a single arm phase I/II study, BV-nivolumab was applied to patients before ASCT, achieving a high CR of 67% and an ORR of 85% (30, 87). Among patients with high-risk R/R HL, consolidation after HCT with BV-nivolumab resulted in an estimated 18 month PFS and OS of 95% and 98% in all 59 patients, respectively (31). In addition, the combination of the two drugs is effective and well tolerated in newly treated elderly patients with HL (32). There are also a variety of new PD-1 inhibitors, such as sintilimab, tirizumab and camerezumab, which are under development. Their ORR is about 80% and CR rate is 30 – 60% (27–29) (Table 1). These studies excluded patients previously treated with PD-1. In the future, it will be interesting to assess the response of patients previously exposed to checkpoint inhibitors to new PD-1 inhibitors. Given the known efficacy in cHL, the use of immune checkpoint inhibitors in PTLD is promising (88). It is reported that after all conventional treatments failed and significant toxicity occurred in a child with PTLD, the salvage treatment with nivolumab achieved CR (33). Similarly, this result was also observed after application of nivolumab in a woman with cHL-like PTLD (34). At present, a phase I clinical trial to explore the efficacy of nivolumab and EBV-specific T cells in patients with R/R EBV-positive lymphoma, including PTLD, is completed, but no results were released (NCT02973113).
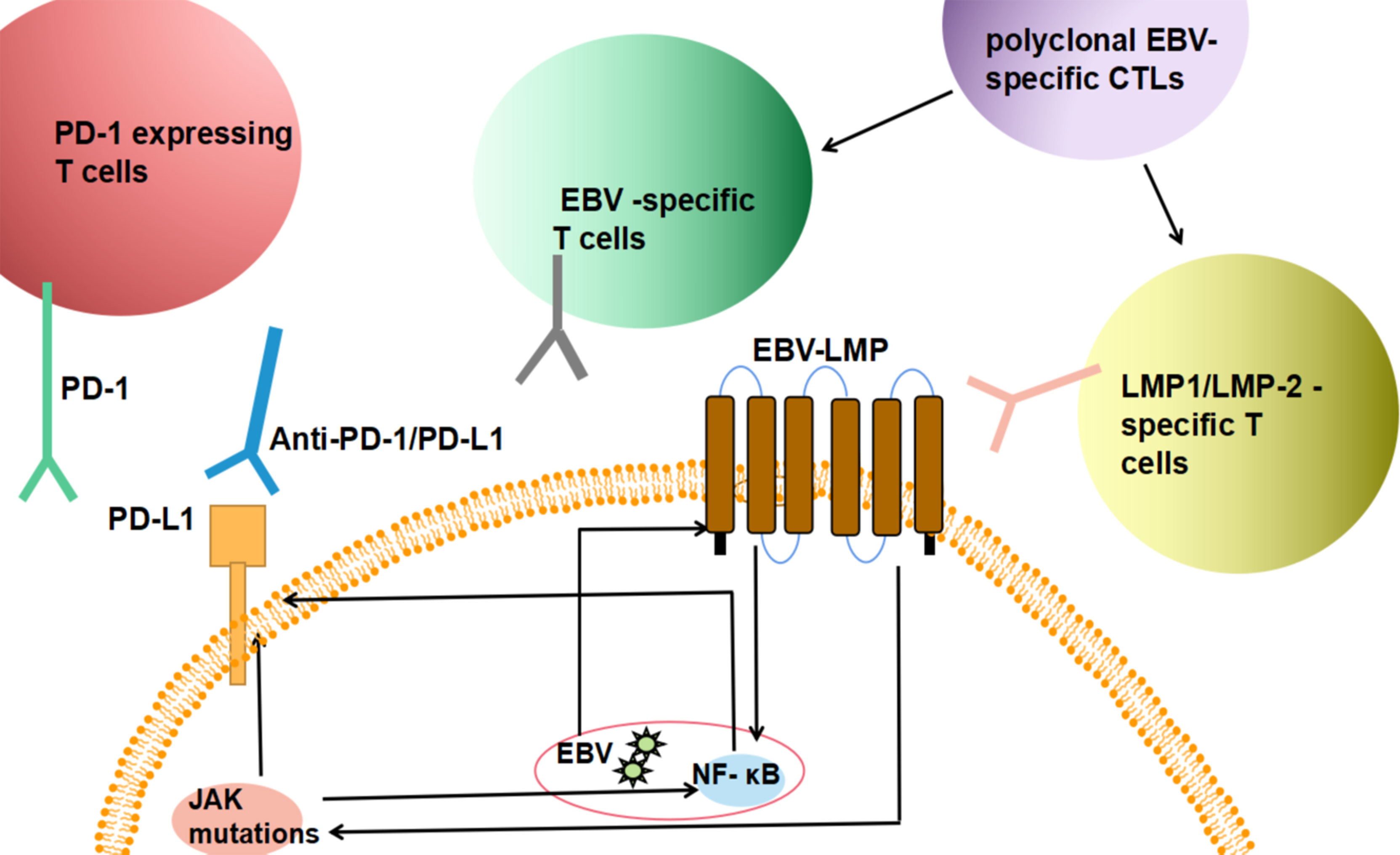
Figure 3 Summary of the immunotherapeutic mechanism of EBV infected tumor cells. EBV infected tumor cells express PD-L1, and binding with PD-1-expressing T cells in microenvironment can inhibit immune killing function; EBV drives the overexpression of latent membrane protein LMP and the activates NF- κB/MAPK and JAK/STAT signaling pathways lead to high expression of PD-L1. Therefore, after the infusion of polyclonal EBV specific CTLs, the increased frequency of EBV and LMP specific T cells and the application of PD-1/PD-1/L1 drugs can reduce immunosuppression and kill tumour cells.
CTLs
Immunotherapy strategies to restore virus-specific immunity are an attractive alternative to antiviral therapy. EBV-specific CTLs of human leukocyte antigens (HLA)-matched donors or autologous lymphocytes can be activated and expanded in vitro and then injected into the recipient, where they can restore cellular immunity after EBV infection and eradicate EBV-infected cells. The most common adverse reaction is graft-versus-host disease (GVHD). Compared with adoptive therapy with monoclonal antibodies, CTLs can actively migrate through the microvascular wall to reach isolated tumour cells and undergo self-expansion. CTLs can kill tumour cells through cytotoxic effectors and have advantages in biological distribution and antitumour activity (89). In some initial single-centre experiments, EBV-CTLs were used to treat viral reactivation after bone marrow transplantation and achieved clear results (90, 91). In 1994, Ekaterina Doublovina et al. reported that five patients with EBV-associated lymphoma achieved sustained clinical remission after receiving peripheral blood mononuclear cells (PBMCs) from EBV-seropositive transplant donors (92). Subsequently, C M Rooney et al. prepared the first EBV CTL line in vitro from donor leukocytes and infused these cells into ten lymphoma patients after allogeneic transplantation to reconstruct EBV-specific immunity; the cells were found to persist for ten weeks in vivo without GVHD (93). The team infused EBV-CTLs in 114 HSCT patients at three different centres over a 12-year period. The CR rate reached 84.6% in 13 patients with PTLD and proved that the duration of functional CTLs was up to 9 years. The other patients who received preventive treatment did not develop PTLD [42]. The main disadvantages of generating EBV-CTLs for specific patients are that it is expensive and time-consuming; it takes three to four months to generate a suitable CTL system. Tanzina Haque et al. generated allogeneic virus-specific CTL line banks from normal donors, overcoming the main limitations. It was found that the higher the HLA match between patients and CTLs was, the better the response. In this phase II clinical trial, the 6-month response rate of 33 patients with PTLD who failed conventional treatment was 52% (94). In addition, the autologous T cell therapy CMD-003 has been approved by the FDA for the treatment of R/R lymphoma and PTLD (3) (Table 1). Due to cost constraints and technical difficulties, EBV-specific CTLs are always used after the patient’s condition has deteriorated, and this treatment strategy is not currently being used in the clinic. A multicentre, open label, single arm, phase III study was completed in March 2021. In the context of allogeneic haematopoietic cell transplantation, the efficacy and safety of an allogeneic T cell immunotherapy called tabelecleucel in the treatment of EBV+ PTLD were evaluated. The results have not been published (NCT03392142). Other relevant clinical trials are still in progress (Table 3).
RS cells in EBV-positive cHL can downregulate immune-dominant EBV nuclear antigens such as EBNA3A, EBNA3B and EBNA3C so that most CTLs against potential antigens lose their effect. However, the latent proteins LMP-1 and LMP-2 expressed by tumour cells in approximately 40% of patients with Hodgkin’s or non-Hodgkin’s lymphoma then become the immunotherapeutic targets of CTLs (95) (Figure 3). M A Roskrow et al. conducted a number of phase I clinical trials in patients with cHL using polyclonal EBV-CTLs and CTLs rich in precursors targeting LMP-2 (37). A large number of EBV-specific T cells were obtained from 9 patients with advanced Hodgkin’s disease and successfully expanded slowly in vitro. After these cells were injected into patients with multiple relapses, the activity of EBV-specific CTLs increased, which enhanced the immune response to EBV for more than 13 weeks. In addition, it was found that these CTLs could recognize LMP2A expressed by tumour cells (Table 1).
Gene Therapy
The transcription factor EBNA2, a latent viral gene expressed by malignant cells latently infected with EBV, is a transactivator of virus and gene expression (96). M Franken et al. showed that use of the BamHI C promoter (CP) of EBNA2 in B-cell lymphoma can regulate the expression of the suicide gene and can selectively enhance the sensitivity of EBNA2-expressing cells to ganciclovir. These results provide in vivo and in vitro support for gene therapy based on the molecular basis of tumour development (96). Jian Hua Li et al. reported the feasibility of adenovirus-mediated wild-type p53 (Ad5CMV-p53) gene therapy for NPC. The adenovirus vector regulates transgene expression through the EBV replication initiation site, which can accurately transmit p53 to EBV-positive cancer cells and induce apoptosis. This approach shows effective cytotoxicity that can be enhanced by ionizing radiation (97). The team then further demonstrated that the results of EBV-targeted gene therapy could be replicated in mouse tumour models, which is expected to support the translation of this strategy into the clinic for NPC patients (98). At present, there is little research on gene therapy in EBV-LPDs, although there is much space for further exploration.
EBV-Associated T- and NK-Cell Lymphoproliferative Disorders
Chemotherapy
Epstein Barr virus (EBV) can cause B-cell lymphoma and is also found in some T or NK cell lymphoid tumours, such as extranodal NK/T-cell lymphoma (ENKTCL), invasive NK cell leukaemia (ANKL) and chronic active Epstein Barr virus infection (CAEBV). The WHO classification of haematopoietic and lymphoid tumours was revised in 2017 (WHO 2017), and CAEBV is defined as an T cell or NK cell tumour (99). The infected cells in CAEBV are activated and clonally proliferate with the characteristics of inflammation and malignancy. Affected patients experience fatal progression after lymphoma or HLH, so treatment needs to be started before this occurs. Chemotherapy can significantly reduce disease activity and the burden of residual EBV-infected T cells, but it is usually impossible to significantly reduce EBV-DNA load before haematopoietic cell transplantation. One of the main goals of chemotherapy is to control disease and reduce the risk of transplantation-related complications. Akihisa Sawada and others selected the combination of steroids, etoposide and cyclosporine or cytotoxic chemotherapy according to the treatment of lymphoma and then performed HSCT. The OS of 79 patients with CAEBV and related diseases reached 87% (100).
The prototype of EBV-driven lymphoma is extranodal NK/T cell lymphoma of the nasal type. Due to the high expression level of P-glycoprotein in NK lymphoma cells, chemotherapy, such as CHOP- and doxorubicin-based regimens, is largely ineffective. However, the lack of asparagine synthase makes ENKTCL sensitive to L-asparaginase. DDGP (cisplatin, dexamethasone, gemcitabine and pegaspargase) was recommended as the first-line chemotherapy for NK/T-cell lymphoma in the National Comprehensive Cancer Network (NCCN) guidelines in 2020. Other schemes based on L-asparaginase, such as SMILE (dexamethasone, methotrexate, ifosfamide, asparaginase, and etoposide), also showed good efficacy; however, this regimen is highly toxic and can even lead to death (55). DDGP, P-Gemox and AspaMetDex are less toxic alternatives to SMILE (56, 57) (Table 2).
EBV+ PTCL-NOS is a highly heterogeneous mature post-thymic T cell tumour. CHOP is the most commonly used first-line treatment for systemic PTCL, but this disease is usually incurable. When EBV-related LPD is pathological grade 3 and the patient has severe systemic symptoms, chemotherapy can be considered to control the disease condition but cannot improve patient prognosis (101).
Allo-HSCT
Numerous studies at home and abroad have proven that allo-HSCT can cure EBV-T/NK-LPD. In 2000, K Kawa et al. first reported the cure of a patient with CAEBV by allogeneic bone marrow transplantation, which also eradicated EBV-infected peripheral T cells and natural killer cells (102). A series of studies have proven an obvious survival benefit for patients treated with HSCT (103, 104). However, the prognosis of patients with active disease at allo-HSCT is worse than that of patients without active disease (105). Arai et al. found that 4 of the 5 patients with active disease at the time of HSCT experienced transplantation failure or recipient cell recovery. These findings suggest the importance of disease control before HSCT. Recently, Ichiro Yonese et al. evaluated 100 patients with newly diagnosed EBV-T/NK-LPD in Japan from January 2003 to March 2016 and found that the 3-year overall survival rates of chemotherapy alone, allo-HSCT after chemotherapy and allo-HSCT alone were 0%, 65% and 82%, respectively (58). Therefore, chemotherapy has not solved the problem of disease activity before transplantation. Although allo-HSCT can cure patients, the risk of complications after transplantation is high, and it is generally reserved for use in severe cases.
Immunotherapy
Monoclonal Antibodies
To date, several monoclonal antibodies against human CD38 have been successfully developed, such as daratumumab, isatuximab (SAR650984) and MOR202 (59). Wang L and colleagues demonstrated that 95% of NK/T cell lymphoma (NKTCL) cases were CD38 positive and half had high CD38 expression, which was significantly correlated with adverse results, indicating the potential role of CD38 as a therapeutic target in ENKTCL (106). A subsequent case report described an Asian woman with R/R NKTCL who achieved CR with daratumumab (107). At the 2018 American Society of Haematology (ASH) meeting, preliminary results reported a good remission rate with daratumumab for the treatment of patients with R/R NKTCL, and the ORR was 35.7% (59). The latest phase 2 study of R/R NKTCL included 32 Asian patients, and the ORR reached 25% after the application of single drug daratumumab. All patients were well tolerated, but none reached CR and the response duration was short (60). This may indicate that daratumumab alone is insufficient to treat patients with invasive characteristics, especially those with poor prognosis. Whether daretouximab can be used in combination with drugs for the treatment of NKTCL remains to be further studied. The CD30 drug–antibody conjugate brentuximab vedotin is effective in NKTCL because CD30 was reported by Feng Y et al. to be expressed in approximately 50% of 622 patients (108). To date, no clinical trials have been conducted specifically for recurrent/refractory NKTCL, but two patients were reported to achieve CR after BV treatment. Hee Kyung Kim et al. reported a case of an R/R NKTCL male patient with skin lesions who received brentuximab vedotin monotherapy and achieved long-term CR after 4 cycles. Subsequently, Li Mei Poon et al. treated ENKTCL that relapsed after chemotherapy with a combination of brentuximab vedotin with bendamustine and achieved CR (61, 62) (Table 2).
Checkpoint Inhibitors
EBV-driven latent membrane protein LMP1 is overexpressed to activate the NF-κB/MAPK and JAK/STAT signalling pathways, which leads to high PD-L1 expression. Based on the involvement of these signalling pathways, anti-PD-1 antibodies and JAK1/2/3 inhibitors have been the subject of specific drug research and applications (59) (Figure 3). Anti-PD-1 antibodies such as pembrolizumab and nivolumab can disrupt the interaction between PD-L1 and PD-1, thereby restoring the antitumour activity of activated T cells (109). Kwong et al. Have confirmed the efficacy of pembrolizumab in NKTCL patients (63); five of the seven patients with advanced disease achieved a CR. Immunohistochemical staining of residual lesions showed only a few CD56+EBER+ cells in infiltrating lymphocytes. This finding is consistent with the hypothesis that pembrolizumab treatment allows T cells to recognize and kill EBV-infected NK/T lymphoma cells. Xin Li et al. applied pembrolizumab in seven patients with R/R ENKL after extensive pretreatment. Two had CR and two had PR. The total response rate was 57% (64). In an independent study, three patients with R/R NKTCL also achieved a clinical response with low-dose nivolumab (65). In addition to these two popular drugs, some other PD-1/PD-L1 studies have preliminary results. In a multicenter, single arm, phase 2 clinical trial, among 28 R/R NKTCL patients, 75% achieved ORR after continuous use of sindilimab for 24 months (66). The PD-L1 inhibitor avelumab also showed monodrug activity in some patients with R/R NKTCL. In the open label phase 2 study, Seok Jin Kim et al. reported a CR of 24% (5/21) and an ORR of 38% (8/21) (67). More relevant prospective studies are still in progress (Table 3). PRN371 is a small molecule selective JAK3 inhibitor. Nairismägi ML and his colleagues conducted a preclinical evaluation of PRN371 and found that it significantly inhibited tumour growth in an NKTCL xenotransplantation model carrying a JAK3 activating mutation, which is consistent with the in vitro results (69). In addition, tofacitinib can significantly inhibit JAK3 activity in vivo and in vitro, but its clinical application in cancer treatment is limited by pan-JAK inhibitory activity. Shotaro Ando et al. found that treatment of EBV-positive T and NK cell lines with tofacitinib reduced phosphorylated STAT5 levels, inhibited proliferation, induced G1 phase arrest and reduced EBV LMP1 and EBNA1 expression (70). In addition, Erika Onozawa et al. determined that the JAK1/2 inhibitor ruxolitinib can inhibit the phosphorylation of STAT3, thereby reducing the survival rate of EBV-positive cells and cytokine production in CAEBV patients (110) (Table 2).
PD-1 expression can be detected in 30–60% of PTCL/NOS cases (111). In a single arm multicenter phase 2 study completed in China, all R/R PTCL patients received at least one dose of geptanolimab. Of the 89 patients with FAS, 40.4% achieved ORR, and 14.6% achieved CR. Patients with PD-L1 expression ≥ 50% benefited more from treatment (68). A large number of trials of PD-1 inhibitors alone or in combination with other drugs in PTCL are still ongoing (Table 3).
CTLs
Greater than 90% of cases of natural killer (NK)/T cell NHL, nasal type, are related to latent type II EBV. Tumour cells can express the weakly immunogenic EBV antigens LMP1, LMP2 and EBNA1. The stimulation of cytotoxic T lymphocytes (CTLs) targeting LMP1 and LMP2 showed efficacy in EBV+ NKTCL. In a clinical trial of LMP-CTLs in 52 EBV-associated lymphomas in the United States, 5 of 11 NKTCL patients received CTLs as consolidation treatment after primary radiotherapy or autologous stem cell transplantation. CR was achieved for 2–6 years, 8 patients survived for at least 2 years, and the longest period of CR was 6 years. In half of the patients, EBV levels could not be detected in plasma during CR (71). One patient with refractory NKTCL after autologous stem cell transplantation also remained in CR for 2 years after CTL infusion. Cho and colleagues studied LMP-CTL treatment in 8 patients with local disease and 2 patients with advanced NKTCL. All patients achieved CR within 4 years, with OS and PFS rates of 100% and 90%, respectively (72). However, since the 5-year survival rate of radiotherapy and chemotherapy can be as high as 90%, it is not clear whether patients with early disease truly benefit from CTL infusion. In conclusion, these trials represent significant advances in NKTCL and EBV-associated lymphoma. The role of CTL treatment in maintaining local disease after first-line treatment remains to be clarified. Longer follow-up and larger studies are needed to confirm these results. At present, there is no phase III clinical trial to guide clinical treatment.
Epigenetic Therapy
Histone Deacetylase Inhibitors
Reactivation of the EBV lytic cycle can enable cytotoxic antiviral drugs to achieve a specific killing effect on EBV-positive cells. These types of therapy include chemical lytic inducers and nucleoside analogue antiviral prodrugs. Studies have shown that HDAC inhibitors can reactivate the lytic cycle and lead to enhanced apoptosis of NPC and gastric cancer cells (112–114). HDAC inhibitors are divided into three categories according to their chemical structure: hydroxamate, cyclic peptide and benzamide. Suberoylanilide hydroxamic acid (SAHA) induces apoptosis and/or cell cycle arrest in some T and NK cell lines. SAHA also inhibited tumour progression and metastasis in a mouse xenograft model, demonstrating that it can inhibit EBV-related T and NK cell lymphoma (73). The cyclic peptide romidepsin has been studied by Bertrand Coiffier et al., who been found that romidepsin monotherapy can induce a complete and lasting response in patients with recurrent or refractory PTCL of all major subtypes, regardless of the number or type of previous treatments, and has controllable toxicity (74). At present, SAHA and romidepsin have been approved by the FDA for the treatment of many types of malignant tumours, such as peripheral and cutaneous T-cell lymphomas (115). Both drugs can induce an EBV lysis cycle at acceptable concentrations in patient plasma (113, 116). Chidamide is a novel oral benzamide HDAC inhibitor. In a phase II study, Y. Shi et al. evaluated the efficacy and safety of chidamide in recurrent or refractory peripheral T-cell lymphoma (PTCL) in a Chinese population and found that it has significant single-agent activity and controllable toxicity (75). Seventy-nine patients were enrolled, and an ORR of 28% was reported; 14% of the patients achieved a CR/CRu, and the OS was 21.4 months. Among these patients, the effects were more pronounced and the response was more durable in AITL patients. Most adverse events were limited to grades 1–2. Based on the results of this critical trial, CFDA approved chidamide for this indication in December 2014. However, it should be noted that HDAC, especially romidepsin, may lead to serious adverse events based on the mechanism of EBV cleavage recirculation activation. In an open label prospective pilot study, SJ Kim et al. found that three of the five NKTCL patients treated with romidepsin had a rapid increase in EBV DNA titer in their blood and an increase in liver enzymes and bilirubin levels (117). Therefore, patients with NKTCL should avoid using romidepsin, but patients with recurrent refractory diseases without other treatment options can consider the combination of two or more research drugs with strong biological principles. Joo Hyun Kim et al. conducted high-throughput screening of FDA approved drugs and tested them in EBV positive cell lines. It was found that phosphodiesterase 5 (PDE5) inhibitors, such as sildenafil, seem to be non-toxic and effective inhibitors of romidepsin induced EBV reactivation (118). A large number of clinical trials of HDAC inhibitors combined with other drugs are ongoing (Table 3).
Other Approaches
Proteasome Inhibitors
Proteasome inhibitors have been shown to inhibit cell growth and promote cell death in a variety of cancers. Bortezomib is the first proteasome inhibitor approved by the FDA in 2003 for the treatment of multiple myeloma (MM) and mantle cell lymphoma (119). The manipulation of normal ubiquitin proteasome system function by EBV is vital for virus replication and the survival of virus-infected cells. EBNA-1, LMP-2A and LMP-1 inhibit proteasome-mediated degradation to maintain virus latency, while bortezomib can reactivate the EBV lytic cycle in EBV-related BL cells (120). Induction of the EBV lytic cycle can activate the radioisotope [125I]2’-fluoro-2’-deoxy-β-D-5-iodouracil-arabinofuranoside, selectively inhibiting the growth of BL xenografts in severe combined immunodeficiency (SCID) mice (121). Granato M et al. found that bortezomib activates endoplasmic reticulum (ER) stress and that C/EBP-β, C-Jun N-terminal kinase (JNK) and autophagy mediate the transformation of a latent to a lytic EBV infection. It is more difficult to induce the EBV lysis cycle in lymphoid cells than in epithelial cells, and this effect is limited to BL cells (122). In addition to reinducing the lysis cycle, bortezomib can affect the apoptosis of EBV-related malignant tumour cells. The latent EBV membrane protein LMP-1 has been shown to activate the NF-κB pathway in BL and NPC, and this pathway has strong tumorigenic activity and may contribute to resistance to apoptosis inducers (123, 124). Bortezomib can protect the inhibitory protein IκBα and block NF-κB activation, leading to malignant cell apoptosis (125–128). It has been reported that bortezomib can induce the apoptosis of NK lymphoma/leukaemia cells, and this result was verified in vivo (76, 77, 129). In a phase I study, bortezomib combined with CHOP was administered to patients with advanced invasive T-cell lymphoma, and the ORR was 61.5%. Pier Luigi Zinzani et al. obtained a 67% ORR in patients with cutaneous T-cell lymphoma and invasive T/NK cell lymphoma treated with bortezomib monotherapy. In a study involving 6 NKTCL patients, the ORR after treatment with the B-GIFOX (bortezomib, gemcitabine, oxaliplatin and ifosfamide) regimen was 42.8% (78).
Selective Nuclear Export Protein Inhibitors
XPO1 is a nuclear export protein responsible for transporting biological macromolecules from the nucleus, including tumour suppressor proteins (TSPs) (e.g. p53, p73, FOXO3a, and IκB), growth regulators (e.g., glucocorticoid receptors), and oncogenic proteins (e.g., mRNA, c-myc, cyclin D, Bcl-2, and Bcl-6). In healthy cells, this process is strictly regulated to maintain an appropriate balance between cell growth and apoptosis (130). Studies have found that high levels of XPO1 are associated with poor clinical prognosis of multiple myeloma, DLBCL, glioblastoma and other diseases (130–132). As a linker protein, the EBV protein SM is involved in the nuclear output of mRNA encoding the soluble EBV gene. XPO1 inhibitors can covalently bind to XPO1 active sites and inhibit the output of corresponding mRNAs to the cytoplasm for translation, thereby effectively inhibiting EBV replication and virus transmission (133). Based on the mechanism of action of XPO1 inhibitors, they have broad application prospects in EBV-LPD and are under clinical investigation. A double-blind, placebo-controlled, phase I study of ATG-527 (KPT-335, verdinexor) for the treatment of CAEBV is ongoing; the aim of this study is to evaluate the safety, tolerability, pharmacokinetics and overall treatment response of different dose levels of ATG-527 in patients with CAEBV.
Exosomes
Exosomes are small membrane-bound vesicles secreted by cells. These extracellular vesicles (EVs) carry a wide range of molecules and affect intercellular communication, which contributes to the pathogenesis of various diseases and infections (134, 135). There is considerable evidence that EBV-related exosomes specifically package a variety of viral components that may promote EBV infection (such as LMP-1, lmp-2a, EBER, viral RNA, and miRNA) (136–139), which may help EBV establish the surrounding tumour microenvironment to promote tumour growth and survival. In addition, Keryer-Bibens C et al. found that galectin-9-containing exosomes inhibited EBV-specific T cell proliferation and induced apoptosis (136). Therefore, blocking these exosomes can restore immune surveillance while resisting tumours. Exosomes have been designated as an effective target for cancer treatment, but they need to be further explored.
Conclusion
In general, there has been great progress in our understanding and treatment of EBV-LPDs in recent years from traditional chemotherapy, changes in immunosuppressants and HSCT to immunotherapy, gene therapy and epigenetic therapy stemming from discoveries of signaling pathways and virus latency and lysis cycles; together, this improved understanding will lead to the development of better treatment options with fewer side effects. Among such potential treatments, proteasome inhibitors, HDAC inhibitors and JAK inhibitors have been tested in vitro and in vivo using xenotransplantation models. The effects of rituximab, nivolumab, pembrolizumab, daratumumab, bortezomib and CTLs have been confirmed in clinical trials. However, we must appreciate that most of the research results are limited to specific groups. For heterogeneous populations of EBV-LPDs, immunotherapy targets, checkpoint inhibitors, CTLs, small molecule targets and even gene therapy are still elusive. We hope that as the data and science related to these methods mature, they will provide alternative treatments as both monotherapy and combination therapy.
Author Contributions
KL and TY design, analysis and draft the manuscript. MY, ZC: supervision, funding acquisition, writing–review and editing. YZ: methodology, and writing–review and editing. FL: design, supervision, funding acquisition, methodology, project administration, writing–review and editing. All authors contributed to the article and approved the submitted version.
Funding
This work was financially supported by grants from the National Natural Science Foundation of China (81960043, 82160043), Natural Science Foundation of Jiangxi Province (20192ACB20030), Science and Technology Innovation Base Construction Project of Jiangxi Province (20211ZDG02006, 20212BCG74001).
Conflict of Interest
The authors declare that the research was conducted in the absence of any commercial or financial relationships that could be construed as a potential conflict of interest.
Publisher’s Note
All claims expressed in this article are solely those of the authors and do not necessarily represent those of their affiliated organizations, or those of the publisher, the editors and the reviewers. Any product that may be evaluated in this article, or claim that may be made by its manufacturer, is not guaranteed or endorsed by the publisher.
References
1. Kimura H, Fujiwara S. Overview of EBV-Associated T/NK-Cell Lymphoproliferative Diseases. Front Pediatr (2018) 6:417:417. doi: 10.3389/fped.2018.00417
2. Hui KF, Chan TF, Yang W, Shen JJ, Lam KP, Kwok H, et al. High Risk Epstein-Barr Virus Variants Characterized by Distinct Polymorphisms in the EBER Locus are Strongly Associated With Nasopharyngeal Carcinoma. Int J Cancer (2019) 144(12):3031–42. doi: 10.1002/ijc.32049
3. Hui KF, Yiu SPT, Tam KP, Chiang AKS. Viral-Targeted Strategies Against EBV-Associated Lymphoproliferative Diseases. Front Oncol (2019) 9:81:81. doi: 10.3389/fonc.2019.00081
4. Israel BF, Kenney SC. Virally Targeted Therapies for EBV-Associated Malignancies. Oncogene (2003) 22(33):5122–30. doi: 10.1038/sj.onc.1206548
5. Kis LL, Takahara M, Nagy N, Klein G, Klein E. Cytokine Mediated Induction of the Major Epstein-Barr Virus (EBV)-Encoded Transforming Protein, LMP-1. Immunol Lett (2006) 104(1-2):83–8. doi: 10.1016/j.imlet.2005.11.003
6. Rowe M, Kelly GL, Bell AI, Rickinson AB. Burkitt's Lymphoma: The Rosetta Stone Deciphering Epstein-Barr Virus Biology. Semin Cancer Biol (2009) 19(6):377–88. doi: 10.1016/j.semcancer.2009.07.004
7. Kis LL, Takahara M, Nagy N, Klein G, Klein E. IL-10 can Induce the Expression of EBV-Encoded Latent Membrane Protein-1 (LMP-1) in the Absence of EBNA-2 in B Lymphocytes and in Burkitt Lymphoma- and NK Lymphoma-Derived Cell Lines. Blood (2006) 107(7):2928–35. doi: 10.1182/blood-2005-06-2569
8. Carbone A, Gloghini A, Dotti G. EBV-Associated Lymphoproliferative Disorders: Classification and Treatment. Oncol (2008) 13(5):577–85. doi: 10.1634/theoncologist.2008-0036
9. Kieff E, Rickinson AB. Epstein Barr virus and its replication. In: Fields BN, Knipe DM, Howley P, et al editors. Fields Virology. Philadelphia, PA: Lippincott–Raven (2001). p. 2511–75
10. Mainou BA, Everly DN, Raab-Traub N. Unique Signaling Properties of CTAR1 in LMP1-Mediated Transformation. J Virol (2007) 81(18):45–. doi: 10.1128/JVI.01001-07
11. Thorley-Lawson DA, Gross A. Persistence of the Epstein-Barr Virus and the Origins of Associated Lymphomas. New Engl J Med (2004) 350(13):1328–37. doi: 10.1056/NEJMra032015
12. Epstein M, Achong B, Barr Y. Virus Particles in Cultured Lymphoblasts from Burkitt's Lymphoma. Lancet (1964) 1(7335):702–3. doi: 10.1016/s0140-6736(64)91524-7
13. Jacobson C, Lacasce A. How I Treat Burkitt Lymphoma in Adults. Blood (2014) 124(19):2913–20. doi: 10.1182/blood-2014-06-538504
14. Molyneux EM, Rochford R, Griffin B, Newton R, Bailey S. Burkitt's Lymphoma. Lancet (2012) 379(9822):1234–44. doi: 10.1016/S0140-6736(11)61177-X
15. Magrath I, Adde M, Shad A, Venzon D, Seibel N, Gootenberg J, et al. Adults and Children With Small non-Cleaved-Cell Lymphoma Have a Similar Excellent Outcome When Treated With the Same Chemotherapy Regimen. J Clin Oncol (1996) 14(3):925–34. doi: 10.1200/jco.1996.14.3.925
16. Barnes JA, Lacasce AS, Feng Y, Toomey CE, Neuberg D, Michaelson JS, et al. Evaluation of the Addition of Rituximab to CODOX-M/IVAC for Burkitt's Lymphoma: A Retrospective Analysis. Ann Oncol Off J Eur Soc Med Oncol (2011) 22(8):1859–64. doi: 10.1093/annonc/mdq677
17. Thomas DA, Faderl S, O'Brien S, Bueso-Ramos C, Cortes J, Garcia-Manero G, et al. Chemoimmunotherapy With Hyper-CVAD Plus Rituximab for the Treatment of Adult Burkitt and Burkitt-Type Lymphoma or Acute Lymphoblastic Leukemia. Cancer (2006) 106(7):1569–80. doi: 10.1002/cncr.21776
18. Rizzieri DA, Johnson JL, Byrd JC, Lozanski G, Blum KA, Powell BL, et al. Improved Efficacy Using Rituximab and Brief Duration, High Intensity Chemotherapy With Filgrastim Support for Burkitt or Aggressive Lymphomas: Cancer and Leukemia Group B Study 10 002. Br J Haematol (2014) 165(1):102–11. doi: 10.1111/bjh.12736
19. Rizzieri DA, Johnson JL, Niedzwiecki D, Lee EJ, Vardiman JW, Powell BL, et al. Intensive Chemotherapy With and Without Cranial Radiation for Burkitt Leukemia and Lymphoma: Final Results of Cancer and Leukemia Group B Study 9251. Cancer (2010) 100(7):1438–48. doi: 10.1002/cncr.20143
20. Dunleavy K, Pittaluga S, Shovlin M, Steinberg SM, Cole D, Grant C, et al. Low-Intensity Therapy in Adults With Burkitt's Lymphoma. N Engl J Med (2013) 369(20):1915–25. doi: 10.1056/NEJMoa1308392
21. van der Velden WJFM, Mori T, Stevens W, Haan AD, Stelma FF, Blijlevens N, et al. Reduced PTLD-Related Mortality in Patients Experiencing EBV Infection Following Allo-SCT After the Introduction of a Protocol Incorporating Pre-Emptive Rituximab. Bone Marrow Transplant (2013) 48(11):1465–71. doi: 10.1038/bmt.2013.84
22. Mika T, Strate K, Ladigan S, Aigner C, Schroers R. Refractory Epstein-Barr Virus (EBV)-Related Post-Transplant Lymphoproliferative Disease: Cure by Combined Brentuximab Vedotin and Allogeneic EBV-Specific T-Lymphocytes. Front Med (2019) 6:295. doi: 10.3389/fmed.2019.00295
23. Strunz PP, Schmalzing M, Heidemeier A, Rasche L, Kortüm KM. Response to Daratumumab in Rituximab-Resistant EBV-Associated PTLD Following Allogenic Stem Cell Transplantation From an EBV Seronegative Donor. Leukemia Lymphoma (2019) 60(14):1–4. doi: 10.1080/10428194.2019.1636981
24. Younes A, Santoro A, Shipp M, Zinzani PL, Timmerman JM, Ansell S, et al. Nivolumab for Classical Hodgkin's Lymphoma After Failure of Both Autologous Stem-Cell Transplantation and Brentuximab Vedotin: A Multicentre, Multicohort, Single-Arm Phase 2 Trial. Lancet Oncol (2016) 17(9):1283–94. doi: 10.1016/S1470-2045(16)30167-X
25. Herrera AF, Chen RW, Palmer J, Tsai NC, Lee HJ. PET-Adapted Nivolumab or Nivolumab Plus ICE As First Salvage Therapy in Relapsed or Refractory Hodgkin Lymphoma. Blood (2019) 134(Supplement_1):239–. doi: 10.1182/blood-2019-123162
26. Chen R, Zinzani PL, Fanale MA, Armand P, Moskowitz CH. Phase II Study of the Efficacy and Safety of Pembrolizumab for Relapsed/Refractory Classic Hodgkin Lymphoma. J Clin Oncol (2017) 35(19):2125–32. doi: 10.1200/JCO.2016.72.1316
27. Pys A, Phs C, Pys D, Pwj E, Pxs F, Pwq G, et al. Safety and Activity of Sintilimab in Patients With Relapsed or Refractory Classical Hodgkin Lymphoma (ORIENT-1): A Multicentre, Single-Arm, Phase 2 Trial. Lancet Haematol (2019) 6(1):e12–e9. doi: 10.1016/S2352-3026(18)30192-3
28. Song Y, Gao Q, Zhang H, Fan L, Zhou J, Zou D, et al. Treatment of Relapsed or Refractory Classical Hodgkin Lymphoma With the Anti-PD-1, Tislelizumab: Results of a Phase 2, Single-Arm, Multicenter Study. Leukemia (2020) 34(2):533–42. doi: 10.1038/s41375-019-0545-2
29. Song Y, Wu J, Chen X, Lin TY, Single-Arm ZJA. Multicenter, Phase II Study of Camrelizumab in Relapsed or Refractory Classical Hodgkin Lymphoma. Clin Cancer Res (2019) 25(18):7363–9. doi: 10.1158/1078-0432.CCR-19-1680
30. Moskowitz AJ, Advani RH, Bartlett NL, Vose JM, Herrera AF. Brentuximab Vedotin and Nivolumab for Relapsed or Refractory Classic Hodgkin Lymphoma: Long-Term Follow-Up Results From the Single-Arm Phase 1/2 Study. Blood (2019) 134(Supplement_1):238–. doi: 10.1182/blood-2019-122576
31. Herrera AF, Chen L, Nieto Y, Holmberg L, Feldman TA. Consolidation With Nivolumab and Brentuximab Vedotin After Autologous Hematopoietic Cell Transplantation in Patients With High-Risk Hodgkin Lymphoma. Blood (2020) 136(Supplement 1):19–20. doi: 10.1182/blood-2020-136384
32. Cheson BD, Bartlett NL, LaPlant B, Lee HJ, Advani RJ, Christian B, et al. Brentuximab Vedotin Plus Nivolumab as First-Line Therapy in Older or Chemotherapy-Ineligible Patients With Hodgkin Lymphoma (ACCRU): A Multicentre, Single-Arm, Phase 2 Trial. (2020) 7(11):e808–15. doi: 10.1016/S2352-3026(20)30275-1
33. Kassa C, Reményi P, Sinkó J, Kállay K, Kertész G, Kriván G. Successful Nivolumab Therapy in an Allogeneic Stem Cell Transplant Child With Post-Transplant Lymphoproliferative Disorder. Pediatr Transplantation (2018) 22(8):e13302. doi: 10.1111/petr.13302
34. Gunes AK, Demir I, Pehlivan M. Classical Hodgkin Lymphoma-Like Post-Transplant Lymphoproliferative Disease After Allogeneic Stem Cell Transplantation for Primary Myelofibrosis is Successfully Treated With Nivolumab: A Case Report. J Oncol Pharm Pract (2021) 27(2):509–12. doi: 10.1177/1078155220946462
35. Chen BJ, Chapuy B, Ouyang J, Sun HH, Roemer M, Xu ML, et al. PD-L1 Expression Is Characteristic of a Subset of Aggressive B-Cell Lymphomas and Virus-Associated Malignancies. Clin Cancer Res (2013) 19(13):3462–73. doi: 10.1158/1078-0432.CCR-13-0855
36. Ouyang J, Juszczynski P, Rodig SJ, Green MR, Shipp MA. Viral Induction and Targeted Inhibition of Galectin-1 in EBV+ Posttransplant Lymphoproliferative Disorders. Blood (2009) 117(16):4315–22. doi: 10.1182/blood-2010-11-320481
37. Roskrow MA, Suzuki N, Gan YJ, Sixbey JW, Rooney CM. Epstein-Barr Virus (EBV)-Specific Cytotoxic T Lymphocytes for the Treatment of Patients With EBV-Positive Relapsed Hodgkin's Disease. Blood (1998) 91(8):2925–34. doi: 10.1182/blood.V91.8.2925.2925_2925_2934
38. Gopal S, Gross TG. How I Treat Burkitt Lymphoma in Children, Adolescents, and Young Adults in Sub-Saharan Africa. Blood (2018) 132(3):254–63. doi: 10.1182/blood-2018-04-844472
39. Wu TC, Mann RB, Charache P, Hayward SD, Staal S, Lambe BC, et al. Detection of EBV Gene Expression in Reed-Sternberg Cells of Hodgkin's Disease. Int J Cancer (2010) 46(5):801–4. doi: 10.1002/ijc.2910460509
40. Pallesen G, Hamilton-Dutoit SJ, Rowe M, Young LS. Expression of Epstein-Barr Virus Latent Gene Products in Tumour Cells of Hodgkin's Disease. Lancet (1991) 337(8737):320. doi: 10.1016/0140-6736(91)90943-J
41. Carbone A, Gloghini A, Larocca LM, Antinori A, Falini B, Tirelli U, et al. Human Immunodeficiency Virus-Associated Hodgkin's Disease Derives From Post-Germinal Center B Cells. Blood (1999) 93(7):2319–26. doi: 10.1182/blood.V93.7.2319
42. Song Y, Guo Y, Huang H, Li W, Ke X, Feng J, et al. Phase II Single-Arm Study of Brentuximab Vedotin in Chinese Patients With Relapsed/Refractory Classical Hodgkin Lymphoma or Systemic Anaplastic Large Cell Lymphoma. Expert Rev Hematol (2021) 14(9):867–75. doi: 10.1080/17474086.2021.1942831
43. Bartlett N, Younes A, Carabasi M, Forero A, Rosenblatt J, Leonard J, et al. A Phase 1 Multidose Study of SGN-30 Immunotherapy in Patients With Refractory or Recurrent CD30+ Hematologic Malignancies. Blood (2008) 111(4):1848–54. doi: 10.1182/blood-2007-07-099317
44. Oyama T, Ichimura K, Suzuki R, Suzumiya J, Ohshima K, Yatabe Y, et al. Senile EBV+ B-Cell Lymphoproliferative Disorders: A Clinicopathologic Study of 22 Patients. Am J Surg Pathol (2003) 27(1):16–26. doi: 10.1097/00000478-200301000-00003
45. Nan FF, Zhang L, Li L, Li X, Sun ZC, Zhang XD, et al. Clinical Features and Survival Impact of EBV-Positive Diffuse Large B-Cell Lymphoma With Different Age Cutoffs. Eur Rev Med Pharmacol Sci (2020) 24(17):8947–56. doi: 10.26355/eurrev_202001_22836
46. Lu TX, Liang JH, Miao Y, Fan L, Wang L, Qu XY, et al. Epstein-Barr Virus Positive Diffuse Large B-Cell Lymphoma Predict Poor Outcome, Regardless of the Age. Sci Rep (2015) 5(1):12168. doi: 10.1038/srep12168
47. Beltran BE, Morales D, Qui?Ones P, Medeiros LJ, Miranda RN, Castillo JJ. EBV-Positive Diffuse Large B-Cell Lymphoma in Young Immunocompetent Individuals. Clin Lymphoma Myeloma Leukemia (2011) 11(6):512–6. doi: 10.1016/j.clml.2011.07.003
48. Cohen M, Narbaitz M, Metrebian F, Matteo ED, Preciado MV, Chabay PA. Epstein-Barr Virus-Positive Diffuse Large B-Cell Lymphoma Association is Not Only Restricted to Elderly Patients. Int J Cancer (2015) 135(12):2816–24. doi: 10.1002/ijc.28942
49. Cohen M, Matteo ED, Narbaitz M, Carreño FA, Preciado MV, Chabay PA. Epstein-Barr Virus Presence in Pediatric Diffuse Large B-Cell Lymphoma Reveals a Particular Association and Latency Patterns: Analysis of Viral Role in Tumor Microenvironment. Int J Cancer (2012) 132(7):1572–80. doi: 10.1002/ijc.27845
50. Uccini S, Al-Jadiry MF, Scarpino S, Ferraro D, Alsaadawi AR, Al-Darraji AF, et al. Epstein-Barr Virus–Positive Diffuse Large B-Cell Lymphoma in Children: A Disease Reminiscent of Epstein-Barr Virus–Positive Diffuse Large B-Cell Lymphoma of the Elderly. Hum Pathol (2015) 46(5):716–24. doi: 10.1016/j.humpath.2015.01.011
51. Hong JY, Yoon DH, Suh C, Huh J, Do IG, Sohn I, et al. EBV-Positive Diffuse Large B-Cell Lymphoma in Young Adults: Is This a Distinct Disease Entity? Ann Oncol (2015) 26(3):548–55. doi: 10.1093/annonc/mdu556
52. Swerdlow SH, Campo E, Pileri SA, Harris NL, Stein H, Siebert R, et al. The 2016 Revision of the World Health Organization Classification of Lymphoid Neoplasms. Blood (2016) 127(20):2375–90. doi: 10.1182/blood-2016-01-643569
53. Park S, Lee J, Ko YH, Han A, Jun HJ, Lee SC. The Impact of Epstein-Barr Virus Status on Clinical Outcome in Diffuse Large B-Cell Lymphoma. Blood (2007) 110(3):972–8. doi: 10.1182/blood-2007-01-067769
54. Crombie JL, Lacasce AS. Epstein Barr Virus Associated B-Cell Lymphomas and Iatrogenic Lymphoproliferative Disorders. Front Oncol (2019) 9:109–. doi: 10.3389/fonc.2019.00109
55. Yamaguchi M, Kwong YL, Kim WS, Maeda Y, Suzuki R. Phase II Study of SMILE Chemotherapy for Newly Diagnosed Stage IV, Relapsed, or Refractory Extranodal Natural Killer (Nk)/T-Cell Lymphoma, Nasal Type: The NK-Cell Tumor Study Group Study. J Clin Oncol (2011) 29(33):4410–6. doi: 10.1200/JCO.2011.35.6287
56. Li X, Cui Y, Sun Z, Zhang L, Li L, Wang X, et al. DDGP Versus SMILE in Newly Diagnosed Advanced Natural Killer/T-Cell Lymphoma: A Randomized Controlled, Multicenter, Open-Label Study in China. Clin Cancer Res (2016), 5223–8. doi: 10.1158/1078-0432.CCR-16-0153
57. Wang JH, Wang L, Liu CC, Xia ZJ, Huang HQ, Lin TY, et al. Efficacy of Combined Gemcitabine, Oxaliplatin and Pegaspargase (P-Gemox Regimen) in Patients With Newly Diagnosed Advancedstage or Relapsed/Refractory Extranodal NK/T-Cell Lymphoma. Oncotarget (2016) 7(20). doi: 10.18632/oncotarget.8647
58. Yonese I, Sakashita C, Imadome KI, Kobayashi T, Yamamoto M, Sawada A, et al. Nationwide Survey of Systemic Chronic Active EBV Infection in Japan in Accordance With the New WHO Classification. Blood Adv (2020) 4(13):2918–26. doi: 10.1182/bloodadvances.2020001451
59. Lv K, Li X, Yu H, Chen X, Wu X. Selection of New Immunotherapy Targets for NK/T Cell Lymphoma. Am J Trans Res (2020) 12(11):7034–47.
60. Huang H, Zhu J, Yao M, Kim TM, Yoon DH, Cho SG, et al. Daratumumab Monotherapy for Patients With Relapsed or Refractory Natural Killer/T-Cell Lymphoma, Nasal Type: An Open-Label, Single-Arm, Multicenter, Phase 2 Study. J Hematol Oncol (2021) 14(1):25. doi: 10.1186/s13045-020-01020-y
61. Kyung KH, Mi MS, Hoon MJ, Eun PJ, Seonggyu B, Seog KW. Complete Remission in CD30-Positive Refractory Extranodal NK/T-Cell Lymphoma With Brentuximab Vedotin. Blood Res (2015) 50(4):254–6. doi: 10.5045/br.2015.50.4.254
62. Poon LM, Kwong YL. Complete Remission of Refractory Disseminated NK/T Cell Lymphoma With Brentuximab Vedotin and Bendamustine. Ann Hematol (2016) 95(5):847–9. doi: 10.1007/s00277-016-2627-9
63. Jaccard A, Hermine O. A Major Turning Point in NK/T-Cell Lymphoma? Blood J Am Soc Hematol (2017). doi: 10.1182/blood-2017-03-769075
64. Li X, Cheng Y, Zhang M, Yan J, Li L, Fu X, et al. Activity of Pembrolizumab in Relapsed/Refractory NK/T-Cell Lymphoma. J Hematol Oncol (2018) 11(1):15. doi: 10.1186/s13045-018-0559-7
65. Chan T, Li J, Loong F, Khong PL, Tse E, Kwong YL. PD1 Blockade With Low-Dose Nivolumab in NK/T Cell Lymphoma Failing L-Asparaginase: Efficacy and Safety. Ann Hematol (2017). doi: 10.1007/s00277-017-3127-2
66. Tao R, Fan L, Song Y, Hu Y, Zhang W, Wang Y, et al. Sintilimab for Relapsed/Refractory Extranodal NK/T Cell Lymphoma: A Multicenter, Single-Arm, Phase 2 Trial (ORIENT-4). Signal Transduction Targeted Ther (2021) 6(1):365. doi: 10.1038/s41392-021-00768-0
67. Kim SJ, Lim JQ, Laurensia Y, Cho J, Yoon SE, Lee JY, et al. Avelumab for the Treatment of Relapsed or Refractory Extranodal NK/T-Cell Lymphoma: An Open-Label Phase 2 Study. Blood (2020) 136(24):2754–63. doi: 10.1182/blood.2020007247
68. Shi Y, Wu J, Wang Z, Zhang L, Wang Z, Zhang M, et al. Efficacy and Safety of Geptanolimab (GB226) for Relapsed or Refractory Peripheral T Cell Lymphoma: An Open-Label Phase 2 Study (Gxplore-002). J Hematol Oncol (2021) 14(1):12. doi: 10.1186/s13045-021-01033-1
69. Nairisma?Gi ML, Gerritsen ME, Li ZM, Wijaya GC, Chia B, Laurensia Y, et al. Oncogenic Activation of JAK3-STAT Signaling Confers Clinical Sensitivity to PRN371, a Novel Selective and Potent JAK3 Inhibitor, in Natural Killer/T-Cell Lymphoma. Leukemia (2018). doi: 10.1038/s41375-017-0004-x
70. Ando S, Kawada JI, Watanabe T, Suzuki M, Kimura H. Tofacitinib Induces G1 Cell-Cycle Arrest and Inhibits Tumor Growth in Epstein-Barr Virus-Associated T and Natural Killer Cell Lymphoma Cells. Oncotarget (2016) 7(47):76793–805. doi: 10.18632/oncotarget.12529
71. Bollard CM, Gottschalk S, Torrano V, Diouf O, Rooney CM. Sustained Complete Responses in Patients With Lymphoma Receiving Autologous Cytotoxic T Lymphocytes Targeting Epstein-Barr Virus Latent Membrane Proteins. J Clin Oncol (2013) 32(8):798. doi: 10.1200/JCO.2013.51.5304
72. Cho SG, Kim N, Sohn HJ, Lee SK, Oh ST, Lee HJ, et al. Long-Term Outcome of Extranodal Nk/T Cell Lymphoma Patients Treated With Postremission Therapy Using EBV LMP1 and LMP2a-Specific CTLs. Mol Ther (2015) (8):1401–9. doi: 10.1038/mt.2015.91
73. Siddiquey MNA, Nakagawa H, Iwata S, Kanazawa T, Suzuki M, Imadome KI, et al. Anti-Tumor Effects of Suberoylanilide Hydroxamic Acid on Epstein–Barr Virus-Associated T Cell and Natural Killer Cell Lymphoma. Cancer Sci (2014) 105(6):713–22. doi: 10.1111/cas.12418
74. Coiffier B, Pro B, Prince HM, Foss F, Sokol L. Results From a Pivotal, Open-Label, Phase II Study of Romidepsin in Relapsed or Refractory Peripheral T-Cell Lymphoma After Prior Systemic Therapy. J Clin Oncol Off J Am Soc Clin Oncol (2012) 30(6):631. doi: 10.1200/JCO.2011.37.4223
75. Shi Y, Dong M, Hong X, Zhang W, Feng J, Zhu J, et al. Results From a Multicenter, Open-Label, Pivotal Phase II Study of Chidamide in Relapsed or Refractory Peripheral T-Cell Lymphoma. Ann Oncol (2015) 26(8):1766. doi: 10.1093/annonc/mdv237
76. Lee S, Kang B-Y, Ryoo H. Phase I Study of Proteasome Inhibitor Bortezomib Plus CHOP in Patients With Advanced, Aggressive T-Cell or NK/T-Cell Lymphoma. Ann Oncol (2008). doi: 10.1093/annonc/mdn431
77. Zinzani PL, Musuraca G, Tani M, Stefoni V, Baccarani M. Phase II Trial of Proteasome Inhibitor Bortezomib in Patients With Relapsed or Refractory Cutaneous T-Cell Lymphoma. J Clin Oncol (2007) 25(27):4293–7. doi: 10.1200/JCO.2007.11.4207
78. Farid M, Yau YW, Tay K, Quek R, Tao M, Koo GC, et al. A Promising New Regimen for the Treatment of Advanced Extranodal NK/T Cell Lymphoma. Acta Oncol (2011) 50(4):589–90. doi: 10.3109/0284186X.2010.516272
79. McKhann CF. Primary Malignancy in Patients Undergoing Immunosuppression for Renal Transplantation. Transplantation (1969) 8(2):209–12 doi: 10.1097/00007890-196908000-00033
80. Penn I, Hammond W, Brettschneider L, Starzl TE. Malignant Lymphomas in Transplantation Patients. Transplant Proc (1969) 1(1):106–12.
81. Thompson MP. Epstein-Barr Virus and Cancer. Clin Cancer Res (2004) 10(3):803–21. doi: 10.1158/1078-0432.CCR-0670-3
82. Dolcetti R. B Lymphocytes and Epstein-Barr Virus: The Lesson of Post-Transplant Lymphoproliferative Disorders. Autoimmun Rev (2008) 7(2):96–101. doi: 10.1016/j.autrev.2007.02.012
83. Llaurador G, Mclaughlin L, Wistinghausen B. Management of Post-Transplant Lymphoproliferative Disorders. Curr Opin Pediatr (2017) 29. doi: 10.1097/MOP.0000000000000445
84. Green MR, Rodig S, Juszczynski P, Ouyang J, Shipp MA. Constitutive AP-1 Activity and EBV Infection Induce PD-L1 in Hodgkin Lymphomas and Posttransplant Lymphoproliferative Disorders: Implications for Targeted Therapy. Clin Cancer Res (2012) 18(6):1611–8. doi: 10.1158/1078-0432.CCR-11-1942
85. Chen R, Zinzani P, Lee H, Armand P, Johnson N, Brice P, et al. Pembrolizumab in Relapsed or Refractory Hodgkin Lymphoma: 2-Year Follow-Up of KEYNOTE-087. Blood (2019) 134(14):1144–53. doi: 10.1182/blood.2019000324
86. Armand P, Chen YB, Redd RA, Joyce RM, Bsat J, Jeter E, et al. PD-1 Blockade With Pembrolizumab for Classical Hodgkin Lymphoma After Autologous Stem Cell Transplantation. Blood (2019) 134(1):22–9. doi: 10.1182/blood.2019000215
87. Herrera AF, Moskowitz AJ, Bartlett NL, Vose JM, Ramchandren R, Feldman TA, et al. Interim Results of Brentuximab Vedotin in Combination With Nivolumab in Patients With Relapsed or Refractory Hodgkin Lymphoma. Blood (2018) 131(11):1183–94. doi: 10.1182/blood-2017-10-811224
88. Armand P. Immune Checkpoint Blockade in Hematologic Malignancies. Blood (2015) 125(22):3393–400. doi: 10.1182/blood-2015-02-567453
89. Foster AE, Rooney CM. Improving T Cell Therapy for Cancer. Expert Opin Biol Ther (2006) 6(3):215–29. doi: 10.1517/14712598.6.3.215
90. Riddell, Watanabe K, Goodrich J, Li C, Agha M, Greenberg P. Restoration of Viral Immunity in Immunodeficient Humans by the Adoptive Transfer of T Cell Clones. Science (1992) 257(5067):238–41. doi: 10.1126/science.1352912
91. Heslop HE, Brenner MK, Rooney CM. Donor T Cells to Treat EBV-Associated Lymphoma. N Engl J Med (1994) 331(10):679–80. doi: 10.1056/NEJM199409083311017
92. Papadopoulos EB, Ladanyi M, Emanuel D, Mackinnon S, Boulad F, Carabasi MH, et al. Infusions of Donor Leukocytes to Treat Epstein-Barr Virus-Associated Lymphoproliferative Disorders After Allogeneic Bone Marrow Transplantation. N Engl J Med (1994) 330(17):1185–91. doi: 10.1056/NEJM199404283301703
93. Doubrovina E, Oflaz-Sozmen B, Prockop SE, Kernan NA, Abramson S, Teruya-Feldstein J, et al. Adoptive Immunotherapy With Unselected or EBV-Specific T Cells for Biopsy-Proven EBV+ Lymphomas After Allogeneic Hematopoietic Cell Transplantation. Blood (2012) 119(11):2644. doi: 10.1182/blood-2011-08-371971
94. Haque T, Wilkie GM, Jones MM, Higgins CD, Crawford DH. Allogeneic Cytotoxic T-Cell Therapy for EBV-Positive Posttransplantation Lymphoproliferative Disease: Results of a Phase 2 Multicenter Clinical Trial. Blood (2007) 110(4):1123–31. doi: 10.1182/blood-2006-12-063008
95. Poppema S, Potters M, Visser L, van den Berg AM. Immune Escape Mechanisms in Hodgkin's Disease. Ann Oncol (1998) 9(suppl 5):S21–S4. doi: 10.1093/annonc/9.suppl_5.S21
96. Franken M, Estabrooks A, Cavacini L, Sherburne B, Scadden DT. Epstein-Barr Virus-Driven Gene Therapy for EBV-Related Lymphomas. Nat Med (1997) 2(12):1379–82. doi: 10.1038/nm1296-1379
97. Li JH, Huang D, Sun BF, Zhang X, Liu FF. Efficacy of Ionizing Radiation Combined With Adenoviral P53 Therapy in EBV-Positive Nasopharyngeal Carcinoma. Int J Cancer (2015) 87(4):606–10. doi: 10.1002/1097-0215(20000815)87:4<606::AID-IJC23>3.0.CO;2-O
98. Li JH, Chia M, Shi W, Ngo D, Strathdee CA, Huang D, et al. Tumor-Targeted Gene Therapy for Nasopharyngeal Carcinoma. Cancer Res (2002) 62(1):171–8.
99. Arai A. Chronic Active Epstein–Barr Virus Infection: The Elucidation of the Pathophysiology and the Development of Therapeutic Methods. Microorganisms (2021) 9(1):180. doi: 10.3390/microorganisms9010180
100. Sawada A, Inoue M, Kawa K. How We Treat Chronic Active Epstein-Barr Virus Infection. Int J Hematol (2017) 105(4):406–18. doi: 10.1007/s12185-017-2192-6
101. Cohen J. Characterization and Treatment of Chronic Active Epstein-Barr Virus Disease: A 28-Year Experience in the United States. Blood (2011) 117(22):5835–49. doi: 10.1182/blood-2010-11-316745
102. Takayuki O, Yoshikazu H, Arai H, Inoue M, Kawa K. Blood Stem-Cell Transplantation for Chronic Active Epstein-Barr Virus With Lymphoproliferation. Lancet (2000) 356(9225):223–4. doi: 10.1016/S0140-6736(00)02488-0
103. Kawa K, Sawada A, Sato M, Okamura T, Sakata N, Kondo O, et al. Excellent Outcome of Allogeneic Hematopoietic SCT With Reduced-Intensity Conditioning for the Treatment of Chronic Active EBV Infection. Bone Marrow Transplantation (2011) 46(1):77–83. doi: 10.1038/bmt.2010.122
104. Kimura H, Ito Y, Kawabe S, Gotoh K, Nakamura S. EBV-Associated T/NK-Cell Lymphoproliferative Diseases in Nonimmunocompromised Hosts: Prospective Analysis of 108 Cases. Blood (2011) 119(3):673–86. doi: 10.1182/blood-2011-10-381921
105. Arai A, Sakashita C, Hirose C, Imadome KI, Yamamoto M, Jinta M, et al. Hematopoietic Stem Cell Transplantation for Adults With EBV-Positive T- or NK-Cell Lymphoproliferative Disorders: Efficacy and Predictive Markers. Bone Marrow Transplantation (2016) 51(6):879–82. doi: 10.1038/bmt.2016.3
106. Wang L, Wang H, Li PF, Lu Y, Xia ZJ, Huang HQ, et al. CD38 Expression Predicts Poor Prognosis and Might be a Potential Therapy Target in Extranodal NK/T Cell Lymphoma, Nasal Type. Ann Hematol (2015) 94(8):1381–8. doi: 10.1007/s00277-015-2359-2
107. Hari P, Raj R, Olteanu H. Targeting CD38 in Refractory Extranodal Natural Killer Cell-T-Cell Lymphoma. New Engl J Med (2016) 375(15):1501–2. doi: 10.1056/NEJMc1605684
108. Feng Y, Rao H, Lei Y, Huang Y, Fang W, Yu Z, et al. CD30 Expression in Extranodal Natural Killer/T-Cell Lymphoma, Nasal Type Among 622 Cases of Mature T-Cell and Natural Killer-Cell Lymphoma at a Single Institution in South China. Chin J Cancer (2017) 36(006):258–66. doi: 10.1186/s40880-017-0212-9
109. Reiss KA, Forde PM, Brahmer JR. Harnessing the Power of the Immune System via Blockade of PD-1 and PD-L1: A Promising New Anticancer Strategy. Immunotherapy (2014) 6(4):459–75. doi: 10.2217/imt.14.9
110. Onozawa E, Shibayama H, Takada H, Imadome KI, Aoki S, Yoshimori M, et al. STAT3 is Constitutively Activated in Chronic Active Epstein-Barr Virus Infection and can be a Therapeutic Target. Oncotarget (2018) 9(57):31077–89. doi: 10.18632/oncotarget.25780
111. Krishnan C, Warnke RA, Arber DA, Natkunam Y. PD-1 Expression in T-Cell Lymphomas and Reactive Lymphoid Entities: Potential Overlap in Staining Patterns Between Lymphoma and Viral Lymphadenitis. Am J Surg Pathol (2010) 34(2):178. doi: 10.1097/PAS.0b013e3181cc7e79
112. Hui KF, Chiang A. Suberoylanilide Hydroxamic Acid Induces Viral Lytic Cycle in Epstein-Barr Virus-Positive Epithelial Malignancies and Mediates Enhanced Cell Death. Int J Cancer (2010) 126(10):2479–89. doi: 10.1002/ijc.24945
113. Hui KF, Ho DN, Tsang CM, Middeldorp JM, Tsao G, Chiang A. Activation of Lytic Cycle of Epstein-Barr Virus by Suberoylanilide Hydroxamic Acid Leads to Apoptosis and Tumor Growth Suppression of Nasopharyngeal Carcinoma. Int J Cancer (2012) 131(8):1930–40. doi: 10.1002/ijc.27439
114. Kawanishi M. Epstein-Barr Virus Induces Fragmentation of Chromosomal DNA During Lytic Infection. J Virol (1993) 67(12):7654–8. doi: 10.1128/jvi.67.12.7654-7658.1993
115. Harrison SJ, Bishton M, Bates SE, Grant S, Piekarz RL, Johnstone RW, et al. A Focus on the Preclinical Development and Clinical Status of the Histone Deacetylase Inhibitor, Romidepsin (Depsipeptide, Istodax()). Epigenomics (2012) 4(5):571–89. doi: 10.2217/epi.12.52
116. Hui FK, Chiang SAK, Cheung LAK, Choi CK, Yeung PL, Middeldorp JM. Inhibition of Class I Histone Deacetylases by Romidepsin Potently Induces Epstein-Barr Virus Lytic Cycle and Mediates Enhanced Cell Death With Ganciclovir. Int J Cancer (2016) 138(1):125–36. doi: 10.1002/ijc.29698
117. Kim SJ, Kim JH, Ki CS, Ko YH, Kim JS, Kim WS. Epstein-Barr Virus Reactivation in Extranodal Natural Killer/T-Cell Lymphoma Patients: A Previously Unrecognized Serious Adverse Event in a Pilot Study With Romidepsin. Ann Oncol Off J Eur Soc Med Oncol (2016) 27(3):508–13. doi: 10.1093/annonc/mdv596
118. Kim JH, Kim WS, Park C. Sildenafil Prevents HDACi-Induced Epstein-Barr Virus Reactivation Through the PKG Pathway in NK/T Cell Lymphoma; Potential Implications for HDACi-Mediated Fatal Complications. Antiviral Res (2021) 189:105063. doi: 10.1016/j.antiviral.2021.105063
119. Kane RC. United States Food and Drug Administration Approval Summary: Bortezomib for the Treatment of Progressive Multiple Myeloma After One Prior Therapy. Clin Cancer Res (2006) 12(10):2955. doi: 10.1158/1078-0432.CCR-06-0170
120. Shirley CM, Chen J, Shamay M, Li H, Ambinder RF. Bortezomib Induction of C/Ebpβ Mediates Epstein-Barr Virus Lytic Activation in Burkitt Lymphoma. Blood (2011) 117(23):6297–303. doi: 10.1182/blood-2011-01-332379
121. Fu DX, Tanhehco Y, Chen J, Foss CA, Fox JJ, Chong JM, et al. Bortezomib-Induced Enzyme-Targeted Radiation Therapy in Herpesvirus-Associated Tumors. Nat Med (2008) 14(10):1118. doi: 10.1038/nm.1864
122. Granato M, Romeo MA, Tiano MS, Santarelli R, Gonnella R, Gilardini Montani MS, et al. Bortezomib Promotes KHSV and EBV Lytic Cycle by Activating JNK and Autophagy. Sci Rep (2017) 7(1):13052. doi: 10.1038/s41598-017-13533-7
123. Vaysberg M, Hatton O, Lambert SL, Snow AL, Wong B, Krams SM, et al. Tumor-Derived Variants of Epstein-Barr Virus Latent Membrane Protein 1 Induce Sustained Erk Activation and C-Fos. J Biol Chem (2008) 283(52):36573–85. doi: 10.1074/jbc.M802968200
124. Morris MA, Dawson CW, Young LS. Role of the Epstein-Barr Virus-Encoded Latent Membrane Protein-1, LMP1, in the Pathogenesis of Nasopharyngeal Carcinoma. Future Oncol (2009) 5(6):811–25. doi: 10.2217/fon.09.53
125. Adams J. Proteasome Inhibition: A Novel Approach to Cancer Therapy. Trends Mol Med (2002) 8(4 Suppl):S49–54. doi: 10.1016/S1471-4914(02)02315-8
126. Zhang XM, Lin H, Chen C, Chen DM. Inhibition of Ubiquitin-Proteasome Pathway Activates a Caspase-3-Like Protease and Induces Bcl-2 Cleavage in Human M-07e Leukaemic Cells. Biochem J (1999) 340(Pt 1):127–33. doi: 10.1042/bj3400127
127. Huynh M, Pak C, Markovina S, Callander NS, Chng KS, Wuerzberger-Davis SM, et al. Hyaluronan and Proteoglycan Link Protein 1 (HAPLN1) Activates Bortezomib-Resistant NF-Kappa B Activity and Increases Drug Resistance in Multiple Myeloma. J Biol Chem (2018) 293(7):2452–65. doi: 10.1074/jbc.RA117.000667
128. Fu DX, Tanhehco YC, Chen J, Foss CA, Fox JJ, Lemas V, et al. Virus-Associated Tumor Imaging by Induction of Viral Gene Expression. Clin Cancer Res (2007) 13(5):1453–8. doi: 10.1158/1078-0432.CCR-06-2295
129. Shen L, Au W, Guo T, Wong K, Wong M, Tsuchiyama J, et al. Proteasome Inhibitor Bortezomib-Induced Apoptosis in Natural Killer (NK)-Cell Leukemia and Lymphoma: An In Vitro and In Vivo Preclinical Evaluation. Blood (2007) 110(1):469–70. doi: 10.1182/blood-2007-02-072900
130. Tai YT, Landesman Y, Acharya C, Calle Y, Zhong MY, Cea M, et al. CRM1 Inhibition Induces Tumor Cell Cytotoxicity and Impairs Osteoclastogenesis in Multiple Myeloma: Molecular Mechanisms and Therapeutic Implications. Leukemia (2014) 28(1):155–65. doi: 10.1038/leu.2013.115
131. Bertucci F, Finetti P, Birnbaum D. XPO1, Therapeutic And Prognostic Target in Sarcomas. Oncoscience (2016) 3(5-6):143–4. doi: 10.18632/oncoscience.304
132. Liu X, Chong Y, Tu Y, Liu N, Yue C, Qi Z, et al. CRM1/XPO1 is Associated With Clinical Outcome in Glioma and Represents a Therapeutic Target by Perturbing Multiple Core Pathways. J Hematol Oncol (2016) 9(1):108. doi: 10.1186/s13045-016-0338-2
133. Boyle SM, Ruvolo V, Gupta AK, Swaminathan S. Association With the Cellular Export Receptor CRM 1 Mediates Function and Intracellular Localization of Epstein-Barr Virus SM Protein, a Regulator of Gene Expression. J Virol (1999) 73(8):6872–81. doi: 10.1128/jvi.73.8.6872-6881.1999
134. Sin-Yeang T, Che NA, Ali SA, Soo-Beng KA. Exosomes in Human Immunodeficiency Virus Type I Pathogenesis: Threat or Opportunity? Adv Virol (2016) 2016:9852494. doi: 10.1155/2016/9852494
135. Milane L, Singh A, Mattheolabakis G, Suresh M, Amiji MM. Exosome Mediated Communication Within the Tumor Microenvironment. J Controlled Release (2015) 219:278–94. doi: 10.1016/j.jconrel.2015.06.029
136. Keryer-Bibens C, Pioche-Durieu C, Villemant C, Souquère S, Nishi N, Hirashima M, et al. Exosomes Released by EBV-Infected Nasopharyngeal Carcinoma Cells Convey the Viral Latent Membrane Protein 1 and the Immunomodulatory Protein Galectin 9. BMC Cancer (2006) 6(1):283. 6, 1(2006-12-08). doi: 10.1186/1471-2407-6-283
137. Meckes DG. Exosomal Communication Goes Viral. J Virol (2015) 89(10):5200–3. doi: 10.1128/JVI.02470-14
138. Aga M, Bentz G, Raffa L, Torrisi MR, Kondo S, Wakisaka N, et al. Exosomal Hif1α Supports Invasive Potential of Nasopharyngeal Carcinoma-Associated LMP1-Positive Exosomes. Oncogene (2014) 33(37):4613–22. doi: 10.1038/onc.2014.66
Keywords: barr virus, lymphoproliferative disorders, lymphoma, therapy, advances
Citation: Lv K, Yin T, Yu M, Chen Z, Zhou Y and Li F (2022) Treatment Advances in EBV Related Lymphoproliferative Diseases. Front. Oncol. 12:838817. doi: 10.3389/fonc.2022.838817
Received: 18 December 2021; Accepted: 11 March 2022;
Published: 19 April 2022.
Edited by:
Hubing Shi, Sichuan University, ChinaReviewed by:
Won Seog Kim, Sungkyunkwan University, South KoreaJoshua Nyagol, University of Nairobi, Kenya
Copyright © 2022 Lv, Yin, Yu, Chen, Zhou and Li. This is an open-access article distributed under the terms of the Creative Commons Attribution License (CC BY). The use, distribution or reproduction in other forums is permitted, provided the original author(s) and the copyright owner(s) are credited and that the original publication in this journal is cited, in accordance with accepted academic practice. No use, distribution or reproduction is permitted which does not comply with these terms.
*Correspondence: Yulan Zhou, d2VueGluX3lsQDE2My5jb20=; Fei Li, bmR5ZnkwMTIzOEBuY3UuZWR1LmNu
†These authors have contributed equally to this work