- Department of Psychology and Anesthesia, McGill University, Montreal, QC, Canada
Pain needs to be measured in order to be studied and managed. Pain measurement strategies in both humans and non-human animals have varied widely over the years and continue to evolve. This review describes the historical development of human and animal algesiometry.
Introduction
A common adage, variously attributed to management consultants Peter Drucker or W. Edwards Deming, asserts that “if you can't measure it, you can't manage it.” This is obviously true for pain, which can neither be managed nor even studied without being measured. The measurement of pain in humans and animals—algesiometry—has been a continuing focus of pain researchers since the late 1800s, coinciding with the development of psychophysics (1). Methods for estimating the intensity of a stimulus, applied to human skin, required to evoke the perception of pain were developed using noxious electrical (2), mechanical (3), and heat (4, 5) stimuli. In the first few decades of the 20th century, such methods were used to establish analgesic dose-response curves of opioids and aspirin (6–8), investigate intra- and inter-individual variability (5), circadian rhythmicity (7), spatial summation (9), counter-irritation (5), and even to demonstrate the effect of modulatory factors such as distraction (5). The first algesiometric techniques for laboratory animals were based on these human methods, with noxious stimuli aimed at easily accessible body parts such as the tail (10, 11) and plantar hind paw (12, 13). The von Frey filament test of mechanical sensitivity is used identically in human and non-human animals, with the exception of the range of filament force employed and the nature (verbal or non-verbal) of the response. Of course, refinements of these procedures have been made over the years and continue to this day (e.g., 14–16).
One might ask why any further developments in algesiometry were (and are) required. I would argue that these historical methods, though useful, fail to suffice for a number of reasons best thought of in terms of the dimensions, continua, and categories shown in Figure 1. Likely the most important of these is duration (see Figure 1A). All algesiometric methods introduced prior to the mid-1900s are measurements of acute pain, in which the time elapsing from stimulus onset to pain threshold can be measured in seconds, and also in seconds from pain threshold to pain tolerance (or, in animals, to withdrawal from the stimulus). The stimuli need to be of sufficient intensity to potentially cause tissue damage, and Woodworth / Sherrington (17) suggested that “pain” was mediated by higher-order systems driven by these stimuli. Although such stimuli clearly produce an aversive condition that is valid as a painful state (e.g., picking up a hot coffee cup), the pain states that researchers and clinicians are most interested in studying and managing, respectively, occur on a much longer time scale: hours to years. Whether mechanisms and treatment strategies applicable to pain measured in seconds are also applicable to pain measured in months is hardly a given. A second dimension of pain highly relevant to algesiometry is its locus (see Figure 1B). The historical algesiometric techniques described above involve noxious stimuli being applied by an experimenter to the skin of the pain-perceiving subject. Again, it's real pain, but different in character from clinical pain, which is usually not superficial but deep (e.g., muscle pain, joint pain, visceral pain) (18), if it can be precisely localized at all, and not evoked by a stimulus external to the body but rather arising within the body itself. The type of pain we really want to understand is spontaneous pathological pain (19, but see 20), which may or may not share underlying mechanisms with evoked pain. As already well appreciated in the 1800s, different noxious stimulus modalities (see Figure 1C) exist. Pain can be caused by mechanical force, electric shock, heat, and cold. But it can also be evoked by irritant chemicals applied to the skin or otherwise introduced into the body. Much clinical pain arises via the presence of inflammation or nerve damage, which are both associated with the release of chemical messengers in the immune and nervous systems. The generation of nociplastic (previously known as functional or idiopathic) pain remains a mystery. The transduction of different pain modalities by nociceptors is dissociable, mediated by different proteins, and there exists considerable evidence that the processing of different modalities of pain is dissociable at more rostral levels of the neuraxis as well. Especially in the context of pain questionnaires, pain associated with different clinical entities may need to be measured in different ways; this is pain's version of the lumping/splitting problem. Finally, the type of response (see Figure 1D) can have serious consequences in algesiometry in both humans and animals. In humans, one can choose to measure pain threshold, pain tolerance, or to elicit quantitative ratings of suprathreshold stimuli. Alternatively, one might eschew quantitative measurement and elicit qualitative descriptors of pain via questionnaires, or try to avoid self report entirely in favor of an “objective” biomarker. Animals, of course, cannot be instructed to respond at threshold, cannot easily be motivated to hold off responding until tolerance, and are incapable of verbal report. For them, nocifensive and other behaviors are necessary for experimenters to interpret the presence of pain. This is often held as a disadvantage of preclinical pain research, although a human subject rating their back pain “an 8” is equally a behavior requiring interpretation.
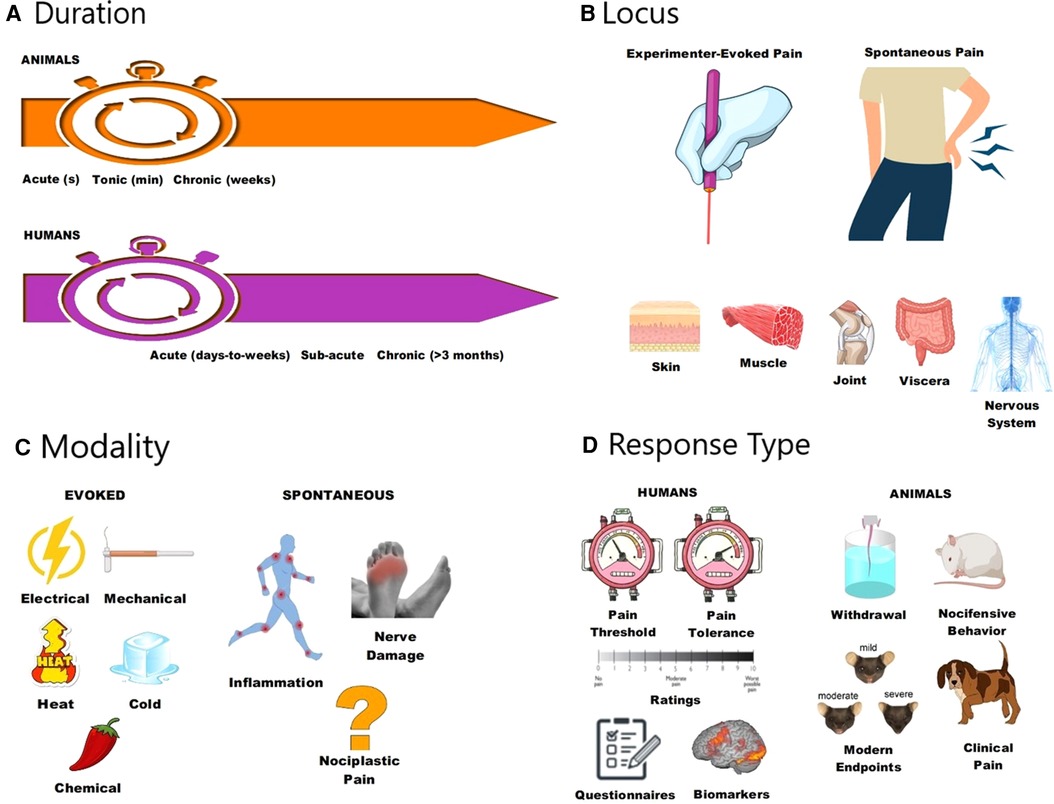
Figure 1. Different ways to think about different types of pain, all with implications for algesiometry in humans and non-human animals. Pain can be dissociated based on its duration (A), locus (B), modality (C), and response type (D).
The history of algesiometry is the story of attempts by pain researchers to broaden available tools so that they cover more of these continua, into the range that more typifies the clinical problem at hand. This review will attempt to briefly summarize developments over the years in humans and non-human animals, with respect to the dimensions described above.
Pain measurement in humans
The measurement of experimental pain in humans using controlled noxious stimuli delivered to the skin (superficially or subdermally), muscles, joints, or viscera has continued unabated since the pioneering psychophysical work in the 1800s. In general, regardless of the noxious stimulus employed, attempts are made to quantify either pain threshold, pain tolerance, ratings of pain on structured scales, magnitude estimation (e.g., by cross-modality matching), or measurement of performance (21). Beyond the many issues surrounding confounds and inter- and intra-individual differences, the obvious limitation is that these are ways to measure sensitivity to the particular noxious stimuli delivered, with no obvious relation (except perhaps in certain pain syndromes such as fibromyalgia) to the clinical pain of a patient, although attempts were often made to compare the magnitude of the experimental pain to a patient's clinical pain.
The first major proponent of abandoning the “dolorimeter” approach to measuring clinical pain was Beecher (22), who advanced the notion of measuring clinical pain by its relief, via subjective ratings. But how exactly to provide such ratings? Solutions to this problem include the use of numerical rating scales (NRS) with descriptive “pegs” at the ends (e.g., from 0, no pain, to 10, the worst pain imaginable), verbal rating scales (VRS) of category judgments (e.g., mild, distressing, excruciating), and visual analog scales (VAS) (23), in which pain is indicated by marking a spot along a 10-cm continuum. These have been modified for use in pediatric and elderly populations (24, 25), and categories and pegs translated into different languages. As it became increasingly clear that pain was multidimensional—consisting of sensory/discriminative, motivational/affective, and cognitive components—research subjects and patients were increasingly asked for multiple ratings (e.g., 26).
A rather different approach to the problem was developed by Melzack and Torgerson (27, 28) with the McGill Pain Questionnaire, in which pain is rated qualitatively via sensory, evaluative, and affective descriptors (e.g., burning, shooting, troublesome, agonizing). Questionnaires are currently used not only to measure different aspects of pain itself, but also to: (1) more thoroughly characterize disease states featuring pain, and their impact on functioning and quality of life (29, 30); (2) help diagnose the presence of particular types of pain, such as neuropathic pain (31); and (3) quantify putative risk factors for chronic pain, such as catastrophizing (32).
Finally, a whole host of observational methods have been developed in which pain is quantified by others. Such methods, valuable especially for use in non-verbal populations, include the FLACC scale for young children (33), in which facial expression, leg position, activity, crying, and consolability are rated on a 0–2 scoring scale, and the Neonatal Facial Coding System (34), in which pain expression is quantified via judgments of facial musculature (e.g., brow bulging, eye squeezing). Other observational methods attempt to bypass patient self-report via the measurement, for example, of visible behaviors (e.g., guarding, limping, rubbing, sighing) by clinicians (35) or changes in a child's daily behaviors (e.g., playing less, complaining more) by parents (36). Such techniques are obviously susceptible to bias, although individuals are likely biased self-observers as well.
Recently, the use of modern versions of venerable acute pain measures has been enjoying a renaissance, more for the purpose of patient stratification (e.g., 37) than pain quantification per se. Known as quantitative sensory testing (QST), the most comprehensive effort has been by a German consortium involving the QST profiling—using 13 different measures of gain and loss of sensory function—of over a thousand patients and non-patients (38, 39). Although different frequencies of sensory abnormalities were observed in different pain disorders (38), it has more recently been shown that QST batteries are far better at quantifying neuropathy itself than neuropathic pain (40, 41).
Ultimately, although the validity and usefulness of self-reported pain, especially by VAS, has been amply demonstrated (42), researchers and clinicians have always longed for an objective measure, or biomarker, that could be used for diagnostic, prognostic, and/or drug development purposes. Although we are counseled to always “trust the patient”, there are obvious (if not necessarily frequent) incentives for both exaggerated and minimized self-reporting. Putative biomarkers over the years have included physiological measurements, blood protein levels, genetic variants, and nervous system electrical (e.g., microneurography, electroencephalography) and metabolic activity [e.g., positron emission tomograph, functional magnetic resonance imaging (fMRI)]. The leading contender as a pain biomarker is likely fMRI, although pain imagers have cautioned against its over-credulous use (43). It seems likely that useful pain biomarkers will need to be composites of many different types of measures (44).
Pain measurement in non-human animals
Despite developments in human pain research such as fMRI, animal models of pain have always been and continue to be necessary for ethical reasons and to obtain causal, mechanistic explanations of pain pathophysiology (45). As described in a prior, comprehensive review (46), algesiometry in laboratory animals has featured several waves of development. The classical assays featured the application of electrical, mechanical, or thermal stimuli to conveniently located body parts, producing pain for as long as it took for the stimulus to reach noxious intensity and the animal to reflexively or consciously withdraw. In the 1950s, several groups demonstrated that intraperitoneal injection of irritants (e.g., weak acids, phenylquinone) produced abdominal constriction (i.e., “writhing”) behavior (47–51) lasting 20–60 min, and that these assays appeared to have higher sensitivity to non-opioid analgesics like aspirin. In addition to lasting longer than the acute assays, these were tests of inescapable, suprathreshold pain (like clinical pain), and the endpoints (i.e., dependent measures) represented total time spent performing a behavior positively correlated with stimulus intensity rather than latency to a first response. Drawbacks included an uncertain location of the pain (visceral? muscle wall?), a non-linear stimulus-response relationship, and concerns over selectivity. An interesting advance occurred in 1977, when Dubuisson and Dennis (52), working in the laboratory of Ron Melzack (who in service of Stephen Dennis’ career declined to take an authorship), reported that formalin (i.e., diluted formaldehyde) injected into the forepaw of cats and rats produced guarding and licking/biting/shaking of the affected paw (In a classic footnote, they described the results of such an injection into their own finger.) In rats, a biphasic time course was noted, with early/acute (0–10 min) and late/tonic phases (>20 min) of pain behavior being interrupted by “a significant dip” lasting for about 10 min, and the two phases and “interphase” or “quiescent period” of the formalin test engendered voluminous study over the next few decades (see 53).
However, even the 60–90-min duration of the formalin test was clearly too short to properly model human chronic pain. A number of longer-lasting assays were developed, by injecting immune system-activating substances used previously to study inflammation—such as carrageenan (54), complete Freund's adjuvant (CFA) (55), zymosan (56), and urate crystals (57, 58)—into the hind paw or knee joint. A curious observation arising from the use of these assays was that the longer lasting the inflammation and the more time elapsed since injection, the less likely was the observation of any obvious nocifensive behaviors (i.e., licking, biting, shaking). For example, in the first paper reporting the effects of hind paw-injected CFA, Stein and colleagues (55) observed changes in body weight, food intake, core temperature, locomotion, defecation, and paw-pressure thresholds over 30 days post-injection, but no nocifensive behaviors. Thus, the advantage of a longer-lasting assay was paired with the disadvantage of needing to employ endpoints of questionable specificity to pain (e.g., hypolocomotion) or endpoints corresponding to comparatively minor human chronic pain symptoms, such as thermal or mechanical hypersensitivity (18, 38, 59).
A similar situation developed in the quest for longer-lasting animal models of neuropathic pain. The first such behavioral model was developed by Wall and colleagues (60), featuring a bizarre endpoint known as autotomy, whereby the animal progressively bites off the digits of the denervated paw. A credible model of phantom limb pain, this assay is almost never used because of its disagreeable aesthetics, controversial interpretation (61), and the fact that most human neuropathic pain is caused by partial, not complete injury to a nerve (62). In appreciation of this, Bennett and Xie (63) in 1988 developed an assay of peripheral mononeuropathy in which the sciatic nerve is slowly strangled by the placement of constrictive ligatures. Other strategies for producing partial disruptions of afferent input from the hind paw soon followed (64–66), and today there exist an alphabet soup of surgically and chemically induced nerve injuries (see 67). Although these differ in their symptom profile (e.g., only some featuring heat hypersensitivity), as with the inflammatory assays apart from (species-specific) guarding behavior there are no overt nocifensive behaviors to measure (68), and very little effect on activities of daily living (69, 70).
As measured by mechanical hypersensitivity, the most robust and thus most-used endpoint (71), the duration of pain varies in these assays. For example, hypersensitivity in the chronic constriction injury (CCI) assay is entirely resolved within 30–90 days post-surgery (63), whereas mechanical hypersensitivity in the spared nerve injury (SNI) lasts an entire lifetime (72). Bonica (73) defined chronic pain as that persists past normal healing time, but the need for a clearer cut off led Merskey (74) to propose (an arbitrary) 3 months as the definition of chronic, and this has been retained (75), although not without controversy (76). The SNI is suited for longer investigations, but 80% of extant preclinical pain studies are completed in less than 4 weeks post-injury (71). The wisdom of this status quo is called into question by two recent mouse studies showing important pathophysiological changes that do not occur until many months after injury (77, 78). It remains quite possible that 3 months in a mouse is…3 months.
Perhaps the strongest and most enduring criticism of the status quo in preclinical pain testing is the continued reliance on reflexive withdrawals to experimenter-delivered stimuli as an endpoint (e.g., 19, 79, 80). The most recent wave of development, therefore, has been to identify new endpoints. As described in recent, comprehensive reviews (81, 82), these can be broadly classified as pain-stimulated behaviors, pain-depressed behaviors, conditioned/motivated behaviors, measures of disability or quality of life, and biomarkers. Notable among these are: (1) operant conditioning to produce place avoidance (83); (2) conditioned place preference to analgesics as a way to infer pain during the classical conditioning (84, 85); (3) depression of previously favored activities such as wheel running (86), nest building (87), burrowing (88, 89), feeding (90), and cage-lid hanging (70); (4) measures of pain-related disability in, for example, grip strength (91); and (5) facial grimacing (92). Each of these have advantages and disadvantages compared to legacy endpoints, and many of these procedures are much more labor-intensive than their predecessors, although automation is proceeding apace (93). In some contexts, instead of behavioral endpoints one can employ non-behavioral proxies of nociceptive activity using powerful electrophysiological or calcium imaging techniques.
Finally, a recent development in preclinical algesiometry, especially at later stages of preclinical research, is to consider assessing new treatments via their effect on clinical pain states (e.g., arthritis, cancer pain) in companion animals (see 94, 95). Combined with more valid endpoints, such as automated measurement of grimacing in cats (96), this might represent a powerful way to predict clinically efficacy in human trials.
The future of algesiometry
It can be argued that algesiometry in both humans and non-human animals have largely failed thus far in their respective aims. The continuing quest for objective biomarkers of pain in humans suggests that self-reported ratings are still not fully trusted, and many pain physicians increasingly avoid soliciting such ratings in favour of broader (but less selective) measures of functional disability and quality of life. Other dimensions of pain are being given more attention as well, including cognitive and social aspects (97), and there is likely to be much more study of “social pain”, aversive states not associated with physical injury and independent of somatic/visceral input (98). The repeated translational failures of analgesic development over the past decades have often, fairly or not, been blamed on the inadequacy of preclinical models (e.g., 99). Whether useful biomarkers and modern preclinical methods can improve the situation will be interesting to monitor over the next few decades. As ever, solutions to the measurement problem are critical for both the understanding and better management of pain.
Author contributions
The author confirms being the sole contributor of this work and has approved it for publication.
Conflict of interest
The author declares that the research was conducted in the absence of any commercial or financial relationships that could be construed as a potential conflict of interest.
Publisher's note
All claims expressed in this article are solely those of the authors and do not necessarily represent those of their affiliated organizations, or those of the publisher, the editors and the reviewers. Any product that may be evaluated in this article, or claim that may be made by its manufacturer, is not guaranteed or endorsed by the publisher.
References
2. Helmholtz H. Ueber die dauer und den verlauf der durch stromesschwankungen inducirten elektrischen strome. Annalen der Physik. (1851) 83:505–40. doi: 10.1002/andp.18511590803
3. von Frey M. Verspatete schmerzempfindungen. Z Gesamte Neurol Psychiat. (1922) 79:324–33. doi: 10.1007/BF02878457
4. Goldscheider A. Thermoreceptoren. I. Temperatursinn des menschen. Handbuch der Normalin und Pathologischen Physiologie. (1926) 11:131–64.
5. Hardy JD, Wolff HG, Goodell H. A new method for measuring pain threshold: observations on spatial summation of pain. J Clin Invest. (1940) 19:649. doi: 10.1172/JCI101168
6. Eddy NB. Studies of morphine, codeine and their derivatives; general methods. J Pharmacol Exp Ther. (1932) 45:339–59.
7. Martin EG. A quantitative study of faradic stimulation. II. The calibration of the inductorium for break shocks. Am J Physiol. (1908) 22:116–32. doi: 10.1152/ajplegacy.1908.22.1.116
8. Macht DI, Herman NB, Levy CS. A quantitative study of the analgesia produced by opium alkaloids, individually and in combination with each other in normal man. J Pharmacol Exp Ther. (1916) 8:1–37.
9. Hardy JD, Oppel TW. Studies in temperature sensation. III. The sensitivity of the body to heat and the spatial summation of end organ responses. J Clin Invest. (1937) 16:533–40. doi: 10.1172/JCI100879
10. D'Amour FE, Smith DL. A method for determining loss of pain sensation. J Pharmacol Exp Ther. (1941) 72:74–9.
11. Nilsen PL. Studies on algesimetry by electrical stimulation of the mouse tail. Acta Pharmacol Toxicol. (1961) 194:967–8.
12. Blake L, Graeme ML, Sigg EB. Grid shock test for analgesics in mice. Med Exp. (1963) 9:146–50.14083334
13. Evans WO. A new technique for the investigation of some analgesic drugs on a reflexive behavior in the rat. Psychopharmacology. (1961) 2:318–25. doi: 10.1007/BF00404119
14. Hargreaves K, Dubner R, Brown F, Flores C, Joris J. A new and sensitive method for measuring thermal nociception in cutaneous hyperalgesia. Pain. (1988) 32:77–88. doi: 10.1016/0304-3959(88)90026-7
15. Allchorne AJ, Broom DC, Woolf CJ. Detection of cold pain, cold allodynia and cold hyperalgesia in freely behaving rats. Mol Pain. (2005) 1:36. doi: 10.1186/1744-8069-1-36
16. Bonin RP, Bories C, De Koninck Y. A simplified up-down method (SUDO) for measuring mechanical nociception in rodents using von frey filaments. Mol Pain. (2014) 10:26. doi: 10.1186/1744-8069-10-26
17. Woodworth RS, Sherrington CS. A pseudoaffective reflex and its spinal path. J Physiol. (1904) 31:234–43. doi: 10.1113/jphysiol.1904.sp001034
18. Scholz J, Mannion RJ, Hord DE, Griffin RS, Rawal B, Zheng H, et al. A novel tool for the assessment of pain: validation in low back pain. PLoS Med. (2009) 6(4):e1000047. doi: 10.1371/journal.pmed.1000047
19. Mogil SJ, Crager SE. What should we be measuring in behavioral studies of chronic pain in animals? Pain. (2004) 112(1-2):12–5. doi: 10.1016/j.pain.2004.09.028
20. Bennett GJ. What is spontaneous pain and who has it? J Pain. (2012) 13(10):921–9. doi: 10.1016/j.jpain.2012.05.008
21. Chapman CR, Casey KL, Dubner R, Foley KM, Gracely RH, Reading AE. Pain measurement: an overview. Pain. (1985) 22:1–31. doi: 10.1016/0304-3959(85)90145-9
22. Beecher HK. The measurement of pain: prototype for the quantitative study of subjective responses. Pharmacol Rev. (1957) 9:59–209.13431416
23. Bond MR, Pilowsky I. Subjective assessment of pain and its relationship to the administration of analgesics in patients with advanced cancer. J Psychosom Res. (1966) 10:203–8. doi: 10.1016/0022-3999(66)90064-X
24. Chambers CT, Hardial J, Craig KD, Court C, Montgomery C. Faces scales for the measurement of postoperative pain intensity in children following minor surgery. Clin J Pain. (2005) 21:277–85. doi: 10.1097/00002508-200505000-00011
25. Herr KA, Mobily PR. Comparison of selected pain assessment tools for use with the elderly. Appl Nurs Res. (1993) 6:39–46. doi: 10.1016/S0897-1897(05)80041-2
26. Gracely RH, McGrath F, Dubner R. Ratio scales of sensory and affective verbal pain descriptors. Pain. (1978) 5:5–18. doi: 10.1016/0304-3959(78)90020-9
27. Melzack R. The McGill pain questionnaire: major properties and scoring methods. Pain. (1975) 1(3):277–99. doi: 10.1016/0304-3959(75)90044-5
28. Melzack R, Torgerson WS. On the language of pain. Anesthesiology. (1971) 34:50–9. doi: 10.1097/00000542-197101000-00017
29. Bellamy N, Buchanan WW, Goldsmith CH, Campbell J, Stitt LW. Validation study of WOMAC: a health status instrument for measuring clinically important patient relevant outcomes to antirheumatic drug therapy in patients with osteoarthritis of the hip or knee. J Rheumatol. (1988) 15:1833–40.3068365
30. Cleeland CS, Ryan KM. Pain assessment: global use of the brief pain inventory. Ann Acad Med Singap. (1994) 23:129–38.8080219
31. Bouhassira D, Attal N, Alchaar H, Bourea F, Brochet B, Bruxelle J, et al. Comparison of pain syndromes associated with nervous or somatic lesions and development of a new neuropathic pain diagnostic questionnaire (DN4). Pain. (2005) 114:29–36. doi: 10.1016/j.pain.2004.12.010
32. Sullivan MJL, Bishop S, Pivik J. The pain catastrophizing scale: development and validation. Psychol Assess. (1995) 7:524–32. doi: 10.1037/1040-3590.7.4.524
33. Merkel S, Voepel-Lewis T, Shaevitz JR, Malviya S. The FLACC: a behavioral scale for scoring postoperative pain in young children. Pediatr Nurs. (1997) 23:293–7.9220806
34. Grunau RV, Craig KD. Pain expression in neonates: facial action and cry. Pain. (1987) 28(3):395–410. doi: 10.1016/0304-3959(87)90073-X
35. Keefe F, Block A. Development of an observational method for assessing pain behavior in chronic pain patients. Behav Ther. (1982) 13:363–75. doi: 10.1016/S0005-7894(82)80001-4
36. Chambers CT, Reid GJ, McGrath PJ, Finley GA. Development and preliminary validation of a postoperative pain measure for parents. Pain. (1996) 68:307–13. doi: 10.1016/S0304-3959(96)03209-5
37. Demant DT, Lund K, Vollert J, Maier C, Segerdahl M, Finnerup NB, et al. The effect of oxcarbazepine in peripheral neuropathic pain depends on pain phenotype: a randomised, double-blind, placebo-controlled phenotype-stratified study. Pain. (2014) 155:2263–73. doi: 10.1016/j.pain.2014.08.014
38. Maier C, Baron R, Tolle TR, Binder A, Birbaumer N, Birklein F, et al. Quantitative sensory testing in the German research network on neuropathic pain (DFNS): somatosensory abnormalities in 1236 patients with different neuropathic pain syndromes. Pain. (2010) 150:439–50. doi: 10.1016/j.pain.2010.05.002
39. Rolke R, Baron R, Maier C, Tolle TR, Treede R-D, Beyer A, et al. Quantitative sensory testing in the German research network on neuropathic pain (DFNS): standardized protocol and reference values. Pain. (2006) 123(3):231–43. doi: 10.1016/j.pain.2006.01.041
40. Schmelz M. Quantitative sensory test correlates with neuropathy, not with pain. Pain. (2018) 159(3):409–10. doi: 10.1097/j.pain.0000000000001142
41. Forstenpointner J, Ruscheweyh R, Attal N, Baron R, Bouhassira D, Enax-Krumova EK, et al. No pain, still gain (of function): the relation between sensory profiles and the presence or absence of self-reported pain in a large multicenter cohort of patients with neuropathy. Pain. (2021) 162:718–27. doi: 10.1097/j.pain.0000000000002058
42. Price DD, McGrath P, Rafii A, Buckingham B. The validation of visual analogue scales as ratio scale measures for chronic and experimental pain. Pain. (1983) 17:45–56. doi: 10.1016/0304-3959(83)90126-4
43. Davis KD, Aghaeepour N, Ahn AH, Angst MS, Borsook D, Brenton A, et al. Discovery and validation of biomarkers to aid the development of safe and effective pain therapeutics: challenges and opportunities. Nat Rev Neurol. (2020) 16:381–400. doi: 10.1038/s41582-020-0362-2
44. Tracey I, Woolf CJ, Andrews NA. Composite pain biomarker signatures for objective assessment and effective treatment. Neuron. (2019) 101:783–800. doi: 10.1016/j.neuron.2019.02.019
45. Mogil JS, Davis KD, Derbyshire SW. The necessity of animal models in pain research. Pain. (2010) 151:12–7. doi: 10.1016/j.pain.2010.07.015
46. Mogil JS. Animal models of pain: progress and challenges. Nat Rev Neurosci. (2009) 10(4):283–94. doi: 10.1038/nrn2606
47. van der Wende C, Margolin S. Analgesic tests based upon experimentally induced acute abdominal pain in rats. Fed Proc. (1956) 15:494.
48. Siegmund E, Cadmus R, Lu G. A method for evaluating both non-narcotic and narcotic analgesics. Proc Soc Exp Biol Med. (1957) 95:729–31. doi: 10.3181/00379727-95-23345
50. Hendershot LC, Forsaith S. Antagonism of the frequency of phenylquinone-induced writhing in the mouse by weak analgesics and non-analgesics. J Pharmacol Exp Ther. (1959) 125:237–40.13642264
52. Dubuisson D, Dennis SG. The formalin test: a quantitative study of the analgesic effects of morphine, meperidine, and brain stem stimulation in rats and cats. Pain. (1977) 4:161–74. doi: 10.1016/0304-3959(77)90130-0
53. Tjolsen A, Berge O-G, Hunskaar S, Rosland JH, Hole K. The formalin test: an evaluation of the method. Pain. (1992) 51:5–17. doi: 10.1016/0304-3959(92)90003-T
54. Winters CA, Risley EA, Nuss GW. Carrageenin-induced edema in hind paw of the rat as an assay for antiinflammatory drugs. Proc Soc Exp Biol Med. (1962) 111:544–7. doi: 10.3181/00379727-111-27849
55. Stein C, Millan MJ, Herz A. Unilateral inflammation of the hindpaw in rats as a model of prolonged noxious stimulation: alterations in behavior and nociceptive thresholds. Pharmacol Biochem Behav. (1988) 31:445–51. doi: 10.1016/0091-3057(88)90372-3
56. Meller ST, Gebhart GF. Intraplantar zymosan as a reliable, quantifiable model of thermal and mechanical hyperalgesia in the rat. Eur J Pain. (1997) 1:43–52. doi: 10.1016/S1090-3801(97)90052-5
57. Coderre TJ, Wall PD. Ankle joint urate arthritis (AJUA) in rats: an alternative animal model of arthritis to that produced by freund's adjuvant. Pain. (1987) 28:379–93. doi: 10.1016/0304-3959(87)90072-8
58. Otsuki T, Nakahama H, Niizuma H, Suzuki J. Evaluation of the analgesic effects of capsaicin using a new rat model for tonic pain. Brain Res. (1986) 365:235–40. doi: 10.1016/0006-8993(86)91634-3
59. Backonja M-M, Stacey B. Neuropathic pain symptoms relation to overall pain rating. J Pain. (2004) 5(9):491–7. doi: 10.1016/j.jpain.2004.09.001
60. Wall PD, Devor M, Inbal R, Scadding JW, Schonfeld D, Seltzer Z, et al. Autotomy following peripheral nerve lesions: experimental anaesthesia dolorosa. Pain. (1979) 7:103–13. doi: 10.1016/0304-3959(79)90002-2
61. Rodin BE, Kruger L. Deafferentation in animals as a model for the study of pain: an alternate hypothesis. Brain Res Rev. (1984) 7:213–28. doi: 10.1016/0165-0173(84)90011-0
63. Bennett GJ, Xie Y-K. A peripheral mononeuropathy in rat that produces disorders of pain sensation like those seen in man. Pain. (1988) 33:87–107. doi: 10.1016/0304-3959(88)90209-6
64. Kim SH, Chung JM. An experimental model for peripheral neuropathic produced by segmental spinal nerve ligation in the rat. Pain. (1992) 50:355–63. doi: 10.1016/0304-3959(92)90041-9
65. Seltzer Z, Dubner R, Shir Y. A novel behavioral model of causalgiform pain produced by partial sciatic nerve injury in rats. Pain. (1990) 43:205–18. doi: 10.1016/0304-3959(90)91074-S
66. Decosterd I, Woolf CJ. Spared nerve injury: an animal model of persistent peripheral neuropathic pain. Pain. (2000) 87:149–58. doi: 10.1016/S0304-3959(00)00276-1
67. Colleoni M, Sacerdote P. Murine models of human neuropathic pain. Biochim Biophys Acta. (2010) 1802:924–33. doi: 10.1016/j.bbadis.2009.10.012
68. Mogil JS, Graham AC, Ritchie J, Hughes SF, Austin J-S, Schorscher-Petcu A, et al. Hypolocomotion, asymmetrically directed behaviors (licking, lifting, flinching, and shaking) and dynamic weight bearing (gait) changes are not measures of neuropathic pain in mice. Mol Pain. (2010) 6(1):34.20529328
69. Urban R, Scherrer G, Goulding EH, Tecott LH, Basbaum AI. Behavioral indices of ongoing pain are largely unchanged in male mice with tissue or nerve injury-induced mechanical hypersensitivity. Pain. (2011) 152:990–1000. doi: 10.1016/j.pain.2010.12.003
70. Zhang H, Lecker I, Collymore C, Dokova A, Pham MC, Rosen SF, et al. Cage-lid hanging behavior as a translationally relevant measure of pain in mice. Pain. (2021) 162:1416–25. doi: 10.1097/j.pain.0000000000002127
71. Sadler KE, Mogil JS, Stucky CL. Innovations and advances in modeling and measuring pain in animals. Nat Rev Neurosci. (2022) 23:70–85. doi: 10.1038/s41583-021-00536-7
72. Millecamps M, Sotocinal SG, Austin J-S, Stone LS, Mogil JS. Sex-specific effects of neuropathic pain on long-term pain behavior and mortality in mice. Pain. (2022); in press.35916733
74. Merskey H. Classification of chronic pain: descriptions of chronic pain syndromes and definitions of pain terms. Pain Suppl. (1986) 3:226.
75. Nicholas M, Vlaeyen JWS, Rief W, Barke A, Aziz Q, Benoliel R, et al. The IASP classification of chronic pain for ICD-11: chronic primary pain. Pain. (2019) 160:28–37. doi: 10.1097/j.pain.0000000000001390
76. Loeser JD. A new way of thinking about pains. Pain. (2022) 163:1670–4. doi: 10.1097/j.pain.0000000000002583
77. Muralidharan A, Sotocinal SG, Yousefpour N, Akkurt N, Lima LV, Parisien M, et al. Long-term male-specific chronic pain via telomere- and p53-mediated spinal cord cellular senescence. J Clin Invest. (2022) 132:e15187. doi: 10.1172/JCI151817
78. Gangadharan V, Zheng H, Taberner FJ, Landry J, Nees TA, Pistolic J, et al. Neuropathic pain caused by miswiring and abnormal end organ targeting. Nature. (2022) 606:137–44. doi: 10.1038/s41586-022-04777-z
79. Mao J. Translational pain research: bridging the gap between basic and clinical research. Pain. (2002) 97:183–7. doi: 10.1016/S0304-3959(02)00109-4
80. Vierck CJ, Hansson PT, Yezierski RP. Clinical and pre-clinical pain assessment: are we measuring the same thing? Pain. (2008) 135:7–10. doi: 10.1016/j.pain.2007.12.008
81. Mogil JS. The measurement of pain in the laboratory rodent. In: Wood JN, editors. The Oxford handbook of the neurobiology of pain. Oxford, U.K.: Oxford Press (2020).
82. Negus SS. Core outcome measures in preclinical assessment of candidate analgesics. Pharmacol Rev. (2019) 71:225–66. doi: 10.1124/pr.118.017210
83. LaBuda CJ, Fuchs PN. A behavioral test paradigm to measure the aversive quality of inflammatory and neuropathic pain in rats. Exp Neurol. (2000) 163:490–4. doi: 10.1006/exnr.2000.7395
84. Sufka KJ. Conditioned place preference paradigm: a novel approach for analgesic drug assessment against chronic pain. Pain. (1994) 58:355–66. doi: 10.1016/0304-3959(94)90130-9
85. King T, Vera-Portocarrero L, Gutierrez T, Vanderah TW, Dussor G, Lai J, et al. Unmasking the tonic-aversive state in neuropathic pain. Nat Neurosci. (2009) 12(11):1361–3. doi: 10.1038/nn.2407
86. Cobos EJ, Ghasemlou N, Araldi D, Segal D, Duong K, Woolf CJ. Inflammation-induced decrease in voluntary wheel running in mice: a nonreflexive test for evaluating inflammatory pain and analgesia. Pain. (2012) 153:876–84. doi: 10.1016/j.pain.2012.01.016
87. Jirkof P, Fleischmann T, Cesarovic N, Rettich A, Vogel J, Arras M. Assessment of postsurgical distress and pain in laboratory mice by nest complexity scoring. Lab Anim. (2013) 47(3):153–61. doi: 10.1177/0023677213475603
88. Jirkof P, Cesarovic N, Rettich A, Nicholls F, Seifert B, Arras M. Burrowing behavior as an indicator of post-laparotomy pain in mice. Front Behav Neurosci. (2010) 4:165. doi: 10.3389/fnbeh.2010.00165
89. Wodarski R, Delaney A, Ultenius C, Morland C, Andrews N, Baastrup C, et al. Cross-centre replication of suppressed burrowing behaviour as an ethologically relevant pain outcome measure in the rat: a prospective multicentre study. Pain. (2016) 157:2350–65. doi: 10.1097/j.pain.0000000000000657
90. Stevenson GW, Bilsky EJ, Negus SS. Targeting pain-suppressed behaviors in preclinical assays of pain and analgesia: effects of morphine on acetic acid-suppressed feeding in C57BL/6J mice. J Pain. (2006) 7(6):408–16. doi: 10.1016/j.jpain.2006.01.447
91. Kehl LJ, Trempe TM, Hargreaves KM. A new animal model for assessing mechanisms and management of muscle hyperalgesia. Pain. (2000) 85:333–43. doi: 10.1016/S0304-3959(99)00282-1
92. Mogil JS, Pang DSJ, Dutra GGS, Chambers CT. The development and use of facial grimace scales for pain measurement in animals. Neurosci Biobehav Rev. (2020) 116:480–93. doi: 10.1016/j.neubiorev.2020.07.013
93. Fried NT, Chamessian A, Zylka MJ, Abdus-Saboor I. Improving pain assessment in mice and rats with advanced videography and computational approaches. Pain. (2020) 161:1420–4. doi: 10.1097/j.pain.0000000000001843
94. Klinck MP, Mogil JS, Moreau M, Lascelles BDX, Flecknell PA, Poitte T, et al. Translational pain assessment: could natural animal models be the missing link? Pain. (2017) 158(9):1633–46. doi: 10.1097/j.pain.0000000000000978
95. Lascelles BDX, Brown DC, Maixner W, Mogil JS. Spontaneous painful disease in companion animals can facilitate the development of chronic pain therapies for humans: osteoarthritis as a leading example. Osteoarthr Cartil. (2018) 26:175–83. doi: 10.1016/j.joca.2017.11.011
96. Feighelstein M, Shimshoni I, Finka LR, Luna SPL, Mills DS, Zamansky A. Automated recognition of pain in cats. Sci Rep. (2022) 12:9575. doi: 10.1038/s41598-022-13348-1
97. Williams ACDC, Craig KD. Updating the definition of pain. Pain. (2016) 157:2420–3. doi: 10.1097/j.pain.0000000000000613
98. Eisenberger NI. Social pain and the brain: controversies, questions, and where to go from here. Annu Rev Psychol. (2015) 66:601–29. doi: 10.1146/annurev-psych-010213-115146
Keywords: algesiometry, history, human, animal, acute, chronic
Citation: Mogil JS (2022) The history of pain measurement in humans and animals. Front. Pain Res. 3:1031058. doi: 10.3389/fpain.2022.1031058
Received: 29 August 2022; Accepted: 31 August 2022;
Published: 15 September 2022.
Edited by:
Tony L. Yaksh, University of California, San Diego, United StatesReviewed by:
Howard Fields, University of California, San Francisco, United States© 2022 Mogil. This is an open-access article distributed under the terms of the Creative Commons Attribution License (CC BY). The use, distribution or reproduction in other forums is permitted, provided the original author(s) and the copyright owner(s) are credited and that the original publication in this journal is cited, in accordance with accepted academic practice. No use, distribution or reproduction is permitted which does not comply with these terms.
*Correspondence: Jeffrey S. Mogil jeffrey.mogil@mcgill.ca
Specialty Section: This article was submitted to Pain Research Methods, a section of the journal Frontiers in Pain Research