- 1Section of Pediatric Hematology/Oncology, Department of Pediatrics, Yale School of Medicine, New Haven, CT, United States
- 2Section of Medical Oncology, Department of Medicine, Yale School of Medicine, New Haven, CT, United States
- 3Department of Pharmacology, Yale School of Medicine, New Haven, CT, United States
- 4Yale Cancer Center, New Haven, CT, United States
Chemotherapy induced peripheral neuropathy (CIPN) is an often severe and debilitating complication of multiple chemotherapeutic agents that can affect patients of all ages, across cancer diagnoses. CIPN can persist post-therapy, and significantly impact the health and quality of life of cancer survivors. Identifying patients at risk for CIPN is challenging due to the lack of standardized objective measures to assess for CIPN. Furthermore, there are no approved preventative treatments for CIPN, and therapeutic options for CIPN remain limited once it develops. Biomarkers of CIPN have been studied but are not widely used in clinical practice. They can serve as an important clinical tool to identify individuals at risk for CIPN and to better understand the pathogenesis and avenues for treatment of CIPN. Here we review promising biomarkers of CIPN in humans and their clinical implications.
Introduction
Chemotherapy induced peripheral neuropathy (CIPN) is a common and debilitating toxicity of cancer therapy. CIPN manifests with distal sensory and motor impairments, including pain, paresthesia, numbness, weakness, stiffness, and muscle atrophy (1), and can lead to impaired physical function and quality of life in cancer survivors (2–4). Patients at risk for CIPN range from children to adults, and span multiple cancer diagnoses (1, 5). Classes of chemotherapy implicated in CIPN include platinums, taxanes, vinca alkaloids, proteosome inhibitors, and angiogenesis inhibitors (5). As many a 68% of adult patients receiving neurotoxic chemotherapy develop CIPN, with one third of cases persisting post-therapy (6).
Despite the high prevalence and morbidity associated with CIPN, there are significant barriers to diagnosis and treatment. There is no standardized measure for CIPN, and current measures have limitations (7, 8). Objective measures including nerve conduction studies and the Total Neuropathy Score (TNS) can be invasive and time consuming, while patient-reported measures can be biased by subjective responses (8). Further, there are no approved treatments to prevent CIPN, and limited therapeutic options once it develops (9).
Biomarkers offer a novel approach to objectively identifying and risk-stratifying patients with CIPN and can provide insight into pathogenesis and treatment. Although studies of biomarkers of CIPN have increased over the past decade, they are still not part of routine clinical care. We present a review of promising biomarkers of CIPN in humans, and their implication for clinical care and future studies.
Protein/Molecular Biomarkers of CIPN
Increasing studies are identifying alterations in serum proteins and molecular markers in CIPN patients (Figure 1).
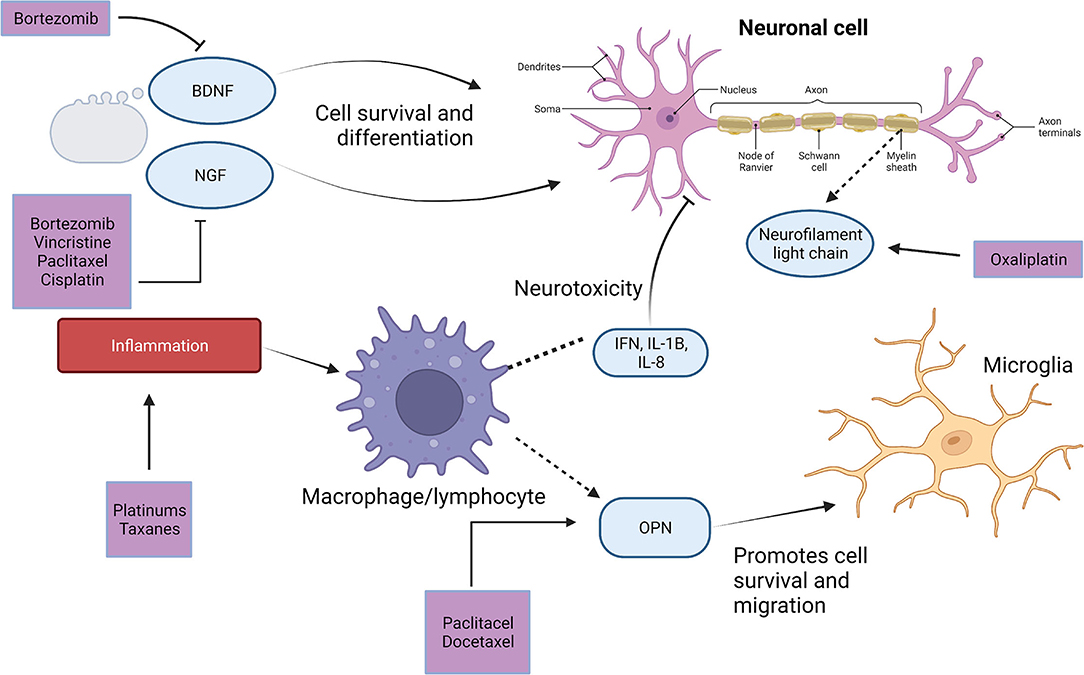
Figure 1. Serum markers of CIPN and their role in CIPN pathogenesis. Adapted from “Neuron Anatomy,” by BioRender.com (2022). Retrieved from https://app.Biorender.com/biorender-templates. CIPN, chemotherapy-induced peripheral neuropathy; BDNF, brain derived neurotrophic factor; NGF, nerve growth factor; OPN, osteopontin.
Neurofilament Light Chain (NfL)
NfL is a neuronal cytoskeletal protein released with axonal damage (10). NfL was initially described as a marker of neurodegenerative diseases (11), and later of inherited neuropathies, and CIPN in animal models (10, 12).
Recently NfL has been studied in adults with CIPN. A prospective study of 43 patients receiving oxaliplatin evaluated serum NfL and CIPN severity by nerve conduction studies throughout therapy (13). Mean serum NfL levels increased over time, with significant differences in serum NfL between low grade (0–2) CIPN vs. high grade (≥3) CIPN at 6 months, and a cut-off of 195 pg/ml being 80% sensitive and 86.2% specific to identify high grade CIPN (13).
Brain Derived Neurotrophic Factor (BDNF)
BDNF is a protein that promotes neuronal survival (14, 15). BDNF was associated with CIPN in 25 bortezomib-treated multiple myeloma patients evaluated for CIPN using the reduced Total Neuropathy Score (TNS-r) before and during therapy (16). Eight participants who developed CIPN had lower mean BDNF levels (2.16 ± 0.72 vs. 4.62 ± 0.61 ng/ml, p = 0.007), and were more likely to have a reduction from baseline BDNF (−1.67 ± 0.67 vs. 0.41 ± 0.71, p = 0.02) than those without CIPN (16). Similarly in 91 multiple myeloma patients treated with bortezomib or thalidomide lower BDNF levels during treatment were associated with CIPN by Common Terminology Criteria for Adverse Events (CTCAE), and a cut-point of 9.11 ng/ml was 76% sensitive and 71.4% specific to identify CIPN (17). Correlation between BDNF levels and CIPN by TNS-r was also reported in 22 non-Hodgkin lymphoma patients treated with vincristine (18).
Single nucleotide polymorphisms (SNPs) in BDNF may also confer increased risk for CIPN in individuals with Met/Met or Val/Met vs. Val/Val genotype (17–20). This association has been shown in bortezomib (16), and taxane-treated patients (20). A study of 35 breast cancer patients treated with taxanes, however, found the risk may be due to increased prevalence of baseline neuropathy, with no difference in prevalence of CIPN between genotypes when excluding patients with baseline neuropathy (21). There was also no association between the met-BDNF genotype and CIPN in 22 non-Hodgkin lymphoma patients treated with vincristine (18).
Nerve Growth Factor (NGF)
NGF is a protein that also regulates neuronal survival (22). In 23 cancer patients receiving taxanes or platinums NGF levels decreased after four to six cycles of chemotherapy, and decline was associated with severity of CIPN by nerve conduction studies (23). Similarly in 129 plasma samples from 34 women treated for cervical cancer with paclitaxel and cisplatin, decrease in NGF from baseline was associated with CIPN severity by TNS (24). A prospective study of 45 patients with hematologic malignancies treated with bortezomib, thalidomide, or vincristine, also found there was a significant decrease in NGF in participants who developed CIPN symptoms, whereas there was no change in NGF in participants who did not develop CIPN symptoms (25). In contrast, in a study specifically evaluating neuropathic pain in 60 patients treated with platinum or taxane therapy, the 13 patients who developed painful neuropathy had higher NGF levels post-therapy than those without neuropathic pain (26).
Osteopontin
Osteopontin is a glycoprotein involved in inflammatory pathways and has been implicated in cancer progression (27). It has been described as a marker of inflammation in other neurologic conditions included multiple sclerosis (28), and has also been implicated in neuronal repair (29). In a study of 50 breast cancer patients treated with taxanes evaluated by the TNS-r lower baseline levels of osteopontin were associated with developing moderate or severe CIPN, and baseline osteopontin levels were inversely associated with the magnitude of change in nerve conduction over time (30).
Inflammatory Markers
The immune system has increasingly been implicated in CIPN in animal models (31, 32), but few studies examine the association of immune markers with CIPN in humans. In 67 breast cancer patients treated with taxanes there was a higher peripheral blood neutrophil-to-lymphocyte ratio in patients with CIPN than those without CIPN (33). In an analysis of cytokines in 55 breast cancer patients treated with taxane and platinum chemotherapy, high IFN-γ, IL-1β, and IL-8 and low IL-10 and IL-6 were associated with CIPN symptoms (34).
MicroRNA, Proteomics, and Metabolomics
A recent approach to biomarker discovery in CIPN has included microRNA and exosome sequencing. In a preclinical model, miRNA-124 was associated with CIPN in mice treated with paclitaxel (35). MicroRNA may be a promising marker in humans as well, in cross-sectional analysis of microRNA expression in 84 breast cancer patients treated with paclitaxel, 15 microRNAs were identified with a significant fold change between CIPN and non-CIPN groups, and miRNA-451a was the most discriminatory (36). Mass spectrometry-based proteomic technology is another novel approach to biomarker discovery used to identify protein signatures associated with CIPN. In a study of 17 patients with breast cancer treated with taxanes, 12 protein signatures identified patients who developed CIPN (37).
Genetic Biomarkers of CIPN
Genetic alterations are increasingly studied as predictors of disease toxicity. The following genetic alterations have been implicated in susceptibility to CIPN (Table 1).
Genes Associated With Microtubule Function
Taxanes and vinca alkaloids interfere with microtubule function and may lead to CIPN pathogenesis (1, 73), therefore genes encoding microtubule function have been studied as predictors of CIPN sensitivity. An SNP in CEP72 (rs924607) is associated with CIPN in children and adults (38–41). In 48 adults with acute lymphoblastic leukemia (ALL) receiving vincristine, 75% with the TT genotype developed CIPN vs. 44% with CC or CT genotypes (39). In a combined sample of pediatric ALL patients treated with vincristine in two large therapeutic trials, the TT genotype was also associated with an increased risk for CIPN (38). This finding was replicated when measuring CIPN with nerve conduction studies (41), and in a separate cohort of pediatric ALL patients (40). However, other studies evaluating CEP72 alterations did not find associations with CIPN in cohorts of Spanish and Arab patients treated with vincrisitne (74, 75). Additionally, there was no association of CEP72 alterations with CIPN in patients treated with taxanes (43).
A polymorphism in TUBB2A, encoding tubulin, was associated with CIPN in 1,303 European patients treated with paclitaxel (42). However this finding has not been replicated in other studies of taxanes and vinca alkaloids (43, 76). Individual polymorphisms in MAPT (43, 76) and GSK3B (43) have not been associated with CIPN in patients treated with taxanes or vinca alkaloids, however additive polymorphisms in MAPT and GSK3B were associated with patient and clinician reported CIPN in 454 ovarian cancer patients treated with paclitaxel and carboplatin (43). SNPs in cytoskeletal protein genes, ACTG1 and CAPG, have also been associated with CIPN in pediatric ALL patients treated with vincristine (44).
Genes Associated With Ion Channels
Disturbance in neuronal function through ion channels may also contribute to CIPN, and alterations in these genes have been associated with CIPN sensitivity (1, 73, 77). In 186 Japanese breast and ovarian cancer patients treated with taxanes a SNP in SCN9A, encoding voltage-gated sodium channels, was associated with developing ≥grade 2 CIPN, and predicted CIPN persistence post-treatment (56). In 94 Spanish patients with gastrointestinal cancer treated with oxaliplatin another polymorphism in SCN9A was associated with a lower risk of acute CIPN by neurologic evaluation (57), and in 228 South Indian gastrointestinal cancer patients treated with oxaliplatin it was associated with increased incidence of chronic CIPN (58). In 200 patients with colorectal cancer treated with platinums, polymorphisms in SCN4A and SCN10A that encode voltage-gated sodium channels were associated with CIPN risk and severity (59). SCN10A has also been associated with chronic CIPN (58). Associations of mutations in voltage-gated potassium channels with CIPN have not been identified (78).
Genes Associated With Inherited Neuropathies
Genes implicated in inherited neuropathies have also been examined in relation to CIPN. SBF2, associated with Charcot-Marie-Tooth disease, was associated with CIPN in 213 African American patients treated with paclitaxel (61). However, another prospective study of 58 paclitaxel-treated patients found FZD3 was associated with CIPN, but not SBF2 (61). In a study of 219 breast cancer patients treated with taxanes, FGD4 was associated with CIPN (46). In a large prospective study of 855 patients of European Ancestry receiving paclitaxel, another polymorphism in FGD4 was associated with patient-reported sensory CIPN, which was replicated in two additional cohorts (62). In the replication cohorts a different polymorphism in FZD3 was also associated with sensory CIPN (62). ARHGEF10 was associated with CIPN in a prospective study of 269 cancer patients treated in Alliance N08C1 that analyzed blood samples for 49 Charcot-Marie-Tooth genes (63). These findings were confirmed in 138 patients treated with paclitaxel in Alliance N08CA (64).
Genes Associated With Inflammatory Pathways
There is a growing body of literature suggesting inflammation contributes to CIPN (73, 79, 80), and genetic alterations in inflammatory pathways have been studied in association with CIPN. In 3,431 breast cancer patients treated with paclitaxel a SNP in FCAMR that encodes the FC receptor, trended toward a significant association with CIPN (60). In 139 patients treated with bortezomib, variations in genes regulating immune function, CTLA4 and CTSS, were associated with time to onset of CIPN, with a similar trend for CTLA4 in a validation cohort (55). Bortezomib-neuropathy has also been associated with alterations in IL17RD, IL10RA, and genes in the NF-KB signaling pathway in 646 patients with multiple myeloma (52). Late-onset bortezomib-neuropathy was associated with polymorphisms in other genes in inflammatory pathways, MBL2 and PPARD in 186 myeloma patients (53). In a meta-analysis of pediatric patients treated with vincristine for ALL from two large clinical trials rs7963521, associated with coding of the protein chemerin implicated in inflammatory pathways (66), was associated with CIPN.
Genes Associated With Drug Metabolism and Transport
Polymorphisms in genes involved in chemotherapy metabolism may also increase CIPN sensitivity. In 107 children treated for ALL with vincristine CYP3A5 polymorphisms were associated with CIPN (45). SNPs in CYP2C8 and CYP3A4 were associated with ≥grade 2 CIPN in two studies of breast cancer patients treated with taxanes (46, 47). In 79 breast cancer patients treated with taxanes SNPs in NR1I3 and UGT2B7 involved in drug metabolism were also associated with CIPN (48). In 1,303 patients treated with taxanes, additional SNPs in genes involved in taxane metabolism including, CYP2C8*4 and CYP1B1*3, were associated with CIPN, as were, ABCB1 and SLCO1B1, involved in drug transport (42). In multiple myeloma patients treated with bortezomib, alterations in PSMB1, encoding drug binding proteins (55), and ABCC1 and ABCC6, encoding drug transport, were also associated with CIPN (52). However, in a separate study of 369 multiple myeloma patients ABCC1 polymorphisms were not associated with bortezomib-neuropathy, but were associated with vincristine-neuropathy, as was DPYD responsible for drug excertion (53). ABCC1 polymorphisms have also been associated with CIPN in pediatric ALL patients treated with vincristine (40, 50). Alterations in, ABCB1, encoding drug transport, has also been widely associated with CIPN in patients treated with vincristine and taxanes (44, 46, 49–51), and alterations in ABCC2 are associated with CIPN in children treated with vincristine (50). An alteration in ABCG2, involved in oxalate metabolism, was associated with oxaliplatin-induced neuropathy in 206 colon cancer patients (54).
Other Genetic Alterations Associated With CIPN
Genes involved in nervous system development and function, and in cellular repair pathways, have also been associated with CIPN.
Alterations in genes encoding ephrin receptors (EPHA4, EPHA5, EPHA6, EPHA8), a family of tyrosine kinase receptors involved in neural development, are associated with CIPN in patients treated with taxanes (62, 69–71, 81). An SNP in TAC1, encoding neuronal signaling hormones, was associated with CIPN in colon cancer patients treated with oxaliplatin (65). A polymorphisms in COCH, encoding cochlin involved in vestibular function and hearing loss, was associated with CIPN in a study of children with ALL treated with vincristine (82). Alterations in SOX10, involved in neuronal development, and GPX7 were associated with CIPN in 107 survivors of gynecologic cancers treated with taxane or platinum (67). Polymorphisms in genes involved in nervous system function, NFATC1, NFATC4, and EDN1 were associated with CIPN in 646 myeloma patients treated with bortezomib (52), as were TCF4, DYNC1I1, and GJE1 in 139 myeloma patients treated with bortezmib (55). GNGT1 encodes a protein in photoreceptors and has been associated with taxane-CIPN (68, 83).
Alterations in genes associated with DNA repair are also associated with CIPN. SNPs in CCNH, encoding cyclin H, involved in cell cycle progression and DNA repair (84), were associated with CIPN in 206 colon cancer patients (54), and in 228 gastrointestinal cancer patients treated with oxaliplatin (72). In myeloma patients treated with bortezomib, ERCC4 and ERCC3 involved in DNA repair were associated with CIPN (53). In a study of 680 testicular cancer survivors treated with cisplatin, lower expression of DNA repair gene RPRD1B, was associated with an increased risk of CIPN, which was replicated in two independent datasets (85).
Pharmacokinetics and CIPN
Evaluation of drug pharmacokinetics may be another promising approach to identifying CIPN sensitivity.
Taxane Pharmacokinetics
In 24 patients who received 12 weekly 3 or 1 h infusions of paclitaxel, longer duration of paclitaxel concentration >0.05 μM was associated with developing CIPN (86). In another prospective evaluation of 60 breast cancer patients receiving weekly paclitaxel infusions neither peak plasma concentration nor time above concentration of 0.05 μM were associated with CIPN, but were associated with increased toxicity-related treatment disruptions (87). An early study of lung cancer patients treated with paclitaxel also found no association between plasma concentration and neuromuscular or neurosensory outcomes (88).
Vincristine Pharmacokinetics
An early study of pharmacokinetics in 54 children treated with vincristine did not find any association between vincristine clearance and neurotixicy (89). In a subsequent study assessing pharmacokinetics of vincristine in 74 pediatric patients, lower vincristine metabolite concentrations were associated with increased CIPN severity (45). Another study assessed vincristine pharmacokinetics in 35 patients receiving vincristine via push or 1 h infusions and found intercompartment clearance of vincristine was associated with an increased risk of CIPN, however other pharmacokinetic measures including maximum concentration were not associated with an increased CIPN risk (90).
Discussion
We described promising biomarkers of CIPN in humans, including serum proteins, genetic polymorphisms, and drug metabolites. There are several limitations to the current studies and areas for future direction.
Serum protein biomarkers such as NfL, BDNF, NGF, osteopontin, and inflammatory markers have all been associated with CIPN, and may be easily translatable tools for detection and risk profiling in clinical practice (13, 18, 19, 24, 28, 91). However, these studies have been limited by small samples and variation in CIPN measurement between studies. Prospective validation studies of these biomarkers using objective CIPN measures would be helpful in confirming their clinical utility. Additionally, preclinical models should continue to be utilized to identify protein biomarkers that can be validated in humans.
Protein biomarkers can also inform therapeutic options that should continue to be explored. For example, in a study of 60 patients with bortezomib-neuropathy, patients randomized to receive NGF injections had better nerve conduction studies than those who did not receive NGF (92). In paclitaxel-treated rats, losartan had anti-inflammatory activity that resulted in lower inflammatory markers and decreased signs of CIPN (31). In addition to immune pathway targets, there are promising therapeutic targets that have been identified in critical CIPN pathways in animal models (73, 93). Neuronal Calcium Sensor-1 (NCS1), a protein involved in calcium signaling that binds taxanes and vinca alkaloids, decreases in CIPN in animal models (94–96). NCS1 may be a therapeutic target since lithium and ibudilast bind NCS1 and prevent CIPN in animal models (96), and retrospective studies show lithium may prevent CIPN in humans (97). Sterile alpha and TIR motif containing protein (SARM1) is another protein implicated in axonal degeneration in CIPN in preclinical models (73), and SARM1 inhibition may prevent CIPN (98, 99). Therefore, preclinical models can help better understand CIPN mechanisms, not only resulting in biomarker discovery that can be translated to the bedside, but also informing therapeutic strategies to prevent and mitigate CIPN that can be tested in humans.
Genetic polymorphisms are another avenue that offer promise in identifying individuals at risk for CIPN. CEP72 has been identified as a risk factor for CIPN in multiple studies (38, 39, 43), and may help classify upfront risk for toxicity. Future studies should focus on whether treatment modification in at risk individuals alters toxicity and survival outcomes. Other genome wide studies identified numerous polymorphisms that may influence CIPN sensitivity, but few were replicated in multiple cohorts, therefore future studies should focus on replicating these findings. Another limitation of genome wide studies is that they only identify proteins with altered expression, however in other models altered function, rather than expression, of cellular components are proposed to initiate CIPN (93). Future studies should continue to elucidate CIPN pathogenesis through complementary mechanisms of genome wide studies and functional pathway analyses to identify therapeutic targets to mitigate this outcome.
Finally, pharmacokinetics is an evolving way to assess drug response and CIPN susceptibility in individuals receiving neurotoxic chemotherapy. Although current studies report mixed results regarding the ability to identify individuals at risk for CIPN (45, 87, 90), it warrants further exploration. Monitoring individual plasma drug concentration could offer a novel method to ensure adequate dosing for cancer treatment while minimizing risk for CIPN.
A limitation across studies is that there is no standardized method to define CIPN. We found most studies used CTCAE for CIPN grading, which lacks sensitivity and can vary by evaluator (100–102). Patient-reported outcome measures for CIPN have been validated in adults, and may be more sensitive for detection and measurement of change over time than the CTCAE (101–103). However, patient-reported outcomes still have limitations and do not always correlate with clinical assessments (104). It is important that future biomarker research incorporate robust, validated measures for CIPN that ideally combine patient-report and clinical evaluations (104).
Overall, there are many promising biomarkers of CIPN that can be valuable tools to aid in detection, risk stratification, and drug development. Future studies should prioritize large-scale validation of these biomarkers using standardized instruments to measure CIPN and expedite their implementation into clinical practice.
Author Contributions
RR and ML contributed to concept, design, writing initial draft, editing final draft, and approval of final version. NS and BE contributed to writing initial draft, editing final draft, and approval of final version. All authors contributed to the article and approved the submitted version.
Funding
RR was supported by the National Cancer Institute through the Yale Cancer Prevention and Control Training Program (T32 CA250803), as well as the Yale Pediatric Scholar Program, the William O. Seery Mentored Research Award for Cancer Research, Bank of America, N.A., Trustee, and Hyundai Hope on Wheels Young Investigator Award.
Conflict of Interest
BE is a cofounder of Osmol Therapeutics, a company that is targeting NCS1 for therapeutic purposes.
The remaining authors declare that the research was conducted in the absence of any commercial or financial relationships that could be construed as a potential conflict of interest. ML is a consultant for Osmol Therapeutics.
Publisher's Note
All claims expressed in this article are solely those of the authors and do not necessarily represent those of their affiliated organizations, or those of the publisher, the editors and the reviewers. Any product that may be evaluated in this article, or claim that may be made by its manufacturer, is not guaranteed or endorsed by the publisher.
References
1. Bjornard KL, Gilchrist LS, Inaba H, Diouf B, Hockenberry MJ, Kadan-Lottick NS, et al. Peripheral neuropathy in children and adolescents treated for cancer. Lancet Child Adolesc Health. (2018) 2:744–54. doi: 10.1016/S2352-4642(18)30236-0
2. Kandula T, Farrar MA, Cohn RJ, Mizrahi D, Carey K, Johnston K, et al. Chemotherapy-induced peripheral neuropathy in long-term survivors of childhood cancer: clinical, neurophysiological, functional, and patient-reported outcomes. JAMA Neurol. (2018) 75:980–8. doi: 10.1001/jamaneurol.2018.0963
3. Eikeland SA, Smeland KB, Mols F, Fagerli UM, Bersvendsen HS, Kiserud CE, et al. Chemotherapy-induced peripheral neuropathy after modern treatment of Hodgkin's lymphoma; symptom burden and quality of life. Acta Oncol. (2021) 60:911–20. doi: 10.1080/0284186X.2021.1917776
4. van de Velde ME, van den Berg MH, Kaspers GJL, Abbink FCH, Twisk JWR, van der Sluis IM, et al. The association between vincristine-induced peripheral neuropathy and health-related quality of life in children with cancer. Cancer Med. (2021) 10:8172–81. doi: 10.1002/cam4.4289
5. Staff NP, Grisold A, Grisold W, Windebank AJ. Chemotherapy-induced peripheral neuropathy: a current review. Ann Neurol. (2017) 81:772–81. doi: 10.1002/ana.24951
6. Seretny M, Currie GL, Sena ES, Ramnarine S, Grant R, MacLeod MR, et al. Incidence, prevalence, and predictors of chemotherapy-induced peripheral neuropathy: a systematic review and meta-analysis. Pain. (2014) 155:2461–70. doi: 10.1016/j.pain.2014.09.020
7. Mohrmann C, Armer J, Hayashi RJ. Challenges evaluating chemotherapy-induced peripheral neuropathy in childhood cancer survivors. J Pediatr Oncol Nurs. (2017) 34:106–14. doi: 10.1177/1043454216651016
8. Haryani H, Fetzer SJ, Wu CL, Hsu YY. Chemotherapy-induced peripheral neuropathy assessment tools: a systematic review. Oncol Nurs Forum. (2017) 44:E111–e23. doi: 10.1188/17.ONF.E111-E123
9. Loprinzi CL, Lacchetti C, Bleeker J, Cavaletti G, Chauhan C, Hertz DL, et al. Prevention and management of chemotherapy-induced peripheral neuropathy in survivors of adult cancers: ASCO guideline update. J Clin Oncol. (2020) 38:3325–48. doi: 10.1200/JCO.20.01399
10. Meregalli C, Fumagalli G, Alberti P, Canta A, Carozzi VA, Chiorazzi A, et al. Neurofilament light chain as disease biomarker in a rodent model of chemotherapy induced peripheral neuropathy. Exp Neurol. (2018) 307:129–32. doi: 10.1016/j.expneurol.2018.06.005
11. Rosengren LE, Karlsson JE, Karlsson JO, Persson LI, Wikkelsø C. Patients with amyotrophic lateral sclerosis and other neurodegenerative diseases have increased levels of neurofilament protein in CSF. J Neurochem. (1996) 67:2013–8. doi: 10.1046/j.1471-4159.1996.67052013.x
12. Sandelius Å, Zetterberg H, Blennow K, Adiutori R, Malaspina A, Laura M, et al. Plasma neurofilament light chain concentration in the inherited peripheral neuropathies. Neurology. (2018) 90:e518–e24. doi: 10.1212/WNL.0000000000004932
13. Kim SH, Choi MK, Park NY, Hyun JW, Lee MY, Kim HJ, et al. Serum neurofilament light chain levels as a biomarker of neuroaxonal injury and severity of oxaliplatin-induced peripheral neuropathy. Sci Rep. (2020) 10:7995. doi: 10.1038/s41598-020-64511-5
14. Hofer MM, Barde YA. Brain-derived neurotrophic factor prevents neuronal death in vivo. Nature. (1988) 331:261–2. doi: 10.1038/331261a0
15. Lipsky RH, Marini AM. Brain-derived neurotrophic factor in neuronal survival and behavior-related plasticity. Ann N Y Acad Sci. (2007) 1122:130–43. doi: 10.1196/annals.1403.009
16. Azoulay D, Lavie D, Horowitz N, Suriu C, Gatt ME, Akria L, et al. Bortezomib-induced peripheral neuropathy is related to altered levels of brain-derived neurotrophic factor in the peripheral blood of patients with multiple myeloma. Br J Haematol. (2014) 164:454–6. doi: 10.1111/bjh.12624
17. Szudy-Szczyrek A, Mlak R, Bury-Kamińska M, Mielnik M, Podgajna M, Kuśmierczuk K, et al. Serum brain-derived neurotrophic factor (BDNF) concentration predicts polyneuropathy and overall survival in multiple myeloma patients. Br J Haematol. (2020) 191:77–89. doi: 10.1111/bjh.16862
18. Azoulay D, Nasser R, Sharon R, Simanovich L, Akria L, Shaoul E, et al. Brain derived neurotropic factor single nucleotide polymorphism Val66Met and serum protein levels are associated with development of vincristine-induced peripheral neuropathy in patients with lymphoma. Br J Haematol. (2019) 185:175–7. doi: 10.1111/bjh.15428
19. Azoulay D, Giryes S, Nasser R, Sharon R, Horowitz NA. Prediction of chemotherapy-induced peripheral neuropathy in patients with lymphoma and myeloma: the roles of brain-derived neurotropic factor protein levels and A gene polymorphism. J Clin Neurol. (2019) 15:511–6. doi: 10.3988/jcn.2019.15.4.511
20. Azoulay D, Leibovici A, Sharoni R, Shaoul E, Gross B, Braester A, et al. Association between Met-BDNF allele and vulnerability to paclitaxel-induced peripheral neuropathy. Breast Cancer Res Treat. (2015) 153:703–4. doi: 10.1007/s10549-015-3546-5
21. Leibovici A, Sharon R, Azoulay D. BDNF Val66Met is associated with pre-existing but not with paclitaxel-induced peripheral neuropathy in an Israeli cohort of breast cancer patients. Isr Med Assoc J. (2018) 20:746–8. https://www.ima.org.il/MedicineIMAJ/viewarticle.aspx?year=2018&month=12&page=746
22. Aloe L, Rocco ML, Bianchi P, Manni L. Nerve growth factor: from the early discoveries to the potential clinical use. J Transl Med. (2012) 10:239. doi: 10.1186/1479-5876-10-239
23. De Santis S, Pace A, Bove L, Cognetti F, Properzi F, Fiore M, et al. Patients treated with antitumor drugs displaying neurological deficits are characterized by a low circulating level of nerve growth factor. Clin Cancer Res. (2000) 6:90–5. https://aacrjournals.org/clincancerres/article/6/1/90/287777/Patients-Treated-with-Antitumor-Drugs-Displaying
24. Cavaletti G, Bogliun G, Marzorati L, Zincone A, Piatti M, Colombo N, et al. Early predictors of peripheral neurotoxicity in cisplatin and paclitaxel combination chemotherapy. Ann Oncol. (2004) 15:1439–42. doi: 10.1093/annonc/mdh348
25. Youk J, Kim YS, Lim JA, Shin DY, Koh Y, Lee ST, et al. Depletion of nerve growth factor in chemotherapy-induced peripheral neuropathy associated with hematologic malignancies. PLoS ONE. (2017) 12:e0183491. doi: 10.1371/journal.pone.0183491
26. Velasco R, Bruna J, Briani C, Argyriou AA, Cavaletti G, Alberti P, et al. Early predictors of oxaliplatin-induced cumulative neuropathy in colorectal cancer patients. J Neurol Neurosurg Psychiatry. (2014) 85:392–8. doi: 10.1136/jnnp-2013-305334
27. Castello LM, Raineri D, Salmi L, Clemente N, Vaschetto R, Quaglia M, et al. Osteopontin at the crossroads of inflammation and tumor progression. Mediators Inflamm. (2017) 2017:4049098. doi: 10.1155/2017/4049098
28. Agah E, Zardoui A, Saghazadeh A, Ahmadi M, Tafakhori A, Rezaei N. Osteopontin (OPN) as a CSF and blood biomarker for multiple sclerosis: a systematic review and meta-analysis. PLoS ONE. (2018) 13:e0190252. doi: 10.1371/journal.pone.0190252
29. Wright MC, Mi R, Connor E, Reed N, Vyas A, Alspalter M, et al. Novel roles for osteopontin and clusterin in peripheral motor and sensory axon regeneration. J Neurosci. (2014) 34:1689–700. doi: 10.1523/JNEUROSCI.3822-13.2014
30. Pizzamiglio C, Ripellino P, Prandi P, Clemente N, Saggia C, Rossi V, et al. Nerve conduction, circulating osteopontin and taxane-induced neuropathy in breast cancer patients. Neurophysiol Clin. (2020) 50:47–54. doi: 10.1016/j.neucli.2019.12.001
31. Kalynovska N, Diallo M, Sotakova-Kasparova D, Palecek J. Losartan attenuates neuroinflammation and neuropathic pain in paclitaxel-induced peripheral neuropathy. J Cell Mol Med. (2020) 24:7949–58. doi: 10.1111/jcmm.15427
32. Krukowski K, Eijkelkamp N, Laumet G, Hack CE, Li Y, Dougherty PM, et al. CD8+ T cells and endogenous IL-10 are required for resolution of chemotherapy-induced neuropathic pain. J Neurosci. (2016) 36:11074–83. doi: 10.1523/JNEUROSCI.3708-15.2016
33. Yamanouchi K, Kuba S, Sakimura C, Morita M, Kanetaka K, Kobayashi K, et al. The relationship between peripheral neuropathy induced by docetaxel and systemic inflammation-based parameters in patients with breast cancer. Anticancer Res. (2017) 37:6947–51. doi: 10.21873/anticanres.12160
34. Kleckner IR, Jusko TA, Culakova E, Chung K, Kleckner AS, Asare M, et al. Longitudinal study of inflammatory, behavioral, clinical, and psychosocial risk factors for chemotherapy-induced peripheral neuropathy. Breast Cancer Res Treat. (2021) 189:521–32. doi: 10.1007/s10549-021-06304-6
35. Peng Q, Mechanic J, Shoieb A, Pardo ID, Schaevitz L, Fenyk-Melody J, et al. Circulating microRNA and automated motion analysis as novel methods of assessing chemotherapy-induced peripheral neuropathy in mice. PLoS ONE. (2019) 14:e0210995. doi: 10.1371/journal.pone.0210995
36. Benbow JH, Mann T, Keeler C, Fan C, Hodsdon ME, Lolis E, et al. Inhibition of paclitaxel-induced decreases in calcium signaling. J Biol Chem. (2012) 287:37907–16. doi: 10.1074/jbc.M112.385070
37. Chen EI, Crew KD, Trivedi M, Awad D, Maurer M, Kalinsky K, et al. Identifying predictors of taxane-induced peripheral neuropathy using mass spectrometry-based proteomics technology. PLoS ONE. (2015) 10:e0145816. doi: 10.1371/journal.pone.0145816
38. Diouf B, Crews KR, Lew G, Pei D, Cheng C, Bao J, et al. Association of an inherited genetic variant with vincristine-related peripheral neuropathy in children with acute lymphoblastic leukemia. JAMA. (2015) 313:815–23. doi: 10.1001/jama.2015.0894
39. Stock W, Diouf B, Crews KR, Pei D, Cheng C, Laumann K, et al. An inherited genetic variant in CEP72 promoter predisposes to vincristine-induced peripheral neuropathy in adults with acute lymphoblastic leukemia. Clin Pharmacol Ther. (2017) 101:391–5. doi: 10.1002/cpt.506
40. Wright GEB, Amstutz U, Drogemoller BI, Shih J, Rassekh SR, Hayden MR, et al. Pharmacogenomics of vincristine-induced peripheral neuropathy implicates pharmacokinetic and inherited neuropathy genes. Clin Pharmacol Ther. (2019) 105:402–10. doi: 10.1002/cpt.1179
41. Kavčič M, Zečkanović A, Jazbec J, Debeljak M. Association of CEP72 rs924607 TT genotype with vincristine-induced peripheral neuropathy measured by motor nerve conduction studies. Klin Padiatr. (2020) 232:331–3. doi: 10.1055/a-1213-5995
42. Abraham JE, Guo Q, Dorling L, Tyrer J, Ingle S, Hardy R, et al. Replication of genetic polymorphisms reported to be associated with taxane-related sensory neuropathy in patients with early breast cancer treated with Paclitaxel. Clin Cancer Res. (2014) 20:2466–75. doi: 10.1158/1078-0432.CCR-13-3232
43. Park SB, Kwok JB, Asher R, Lee CK, Beale P, Selle F, et al. Clinical and genetic predictors of paclitaxel neurotoxicity based on patient-versus clinician-reported incidence and severity of neurotoxicity in the ICON7 trial. Ann Oncol. (2017) 28:2733–40. doi: 10.1093/annonc/mdx491
44. Ceppi F, Langlois-Pelletier C, Gagné V, Rousseau J, Ciolino C, De Lorenzo S, et al. Polymorphisms of the vincristine pathway and response to treatment in children with childhood acute lymphoblastic leukemia. Pharmacogenomics. (2014) 15:1105–16. doi: 10.2217/pgs.14.68
45. Egbelakin A, Ferguson MJ, MacGill EA, Lehmann AS, Topletz AR, Quinney SK, et al. Increased risk of vincristine neurotoxicity associated with low CYP3A5 expression genotype in children with acute lymphoblastic leukemia. Pediatr Blood Cancer. (2011) 56:361–7. doi: 10.1002/pbc.22845
46. Kus T, Aktas G, Kalender ME, Demiryurek AT, Ulasli M, Oztuzcu S, et al. Polymorphism of CYP3A4 and ABCB1 genes increase the risk of neuropathy in breast cancer patients treated with paclitaxel and docetaxel. Onco Targets Ther. (2016) 9:5073–80. doi: 10.2147/OTT.S106574
47. Hertz DL, Roy S, Motsinger-Reif AA, Drobish A, Clark LS, McLeod HL, et al. CYP2C8*3 increases risk of neuropathy in breast cancer patients treated with paclitaxel. Ann Oncol. (2013) 24:1472–8. doi: 10.1093/annonc/mdt018
48. Arbitrio M, Scionti F, Altomare E, Di Martino MT, Agapito G, Galeano T, et al. Polymorphic variants in NR1I3 and UGT2B7 predict taxane neurotoxicity and have prognostic relevance in patients with breast cancer: a case-control study. Clin Pharmacol Ther. (2019) 106:422–31. doi: 10.1002/cpt.1391
49. Tanabe Y, Shimizu C, Hamada A, Hashimoto K, Ikeda K, Nishizawa D, et al. Paclitaxel-induced sensory peripheral neuropathy is associated with an ABCB1 single nucleotide polymorphism and older age in Japanese. Cancer Chemother Pharmacol. (2017) 79:1179–86. doi: 10.1007/s00280-017-3314-9
50. Lopez-Lopez E, Gutierrez-Camino A, Astigarraga I, Navajas A, Echebarria-Barona A, Garcia-Miguel P, et al. Vincristine pharmacokinetics pathway and neurotoxicity during early phases of treatment in pediatric acute lymphoblastic leukemia. Pharmacogenomics. (2016) 17:731–41. doi: 10.2217/pgs-2016-0001
51. Sissung TM, Baum CE, Deeken J, Price DK, Aragon-Ching J, Steinberg SM, et al. ABCB1 genetic variation influences the toxicity and clinical outcome of patients with androgen-independent prostate cancer treated with docetaxel. Clin Cancer Res. (2008) 14:4543–9. doi: 10.1158/1078-0432.CCR-07-4230
52. Campo C, Da Silva Filho MI, Weinhold N, Goldschmidt H, Hemminki K, Merz M, et al. Genetic susceptibility to bortezomib-induced peripheral neuroropathy: replication of the reported candidate susceptibility loci. Neurochem Res. (2017) 42:925–31. doi: 10.1007/s11064-016-2007-9
53. Broyl A, Corthals SL, Jongen JL, van der Holt B, Kuiper R, de Knegt Y, et al. Mechanisms of peripheral neuropathy associated with bortezomib and vincristine in patients with newly diagnosed multiple myeloma: a prospective analysis of data from the HOVON-65/GMMG-HD4 trial. Lancet Oncol. (2010) 11:1057–65. doi: 10.1016/S1470-2045(10)70206-0
54. Custodio A, Moreno-Rubio J, Aparicio J, Gallego-Plazas J, Yaya R, Maurel J, et al. Pharmacogenetic predictors of severe peripheral neuropathy in colon cancer patients treated with oxaliplatin-based adjuvant chemotherapy: a GEMCAD group study. Ann Oncol. (2014) 25:398–403. doi: 10.1093/annonc/mdt546
55. Favis R, Sun Y, van de Velde H, Broderick E, Levey L, Meyers M, et al. Genetic variation associated with bortezomib-induced peripheral neuropathy. Pharmacogenet Genomics. (2011) 21:121–9. doi: 10.1097/FPC.0b013e3283436b45
56. Tanabe Y, Shiraishi S, Hashimoto K, Ikeda K, Nishizawa D, Hasegawa J, et al. Taxane-induced sensory peripheral neuropathy is associated with an SCN9A single nucleotide polymorphism in Japanese patients. BMC Cancer. (2020) 20:325. doi: 10.1186/s12885-020-06834-0
57. Sereno M, Gutiérrez-Gutiérrez G, Rubio JM, Apellániz-Ruiz M, Sánchez-Barroso L, Casado E, et al. Genetic polymorphisms of SCN9A are associated with oxaliplatin-induced neuropathy. BMC Cancer. (2017) 17:63. doi: 10.1186/s12885-016-3031-5
58. Palugulla S, Thakkar DN, Kayal S, Narayan SK, Dkhar SA. Association of voltage-gated sodium channel genetic polymorphisms with oxaliplatin-induced chronic peripheral neuropathy in south indian cancer patients. Asian Pac J Cancer Prev. (2017) 18:3157–65. doi: 10.22034/APJCP.2017.18.11.3157
59. Argyriou AA, Cavaletti G, Antonacopoulou A, Genazzani AA, Briani C, Bruna J, et al. Voltage-gated sodium channel polymorphisms play a pivotal role in the development of oxaliplatin-induced peripheral neurotoxicity: results from a prospective multicenter study. Cancer. (2013) 119:3570–7. doi: 10.1002/cncr.28234
60. Schneider BP, Li L, Radovich M, Shen F, Miller KD, Flockhart DA, et al. Genome-wide association studies for taxane-induced peripheral neuropathy in ECOG-5103 and ECOG-1199. Clin Cancer Res. (2015) 21:5082–91. doi: 10.1158/1078-0432.CCR-15-0586
61. Chen Y, Fang F, Kidwell KM, Vangipuram K, Marcath LA, Gersch CL, et al. Genetic variation in Charcot-Marie-Tooth genes contributes to sensitivity to paclitaxel-induced peripheral neuropathy. Pharmacogenomics. (2020) 21:841–51. doi: 10.2217/pgs-2020-0053
62. Baldwin RM, Owzar K, Zembutsu H, Chhibber A, Kubo M, Jiang C, et al. A genome-wide association study identifies novel loci for paclitaxel-induced sensory peripheral neuropathy in CALGB 40101. Clin Cancer Res. (2012) 18:5099–109. doi: 10.1158/1078-0432.CCR-12-1590
63. Beutler AS, Kulkarni AA, Kanwar R, Klein CJ, Therneau TM, Qin R, et al. Sequencing of Charcot-Marie-Tooth disease genes in a toxic polyneuropathy. Ann Neurol. (2014) 76:727–37. doi: 10.1002/ana.24265
64. Boora GK, Kulkarni AA, Kanwar R, Beyerlein P, Qin R, Banck MS, et al. Association of the Charcot-Marie-Tooth disease gene ARHGEF10 with paclitaxel induced peripheral neuropathy in NCCTG N08CA (Alliance). J Neurol Sci. (2015) 357:35–40. doi: 10.1016/j.jns.2015.06.056
65. Won HH, Lee J, Park JO, Park YS, Lim HY, Kang WK, et al. Polymorphic markers associated with severe oxaliplatin-induced, chronic peripheral neuropathy in colon cancer patients. Cancer. (2012) 118:2828–36. doi: 10.1002/cncr.26614
66. Li L, Sajdyk T, Smith EML, Chang CW, Li C, Ho RH, et al. Genetic variants associated with vincristine-induced peripheral neuropathy in two populations of children with acute lymphoblastic leukemia. Clin Pharmacol Ther. (2019) 105:1421–8. doi: 10.1002/cpt.1324
67. Thomaier L, Darst BF, Jewett P, Hoffmann C, Brown K, Makaram A, et al. Genetic variants predictive of chemotherapy-induced peripheral neuropathy symptoms in gynecologic cancer survivors. Gynecol Oncol. (2021) 163:578–82. doi: 10.1016/j.ygyno.2021.10.006
68. Sucheston-Campbell LE, Clay-Gilmour AI, Barlow WE, Budd GT, Stram DO, Haiman CA, et al. Genome-wide meta-analyses identifies novel taxane-induced peripheral neuropathy-associated loci. Pharmacogenet Genomics. (2018) 28:49–55. doi: 10.1097/FPC.0000000000000318
69. Leandro-García LJ, Inglada-Pérez L, Pita G, Hjerpe E, Leskelä S, Jara C, et al. Genome-wide association study identifies ephrin type A receptors implicated in paclitaxel induced peripheral sensory neuropathy. J Med Genet. (2013) 50:599–605. doi: 10.1136/jmedgenet-2012-101466
70. Marcath LA, Kidwell KM, Vangipuram K, Gersch CL, Rae JM, Burness ML, et al. Genetic variation in EPHA contributes to sensitivity to paclitaxel-induced peripheral neuropathy. Br J Clin Pharmacol. (2020) 86:880–90. doi: 10.1111/bcp.14192
71. Apellániz-Ruiz M, Tejero H, Inglada-Pérez L, Sánchez-Barroso L, Gutiérrez-Gutiérrez G, Calvo I, et al. Targeted sequencing reveals low-frequency variants in EPHA genes as markers of paclitaxel-induced peripheral neuropathy. Clin Cancer Res. (2017) 23:1227–35. doi: 10.1158/1078-0432.CCR-16-0694
72. Palugulla S, Devaraju P, Kayal S, Narayan SK, Mathaiyan J. Genetic polymorphisms in cyclin H gene are associated with oxaliplatin-induced acute peripheral neuropathy in South Indian digestive tract cancer patients. Cancer Chemother Pharmacol. (2018) 82:421–8. doi: 10.1007/s00280-018-3629-1
73. Staff NP, Fehrenbacher JC, Caillaud M, Damaj MI, Segal RA, Rieger S. Pathogenesis of paclitaxel-induced peripheral neuropathy: a current review of in vitro and in vivo findings using rodent and human model systems. Exp Neurol. (2020) 324:113121. doi: 10.1016/j.expneurol.2019.113121
74. Gutierrez-Camino A, Martin-Guerrero I, Lopez-Lopez E, Echebarria-Barona A, Zabalza I, Ruiz I, et al. Lack of association of the CEP72 rs924607 TT genotype with vincristine-related peripheral neuropathy during the early phase of pediatric acute lymphoblastic leukemia treatment in a Spanish population. Pharmacogenet Genomics. (2016) 26:100–2. doi: 10.1097/FPC.0000000000000191
75. Zgheib NK, Ghanem KM, Tamim H, Aridi C, Shahine R, Tarek N, et al. Genetic polymorphisms in candidate genes are not associated with increased vincristine-related peripheral neuropathy in Arab children treated for acute childhood leukemia: a single institution study. Pharmacogenet Genomics. (2018) 28:189–95. doi: 10.1097/FPC.0000000000000345
76. Martin-Guerrero I, Gutierrez-Camino A, Echebarria-Barona A, Astigarraga I, Garcia de Andoin N, Navajas A, et al. Variants in vincristine pharmacodynamic genes involved in neurotoxicity at induction phase in the therapy of pediatric acute lymphoblastic leukemia. Pharmacogenomics J. (2019) 19:1–6. doi: 10.1038/s41397-019-0081-5
77. Zajaczkowska R, Kocot-Kepska M, Leppert W, Wrzosek A, Mika J, Wordliczek J. Mechanisms of chemotherapy-induced peripheral neuropathy. Int J Mol Sci. (2019) 20:1451. doi: 10.3390/ijms20061451
78. Argyriou AA, Antonacopoulou AG, Alberti P, Briani C, Bruna J, Velasco R, et al. Liability of the voltage-gated potassium channel KCNN3 repeat polymorphism to acute oxaliplatin-induced peripheral neurotoxicity. J Peripher Nerv Syst. (2019) 24:298–303. doi: 10.1111/jns.12347
79. Peters CM, Jimenez-Andrade JM, Jonas BM, Sevcik MA, Koewler NJ, Ghilardi JR, et al. Intravenous paclitaxel administration in the rat induces a peripheral sensory neuropathy characterized by macrophage infiltration and injury to sensory neurons and their supporting cells. Exp Neurol. (2007) 203:42–54. doi: 10.1016/j.expneurol.2006.07.022
80. Vashistha B, Sharma A, Jain V. Ameliorative potential of ferulic acid in vincristine-induced painful neuropathy in rats: an evidence of behavioral and biochemical examination. Nutr Neurosci. (2017) 20:60–70. doi: 10.1179/1476830514Y.0000000165
81. Flanagan JG, Vanderhaeghen P. The ephrins and Eph receptors in neural development. Annu Rev Neurosci. (1998) 21:309–45. doi: 10.1146/annurev.neuro.21.1.309
82. Li H, Sisoudiya SD, Martin-Giacalone BA, Khayat MM, Dugan-Perez S, Marquez-Do DA, et al. Germline cancer-predisposition variants in pediatric rhabdomyosarcoma: a report from the children's oncology group. J Natl Cancer Inst. (2021) 113:875–83. doi: 10.1093/jnci/djaa204
83. Scherer SW, Feinstein DS, Oliveira L, Tsui LC, Pittler SJ. Gene structure and chromosome localization to 7q213 of the human rod photoreceptor transducin gamma-subunit gene (GNGT1). Genomics. (1996) 35:241–3. doi: 10.1006/geno.1996.0346
84. Lolli G, Johnson LN. CAK-Cyclin-dependent Activating Kinase: a key kinase in cell cycle control and a target for drugs? Cell Cycle. (2005) 4:572–7. doi: 10.4161/cc.4.4.1607
85. Dolan ME, El Charif O, Wheeler HE, Gamazon ER, Ardeshir-Rouhani-Fard S, Monahan P, et al. Clinical and genome-wide analysis of cisplatin-induced peripheral neuropathy in survivors of adult-onset cancer. Clin Cancer Res. (2017) 23:5757–68. doi: 10.1158/1078-0432.CCR-16-3224
86. Mielke S, Sparreboom A, Steinberg SM, Gelderblom H, Unger C, Behringer D, et al. Association of Paclitaxel pharmacokinetics with the development of peripheral neuropathy in patients with advanced cancer. Clin Cancer Res. (2005) 11:4843–50. doi: 10.1158/1078-0432.CCR-05-0298
87. Hertz DL, Kidwell KM, Vangipuram K, Li F, Pai MP, Burness M, et al. Paclitaxel plasma concentration after the first infusion predicts treatment-limiting peripheral neuropathy. Clin Cancer Res. (2018) 24:3602–10. doi: 10.1158/1078-0432.CCR-18-0656
88. Rowinsky EK, Jiroutek M, Bonomi P, Johnson D, Baker SD. Paclitaxel steady-state plasma concentration as a determinant of disease outcome and toxicity in lung cancer patients treated with paclitaxel and cisplatin. Clin Cancer Res. (1999) 5:767–74.
89. Crom WR, de Graaf SS, Synold T, Uges DR, Bloemhof H, Rivera G, et al. Pharmacokinetics of vincristine in children and adolescents with acute lymphocytic leukemia. J Pediatr. (1994) 125:642–9. doi: 10.1016/S0022-3476(94)70027-3
90. van de Velde ME, Panetta JC, Wilhelm AJ, van den Berg MH, van der Sluis IM, van den Bos C, et al. Population pharmacokinetics of vincristine related to infusion duration and peripheral neuropathy in pediatric oncology patients. Cancers. (2020) 12:1789. doi: 10.3390/cancers12071789
91. Apfel SC, Kessler JA. Neurotrophic factors in the therapy of peripheral neuropathy. Baillieres Clin Neurol. (1995) 4:593–606.
92. Yan M, Li Y, Zeng H, Zhao X, Wu H, Qian W, et al. The effect of rat nerve growth factor combined with vitamin B on peripheral neuropathy in multiple myeloma patients. Hematology. (2020) 25:264–9. doi: 10.1080/16078454.2020.1784615
93. Ibrahim EY, Ehrlich BE. Prevention of chemotherapy-induced peripheral neuropathy: a review of recent findings. Crit Rev Oncol Hematol. (2020) 145:102831. doi: 10.1016/j.critrevonc.2019.102831
94. Boehmerle W, Splittgerber U, Lazarus MB, McKenzie KM, Johnston DG, Austin DJ, et al. Paclitaxel induces calcium oscillations via an inositol 1,4,5-trisphosphate receptor and neuronal calcium sensor 1-dependent mechanism. Proc Natl Acad Sci USA. (2006) 103:18356–61. doi: 10.1073/pnas.0607240103
95. Boehmerle W, Zhang K, Sivula M, Heidrich FM, Lee Y, Jordt S-E, et al. Chronic exposure to paclitaxel diminishes phosphoinositide signaling by calpain-mediated neuronal calcium sensor-1 degradation. Proc Natl Acad Sci USA. (2007) 104:11103–8. doi: 10.1073/pnas.0701546104
96. Mo M, Erdelyi I, Szigeti-Buck K, Benbow JH, Ehrlich BE. Prevention of paclitaxel-induced peripheral neuropathy by lithium pretreatment. FASEB J. (2012) 26:4696–709. doi: 10.1096/fj.12-214643
97. Wadia RJ, Stolar M, Grens C, Ehrlich BE, Chao HH. The prevention of chemotherapy induced peripheral neuropathy by concurrent treatment with drugs used for bipolar disease: a retrospective chart analysis in human cancer patients. Oncotarget. (2018) 9:7322–31. doi: 10.18632/oncotarget.23467
98. Gould SA, White M, Wilbrey AL, Pór E, Coleman MP, Adalbert R. Protection against oxaliplatin-induced mechanical and thermal hypersensitivity in Sarm1(-/-) mice. Exp Neurol. (2021) 338:113607. doi: 10.1016/j.expneurol.2021.113607
99. Chen YH, Sasaki Y, DiAntonio A, Milbrandt J. SARM1 is required in human derived sensory neurons for injury-induced and neurotoxic axon degeneration. Exp Neurol. (2021) 339:113636. doi: 10.1016/j.expneurol.2021.113636
100. Gilchrist LS, Marais L, Tanner L. Comparison of two chemotherapy-induced peripheral neuropathy measurement approaches in children. Support Care Cancer. (2014) 22:359–66. doi: 10.1007/s00520-013-1981-6
101. Park SB, Alberti P, Kolb NA, Gewandter JS, Schenone A, Argyriou AA. Overview and critical revision of clinical assessment tools in chemotherapy-induced peripheral neurotoxicity. J Peripher Nerv Syst. (2019) 24(Suppl. 2):S13–s25. doi: 10.1111/jns.12333
102. Molassiotis A, Cheng HL, Leung KT, Li YC, Wong KH, Au JSK, et al. Risk factors for chemotherapy-induced peripheral neuropathy in patients receiving taxane- and platinum-based chemotherapy. Brain Behav. (2019) 9:e01312. doi: 10.1002/brb3.1312
103. Tan AC, McCrary JM, Park SB, Trinh T, Goldstein D. Chemotherapy-induced peripheral neuropathy-patient-reported outcomes compared with NCI-CTCAE grade. Support Care Cancer. (2019) 27:4771–7. doi: 10.1007/s00520-019-04781-6
Keywords: chemotherapy-induced peripheral neuropathy (CIPN), paclitaxel, vincristine, bortezomib, biomarkers, oxaliplatin
Citation: Rodwin RL, Siddiq NZ, Ehrlich BE and Lustberg MB (2022) Biomarkers of Chemotherapy-Induced Peripheral Neuropathy: Current Status and Future Directions. Front. Pain Res. 3:864910. doi: 10.3389/fpain.2022.864910
Received: 29 January 2022; Accepted: 16 February 2022;
Published: 14 March 2022.
Edited by:
Alexandre Chan, University of California, Irvine, United StatesReviewed by:
Paola Alberti, University of Milano-Bicocca, ItalyCopyright © 2022 Rodwin, Siddiq, Ehrlich and Lustberg. This is an open-access article distributed under the terms of the Creative Commons Attribution License (CC BY). The use, distribution or reproduction in other forums is permitted, provided the original author(s) and the copyright owner(s) are credited and that the original publication in this journal is cited, in accordance with accepted academic practice. No use, distribution or reproduction is permitted which does not comply with these terms.
*Correspondence: Maryam B. Lustberg, bWFyeWFtLmx1c3RiZXJnQHlhbGUuZWR1