- 1Division of Medical Genetics, Department of Pediatrics, King Abdulaziz Medical City, Ministry of National Guard Health Affairs (MNG-HA), Riyadh, Saudi Arabia
- 2Medical Genomics Research Department, King Abdullah International Medical Research Center (KAIMRC), King Saud Bin Abdulaziz University for Health Sciences, Ministry of National Guard Health Affairs (MNGH), Riyadh, Saudi Arabia
- 3College of Medicine, King Saud bin Abdulaziz University for Health Sciences, King Abdulaziz Medical City, Ministry of National Guard Health Affairs (MNG-HA), Riyadh, Saudi Arabia
- 4Division of Neurology, Department of Pediatrics, King Abdullah Specialist Children's Hospital, King Abdulaziz Medical City, Ministry of National Guard Health Affairs (MNG-HA), Riyadh, Saudi Arabia
- 5Section of Medical Genetics, Children's Specialist Hospital, King Fahad Medical City, Riyadh, Saudi Arabia
- 6Medical Genetics Division, Department of Pediatrics, College of Medicine, King Saud University, Riyadh, Saudi Arabia
- 7Division of Neurology, Department of Pediatrics, College of Medicine, King Saud University, Riyadh, Saudi Arabia
- 8Pathology and Laboratory Medicine, King Abdulaziz Medical City, Riyadh, Saudi Arabia
- 9Center for Genetics and Inherited Diseases, Taibah University, Almadinah Almunwarah, Saudi Arabia
- 10Faculty of Applied Medical Sciences, Taibah University, Almadinah Almunwarah, Saudi Arabia
- 11Division of Genetics, Department of Pediatrics, Prince Sultan Military Medical City, Riyadh, Saudi Arabia
- 12Department of Anatomy and Cell Biology, College of Medicine, Alfaisal University, Riyadh, Saudi Arabia
- 13Division of Neurology, Department of Pediatrics, Prince Sultan Military Medical City, Riyadh, Saudi Arabia
- 14CENTOGENE AG, Rostock, Germany
- 15Department of Pediatrics, Almulyda, Saudi Arabia. King Abdullah International Medical Research Center (KAIMRC), Qassim University, Riyadh, Saudi Arabia
Background: Leukodystrophies (LDs) are inherited heterogeneous conditions that affect the central nervous system with or without peripheral nerve involvement. They are individually rare, but collectively, they are common. Thirty disorders were included by the Global Leukodystrophy Initiative Consortium (GLIA) as LDs.
Methods: We conducted a retrospective chart review of a consecutive series of patients diagnosed with different types of LD from four large tertiary referral centers in Riyadh, Saudi Arabia. Only those 30 disorders defined by GLIA as LDs were included.
Results: In total, 83 children from 61 families were identified and recruited for this study. The male-to-female ratio was 1.5:1, and a consanguinity rate of 58.5% was observed. An estimated prevalence of 1:48,780 or 2.05/100,000 was observed based on the clinical cohort, whereas a minimum of 1:32,857 or 3.04/100,000 was observed based on the local genetic database. The central region of the country exhibited the highest prevalence of LDs (48.5%). The most common LD was metachromatic leukodystrophy (MLD), and it accounted for 25.3%. The most common disorder based on carrier frequency was AGS. Novel variants were discovered in 51% of the cases, but 49% possessed previously reported variants. Missense variants were high in number and accounted for 73% of all cases. Compared with other disorders, MLD due to saposin b deficiency was more common than expected, Pelizaeus-Merzbacher-like disease was more prevalent than Pelizaeus-Merzbacher disease, and X-linked adrenoleukodystrophy was less common than expected. The mortality rate among our patients with LD was 24%.
Conclusion: To the best of our knowledge, this is the largest cohort of patients with LD from Saudi Arabia. We present epidemiological, clinical, radiological, and genetic data. Furthermore, we report 18 variants that have not been reported previously. These findings are of great clinical and molecular utility for diagnosing and managing patients with LD.
Introduction
Leukodystrophies (LDs) are inherited heterogeneous conditions affecting the central nervous system with or without peripheral nerve involvement (1, 2). They are individually rare but are collectively common. Their prevalence is not yet known because of challenges in diagnosis; however, it is estimated to be in the range of 1:7663–1:50,000 (1). The exact definition of LD is not well-described and is further complicated by the existence of other LD terms used in the literature (2). In addition to primary hereditary LD, this condition can be secondary to vascular, infectious, toxic, and other insults to the white matter. In 2015, 13 experts representing the Global Leukodystrophy Initiative Consortium (GLIA) used a modified Delphi-based approach to achieve a case definition of LDs. Thirty disorders were included in this category (Table 1) (2).
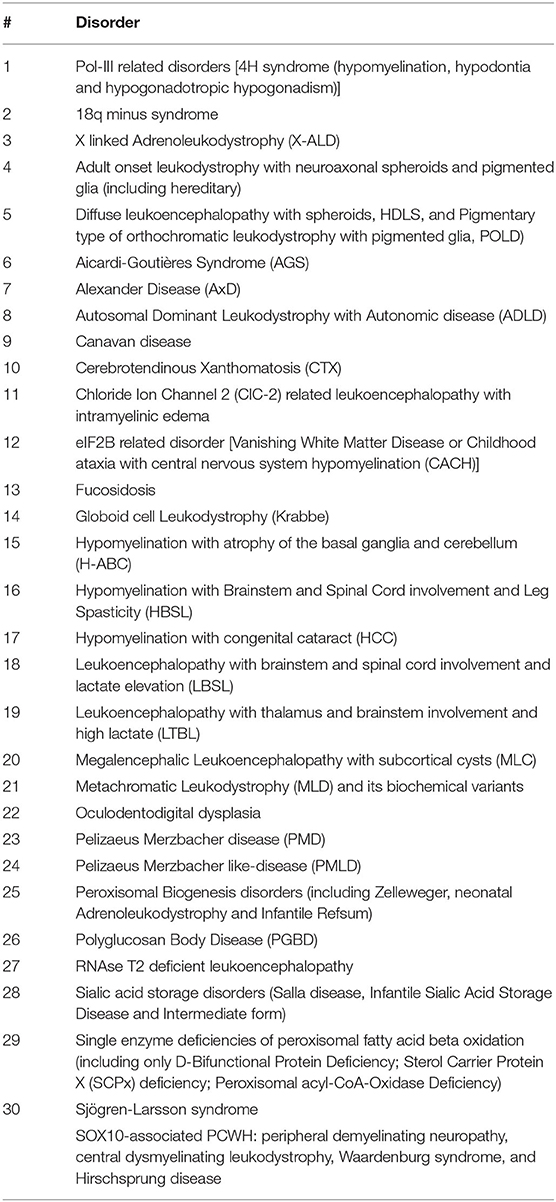
Table 1. Thirty disorders classified as leukodystrophy by Global Leukodystrophy Initiative Consortium (GLIA).
The clinical features of LD can present at or shortly after birth for some types of LDs, whereas others develop later in childhood, adolescence, or even during adulthood. All LDs share selective white matter abnormalities upon neuroradiological imaging of the brain. White matter deterioration occurs concurrently with clinical neuroregression of the associated skills. Several LDs are degenerative; however, some are limited to white matter function impairment. The clinical course is heterogeneous in nature; some patients experience progressive disease, and others may have static disease or exhibit improvement over time. The typical clinical presentation in childhood is neurological and mainly affects motor functions. This is dissimilar to primary neuronal disorders that present initially with cognitive defects and epilepsy (3, 4). The common scenarios include but are not limited to neuroregression in a proband after normal development with loss of milestones and hypotonia that progresses to peripheral spasticity, optic atrophy, and global developmental delay. The initial symptoms in older children can manifest as frequent falls or a clumsy gait. Adult-onset LD is largely similar to child-onset LD with typical progressive cognitive or neuropsychiatric difficulties. Cerebral defects are characterized by changes in personality, emotional disturbances, impaired attention, and memory loss. Several LDs possess specific clinical features, such as X-linked adrenoleukodystrophy (X-ALD), that is characterized by adrenal insufficiency, patients possessing metachromatic leukodystrophy (MLD), Krabbe disease that is characterized by peripheral neuropathy, and macrocephaly that occurs in Canavan and Alexander diseases. Patients with 4H syndrome suffer from hypogonadotropic hypogonadism. Optic atrophy is notable in Canavan disease and vanishing white matter disease, whereas brain calcification is common in Aicardi-Goutieres syndrome (AGS) (3, 4).
In this study, we report the largest cohort of patients with LD in Saudi Arabia. We estimated the prevalence, common LD types, and radiological and molecular data associated with LD.
Methods
Ethical Approval
The study was approved by the Institutional Review Board of King Abdullah International Medical Research Centre (KAIMRC), Riyadh (Ref. RC19/120/R). Written informed consent for the publication of related data was obtained from the parents of the enrolled patients.
Patients
This is a retrospective chart review study of a consecutive series of patients diagnosed with different types of LD from four large tertiary referral centers in Riyadh, Saudi Arabia (January 2015 to January 2020). Patient records were reviewed to collect data on variables that included clinical diagnoses, number of patients, sex, age of onset, age at diagnosis, current age, neuroregression, global developmental delay, seizure and its types, magnetic resonance imaging (MRI) findings, long-term outcomes, and genetic results. The standard threshold criteria were applied to diagnose LD, and these included neurological clinical presentation in addition to white matter abnormalities in MRI of the brain. These diagnoses were also confirmed using DNA molecular genetic testing.
Genetic Testing
The diagnosis of LD was confirmed by whole exome sequencing (WES) or whole genome sequencing (WGS) of genomic DNA for the majority of patients, but a small number of patients were diagnosed according to relevant gene panels or single gene testing. All molecular genetic studies were performed by accredited commercial laboratories, such as CENTOGENE (https://www.centogene.com/). Cases with negative gene panels were reflexed to WES/WGS as described elsewhere (5, 6). The variants were classified according to the guidelines of the ACMG (PMID: 25741868). A minor allele frequency of <0.001 was selected based on our internal database (1,410 exomes), and 13,180 WES/WGS were performed at CENTOGENE (https://www.centogene.com/), the Genome Aggregation Database (gnomAD), and Exome Aggregation Consortium (ExAC) databases. Additionally, the variants should be fully segregated within available family members. Variants obtained after WES/WGS were analyzed and filtered using standard methods (Supplementary Figure 1). All discovered variants were confirmed by bidirectional Sanger sequencing following standard methods. For missense variants, in silico analysis tools, including Polyphen2 (http://www.genetics.bwh.harvard.edu/pph2/), SIFT (http://sift.bii.a-star.edu.sg/), Varsome (https://varsome.com/), and MutationTaster (http://www.mutationtaster.org/), were used to predict the pathogenicity of the variants. For splice site variants, Human Splicing Finder (HSF; http://www.umd.be/HSF3/index.html) was used as a prediction tool.
Results
Cohort Description
A total of 83 children from 61 families were identified and recruited for this retrospective study. The consanguinity rate among the study participants was 58.5%. The age of LD onset ranged from 0 to 7 years, age at diagnosis ranged from 0 to 19 years, and age at follow-up ranged from 0.3 to 25 years. Tables 2, 3 summarize the clinical and genetic information for all of the cases. The estimated prevalence of LD was 1:48,780 or 2.05/100,000. Upon reviewing our in-house genome database of 1,410 individuals, the estimated prevalence was calculated to be in the range of 1:32,857 to 3.04/100,000. Common clinical features included global developmental delay (97.5%), neuroregression (40%), and seizure (50%). The types of seizures included generalized tonic-clonic, focal colonic, myoclonic, and infantile spasms.
Clinical and Molecular Landscapes of LD
The most common LD disorder in our cohort was MLD, which represented 25.30% of the cases. The other ordered distribution of other LD included leukoencephalopathy with vanishing white matter (VWM; 13.25%), peroxisome biogenesis disorders (PBD; 12.04%), AGS (10.84%), Krabbe disease (KD; 8.43%), Pelizaeus-Merzbacher-like disease (PMLD; 7.22%), megalencephalic leukoencephalopathy with subcortical cyst (MLC; 6.02%), Canavan disease (CD; 4.81%), X-ALD (3.61%), Alexandar disease (AD; 2.40%), Pelizaeus-Merzbacher disease (2.40%), Salla disease (SD; 2.40%), and oculodentodigital dysplasia (1.20%; Figure 1A). The central region of the country exhibited the highest prevalence of LD (48.5%). The ordered regional distribution of LD included the South Region (21%), North Region (15%), Eastern Province (10.5%), and Western regions (5%; Figure 2). However, based on our local genetic database combined with other available databases, the ordered carrier frequency of various LDs was estimated to be the highest in the Saudi population, and these LDs included AGS, MLD, leukoencephalopathy with brainstem and spinal cord involvement, and lactate elevation (LBSL, OMIM#611105), PBD, and VWM diseases (Table 2). Interestingly, polyglucosan body disease (PGBD; OMIM #263570) was present in the database but not in the clinical cohort, in which the estimated mean allele frequency of the identified pathogenic variant in GBE1 (NM_000158.4: c.998A>T; p.[Glu333Val)] was 15 in 100,000. Similarly, LBSL with identified pathogenic variants in DARS2 (NM_018122.4: c.1762C>G; p.[Leu588Val] was present with a mean allele frequency of 57 in 100,000.
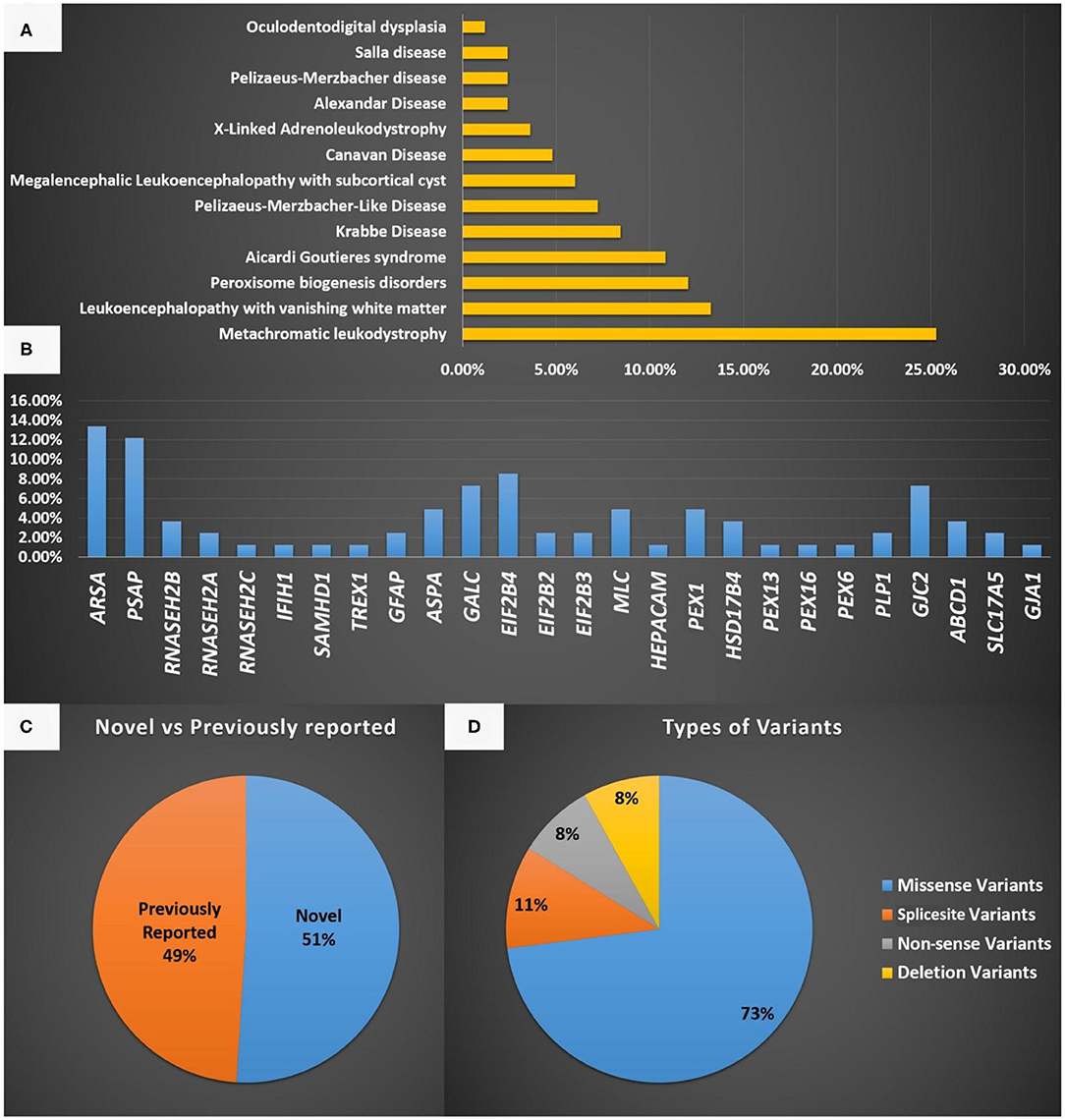
Figure 1. (A) A representation of the different LD disorders included in our cohort and their percentages. (B) The genes reported in the LD disorder cohort in the present study. (C) Representation of novel and previously reported variants identified in the present study and the type of mutations identified. (D) Represent the type of variants identified in the present study.
The most common gene defects in the clinical cohort occurred in ARSA and PSAP and accounted for 13.25 and 12.04% of the cases, respectively. The most common gene for VWM was EIF2B4, for PBD was PEX1, for AGS was RNASEH2B, and for MLC was MLC1 (Figure 1B).
Novel variants were discovered in 51% of the cases, whereas variants in 49% of the cases were previously reported. The most common variants were missense (73%), splice site (10.8%), non-sense (8.1%), and deletion variants (8.1%; Figures 1C,D). Most of the variants were detected in autosomal recessive genes, whereas only five variants were detected in autosomal dominant genes (13.5%). This is consistent with the high rate of consanguinity observed in our cohort (58.5%). All the identified novel variants were highly conserved across multiple species. All variants identified in the present study were uploaded to the public repository LOVD (https://www.lovd.nl/2.0/index.php).
Radiological Spectrum of LD
Brain MRI findings (Table 3; Figures 3A–H) revealed diffuse white matter disorders with demyelination in MLD, X-ALD, KD, and AD. A tigroid pattern was found in MLD and VWM disease. Periventricular predominance was observed in the MLD and KD groups. Parieto-occipital predominance was observed in X-ALD with post-gadolinium peripheral enhancement. Hypomyelination was observed in patients with SD. White matter disorders with vacuolization were found in patients with CD, VWM disease, and MLC. Brain calcification was present in the AGS and Zellweger syndrome patients. Hyperintensity in the peritrigonal white matter on both sides with splenium involvement of the corpus callosum and thinning of the corpus callosum was observed in PBD.
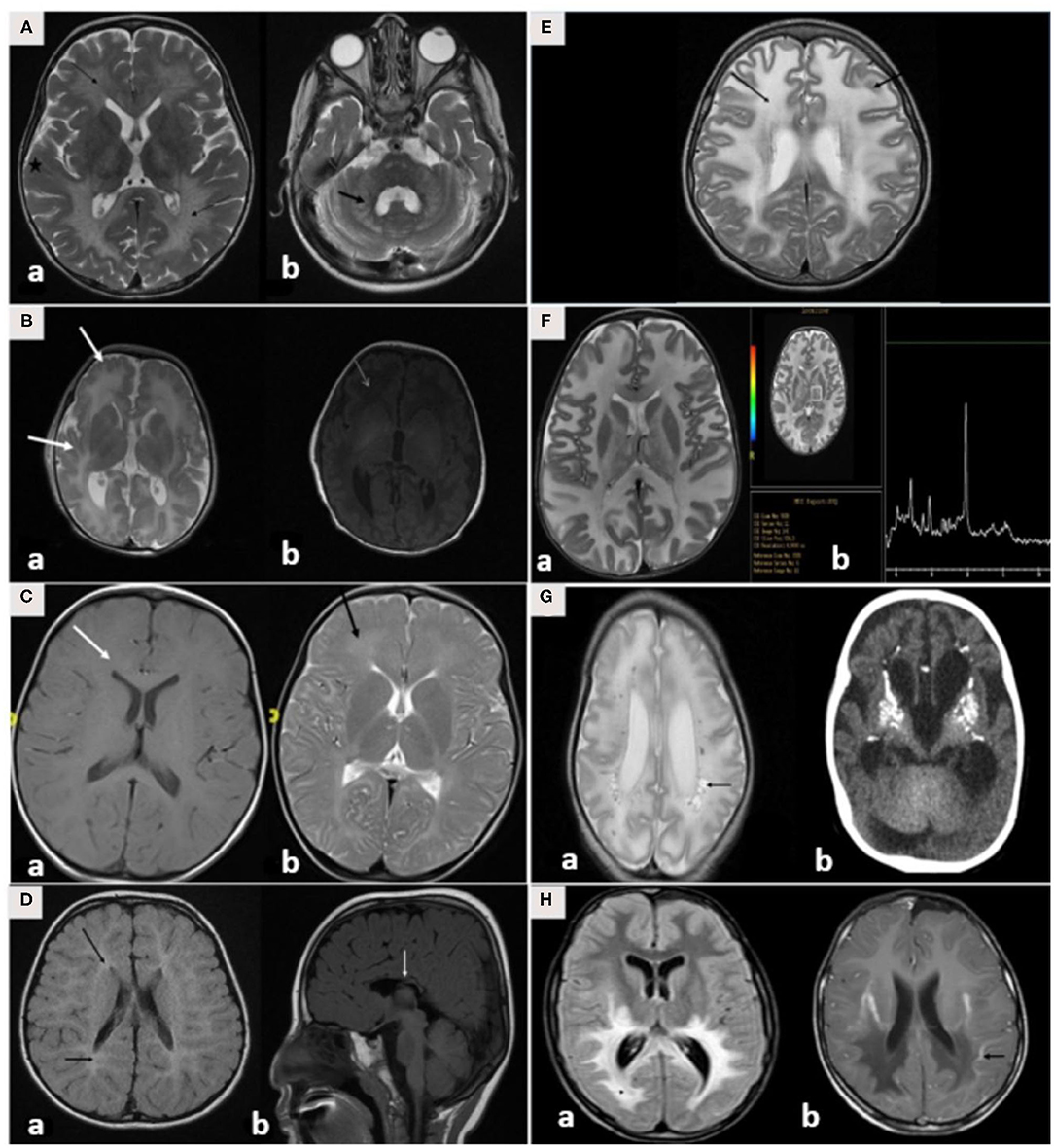
Figure 3. (A–H) MRI/CT brain of patients included in this study. (A) Brain MRI of a 30-month-old boy with metachromatic leukodystrophy. (a) Axial T2WI demonstrates bilateral symmetrical confluent areas of periventricular deep white matter signal change (thin arrow) with sparing of subcortical U-fibers (star). Note the classic tigroid pattern. (b) Axial T2WI exhibits bilateral involvement of the cerebellar white matter (thick arrow) and the pons. (B) Brain MRI of a 10-day-old boy with Zellweger syndrome. (a) Axial T2WI shows malformation of the cerebral cortex in both sylvian fissures (thick arrow) extending into the pericentral cortex and right parietal cortex as well as at the mesial aspect of the hemispheres in the form of a polymicrogyric pattern. (b) Axial T1WI shows an excessive hypointensity of the deep and subcortical white matter and also noted in the bilateral frontoparietal white matter (thin arrow). (C) Brain MRI of a 3-year-old boy with GJC2-hypomyelating leukodystrophy. (a) Axial T1WI shows iso/low signal intensity of the white matter (white arrow). (b) Axial T2WI exhibits an increased signal intensity of cerebral hemispheric white matter (black arrow). (D) Brain MRI of a 4-year-old boy with Salla disease. (a) FLAIR-WI demonstrates abnormal periventricular white matter signals (black arrow). (b) Sagittal T1WI shows thin dysplastic corpus callosum (white arrow). (E) Brain MRI of 11-year-old girl with megaloencephalic leukodystrophy with subcortical cysts. Axial T2WI shows diffuse superficial and deep white matter disease (thin arrow) with subcortical cysts (thick arrow). (F) Brain MRI of a 13-month-old boy with Canavan disease. (a) Axial T2WI demonstrates diffuse high signal intensity in the subcortical, deep, and periventricular white matter. The abnormalities also involve both globi pallidi, the thalami, and the posterior portion of the corpus callosum. (b) MR spectroscopy demonstrates increased N-acetylaspartate (NAA) peak intensity at 200 ms. (G) A 7-month-old-boy with Aicardi-Goutieres syndrome. (a) Brain MRI with axial T2WI at 29 days of age shows diffuse white matter with cystic changes in the periventricular areas (black arrow). (b) CT brain at the age of 6 months shows diffuse calcification in the basal ganglia. (H) Brain MRI of a 7-year-old boy with X-linked adrenoleukodystrophy. (a) Axial FLAIR-WI demonstrates extensive symmetrical white matter changes in the parieto-occipital region (thin arrow). (b) Gadolinium T1WI shows peripheral enhancement (thick arrow).
Discussion
Herein, we report the largest cohort to date of LD in Saudi Arabia. Most LD cases were recruited from hospitals in the Riyadh region (Figure 2). The referral centers in this study were based in Riyadh, the capital city of Saudi Arabia. However, as inferred from their tribal origins, specific LD was prevalent in other regions. For example, CD was more prevalent in the Jazan region, PMLD was more common in the Qassim region, and MLD (due to saposin b deficiency) was more common in Dawadmi city (Figure 2).
Interestingly, the most common disorder in the clinical cohort is MLD; however, the most common disorder based on the carrier frequency of our local Saudi genome database is AGS. However, if we add the previously published data regarding AGS by Al Mutairi et al. (7) to this cohort, AGS becomes the most common LD in Saudi Arabia (8). MLD due to saposin b deficiency is more common in our population than it is in the other populations as it accounted for 12% of the total LDs.
PSAP defects are rare, with fewer than 30 cases reported in the literature from Italy, Turkey, India, Mexico, Canada, the Czech Republic, and Nigeria (9). Remarkably, this disease was ranked among the highest by both our clinical cohort and the local genome data, thus supporting its prevalence in Saudi Arabia. Of note, PMLD was more common than was PM. However, the prevalence of X-ALD was lower than expected in the present study.
In the present study, we estimated a minimum prevalence of 2.2/100,000 to 3.1/100,000 in Saudi Arabia. The incidence of LD is estimated to be 2.0/100,000 in Germany, 3.1/100,000 in the United Kingdom, 0.5/100,000 in France, 2.5/100,000 in the United States, and 1/100,000 in the Netherlands (9–11); however, we believe that the reported prevalence in this study is underestimated as the current study is limited to pediatric patients from hospitals located in Riyadh only.
Unsurprisingly, this study reports a high rate of novel variants (51%) as this is the first relatively large-scale report of LDs from Saudi Arabia. In correlation with the clinical, segregation, and biochemical data, the pathogenic effects of all identified novel variants were further supported by several online in silico prediction tools. Additionally, all of these novel variants were absent or very rare in general population databases and were not found in our in-house 2000 WES and WGS. Furthermore, all identified variants were perfectly segregated with other family members.
Naidu et al. (8) classified LD into four major categories that included hypomyelinating disorders (HMDs) that are defined as a lack of myelin accumulation, demyelinating LD that is defined by the loss of previously formed myelin, dysmyelinating disorders that possess the deposition of biochemically or structurally abnormal myelin, and myelinolytic diseases that are characterized by the vacuolization of the myelin (12). These categorical classifications of LD were based on the mechanism of white matter injury.
Subsequently, Van der Knaap and Bugiani (13) propose a classification that depends predominantly upon the primary involvement of any white matter constituent. The categories in this classification are myelin disorders due to a primary defect in oligodendrocytes or myelin (hypomyelinating and demyelinating LDs or LDs with myelin vacuolization), leuko-axonopathies, microgliopathies, leukovasculopathies, and astrocytopathies (13). Finally, Vanderver et al. (2) defined LD based on the Delphi approach, and they included 30 disorders as LDs.
We prefer to follow Vanderver's classification in the present study. This made our study more systematic and resulted in the exclusion of important diseases that were among Van der Knaap's classification (2). For example, multiple sulfatase deficiency is not included in the GLIA classification. We suggest that multiple sulfatase deficiencies should be included as a type of LD as it is a demyelinating disease. Multiple sulfatase deficiency is an autosomal recessive disorder caused by formylglycine-generating enzyme deficiency due to SUMF1 defects. The typical clinical presentation is developmental regression, periventricular white matter disease, intellectual disability, and ichthyosis. Hijazi et al. (14) reported the presence of this disease in six children from Saudi Arabia ranging from 5 to 13 years of age, indicating that the disease is not uncommon in the Saudi population (14). Taken together, heterogeneity in the LD spectrum causes the estimation of their collective prevalence to become very difficult and to be underestimated worldwide. The clinical features and MRI findings of the current cohort were similar to those reported previously (Table 3; Figure 3).
A pattern recognition approach using brain MRI in addition to detailed history and examination would be very useful to achieve a diagnosis. Three brain MRI findings can differentiate between different types of LD. The first is the presence or absence of hypomyelination, and this is defined as no change in the MRI pattern of deficient myelination in at least two MRIs (6 months apart) in children older than 1 year. Second, to determine if the white matter defects are isolated, multifocal, or confluent. Multifocal changes indicate acquired causes, such as structural chromosomal disorders, infectious diseases, and vasculopathies, whereas confluent abnormalities indicate LD. Finally, the predominant localization of white matter abnormalities, such as parieto-occipital predominance in X-ALD, periventricular abnormalities in MLD, subcortical in CD, and diffuse cerebral in VWM diseases, must be assessed (3).
The diagnosis is achieved through a detailed clinical history, examination, and brain MRI. MRI patterns help differentiate among different types of LD. Additionally, biochemical abnormalities such as metabolites and enzyme assays are very useful tools (e.g., very long chain fatty acids for X-ALD and arylsulfatase A enzyme assays in patients with MLD) (3, 4). However, a definitive diagnosis is achieved through molecular testing and identification of pathogenic variants in the culprit genes. The advent of WES and WGS has led to an improvement in the diagnostic yield of molecular testing in LD and a significant decrease in the percentage of unsolved cases (<30%) (15). Additionally, WES and WGS provide a unique opportunity for the discovery of candidate genes such as ISCA1 and ISCA2 (12, 16, 17).
Treatments are typically supportive, and there are no curative treatments for LD. Patients and families require frequent counseling sessions, discussions regarding the importance of family screening, preventive measures for future pregnancies, and the establishment of multidisciplinary team care (4, 18).
Anecdotal data exist for specific diseases, but in general, this is not the case for LD. Al-Hassnan et al. (19) reported nine children from four unrelated Saudi families who were diagnosed with metachromatic LD due to sphingolipid activator protein B deficiency (19). Additionally, our group described 24 patients with AGS (7). However, the incidence, prevalence, and genetic landscape of LD in Saudi Arabia remain unknown.
Various promising therapeutic approaches currently exist in experimental settings with potential clinical translational utilities, including enzyme-replacement therapy, bone marrow transplantation, gene therapy by ex vivo transplantation of genetically modified hematopoietic stem cells and adeno-associated virus (AAV)-mediated gene therapy delivered to the CNS (20). Several clinical trials are currently underway that involve injecting genes via intraparenchymal, intracerebroventricular, and intracisternal routes using AAV. This method expresses the protein locally, persistently, and permanently through the use of a one-time administration (20).
Conclusion
To the best of our knowledge, this is the largest cohort of LD from Saudi Arabia that presents epidemiological, clinical, radiological, and genetic data. Furthermore, we report 18 novel variants in 61 families that were not previously associated with LD. National and international registries are required to further delineate the epidemiological, clinical, radiological, and genetic data of the LD spectrum. Sharing the genotypic and phenotypic data of a large molecularly characterized LD cohort will improve the diagnostic rate of LD patients in the targeted population and beyond. Future multicentric studies will undoubtedly improve our understanding of the natural history of these diseases and facilitate evidence-based guidelines stipulating the disease pathogenesis that may lead to the design of new therapies and prompt treatment.
Data Availability Statement
The datasets presented in this study can be found in online repositories. The names of the repository/repositories and accession number(s) can be found at: https://www.lovd.nl/, Individual #00358887; https://www.lovd.nl/, Individual #00335517; https://www.lovd.nl/, Individual #00334384; https://www.lovd.nl/, Individual #00335515; https://www.lovd.nl/, Individual #00335516; https://www.lovd.nl/, Individual #00335518; https://www.lovd.nl/, Individual #00358876.
Ethics Statement
The studies involving human participants were reviewed and approved by KAIMRC. Written informed consent to participate in this study was provided by the participants' legal guardian/next of kin. Written informed consent was obtained from all participating family members (or parents in the case of minors) for publication of this research and any accompanying clinical data and images after a genetic counsellor explained the nature and possible consequences of the study to them.
Author Contributions
MAlf: performed the majority of work associated with preparing, writing and submitting the manuscript and contributed to the clinical diagnosis and management of the patients from KASCH, KAMC. MAlma, FAM, MU, MAlg, HA, RA, FB, AAls, WE, WAlt, and AAlf: edited the manuscript, collected the data and contributed to the clinical diagnosis and management of the patients from KASCH, KAMC. MAlma, MS, AA-A, and EF: collected the data and contributed to the clinical diagnosis and management of the patients from KFMC. MAlg: collected the data and contributed to the clinical diagnosis and management of the patients from KKUH. FA and WAls: edited the manuscript, collected the data and contributed to the clinical diagnosis and management of the patients from KKUH. DB-A and AA-R: edited the manuscript, collected the data and contributed to the clinical diagnosis and management of neurological features of the patients. MB: edited the manuscript, collected the molecular genetic data. NA: collected the data and interpret WES data from KFMC. AAlh, AAls, KH, and BT: edited the manuscript collected the genetic data from Prince Sultan Military Medical City. AB-A, PB, and CB: edited the manuscript, collected the genome data from CENTOGEN, Germany. MAlmu: performed clinical evaluation of patients and collected clinical data. The final version of the manuscript read and approved by all named authors.
Funding
This study was funded by King Abdullah International Medical Research Center (KAIMRC).
Conflict of Interest
The authors declare that the research was conducted in the absence of any commercial or financial relationships that could be construed as a potential conflict of interest.
Acknowledgments
We are thankful to the patients and their families.
Supplementary Material
The Supplementary Material for this article can be found online at: https://www.frontiersin.org/articles/10.3389/fped.2021.633385/full#supplementary-material
Supplementary Figure 1. WGS/WES variant filtration steps.
Abbreviations
LD, Leukodystrophies; MLD, Metachromatic leukodystrophy; VWM, leukoencephalopathy with vanishing white matter; PBD, Peroxisome biogenesis disorders; AGS, Aicardi Goutieres syndrome; LBSL, Leukoencephalopathy with brainstem and spinal cord involvement and lactate elevation; PMLD, Pelizaeus-Merzbacher like disease; GLIA, Global Leukodystrophy Initiative Consortium.
References
1. Kufs H, Lange-Cosack H, Suckow J. [Clinical observations, histopathology and hereditary pathology in a family with familial juvenile diffuse sclerosis (leukodystrophia cerebri hereditaria progressiva)]. Psychiatr Neurol Med Psychol (Leipz). (1954) 6:12–24.
2. Vanderver A, Prust M, Tonduti D, Mochel F, Hussey HM, Helman G, et al. Case definition and classification of leukodystrophies and leukoencephalopathies. Mol Genet Metab. (2015) 114:494–500. doi: 10.1016/j.ymgme.2015.01.006
3. Parikh S, Bernard G, Leventer RJ, van der Knaap MS, van Hove J, Pizzino A, et al. A clinical approach to the diagnosis of patients with leukodystrophies and genetic leukoencephelopathies. Mol Genet Metab. (2015) 114:501–15. doi: 10.1016/j.ymgme.2014.12.434
4. Costello DJ, Eichler AF, Eichler FS. Leukodystrophies: classification, diagnosis, and treatment. Neurologist. (2009) 15:319–28. doi: 10.1097/NRL.0b013e3181b287c8
5. Trujillano D, Bertoli-Avella AM, Kumar Kandaswamy K, Weiss MER, Köster J, Marais A, et al. Clinical exome sequencing: results from 2819 samples reflecting 1000 families. Eur J Hum Genet. (2017) 25:176–82. doi: 10.1038/ejhg.2016.146
6. Alfares A, Alfadhel M, Wani T, Alsahli S, Alluhaydan I, Mutairi FA, et al. A multicenter clinical exome study in unselected cohorts from a consanguineous population of Saudi Arabia demonstrated a high diagnostic yield. Mol Genet Metab. (2017) 121:91–5. doi: 10.1016/j.ymgme.2017.04.002
7. Al Mutairi F, Alfadhel M, Nashabat M, El-Hattab AW, Ben-Omran T, Hertecant J, et al. Phenotypic and molecular spectrum of aicardi-goutieres syndrome: a study of 24 patients. Pediatr Neurol. (2018) 78:35–40. doi: 10.1016/j.pediatrneurol.2017.09.002
9. van Geel BM, Assies J, Weverling GJ, Barth PG. Predominance of the adrenomyeloneuropathy phenotype of X-linked adrenoleukodystrophy in The Netherlands: a survey of 30 kindreds. Neurology. (1994) 44:2343–6. doi: 10.1212/WNL.44.12.2343
10. Sereni C, Paturneau-Jouas M, Aubourg P, Baumann N, Feingold J. Adrenoleukodystrophy in France: an epidemiological study. Neuroepidemiology. (1993) 12:229–33. doi: 10.1159/000110321
11. Stellitano LA, Winstone AM, van der Knaap MS, Verity CM. Leukodystrophies and genetic leukoencephalopathies in childhood: a national epidemiological study. Dev Med Child Neurol. (2016) 58:680–9. doi: 10.1111/dmcn.13027
12. Shukla A, Hebbar M, Srivastava A, Kadavigere R, Upadhyai P, Kanthi A, et al. Homozygous p.(Glu87Lys) variant in ISCA1 is associated with a multiple mitochondrial dysfunctions syndrome. J Hum Genet. (2017) 62:723–7. doi: 10.1038/jhg.2017.35
13. van der Knaap MS, Bugiani M. Leukodystrophies: a proposed classification system based on pathological changes and pathogenetic mechanisms. Acta Neuropathol. (2017) 134:351–82. doi: 10.1007/s00401-017-1739-1
14. Hijazi L, Kashgari A, Alfadhel M. Multiple Sulfatase Deficiency: A Case Series With a Novel Mutation. J Child Neurol. (2018) 33:820–4. doi: 10.1177/0883073818790851
15. Vanderver A, Simons C, Helman G, Crawford J, Wolf NI, Bernard G, et al. Whole exome sequencing in patients with white matter abnormalities. Ann Neurol. (2016) 79:1031–7. doi: 10.1002/ana.24650
16. Alfadhel M. Multiple mitochondrial dysfunctions syndrome 4 due to ISCA2 gene defects: a review. Child Neurol Open. (2019) 6:2329048X19847377. doi: 10.1177/2329048X19847377
17. Alfadhel M, Nashabat M, Alrifai MT, Alshaalan H, Al Mutairi F, Al-Shahrani SA, et al. Further delineation of the phenotypic spectrum of ISCA2 defect: a report of ten new cases. Eur J Paediatr Neurol. (2018) 22:46–55. doi: 10.1016/j.ejpn.2017.10.003
18. Heim P, Claussen M, Hoffmann B, Conzelmann E, Gärtner J, Harzer K, Hunneman DH, et al. Leukodystrophy incidence in Germany. Am J Med Genet. (1997) 71:475–8. doi: 10.1002/(SICI)1096-8628(19970905)71:4<475::AID-AJMG20>3.0.CO;2-C
19. Al-Hassnan ZN, Al Dhalaan H, Patay Z, Faqeih E, Al-Owain M, Al-Duraihem A, et al. Sphingolipid activator protein B deficiency: report of 9 Saudi patients and review of the literature. J Child Neurol. (2009) 24:1513–9. doi: 10.1177/0883073809341269
Keywords: leukodystrophy, Saudi Arabia, neurometabolic, metachromatic leukodystrophy, novel mutations
Citation: Alfadhel M, Almuqbil M, Al Mutairi F, Umair M, Almannai M, Alghamdi M, Althiyab H, Albarakati R, Bashiri FA, Alshuaibi W, Ba-Armah D, Saleh MA, Al-Asmari A, Faqeih E, Altuwaijri W, Al-Rumayyan A, Balwi MA, Ababneh F, Alswaid AF, Eyaid WM, Almontashiri NAM, Alhashem A, Hundallah K, Bertoli-Avella A, Bauer P, Beetz C, Alrifai MT, Alfares A and Tabarki B (2021) The Leukodystrophy Spectrum in Saudi Arabia: Epidemiological, Clinical, Radiological, and Genetic Data. Front. Pediatr. 9:633385. doi: 10.3389/fped.2021.633385
Received: 25 November 2020; Accepted: 22 March 2021;
Published: 13 May 2021.
Edited by:
Marco Carotenuto, University of Campania Luigi Vanvitelli, ItalyReviewed by:
Michele Roccella, University of Palermo, ItalyAhmad Farooq, International Islamic University, Pakistan
Copyright © 2021 Alfadhel, Almuqbil, Al Mutairi, Umair, Almannai, Alghamdi, Althiyab, Albarakati, Bashiri, Alshuaibi, Ba-Armah, Saleh, Al-Asmari, Faqeih, Altuwaijri, Al-Rumayyan, Balwi, Ababneh, Alswaid, Eyaid, Almontashiri, Alhashem, Hundallah, Bertoli-Avella, Bauer, Beetz, Alrifai, Alfares and Tabarki. This is an open-access article distributed under the terms of the Creative Commons Attribution License (CC BY). The use, distribution or reproduction in other forums is permitted, provided the original author(s) and the copyright owner(s) are credited and that the original publication in this journal is cited, in accordance with accepted academic practice. No use, distribution or reproduction is permitted which does not comply with these terms.
*Correspondence: Majid Alfadhel, ZHJhbGZhZGhlbG1AZ21haWwuY29t