- 1Department of Pediatrics, Morsani College of Medicine, University of South Florida, Tampa, FL, United States
- 2College of Public Health, University of South Florida, Tampa, FL, United States
- 3Department of Anthropology, College of Arts and Sciences, University of South Florida, Tampa, FL, United States
Background: Preterm infants are at high risk for growth failure and childhood weight problems due to the disruption of normal intrauterine growth and nutrition. Early nutritional support and microbiome acquisition can play an important role in childhood growth.
Objective: Our study examined potential postnatal indicators, including gut bacterial compositions, macronutrients, and catch-up growth, of growth pattern from infancy into early childhood.
Methods: This is a retrospective study of preterm infants born < 35 weeks who were followed up in the university complex care clinic from 2012–2018. Weight and length z-scores at birth, 1, 2, 4, 6, 12 and 15 months, and body mass index (BMI) and length z-scores from 2 to 5 years of age were collected. Catch-up growths were calculated by changes in z-scores and divided into early (birth-4 months) and late (4–18 months). Postnatal nutritional data and fecal samples were collected. Fecal microbiome data obtained from 16S RNA V4 sequencing was analyzed against clinical and growth data using a regression model.
Results: 160 infants included in the final analysis had birth weight and gestational age of 1,149 ± 496 grams and 28 ± 3 weeks. Early weight gain positively correlated with length z-scores but not with BMI at 2 years of age. BMI at 2 years of age strongly correlated with BMI at 3, 4, and 5 years of age. Postnatal abundance of Gammaproteobacteria was negatively associated with early growth while Bacteroides and Lactobacillus were positively associated with childhood BMI.
Conclusion: Our findings suggest that optimal postnatal nutrition promoted early catch-up growth in weight as well as improved linear growth without influence on childhood BMI. Postnatal gut microbial colonization, which is a modifiable factor, was associated with childhood growth in preterm infants.
Introduction
Due to prematurity and compromised intrauterine environment, preterm infants commonly experience growth deficit, which often presents as a low birth weight for age and/or growth failure occurring shortly after birth. Combating this growth deficit is important for the long-term outcomes of these infants. The trajectory of post-natal growth of preterm infants correlates with childhood growth patterns and cognitive development (1). Insufficient growth can negatively impact immune, gastrointestinal, and neurological development. Further, some studies have demonstrated that timing of catch-up growth is critical for optimal development and missed opportunities may not be reversible (2). Catch-up growth is a phase of rapid linear growth that allows an infant to accelerate toward and resume their pre-restriction growth curve. Although catch-up growth could help preterm infants reach their ideal anthropometrics, many preterm infants experience post-natal growth failure. Understanding the relationships among early nutritional support, gut microbiota, and childhood growth as well as identifying predictors of childhood growth assist clinicians to optimize early postnatal nutritional care.
To assess growth from birth into late adolescence and early adulthood, the latest World Health Organization (WHO) guidelines provide seamless standards and references (3). Preterm growth is measured using the Fenton Growth charts, which transition to the WHO Childhood Growth Charts (4, 5). Changes in growth can be assessed through change in z scores to indicate height and weight changes in reference to the WHO Childhood Growth Standards. Body mass index (BMI), a simple index of weight-for-height, is commonly used to classify overweight and obesity. To gain a better understanding of the growth trajectory during infancy, growth can be classified as early from birth—4 months of age, and late, from 4–18 months of age (6, 7).
The postnatal growth failure that preterm infants often experience has been linked to the immaturity of gut microbiota (8, 9). The microbiota of infants experiencing growth failure has been previously characterized by low diversity, increased abundance of Staphylococcaceae and Enterobacteriaceae, and lack of Veillonella as compared to infants with appropriate growth (8). Nutrition in early infancy plays an important role in both growth and bacterial colonization of the gut (10). The composition of the microbiota is vital in the breakdown of food and utilization of nutrients to support growth during infancy. The early bacterial colonizers of the infant gut help regulate future changes throughout childhood (11). However, there is no consensus on which bacterial taxa predispose these infants to growth deficit. Determining which bacterial taxa aid and inhibit catch-up growth in preterm infants is important to optimize healthy childhood anthropometrics.
Few studies have investigated the role early microbial acquisition on childhood growth among preterm infants. Thus far, early microbiome volatility, potentially indicating greater bacterial diversity, correlates with improved growth in length at ages 2 through 4 (12). Identifying factors that can help combat post-natal growth deficit of preterm infants is critical to their later development. This study aims to examine the relationship among early postnatal nutrition and microbiome, infancy growth, and early childhood BMI. These relationships may identify early life predictors of optimal childhood growth for preterm infants.
Materials and methods
Patient selection and data collection
This was a retrospective study of infants who were born with birth gestational age (GA) < 35 weeks, admitted to the level 3 academic neonatal intensive care unit (NICU) at Tampa General Hospital between January 1, 2012 to December 31, 2018, and had at least one well child visit between 2 and 5 years of age at the University of South Florida (USF) Complex Care Clinic. Exclusion criteria were congenital anomalies or chromosomal disorders. The study was approved by the USF Institutional Review Board.
The data were collected from both inpatient and outpatient electronic medical records of the infants on NICU admission and outpatient care. The collected demographic and clinical information included birth weight (BW), birth gestational age (GA), gender, ethnicity, race, maternal age, intrauterine growth restriction (IUGR) and small for gestational age (SGA) status, maternal comorbidities (hypertension, preeclampsia, diabetes mellitus), maternal BMI, multiple gestations, NICU length of stay (LOS), grade 3 or 4 intraventricular hemorrhage (IVH), necrotizing enterocolitis (NEC), and chronic lung disease (CLD) (defined as oxygen requirement at 36 weeks corrected gestational age). We collected detailed information on nutritional supports during their NICU admissions and their first 5 years of life, such as the daily caloric intake, days to full feeds, and macronutrient supplementations—protein and medium-chain triglycerides (MCT). The standard caloric concentration is 24 kcal/oz for preterm infants born < 32 weeks and daily caloric concentration > 24 kcal/oz was considered high caloric concentration.
Growth curves and catch-up growth
We recorded each infant’s anthropometric data from birth to 5 years of age including weekly weight and length along with their z-scores on the revised Fenton growth charts up to 2 months chronological age and on WHO growth charts thereafter, and their BMI at ages 2–5 years. For children under 5 years of age, overweight is defined as BMI greater than 1 standard deviations and obesity is defined as BMI greater than 2 standard deviations above the WHO Child Growth Standards median (5). In our study, early catch-up growth was defined as from birth to 4 months, often during NICU admission, and the late catch-up growth as from 4 to 18 months, often post NICU admission.
Stool collection
A number of the above infants (n = 34) had weekly fecal samples collected during their NICU admission in an observational study approved by the University of South Florida Institutional Review Board. The inclusion criteria for this cohort are birth weight < 1,500 g or birth gestational age < 33 weeks. Infant fecal samples were collected weekly from diapers and stored immediately at −80°C until processing for DNA extraction for microbiome analysis. Stool samples collected at < 28 days and at 36-week corrected Ga (cGA) or prior to discharge were included in the final analysis. Stool samples were categorized by postnatal age at collection: during the first 14 days of life, 14–28 days of life, and at 36-week cGA.
DNA extraction and 16s rRNA gene sequencing library preparation
Total DNA was extracted from thawed fecal samples using QIAamp PowerFecal DNA extraction kit (Qiagen, Carlsbad, CA, United States) following the manufacturer’s instructions. The amount of DNA was measured with the Qubit 3.0 fluorometer (Life technologies, Carlsbad, CA, United States). The bacterial 16S rRNA V4 gene region was amplified by polymerase chain reaction using 515F and 806R primers (IDT, Coralville, IA, United States). The gene was then sequenced using the 2 bp × 300 bp chemistry in a paired-end manner on the Illumina Miseq platform (Illumina, San Diego, CA, United States).
16S rRNA analysis
All the raw demultiplexed fastq files were imported in R version 4.1.0 and were analyzed by the dada2 package v1.10.1 (13). Briefly, the forward and reverse reads were trimmed to 270 and 220 bases and the expected error for forward and reverse was set to 2 and 4 respectively. All ambiguous bases were discarded and the phiX genome was removed. The amplicon sequence variants (ASVs) were identified separately for the forward and reverse sequences and were merged subsequently. All identified chimeras were removed and the filtered ASVs were matched against the Silva v132 database to obtain their bacterial classification. Finally, the bacterial distribution table for all samples were obtained and a phyloseq (14) object was created for further analysis.
Statistical analysis
Continuous variables were described by mean and standard deviations (SD) or median and interquartile range (IQR) while categorical variables were described by percentages. The association between categorical variables was evaluated by the Fisher’s exact test. For continuous variables, independent samples t-tests and Mann–Whitney U tests were applied for normally distributed and skewed data, respectively. Because many comorbidities, such as CLD and NEC, occurred more frequently in infants born at lower birth GA and weight, we controlled for birth GA and weight in our analysis. The 95% confidence intervals were used to describe the precision of the estimates. The two-tailed statistical tests were considered significant at p < 0.05. All non-microbiome analyses were performed using IBM SPSS statistical software package (IBM Corp., IBM SPSS Statistics, Version 27.0 Armonk, NY: IBM Corp.). We also used SPSS to calculate the power with a linear regression model and two controlling factors (birth GA and weight) to predict childhood growth measurement with postnatal microbiome. A sample size of 34 subjects will detect an effect size of 0.3 at 0.871 power with a significance alpha level of 0.05. For Pearson correlation between BMI datasets, a sample size of 24 will detect a correlation coefficient of 0.6 at 0.901 power and alpha level of 0.05. The associations for BMI, length z-scores and weight z-scores with the microbiome were tested separately employing optimal microbiome-based association test (OMiAT) in R (15). OMiAT performs microbiome regression-based kernel association tests (MiRKATs) based on various distance measures. Here, all the associations were tested with 5,000 permutations after correcting for gestational weight, gender, current weight, maximum calorie intake, discharge weight, and maximum caloric intake. To determine whether the individual ASVs were associated with the phenotypes, the Microbiome Comprehensive Association Mapping (MiCAM) function implemented in the OMiAT package was used with 5,000 permutations. For all tests, only those ASVs were considered if they had a minimum abundance of 0.01% in at least one sample, and the ASV was observed in a minimum of 10% of all the samples. The associations with a p-value ≤ 0.05 are reported here.
Results
Maternal and infant characteristics
We screened 445 infants who had at least one well child visit at the USF complex care clinic. We included 160 infants who met our inclusion and exclusion criteria and 34 out of 160 infants had stool samples collected for microbiome analysis (Supplementary Figure 1). Their mean BW and GA were 1,149 ± 496 grams and 28 ± 3 weeks, respectively, with 81% (n = 130) very-low-birth-weight (VLBW, < 1,500 g) infants and 12% (n = 19) SGA (Table 1).
Early childhood growth
The mean changes in weight z scores from birth to 4 months and from 4 to 18 months were −3.53 ± 1.85 and 4.07 ± 2.29. The mean changes in length z scores from birth to 4 months and from 4 to 18 months were −3.39 ± 1.87 and 3.14 ± 1.72 respectively. Length z scores at 2 years correlated positively with the changes in weight z scores from birth to 4 months and negatively with that change from 4 to 18 months (r = 0.302, p = 0.001 and r = −0.317, p = 0.001 respectively) (Figure 1).
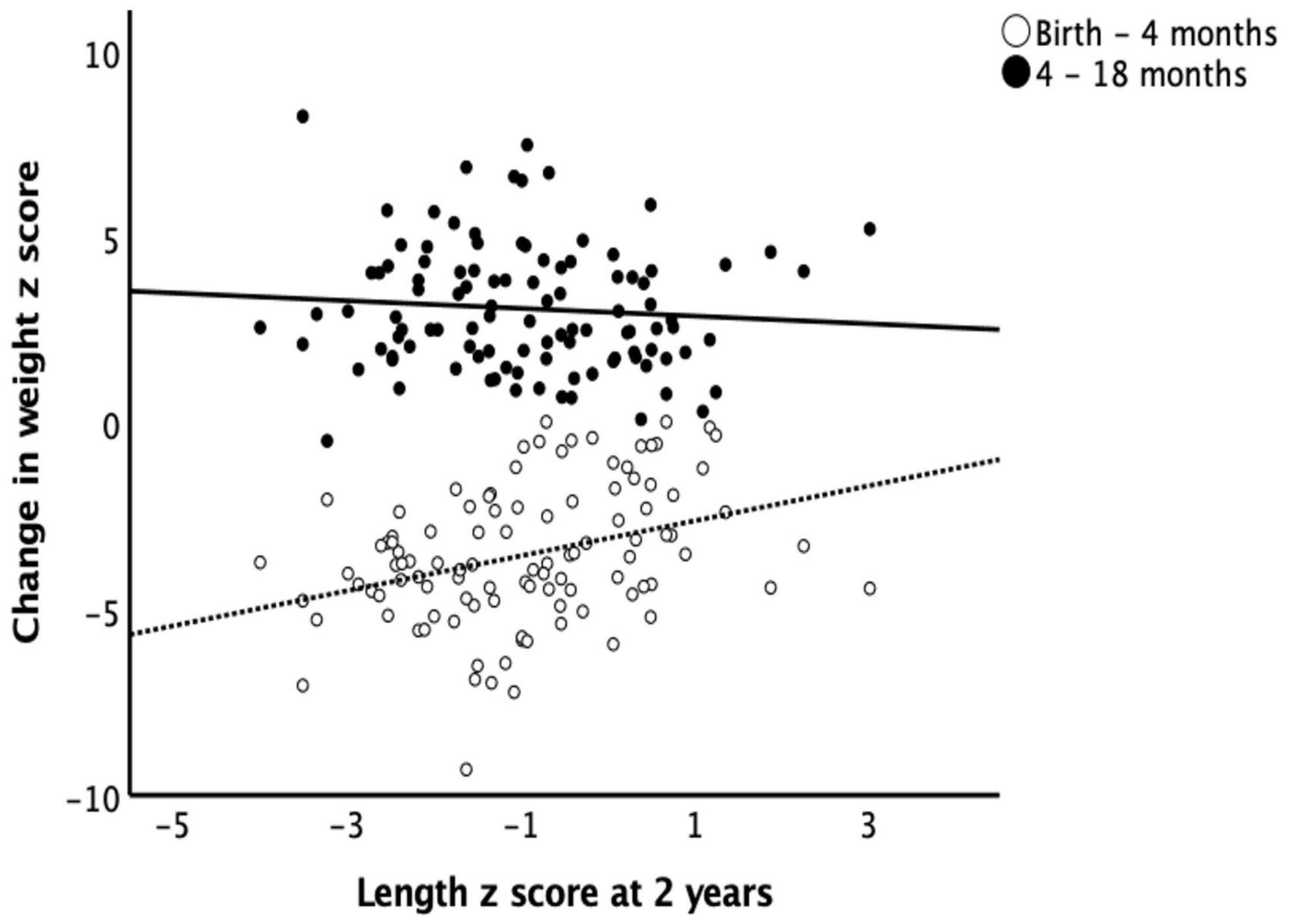
Figure 1. Length at 2 years and catch-up growth. Early catch-up growth, defined as change in weight z score from birth to 4 months of age, had a positive correlation (r = 0.302, p = 0.001) while late catch-up growth, defined as change in weight z score from 4 to 18 months of age, had a negative correlation (r = –0.317, p = 0.001) with length z scores at 2 years of age.
Childhood BMI
A greater number of infants had a recorded BMI at 2 and 3 years than at 4 and 5 years. Of the 160 infants, the numbers of infants that had a documented BMI were: 93% (n = 149) at 2 years of age, 65% (n = 105) at 3 years, 25% (n = 40) at 4 years, and 15% (n = 24) at 5 years of age. At 2 years, the mean BMI was 16.1 ± 1.8 kg/m2, 11% (n = 17) of infants were overweight (BMI > 1 standard deviation above the mean), and 6% (n = 10) infants were obese (BMI > 2 standard deviations above the mean) based on the WHO definitions. Most infants (n = 114, 71%) had a BMI within the healthy range at 2 years of age (Table 2). The mean BMI was 15.8 ± 2.2 kg/m2 at 3 years of age, 16.1 ± 3.5 kg/m2 at 4 years of age, and 16.4 ± 4.1 kg/m2 at 5 years of age. BMIs at 2 years of age positively correlated with BMIs at 3, 4, and 5 years of age (r = 0.753, p < 0.001; r = 0.652, p < 0.001; and r = 0.836, p < 0.001 respectively) (Figure 2). We used BMI at 2 years as the representative of the childhood BMI in the following analyses.
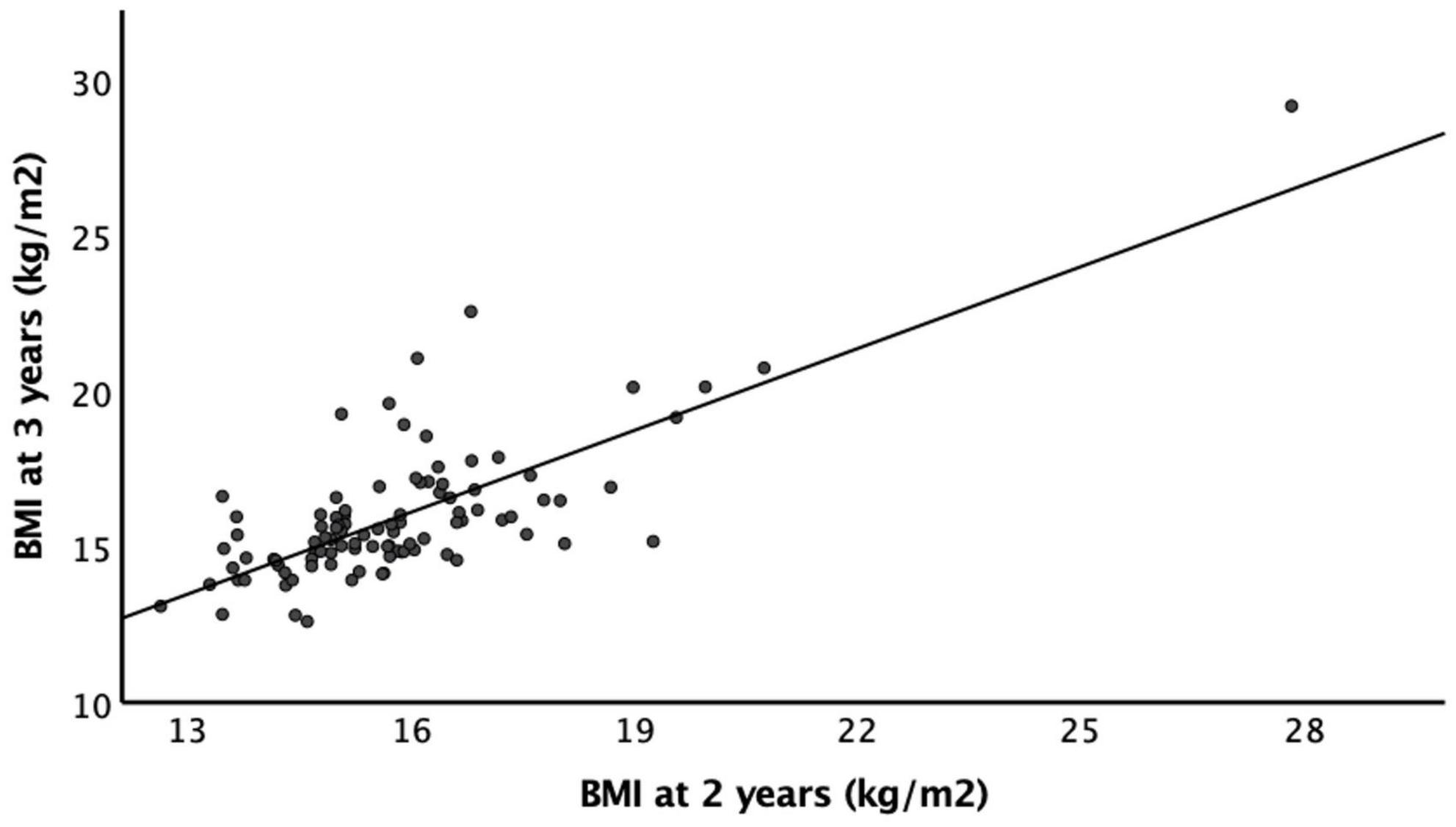
Figure 2. Body mass index as 2 and 3 years of age. There is a strong positive correlation between BMI at 2 years of age and BMI at 3 years of age (r = 0.753, p < 0.001).
Early childhood growth and BMI
The change in weight z scores from birth to 4 months did not correlate with BMI at 2 years with and without controlling for birth GA and BW. The change in weight z scores from 4 to 18 months positively correlated with BMI at 2 years of age (r = 0.417, p = 0.001) and the correlation remains significant with controlling for birth GA and BW (r = 0.469, p < 0.001). BMI at 2 years of age also had positive correlations with the change in length z scores from 4 to 18 months of age (r = 0.31, p = 0.002) but not with the change in length z scores from birth to 4 months (Figure 3).
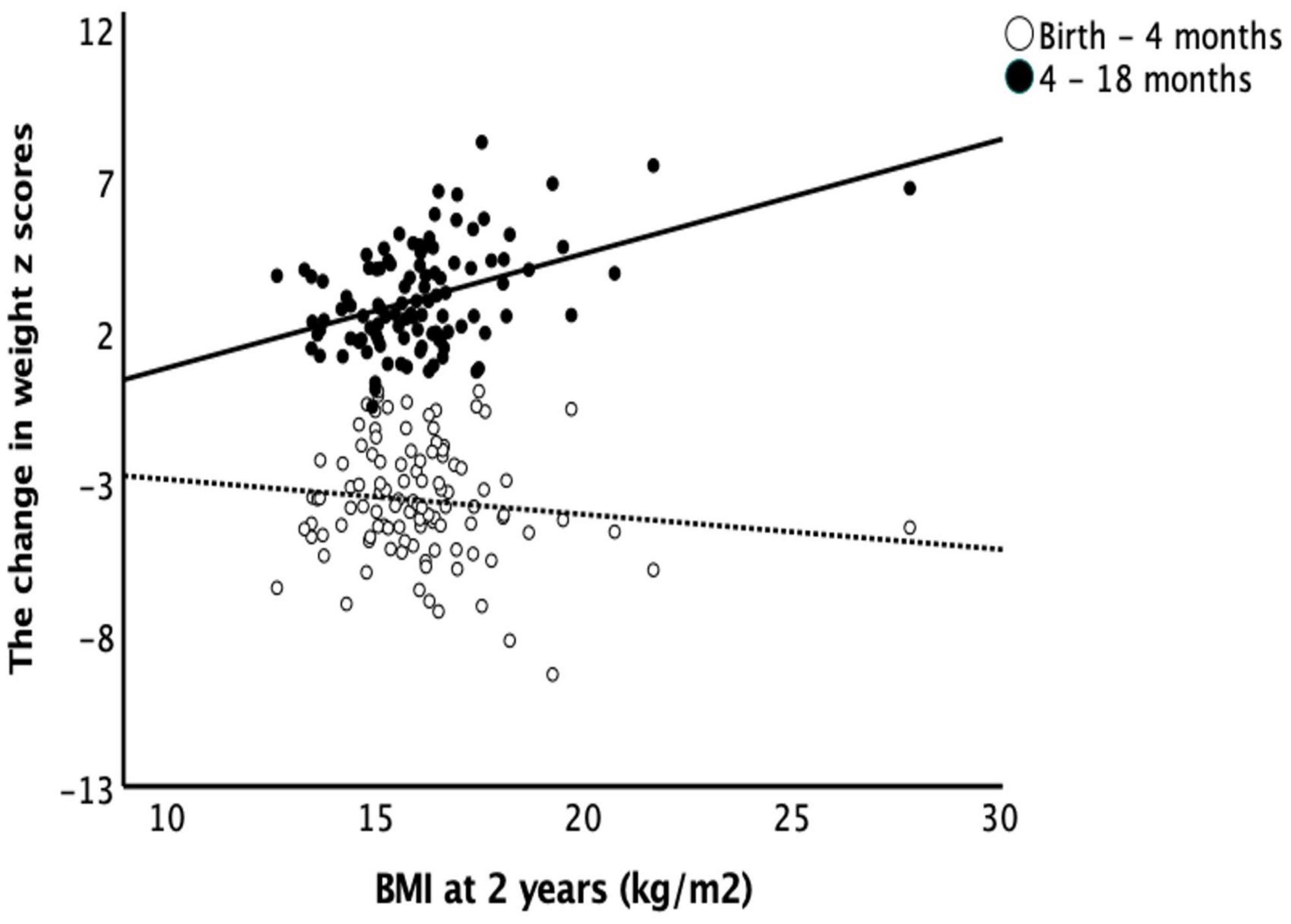
Figure 3. Body mass index at 2 years and catch-up growth. Early catch-up growth, defined as change in weight z score from birth to 4 months of age, did not correlate with BMI at 2 years of age (r = –0.103, p = 0.256). Late catch-up growth, defined as change in weight z scores from 4 to 18 months of age, had a positive correlation with BMI at 2 years of age (r = 0.417, p = 0.001).
NICU nutrition in relation to postnatal microbiome development and childhood growth
About half (49%) of the infants (n = 78) were fed human milk (including donor breast milk, mother’s own breast milk, or a combination of the two) and 25% (n = 40) were exclusively breastfed at discharge (Table 1). Within the population, 41% received at least one macronutrient supplementation with 14% (n = 22) receiving both protein and MCT. About a third (31%) received high caloric concentration (>24 kcal/oz) during NICU admission, 31% received high daily caloric intake (≥140 kcal/kg/day), and 39% were discharged home with ≥24 kcal/oz feeding.
Length z scores at 2 years had a negative correlation with caloric supports measured as maximum caloric density (calories/ounce) and maximum caloric intake (calories/kg/day) during NICU (r = −0.357, p < 0.001 and r = −0.323, p < 0.001 respectively) and caloric density (calories/oz) of discharge feed (r = −0.302, p < 0.001). There was no association between nutritional supports (macronutrient supplementation and caloric density) and childhood BMI. We did not find any significant association between postnatal microbiome development and feeding types, caloric supports, or macronutrient supplementation.
Postnatal microbiome development and early childhood growth
Birth to 4 month growth
Using the stated regression analysis, the change in length z scores from birth to 4 months positively associated with the fecal abundances of Streptococcus, Veillonella, and Haemophilus (p = 0.031, p = 0.044, and p = 0.002, respectively) from 0 to 14 days of age, negatively associated with the abundance of Proteus (p = 0.04) from 14 to 28 days of age and was not significantly associated with microbiome composition after 28 days of life.
The change in weight z scores from birth to 4 months positively associated with abundance of Bacteroidales and Haemophilus (p = 0.042 and p = 0.024, respectively) from 0 to 14 days of age, negatively associated with abundances of Gemella (p = 0.032) from 14 to 28 days of age, and negatively associated with abundance of Gammaproteobacteria, a class of Proteobacteria (p = 0.018) at 36 weeks cGA (Figure 4).
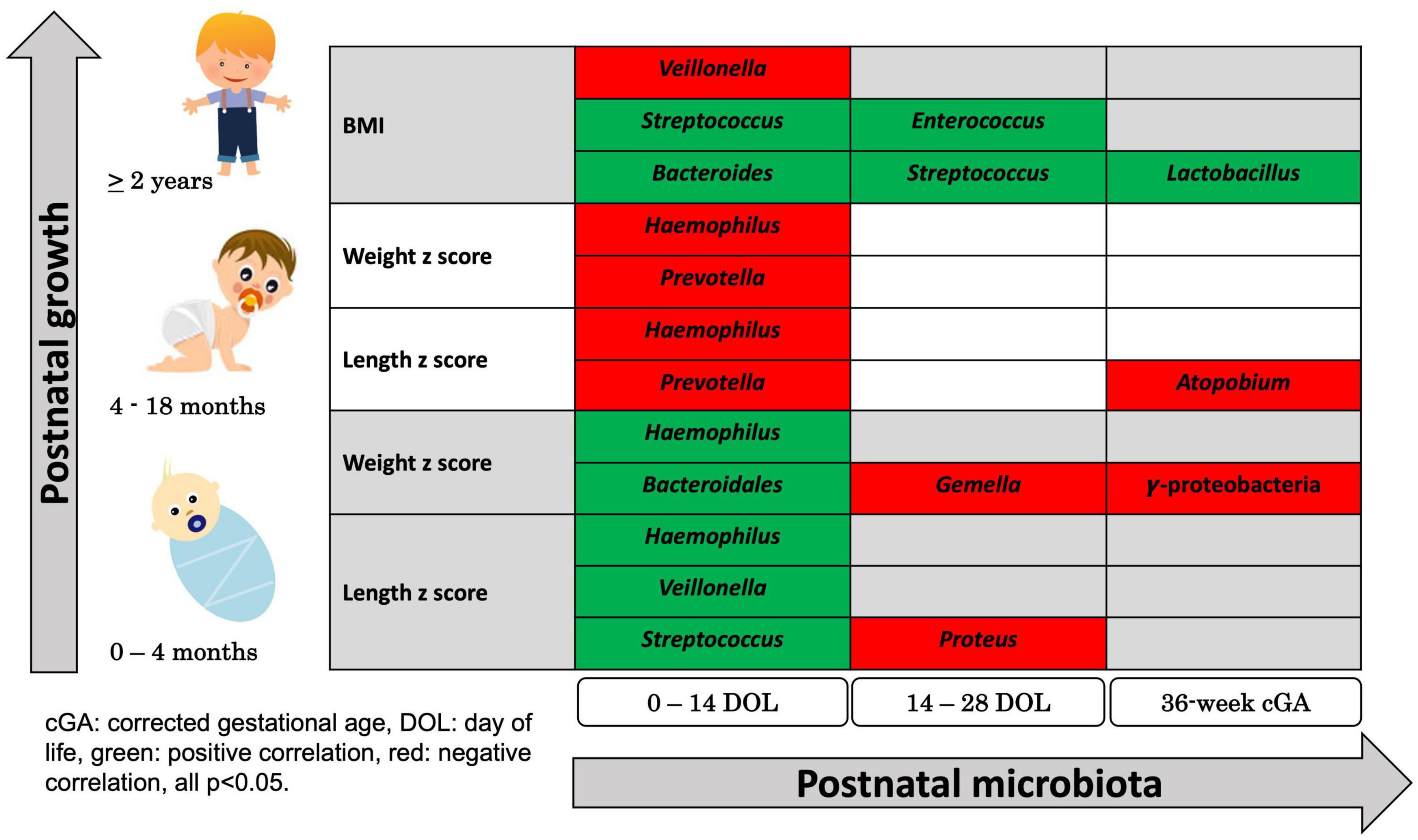
Figure 4. Summary of Significant Correlations Between Postnatal microbial abundance and early childhood growth. Using regression-based association tests between individual bacterial classification and growth, bacterial abundances 0–14 days, 14–28 days, and at 36 weeks corrected gestational age were associated with childhood growth measured by change in z-scores in weight and in length during early infancy, z-scores in weight and in length during late infancy, and BMI at 2 years of age. Green colored bacterial names had positive correlation with growth and red colored bacterial names had negative correlation with growth (all p < 0.05).
4–18 month growth
The change in length and weight z scores from 4–18 months were negatively associated with the abundances of Prevotella and Haemophilus (p = 0.034 and p = 0.031, respectively, for length and p = 0.02 and p = 0.029, respectively, for weight) from 0 to 14 days of age. The length z scores negatively associated with abundance of Atopobium (p = 0.027) after 28 days of age (Figure 4).
BMI at 2 years of age
BMIs at 2 years of age positively associated with abundance of Bacteroides and Streptococcus (p = 0.037 and p = 0.003, respectively) and negatively correlated with abundance of Veillonella (p = 0.018) from 0 to 14 days of age. BMI at 2 years of age was positively associated with abundance of Streptococcus and Enterococcus (p = 0.021 and p = 0.018, respectively) from 14 to 28 days of age and was positively associated with abundance of Lactobacillus (p = 0.035) at 36-week cGA (Figure 4).
Discussion
Our study examined the nutritional supports and gut microbiota development of preterm infants in the NICU as predictors of early childhood anthropometrics. The data showed that optimized early postnatal growth in the NICU improved childhood linear growth without an impact on childhood BMI. Infants who required higher caloric intake to improve growth in NICU were at higher risk for poor linear growth at 2 years of age. The NICU microbiome was associated with anthropometrics from birth to 2 years. The trajectory of childhood BMI can be identified as early as 2 years of age and influenced by late infancy growth but not by early infancy growth. These findings support the benefits of early postnatal catch-up growth and the role of gut microbiota acquisition on childhood growth in preterm infants.
The importance of optimizing nutrition and growth in preterm infants in the NICU to improve long term neurodevelopmental outcomes has been well studied and documented (16). Our results show that optimal growth in the NICU establishes desired childhood growth patterns in preterm infants. Preterm growth can be improved through nutritional supplementation and increased caloric density (17) but some fear aggressive caloric supports in NICU can lead to childhood obesity (6, 7, 18). Ideally, nutritional intervention supports linear growth and lean body mass more so than fat deposition to achieve a healthy BMI (18). In our study, requiring increased caloric density and/or daily caloric intake using protein and MCT supplementations during the postnatal period in the NICU was an indicator of poorer growth in length at 2 years. However, the resulting early catch-up growth correlated with better linear growth but not associated with BMI ay 2 years. Our findings are in line with the findings of Regan et al. (19), which showed no significant association between macronutrient or energy intake and insulin sensitivity in preterm infants at 4–10 years of age. These findings suggest that using macronutrient and caloric supplementations to improve growth in the NICU may benefit metabolic health into childhood (20). Raaijmakers et al. (21) found that catch-up growth in infancy of low-birth-weight infants correlated with lower body fat in young adolescence. Other studies note concerns that accelerated growth velocity may predispose children to later obesity (22). Lapillonne et al. (23) discusses how growth in infancy and childhood can predispose patients to metabolic syndrome and hypertension. Our study showed that early catch-up growth in NICU did not appear to influence childhood BMI.
An early predictor of healthy childhood body weight is important for interventions to prevent the long-term negative health impacts from overweight and obesity. Even though early infancy growth did not predict childhood BMI, late infancy growth did. Weight gain during early infancy from birth to 4 months, often while the preterm infant was in the NICU, did not correlate with BMI at 2 years even after controlling for birth GA and BW. Whereas weight gain during late infancy from 4 to 18 months, usually after the preterm infant was discharged from the NICU, positively correlated with BMI at 2 years of age; this correlation remained significant after controlling for birth GA and BW. In our population, 2-year BMI predicted BMI through 5 years of age, which is consistent with the finding by Pryor et al. (24). This implies outpatient nutrition education for parents of preterm infants may be a high impact intervention to promote healthy childhood body weight.
Our study also found infant gut microbiota acquisition to be associated with childhood growth. Bacterial exposure from delivery mode, breast feeding, and hospitalization greatly influence the infant microbiome in the postnatal stage (9, 25). Our group and collaborators have shown the impact of perinatal factors, genetic relatedness, and NICU diet on postnatal microbiota development as well as the link between microbiota and growth during NICU admission (12, 26–30). In this study, we focused on the association between postnatal microbiota development and childhood growth into preschool age in preterm infants. We found links among bacterial abundance, catch-up growth, and childhood BMI; further supporting previous data showing that specific bacterial abundances correlate with growth deviance in childhood (8, 11). During NICU stay, weight gain negatively correlated with the abundance of Gammaproteobacteria. Enterobacteriaceae, a member of the Gammaproteobacteria taxa, has been implicated in delayed growth (27). These bacteria utilize metabolic pathways that produce less adenosine 5′-triphosphate (ATP) compared to beneficial Bifidobacteria and produce pro-inflammatory lipopolysaccharides (LPS) which decreases nutrient absorption in the colon (27). Our data also showed that decreased abundance of Prevotella correlates with higher childhood BMI and increased late infancy growth in weight and in height. Although Prevotella is classified as commensal bacteria, there are emerging studies suggesting its role in inflammatory disease and obesity. As most of the infants in our study were classified in the healthy BMI range at 2 years of age (71%), our finding most likely supports that decreased abundance of Prevotella limits inflammatory mechanisms involved in obesity development (31). Additionally, the data demonstrated that abundance of Bacteroides positively correlating with childhood BMI and infancy growth. Similar to our findings, Indiani et al. (32) found that the abundance of Bacteroides positively correlates with childhood BMI as well as childhood obesity. Due to the small number of children in our study classified as obese, our conclusions on the role of microbiome abundances in preterm infants on childhood obesity are limited. Instead, our results indicate that neonatal microbiota acquisition in preterm infants correlates with growth measures throughout infancy and childhood.
Nutrition in early infancy has been shown to impact the growth of preterm infants as well as influence bacterial diversity (10, 11). Breast milk in early infancy can stimulate beneficial bacteria such as Bacteroides fragilis, Bifidobacteria infantis, and Lactobacillus acidophilus through the production of immunoglobulin A (IgA), activation of regulatory T cells, and promotion of anti-inflammatory mediators (10). In a longitudinal study of term and preterm infants, the main factor in microbiome development during the first year of life was the receipt of breast milk, which was significantly associated with Bifidobacterium species throughout the first 3 years of life (33). In our study, about half (49%) of the infants were fed breast milk (including donor breast milk, mother’s own breast milk, or a combination of the two) and 25% were exclusively breastfed at discharge. Our microbiome pattern may not reflect the colonization and establishment of gut microbiota in a population with higher exposure to human milk or mother’s own milk. Although we did not find any significant associations between postnatal microbiome development and nutritional supports, a larger sample for microbiome analysis would be beneficial. Further study is needed to understand the relationship between NICU nutrition and the microbiome in early infancy.
Postnatal bacterial colonization and growth are early influences that impact childhood growth for preterm infants. The strength of this study was a cohort of preterm infants who were treated in a single center and followed up at one NICU follow-up clinic. The subjects were all under the same standards of care, feeding protocols, and monitoring of growth parameters. The longitudinal data and stool collections strengthened the findings of this study, but microbiome analysis for a greater number of the infants would be more desirable. In the future, a larger sample size should be evaluated to determine trends in microbiome diversity and childhood BMI.
Conclusion
In preterm infants, maximizing childhood health outcomes begins with early interventions and exposures. Our study shows that early catch-up growth and microbiome establishment are important for supporting optimal childhood growth in preterm infants. Further, growth parameters in infancy can predict metabolic health into childhood. These findings offer an opportunity for clinicians and caregivers to cultivate positive health outcomes for preterm infants. The extended effects of early NICU interventions may be amplified by continued comprehensive care outside the NICU, involving NICU follow up clinic, primary care providers, and caregivers.
Data availability statement
The datasets presented in this study can be found in online repositories. The names of the repository/repositories and accession number(s) can be found below: https://www.ncbi.nlm.nih.gov/, SRP171050.
Ethics statement
The studies involving human participants were reviewed and approved by University of South Florida IRB. Written informed consent to participate in this study was provided by the participants’ legal guardian/next of kin.
Author contributions
JT, EM, HG, and TH designed research. JT, RJ, AL, and TH conducted research. TH and AS analyzed data. JT, AL, AS, and TH prepared the manuscript. JT, AL, and TH had primary responsibility for final content. All authors read and approved the final manuscript.
Funding
This work received funding from the source 1K23HL150300-01A1 by NIH/NHLBI to support research time for TH.
Acknowledgments
We would like to thank our research nurse, Marcia Kneusel, for enrolling patients and collecting stool samples. AS’s effort is supported by the Genomics Program at USF.
Conflict of interest
The authors declare that the research was conducted in the absence of any commercial or financial relationships that could be construed as a potential conflict of interest.
Publisher’s note
All claims expressed in this article are solely those of the authors and do not necessarily represent those of their affiliated organizations, or those of the publisher, the editors and the reviewers. Any product that may be evaluated in this article, or claim that may be made by its manufacturer, is not guaranteed or endorsed by the publisher.
Supplementary material
The Supplementary Material for this article can be found online at: https://www.frontiersin.org/articles/10.3389/fped.2022.850629/full#supplementary-material
References
1. Simon L, Nusinovici S, Flamant C, Cariou B, Rouger V, Gascoin G, et al. Post-term growth and cognitive development at 5 years of age in preterm children: evidence from a prospective population-based cohort. PLoS One. (2017) 12:e0174645. doi: 10.1371/journal.pone.0174645
2. Cooke RJ. Improving growth in preterm infants during initial hospital stay: principles into practice. Arch Dis Child Fetal Neonatal Ed. (2016) 101:F366–70. doi: 10.1136/archdischild-2015-310097
3. Hruby A, Hu FB. The epidemiology of obesity: a big picture. Pharmacoeconomics. (2015) 33:673–89. doi: 10.1007/s40273-014-0243-x
4. Fenton TR, Nasser R, Eliasziw M, Kim JH, Bilan D, Sauve R. Validating the weight gain of preterm infants between the reference growth curve of the fetus and the term infant. BMC Pediatr. (2013) 13:92. doi: 10.1186/1471-2431-13-92
6. Barker DJ, Osmond C. Infant mortality, childhood nutrition, and ischaemic heart disease in England and Wales. Lancet. (1986) 1:1077–81. doi: 10.1016/s0140-6736(86)91340-1
7. Desmond C, Casale D. Catch-up growth in stunted children: definitions and predictors. PLoS One. (2017) 12:e0189135. doi: 10.1371/journal.pone.0189135
8. Younge NE, Newgard CB, Cotten CM, Goldberg RN, Muehlbauer MJ, Bain JR, et al. Disrupted maturation of the microbiota and metabolome among extremely preterm infants with postnatal growth failure. Sci Rep. (2019) 9:8167. doi: 10.1038/s41598-019-44547-y
9. Robertson RC, Manges AR, Finlay BB, Prendergast AJ. The human microbiome and child growth - first 1000 days and beyond. Trends Microbiol. (2019) 27:131–47. doi: 10.1016/j.tim.2018.09.008
10. Walker WA. The importance of appropriate initial bacterial colonization of the intestine in newborn, child, and adult health. Pediatr Res. (2017) 82:387–95. doi: 10.1038/pr.2017.111
11. Milani C, Duranti S, Bottacini F, Casey E, Turroni F, Mahony J, et al. The first microbial colonizers of the human gut: composition, activities, and health implications of the infant gut microbiota. Microbiol Mol Biol Rev. (2017) 81:17. doi: 10.1128/MMBR.00036-17
12. Yee AL, Miller E, Dishaw LJ, Gordon JM, Ji M, Dutra S, et al. Longitudinal microbiome composition and stability correlate with increased weight and length of very-low-birth-weight infants. mSystems. (2019) 4:18. doi: 10.1128/mSystems.00229-18
13. Callahan BJ, McMurdie PJ, Rosen MJ, Han AW, Johnson AJ, Holmes SP. DADA2: high-resolution sample inference from Illumina amplicon data. Nat Methods. (2016) 13:581–3. doi: 10.1038/nmeth.3869
14. Lu J, Salzberg SL. Ultrafast and accurate 16S rRNA microbial community analysis using Kraken 2. Microbiome. (2020) 8:124. doi: 10.1186/s40168-020-00900-2
15. Koh H, Blaser MJ, Li H. A powerful microbiome-based association test and a microbial taxa discovery framework for comprehensive association mapping. Microbiome. (2017) 5:45. doi: 10.1186/s40168-017-0262-x
16. Kesavan K, Devaskar SU. Intrauterine growth restriction: postnatal monitoring and outcomes. Pediatr Clin North Am. (2019) 66:403–23. doi: 10.1016/j.pcl.2018.12.009
17. Gat-Yablonski G, Phillip M. Nutritionally-induced catch-up growth. Nutrients. (2015) 7:517–51. doi: 10.3390/nu7010517
18. Belfort MB, Gillman MW, Buka SL, Casey PH, McCormick MC. Preterm infant linear growth and adiposity gain: trade-offs for later weight status and intelligence quotient. J Pediatr. (2013) 163:1564–9e2. doi: 10.1016/j.jpeds.2013.06.032
19. Regan FM, Cutfield WS, Jefferies C, Robinson E, Hofman PL. The impact of early nutrition in premature infants on later childhood insulin sensitivity and growth. Pediatrics. (2006) 118:1943–9. doi: 10.1542/peds.2006-0733
20. Asbury MR, Unger S, Kiss A, Ng DVY, Luk Y, Bando N, et al. Optimizing the growth of very-low-birth-weight infants requires targeting both nutritional and nonnutritional modifiable factors specific to stage of hospitalization. Am J Clin Nutr. (2019) 110:1384–94. doi: 10.1093/ajcn/nqz227
21. Raaijmakers A, Jacobs L, Rayyan M, van Tienoven TP, Ortibus E, Levtchenko E, et al. Catch-up growth in the first two years of life in Extremely Low Birth Weight (ELBW) infants is associated with lower body fat in young adolescence. PLoS One. (2017) 12:e0173349. doi: 10.1371/journal.pone.0173349
22. Hay WW. Optimizing nutrition of the preterm infant. Zhongguo Dang Dai Er Ke Za Zhi. (2017) 19:1–21.
23. Lapillonne A, Griffin IJ. Feeding preterm infants today for later metabolic and cardiovascular outcomes. J Pediatr. (2013) 162:S7–16. doi: 10.1016/j.jpeds.2012.11.048
24. Pryor LE, Tremblay RE, Boivin M, Touchette E, Dubois L, Genolini C, et al. Developmental trajectories of body mass index in early childhood and their risk factors: an 8-year longitudinal study. Arch Pediatr Adolesc Med. (2011) 165:906–12. doi: 10.1001/archpediatrics.2011.153
25. Mesa MD, Loureiro B, Iglesia I, Fernandez Gonzalez S, Llurba Olive E, Garcia Algar O, et al. The evolving microbiome from pregnancy to early infancy: a comprehensive review. Nutrients. (2020) 12:12010133. doi: 10.3390/nu12010133
26. Aguilar-Lopez M, Dinsmoor AM, Ho TTB, Donovan SM. A systematic review of the factors influencing microbial colonization of the preterm infant gut. Gut Microbes. (2021) 13:1–33. doi: 10.1080/19490976.2021.1884514
27. Groer M, Miller EM, Sarkar A, Dishaw LJ, Dutra SV, Youn Yoo J, et al. Predicted metabolic pathway distributions in stool bacteria in very-low-birth-weight infants: potential relationships with NICU faltered growth. Nutrients. (2020) 12:12051345. doi: 10.3390/nu12051345
28. Groer MW, Miller EM, D’Agata A, Ho TTB, Dutra SV, Yoo JY, et al. Contributors to dysbiosis in very-low-birth-weight infants. J Obstet Gynecol Neonatal Nurs. (2020) 2020:3. doi: 10.1016/j.jogn.2020.02.003
29. Ho TTB, Groer MW, Kane B, Yee AL, Torres BA, Gilbert JA, et al. Dichotomous development of the gut microbiome in preterm infants. Microbiome. (2018) 6:157. doi: 10.1186/s40168-018-0547-8
30. Lim SJ, Aguilar-Lopez M, Wetzel C, Dutra SVO, Bray V, Groer MW, et al. The effects of genetic relatedness on the preterm infant gut microbiota. Microorganisms. (2021) 9:9020278. doi: 10.3390/microorganisms9020278
31. Larsen JM. The immune response to Prevotella bacteria in chronic inflammatory disease. Immunology. (2017) 151:363–74. doi: 10.1111/imm.12760
32. Indiani C, Rizzardi KF, Castelo PM, Ferraz LFC, Darrieux M, Parisotto TM. Childhood obesity and firmicutes/bacteroidetes ratio in the gut microbiota: a systematic review. Child Obes. (2018) 14:501–9. doi: 10.1089/chi.2018.0040
Keywords: preterm infants, gut microbiome, catch-up growth, nutrition, childhood BMI
Citation: Tadros JS, Llerena A, Sarkar A, Johnson R, Miller EM, Gray HL and Ho TTB (2022) Postnatal growth and gut microbiota development influenced early childhood growth in preterm infants. Front. Pediatr. 10:850629. doi: 10.3389/fped.2022.850629
Received: 07 January 2022; Accepted: 19 July 2022;
Published: 09 August 2022.
Edited by:
Shi Yuan, Children’s Hospital of Chongqing Medical University, ChinaReviewed by:
Hui Wu, First Affiliated Hospital of Jilin University, ChinaRoberto Murgas Torrazza, Secretaría Nacional de Ciencia, Tecnología e Innovación, Panama
Copyright © 2022 Tadros, Llerena, Sarkar, Johnson, Miller, Gray and Ho. This is an open-access article distributed under the terms of the Creative Commons Attribution License (CC BY). The use, distribution or reproduction in other forums is permitted, provided the original author(s) and the copyright owner(s) are credited and that the original publication in this journal is cited, in accordance with accepted academic practice. No use, distribution or reproduction is permitted which does not comply with these terms.
*Correspondence: Thao T. B. Ho, dGhvMUB1c2YuZWR1