- 1Endocrinology Department, Sant Joan de Déu Research Institute, University of Barcelona, Barcelona, Spain
- 2Centro de Investigación Biomédica en Red de Diabetes y Enfermedades Metabólicas Asociadas, ISCIII, Madrid, Spain
- 3Department of Biochemistry and Molecular Biomedicine, Institute of Biomedicine, Sant Joan de Déu Research Institute, University of Barcelona, Barcelona, Spain
- 4Centro de Investigación Biomédica en Red Fisiopatología de la Obesidad y Nutrición, ISCIII, Madrid, Spain
- 5Department of Development and Regeneration, KU Leuven, Leuven, Belgium
- 6Pediatric Endocrinology Research Group, Dr. Josep Trueta Hospital, Girona Biomedical Research Institute (IDIBGI), Girona, Spain
Objective: Bone morphogenetic protein-8B (BMP8B) is an adipokine produced by brown adipose tissue (BAT) contributing to thermoregulation and metabolic homeostasis in rodent models. In humans, BAT activity is particularly relevant in newborns and young infants. We assessed BMP8B levels and their relationship with BAT activity and endocrine-metabolic parameters in young infants to ascertain its potentiality as biomarker in early life.
Materials and Methods: BMP8B concentrations were assessed longitudinally by ELISA in a cohort of 27 girls and 23 boys at birth, and at age 4 and 12 months, together with adiposity parameters (DXA), and circulating endocrine-metabolic variables. BAT activity was measured by infrared thermography. BMP8B gene expression (qRT-PCR) was determined in BAT, white fat, and liver samples from neonatal necropsies, and in placenta and cord blood.
Results: BMP8B levels were high at birth, particularly in boys (P = 0.04 vs. girls), declined progressively, and remained well above those in healthy adults and pregnant women at age 1 year (P < 0.05 and P < 0.001, respectively). Neonatal BMP8B transcript levels were higher in BAT than in white fat, liver and cord blood. Circulating BMP8B levels during the first year of life marginally correlated with bone mineral density and gains in lean mass.
Conclusion: BMP8B levels are high at birth and decline progressively over the first year of life remaining above adult levels. Although changes in BMP8B concentrations overall reflect those in BAT activity during development, BMP8B levels are unlikely to be useful to predict individual variations in endocrine-metabolic status and BAT activity in healthy young infants.
Introduction
Brown adipose tissue (BAT) possesses marked metabolic and thermogenic properties. In humans, BAT is especially abundant in newborns (1). BAT secretes regulatory proteins, termed brown adipokines or batokines, which hold autocrine, paracrine and endocrine attributes (2). The identification of batokines that could serve as circulating biomarkers of BAT activity could be useful in clinical practice. In this regard, we recently showed that BAT activity and the levels of the batokine C-X-C motif chemokine ligand 14 (CXCL14) -a purported surrogate of BAT activity- are sex-specific in the first year of life (3).
Bone morphogenetic protein-8B (BMP8B) is a member of the transforming growth factor β superfamily (TGF-β), a large group of regulatory factors involved in embryo development and adult tissue homeostasis. Experimental data in adult rodent models has revealed a thermogenic role for BMP8B, which has been demonstrated to be produced in BAT as a brown adipokine and to induce thermogenesis by acting both peripherally and centrally (4, 5). Other data in experimental models have shown that, despite BMP8B expression is minimal in healthy liver, hepatic BMP8B expression is increased under conditions of non-alcoholic steatohepatitis (6, 7). Recently, blood BMP8B levels have been found to be positively associated with body temperature and thermal stress-induced BAT activity in human neonates, thus indicating that BMP8B may be relevant for thermoregulation in human early life (8). It is known that BAT is highly present in the first years of human postnatal development (1), and considering that BAT is a major site of BMP8B release in rodent models (4), it could be hypothesized that BAT may be a major source of circulating BMP8B in human neonates. Thus, BMP8B levels might be a biomarker indicative of the extent of BAT activity in early life and, considering the association of BAT activity and a healthy metabolic status in adults (9), BMP8B could also reflect postnatal metabolic status.
Here, we determined for the first time the longitudinal outcome of circulating BMP8B levels over the first year of life of humans and analyzed their association with endocrine and metabolic parameters as well as with BAT activity.
Methods
Study Population
The study cohort consisted of 50 infants (27 girls and 23 boys) who were enrolled prenatally during the customary third trimester visit among those Caucasian pregnant mothers (age, 34.0 ± 0.7 years) consecutively seen in the outpatient clinic of Hospital Sant Joan de Déu and Hospital de Sant Boi – Parc Sanitari Sant Joan de Déu (Barcelona, Spain) (Supplementary Figure 1). Forty-three out of those 50 infants (23 girls and 20 boys) had previously participated in a longitudinal study assessing BAT activity and the circulating levels of CXCL14 in the first year of life (3).
Specific inclusion and exclusion criteria have been previously described in detail (3). Briefly, the inclusion criteria were: singleton uncomplicated pregnancies at term (37–42 weeks), exclusive breastfeeding or formula-feeding in the first 4 months, postnatal follow-up completed (at 15 days, 4 and 12 months) and written informed consent. Exclusion criteria were maternal disease, alcohol or drug abuse, congenital malformations and complications at birth. Birth weight was not considered as inclusion or exclusion criterium; accordingly, the study population included infants with a wide range of birth weight Z-scores (between −2.9 and +1.0).
Circulating BMP8B was measured in a subset of infants who had spare serum sample available at birth (22 girls and 20 boys), and in the whole study cohort (27 girls and 23 boys) at age 4 and 12 months. Serum BMP8B was also measured in 42 mothers of those infants during the third trimester of pregnancy (age, 34.0 ± 0.7 years) (Supplementary Figure 1). In addition, serum BMP8B concentrations were measured cross-sectionally in apparently healthy adult women (N = 15; age, 22.2 ± 0.8 years) and men (N = 11; age, 21.9 ± 0.6 years) and in 11 healthy newborns (N = 6 girls and N = 5 boys; birth weight, 3.3 ± 0.1 kg) sampled at the postnatal age of 36 h (10).
Bone morphogenetic protein-8B mRNA expression levels were assessed in: (a) dorso-interscapular BAT (N = 5), omental/perirenal white adipose tissue (WAT, N = 5) and liver (N = 4) post-mortem samples obtained on occasion of autopsies (2–3 h after the death) of Caucasian newborns with a gestational age of 22–36 weeks who survived, at most, 3 days post-partum, as described previously (11) (see Supplementary Table 1 for details), (b) placental samples from healthy women (N = 3) with uncomplicated pregnancies undergoing cesarean section at term, as previously described (12), (c) whole cord blood (N = 4; 2 girls, 2 boys) obtained from healthy neonates randomly selected among those composing the cohort reported above.
Assessments
Maternal data were retrieved from the hospital clinical records. Gestational age was estimated based on the last menses and validated by first-trimester ultrasound. Weight and length were measured immediately after delivery, and again at age 4 and 12 months, and Z-scores were derived using regional normative data (13).
Maternal venous samples were obtained during the third trimester of gestation (gestational age, 35.3 ± 0.4 weeks). Infant blood samples were obtained at birth [from the umbilical cord before placenta separation (14)] and in the fasting state at age 4 and 12 months. Whole blood collected in EDTA tubes was used for RNA extraction. The serum fraction was also obtained and stored at −80°C until analysis.
Serum glucose, insulin, insulin-like growth factor (IGF)-I, high-molecular-weight (HMW) adiponectin and CXCL14 were assessed as reported (3). Serum BMP8B levels were determined with a specific human enzyme-linked immunosorbent assay kit (MBS944757, MyBioSource, San Diego, CA, United States; sensitivity: 2.34 pg/ml; intra-assay coefficient of variation (CV): <8%; inter-assay CV: <10%). Body composition was assessed by dual-energy X-ray absorptiometry (DXA) with a Lunar Prodigy and Lunar software (version 3.4/3.5; Lunar Corp., Madison, WI, United States) adapted to for infants (14).
As previously detailed (3), BAT activity at age 12 months was estimated through the infrared thermography-based measurement of the skin temperature overlying BAT depots. The parameters assessed included the maximal temperature at the posterior cervical (TPCR) and supraclavicular (TSCR) regions, and the extent of active BAT in these regions (AreaPCR and AreaSCR).
RNA was extracted from tissues using an affinity-based method (NucleoSpin, MACHEREY-NAGEL, Germany) and from cord blood using TriPure (Roche Diagnostics, Indianapolis, IN, United States). BMP8B transcript levels were determined by qRT-PCR using TaqMan technology (Thermo Fisher Scientific, Waltham, MA, United States). 0.5 μg RNA were retrotranscribed using random hexamer primers (Thermo Fisher Scientific, Waltham, MA, United States). For qRT-PCR, the BMP8B TaqMan Gene Expression assay probe Hs01629120 was used, with reaction mixtures containing 1 μL cDNA, 10 μL TaqMan Universal PCR Master Mix (Thermo Fisher Scientific, Waltham, MA, United States), 250 nM probes and 900 nM of primers from the Assays-on-Demand Gene Expression Assay Mix (Thermo Fisher Scientific, Waltham, MA, United States). The 18S rRNA transcript (Hs99999901) was measured as housekeeping reference gene. The mRNA level of BMP8B in each sample was normalized to that of the reference control using the comparative (2–ΔCT) method.
Statics and Ethics
Data were analyzed using the SPSS version 27.0 (SPSS software, IBM, Armonk, NY, United States). Results are shown as mean ± SEM. Variables with normal distribution were compared with two-tailed Student’s t-test. Chi-square test was used to compare qualitative variables. Correlation and stepwise multi-regression analysis were used to study associations between circulating BMP8B levels and the assessed variables. Covariance analysis was used to adjust for ponderal index and breastfeeding. A P-value < 0.05 was considered statistically significant.
The study was approved by the Institutional Review Board of University of Barcelona, Sant Joan de Déu University Hospital; informed written consent was obtained from mothers at the time of recruitment.
Results
The anthropometric data in the mothers and the longitudinal infant data split by sex are summarized in Table 1. The infants’ results confirmed the previously reported sex-specificity of lean mass, CXCL14 and BAT activity (3).
Figure 1 depicts circulating BMP8B concentrations in the infants from birth to age 12 months, as well as in the mothers of those infants in the third trimester of gestation and in healthy adults. Over the first year of life, circulating BMP8B levels decreased. When pooling BMP8B concentrations independently of sex, serum BMP8B levels were significantly higher at birth and at age 4 months as compared to age 12 months (P < 0.001) and remained higher versus those in healthy adults at all time points. In addition, serum BMP8B concentrations in pregnant women in late gestation were significantly lower than those in healthy adults or in human infants (P < 0.001) (Figure 1A). When BMP8B levels were split by sex, boys showed significantly higher concentrations than girls only at birth (P = 0.04) (Figure 1B).
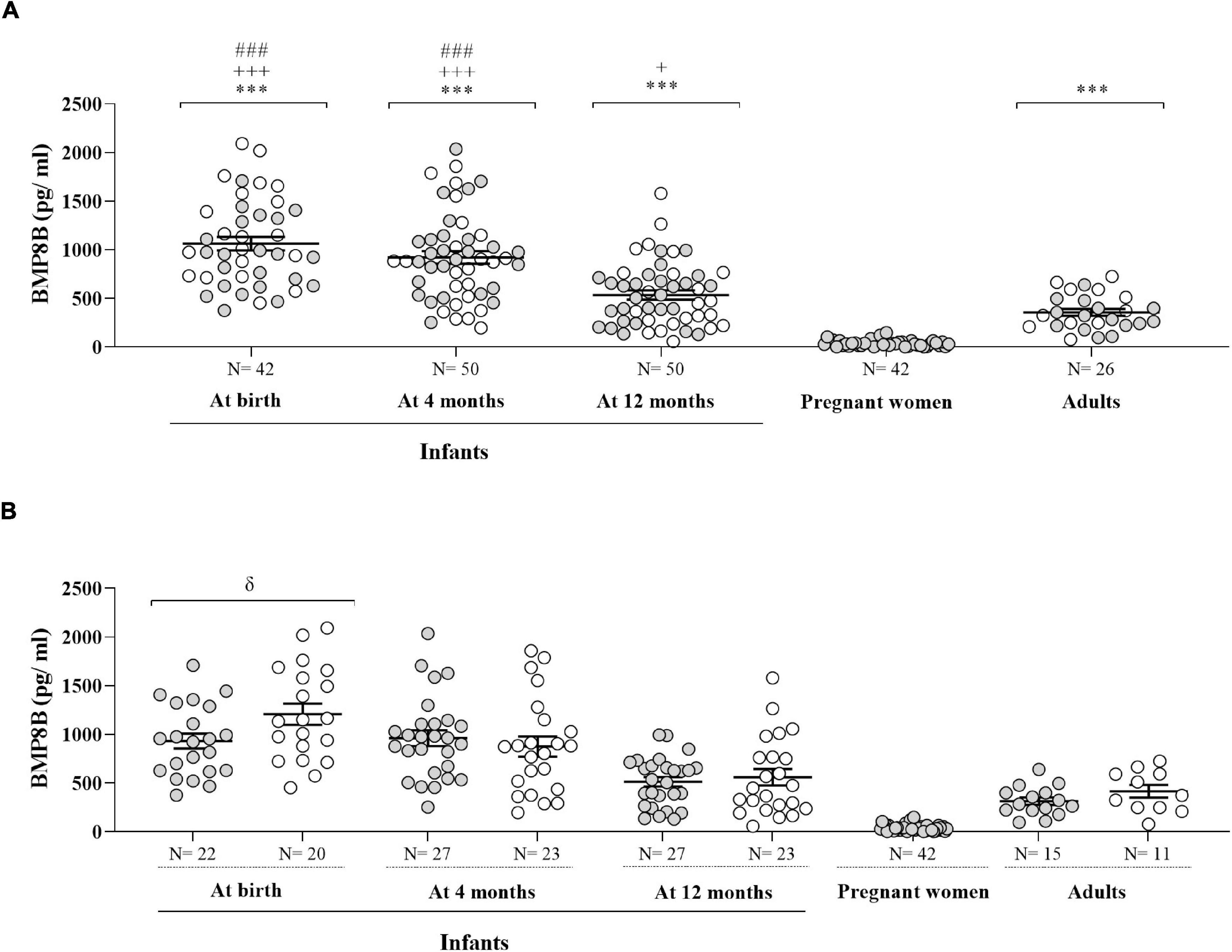
Figure 1. Circulating BMP8B concentrations in human infants at birth and at age 4 and 12 months, in mothers of those infants during the third trimester of pregnancy (gestational age: 35.3 ± 0.39 weeks) and in healthy adult women and men. Data are shown for the two sexes pooled (A) and split by sex (B). Gray dots represent girls, pregnant women and healthy adult women, and white dots correspond to boys and to healthy adult men. ***P < 0.001 vs. pregnant women; +P < 0.05 and +++P < 0.001 vs. healthy adults; ###P < 0.001 vs. 12 months; δ P = 0.04 vs. boys.
Bone morphogenetic protein-8B levels decreased in a different manner from birth to age 12 months in girls and in boys (Figure 2). While in girls BMP8B levels remained elevated from birth to 4 months and dropped significantly between age 4 and 12 months (Figure 2A), boys experienced a progressive decrease throughout the first year of life (Figure 2B). Indeed, significant differences between BMP8B concentrations at birth and at 4 months were only detected in boys. Interestingly, BMP8B levels were still elevated 36 h after birth in both girls (928 ± 390 pg/ml) and boys (1,656 ± 390 pg/ml) and tended to be higher in boys.
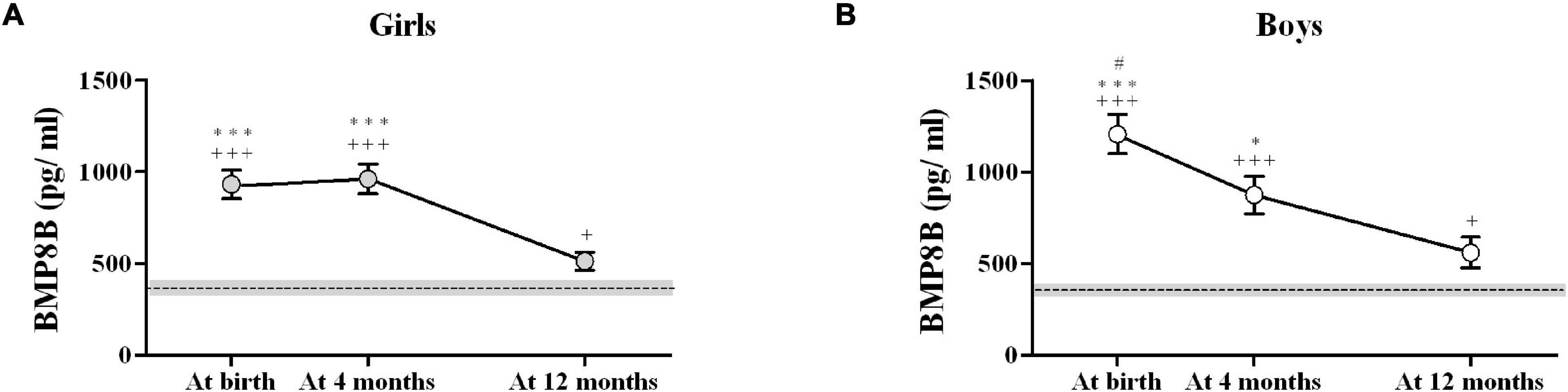
Figure 2. Longitudinal results of circulating BMP8B concentrations in infant girls (A) and boys (B). The dotted line depicts the mean values in apparently healthy adults (N = 26; 15 women and 11 men); the shaded area represents the standard error (SEM) in those healthy adults. #P < 0.05 vs. 4 months; *P < 0.05 and ***P < 0.001 vs. 12 months; +P < 0.05 and +++P < 0.001 vs. healthy adults.
The associations between circulating BMP8B concentrations and clinical, endocrine-metabolic, body composition and BAT activity variables are depicted in Supplementary Table 2. At 4 months, circulating BMP8B levels significantly correlated with bone mineral density (R = −0.383; P = 0.031) in the entire population, and with serum glucose levels (R = 0.467; P = 0.042) only in girls. In addition, the decrease in BMP8B levels in the entire population over the first year of life positively correlated with the increase in lean mass (R = 0.412; P = 0.036).
The expression levels of BMP8B mRNA in BAT, WAT and liver samples from human neonates and in human placenta and umbilical cord blood cells are shown in Figure 3. BMP8B expression was found to be significantly higher in BAT as compared to WAT, liver and cord blood cells.
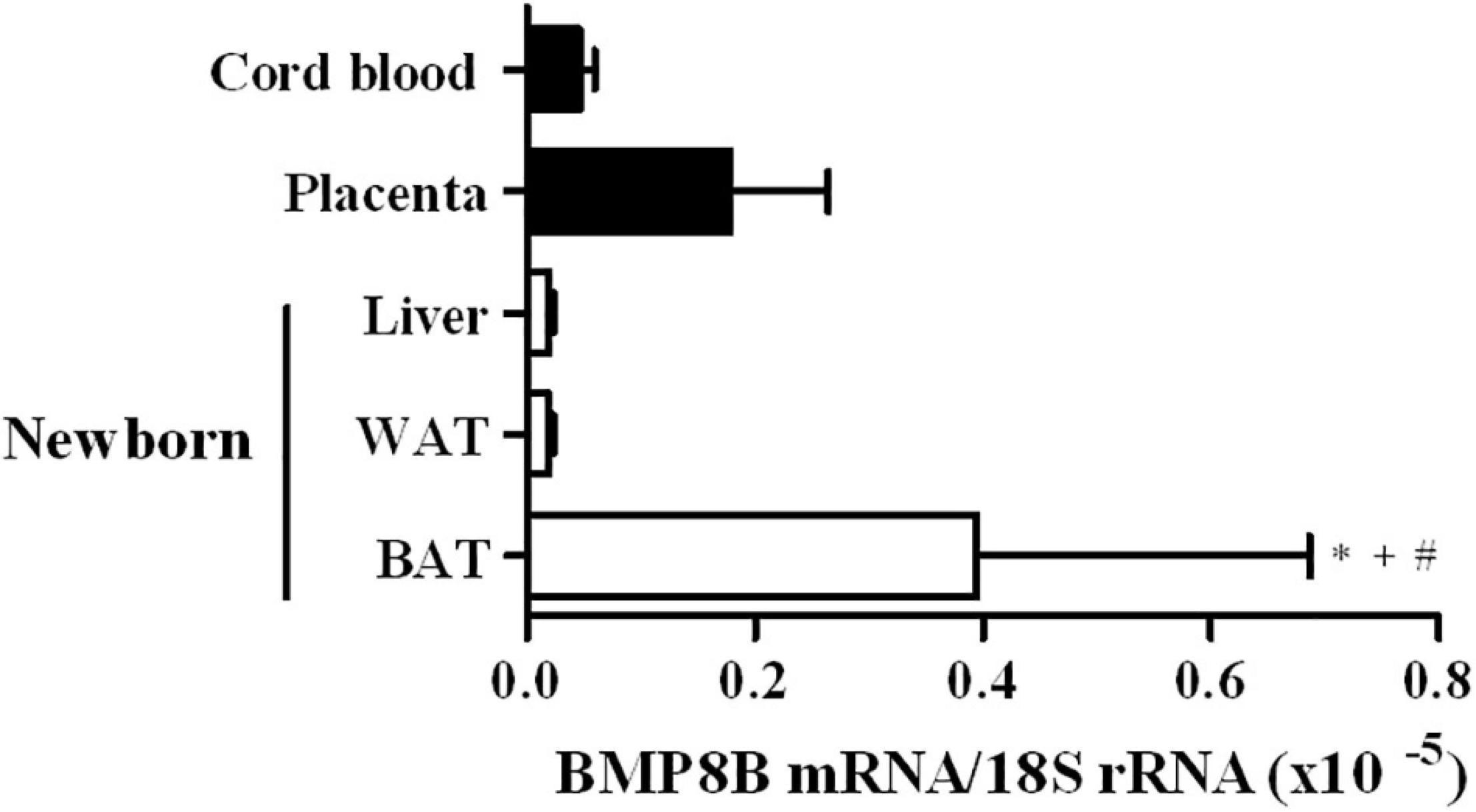
Figure 3. BMP8B expression levels in brown adipose tissue (BAT, N = 5), white adipose tissue (WAT, N = 5) and liver (N = 3) from newborns, and in placenta (N = 4) and cord blood cells (N = 4). Data are mean ± SEM of relative levels of BMP8B mRNA (BMP8B mRNA/18S rRNA). *P = 0.031 vs. cord blood cells; +P = 0.035 vs. liver; #P = 0.007 vs. WAT.
Discussion
The present study constitutes the first one reporting the levels of BMP8B–a brown adipokine involved in thermoregulation and metabolic homeostasis recently identified in experimental studies-across the first year of human development. The highest levels of BMP8B were found at birth, and declined progressively throughout the first year of life, where they were still above adult levels. This pattern is consistent with the especially high requirements of thermoregulation and high BAT activity in neonates early after birth (1). In fact, it is known that maintenance of normothermia at birth is of critical importance, as hypothermia is a challenging and not infrequent condition in newborns (15). Our findings are also consistent with a recent report indicating a positive correlation between BMP8B levels and BAT activity in neonates subjected to thermal stress stimulation in the first 2 days of life (8). Indeed, we found that BMP8B gene expression is the highest in BAT among other neonatal tissues analyzed, which provides the first direct evidence that BAT may be a potential major source of BMP8B in human neonates.
Interestingly, BMP8B levels at birth were higher in boys than in girls, and this trend persisted for at least the next 36 h. This may be in part related to reports of a lower body temperature in boys at birth (16) which may result in enhanced thermal stress, high BAT activation and subsequent BMP8B release. Most reports point to higher BAT activity in adult women than in adult men (17) and there are experimental data indicating that estrogens activate BAT thermogenesis (18) and BMP8B gene expression (19). Along these lines, we disclosed the presence of higher posterior-cervical BAT activity in 1 year-old girls relative to boys (3). In contrast, studies on sex differences in BAT activity in pre-school children have provided heterogeneous conclusions (20–22). Further research is warranted as there are currently no data on sex-related differences in BAT activity in human neonates in the first months of postnatal life.
We did not find significant correlations between BAT activity and BMP8B levels at age 1 year. This may indicate that the reported association between BMP8B levels and inducible BAT activity in 1–2-day-old neonates (8) is restricted to this very early development period characterized by intense thermoregulatory needs and does not happen later in development. It is also possible that the association between BMP8B and BAT activity is evidenced only when BAT activity is determined under acute induction of thermal stress, as in Urisarri et al., (8), and not in basal conditions. Moreover, we did not find significant associations between BMP8B levels and the main auxological, metabolic and endocrine parameters across the first year of life. Indeed, only marginal associations were found between BMP8B levels and each bone mineral density and gains in lean mass in the whole population; these associations could be reminiscent of the role of BMP8B, as member of the bone morphogenetic protein sub-family, in the ossification process (23) and also reflect the effects of gonadal steroids on both lean mass and circulating BMP8B levels (19). Most likely, though, the lack of strong associations could be due to the narrow range of variation in metabolic and endocrine parameters as well as in BAT activity among individuals in the first year of life under healthy conditions. In any case, and according to our findings, variations in BMP8B levels do not appear to be particularly informative of the individual metabolic status and BAT activity in apparently healthy human infants in the first year of life.
An additional noteworthy result in our study is the intense downregulation of BMP8B levels observed during late pregnancy relative to BMP8B levels in non-pregnant women. It has long been known that the thermogenic activity of BAT is decreased at term gestation in rodent models (24, 25). Although no such data on BAT activity are currently available in human pregnancy, low BMP8B levels in pregnant women may reflect reduced BAT thermogenic activity and subsequently reduced BMP8B secretion. On the other hand, the very low BMP8B levels in pregnant women suggest that the substantial expression of BMP8B mRNA in placenta does not result in relevant release of BMP8B to maternal circulation.
The current study has several limitations mostly related to obvious ethical restrictions for the invasiveness of some analytical procedures in healthy neonates. For example, BAT activity data couldn’t be obtained in unrestrained neonates immediately after birth and tissue BAT sampling had to be limited to necropsies from severely ill neonates and was obviously unfeasible in healthy 1-year old infants. However, the longitudinal nature of the study, with extensive information about endocrine-metabolic and body composition parameters to be related to BMP8B levels is a strength of this study, which is to our knowledge the first reporting the outcome of circulating BMP8B levels in humans in the first year of life.
In conclusion, changes in BMP8B levels appear to reflect overall changes in BAT activity in development. However, circulating BMP8B concentrations do not seem to be particularly useful as biomarker of individual variations in the metabolic-endocrine status and BAT activity of healthy infants in the first year of life.
Author’s Note
CG-B and LI are clinical investigators of CIBERDEM (Centro de Investigación Biomédica en Red de Diabetes y Enfermedades Metabólicas Asociadas, Instituto de Salud Carlos III, Madrid, Spain). AL-B is a clinical investigator of the I3 Fund for Scientific Research (Ministry of Science and Innovation, Spain). FV is an ICREA Academia researcher.
Data Availability Statement
The original contributions presented in the study are included in the article/Supplementary Material, further inquiries can be directed to the corresponding author/s.
Ethics Statement
The studies involving human participants were reviewed and approved by the Institutional Review Board of University of Barcelona, Sant Joan de Déu University Hospital. Written informed consent to participate in this study was provided by the participants’ legal guardian/next of kin.
Author Contributions
CG-B contributed to literature research, design of figures and tables, data collection, analysis, and interpretation, and wrote the manuscript. JV, CP, AG-N, and RC contributed to data collection, analysis, and interpretation. PC contributed to data analysis and interpretation. FZ and AL-B contributed to data interpretation, and reviewed and edited the manuscript. LI and FV contributed to study design, data interpretation, wrote the manuscript, and reviewed and edited the manuscript. All authors contributed to the article and approved the submitted version.
Funding
This work was supported by grants SAF2017-85772-R and PID2020-114112RB-100 from MICIN/AEI/10.13039/50110 0011033, Spain, grant PI18/00109 from Ministerio de Ciencia e Innovación, Instituto de Salud Carlos III, by the fondo Europeo de Desarrollo Regional (FEDER), grant (BR201601) from Sant Joan de Déu Research Institute, and grant PERIS-SLT017/20/000228 from the Agéncia de Qualitat i Avaluació Sanitaries de Catalunya, Generalitat de Catalunya, Spain.
Conflict of Interest
The authors declare that the research was conducted in the absence of any commercial or financial relationships that could be construed as a potential conflict of interest.
The reviewer ML, declared past co-authorship with the authors AG-N, RC, and FV, and the absence of any ongoing collaboration with any of the authors to the handling editor.
Publisher’s Note
All claims expressed in this article are solely those of the authors and do not necessarily represent those of their affiliated organizations, or those of the publisher, the editors and the reviewers. Any product that may be evaluated in this article, or claim that may be made by its manufacturer, is not guaranteed or endorsed by the publisher.
Acknowledgments
We thank Merche Morales for technical support. We acknowledge J. Kopecky, Academy of Sciences of the Czech Republic, for providing access to neonatal necropsy samples.
Supplementary Material
The Supplementary Material for this article can be found online at: https://www.frontiersin.org/articles/10.3389/fped.2022.869581/full#supplementary-material
References
1. Lidell ME. Brown adipose tissue in human infants. In: A Pfeifer, M Klingenspor, S Herzig editors. Brown Adipose Tissue. Handbook of Experimental Pharmacology. Vol. 251. Heidelberg: Springer (2019). p. 107–23. doi: 10.1007/164_2018_118
2. Villarroya J, Cereijo R, Gavaldà-Navarro A, Peyrou M, Giralt M, Villarroya F. New insights into the secretory functions of brown adipose tissue. J Endocrinol. (2019) 243:R19–27. doi: 10.1530/JOE-19-0295
3. Garcia-Beltran C, Cereijo R, Plou C, Gavaldà-Navarro A, Malpique R, Villarroya J, et al. Posterior cervical brown fat and CXCL14 levels in the first year of life: sex differences and association with adiposity. J Clin Endocrinol Metab. (2021) 107:e1148–58. doi: 10.1210/clinem/dgab761
4. Whittle AJ, Carobbio S, Martins L, Slawik M, Hondares E, Vázquez MJ, et al. BMP8B increases brown adipose tissue thermogenesis through both central and peripheral actions. Cell. (2012) 149:871–85. doi: 10.1016/j.cell.2012.02.066
5. Martins L, Seoane-Collazo P, Contreras C, González-García I, Martínez-Sánchez N, González F, et al. A functional link between AMPK and orexin mediates the effect of BMP8B on energy balance. Cell Rep. (2016) 16:2231–42. doi: 10.1016/j.celrep.2016.07.045
6. Vacca M, Leslie J, Virtue S, Lam BYH, Govaere O, Tiniakos D, et al. Bone morphogenetic protein 8B promotes the progression of non-alcoholic steatohepatitis. Nat Metab. (2020) 2:514–31. doi: 10.1038/s42255-020-0214-9
7. Mahli A, Seitz T, Beckröge T, Freese K, Thasler WE, Benkert M, et al. Bone Morphogenetic Protein-8B expression is induced in steatotic hepatocytes and promotes hepatic steatosis and inflammation in vitro. Cells. (2019) 8:457. doi: 10.3390/cells8050457
8. Urisarri A, González-García I, Estévez-Salguero Á, Pata MP, Milbank E, López N, et al. BMP8 and activated brown adipose tissue in human newborns. Nat Commun. (2021) 12:5274. doi: 10.1038/s41467-021-25456-z
9. Becher T, Palanisamy S, Kramer DJ, Eljalby M, Marx SJ, Wibmer AG, et al. Brown adipose tissue is associated with cardiometabolic health. Nat Med. (2021) 27:58–65. doi: 10.1038/s41591-020-1126-7
10. de Zegher F, Díaz M, Sebastiani G, Martín-Ancel A, Sánchez-Infantes D, López-Bermejo A, et al. Abundance of circulating preadipocyte factor 1 in early life. Diabetes Care. (2012) 35:848–9. doi: 10.2337/dc11-1990
11. Hondares E, Gallego-Escuredo JM, Flachs P, Frontini A, Cereijo R, Goday A, et al. Fibroblast growth factor-21 is expressed in neonatal and pheochromocytoma-induced adult human brown adipose tissue. Metabolism. (2014) 63:312–7. doi: 10.1016/j.metabol.2013.11.014
12. Díaz M, Aragonés G, Sánchez-Infantes D, Bassols J, Pérez-Cruz M, de Zegher F, et al. Mitochondrial DNA in placenta: associations with fetal growth and superoxide dismutase activity. Horm Res Paediatr. (2014) 82:303–9. doi: 10.1159/000366079
13. Ferrández-Longás A, Mayayo E, Labarta JI, Bagué L, Puga B, Rueda C, et al. Estudio longitudinal de crecimiento y desarrollo. Centro Andrea Prader. Zaragoza 1980-2002. In: A García-Dihinx, A Romo, A Ferrández-Long editors. Patrones de Crecimiento y Desarrollo en España. Atlas de Gráficas y Tablas. 1st ed. Madrid: Ergon (2004). p. 61–115.
14. Díaz M, Garcia C, Sebastiani G, de Zegher F, Lopez-Bermejo A, Ibáñez L. Placental and cord blood methylation of genes involved in energy homeostasis: association with fetal growth and neonatal body composition. Diabetes (2017) 66:779–84. doi: 10.2337/db16-0776
15. World Health Organization [WHO]. Maternal and Newborn Health/Safe Motherhood, Thermal Protection of the Newborn: A Practical Guide. Geneva: World Health Organization (1997).
16. Nagy E. Gender-related differences in rectal temperature in human neonates. Early Hum Dev. (2001) 64:37–43. doi: 10.1016/s0378-3782(01)00169-4
17. Kaikaew K, Grefhorst A, Visser JA. Sex differences in brown adipose tissue function: sex hormones, glucocorticoids, and their crosstalk. Front Endocrinol (Lausanne). (2021) 12:652444. doi: 10.3389/fendo.2021.652444
18. González-García I, Tena-Sempere M, López M. Estradiol regulation of brown adipose tissue thermogenesis. Adv Exp Med Biol. (2017) 1043:315–35. doi: 10.1007/978-3-319-70178-3_15
19. Grefhorst A, van den Beukel JC, van Houten EL, Steenbergen J, Visser JA, Themmen AP. Estrogens increase expression of bone morphogenetic protein 8b in brown adipose tissue of mice. Biol Sex Differ. (2015) 6:7. doi: 10.1186/s13293-015-0025-y
20. Malpique R, Gallego-Escuredo JM, Sebastiani G, Villarroya J, López-Bermejo A, de Zegher F, et al. Brown adipose tissue in prepubertal children: associations with sex, birthweight, and metabolic profile. Int J Obes (Lond). (2019) 43:384–91. doi: 10.1038/s41366-018-0198-7
21. Robinson LJ, Law J, Astle V, Gutiérrez-García M, Ojha S, Symonds ME, et al. Sexual dimorphism of brown adipose tissue function. J Pediatr. (2019) 210:166–72.e1. doi: 10.1016/j.jpeds.2019.03.003
22. Robinson L, Ojha S, Symonds ME, Budge H. Body mass index as a determinant of brown adipose tissue function in healthy children. J Pediatr. (2014) 164:318–22.e1. doi: 10.1016/j.jpeds.2013.10.005
23. Gelse K, Ekici AB, Cipa F, Swoboda B, Carl HD, Olk A, et al. Molecular differentiation between osteophytic and articular cartilage–clues for a transient and permanent chondrocyte phenotype. Osteoarthr Cartil. (2012) 20:162–71. doi: 10.1016/j.joca.2011.12.004
24. Trayhurn P. Thermogenesis and the energetics of pregnancy and lactation. Can J Physiol Pharmacol. (1989) 67:370–5. doi: 10.1139/y89-060
Keywords: bone morphogenetic protein-8B, brown adipose tissue, neonate, perinatal metabolism, adipokine
Citation: Garcia-Beltran C, Villarroya J, Plou C, Gavaldà-Navarro A, Casano P, Cereijo R, de Zegher F, López-Bermejo A, Ibáñez L and Villarroya F (2022) Bone Morphogenetic Protein-8B Levels at Birth and in the First Year of Life: Relation to Metabolic-Endocrine Variables and Brown Adipose Tissue Activity. Front. Pediatr. 10:869581. doi: 10.3389/fped.2022.869581
Received: 04 February 2022; Accepted: 01 March 2022;
Published: 24 March 2022.
Edited by:
Diego Gazzolo, SS. Annunziata Polyclinic Hospital, Chieti, ItalyReviewed by:
Miguel López, University of Santiago de Compostela, SpainP. Trayhurn, University of Liverpool, United Kingdom
Copyright © 2022 Garcia-Beltran, Villarroya, Plou, Gavaldà-Navarro, Casano, Cereijo, de Zegher, López-Bermejo, Ibáñez and Villarroya. This is an open-access article distributed under the terms of the Creative Commons Attribution License (CC BY). The use, distribution or reproduction in other forums is permitted, provided the original author(s) and the copyright owner(s) are credited and that the original publication in this journal is cited, in accordance with accepted academic practice. No use, distribution or reproduction is permitted which does not comply with these terms.
*Correspondence: Lourdes Ibáñez, bG91cmRlcy5pYmFuZXpAc2pkLmVz; Francesc Villarroya, ZnZpbGxhcnJveWFAdWIuZWR1
†These authors have contributed equally to this work and share first authorship
‡These authors have contributed equally to this work and share last authorship