- 1Université Paris Cité, CNRS, Integrative Neuroscience and Cognition Center (INCC), Paris, France
- 2CHArt Laboratory (Human and Artificial Cognition), EPHE-PSL, Paris, France
- 3Marian Wright Edelman Institute, San Francisco State University, San Francisco, CA, United States
- 4Service de Néonatologie, Centre Hospitalier Intercommunal, Créteil, France
- 5Service de Néonatologie, Groupe Hospitalier Paris Saint-Joseph, Paris, France
- 6Service de Néonatologie, AP-HP, Maternité Port Royal, Paris, France
- 7Service de Néonatologie, AP-HP, Hôpital Robert Debré, Paris, France
Aim: To examine the effects of an early home-based 8-week crawling intervention performed by trained therapists on the motor and general development of very premature infants during the first year of life.
Methods: At term-equivalent age, immediately following discharge from the Neonatal Intensive Care Unit (NICU), we randomly allocated 44 premature infants born before 32 weeks' gestation without major brain damage to one of three conditions in our intervention study: crawling on a mini-skateboard, the Crawliskate (Crawli), prone positioning control (Mattress), or standard care (Control). The Crawli and Mattress groups received 5 min daily at-home training administered by trained therapists for 8 consecutive weeks upon discharge from the NICU. The outcomes of greatest interest included gross motor development (Bayley-III) at 2, 6, 9, and 12 months (primary outcome) corrected age (CA), mature crawling at 9 months CA and general development at 9 and 12 months CA [Ages and Stages Questionnaires-3 (ASQ-3)]. The study was registered at www.clinicaltrials.gov; registration number: NCT05278286.
Results: A 3 (Condition) × 4 (Age) repeated measures ANOVA revealed that Crawli group infants had significantly higher Bayley-III gross motor development scores than Mattress and Control group infants. Crawli group infants also scored significantly higher on groups of Bayley-III items related to specific motor skills than infants in the other groups, including crawling at 9 months CA. We found significant differences in favor of the Crawli group in separate one-way ANOVAs at each of the ages we examined. A 3 (Condition) × 2 (Age) repeated measures ANOVA revealed that the Crawli group scored significantly higher than the Control group for the ASQ-3 total score and communication score and significantly higher for the fine motor score than the Control and Mattress groups. We found additional significant differences in favor of the Crawli group for other dimensions of the ASQ-3 in separate one-way ANOVAs at 9 and 12 months CA.
Interpretation: Early crawling training on a Crawliskate provides an effective way to promote motor and general development in very premature infants. The findings also provide clear evidence for a link between newborn crawling and more mature crawling later in development.
1. Introduction
Preterm birth, defined as delivery prior to 37 weeks gestational age (WGA), is a worldwide epidemic with a world global incidence of approximately 15 million preterm births per year (1). Prematurity continues to increase at a constant rate even though it appears to have stabilized recently in some countries (2). In parallel, the survival rate of children born prematurely has increased, particularly for very premature babies born before 32 WGA (around 20% of premature births), thanks to advances in medical practices and the quality of neonatal services. Paradoxically, this situation creates a real public health problem because the increase in the survival of very premature infants leads to an increase in children with disabilities during their development. Yearly, 5%–8% of very preterm born survivors develop cerebral palsy (CP), which represents the main cause of childhood disability (3, 4), resulting in significant delays and/or impairments in postural, manual and locomotor development, depending on the severity of the CP. Even if they do not develop CP, very premature babies remain at risk for motor problems, with 40% presenting developmental delays or impairments, including later sensorimotor anomalies that can impact gross motor development (5).
1.1. The centrality of motor/locomotor development in human development
Given the fundamental role movement plays in all human behavior, delays and impairments in the acquisition of motor skills during the first year of life have significant implications for the subsequent development of the brain and behavior and for an individual's ultimate quality of life. Researchers have established that the acquisition of motor skills during the first year of life has cascading effects on the later development of skills in the motor and psychological domains in at-term and preterm infants (6–13). Several studies have shown that the quality of early movements in preterm infants predict the quality of motor skills at later ages (12, 14, 15). Hua and colleagues recently showed that even a mild delay in crawling and walking onsets increases the risk for subsequent motor impairments in childhood (16). Research on the psychological revolutions that follow the acquisition of independent crawling and walking provides some of the strongest evidence for the fundamental contribution motor development makes to broader developmental outcomes (6, 7, 17–19). Researchers have linked the emergence and practice of independent crawling around 8–9 months of age to changes in perceptual-action coupling, spatial cognition, memory, social and emotional functioning and brain functioning (6, 17, 20–25) and the acquisition of walking to a range of developmental changes (26), particularly in the language domain (8, 27, 28).
The pervasive effect of locomotor experience on a child's development represents one of the primary reasons clinicians target locomotor skills for therapeutic intervention for children at risk for developmental delay. However, most of the interventions designed to promote locomotion begin well after the age of 12 months, largely because clinicians cannot diagnose locomotor problems and neurological disorders like CP until infants have already demonstrated delayed acquisition of motor skills. A recent systematic review by Dumuids-Vernet and collaborators reported that since the year 2000, only ten motor/locomotor interventions commenced before the age of one year in infants at risk for motor delays and only three targeted infants born preterm, the other studies targeted infants with an established disability at birth (Myelomeningocele, Down syndrome or high brain damage) (29).
A clear exception to the prevailing tendency to initiate interventions after the infant's first birthday is the Neurohabilitation method developed by Ferenc Katona over 30 years ago in Hungary (30, 31). The method focuses on frequent stimulation of “elementary neuromotor patterns” that contribute towards upright postures and locomotion, from the first months of life and continuing for several months thereafter. Unfortunately, much of the world knows little about Katona's work and methods because Katona published most of his findings in Hungarian (32, 33) and he believed it was ethically wrong to conduct a randomized controlled trial to compare a group of infants who had received his treatment to a group who had not (personal communication, April, 2013). However, Thalia Harmony and her colleagues have written comprehensively about the implementation of Katona's method in Mexico and its effectiveness for preterm and term infants with and without perinatal brain damage (34–36). For example, in one study she and her colleagues compared the neurodevelopmental outcomes at six to eight years of age of 20 children who had received Katona's Neurohabilitation treatment to the outcomes of 13 infants whose families had voluntarily discontinued treatment after one month. The treated infants received treatment for several months after discharge from the hospital and received consistent follow up evaluations until the evaluations between six and eight years of age. All infants had brain damage, as indicated by their first clinical and MRI examinations, and were matched in terms of age, gender, birth weight, Apgar, risk factors and diagnosis. The findings revealed that the treated group had a higher percentage (90%) of children with normal neurodevelopmental outcomes between six and eight years of age than the non-treated group (38%), with particularly clear differences for children born at or before 34 weeks gestational age.
1.2. The need for early motor/locomotor interventions for very premature infants
Given the heightened risk of motor/locomotor delays and impairments in very preterm infants, it is essential to have early intervention programs that clinicians could start even before the diagnosis of a behavioral or neurological disorder to improve long-term motor outcomes for these infants (37, 38). As indicated by a growing body of literature (37, 39) these programs should be implemented as early as possible to take advantage of the heightened plasticity of body and supra spinal structures (i.e., brain and corticospinal tract) and spinal circuitries (40–42). It is also essential (37) that these programs: (1) promote active, self-generated movements, (2) are high in frequency (37, 38, 42–44), and (3) target specific functions that are likely to generalize more broadly to motor and muscular development and be feasible for infants limited in their postural control and mobility (29, 45). Aside from Katona's Neurohabilitation method, what type of very early intervention could be implemented to stimulate active locomotion in very premature infants while adhering to these recommendations? Could an intervention designed to stimulate locomotor development be started as early as term equivalent age and would such an intervention facilitate the development of mature locomotion and other gross motor skills?
1.3. The link between primitive neonatal locomotion and mature crawling and walking
Human newborns can perform alternating locomotor movements of their legs at birth when supported upright in a stepping position on a table (46, 47) and can propel themselves forward when breast crawling on their mother's abdomen (48, 49) and via swimming movements when supported in the prone position in water (50). Researchers and clinicians have observed these patterns in preterm infants in neonatal care units when they crawl occasionally in their incubators (51) and when they perform stepping movements while supported upright under their armpits (30, 31). Though traditionally considered simple spinal reflexes, destined to rapidly disappear under the increasing influence of cortical maturation and playing no role in the development of independent locomotion, a growing body of literature has revealed that these primitive locomotor behaviors display much greater complexity than simple reflexes and serve as important precursors to mature crawling and walking. First, primitive locomotion does not disappear during development; biomechanical constraints mask its expression. For example, a rapid increase in fat mass during the first two months of life make the legs difficult to lift in the upright position if the infant has not received stepping practice, leading to an apparent disappearance of the stepping pattern. However, minimizing the gravitational force on the legs by submerging the infant in water (52, 53) or driving leg extension with a moving treadmill belt (54) can reactivate the stepping pattern between 2 and 6 months of age.
The second reason researchers now consider early motor patterns as complex behaviors is that several studies have shown that a range of higher order stimuli processed supra spinally can initiate and modulate newborn stepping and crawling, including visual, olfactory, and auditory stimuli (55–59). Finally, researchers have used a variety of experimental approaches to document a link between primitive and mature walking. One approach has used EMG recordings to show common patterns of muscle activation in newborn stepping and mature walking (60), even though such patterns remain plastic during development and can be fractioned into more controlling units (61). In another approach, researchers have shown that two months of daily stepping on a solid surface from birth leads to an earlier emergence and/or higher quality of mature walking in typically-developing infants (62, 63) and training stepping on a treadmill leads to an earlier emergence and higher quality of walking in infants with Down syndrome (64–68). Though less studied, researchers have shown that daily training of supported crawling in typically developing infants from four months of age onward leads not only to an earlier emergence of independent crawling and walking but boosts cognitive development (19). In addition, researchers have shown that Katona's method, which includes crawling as part of more comprehensive intervention for very young infants, also boosts motor and cognitive development (31, 35).
In summary, a large body of literature provides evidence for three important linkages in early development, each with connections to the other linkages. First, infants born very preterm have a much greater risk for delayed and/or impaired motor development, including locomotor development. Second, early primitive locomotor patterns appear to serve as precursors for later emerging independent crawling and walking. Third, the acquisition of crawling and walking make fundamental contributions to the development of a diversity of skills in the motor and psychological domains. Taken together, these linkages suggest that training locomotion in infants born very preterm could have positive and pervasive effects on their developmental outcomes. This suggestion raises some interesting questions. For example, what type of active locomotor movements should clinicians stimulate? Should the infant's movements be active or passive? When should the stimulation begin? Is there a preferred method or paradigm for providing the stimulation? Researchers have used a solid surface or treadmill to promote stepping in young infants, however stepping on these surfaces could create problems for very preterm infants, who are more fragile and often hypo or hypertonic. Furthermore, this type of stepping practice requires an experimenter to support the infant's body and head and constrains independent movement and forward propulsion. Moreover, supporting the infant's head and body under the armpits blocks potential locomotor movements of the arms and associated trunk and head movements, important in mature crawling and walking (69, 70).
1.4. The potential benefits of stimulating quadrupedal locomotion early in life
The stimulation of quadrupedal locomotion has several advantages over the stimulation of other motor activities, such as isolated head movements, stepping on a treadmill, or repetitive limb movements. Crawling represents a whole-body activity that involves the coordination and sequencing of multiple muscles and joints, leading to observable propulsion and reorientation of the infant to her environment, even in the newborn (30, 31, 71). Notably, the neuromuscular substrate underlying the quadrupedal organization of crawling also seems to underlie bipedal stepping and walking (69, 70). Consequently, the active practice of crawling potentially facilitates the development of not only quadrupedal locomotion but also bipedal locomotion, holding of the head, unsupported sitting (by strengthening the muscles of the neck and trunk) and standing. However, due to the weight of their head, even typically developing newborns cannot lift their trunk and head to move forward by themselves in a prone position at birth; an experimenter must support very young infants (30, 31, 72), preventing them from moving actively in any direction in their environment.
1.5. The Crawliskate
Given the aforementioned limitations in newborns' ability to propel themselves via crawling movements, we designed and constructed a mini-skateboard, which raises the head and trunk off the ground and allows the arms to move freely, to facilitate and stimulate quadrupedal locomotion during the first months of life (71). This mini-skateboard, known as the Crawliskate, promotes a lengthening of the spine and a neutral position of the head to facilitate the arm and leg movements necessary to generate independent propulsion (see a detailed description in the methods). The results of a previous study on 60 typically-developing newborns highlighted the advantages of using the Crawliskate to promote newborn crawling (71). The newborns propelled themselves significantly further on the Crawliskate and they demonstrated more mature crawling patterns, in terms of limb kinematics and interlimb coordination, than when observed crawling without the Crawliskate.
This current study investigates the feasibility and the effect of early crawling training using the Crawliskate on the locomotor and motor development of very preterm infants. The intervention complies with the essential program elements for early interventions described earlier in the introduction, particularly for infants with limited postural control or who are hypotonic, and it preserves the natural alignment of the head, neck and spine when the infant is in the prone position, which is particularly important for infants born premature. The “Crawliskate” can be used at even younger ages than a robotic device recently designed to train crawling in infants with or at risk for cerebral palsy (73, 74) and it has the added benefits of being portable and easy to use in the home. As noted earlier, studies of more than four hundred typically-developing newborns revealed that neonatal crawling can be regulated by visual, olfactory and auditory stimuli processed supra spinally, and it appears to engage and stimulate the neural circuitry underlying the control of mature bipedal and quadrupedal locomotion (58, 59, 71). Consequently, newborn crawling represents an ideal target for early intervention. Early crawling training has the potential to influence the plasticity and development of the supra spinal tracts, ultimately contributing to the development of not only locomotion but a range of gross motor skills and the cognitive and psychological skills to which these motor skills have been linked (6, 17, 19).
1.6. Objectives
Given the aforementioned observations of typically-developing newborns, we hypothesized that stimulating very premature infants' crawling on the Crawliskate would enhance their mature crawling (and possibly their walking), gross motor, and general development during the first year of life. We based our expectations on the findings from studies referred to earlier showing that five minutes of daily upright stepping practice over the first eight weeks of life accelerates walking onset of typically developing infants (62, 63), presumably because of the well-established neurological links between newborn stepping and later walking (60), and 15 min of daily crawling training in 5–9 month-old typically developing infants accelerates crawling onset, motor development and intellectual development relative to controls (19). We anticipated these latter training effects would be more pronounced in very young infants given their heightened body and brain plasticity (41, 42). The long-term aim of the study was to compare the motor and general development of infants up to 60 months corrected age (CA), however, in the current paper we present the motor and general outcomes up to 12 months CA.
2. Method
2.1. Trial design
The study was registered at www.clinicaltrials.gov; registration number: NCT05278286. Because of the limited number of subjects in our study due to the home-based nature of our intervention (see training protocol below), we chose not to include very preterm infants with major brain lesions because this would have required us to match lesion levels and locations across the three groups and we could not test a large enough sample to accomplish such matching. We randomly allocated forty-four very preterm infants without major brain lesions to one of three groups: Crawling training on the Crawliskate (Crawli), prone positioning on a Mattress training without the Crawliskate (Mattress), or standard care (Control), in which infants received pediatric checkups at 4, 9, 12, 18 and 24 months CA but no other treatment unless recommended by the pediatrician. It is important to note that we designed the Mattress condition to act as an active Hawthorne-effect control for the effect of prone positioning on the Crawliskate [prone positioning has positive effects on motor development, e.g., (75)] and the effect of visits from the osteopaths who implemented the training in the infants' homes. Because infants born between 24 + 0 and 27 + 6 (GA + Days) have higher risk for developmental delays than those born between 28 + 0 and 32 + 0 GA (76), the randomization process stratified infants on gestational age (24–27 + 6 GA vs. 28–32 GA) to ensure equal rates of GA at term equivalent age between groups.
2.2. Participants
We recruited eligible infants between March 2017 and October 2019 from four Neonatal Intensive Care Units (NICU) in Paris, France. Infants were included if they were born between 24 and 32 weeks of gestation (WG), lived within 10 kms of our laboratory (because of daily home visits by our team for the training groups), and could leave the NICU and begin training between 37 and 42 WG. Exclusion criteria included major brain damage defined by transcranial ultrasound, such as uni or bilateral IVH grade 3 and 4, periventricular leukomalacia, punctate lesions of the white matter and non-isolated lesions of posterior fossa (MRI was not used to screen for brain lesions as MRI was not available for all participants). Exclusion criteria also included Hypoxic-Ischemic Encephalopathy defined as infants presenting Apgar scores <5 at 5- and 10 min and a pH < 7 of the umbilical artery. Finally, exclusion criteria further included congenital anomalies, bronchopulmonary dysplasia with oxygen dependence after 36 WG, any digestive or other problems preventing prone positioning, limb deformities, and presence of retinopathy or sensory pathology that may delay motor development. We excluded infants if only one of the criteria was present. The study was approved by the ethical committee Ile de France 3 (CPP n°3465; ANSM n°2016-A01320-51). All parents provided written informed consent for their infants to participate in the study.
2.3. Detailed description of the Crawliskate
The Crawliskate, or Crawli, is a mini skateboard, adapted to newborns and young infants, that permits comfortable positioning on the belly. The slight upward slope (15 degrees) of the Crawli allows the upper part of the trunk and the head to be elevated, with the head resting on a platform in front (Figure 1D). The slope also allows maintenance of the natural flexion of the trunk and head, avoiding the possible hyper-extension of the head that can occur when the infant is placed directly in a prone position on a flat surface. The other advantage of the Crawli is that it frees the movements of the arms because the upper trunk is raised. Under these conditions, the arms can actively participate in the child's propulsion along with the legs (71). With the minimum slope of the Crawli at the infant's pelvis, the infant's legs rest on the surface of support, which allows the infant to learn to elevate the pelvis to make effective propulsive thrusts. To ensure the safety of the infant, he/she is secured to the Crawli by a system of fabric fins and straps that wrap the child on the skate (Figures 1A,B). Two stabilizers placed on each side and in front of the headrest prevent any lateral imbalance during the infant's propulsive pushes. The wheels placed under the Crawli allow the infant to move 360 degrees. The Crawli thus allows the infant to move actively in any direction by pushing with all four limbs (Figures 1B,C for a newborn on the Crawliskate).
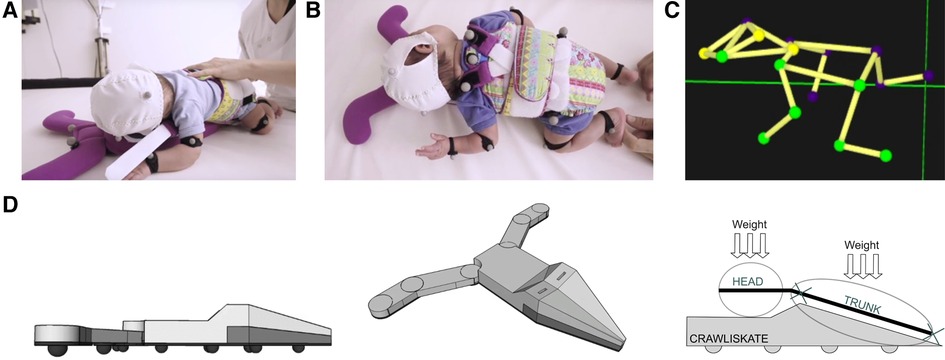
Figure 1. (A) A 2-day-old newborn resting on its belly and wrapped on the Crawli; (B) stimulation of quadrupedal propulsion on the Crawli; (C) 3D reconstruction of quadrupedal crawling on the Crawli; (D) structure of the Crawli. Patent: Barbu-Roth, M., Forma, V., Teulier, C., Anderson, D., Provasi, J., & Schaal, B. (2016). -Device for assisting the crawling of an infant. Patent WO2016009022. https://patentscope.wipo.int/search/en/detail.jsf?docId=WO2016009022.
2.4. Intervention at home
2.4.1. Training of the osteopaths in charge of the Crawli and Mattress training at home
We recruited thirty professionals to conduct the intervention. Before they implemented the intervention, Evelyne Soyez, a senior physiotherapist, expert in neurodevelopmental disorders, trained them. This training included learning Albert Grenier's method for handling fragile infants (77), an introduction to the issue of neurodevelopmental disorders, and an introduction to Claudine Amiel-Tison's neurological examination: ATNAT (78). Each osteopath also had to complete a Red Cross training course and earn a diploma in pediatric emergency care (IPSEN training, Initiation Premier Secours Enfants Nourrissons).
Then, the lead osteopath on our team, Marie-Victorine Dumuids-Vernet, who organized all training sessions for this study, taught the 30 osteopaths the protocol for the home visits and the training for the infants in the Crawli and Mattress training groups. The lead osteopath conducted the training initially in a classroom at our laboratory using a baby doll and the Crawliskate and then at home with the infant and parents. The lead osteopath also supervised each of the other osteopaths as they conducted the first seven days of training in the home. We monitored the quality of the training implemented by each osteopath by viewing daily videos of the training sessions at the end of each week of training. This standardized protocol allowed us to ensure the homogeneity of the trainings across the different trainers.
2.4.2. Training protocol
Each infant included in the Crawli and Mattress groups received 5 min of daily training for 8 consecutive weeks at home by an osteopath. The training started as soon as the infant was between 37 and 42 WG and left the NICU. The five minutes duration of crawling training on the Crawli is consistent with a study that trained upright stepping (63) and similar to a study that used a robotic device to train crawling in older infants (73, 74).
2.4.2.1. Crawli training
The osteopath gently placed the infant on the Crawli, with the head at the level of the clean bib on the headrest platform, and so the infant's hands and feet contacted a thin 1 meter × 2 meters mat beneath the device. The infants propelled themselves independently on the device. During the session, particularly during the first week of training, the osteopath occasionally placed a hand behind the infant's foot or hand to provide a surface to push against and/or extended the fingers of the infant's hand to facilitate pushing and teach the infant how to place her feet and hands to push effectively and efficiently (see Figure 2A and the beginning of the Supplementary video Crawli Home). The osteopath was careful not to create any forward propulsion, as the infant should be the only one actively initiating propulsion (see the Supplementary videos Crawli Home or Crawli Lab for a full independent crawling of the infant on the Crawli). During the five minutes of training, the caregiver could initiate a break if the infant became too agitated or regurgitated.
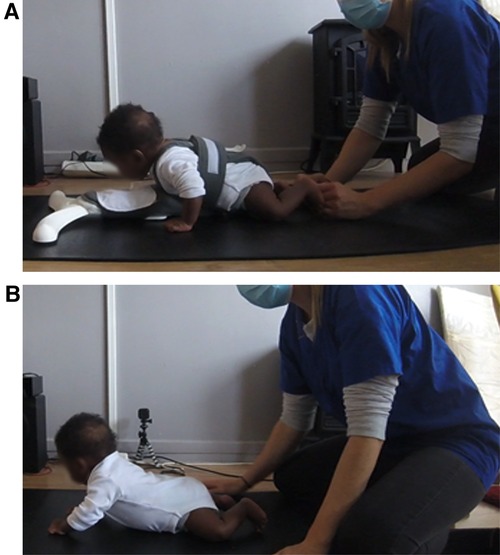
Figure 2. (A) photo of the beginning of a Crawli training session at home with the osteopath assisting placement of the feet of the infant on the mat. (B) Photo of a Mattress training session at home.
2.4.2.2. Mattress training
The mattress training was almost identical to the Crawliskate training, except that the infant was placed directly on his belly on the mat (Figure 2B and Supplementary video Mat Home) and the osteopaths did not use their hands to provide a surface for the infants to push against because pushing this way while the friction from the surface blocked the infant's forward progression could have increased the stress on the infant's neck to a harmful level. With the infant lying prone on the mat, the osteopath allowed the infant to make his or her own movements, taking care to monitor the upper airway clearance. Again, breaks were provided if the infant became too agitated or regurgitated and the session could be shortened if the infant could not be calmed down or showed signs of fatigue.
It is important to note that the parents played no role in training their infants other than to be present to calm their infants if they experienced distress. The osteopaths conducted all of the training. To ensure that parents did not practice training outside of the sessions performed by our team, we stored the training material (Crawliskate, mats, cameras) in a locked bag stored at the parents' home.
2.4.3. Safety monitoring
2.4.3.1. Before training
To ensure infants tolerated prone positioning, we performed a one-minute test with each infant on the Crawliskate or without the Crawliskate but prone on the mat before training started. During this test, we monitored the infant's oxygen saturation and heart rate while observing their behavior and checking how well they tolerated the position (see Supplementary videos Crawli Lab and Mat Lab to see infants tested at term equivalent age at our babylab). All infants tolerated the prone position on the Crawliskate or the mat.
2.4.3.2. During training
Diaries kept by the osteopaths and videos of the home trainings allowed us to check for any adverse events. We observed no adverse events.
2.4.3.3. After training
Each infant was followed by our senior physical therapist and osteopath, Evelyne Soyez, who assessed the infants using the ATNAT up to 12 months CA and discussed with the parents any concerns they had about their infants' development after 2 months CA (the senior physical therapist was blind to the infant's group). Parents were also asked to report activities they engaged in with their infants when their infants were 2, 6, 9, and 12 months CA, which was designed to track how the infants were stimulated after the training sessions. Neither the osteopaths, parents, nor the experimenters observed or reported any adverse events.
2.4.4. Recording of training and evaluation of traveled distance
All training sessions at home were videotaped with two GoPro cameras placed on each side of the mat to ensure the osteopaths implemented the training correctly and to measure distances traveled by the infants at each session. We measured the traveled distance covered by the infant at each session in cm by coding the videos of each session. We compared the mean traveled distances of the infants in the Crawli and Mattress groups to evaluate how well they could propel themselves as a function of the training protocol. We also charted the progression over time in the average distances covered each week between the first and last training session for the Crawli group (the Mattress group showed very limited propulsion—see the results section).
2.5. Outcomes
Figure 3 summarizes the outcome assessments. The assessors of all outcomes were blind to the infants’ group and the osteopaths who performed the training had no contact with the assessors. Note, we do not present the crawling efficiency data (assessed via three-dimensional kinematics) at term equivalent age, 2, 6, 9, and 12 months CA in this manuscript because we continue to reduce and analyze those data.
2.5.1. Gross and fine motor bayley assessments
We administered the Bayley III gross and fine motor assessments at 2, 6, 9, 12, and a full Bayley III assessment at 24 months CA (BSID-III ed (79)) to assess general motor development and to evaluate the development of specific motor milestones, especially mature crawling, but also head control, sitting, standing and walking (note, we do not include the Bayley III data at 24 months in this report as we plan to publish those data in a later report on the long-term effects of the intervention). Two trained testers administered the assessments. They both had over 20 years of experience assessing infant development and 10 and 8 years of experience administering the Bayley assessments. Both were blinded to infants' group assignment. Scaled scores were calculated. Before the start of the study the raters assessed 8 infants between 2 and 12 months of age using the BSID-III and were required to reach a high interrater reliability [intra class correlation coefficient (ICC) > 0.9]. During the study, 10% of the assessments were double scored by the two raters in real time and all assessments were videotaped so disagreements could be resolved afterward. The interrater reliability was excellent (ICC = 0.92).
2.5.2. ASQ-3 general assessment
We asked parents to complete the ASQ-3 questionnaire to evaluate their infants' general development in the communication, gross motor, fine motor, problem solving, and personal-social domains at 9, 12, 18, 24 and 60 months CA (note, we do not include the ASQ-3 at 18, 24 and 60 months in this report as we plan to publish those data in a later report on the long-term effects of the intervention), as performed in previous studies on premature infants (4, 5). We chose to administer the ASQ-3 from 9 months of age onward for two reasons. First, we did not want to overwhelm the parents at the beginning of the study by making too many demands on them. Second, we expected differences between groups to appear in the general domains assessed by the ASQ-3 after 8–9 months of age, when typically-developing infants generally acquire the ability to crawl independently. We based our expectations on the range of psychological changes researchers have documented following the onset of independent crawling.
2.5.3. Amiel-Tison neurological assessment (ATNAT)
The ATNAT clinical examination assesses the quality of musculoskeletal and neuromotor function responses to tonic and active stimulation (80). ATNAT scores were evaluated at term equivalent age and at 2, 6, 9 and 12 months CA by our senior physiotherapist, Evelyne Soyez, with 30 years of experience administering the ATNAT to ensure the groups were equivalent at the beginning of the study, to monitor for any deleterious effects of the training, and to diagnose any infants at heightened risk for a neurological disorder. She was blinded to the infants' group assignment.
2.5.4. Prechtl's general movement assessment (GMA)
The GMA examines the quality of the spontaneous movements of the infant while she is lying supine on a flat surface in a calm but alert state, stage 3 (81), without any external stimulation. The GMA was performed at term equivalent age and at 2 months CA. A specialist, Joëlle Provasi, blind to the infants' group allocation and trained to the Expert level by the Einspieler team, analyzed the videos and calculated a Motor Optimality Score (MOS) for each infant to compare the mean of the MOS between the groups at term equivalent age and at 2 months CA.
2.6. Sample size and randomization
The planned 1,600 home training sessions supervised by the 30 osteopaths constrained the sample size to 44. The stratified randomization process ensured we had a similar number of 24–27 + 6 and 28–32 GA infants in each group.
2.7. Statistical methods
We used Statistica software (TIBCO Software Inc.) to run the analyses. Differences are given as mean differences with 95% confidence intervals and effect sizes are reported with confidence intervals when the effects are significant.
2.7.1. Baseline data
We compared the perinatal characteristics of the infants and the parents' education level among groups using separate univariate ANOVAs.
2.7.2. Traveled distances during training
We compared the mean traveled distances by each infant for all the training sessions between the Crawli and Mattress groups using a student's T-test and reported effect sizes using Cohen's d.
2.7.3. ATNAT and MOS scores
We compared the ATNAT and MOS scores among the three groups using separate univariate ANOVAs at the different ages tested because the total scores change according to the age at which the test is administered.
2.7.4. Bayley gross and fine motor scaled scores
2.7.4.1. Primary analyses
The Bayley Gross and Fine Motor Scaled Scores at 2, 6, 9, and 12 months CA, which were normally distributed according to the Shapiro-Wilk statistic, were analyzed with 3(GROUP) × 4(AGE) ANOVAs with repeated measures on the AGE factor. We then performed separate one-way ANOVAs on GROUP at the four ages. Tukey's tests were used to specify main effects. Differences are given as mean differences with 95% confidence intervals and effect sizes are reported using partial η2.
2.7.4.2. Secondary analyses
To deepen the Bayley Gross Motor results, we performed a second level of analysis of the Gross Motor scores by grouping certain items of interest reflecting the acquisition of a motor function: head holding, sitting, crawling, static standing, position transfers, and walking. For this purpose, the Gross Motor items grouped under the following functions are:
- Head Control: Item 3 “Lift his/her head less than 3 s from the shoulder of the experimenter who holds him/her vertically in his/her arms”; Item 4 “Lift his/her head for 3 s from the shoulder of the experimenter who holds him/her vertically in his/her arms”
Item 9 “Lift his/her head for 15 s from the shoulder of the experimenter who holds him/her vertically in his/her arms”; Item 11 “Stable head when carried vertically in the arms of an experimenter who is moving around the room”.
- Sitting: Item 22 “Sitting alone 5 s without support”; Item 26 “Sitting alone 30 s without support”; Item 27 “Sitting alone 60 s while manipulating an object”; Item 28 “Sitting alone while rotating the trunk to catch objects bilaterally”.
- Crawling: Item 30 “Belly-crawling for 1 meter”; Item 31 “Stand in a four-legged position”
Item 34 “Crawling on his or her hands and knees over 5 feet”.
- Static Standing: Item 33 “Standing for at least 2 s with light hand support”; Item 36 “Bouncing movements while standing with support of both hands”; Item 40 “Standing alone at least 3 s without support”.
- Walking: Item 37 “Walking with light hand support”; Item 42 “Three steps without support”; Item 43 “Walking at least five independent controlled steps”.
- Transfers: Item 41 “Lying on his/her back, the child is able to get up and stand, without any assistance or support. He/she first rolls to a prone or quadruped position and then stands up on his/her feet”; Item 46 “Lying on his/her back, the child is able to get up and stand, without any assistance or support and without rolling into the prone or quadruped position first”.
To perform the item grouping analysis for each stage of gross motor maturation, we summed the raw scores of the items comprising each grouping. Because the data were not distributed normally, we used a non-parametric Kruskal-Wallis test. We weighted ranks by the age of the subject (the youngest child having the highest rank). For this analysis, we compared the data from the Crawli group with those from the Control group and those from the Mattress group.
2.7.5. Ages and stages questionnaires-3 (ASQ-3)
Consistent with our analysis of the Bayley Gross and Fine Motor scaled scores, we analyzed the Ages and Stages Questionnaires-3 scores at 9 and 12 months CA using 3(GROUP) × 2(AGE) ANOVAs with repeated measures on the AGE factor for the Total score and the scores for each of the five subscales of the questionnaire. We followed up any main effects of GROUP with Tukey tests. The Shapiro-Wilk test revealed the data were normally distributed. To provide greater specificity for the ASQ-3 results, we conducted separate one-way ANOVAs for the Total score and each of the subscale scores at 9 and 12 months CA. Tukey's tests were used to specify main effects. Differences are given as mean differences with 95% confidence intervals and effect sizes are reported using partial η2.
3. Results
As some subjects missed some tests during the longitudinal follow-up, not all the participants were included in each analysis. However, we did not use imputation to replace missing data with substituted values. That accounts for the different degrees of freedom for the various tests.
3.1. Participant flow and recruitment
Among 107 participants assessed for eligibility, 49 infants were included and randomly assigned to the Crawli (n = 17), Mattress (n = 15) or Control (n = 17) group. Five infants were excluded immediately after inclusion. Forty-four subjects were included in the final analysis with 15 in the Crawli group, 14 in the Mattress group and 15 in the Control group (See full description in Figure 4). The participants were predominantly Caucasian 11/15, 10/14 and 11/15 respectively in the Crawli, Mattress and Control groups. The others were of North or Central African descent.
3.2. Baseline data
There was no difference in perinatal characteristics and parental education level between groups (See full description in Table 1). There was no difference in either the ATNAT scores [F(2,41) = 0.21, p = 0.81, partial η2 = 0.01; Figure 5] or the Motor Optimality scores [F(2,33) = 1.71, p = 0.20, partial η2 = 0.93; Table 2] between groups at inclusion, confirming the homogeneity of the groups at inclusion.
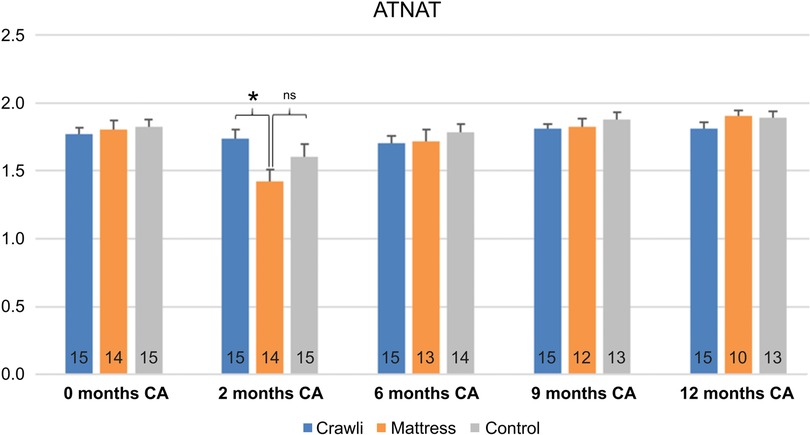
Figure 5. Comparison of ATNAT scores between groups at each age. The number of subjects is displayed at the bottom of each histogram. A score of 2 (on the y-axis) is considered as an optimal ATNAT score. Ages are in corrected age. Significant differences are indicated by * for p < .05 and ns indicates a non-significant difference.
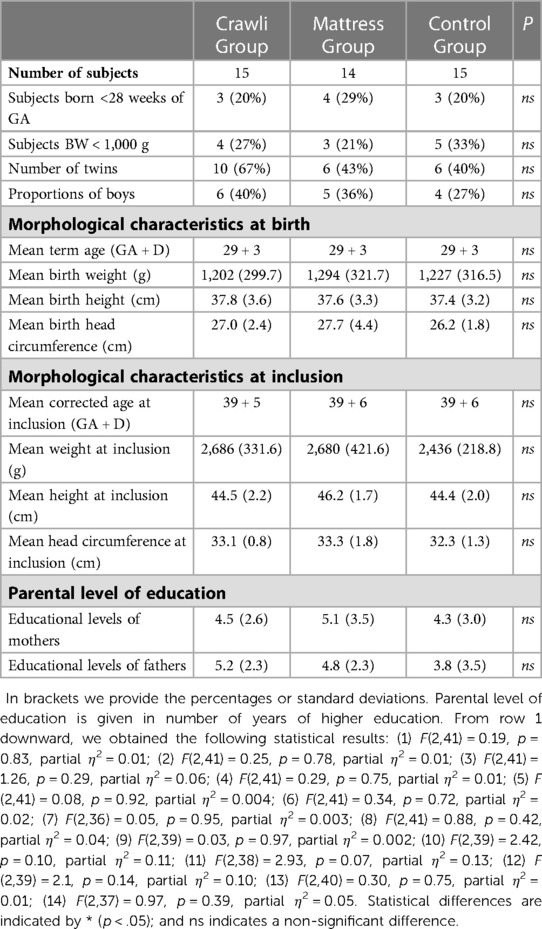
Table 1. Morphological characteristics (GA gestational age, BW birthweight, HC head circumference) and parents level of education.

Table 2. Motor optimality score (MOS) at term equivalent age (i.e., moment of inclusion in the study).
3.3. Training adherence, traveled distances during the sessions and possible harms
Adherence to the training was high, likely because the osteopaths were the ones who conducted the training in the infants' homes each day and this motivated the parents' compliance with the study. Of the 56 sessions, the Crawli group and Mattress groups completed an average of 52 (SD = 2) and 51 (SD = 2) sessions, respectively. The difference was not significant. The maximum number of sessions missed by a single infant was nine.
All infants trained in the Crawli group were able to move forward on the Crawliskate with a mean traveled distance per session of 138.7 cm (SD = 61.2) and a range from 68.1 to 242.3 cm (see Figure 6A). As expected, in contrast to the Crawli group, infants positioned prone on the mattress were only able to move between 0.12 and 12.7 cm (mean = 6.4 cm, SD = 4.4 cm) (T(27) = 8.07, p < 0.00001, Cohen's d = 3.0 [CI 95%(1.91- 4.06)]; Figure 6A). We observed a high variability in the mean distances traveled by the infants on the Crawliskate during each of the eight weeks (Figure 6B).
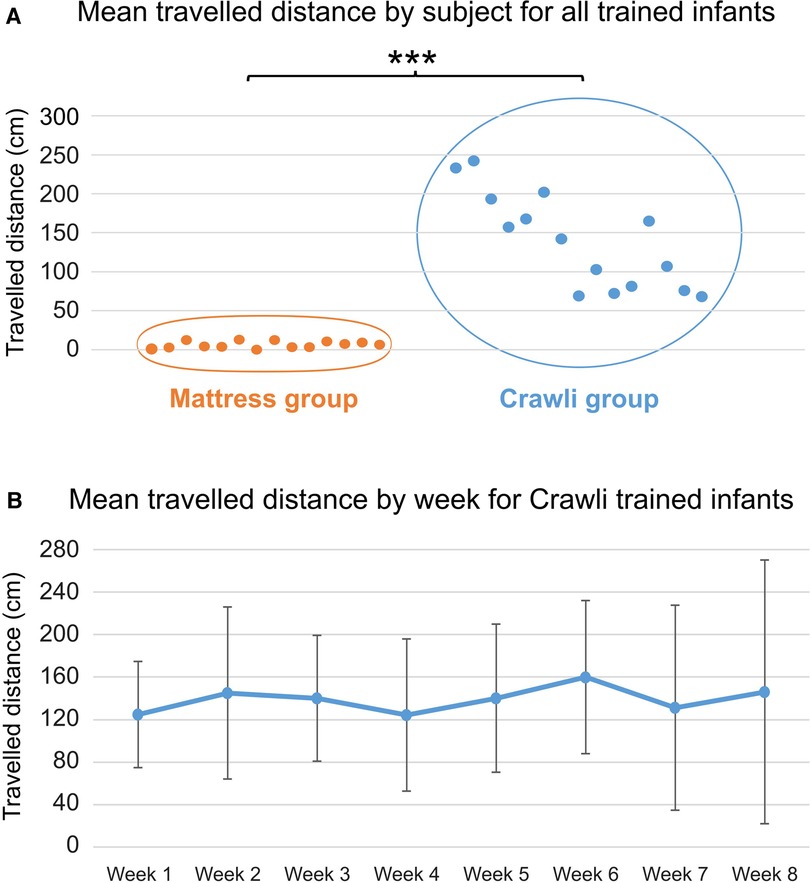
Figure 6. (A) mean distance covered per session by each subject in each group (orange = mattress group; blue = Crawli group) during his/her entire training. The distance covered (in cm) is represented on the y-axis and the subjects are distributed on the x-axis. The *** indicates a p-value <0.001. (B) Graphical representation of the mean distance (and SD) covered during each of the eight weeks of training for the Crawli group.
The osteopaths reported no harms to the trained infants and the ATNAT follow-up assessment at each age up to 12 months CA showed no deleterious effects of training in the Crawli and Mattress groups: similar ATNAT scores were observed between Crawli, Mattress and Control groups except for an unexpected lower score for the Mattress group than the Crawli group at 2 months CA (F(2,40) = 3.62, p = 0.04, partial η2 = 0.15 [CI 95%(0.08–0.55)]; HSD Tukey Crawli > Mattress, p = 0.03; Mattress = control, p = 0.28 and Crawli = control, p = 0.51) but not thereafter (ATNAT 6: F(2,40) = .13, p = 0.88, partial η2 = 0.01; ATNAT 9: F(2,39) = 1.00, p = 0.37, partial η2 = 0.05; ATNAT 12: F(2,38) = 0.98, p = 0.39, partial η2 = 0.05; Figure 5). The temporary lower score for the Mattress group at 2 months CA was due to a greater spinal axis hyper extension on the corresponding specific ATNAT item on spinal axis (data not shown). The groups demonstrated similar MOS at 2 months CA [F(2,38) = 1.32, p = 0.28, partial η2 = 0.06; Table 3].
3.4. Bayley gross motor scaled scores
All Gross Motor (GM) scaled scores are summarized in Figure 7 and Table 4.
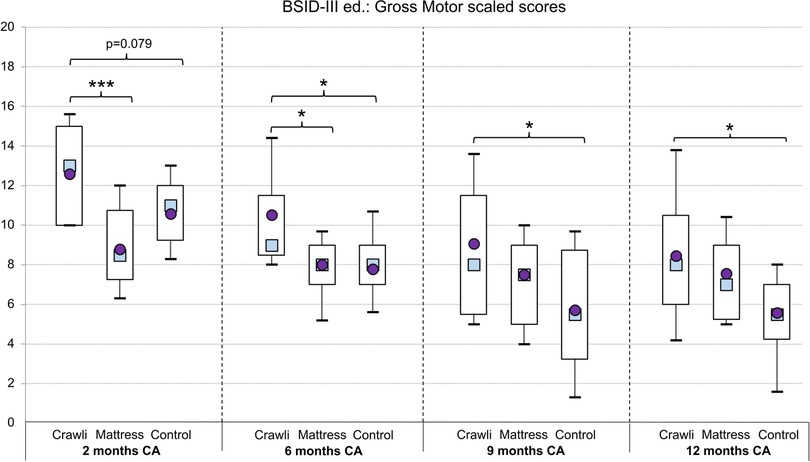
Figure 7. Gross motor scaled score. The boxes and whiskers represent the 10th, 25th, 75th and 90th percentiles with the mean represented by a circle and the median by a square. Significant differences are indicated with * for p < 0.05; ** for p < 0.01; *** for p < 0.001; p-values between 0.05 and 0.1 are reported in superscript.
3.4.1. Comprehensive ANOVA
The main effect of GROUP was significant, [F(2,37) = 7.39, p < 0.001], partial η2 = 0.28 [CI 95% (0.05–0.46)]. Tukey's test revealed the Crawli group scaled score was significantly higher than that of the Control group (p < 0.01) and the Mattress group (p < 0.05). The difference between the Control and Mattress group was not significant (p = 0.6). The main effect of AGE was significant, [F(3,111) = 21.07, p < 0.0001], partial η2 = 0.36 [CI 95% (0.21–0.47)], and the AGE x GROUP interaction was significant, [F(6,111) = 2.53, p = 0.02], partial η2 = 0.12 [CI 95% (0–0.20)]. However, because we used the scaled Bayley Gross Motor score, we did not use the Tukey test to follow up on the main effect of AGE in the ANOVA. Comparison of scaled scores across age groups is not particularly meaningful.
3.4.2. Univariate ANOVA at 2 months CA
The ANOVA at 2 months of corrected age (CA) revealed a significant GROUP effect [F(2,40) = 8.68, p < 0.001], partial η2 = 0.30 [CI 95% (0.07–0.47)]. The post-hoc test revealed the Crawli group scored significantly higher than the Mattress group (p < 0.001) and there was a trend for the Crawli group to score higher than the Control group (p = 0.08).
3.4.3. Univariate ANOVA at 6 months CA
The ANOVA at 6 months CA revealed a significant GROUP effect [F(2,40) = 5.30, p < 0.01], partial η2 = 0.21 [CI 95% (0.02–0.39)]. The post-hoc test revealed the Crawli group scores were significantly higher than those of the Control group (p < 0.05) and the Mattress group (p < 0.05).
3.4.4. Univariate ANOVA at 9 months CA
The ANOVA at 9 months CA revealed a significant GROUP effect [F(2,40) = 3.74, p < 0.05], partial η2 = 0.16 [CI 95% (0–0.33)]. The post-hoc test revealed the Crawli group scores were significantly higher than those of the Control group (p < 0.05).
3.4.5. Univariate ANOVA at 12 months CA
The ANOVA at 12 months CA revealed a significant GROUP effect [F(2,40) = 3.33, p < 0.05], partial η2 = 0.14 [CI 95% (0–0.32)]. The post-hoc test revealed the Crawli group scores were significantly higher than those of the Control group (p < 0.05).
3.4.6. Specific items
With reference to the secondary analyses of the Bayley items (Figure 8), the analyses revealed that the scores of the Crawli group were significantly higher: 1) for head control at 2 months CA compared to the Mattress group (p < 0.001; η2 = 0.62) and the Control group (p < 0.05; η2 = 0.18), 2) for mature crawling at 9 months CA compared to the Mattress group (p < 0.05; η2 = 0.11) and the Control group (p < 0.05; η2 = 0.12), and 3) for static standing (p < 0.01; η2 = 0.23) and the position transfers (p < 0.01; η2 = 0.26) compared to the Control group at 12 months CA. The Crawli group had scores higher than the Mattress group for sitting at 6 months CA (tendency p = 0.066; η2 = 0.09) and higher than the Control group for walking at 9 months CA (tendency p = 0.066; η2 = 0.09).
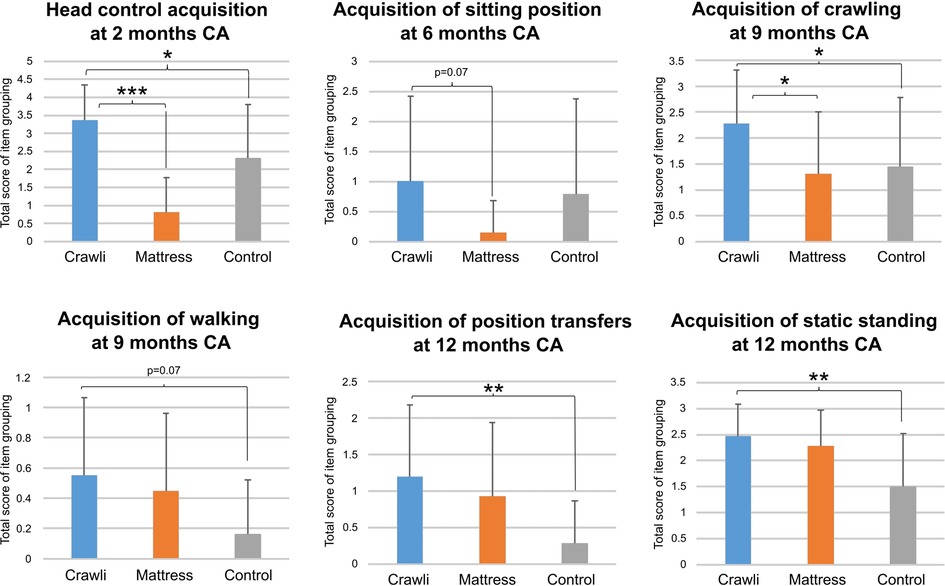
Figure 8. Graphical representation of raw scores of grouped items of the Bayley scales reflecting major gross motor skills. Statistical analyses were done using Kruskal-Wallis tests. Significant differences and trends are shown in bold: * for p < 0.05; ** for p < 0.01; *** for p < 0.001.
3.5. Bayley fine motor scaled scores
The 3 groups were similar in their Bayley Fine Motor scaled scores (results are summarized in Table 5).
3.5.1. Comprehensive ANOVA
The main effect of GROUP was not significant, [F(2,37) = 1.07, p = 0.38, partial η2 = 0.07]. The main effect of AGE was significant, (F(3,90) = 6.06, p = 0.0008, partial η2 = 0.17 [CI 95% (0.03–0.28)] and the AGE x GROUP interaction was not significant, [F(2,37) = 0.94, p = 0.45 partial η2 = 0.06]. However, because we used the scaled Bayley Fine Motor score, we did not use the Tukey test to follow up on the main effect of AGE in the ANOVA. Comparison of scaled scores across age groups is not particularly meaningful. Despite the non-significant findings for GROUP and the AGE x GROUP interaction, we conducted the univariate ANOVAs at each age group for the sake of completeness.
3.5.2. Univariate ANOVA at 2 months CA
The ANOVA at 2 months of corrected age (CA) did not reveal a significant GROUP effect [F(2,37) = 2.62, p = 0.09, partial η2 = 0.12].
3.5.3. Univariate ANOVA at 6 months CA
The ANOVA at 6 months CA did not reveal a significant GROUP effect [F(2,39) = 3.08, p = 0.06, partial η2 = 0.14].
3.5.4. Univariate ANOVA at 9 months CA
The ANOVA at 9 months CA did not reveal a significant GROUP effect [F(2,38) = 0.75, p = 0.48, partial η2 = 0.04].
3.5.5. Univariate ANOVA at 12 months CA
The ANOVA at 12 months CA did not reveal a significant GROUP effect [F(2,36) = 2.10, p = 0.14, partial η2 = 0.10].
3.6. ASQ-3 scores
ASQ-3 results are summarized in Tables 6, 7.
3.6.1. Comprehensive ANOVA for total score
The main effect for GROUP [F(2,35) = 6.02, p < 0.01], partial η2 = 0.26 [CI 95% (0.03–0.44)], was significant for the Total score. The post-hoc test revealed the Crawli total score was significantly higher than that of the Control group (p < 0.01). The difference between the Crawli and Mattress group and between the Control and Mattress group were not significant (respectively p = 0.1 and p = 0.4). The main effect for AGE [F(1,35) = 15.81, p < 0.001], partial η2 = 0.31 [CI 95% (0.08–0.50)] was also significant but not the AGE x GROUP interaction [F(2,35) = 0.35, p = 0.71], partial η2 = 0.02). However, because the Ages and Stages Questionnaires scores are also scaled according the child's age, we did not use the Tukey test to follow up on the main effect of AGE in the ANOVA. Comparison of scores across age groups is not particularly meaningful.
3.6.2. Comprehensive ANOVA for gross motor subscale
For the Gross Motor subscale, neither the main effect for GROUP [F(2,35) = 2.75, p = 0.08; partial η2 = 0.14], nor AGE [F(1,35) = 3.06, p = 0.09, partial η2 = 0.08], nor the AGE x GROUP interaction were significant [F(2,35) = 0.41, p = 0.67, partial η2 = 0.02].
3.6.3. Comprehensive ANOVA for fine motor subscale
For the Fine Motor subscale, the main effect of GROUP was significant, [F(2,35) = 6.75, p < 0.01], partial η2 = 0.28 [CI 95% (0.40–0.46)], however, neither the main effect of AGE [F(1,35) = 0.45, p = 0.51, partial η2 = 0.01], nor the AGE x GROUP interaction were significant [F(2,35) = 0.03, p = 0.97], partial η2 = 0.001). The post-hoc test revealed the Crawli group scored significantly higher than the Mattress group (p < 0.05) and the Control group (p < 0.01).
3.6.4. Comprehensive ANOVA for problem solving subscale
For the Problem Solving subscale, neither the main effect of GROUP [F(2,35) = 1.28, p = 0.29, partial η2 = 0.07], nor AGE [F(1,35) = 0.82, p = 0.37, partial η2 = 0.02], nor the AGE x GROUP interaction [F(2,35) = 2.43, p = 0.10; partial η2 = 0.12] were significant.
3.6.5. Comprehensive ANOVA for communication subscale
For the Communication subscale, the main effect of GROUP [F(2,35) = 4.05, p < 0.05], partial η2 = 0.19 [CI 95% (0–0.37)] and AGE [F(1,35) = 5.78, p < 0.05], partial η2 = 0.14 [CI 95% (0–0.35)] and the AGE x GROUP interaction [F(2,35) = 3.54, p < 0.05], partial η2 = 0.17 [CI 95% (0–0.35)] were significant. The post-hoc test revealed the Crawli group scored significantly higher than the Control group (p < 0.05).
3.6.6. Comprehensive ANOVA for personal social subscale
For the Personal Social subscale, the main effect of AGE [F(1,35) = 20.38, p < 0.001], partial η2 = 0.37 [CI 95% (0.12–0.55)] was significant, however neither the main effect of GROUP [F(2,35) = 1.99, p = 0.15, partial η2 = 0.10] nor the AGE x GROUP interaction [F(2,35) = 1.02, p = 0.37, partial η2 = 0.05] were significant.
3.6.7. Univariate ANOVAs at 9 months CA
For the Total score at 9 months CA, the ANOVA was significant [F(2,35) = 5.95, p < 0.01], partial η2 = 0.25 [CI 95% (0.03–0.44)]. The post-hoc test revealed the Crawli group scored significantly higher than the Control group (p < 0.01). For the Gross Motor score at 9 months CA, the ANOVA was significant [F(2,35) = 3.54, p < 0.05], partial η2 = 0.17 [CI 95% (0–0.35)]. The post-hoc test revealed the Crawli group scored significantly higher than the Control group (p < 0.05). For the Fine Motor score at 9 months CA, the ANOVA was significant [F(2,35) = 3.52, p < 0.05], partial η2 = 0.17 [CI 95% (0–0.35)]. The post-hoc test revealed the Crawli group scored significantly higher than the Control group (p < 0.05). For the Problem Solving score at 9 months CA, the ANOVA was not significant [F(2,35) = 0.79, p = 0.46, partial η2 = 0.04]. For the Communication score at 9 months CA, the ANOVA was significant [F(2,35) = 4.96, p < 0.05], partial η2 = 0.22 [CI 95% (0.01–0.41)]. The post-hoc test revealed the Crawli group scored significantly higher than the Control group (p < 0.05). There was a trend for the Crawli group to score higher than the Mattress group (p = 0.08). For the Personal Social score at 9 months CA, the ANOVA trended toward significance [F(2,35) = 2.80, p = 0.07], partial η2 = 0.14 [CI 95% (0–0.32)]. The Crawli group scores were almost significantly higher than those of the Control Group (p = 0.07).
3.6.8. Univariate ANOVAs at 12 months CA
For the Total score at 12 months CA, the ANOVA was significant [F(2,40) = 4.77, p < 0.05], partial η2 = 0.19 [CI 95% (0.01–0.37)]. The post-hoc test revealed the Crawli group scored significantly higher than the Control group (p < 0.05). For the Gross Motor score at 12 months CA, the ANOVA was not significant [F(2,40) = 1.95, p = 0.16, partial η2 = 0.09]. For the Fine Motor score at 12 months CA, the ANOVA was significant [F(2,40) = 5.21, p < 0.001], partial η2 = 0.21 [CI 95% (0.01–0.38)]. The post-hoc test revealed the Crawli group scored significantly higher than the Control group (p < 0.05) and the Mattress group (p < 0.05). For the Problem Solving score at 12 months CA, the ANOVA trended towards significance [F(2,40) = 3.13, p = 0.05], partial η2 = 0.13 [CI 95% (0–0.31)]. The Crawli group scores tended to be higher than those of the Mattress group (p = 0.06). For the Communication score at 12 months CA, the ANOVA was significant [F(2,40) = 3.45, p < 0.05], partial η2 = 0.15 [CI 95% (0–0.32)]. However, the post-hoc test failed to identify the significant differences with the Crawli group scores tending to be higher than those of the Control group (p = 0.07) and the Mattress group (p = 0.07); and the Mattress group scores tending to be higher than those of the Control group (p = 0.07). For the Personal Social score at 12 months CA, the ANOVA was not significant [F(2,40) = 0.45, p = 0.64, partial η2 = 0.02].
4. Discussion
Prematurity continues to increase at a constant rate, even though it appears to have stabilized recently in some countries (2). However, we lack consensus on methods to train the locomotor and gross motor skills of premature infants at risk of disability at a very early age (29). The goal of the present study was to test the feasibility of using a mini-skateboard, the Crawliskate, to train very premature infants without major brain injuries, but who were nevertheless at heightened risk for developmental delay and/or disability, to crawl daily in the home from term equivalent age onward and to determine the effects of this type of training on their motor and general development up to 12 months CA. Although we had a small sample size, our results show not only the feasibility of our intervention but more importantly that daily stimulation of the quadrupedal gait of premature infants upon discharge from the NICU has positive effects on their development of mature crawling at 9 months CA and on their gross motor and general development.
4.1. Training adherence and possible harms
First, we observed excellent adherence to the training, likely because the osteopaths we recruited conducted the training in the infants' homes each day. The results show the feasibility of such early home-based crawling training performed 5 min/day for 8 consecutive weeks following discharge from the NICU. Specifically, all infants in the Crawli groups were able to propel themselves using quadrupedal movements with the help of the Crawliskate without deleterious effects, as shown by similar scores on the ATNAT at 2, 6, 9 and 12 months CA and GMA at 2 months CA. As predicted, infants positioned directly on the mattress without the Crawliskate were unable to move very far and unexpectedly developed a temporary head and trunk hyper extension at 2 months CA.
4.2. Traveled distances during Crawli training
It is remarkable that even at this very early age, premature infants could travel long distances with the help of the Crawliskate, up to a maximum of 2.5 meters in only 5 min for some of the infants. However, we observed a large variability within and between infants in traveled distance. We can likely attribute the variability in distance traveled to variation in the infant's state of alertness at each of the training sessions. Sometimes infants appeared too tired or sleepy to move much during the session. We also saw variability within sessions, with infants often starting to crawl, then stopping, then resuming again at the end of the session. Consequently, we found it difficult to construct a learning profile for crawling during the 8 consecutive weeks of training for each infant. We are presently working to code the number of leg and arm steps during each session to determine the distance traveled per step, which should provide a better representation of the progression of crawling skill during the training.
4.3. Effect of the neonatal crawling intervention on mature Crawling
One of the remarkable effects we saw from stimulating the very premature infants crawling at an early age was the impact it had on the development of mature crawling at 9 months CA, with infants in the Crawli group showing significantly more mature crawling than infants in the Mattress and Control groups. This result is not simply the consequence of prone positioning because the Mattress group infants demonstrated a similar level of crawling competence as infants in the Control group. The effect of early crawling training on mature crawling confirms, with a new paradigm, the existence of a link between neonatal and mature locomotion. Researchers have reported previously a link between stepping and walking by showing that daily stepping on a solid surface from birth enhances the emergence and quality of mature walking in typically-developing infants (62, 63) and training stepping on a treadmill leads to an earlier emergence and higher quality of walking in infants with Down syndrome (64, 65, 67, 68, 82). Our results on crawling training also confirm, but with a much earlier intervention, results reported 40 years ago showing that daily training of supported crawling in typically developing infants from four months of age onward leads to an earlier emergence of independent crawling and walking (19). However, we did not find an effect of our intervention on walking, as scored by Bayley items at 12 months CA, only a strong trend for the Crawli infants to have higher scores relative to the Control group on the items related to walking at 9 months CA (p = 0.07). This result for walking could result from the fact that, in contrast to typically-developing infants, very premature infants may need more than 5 min per day and/or more than 8 weeks of crawling training to accelerate the development of their walking proficiency. Alternately, the Bayley items related to walking at 12 months CA may lack the sensitivity to detect subtle differences in the quality of walking between groups of very preterm infants without brain damage. As we also collected the spatio-temporal parameters of these infants walking patterns on a GAITRite pressure sensitive walkway at 24 months CA, we may still find an effect of our intervention on walking.
4.4. Effect of the crawling intervention on gross motor development
The crawling intervention had positive effects on infants' gross motor development when the Bayley scores were aggregated over the four age periods. When looking by age, the Crawli group had higher scores than the Control group at 6, 9 and 12 months CA, that is 10 months after the end of the crawling intervention. The Crawli group had higher scores than the Mattress group at 2 and 6 months CA. The similar gross motor scores between the Crawli and Mattress groups at 9 and 12 months CA may be the result of the limited duration of the training, although it is important to note (see the previous section) that the Crawli group had significantly higher scores on the specific Bayley items related to mature crawling at 9 months CA relative to the Mattress group. If the training lasted longer than two months and/or the duration of each training session was increased, the group differences at 9 and 12 months may have been larger and more likely to be significant, particularly with a larger sample. For example, in Katona's method the frequency and duration of training is much higher and the intervention continues for a much longer period of time (30, 31, 35, 36). However, we think a more likely explanation for the attenuated differences between Crawli and Mattress groups at older ages is the additional time parents in the Mattress group reported continuing to place their infants in the prone position for more than two hours per day after the training (58% and 60% for the Mattress group between 2 and 6 months CA and 6 to 9 months CA, respectively and 21% and 38% for the Crawli group during the same age periods). Tummy time, practiced when the infant is older, is known to have beneficial effects on infant motor development (75). Although the Bayley Gross Motor and ASQ-3 scores of the Crawli and Mattress groups did not differ significantly between 9 and 12 months CA, it is pertinent to note that the Crawli group's scores were always higher than the Mattress group's scores. Moreover, the Mattress group's scores were never significantly higher than the Control group's scores at any age tested.
The generalized and enduring effects of the crawling training on the infants' motor development, particularly relative to the Control group, are quite surprising in the context of prior work which generally shows that motor practice effects are task specific (83). The generalized effects on gross motor development may reflect the whole-body nature of crawling, which not only stimulates the central and peripheral nervous system but also engages a large percentage of the infant's musculature.
4.5. Effect of the crawling intervention on general development
The broader effects of crawling training on the total ASQ-3 score, and specifically on the scores representing the communication domain, is likely a result of the additional experiences associated with moving through space and their perceptual consequences. These experiences occurred during the eight weeks of training and as a function of the faster acquisition of crawling in the Crawli group. As noted in the introduction, considerable research has linked locomotor development to developmental changes in perception-action coupling, spatial cognition, memory, and social and emotional functioning during the first year of life (6, 17, 84) and to language development at 12 months of age (8, 28). Researchers have speculated that independent locomotion allows infants to have new experiences which in turn recruit the processes that drive developmental change in domains far removed from the locomotor domain (6, 17). Taken together, these findings highlight that an intervention focused on training a specific motor skill, crawling, can have broad effects on the motor domain of functioning as well as on other domains of functioning seemingly unconnected to the motor domain.
4.6. Possible mechanisms underlying the effects of the early crawling intervention
The fact that Crawli but not Mattress training facilitates the development of mature crawling permits different hypotheses. Although when infants lie prone on a mattress they cannot propel themselves very far, they make numerous leg movements (not arm movements), which are inefficient but present. This observation suggests that one of the central components of the “Crawli effect” is propulsion and/or the ability to perform coordinated quadrupedal leg and arm movements. It is impossible to list here the many possible mechanisms behind the effects of Crawli training but some would be especially interesting to investigate in the future. These include the effect of training on the morphology and composition of the leg and arm muscles and on the quadrupedal inter-limb coordination and the intra-limb coordination. It would also be interesting to investigate the evolution of muscle synergies using electromyography in relation to training. Another mechanism likely playing an important role in the effect of Crawli training is the stimulation of perception-action loops between the propulsion actively generated by the infant and the resulting proprioceptive, visual and auditory consequences associated with movement through the environment (36). We intentionally restricted motivational and visual/auditory stimuli in this study during training to minimize potential bias. However, it would be particularly interesting to add visual, auditory, and/or olfactory stimuli during clinical trainings given prior research showing that these stimuli can facilitate newborn stepping and crawling (55–59), especially if these stimuli were combined with parental encouragements.
4.7. Limitations
The main limitation of this intervention is the restricted number of participants, which was a function of the number of osteopaths needed to supervise the large number of home training sessions. However, this design feature was necessary to ensure the training protocol was administered faithfully and to monitor for any possible adverse effects of the training. The small sample size meant that it was not possible to do subgroup analyses within the Crawli group to see, for example, whether any individual difference characteristics explained why some infants derived more benefit from the intervention than others or to see if infants who traveled greater distances during training also had better developmental outcomes. Another limitation of the intervention was the decision to exclude infants with major brain lesions. However, the success of the current intervention suggests the Crawli intervention should now be tested on infants with brain damage. A final design limitation was the daily training time limited to 5 min and the decision to end training after 8 weeks. A longer duration of training may have further increased the efficacy of the intervention.
5. Conclusion
Our early training in crawling intervention facilitated the acquisition of mature independent crawling and had broad effects on development, which were evident from 2 to 12 months of age, but were strongest at two and six months of age. With a longer training duration, the effects might have been even stronger. The feasibility and efficacy of our intervention for very premature infants without major cerebral lesions opens new opportunities to apply this method to a larger number of infants and to infants with brain lesions. Training early crawling on a Crawliskate is a relatively inexpensive, simple, and effective way to stimulate premature infants' motor and general development during the first year of life.
Author's note
Part of our research was supported by the SATT-Erganeo, a French agency linked to the University and established to help researchers take innovative ideas and products to market. The funding they provide is quite similar to the funding provided by NIH SBIR and STTR programs in the US, both of which are designed to stimulate technological innovation. However, SATTErganeo had no influence on any aspect of this study as they had no role in the design of our study, our data collection, analysis process and interpretation.
Data availability statement
The raw data supporting the conclusions of this article will be made available by the authors, without undue reservation.
Ethics statement
The studies involving human participants were reviewed and approved by Ethical committee Ile de France 3 who gave ethical approval for this work (authorization: CPP [Comité de Protection des Personnes] n°3465; ANSM [Agence Nationale de Sécurité du Médicament et des produits de santé] n°2016-A01320-51). Written informed consent to participate in this study was provided by the participants’ legal guardian/next of kin.
Written informed consent was obtained from the minor(s)' legal guardian/next of kin for the publication of any potentially identifiable images or data included in this article.
Author contributions
M-VD-V co-supervised trainings, collected the data, coded crawling movements, carried out the statistical analyses and drafted the initial manuscript. VF supervised trainings at home and crawling testing. JP administered Prechtl's GMA, the Bayley assessments and the ASQ-3 Questionnaires. DIA assisted with the study design, provided input on the statistical analyses, reviewed and revised the manuscript. EH provided input on the statistical analyses and revised the manuscript. ES administered the Amiel-Tison Neurological Assessment. MS, CH and LC coded crawling movements. LG coded crawling distance during trainings. VH administered the Bayley assessments and the ASQ-3 Questionnaires. LG performed statistical analyses. LB, CD and GD recruited the infants, analyzed their characteristics, and recorded the parental level of education. VB assisted with the study design, supervise the ethical approval and recruited infants. MB-R designed the study, supervised the ethical approval, data collection and statistical analyses and drafted, reviewed and revised the manuscript. All authors approved the final manuscript as submitted and agree to be accountable for all aspects of the work. All authors had complete access to the study data that support the publication. All authors contributed to the article and approved the submitted version.
Funding
This work was supported by the grant ANR-20-CE17-0014 from the Agence Nationale de la Recherche, the grant Project-106 from the SATT-Erganeo (Société Accélération des Transferts de Technologies) and a fellowship (ED261) from the French Government to support the doctoral thesis of Marie-Victorine Dumuids-Vernet.
Acknowledgments
A preprint of this paper is available on medRxiv (85). We would like to thank all the infants and parents who participated in this study and all the osteopaths who performed the 1,600 total home visits for their enthusiastic support during this study.
Conflict of interest
MB-R, DIA and JP are co-authors on the patent for the crawling device used in the intervention.
The remaining authors declare that the research was conducted in the absence of any commercial or financial relationships that could be construed as a potential conflict of interest.
Publisher's note
All claims expressed in this article are solely those of the authors and do not necessarily represent those of their affiliated organizations, or those of the publisher, the editors and the reviewers. Any product that may be evaluated in this article, or claim that may be made by its manufacturer, is not guaranteed or endorsed by the publisher.
Supplementary material
The Supplementary Material for this article can be found online at: https://www.frontiersin.org/articles/10.3389/fped.2023.1198016/full#supplementary-material
Video Crawli Home
Video of a premature girl experiencing her first session on the Crawliskate at home. While this is her first experience with the Crawliskate, the infant is able to propel herself in a short time. The beginning of the video shows the osteopath helping the infant by repositioning her feet and arms on the mattress.
Video Crawli Lab
The video shows a test on the Crawliskate at our babylab of a premature infant just discharged from the NICU before the home training started. His tolerance for the Crawliskate was evaluated by recording his oxygen saturation and heart rate.
Video Mat Home
Video of a premature infant prone on the Mattress without the help from the Crawliskate. While the infant preforms many leg movements, arm movements are mostly blocked and propulsion is impeded.
Video Mat Lab
The video shows a test on the Mattress at our babylab of a premature infant just discharged from the NICU before the home training started.
Abbreviations
CA, corrected age; NICU, neonatal intensive care unit; CP, cerebral palsy; ATNAT, Amiel-Tison neurological assessment; BSID-III, bayley scale of infant development: third edition; ASQ-3, ages and stages questionnaires -3; GMA, general movements assessment; WG, weeks of gestation; GA, gestational age; WGA, weeks gestational age; GM, gross motor; FM, fine motor.
References
1. Purisch SE, Gyamfi-Bannerman C. Epidemiology of preterm birth. Semin Perinatol. (2017) 41:387–91. doi: 10.1053/j.semperi.2017.07.009
3. Rosenbaum P, Paneth N, Leviton A, Goldstein M, Bax M, Damiano D, et al. A report: the definition and classification of cerebral palsy April 2006. Dev Med Child Neurol. (2007) 49:8–14. doi: 10.1111/j.1469-8749.2007.tb12610.x
4. Pierrat V, Marchand-Martin L, Arnaud C, Kaminski M, Resche-Rigon M, Lebeaux C, et al. Neurodevelopmental outcome at 2 years for preterm children born at 22 to 34 weeks’ gestation in France in 2011: ePIPAGE-2 cohort study. Br Med J. (2017) 16:j3448. doi: 10.1136/bmj.j3448
5. Pierrat V, Marchand-Martin L, Marret S, Arnaud C, Benhammou V, Cambonie G, et al. Neurodevelopmental outcomes at age 5 among children born preterm: EPIPAGE-2 cohort study. Br Med J. (2021) 28:n741. doi: 10.1136/bmj.n741
6. Campos JJ, Anderson DI, Barbu-Roth MA, Hubbard EM, Hertenstein MJ, Witherington D. Travel broadens the mind. Infancy. (2000) 1:149–219. doi: 10.1207/S15327078IN0102_1
7. Flensborg-Madsen T, Mortensen EL. Infant developmental milestones and adult intelligence: a 34-year follow-up. Early Hum Dev. (2015) 91:393–400. doi: 10.1016/j.earlhumdev.2015.04.006
8. Iverson JM. Developmental variability and developmental cascades: lessons from motor and language development in infancy. Curr Dir Psychol Sci. (2021) 30:228–35. doi: 10.1177/0963721421993822
9. Libertus K, Hauf P. Editorial: motor skills and their foundational role for perceptual, social, and cognitive development. Front Psychol. (2017) 8:301. doi: 10.3389/fpsyg.2017.00301
10. Oudgenoeg-Paz O, Mulder H, Jongmans MJ, van der Ham IJM, Van der Stigchel S. The link between motor and cognitive development in children born preterm and/or with low birth weight: a review of current evidence. Neurosci Biobehav Rev. (2017) 80:382–93. doi: 10.1016/j.neubiorev.2017.06.009
11. Wang T-N, Howe T-H, Hinojosa J, Weinberg SL. Relationship between postural control and fine motor skills in preterm infants at 6 and 12 months adjusted age. Am J Occup Ther. (2011) 65:695–701. doi: 10.5014/ajot.2011.001503
12. Zuccarini M, Guarini A, Savini S, Faldella G, Sansavini A. Do 6-month motor skills have cascading effects on 12-month motor and cognitive development in extremely preterm and full-term infants? Front Psychol. (2020) 11:1297. doi: 10.3389/fpsyg.2020.01297
13. Flensborg-Madsen T, Mortensen EL. Predictors of motor developmental milestones during the first year of life. Eur J Pediatr. (2017) 176:109–19. doi: 10.1007/s00431-016-2817-4
14. Fallang B, Øien I, Hellem E, Saugstad OD, Hadders-Algra M. Quality of reaching and postural control in young preterm infants is related to neuromotor outcome at 6 years. Pediatr Res. (2005) 58:347–53. doi: 10.1203/01.PDR.0000170898.60160.09
15. Lefebvre F, Gagnon M-M, Luu TM, Lupien G, Dorval V. In extremely preterm infants, do the movement assessment of infants and the Alberta infant motor scale predict 18-month outcomes using the bayley-III? Early Hum Dev. (2016) 94:13–7. doi: 10.1016/j.earlhumdev.2016.01.012
16. Hua J, Williams GJ, Jin H, Chen J, Xu M, Zhou Y, et al. Early motor milestones in infancy and later motor impairments: a population-based data linkage study. Front Psychiatry. (2022) 13:31. doi: 10.3389/fpsyt.2022.809181
17. Anderson DI, Campos JJ, Witherington DC, Dahl A, Rivera M, He M, et al. The role of locomotion in psychological development. Front Psychol. (2013) 4:440. doi: 10.3389/fpsyg.2013.00440
18. Diamond A. Close interrelation of motor development and cognitive development and of the cerebellum and prefrontal Cortex. Child Dev. (2000) 71:44–56. doi: 10.1111/1467-8624.00117
19. Lagerspetz K, Nygåkd M, Strandvik C. The effects of training in crawling on the motor and mental development of infants. Scand J Psychol. (1971) 12:192–7. doi: 10.1111/j.1467-9450.1971.tb00623.x
20. Bell MA, Fox NA. Individual differences in object permanence performance at 8 months: locomotor experience and brain electrical activity. Dev Psychobiol. (1997) 31:287–97. doi: 10.1002/(SICI)1098-2302(199712)31:4%3C287::AID-DEV6%3E3.0.CO;2-N
21. Burnay C, Cordovil R. Crawling experience predicts avoidance of real cliffs and water cliffs: insights from a new paradigm. Infancy. (2016) 21:677–84. doi: 10.1111/infa.12134
22. Herbert J, Gross J, Hayne H. Crawling is associated with more flexible memory retrieval by 9-month-old infants. Dev Sci. (2007) 10:183–9. doi: 10.1111/j.1467-7687.2007.00548.x
23. Kretch KS, Franchak JM, Adolph KE. Crawling and walking infants see the world differently. Child Dev. (2014) 85:1503–18. doi: 10.1111/cdev.12206
24. Schwarzer G. How motor and visual experiences shape infants’ visual processing of objects and faces. Child Dev Perspect. (2014) 8:213–7. doi: 10.1111/cdep.12093
25. Schwarzer G, Gehb G, Kelch A, Gerhard-Samunda T, Jovanovic B. Locomotion training contributes to 6-month-old infants’ mental rotation ability. Hum Mov Sci. (2022) 85:102979. doi: 10.1016/j.humov.2022.102979
26. Mulder H, Oudgenoeg-Paz O, Verhagen J, van der Ham IJM, Van der Stigchel S. Infant walking experience is related to the development of selective attention. J Exp Child Psychol. (2022) 220:105425. doi: 10.1016/j.jecp.2022.105425
27. Iverson J. Developing language in a developing body: the relationship between motor development and language development. J Child Lang. (2010) 37:229–61. doi: 10.1017/S0305000909990432
28. Walle EA, Campos JJ. Infant language development is related to the acquisition of walking. Dev Psychol. (2014) 50:336–48. doi: 10.1037/a0033238
29. Dumuids-Vernet M-V, Provasi J, Anderson DI, Barbu-Roth M. Effects of early motor interventions on gross motor and locomotor development for infants at-risk of motor delay: a systematic review. Front Pediatr. (2022) 10:589. doi: 10.3389/fped.2022.877345
30. Katona F. Clinical neuro-developmental diagnosis and treatment. In: Zelazo P, Barr R, Zelazo P, editors. Challenges to developmental paradigms: Implications for theory, assessment and treatment. New York: Grune & Stratton (1989). p. 167–87.
31. Katona F. Developmental clinical neurology and neurohabilitation in the secondary prevention of pre and perinatal injuries of the brain. In: Vietze P, Vaughan H, editors. Early identification of infant with developmental disabilities. Philadelphia: Grune & Stratton (1988). p. 121–44.
32. Katona F. Ontogenesis of the human nervous system (in Hungarian). 1st ed. Budapest: Medicina Konyvkiadó Rt (2001).
33. Katona F. An orienting diagnostic system in neonatal and infantile neurology. Acta Paediatr Hung. (1983) 24:299–314.6673734
34. Harmony T. Outcome of infants at risk of brain damage after katona neurohabilitation therapy. Int J Neurorehabil. (2017) 04:277. doi: 10.4172/2376-0281.1000277
35. Harmony T, Barrera-Reséndiz J, Juárez-Colín ME, Carrillo-Prado C, del Consuelo Pedraza-Aguilar M, Asprón Ramírez A, et al. Longitudinal study of children with perinatal brain damage in whom early neurohabilitation was applied: preliminary report. Neurosci Lett. (2016) 611:59–67. doi: 10.1016/j.neulet.2015.11.013
36. Harmony T. Early diagnosis and treatment of infants with prenatal and perinatal risk factors for brain damage at the neurodevelopmental research unit in Mexico. Neuroimage. (2021) 235:117984. doi: 10.1016/j.neuroimage.2021.117984
37. Morgan C, Fetters L, Adde L, Badawi N, Bancale A, Boyd RN, et al. Early intervention for children aged 0 to 2 years with or at high risk of cerebral palsy: international clinical practice guideline based on systematic reviews. JAMA Pediatr. (2021) 175:846–58. doi: 10.1001/jamapediatrics.2021.0878
38. Novak I, Morgan C, Fahey M, Finch-Edmondson M, Galea C, Hines A, et al. State of the evidence traffic lights 2019: systematic review of interventions for preventing and treating children with cerebral palsy. Curr Neurol Neurosci Rep. (2020) 20:3. doi: 10.1007/s11910-020-1022-z
39. Spittle A, Orton J, Anderson PJ, Boyd R, Doyle LW. Early developmental intervention programmes provided post hospital discharge to prevent motor and cognitive impairment in preterm infants. Cochrane Database Syst Rev. (2015) 2015. doi: 10.1002/14651858.CD005495.pub4
40. Eyre J. Corticospinal tract development and its plasticity after perinatal injury. Neurosci Biobehav Rev. (2007) 31:1136–49. doi: 10.1016/j.neubiorev.2007.05.011
41. Dewolf AH, Sylos Labini F, Ivanenko Y, Lacquaniti F. Development of locomotor-related movements in early infancy. Front Cell Neurosci. (2021) 14:623759. doi: 10.3389/fncel.2020.623759
42. Ulrich BD. Opportunities for early intervention based on theory, basic neuroscience, and clinical science. Phys Ther. (2010) 90:1868–80. doi: 10.2522/ptj.20100040
43. Cappellini G, Sylos-Labini F, Dewolf AH, Solopova IA, Morelli D, Lacquaniti F, et al. Maturation of the locomotor circuitry in children with cerebral palsy. Front Bioeng Biotechnol. (2020) 8:998. doi: 10.3389/fbioe.2020.00998
44. Anderson PJ, Treyvaud K, Spittle AJ. Early developmental interventions for infants born very preterm—what works? Semin Fetal Neonatal Med. (2020) 25:101119. doi: 10.1016/j.siny.2020.101119
45. Blauw-Hospers CH, Hadders-Algra M. A systematic review of the effects of early intervention on motor development. Dev Med Child Neurol. (2005) 47:421–32. doi: 10.1017/S0012162205000824
46. Forssberg H. Ontogeny of human locomotor control I. Infant stepping, supported locomotion and transition to independent locomotion. Exp Brain Res. (1985) 57:480–93. doi: 10.1007/BF00237835
47. McGraw MB. From reflex to muscular control in the assumption of an erect posture and ambulation in the human infant. Child Dev. (1932) 3:291. doi: 10.2307/1125356
48. Widström A-M, Ransjö-Arvidson AB, Christensson K, Matthiesen A-S, Winberg J, Uvnäs-Moberg K. Gastric suction in healthy newborn infants effects on circulation and developing feeding behaviour. Acta Paediatr. (1987) 76:566–72. doi: 10.1111/j.1651-2227.1987.tb10522.x
49. Widström A-M, Lilja G, Aaltomaa-Michalias P, Dahllöf A, Lintula M, Nissen E. Newborn behaviour to locate the breast when skin-to-skin: a possible method for enabling early self-regulation. Acta Paediatr. (2011) 100:79–85. doi: 10.1111/j.1651-2227.2010.01983.x
50. McGraw MB. Swimming behavior of the human infant. J Pediatr. (1939) 15:485–90. doi: 10.1016/S0022-3476(39)80003-8
51. Thoman EB, Ingersoll EW. Learning in premature infants. Dev Psychol. (1993) 29:692–700. doi: 10.1037/0012-1649.29.4.692
52. Thelen E, Fisher DM. Newborn stepping: an explanation for a “disappearing” reflex. Dev Psychol. (1982) 18:760–75. doi: 10.1037/0012-1649.18.5.760
53. Thelen E, Fisher DM, Ridley-johnson R. The relationship between physical growth and a newborn reflex. Infant Behav Dev. (1984) 7:479–93. doi: 10.1016/S0163-6383(84)80007-7
54. Thelen E, Ulrich BD. Hidden skills: a dynamic systems analysis of treadmill stepping during the first year. Monogr Soc Res Child Dev. (1991) 56:1–103. doi: 10.2307/1166099
55. Barbu-Roth M, Anderson DI, Desprès A, Provasi J, Cabrol D, Campos JJ. Neonatal stepping in relation to terrestrial optic flow. Child Dev. (2009) 80:8–14. doi: 10.1111/j.1467-8624.2008.01241.x
56. Barbu-Roth M, Anderson DI, Despre A, Streeter RJ, Cabrol D, Trujillo M, et al. Air stepping in response to optic flows that move toward and away from the neonate. Dev Psychobiol. (2014) 56:1142–9. doi: 10.1002/dev.21174
57. Forma V, Anderson DI, Goffinet F, Barbu-Roth M. Effect of optic flows on newborn crawling. Dev Psychobiol. (2018) 60:497–510. doi: 10.1002/dev.21634
58. Hym C, Forma V, Anderson DI, Provasi J, Granjon L, Huet V, et al. Newborn crawling and rooting in response to maternal breast odor. Dev Sci. (2021) 24:e13061. doi: 10.1111/desc.13061
59. Hym C, Dumuids M, Anderson DI, Forma V, Provasi J, Brière-Dollat C, et al. Newborns modulate their crawling in response to their native language but not another language. Dev Sci. (2022) 26:e13248. doi: 10.1111/desc.13248
60. Dominici N, Ivanenko YP, Cappellini G, d’Avella A, Mondì V, Cicchese M, et al. Locomotor primitives in newborn babies and their development. Science (1979). (2011) 334:997–9. doi: 10.1126/science.1210617
61. Hinnekens E, Barbu-roth M, Do M, Berret B, Teulier C. Generating variability from motor primitives during infant locomotor development. bioRxiv [Preprint] (2022). doi: 10.1101/2022.05.05.490063
62. André-Thomas AS. In: Spastics Society. Medical Education and Information Unit, editor. Locomotion from pre- to post-natal life: How the newborn begins to acquire psycho-sensory functions. 1st ed. London, Kent, England: William Heinemann Medical Books Ltd. (1966).
63. Zelazo PR, Zelazo NA, Kolb S. “Walking” in the newborn. Science (1979). (1972) 176:314–5. doi: 10.1126/science.176.4032.314
64. Angulo-Barroso RM, Wu J, Ulrich DA. Long-term effect of different treadmill interventions on gait development in new walkers with down syndrome. Gait Posture. (2008) 27:231–8. doi: 10.1016/j.gaitpost.2007.03.014
65. Ulrich DA, Ulrich BD, Angulo-Kinzler RM, Yun J. Treadmill training of infants with down syndrome: evidence-based developmental outcomes. Pediatrics. (2001) 108:1–7. doi: 10.1007/s00737-013-0357-8.A
66. Rademacher N, Black DP, Ulrich BD. Early spontaneous leg movements in infants born with and without myelomeningocele. Pediatr Phys Ther. (2008) 20:137–45. doi: 10.1097/PEP.0b013e3181720248
67. Wu J, Ulrich BD, Ulrich DA, Angulo-barroso RM. Exploring effects of different treadmill interventions on walking onset and gait patterns in infants with down syndrome. Dev Med Child Neurol. (2007) 49(11):839–45. doi: 10.1111/j.1469-8749.2007.00839.x
68. Wu J, Ulrich DA, Looper J, Angulo-barroso CWTRM. Strategy adoption and locomotor adjustment in obstacle clearance of newly walking toddlers with down syndrome after different treadmill interventions. Exp Brain Res. (2008) 186(2):261–72. doi: 10.1007/s00221-007-1230-7
69. Dietz V. Do human bipeds use quadrupedal coordination? Trends Neurosci. (2002) 25:462–7. doi: 10.1016/S0166-2236(02)02229-4
70. Sylos-Labini F, Ivanenko YP, MacLellan MJ, Cappellini G, Poppele RE, Lacquaniti F. Locomotor-like leg movements evoked by rhythmic arm movements in humans. PLoS One. (2014) 9:e90775. doi: 10.1371/journal.pone.0090775
71. Forma V, Anderson DI, Huet V, Granjon L, Barbu-roth M. What does prone skateboarding in the newborn tell US about the ontogeny of human locomotion ? Child Dev. (2019) 90:1286–302. doi: 10.1111/cdev.13251
72. McGraw MB. Development of neuro-muscular mechanisms as reflected in the crawling and creeping behavior of the human infant. Pedagog Semin J Genet Psychol. (1941) 58:83–111. doi: 10.1080/08856559.1941.10534556
73. Kolobe THA, Fagg AH. Robot reinforcement and error-based movement learning in infants with and without cerebral palsy. Phys Ther. (2019) 99:677–88. doi: 10.1093/ptj/pzz043
74. Chandrashekhar R, Wang H, Rippetoe J, James SA, Fagg AH, Kolobe THA. The impact of cognition on motor learning and skill acquisition using a robot intervention in infants with cerebral palsy. Front Robot AI. (2022) 9:805258. doi: 10.3389/frobt.2022.805258
75. Wentz EE. Importance of initiating a “tummy time” intervention early in infants with down syndrome. Pediatr Phys Ther. (2017) 29:68–75. doi: 10.1097/PEP.0000000000000335
76. Larroque B, Ancel P-Y, Marret S, Marchand L, André M, Arnaud C, et al. Neurodevelopmental disabilities and special care of 5-year-old children born before 33 weeks of gestation (the EPIPAGE study): a longitudinal cohort study. Lancet. (2008) 371:813–20. doi: 10.1016/S0140-6736(08)60380-3
77. Amiel-Tison C, Grenier A. Expression of liberated motor activity (LMA) following manual immobilization of the head. In: Amiel-Tison C, Grenier A, editors. Neurological evaluation of the newborn and the infant. New York, NY: Masson Publishing USA (1983). p. 87–109.
78. Gosselin J, Amiel-Tison C. Neurological assessment from birth to 6 (second edition). Montreal: CHU Sainte-Justine Eds (2007).
79. Bayley N. Bayley scales of infant and toddler development—third edition: Technical manual. San Antonio: Harcout (2006).
80. Paro-Panjan D, Neubauer D, Kodric J, Bratanic B. Amiel-Tison neurological assessment at term age: clinical application, correlation with other methods, and outcome at 12 to 15 months. Dev Med Child Neurol. (2005) 47:805258. doi: 10.1017/S0012162205000046
81. Prechtl HFR. The behavioural states of the newborn infant (a review). Brain Res. (1974) 76:185–212. doi: 10.1016/0006-8993(74)90454-5
82. Ulrich DA, Lloyd MC, Tiernan CW, Looper JE, Angulo-Barroso RM. Effects of intensity of treadmill training on developmental outcomes and stepping in infants with down syndrome: a randomized trial. Phys Ther. (2008) 88:114–22. doi: 10.2522/ptj.20070139
83. Zelazo NA, Zelazo PR, Cohen KM, Zelazo PD. Specificity of practice effects on elementary neuromotor patterns. Dev Psychol. (1993) 29:686–91. doi: 10.1037/0012-1649.29.4.686
Keywords: early intervention, cerebral palsy, crawliskate, locomotion, neonate, newborn
Citation: Dumuids-Vernet M-V, Forma V, Provasi J, Anderson DI, Hinnekens E, Soyez E, Strassel M, Guéret L, Hym C, Huet V, Granjon L, Calamy L, Dassieu G, Boujenah L, Dollat C, Biran V and Barbu-Roth M (2023) Stimulating the motor development of very premature infants: effects of early crawling training on a mini-skateboard. Front. Pediatr. 11:1198016. doi: 10.3389/fped.2023.1198016
Received: 31 March 2023; Accepted: 18 May 2023;
Published: 6 June 2023.
Edited by:
Gianluca Lista, Ospedale dei Bambini Vittore Buzzi, ItalyReviewed by:
Thalia Harmony, National Autonomous University of Mexico, MexicoDarja Paro-Panjan, University Children's Hospital Ljubljana, Slovenia
© 2023 Dumuids-Vernet, Forma, Provasi, Anderson, Hinnekens, Soyez, Strassel, Guéret, Hym, Huet, Granjon, Calamy, Dassieu, Boujenah, Dollat, Biran and Barbu-Roth. This is an open-access article distributed under the terms of the Creative Commons Attribution License (CC BY). The use, distribution or reproduction in other forums is permitted, provided the original author(s) and the copyright owner(s) are credited and that the original publication in this journal is cited, in accordance with accepted academic practice. No use, distribution or reproduction is permitted which does not comply with these terms.
*Correspondence: Marianne Barbu-Roth YmFyYnVyb3RoQGdtYWlsLmNvbQ==