- 1Department of Paediatric Medicine, Alder Hey Children’s NHS Foundation Trust, Liverpool, United Kingdom
- 2Department of Paediatric Rheumatology, Alder Hey Children’s NHS Foundation Trust, Liverpool, United Kingdom
- 3Department of Biostatistics, Institute of Infection, Veterinary and Ecological Sciences, University of Liverpool, Liverpool, United Kingdom
- 4Department of Paediatric Infectious Diseases and Immunology, Alder Hey Children’s NHS Foundation Trust, Liverpool, United Kingdom
- 5Department of Women’s and Children’s Health, Institute of Life Course and Medical Sciences, University of Liverpool, Liverpool, United Kingdom
Background: Paediatric symptomatic SARS-CoV-2 infections associate with two presentations, acute COVID-19 and paediatric inflammatory multisystem syndrome temporally associated with SARS-CoV-2 (PIMS-TS). Phenotypic comparisons, and reports on predictive markers for disease courses are sparse and preliminary.
Methods: A chart review of COVID-19 and PIMS-TS patients (≤19 years) admitted to Alder Hey Children's NHS Foundation Trust, a tertiary centre in the North-West of England, was performed (02/2020–09/2022).
Results: A total of 161 symptomatic COVID-19 and 50 PIMS-TS patients were included. Peaks in admissions of patients with PIMS-TS occurred approximately 4 weeks after those for acute COVID-19. The incidence of in-patients with PIMS-TS reduced over time, and there were no admissions after February 2022. When compared to acute COVID-19, PIMS-TS patients were older (median: 10.3 years vs. 2.03 years; p < 0.001). There were no differences in gender distribution, but minority ethnicities were over-represented among PIMS-TS patients. Regional ethnic distribution was reflected among acute COVID-19 patients (66% vs. 84.5% White Caucasian, p = 0.01). Pre-existing comorbidities were more common among acute COVID-19 patients (54.7% vs. 8%, p < 0.001). PIMS-TS patients more commonly presented with abdominal symptoms (92% vs. 50.3%), neurological symptoms (28% vs. 10.6%) and skin rashes (72% vs. 16.8%), (p ≤ 0.01) when compared with acute COVID-19, where respiratory symptoms were more common (51.6% vs. 32%, p = 0.016). PIMS-TS more frequently required intensive care admission (64% vs. 16.8%), and inotropic support (64% vs. 9.3%) (all p < 0.05). More deaths occurred among acute COVID-19 patients [0 vs. 7 (4.4%)], with 5/7 (71%) in the context of pre-existing comorbidities. When compared to acute COVID-19, PIMS-TS patients exhibited more lymphopenia and thrombocytopenia, a more pronounced acute phase reaction, and more hyponatraemia (p < 0.05). Partial least square discriminant analysis of routine laboratory parameters allowed (incomplete) separation of patients at diagnosis, and variable importance projection (VIP) scoring revealed elevated CRP and low platelets as the most discriminatory parameters.
Conclusion: Admissions for PIMS-TS reduced with increasing seroconversion rates in the region. Young age and pre-existing comorbidities associate with hospital admission for acute COVID-19. While PIMS-TS may present more acutely with increased need for intensive care, acute COVID-19 had an increased risk of mortality in this cohort.
1. Introduction
Severe acute respiratory syndrome coronavirus 2 (SARS-CoV-2) is the pathogen responsible for the COVID-19 pandemic (1). Since December 2019, COVID-19 presented in waves that associated with various SARS-CoV-2 variants of concern (VoC) which emerged over time (1, 2). New variants were the result of hypermutation, predominantly affecting the spike region of the virus' RNA genome (1, 3). Because the spike protein is responsible for the infection of host cells, virus variants exhibit variable pathogenicity (3).
Notably, SARS-CoV-2 infections can manifest in a variety of clinical pictures. While most patients, across age groups, are asymptomatic or exhibit mild upper respiratory symptoms, others become severely unwell, particularly those with underlying comorbidities and/or the elderly (4–6). Severe COVID-19 can result in organ failure and death (7, 8). Mortality rates in the United Kingdom (UK) between 2020 and 2021 were 113.8 per 100,000 (9). Mortality was 62 times higher among patients aged >65 years (6).
When compared to adults, children and young people (CYP) infected with SARS-CoV-2 exhibit reduced disease severity and lower mortality, with infections typically being mild or asymptomatic (10). However, a subset of children develop severe acute COVID-19 requiring hospitalisation (11–13). Mortality associated with acute COVID-19 is low in children when compared to adults (estimated 1.83/million CYP in England) (14). Similar to adults, CYP presenting with severe COVID-19 are more likely to have comorbidities (11, 13, 15–17). Pre-existing conditions associated with severe COVID-19 in CYP include chronic lung disease, airway anomalies, neurodevelopmental disorders, cardiovascular disease, prematurity, diabetes mellitus, obesity (13, 15), immunosuppression, and sickle cell disease (16). However, published reports on underlying risk factors for severe acute COVID-19 and hospitalisation in children were descriptive, limited in numbers, and sometimes contradictory.
A subset of CYP present with a post-infectious hyperinflammatory syndrome termed Paediatric Multisystem Inflammatory Syndrome Temporally Associated with SARS-CoV-2 (PIMS-TS) or multisystem inflammatory syndrome in children (MIS-C) (18, 19). PIMS-TS typically presents between two and six weeks after the exposure to SARS-CoV-2 (20). It is characterised by persistent fever, elevated laboratory markers of inflammation, and evidence of single or multi-organ dysfunction (21, 22). PIMS-TS represents a disease spectrum, and mild cases may be missed. The full clinical picture of PIMS-TS frequently includes gastrointestinal and cardiovascular involvement (23–25). In a study in the Czech Republic reporting cases between November 2020 and March 2022, the incidence of PIMS-TS was estimated as 53/100,000 SARS-CoV-2 positive children (26).
This study aimed to compare incidences (over time), clinical and laboratory features of PIMS-TS and acute COVID-19 in CYP admitted to a tertiary paediatric hospital in the North-West of England.
2. Methods
2.1. Study design and participants
A retrospective chart review was undertaken in CYP (≤19 years) admitted to Alder Hey Children's NHS Foundation Trust, Liverpool, for acute COVID-19 or PIMS-TS between 10/02/2020 to 31/08/2022. The study was approved as a service evaluation by the local audit committee.
2.2. Case definitions and ascertainment
Several different diagnostic criteria exist to define PIMS-TS/MIS-C. For this study, the case definition of the Royal College of Paediatrics and Child Health (RCPCH) was used (19). Patients were classified by the centre's PIMS-TS multidisciplinary team (including infectious disease, rheumatology, general paediatric and cardiology specialists).
Acute COVID-19 cases were defined as CYP with an acute hospital admission who tested positive for SARS-CoV-2 by RT-PCR (real time polymerase chain reaction) AND the admission was due to COVID-19. Hospital acquired acute COVID-19 cases were also included if patients tested positive for SARS-CoV-2 AND developed symptoms suggestive of acute COVID-19 during their admission. All in-patients testing positive for SARS-CoV-2 (RT-PCR) between 10/02/2020 to 31/08/2022 were identified from the microbiology database. Cases were screened and case definitions for symptomatic acute COVID-19 were applied by PJ and MM to exclude patients with incidentally positive SARS-CoV-2 RT-PCR (e.g., elective admissions, surgical procedures, admissions for mental health issues). Unclear cases were reviewed by CH, CP, WW.
Electronic medical records of inpatients meeting the definition of PIMS-TS or acute COVID-19 were accessed to record demographic (age, sex, and ethnicity) and clinical information, including date of admission, comorbidities, clinical symptoms and signs, laboratory parameters within 24 h of admission and peak abnormal values (including white blood cells [WBC], neutrophils, lymphocytes, C-reactive protein [CRP], ferritin, triglycerides, platelets, INR, fibrinogen, D-dimer, haemoglobin [Hb], sodium, urea, creatinine, bilirubin, alanine transaminase [ALT], aspartate aminotransferase [AST], and treatment. Disease severity as estimated by recording duration of admission [days], admission to critical care, level of medical intervention required (e.g., fluid resuscitation, inotropic support, non-invasive or invasive ventilatory support), and mortality was recorded.
2.3. Statistical analysis
Statistical analyses were performed in Rstudio 2022.07.2 (27), utilising R version 4.2.2 and the “tidyverse” package (28). Quantitative variables were reported using median and interquartile range (IQR), as most variables followed a non-parametric distribution. Continuous variables were tested for normal distribution using Shapiro-Wilk test (p > 0.05); t-tests were used for comparisons between groups if following normal distribution; Mann–Whitney tests were used for variables not following normal distribution. Categorical variables were compared between groups using Chi-Square tests or Fisher's exact test. Statistical significance was determined as a p-value below or equal to 0.05 (p ≤ 0.05). Bonferroni correction was utilised when comparing clinical characteristics to adjust for multiple comparisons. Patients with missing laboratory data were excluded in the univariate analysis. Laboratory parameters with ≥95% missing data in either group were not compared in univariate or multivariate analysis. A partial least squares-discriminant analysis (PLS-DA) model and a variable importance plot of laboratory tests, were conducted on Rstudio using the “mixOmics” (29) and “tidyverse” package (28). For analysis of admission blood tests, laboratory tests with the fewest missing datapoints (≤16% for either group) were selected, and missing values were imputed by using the mean of the variable for each group. For analysis of peak blood tests, no imputation was done due to a greater percentage of missing datapoints, and any patients with missing values were omitted.
We chose the PLS-DA model, a supervised multivariate statistical method, as a dimensionality reduction tool to produce latent variables, which are a linear combination of the laboratory parameters. 95% confidence ellipses were used to visually estimate whether groups can be separated based on the laboratory parameters. A variable importance plot (VIP) of laboratory parameters was used to display which laboratory variables most contributed to the PLS-DA model. The variable importance plot produces VIP scores for each independent variable in the PLS-DA model, and thus the importance of each variable in contributing to the separation in the two groups of the PLS-DA model. This approach was used to identify which laboratory variables best predict whether patients would belong to the COVID-19 or PIMS-TS group.
3. Results
3.1. Demographics and epidemiology
During the study period a total of 50 patients met the case definition for PIMS-TS. Over the same interval, 848 CYP (≤19 years) tested positive for SARS-CoV-2 by RT-PCR. Of these, 687 CYP (81%) were asymptomatic and/or incidental and were excluded from the analysis. This left 161 patients who were admitted to hospital due to acute COVID-19 or developed symptomatic disease during hospital admission. Two CYP had two separate acute COVID-19 infections and were included in the analysis twice.
PIMS-TS patients were significantly older (median: 10.3 [IQR: 5.65] vs. 2.03 [IQR: 10.6] years; p < 0.001) (Table 1). No differences were recorded in gender distribution. Notably, while in the PIMS-TS cohort ethnic minorities were over-represented, the acute COVID-19 cohort reflected the regional ethnic distribution (63.6% vs. 83.8% White Caucasians, p < 0.01), with the White Caucasian ethnic group representing 84% of the regional population (National Census, 2021) (30).
Pre-existing comorbidities were more common among acute COVID-19 patients (42.2% vs. 4%, p < 0.001), and 28% had more than one comorbidity. Most common pre-existing diseases included cardiovascular disease (15.5%), then prematurity in infant (<1 year old), respiratory and secondary immunodeficiency (all 9.9%) (Table 1, Supplementary Table 1). The two PIMS-TS patients with comorbidities had asthma.
As previously reported, hospital admissions for PIMS-TS lagged approximately 4 weeks behind those for acute COVID-19 (Figure 1) (20, 33). No cases of PIMS-TS were recorded from February 2022, which coincided with the predominance of the SARS-CoV-2 Omicron variant (31).
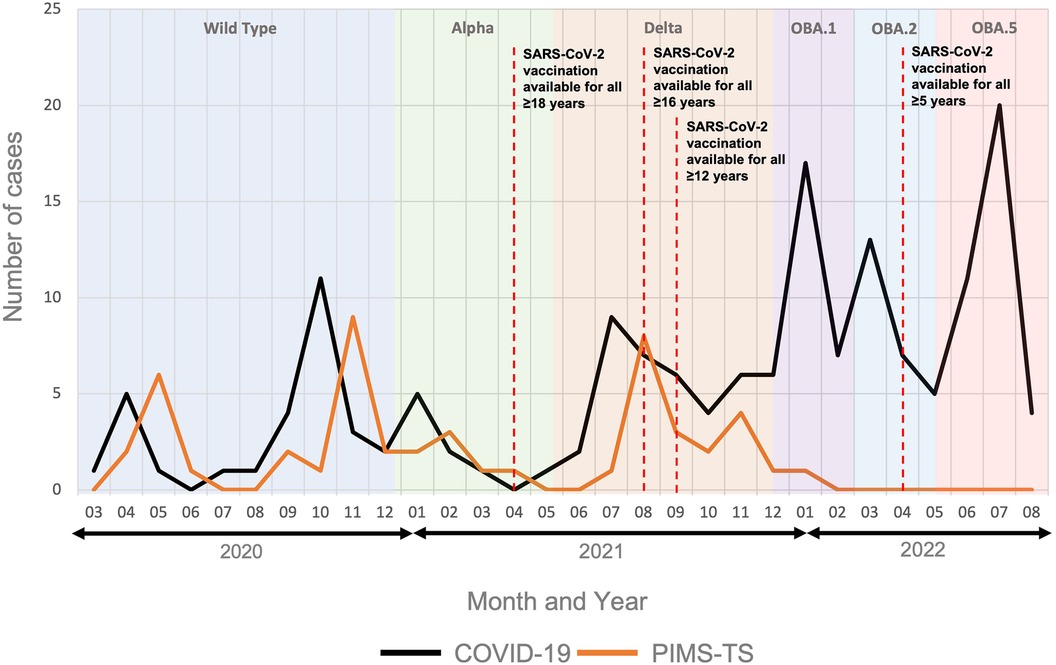
Figure 1. Hospital admissions for PIMS-TS and acute COVID-19. The figure includes data from 50 patients admitted for PIMS-TS and 161 acute COVID-19 patients. Predominant SARS-CoV-2 variants in the region are indicated over time. OBA.1: Omicron variant BA1; OBA.2: Omicron variant BA2; OBA.5: Omicron variant BA5 (31). Timelines of the SARS-CoV-2 vaccination roll out are indicated (32).
3.2. Clinical presentation and disease severity
As per case definition, all PIMS-TS patients exhibited fevers during admission which compared to 72% of acute COVID-19 patients (p < 0.001) (Table 2). PIMS-TS patients more frequently presented with gastrointestinal symptoms (all p < 0.001), neurological symptoms (28% vs. 10.6%, p = 0.0023) and/or headaches (52 vs. 12.4%, p < 0.001). Furthermore, PIMS-TS patients more commonly exhibited symptoms also associated with “historic” Kawasaki disease. Patients admitted with acute COVID-19 more frequently exhibited upper respiratory symptoms (51.6% vs. 32%, p = 0.015). A total of 27 (16.8%) acute COVID-19 patients were admitted with feeding concerns, while no PIMS-TS patients were (p = 0.001).
To assess disease severity, admission to critical care, cardiorespiratory supportive therapy and duration of hospital admissions were compared (Table 3). Patients with PIMS-TS more frequently required oxygen supplementation and inotropic support (64% vs. 9.3%, p < 0.001) but no significant differences were observed in escalating respiratory support via non-invasive (NIV) or invasive ventilation. A higher proportion of critical care admissions occurred in the PIMS-TS when compared to the acute COVID-19 cohort (64% vs. 16.8%, p < 0.001). No PIMS-TS patients required extracorporeal membrane oxygenation (ECMO) whereas 3 (1.9%) patients with acute COVID-19 did (p = 0.3). PIMS-TS patients had a longer median hospitalisation (8 vs. 3 days, p < 0.001). Seven patients admitted with acute COVID-19 died, while all PIMS-TS patients in this cohort recovered (Box 1).
3.3. Laboratory phenotype and predictive markers
PIMS-TS patients exhibited higher systemic markers of inflammation when compared to acute COVID-19 patients, including leucocytosis (p = 0.01), neutrophilia and elevated CRP (p < 0.001) (Table 4). While ferritin (p = 0.01), fibrinogen (p < 0.001) and D-dimers (p = 0.028) were higher in PIMS-TS patients, they were infrequently tested in acute COVID-19. PIMS-TS patients exhibited increased overall WBC but more frequent lymphocytopenia (p < 0.001) and thrombocytopenia (p < 0.001). Patients with PIMS-TS more frequently exhibited hyponatraemia (p < 0.001).
To investigate whether laboratory tests at admission differentiate PIMS-TS from acute COVID-19 patients, partial least-squares discriminant analysis (PLS-DA) was performed, including the following parameters: haemoglobin, platelets, white blood cells, neutrophils, lymphocyte counts, CRP, sodium, urea, and creatinine (Figure 2). While PLS-DA did not completely separate PIMS-TS from acute COVID-19 patients, variable importance projection (VIP) analysis showed that CRP elevation and reduced platelet numbers were more pronounced in PIMS-TS as compared to acute COVID-19 patients.
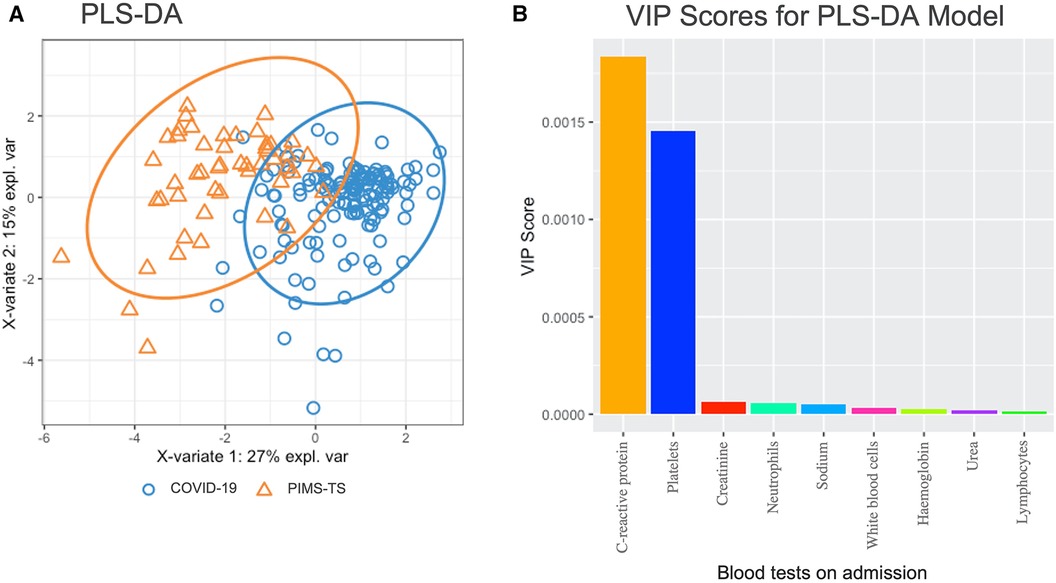
Figure 2. Partial least squares-discriminant analysis (PLS-DA) in PIMS-TS (n = 50) and acute COVID-19 (n = 161) patients at admission. Blood tests included were haemoglobin, platelets, white blood cells, neutrophils, lymphocytes, C-reactive protein, sodium, urea, creatinine. (A) PLS-DA analysis showing individual samples with the confidence ellipses of PIMS-TS and COVID-19. (B) VIP analysis showing variable importance plots of blood tests included in the PLS-DA model.
Considering peak changes of laboratory parameters, overall, PIMS-TS patients exhibited a more pronounced acute phase reaction when compared to COVID-19 infection (elevated WBC, neutrophils, CRP, fibrinogen) (Supplementary Table 2). However, features overlapped more than at admission and did not allow for clear separation between groups (Supplementary Figure 1). While overlapping significantly between groups, VIP analysis suggested elevated CRP and thrombopenia to be associated with PIMS-TS more than active COVID-19.
3.4. Treatment of PIMS-TS and COVID-19
Various drug therapies were used to treat PIMS-TS and acute COVID-19 (Table 5). The most common treatments in PIMS-TS included intravenous immunoglobulins (IVIG) (82%, 41/50) and methylprednisolone (76%, 38/50) Dexamethasone was prescribed in 14.9% (23/161) of acute COVID-19 patients. Furthermore, 16% (8/50) of PIMS-TS patients received biologic disease modifying anti-inflammatory therapy, which compared to 3.7% of acute COVID-19 patients. Treatments included the recombinant interleukin receptor antagonist anakinra (8%) and the IL-6 receptor antagonist tocilizumab (8%).
Remdesivir, a viral RNA polymerase inhibitor, was used in 10.6% of acute COVID-19 patients. Four patients (2.5%) received the recombinant anti-SARS-CoV-2 monoclonal antibody Sotrovimab and 1 patient (0.6%) received Ronapreve, a combination of anti-SARS-CoV-2 recombinant antibodies casirivimab and imdevimab. Almost all PIMS-TS patients (94%) received intravenous antimicrobial therapy, compared to 58.4% of acute COVID-19 patients (p < 0.001).
4. Discussion
This single centre retrospective study from a large tertiary children's hospital in the North-West of England, which provides healthcare to over 330,000 children and young people each year (34), reports varying proportions of COVID-19 and PIMS-TS patients over time. While PIMS-TS was the dominant cause of SAR-CoV-2 associated hospital admissions early in the pandemic, cases of admissions due to acute COVID-19 rose sharply from January 2022 with the emergence of the Omicron variant in the region. This coincided with a decline in hospitalisations for PIMS-TS. The incidence of admissions for SARS-CoV-2 associated disease at Alder Hey Hospital follows the pattern seen nationally with corresponding spikes in infection and admission at similar times (35). Several groups previously suggested decreasing numbers of PIMS-TS cases with the Delta (20, 36–38) and especially the Omicron variant (20, 36–40), despite a significant rise in the number of hospitalised acute COVID-19 cases. Notably, in contrast to some of these reports, we observed an increase in both acute COVID-19 and PIMS-TS cases with the Delta variant (41).
Two main theories exist on why PIMS-TS numbers declined with the Delta and/or the Omicron variant:
• One hypothesis suggests that increasing SARS-CoV-2 seroprevalence, through infection or vaccination, may have reduced the PIMS-TS risk (39, 42, 43). However, Sorg et al. suggested that vaccination did not significantly alter the PIMS-TS risk in Germany because all age groups, including those not eligible for vaccination, had falling rates of PIMS-TS (37). In the here presented study, it was difficult to capture whether children had been vaccinated due to a lack of documentation in medical records. However, a significant proportion of the study population were likely not vaccinated because, in the UK, vaccination programmes for 12- to 15-year-olds only began in September 2021, and for 5- to 11-year-olds in April 2022. Furthermore, vaccination rates in the North-West were the lowest in England (44). Notably, in the here reported cohort and others (23, 45, 46), children admitted for PIMS-TS were older when compared to those admitted for COVID-19 (median: 10.3 vs. 2.03 years). This may suggest increased risk for PIMS-TS in individuals that first encounter SARS-CoV-2 in their school-age years as compared to younger children. Vaccination or infection early in life, which results in the acquisition of immune memory and an orchestrated innate and adaptive immune response against SARS-CoV-2, however, may prevent the development of PIMS-TS upon re-exposure later in life. Increasing and then constantly high case numbers among children in the region resulted in an estimated 93% of children having experienced SARS-CoV-2 infection by September 2022 (unpublished, UK Health Security Agency, UKHSA). This may explain the absence of admissions for PIMS-TS in the region after February 2022. Because vaccination may offer even better protection from PIMS-TS (including strong T cell responses) (47, 48), the observed disappearance of PIMS-TS over time may demonstrate the success of vaccination programmes, preventing hospitalisation and complications. Vaccination not only reduced COVID-19 severity (49), but may also have conferred protection against PIMS-TS (50–52). Therefore, a cornerstone of future public health planning in the face of disease outbreaks should focus on ensuring vaccine uptake remains high.
• A second theory claims that mutations in the virus' spike protein may affect infectivity, virulence, and disease outcomes (3, 53, 54). Reducing rates of PIMS-TS during the pre-vaccine Delta period in some studies suggests that changes to the SARS-CoV-2 virus may have been responsible. However, observations from the here presented study, namely increasing numbers of both COVID-19 and PIMS-TS during the “Delta variant wave”, argue for increasing seroconversion through infection or vaccination having contributed to reduced numbers of PIMS-TS patient cases. Initially, during the first waves with the wild-type and alpha variants, children may have been less affected because of reduced travel activity, shielding, school closures in the regions and reduced infectivity and virulence within the age group (55–57). The proportion of PIMS-TS (as compared to COVID-19) were relatively high throughout the “Wild-type”, “Alpha” and “Delta waves”. Across the UK, approximately 40% of 5- to 11-year-old and 82% of 12- to 18-year-old children and young people had detectable SARS-CoV-2 antibodies by December 2021, the end of the “Delta wave” (58). In adults, at a similar time point, >95% tested positive for SARS-CoV-2 antibodies (59). Of the 82.4% of 12- to 18-year-olds who tested positive for SARS-CoV-2 antibodies, less than half (43.1%) had received at least one COVID-19 vaccine dose (58).
Findings from this study support the first hypothesis, however it is likely, in our view that both theories have a part to play. At later stages, increased infectivity and virulence may have contributed to both increasing numbers of hospital admissions for acute COVID-19 and reduced proportions and absolute numbers of PIMS-TS patients.
This study confirms previous reports on PIMS-TS disproportionately affecting minority ethnic groups (23, 45, 60, 61). Notably, this contrasted with acute COVID-19 which reflected the regional composition of ethnic groups (62). While the exact reason for the predominance of ethnic minorities in PIMS-TS remain unknown, it suggests the involvement of genetic factors.
As mentioned above, children admitted for PIMS-TS were older children when compared to acute COVID-19, which is in line with previous reports (45, 46). However, young age also represents a possible risk factor for severe COVID-19 in children. Indeed, 39.8% (64/161) of patients admitted for COVID-19 in this study were younger than 1 year. This study also highlighted that children <1 year of age are at particular risk of becoming hospitalised with severe acute COVID-19. Notably, children >6 months have only recently become eligible for vaccination, but only if they are of an at-risk group (63). Given the risk profile, universal access to SARS-CoV-2 vaccines for infants, possibly through its incorporation into the childhood vaccination schedule, may prevent hospitalisation and even deaths in future wave outbreaks. Currently, the COVID-19 vaccine is not licenced for neonates, leaving this group highly vulnerable to severe acute infection. Thus, pregnant women should not only be encouraged to receive vaccines due to the increased risk of developing severe disease themselves, but also to allow effective maternal antibody transfer that may potentially protect infants in the crucial first 6 months of life (64, 65). As an alternative, in young children and infants who have not received vaccination, the use of neutralising monoclonal antibody products may incur survival benefit during severe acute COVID-19 disease (66), but more robust safety and efficacy data is required for its routine use.
Another factor associated with admission for COVID-19 is the presence of pre-existing comorbidities. While, in this study and others, only a few PIMS-TS patients (here 4%) had reported comorbidities (17, 23, 67, 68), 42.2% of COVID-19 patients had underlying disease (15, 69). Admissions for acute COVID-19 associate with a history of premature birth (13, 45), young age (<12 months) at infection (45, 61, 69, 70), type 1 diabetes (70), neurologic disorders (13, 45, 69, 70), cardiac disease (13, 45, 69, 70), respiratory disease (13, 45, 69), gastro-intestinal disease (45), malignancy (69), immunosuppression (16), chromosomal disorders including trisomy 21 (69), chronic kidney disease (69) and obesity (61). This suggests that the presence of comorbidities should be another key factor informing decisions on who to target in vaccination programmes and with therapies for non-hospitalised acute COVID-19 infections.
Patients admitted to hospital with PIMS-TS exhibited acute and severe disease but responded to anti-inflammatory and supportive treatment, which was in agreement with previous reports from us and others (45, 46, 61). When compared to COVID-19 patients, PIMS-TS patients had a greater length of admission (46), and an increased likelihood of critical care admission (45, 46). As with other studies, PIMS-TS associated with more gastrointestinal and neurological manifestations as well as mucocutaneous features (23, 45, 46, 60, 61, 71). Patients admitted for COVID-19 were, overall, less acutely unwell at admission. Nonetheless, 7/161 (4.3%) COVID-19 patients did not survive. In line with previous reports, and similar to the adult patient population, mortality in acute COVID-19 was associated with co-morbidities (15, 72). These observations are of relevance when, e.g., considering pre-hospitalisation treatment in high-risk patients (below).
At admission, the differentiation between acute COVID-19 and PIMS-TS can be challenging. Notably, while usually acute infection with SARS-CoV-2 precedes the onset of PIMS-TS by several weeks, some patients with PIMS-TS can remain PCR positive. This may be associated with persistent primary infection (73), or be the result of re-infection. In line with its hyper-inflammatory nature and previously reported features of “cytokine storm” (25, 74), PIMS-TS patients displayed markedly elevated markers of inflammation (white cell counts, neutrophils, C-reactive protein) (23, 45, 46, 60, 61, 71, 75). When compared to COVID-19, PIMS-TS associated with prominent lymphopenia and thrombocytopenia (23, 45, 46, 60, 61, 71, 75). However, severe COVID-19 may also present features of cytokine storm, such as pro-inflammatory cytokines and acute phase reactants, lymphopenia, and coagulopathy (76). Applying PLS-DA and VIP scoring, we identified CRP elevation and reduced platelet numbers as laboratory parameters with the highest contribution to differentiating PIMS-TS from acute COVID-19. Hyponatraemia, which was more common among PIMS-TS patients (46), may, as in “historic” Kawasaki disease, be explained by inappropriate antidiuretic hormone release (77). While these phenotype-associated features may aid in discriminating PIMS-TS from COVID-19, they are overlapping and require validation in larger independent patient cohorts, including further differential diagnoses such as other viral infections and bacterial sepsis (23, 45, 46, 60, 61, 71, 75).
In CYP, the evidence for treatment choices and associated risk is less robust when compared to the adult COVID-19 patient cohort (78, 79). At Alder Hey, treatment decisions were made by a multi-disciplinary team (including members from the infectious disease, rheumatology, general paediatrics, cardiology, and respiratory departments), which aided with balancing the relative effectiveness and safety of therapeutic options with changing virus variants over time. Treatment of acute COVID-19 with glucocorticosteroids was recommended from September 2020 in the UK, and was the mainstay of treatment for children requiring oxygen therapy (78). Remdesivir was licensed in July 2020, tocilizumab in December 2021 (78). Thus, these agents were only used in a minority of COVID-19 patients included here. For pre-hospitalisation treatments to prevent severe COVID-19, Ronapreve (August 2021) and Sotrovimab (December 2021) were available for CYP aged 12 years old and older weighing at least 40 kg (78). Among PIMS-TS patients in this cohort, 60% received IVIG and intravenous glucocorticosteroids, and 4% received tocilizumab or anakinra, to which they had been randomised through the RECOVERY trial (80).
While adding to the understanding of phenotypical, laboratory and prognostic differences between PIMS-TS and COVID-19, this study has limitations. In this single-centre retrospective study, virus variant data was not available for the majority of patients and had to be estimated based on regional surveillance data over time. Medical records did not always contain ethnicity data, SARS-CoV-2 vaccination status or body mass index. Thus, we were unable to determine whether for e.g., obesity was a risk factor. Laboratory tests were more frequently performed in PIMS-TS patients when compared to acute COVID-19 patients, and patients with milder acute COVID-19 were less likely to undergo laboratory testing. Only complete cases were compared in univariate analysis of laboratory tests, and multi-variate analysis of peak laboratory tests, possibly allowing bias.
5. Conclusions
Both alterations to the SARS-CoV-2 spike protein and increasing seroconversion likely contributed to decreasing admissions for PIMS-TS over time. Especially in times with low PIMS-TS case numbers, its diagnosis and differentiation from acute COVID-19 can be challenging. Clinical features, including mucocutaneous and enteric symptoms, thrombocytopenia, and a significantly elevated CRP associate with PIMS-TS, which may aid diagnosis, and rapid commencement of effective therapy. Patients with PIMS-TS can be acutely ill but usually respond to anti-inflammatory and supportive measures. While a smaller proportion of paediatric patients with acute COVID-19 require intensive care admission, 4.3% did not survive. Severe disease and mortality in COVID-19 are associated with young age (infancy) and pre-existing health conditions, including cardiovascular, mitochondrial, and neurological disease. Thus, vaccine programmes should include young age groups and especially target children with pre-existing diseases.
Data availability statement
The original contributions presented in the study are included in the article/Supplementary Material, further inquiries can be directed to the corresponding author.
Ethics statement
Ethical approval was not required for the study involving humans in accordance with the local legislation and institutional requirements. Written informed consent to participate in this study was not required from the participants or the participants’ legal guardians/next of kin in accordance with the national legislation and the institutional requirements.
Author contributions
PJ and MM collected data. WW, CP and CH provided guidance on unclear cases of inclusion and exclusion. PJ, CP and CH wrote the manuscript. SL provided advice on statistics. All authors contributed to the article and approved the submitted version.
Acknowledgments
We thank the microbiology and infectious diseases department of Alder Hey Children's NHS Foundation Trust for their support with compiling the list of SARS-CoV-2 RT-PCR positive patients. We thank all members of the PIMS-TS and acute-COVID multidisciplinary team. We would like to acknowledge the multitude of professionals involved in delivering the care of all patients included. This work was further supported by the UK's Experimental Arthritis Treatment Centre for Children (supported by Versus Arthritis, Alder Hey Children's NHS Foundation Trust, the Alder Hey Charity, and the University of Liverpool).
Conflict of interest
The authors declare that the research was conducted in the absence of any commercial or financial relationships that could be construed as a potential conflict of interest.
Publisher's note
All claims expressed in this article are solely those of the authors and do not necessarily represent those of their affiliated organizations, or those of the publisher, the editors and the reviewers. Any product that may be evaluated in this article, or claim that may be made by its manufacturer, is not guaranteed or endorsed by the publisher.
Supplementary material
The Supplementary Material for this article can be found online at: https://www.frontiersin.org/articles/10.3389/fped.2023.1219654/full#supplementary-material
References
1. Hu B, Guo H, Zhou P, Shi Z-L. Characteristics of SARS-CoV-2 and COVID-19. Nat Rev Microbiol. (2021) 19(3):141–54. doi: 10.1038/s41579-020-00459-7
2. Office of National Statistics. Department for Health and Social Care U.K. Regional and sub-regional estimates of coronavirus (COVID-19) positivity over time, UK: 12 January 2023. (2023). Available at: https://www.ons.gov.uk/peoplepopulationandcommunity/healthandsocialcare/conditionsanddiseases/articles/regionalandsubregionalestimatesofcoronaviruscovid19positivityovertimeuk12january2023/2023-01-12#:∼:text=the%20first%2C%20from%20the%20week,the%20Alpha%20variant%20was%20dominant
3. Harvey WT, Carabelli AM, Jackson B, Gupta RK, Thomson EC, Harrison EM, et al. SARS-CoV-2 variants, spike mutations and immune escape. Nat Rev Microbiol. (2021) 19(7):409–24. doi: 10.1038/s41579-021-00573-0
4. Tagarro A, Cobos-Carrascosa E, Villaverde S, Sanz-Santaeufemia FJ, Grasa C, Soriano-Arandes A, et al. Clinical spectrum of COVID-19 and risk factors associated with severity in spanish children. Eur J Pediatr. (2022) 181(3):1105–15. doi: 10.1007/s00431-021-04306-6
5. Eythorsson E, Helgason D, Ingvarsson RF, Bjornsson HK, Olafsdottir LB, Bjarnadottir V, et al. Clinical spectrum of coronavirus disease 2019 in Iceland: population based cohort study. Br Med J. (2020) 371:m4529. doi: 10.1136/bmj.m4529
6. Yanez ND, Weiss NS, Romand J-A, Treggiari MM. COVID-19 mortality risk for older men and women. BMC Public Health. (2020) 20(1):1742. doi: 10.1186/s12889-020-09826-8
7. Rodriguez-Morales AJ, Cardona-Ospina JA, Gutiérrez-Ocampo E, Villamizar-Peña R, Holguin-Rivera Y, Escalera-Antezana JP, et al. Clinical, laboratory and imaging features of COVID-19: a systematic review and meta-analysis. Travel Med Infect Dis. (2020) 34:101623. doi: 10.1016/j.tmaid.2020.101623
8. Mehta OP, Bhandari P, Raut A, Kacimi SEO, Huy NT. Coronavirus disease (COVID-19): comprehensive review of clinical presentation. Front Public Health. (2021) 8:582932. doi: 10.3389/fpubh.2020.582932
10. Milani GP, Bottino I, Rocchi A, Marchisio P, Elli S, Agostoni C, et al. Frequency of children vs adults carrying severe acute respiratory syndrome coronavirus 2 asymptomatically. JAMA Pediatr. (2021) 175(2):193–4. doi: 10.1001/jamapediatrics.2020.3595
11. Preston LE, Chevinsky JR, Kompaniyets L, Lavery AM, Kimball A, Boehmer TK, et al. Characteristics and disease severity of US children and adolescents diagnosed with COVID-19. JAMA Network Open. (2021) 4(4):e215298-e. doi: 10.1001/jamanetworkopen.2021.5298
12. Bahl A, Mielke N, Johnson S, Desai A, Qu L. Severe COVID-19 outcomes in pediatrics: an observational cohort analysis comparing alpha, delta, and omicron variants. Lancet Reg Health Am. (2023) 18:100405. doi: 10.1016/j.lana.2022.100405
13. Woodruff RC, Campbell AP, Taylor CA, Chai SJ, Kawasaki B, Meek J, et al. Risk factors for severe COVID-19 in children. Pediatrics. (2022) 149(1):e2021053418. doi: 10.1542/peds.2021-053418
14. Smith C, Odd D, Harwood R, Ward J, Linney M, Clark M, et al. Deaths in children and young people in England after SARS-CoV-2 infection during the first pandemic year. Nat Med. (2022) 28(1):185–92. doi: 10.1038/s41591-021-01578-1
15. Gonzalez-Dambrauskas S, Vasquez-Hoyos P, Camporesi A, Cantillano EM, Dallefeld S, Dominguez-Rojas J, et al. Paediatric critical COVID-19 and mortality in a multinational prospective cohort. Lancet Reg Health Am. (2022) 12:100272. doi: 10.1016/j.lana.2022.100272
16. Campbell JI, Dubois MM, Savage TJ, Hood-Pishchany MI, Sharma TS, Petty CR, et al. Comorbidities associated with hospitalization and progression among adolescents with symptomatic coronavirus disease 2019. J Pediatr. (2022) 245:102–10.e2. doi: 10.1016/j.jpeds.2022.02.048
17. Ward JL, Harwood R, Smith C, Kenny S, Clark M, Davis PJ, et al. Risk factors for PICU admission and death among children and young people hospitalized with COVID-19 and PIMS-TS in England during the first pandemic year. Nat Med. (2022) 28(1):193–200. doi: 10.1038/s41591-021-01627-9
18. (CDC) CfDCaP. Multisystem Inflammatory Syndrome in Children (MIS-C) Associated with Coronavirus Disease 2019 (COVID-19). (2020) [23/03/2023]. Available at: https://emergency.cdc.gov/han/2020/han00432.asp
19. Royal College of Paediatrics and Child Health (RCPCH). Guidance: paediatric multisystem inflammatory syndrome temporally associated with COVID-19 [Internet]. (2020). Available from: https://www.rcpch.ac.uk/resources/paediatric-multisystem-inflammatory-syndrome-temporally-associated-covid-19-pims-guidance
20. Shingleton J, Burton L, Williams HE, Finnie TJR, Bennett E, Birrell P, et al. Risk of paediatric multisystem inflammatory syndrome (PIMS-TS) during the SARS-CoV-2 alpha and delta variant waves: national observational and modelling study, 2020–21, England. Front Pediatr. (2022) 10:1034280. doi: 10.3389/fped.2022.1034280
21. Singh-Grewal D, Lucas R, McCarthy K, Cheng AC, Wood N, Ostring G, et al. Update on the COVID-19-associated inflammatory syndrome in children and adolescents; paediatric inflammatory multisystem syndrome-temporally associated with SARS-CoV-2. J Paediatr Child Health. (2020) 56(8):1173–7. doi: 10.1111/jpc.15049
22. Sharma C, Ganigara M, Galeotti C, Burns J, Berganza FM, Hayes DA, et al. Multisystem inflammatory syndrome in children and kawasaki disease: a critical comparison. Nat Rev Rheumatol. (2021) 17(12):731–48. doi: 10.1038/s41584-021-00709-9
23. Feldstein LR, Tenforde MW, Friedman KG, Newhams M, Rose EB, Dapul H, et al. Characteristics and outcomes of US children and adolescents with multisystem inflammatory syndrome in children (MIS-C) compared with severe acute COVID-19. JAMA. (2021) 325(11):1074–87. doi: 10.1001/jama.2021.2091
24. Felsenstein S, Duong P, Lane S, Jones C, Pain CE, Hedrich CM. Cardiac pathology and outcomes vary between kawasaki disease and PIMS-TS. Clin Immunol. (2021) 229:108780. doi: 10.1016/j.clim.2021.108780
25. Felsenstein S, Willis E, Lythgoe H, McCann L, Cleary A, Mahmood K, et al. Presentation, treatment response and short-term outcomes in paediatric multisystem inflammatory syndrome temporally associated with SARS-CoV-2 (PIMS-TS). J Clin Med. (2020) 9(10):3293. doi: 10.3390/jcm9103293
26. David J, Hradsky O, Jabandziev P, Klaskova E, Jirincova H, Lebl J. Impact of SARS-CoV-2 variants on the incidence of paediatric inflammatory multisystem syndrome (PIMS-TS). J Paediatr Child Health. (2022) 58(10):1901–3. doi: 10.1111/jpc.16204
27. RStudio Team. RStudio: Integrated development for R [Internet]. Boston, MA, U.S.A. (2020). Available from: http://www.rstudio.com/
28. Wickham H, Averick M, Bryan J, Chang W, McGowan L, François R, et al. Welcome to the tidyverse. J Open Source Softw. (2019) 4:1686. doi: 10.21105/joss.01686
29. Rohart F, Gautier B, Singh A, Lê Cao K-A. Mixomics: an R package for ‘omics feature selection and multiple data integration. PLoS Comput Biol. (2017) 13(11):e1005752. doi: 10.1371/journal.pcbi.1005752
30. Office for National Statistics. How life has changed in Liverpool: Census 2021. (2023). Available at: https://www.ons.gov.uk/visualisations/censusareachanges/E08000012/
31. UK Health Security Agency. Cases in North West [20/03/2023]. Available at: https://coronavirus.data.gov.uk/details/cases?areaType=region&areaName=North%20West
32. U.K. Government DoHSC. The rollout of the COVID-19 vaccination programme in England. (2022). Available at: https://www.nao.org.uk/wp-content/uploads/2022/02/The-rollout-of-the-COVID-19-vaccination-programme-in-England.pdf
33. Flood J, Shingleton J, Bennett E, Walker B, Amin-Chowdhury Z, Oligbu G, et al. Paediatric multisystem inflammatory syndrome temporally associated with SARS-CoV-2 (PIMS-TS): prospective, national surveillance, United Kingdom and Ireland, 2020. Lancet Reg Health Eur. (2021) 3:100075. doi: 10.1016/j.lanepe.2021.100075
36. Lopez L, Burgner D, Glover C, Carr J, Clark J, Boast A, et al. Lower risk of multi-system inflammatory syndrome in children (MIS-C) with the omicron variant. Lancet Reg Health West Pac. (2022) 27:100604. doi: 10.1016/j.lanwpc.2022.100604
37. Sorg AL, Schönfeld V, Siedler A, Hufnagel M, Doenhardt M, Diffloth N, et al. SARS-CoV-2 variants and the risk of pediatric inflammatory multisystem syndrome temporally associated with SARS-CoV-2 among children in Germany. Infection. (2022) 51(3):729–35. doi: 10.1007/s15010-022-01908-6
38. Cohen JM, Carter MJ, Cheung CR, Ladhani S, Group ftEPIMSTrtS-C-S. Lower risk of multisystem inflammatory syndrome in children with the Delta and omicron variants of severe acute respiratory syndrome coronavirus 2. Clin Infect Dis. (2022) 76(3):e518–21. doi: 10.1093/cid/ciac553
39. Holm M, Espenhain L, Glenthøj J, Schmidt LS, Nordly SB, Hartling UB, et al. Risk and phenotype of multisystem inflammatory syndrome in vaccinated and unvaccinated danish children before and during the omicron wave. JAMA Pediatr. (2022) 176(8):821–3. doi: 10.1001/jamapediatrics.2022.2206
40. Abraham DR, Butters C, Abdulbari Yunis N, Lishman J, Scott C, van der Zalm MM, et al. The impact of SARS-CoV-2 variants on the clinical phenotype and severity of multisystem inflammatory syndrome in children in South Africa. Pediatr Infect Dis J. (2022) 41(12):e510–e2. doi: 10.1097/INF.0000000000003691
41. Shingleton J, Williams H, Oligbu G, Powell A, Cohen J, Arditi M, et al. The changing epidemiology of PIMS-TS across COVID-19 waves: prospective national surveillance, January 2021 to July 2022, England. J Infect. (2022) 85(6):702–69. doi: 10.1016/j.jinf.2022.10.017
42. Levy M, Recher M, Hubert H, Javouhey E, Fléchelles O, Leteurtre S, et al. Multisystem inflammatory syndrome in children by COVID-19 vaccination Status of adolescents in France. JAMA. (2022) 327(3):281–3. doi: 10.1001/jama.2021.23262
43. Zambrano LD, Newhams MM, Olson SM, Halasa NB, Price AM, Boom JA, et al. Effectiveness of BNT162b2 (pfizer-BioNTech) mRNA vaccination against multisystem inflammatory syndrome in children among persons aged 12–18 years—united States, July-December 2021. MMWR Morb Mortal Wkly Rep. (2022) 71(2):52–8. doi: 10.15585/mmwr.mm7102e1
44. Office for National Statistics. Coronavirus (COVID-19) vaccination uptake in school pupils, England. (2022).
45. Swann OV, Holden KA, Turtle L, Pollock L, Fairfield CJ, Drake TM, et al. Clinical characteristics of children and young people admitted to hospital with COVID-19 in United Kingdom: prospective multicentre observational cohort study. Br Med J. (2020) 370:m3249. doi: 10.1136/bmj.m3249
46. Venkataraman A, Kumar NP, Hanna LE, Putlibai S, Karthick M, Rajamanikam A, et al. Plasma biomarker profiling of PIMS-TS, COVID-19 and SARS-CoV2 seropositive children – a cross-sectional observational study from southern India. eBioMedicine. (2021) 66:103317. doi: 10.1016/j.ebiom.2021.103317
47. Moss P. The T cell immune response against SARS-CoV-2. Nat Immunol. (2022) 23(2):186–93. doi: 10.1038/s41590-021-01122-w
48. Gao F, Mallajoysula V, Arunachalam PS, van der Ploeg K, Manohar M, Röltgen K, et al. Spheromers reveal robust T cell responses to the pfizer/BioNTech vaccine and attenuated peripheral CD8+ T cell responses post SARS-CoV-2 infection. Immunity. (2023) 56(4):864–78.e4. doi: 10.1016/j.immuni.2023.03.005
49. Lauring AS, Tenforde MW, Chappell JD, Gaglani M, Ginde AA, McNeal T, et al. Clinical severity of, and effectiveness of mRNA vaccines against, COVID-19 from omicron, delta, and alpha SARS-CoV-2 variants in the United States: prospective observational study. Br Med J. (2022) 376:e069761. doi: 10.1136/bmj-2021-069761
50. Hu S, Xiang D, Zhang X, Zhang L, Wang S, Jin K, et al. The mechanisms and cross-protection of trained innate immunity. Virol J. (2022) 19(1):210. doi: 10.1186/s12985-022-01937-5
51. Schaltz-Buchholzer F, Biering-Sørensen S, Lund N, Monteiro I, Umbasse P, Fisker AB, et al. Early BCG vaccination, hospitalizations, and hospital deaths: analysis of a secondary outcome in 3 randomized trials from Guinea-Bissau. J Infect Dis. (2018) 219(4):624–32. doi: 10.1093/infdis/jiy544
52. Tannous J, Pan AP, Potter T, Bako AT, Dlouhy K, Drews A, et al. Real-world effectiveness of COVID-19 vaccines and anti-SARS-CoV-2 monoclonal antibodies against postacute sequelae of SARS-CoV-2: analysis of a COVID-19 observational registry for a diverse US metropolitan population. BMJ Open. (2023) 13(4):e067611. doi: 10.1136/bmjopen-2022-067611
53. Hirabara SM, Serdan TDA, Gorjao R, Masi LN, Pithon-Curi TC, Covas DT, et al. SARS-COV-2 variants: differences and potential of immune evasion. Front Cell Infect Microbiol. (2022) 11:781429. doi: 10.3389/fcimb.2021.781429
54. Escalera A, Gonzalez-Reiche AS, Aslam S, Mena I, Laporte M, Pearl RL, et al. Mutations in SARS-CoV-2 variants of concern link to increased spike cleavage and virus transmission. Cell Host Microbe. (2022) 30(3):373–87.e7. doi: 10.1016/j.chom.2022.01.006
55. Felsenstein S, Hedrich CM. SARS-CoV-2 infections in children and young people. Clin Immunol. (2020) 220:108588. doi: 10.1016/j.clim.2020.108588
56. Mueed A, Aliani R, Abdullah M, Kazmi T, Sultan F, Khan A. School closures help reduce the spread of COVID-19: a pre- and post-intervention analysis in Pakistan. PLOS Global Public Health. (2022) 2(4):e0000266. doi: 10.1371/journal.pgph.0000266
57. Liyaghatdar Z, Pezeshkian Z, Mohammadi-Dehcheshmeh M, Ebrahimie E. Fast school closures correspond with a lower rate of COVID-19 incidence and deaths in most countries. Inform Med Unlocked. (2021) 27:100805. doi: 10.1016/j.imu.2021.100805
58. Office for National Statistics. COVID-19 Schools Infection Survey, England: pupil antibody data, November to December 2021. (2021). Available at: https://www.ons.gov.uk/peoplepopulationandcommunity/healthandsocialcare/conditionsanddiseases/bulletins/covid19schoolsinfectionsurveyengland/pupilantibodydatanovembertodecember2021
59. Office for National Statistics. Coronavirus (COVID-19) Infection Survey, antibody and vaccination data, UK: 8 December 2021. (2021) Available at: https://www.ons.gov.uk/peoplepopulationandcommunity/healthandsocialcare/conditionsanddiseases/bulletins/coronaviruscovid19infectionsurveyantibodyandvaccinationdatafortheuk/8december2021
60. Abo-Haded HM, Alshengeti AM, Alawfi AD, Khoshhal SQ, Al-Harbi KM, Allugmani MD, et al. Cytokine profiling among children with multisystem inflammatory syndrome versus simple COVID-19 infection: a study from northwest Saudi Arabia. Biology (Basel). (2022) 11(7):946. doi: 10.3390/biology11070946
61. Fernandes DM, Oliveira CR, Guerguis S, Eisenberg R, Choi J, Kim M, et al. Severe acute respiratory syndrome coronavirus 2 clinical syndromes and predictors of disease severity in hospitalized children and youth. J Pediatr. (2021) 230:23–31.e10. doi: 10.1016/j.jpeds.2020.11.016
62. Liverpool John Moores University (LJMU). Children and young people health and wellbeing profile: Liverpool city region Update 2020 [pdf]. (2020). Available from: https://www.ljmu.ac.uk/~/media/phi-reports/pdf/2020-08-children-and-yp-health-wb-profile-liverpool-city-region.pdf
63. U.K. Government DoHaSC. COVID-19 vaccination of children aged 6 months to 4 years: JCVI advice. (2022).
64. Martin-Vicente M, Carrasco I, Muñoz-Gomez MJ, Lobo AH, Mas V, Vigil-Vázquez S, et al. Antibody levels to SARS-CoV-2 spike protein in mothers and children from delivery to six months later. Birth. (2023) 50(2):418–27. doi: 10.1111/birt.12667
65. Kashani-Ligumsky L, Lopian M, Cohen R, Senderovich H, Czeiger S, Halperin A, et al. Titers of SARS CoV-2 antibodies in cord blood of neonates whose mothers contracted SARS CoV-2 (COVID-19) during pregnancy and in those whose mothers were vaccinated with mRNA to SARS CoV-2 during pregnancy. J Perinatol. (2021) 41(11):2621–4. doi: 10.1038/s41372-021-01216-1
66. Rau CA-O, Auer-Hackenberg L, Deubzer HE, Schwabel E, Jaros M, Diederichs A, et al. Treatment of infants and children with SARS-CoV-2 monoclonal antibodies: a European case series. J Pediatr Infect Dis. (2023) 42(2):125–9. doi: 10.1097/INF.0000000000003773
67. Feldstein LR, Rose EB, Horwitz SM, Collins JP, Newhams MM, Son MBF, et al. Multisystem inflammatory syndrome in U.S. Children and adolescents. N Engl J Med. (2020) 383(4):334–46. doi: 10.1056/NEJMoa2021680
68. Jatczak-Pawlik I, Lewek J, Czkwianianc E, Blomberg A, Krysiak N, Zeman K, et al. Biochemical and cardiovascular predictors of PIMS-TS risk in children after COVID-19 recovery: preliminary results of the LATE-COVID-kids study. Arch Med Sci. (2022) 18(2):545–52. doi: 10.5114/aoms/146827
69. Götzinger F, Santiago-García B, Noguera-Julián A, Lanaspa M, Lancella L, Calò Carducci FI, et al. COVID-19 in children and adolescents in Europe: a multinational, multicentre cohort study. Lancet Child Adolesc Health. (2020) 4(9):653–61. doi: 10.1016/S2352-4642(20)30177-2
70. Saatci D, Ranger TA, Garriga C, Clift AK, Zaccardi F, Tan PS, et al. Association between race and COVID-19 outcomes among 2.6 million children in England. JAMA Pediatr. (2021) 175(9):928–38. doi: 10.1001/jamapediatrics.2021.1685
71. Gurlevik SL, Ozsurekci Y, Sağ E, Derin Oygar P, Kesici S, Akca ÜK, et al. The difference of the inflammatory milieu in MIS-C and severe COVID-19. Pediatr Res. (2022) 92(6):1805–14. doi: 10.1038/s41390-022-02029-4
72. González-García N, Castilla-Peón MF, Solórzano Santos F, Jiménez-Juárez RN, Martínez Bustamante ME, Minero Hibert MA, et al. COVID-19 incidence and mortality by age Strata and comorbidities in Mexico city: a focus in the pediatric population. Front Public Health. (2021) 9:738423. doi: 10.3389/fpubh.2021.738423
73. Tiwari L, Gupta P, Singh CM, Singh PK. Persistent positivity of SARS-CoV-2 nucleic acid in asymptomatic healthcare worker: infective virion or inactive nucleic acid? BMJ Case Rep. (2021) 14(3):e241087. doi: 10.1136/bcr-2020-241087
74. Cron RQ. Biologic disease-modifying antirheumatic drugs to treat multisystem inflammatory syndrome in children. Curr Opin Rheumatol. (2022) 34(5):274–9. doi: 10.1097/BOR.0000000000000889
75. Roberts JE, Campbell JI, Gauvreau K, Lamb GS, Newburger J, Son MB, et al. Differentiating multisystem inflammatory syndrome in children: a single-centre retrospective cohort study. Arch Dis Child. (2022) 107(3):e3. doi: 10.1136/archdischild-2021-322290
76. Shahin W, Rabie W, Alyossof O, Alasiri M, Alfaki M, Mahmoud E, et al. COVID-19 in children ranging from asymptomatic to a multi-system inflammatory disease. A single-center study. Saudi Med J. (2021) 42(3):299–305. doi: 10.15537/smj.2021.42.3.20200625
77. Hedrich CM, Schnabel A, Hospach T. Kawasaki disease. Front Pediatr. (2018) 6:198. doi: 10.3389/fped.2018.00198
78. National Institute for Health and Care Excellence. COVID-19 rapid guideline: managing COVID-19. (2022).
79. NHS England. Commissioning Framework: COVID-19 therapeutics for non-hospitalised patients. (2022). Available at: https://www.england.nhs.uk/coronavirus/publication/commissioning-framework-covid-19-therapeutics-for-non-hospitalised-patients/ (Updated March 29, 2023).
80. RECOVERY Trial. Randomised Evaluation of COVID-19 Therapy (RECOVERY). (2020). Available at: https://clinicaltrials.gov/ct2/show/NCT04381936
Keywords: acute COVID-19 children, MIS-C multisystem inflammatory syndrome in children, PIMS-TS, retrospective study, epidemiology, clinical feature
Citation: Jiju P, Matalliotakis M, Lane S, Wong W, Hedrich CM and Pain CE (2023) Demographic, clinical and laboratory differences between paediatric acute COVID-19 and PIMS-TS—results from a single centre study in the UK. Front. Pediatr. 11:1219654. doi: 10.3389/fped.2023.1219654
Received: 9 May 2023; Accepted: 20 October 2023;
Published: 10 November 2023.
Edited by:
Giovanna Ferrara, Azienda USL Toscana Centro, ItalyReviewed by:
Claire Deakin, University College London, United KingdomAleš Janda, Ulm University Medical Center, Germany
© 2023 Jiju, Matalliotakis, Lane, Wong, Hedrich and Pain. This is an open-access article distributed under the terms of the Creative Commons Attribution License (CC BY). The use, distribution or reproduction in other forums is permitted, provided the original author(s) and the copyright owner(s) are credited and that the original publication in this journal is cited, in accordance with accepted academic practice. No use, distribution or reproduction is permitted which does not comply with these terms.
*Correspondence: Christian M. Hedrich Y2hyaXN0aWFuLmhlZHJpY2hAbGl2ZXJwb29sLmFjLnVr
†These authors share last authorship