- 1School of Pharmacy, Anhui University of Chinese Medicine, Hefei, China
- 2Department of Pharmacy, The First Affiliated Hospital of Anhui University of Traditional Chinese Medicine, Hefei, China
Cell necrosis and neuroinflammation play an important role in brain injury induced by ischemic stroke. Previous studies reported that Taohong Siwu decoction (THSWD)can reduce heart muscle cell necrosis and has anti-inflammatory properties. In this study, we investigated the effects of THSWD on cell necrosis and neuroinflammation in a rat model of middle cerebral artery occlusion (MCAO). Thirty-six male Sprague-Dawley (SD) rats were randomly divided into three groups with 12 rats in each group. They were the sham operation group, MCAO model group, and MCAO + THSWD group. We used ELISA to determine the levels of TNF-α, Mcp-1, and IL-1β inflammatory factors in rat serum, qRT‐PCR to detect the expression of TNF‐α, Mcp‐1 and IL‐1β mRNA in rat brain, and immunohistochemistry to detect the number of microglia and neutrophils in rat brain. qRT-PCR and Western blot were used to detect the mRNA and protein expression levels of IBA-1 and MPO inflammatory factors and the TNF-α/RIP1/RIP3/MLKL pathway in the rat brain and protein expression levels. Compared with the sham operation group, the expression of MCP-1, IL-1β, IBA-1, and MPO inflammatory factors and the TNF-α/RIP1/RIP3/MLKL pathway were significantly upregulated in the MCAO group. Compared with the MCAO group, the expressions of MCP-1, IL-1β, IBA-1, and MPO inflammatory factors and the TNF-α/RIP1/RIP3/MLKL pathway were significantly downregulated in the MCAO + THSWD group. THSWD can reduce the expression levels of MCP-1, IL-1β, IBA-1, and MPO inflammatory factors as well as the TNF-α/RIP1/RIP3/MLKL pathway. Meanwhile, it can reduce the necrosis and inflammation of brain cells after cerebral ischemia, so as to protect the brain tissue of rats.
Introduction
Ischemic stroke is a disorder of cerebral blood circulation, which causes cerebral ischemia and hypoxia and leads to localized ischemic necrosis of brain tissue. Meanwhile, it can activate nonspecific inflammation of local tissue, which is an important factor for aggravating ischemic brain injury. Cell necrosis and neuroinflammation play an important role in transient ischemic stroke and reperfusion (Chen et al., 2016; Wang et al., 2021). For example, the neuroinflammatory response to ischemic stroke is characterized by astrocyte activation, microglial residence, infiltration of peripheral leukocytes, and release of pro-inflammatory mediators. In addition, infiltrating neutrophils and activated microglia produce free radicals and oxidants that damage central nervous system tissues, which lead to long-term disability and death in stroke patients (Roger et al., 2012). Therefore, the development of a protective strategy against cell necrosis and neuroinflammation may be an effective approach in the treatment of patients with ischemic stroke.
Taohong Siwu Decoction (THSWD) is from Wu Qian’s “The Golden Guide to Medicine” of the Qing Dynasty. The formula consists of safflower and peach seeds, which promote blood circulation and remove blood stasis. Meanwhile, it also uses angelica and rehmanniae to replenish qi and produce blood (Zhang, 2014). Studies have shown that THSWD have many advantages for dong sth. For example, inhibiting the expression of pro-apoptotic protein Bax, promoting the expression of anti-apoptotic protein Bcl2, reducing the exudation of lactate dehydrogenase (LDH), increasing the activity of antioxidant enzyme superoxide dismutase (SOD), and reducing the content of lipid oxide malondialdehyde (MDA) in a simulated ischemic animal model, etc. The mechanism may be related to the inhibition of cell oxidation and apoptosis (Mao et al., 2016). In the rat model of postherpetic neuralgia, THSWD has an analgesic effect on postherpetic neuralgia in rats by inhibiting the release of inflammatory factors such as tumor necrosis factor-α (TNF-α) and interleukin-1β (IL-1β) and reducing the apoptosis of spinal nerve cells (Xu et al., 2021). Clinically, studies have shown that THSWD can effectively dredge the blood and qi in patients with ischemic stroke, and improve the microcirculation of the brain tissue. Especially, it can reduce the patient’s body inflammation and nerve damage, which more effectively promotes restoration of nerve function in the patient (Zhang, 2019). The research by Wang et al. (2020) shows that THSWD can decrease the activation of NLRP3 inflammasome, downregulate GSDMD, and inhibit cell pyrotosis in MCAO/R rats. Chen et al., 2020 research shows that THSWD can promote angiogenesis after cerebral ischemia in rats by regulating platelet particles, and then treating cerebral ischemia. Other studies have shown that THSWD may regulate the survival of neurons by upregulating the expression of brain-derived neurotrophic factor (BDNF), activating the BDNF-TrkB-ERK1/2 signaling pathway, there by promoting the recovery of cerebral ischemic injury (Wu, 2018).
From the perspective of apoptosis, programmed cell necrosis initiated by the tumor necrosis factor receptor (TNFR) family and toll-like receptor (TLRS) family is the most studied necrosis pathway at present. In the cell, it is mainly mediated by receptor-interacting protein kinases RIPK1 and RIPK3, recruited, and phosphorylated into a mixed lineage kinase-domain-like protein (MLKL), which eventually forms necrotic bodies and causes cell death (Shan et al., 2018).
By replicating the rat model of cerebral ischemia, this study explored whether THSWD can reduce cell necrosis and neuroinflammation in the rat model of MCAO by inhibiting the expression levels of MCP-1, IL-1β, IBA-1, and MPO inflammatory factors as well as the TNF-α/RIP1/RIP3/MLKL pathway. It provides an experimental basis and theoretical basis for the further development of THSWD.
Materials and Methods
Animals
A total of 36 adult male Sprague-Dawley rats (230–270 g) were obtained from the Experimental Animal Center of Anhui Medical University. Rats were kept in a humidity and temperature-controlled chamber with a 12-h light/dark cycle and free access to water and food.
Medicinal Materials
THSWD composition: angelica (Angelicae Sinensis Radix, batch number: 16070501), rehmanniae (Rehmanniae Radix Praeparata, batch number: 17042501) and chuanxiong (Chuanxiong Rhizoma, batch number: 17061601), radix paeoniae alba (Paeoniae Radix Alba, batch number: 17050301), peach kernel (Persicae Semen, batch number: 17033101), safflower (Carthami Flos, batch number: 17041401) each 100 g, All purchased from the pharmacy of the First Affiliated Hospital of Anhui University of Traditional Chinese Medicine. According to the ratio of peach kernel: safflower: rehmanniae: angelica: radix paeoniae alba: chuanxiong = 3:2:4:3:3:2, put the herbal medicine in a beaker, first extract with 10 times the amount of water for 2 h, filter, save the filtrate, the filtrate is extracted with 8 times the amount of water for 1.5 h, filter, combine the two filtrates, concentrate the filtrate to make a concentrate containing 0.9 g/ml of herbal medicine, and store it in a low temperature place away from light (Duan et al., 2020).
Animal Groups
After adaptive feeding for 1 week, 36 rats were randomly divided into three groups with 12 mice in each group: sham operation group, MCAO group and MCAO + THSWD group. In the MCAO + THSWD group, 9 g/(kg-d) of THSWD was administered by gavage on the second day of modeling, and the same amount of saline was administered by gavage on the second day of modeling in the MCAO and sham operation groups once a day for 7 days. All rats were anesthetized by intraperitoneal injection of chloral hydrate (350 mg-kg) 2 h after the last dose, and the brain tissues were removed by severing the head, and the brain tissues of each group were fixed with 4% paraformaldehyde and frozen at −80°C in the refrigerator.
Preparation of MCAO
The model of middle cerebral artery occlusion (MCAO) in rats was established according to the method of Longa et al. (Longa et al., 1989). After fasting for 12 h before surgery, the rats were anesthetized by intraperitoneal injection of 10% chloral hydrate (350 mg/kg−1). The right common carotid artery, external carotid artery, and internal carotid artery were successively separated. The main external carotid artery was ligated and dissociated. When the nylon thread was inserted 18–20 mm from the bifurcation of the common carotid artery and there was slight resistance, the thread was stopped. After 2 h of ischemia, the thread was gently pulled out to restore blood perfusion. The external carotid artery was ligated and the skin was sutured. In the sham operated group, only a surgical incision was made and no wire plugs were inserted. It was assessed using the Zea Longa 5-point scale (Longa et al., 1989): 0 points–no neurological deficit; 1 points–when lifting the tail, it was seen that the left front paw could not be fully extended; 2 points–when lifting the tail, it was seen that the left front paw could not be fully extended and turned in a circle to the left; 3 points–it was seen that the rat rotated or tilted to the left when walking; 4 points–it was seen that the rat could not walk spontaneously and lost consciousness. Those with scores of 1–3 were included in the experiment, and those with scores of 0 or 4 were excluded.
ELISA
Blood was collected from the rat abdominal aorta and centrifuged at 2000 rpm/min for 15 min after 30 min at room temperature, and the serum was extracted. The relevant indexes were tested according to the Elisa kit procedure.
Immunohistochemistry
The brain tissue fixed in 4% paraformaldehyde solution was removed for paraffin-embedding and made into paraffin sections. The procedure was as follows: Dewaxing → hydration → antigen repair → normal serum sealing → drop addition of MPO and IBA-1 antibodies anti-Iba1 (abclonal, A1527, 1:100) and anti-Mpo (abclonal, A1374, 1:100) → DAB color rendering → redyeing → blue return → gradient alcohol dehydration → transparent sealing → the number and morphology of microglia and neutrophils were analyzed by microscopic observation.
Quantitative Real-Time PCR
Total RNA was extracted from each group of rat brain tissue according to the instructions of EZ-10 Total RNA Mini-Preps Kit (B618583, Sangon Biotech). Quantitative PCR primers were designed according to Primer Premier version 6.0 software, and reverse transcription was performed according to ABScript II RT Mix for qPCR with gDNA Remover (RK20403, Abclonal) reverse transcription kit. qPCR reactions were performed using a Biorad IQ5 real-time PCR instrument with 2× Universal SYBR Green Fast qPCR Mix (RK21203, Abclonal) in sybr green (Supplementary Table S1).
Western Blot
The rats were treated with the above method by Western blot. The left brain tissue was taken and put into a 5 ml enzyme-free centrifuge tube. Magnetic beads and RIPA solution containing PMSF were added, and the tissue was smashed with a tissue grinding instrument. The nuclear proteins were extracted according to the instructions of the nuclear protein and cytoplasmic protein extraction kit. According to the instructions of BCA Protein Assay Kit, determine the protein concentration of the sample, make a standard curve, calculate the protein concentration, add the corresponding SDS Protein Loading Buffer, mix well and heat in a metal bath thermostat at 100°C for 10 min, and place on ice. The proteins were separated by SDS-PAGE gel electrophoresis, then transferred to PVDF membrane and closed at room temperature for 2 h using 5% skimmed milk powder. The PVDF membrane was added to TBST and washed 3 times for 8 min each. Add the corresponding primary antibody, the membrane was incubated overnight at 4°C with primary antibodies including anti-Actb (abclonal, AC038, 1:10000), anti-Iba-1 (abclonal, A1527, 1:500), anti-Mpo (abclonal, A1374, 1:1000), anti-Tnfa (abclonal, A0277, 1:1000), anti-Rip1 (abclonal, A7414, 1:1000), anti-Rip3 (abclonal, A12996, 1:1000), anti-Mlkl (A5579, 1:1000) and wash the membrane 3 times with TBST for 8 min each time the next day. The PVDF membranes were then incubated with the secondary antibody (HRP Goat Anti-Rabbit IgG (H+L) (abclonal, AS014, 1:8000), and developed with enhanced chemiluminescence (ECL, beyotime, P0018AM). The PVDF membrane was put into the ultra-sensitive multifunctional imager, the developing solution was added, and the bands with different objectives were obtained by exposure after the reaction, and the protein grayscale values were analyzed by Image J software.
Statistical Methods
SPSS 20.0 software was used to statistically analyze the data. Normally distributed measures were expressed as mean ± standard deviation (x ¯± s), and independent samples t-test was used for comparison between groups, paired t-test for comparison within groups, and one-way ANOVA was used for comparison of data between multiple groups. p < 0.05 was considered a statistically significant difference.
Results
Neurological Deficit Score
Compared with the sham operation group, the neurological deficit score was significantly higher in the MCAO group (p < 0.01), indicating successful modeling, and the neurological deficit score was significantly lower in the MCAO+THSWD group compared with the MCAO group (p < 0.05), as shown in Figure 1.
THSWD Inhibited TNF-α, MCP-1, and IL-1β
Elisa results showed that the levels of TNF-α, Mcp-1 and IL-1β were significantly increased in the MCAO group compared with the sham operation group (p < 0.01), and the levels of TNF-α, Mcp-1 and IL-1β were significantly decreased in the MCAO+THSWD group compared with the MCAO group (p < 0.01), as shown in Figure 2. The qPCR results showed that TNF-α, Mcp-1, and IL-1β mRNA expressions were significantly increased in the MCAO group compared with the sham operation group (p < 0.01), and TNF-α, Mcp-1, and IL-1β mRNA expressions were significantly decreased in the MCAO+THSWD group compared with the MCAO group (p < 0.05, p < 0.01), as shown in Figure 3. It indicates that THSWD can inhibit the release of TNF-α, Mcp-1, and IL-1β inflammatory factors.
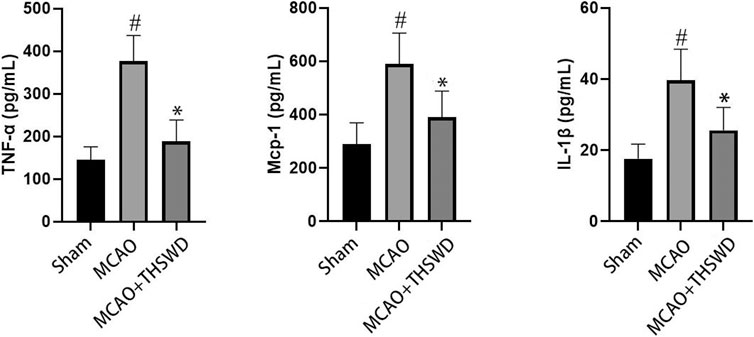
FIGURE 2. TNF-α, MCP-1, and IL-1β were detected by ELISA. Notes: #P<0.01 vs Sham operation group; *P<0.01 vs MCAO group.
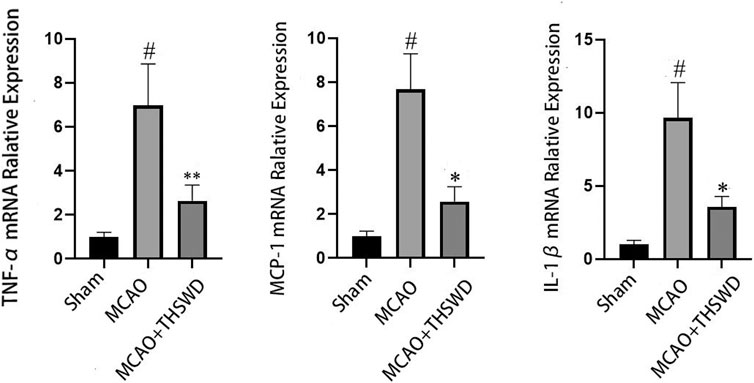
FIGURE 3. mRNA expression of TNF-α, MCP-1, and IL-1β. Notes: #P<0.01 vs Sham operation group; *P<0.01, **P<0.05 vs MCAO group.
THSWD Inhibited IBA-1 and MPO
IBA-1 levels are used to assess microglial activation in brain tissue (Chen et al., 2018). MPO levels are used to assess neutrophil infiltration (Yu et al., 2018.). The qPCR results showed that the mRNA expressions of IBA-1 and MPO significantly increased in the MCAO group compared with the sham operation group (p < 0.01); compared with MCAO group, the mRNA expression of IBA-1 and MPO in the MCAO + THSWD group decreased significantly (p < 0.01), as shown in Figure 4. The results of Western blot showed that the protein expression of IBA-1 and MPO in the MCAO group significantly increased compared with the sham operation group (p < 0.05). Compared with the MCAO group, the expression of IBA-1 and MPO protein in the MCAO + THSWD group decreased significantly (p < 0.05), as shown in Figures 5, 6.
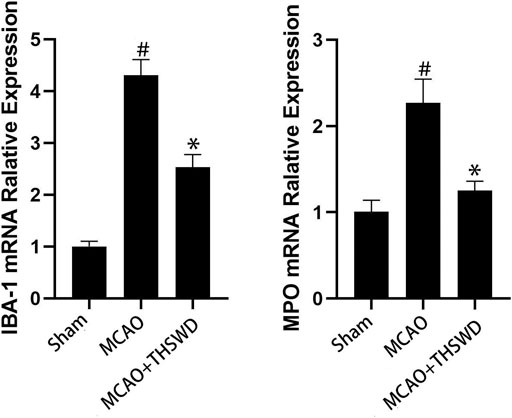
FIGURE 4. mRNA expression of IBA-1 and MPO. Notes: #P<0.01 vs Sham operation group; *P<0.01 vs MCAO group.
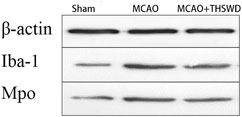
FIGURE 5. β-actin is an internal reference. The blacker the band, the higher the protein expression level.
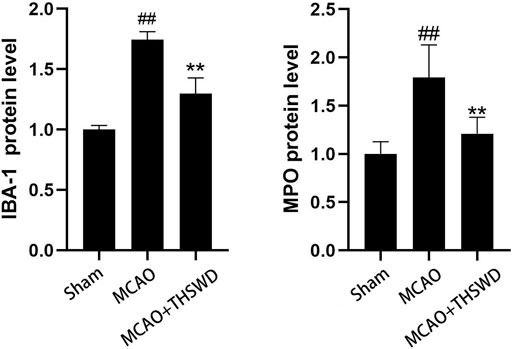
FIGURE 6. Protein expression of IBA-1 and MPO Notes: ##P<0.05 vs Sham operation group; **P<0.05 vs MCAO group.
We next evaluated the activation status of microglia and the degree of neutrophil infiltration in brain tissue using IBA-1 and MPO. Immunohistochemical results (Figure 7) showed that microglia and neutrophils were mostly in a resting state in normal conditions; microscopic observation revealed that microglia and neutrophils were heavily activated and infiltrated in the ischemic parts of the brain of rats in the MCAO group, with increased numbers and larger cytosomes. There was an increased number of microglia and neutrophils in the same part of the rat brain compared to the sham operated group. After administration of THSWD treatment to MCAO model rats, microglia and neutrophil activation and infiltration at the ischemic site of the rat brain were significantly inhibited, and the number of IBA-1 and MPO positive cells was significantly reduced compared with the MCAO group. The results indicate that THSWD can inhibit the hyperactivation and infiltration of microglia and neutrophils at the ischemic site in the brain of MCAO model rats.
THSWD Inhibited the TNF-α/RIP1/RIP3/MLKL Pathway
TNF level is used to evaluate the expression of cellular inflammatory factors (Zhao and Zhang, 2021). The RIP1-RIP3-MLKL pathway is used to evaluate programmed apoptosis (Zhang et al., 2020). The qPCR results showed that the mRNA expression of TNF-α/RIP1/RIP3/MLKL significantly increased in the MCAO group compared with the sham operation group (p < 0.01); compared with the MCAO group, the mRNA expression of TNF-α/RIP1/RIP3/MLKL in the MCAO + THSWD group significantly decreased (p < 0.01), as seen in Figure 8. Western blot results showed that compared with the sham operation group, the expression of TNF-α/RIP1/RIP3/MLKL proteins significantly increased in the MCAO group (p < 0.05); compared with the MCAO group, the protein expression of TNF-α/RIP1/RIP3/MLKL in the MCAO + THSWD group significantly decreased (p < 0.05), seen in Figures 9, 10.
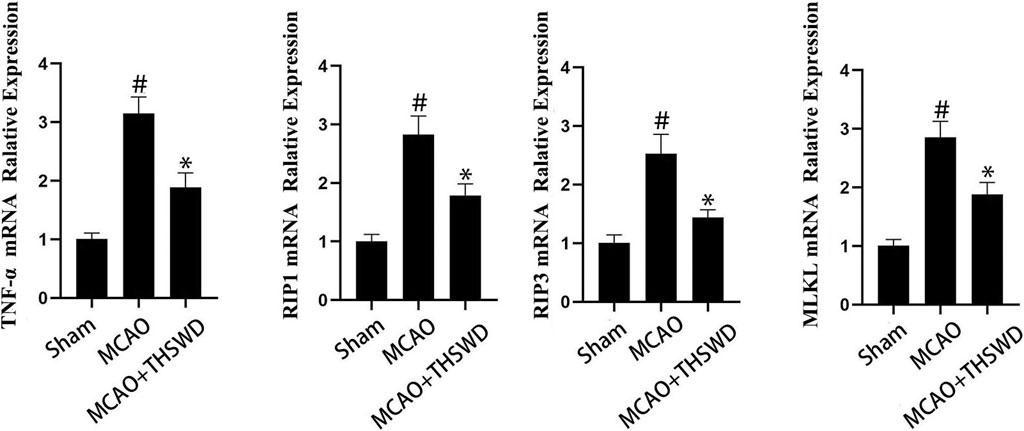
FIGURE 8. mRNA expression of TNF-α/RIP1/RIP3/MLKL. Notes: #P<0.01 vs Sham operation group; *P<0.01 vs MCAO group.
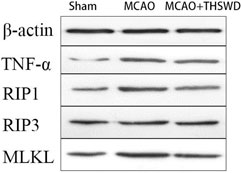
FIGURE 9. β-actin is an internal reference. The blacker the band, the higher the protein expression level.
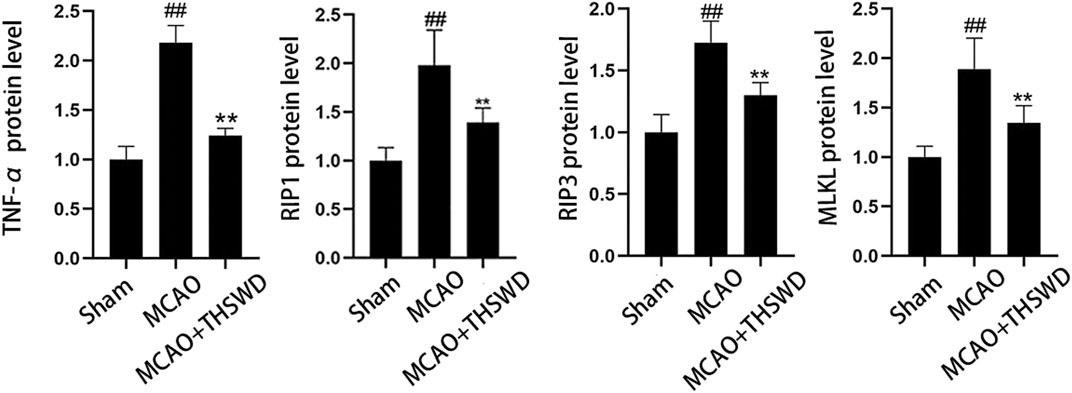
FIGURE 10. Protein expression of TNF-α/RIP1/RIP3/MLKL Notes: ##P<0.05 vs Sham operation group; **P<0.05 vs MCAO group.
Discussion
In this study, we demonstrated that THSWD alleviated cell necrosis and neuroinflammation after MCAO in rats. The following observations were made: 1) THSWD inhibited neutrophil infiltration, microglia activation, and cell necrosis in the ischemic area after MCAO; 2) by inhibiting the expression levels of MCP-1, IL-1β, IBA-1, and MPO inflammatory factors and the TNF-α/RIP1/RIP3/MLKL pathway, the anti-inflammatory effect of THSWD was promoted and the release of TNF-α, IL-6, and other inflammatory factors was reduced. In summary, our results suggest that THSWD alleviates cell necrosis and neuroinflammation in MCAO by reducing the expression levels of MCP-1, IL-1β, IBA-1, and MPO inflammatory factors and inhibiting the TNF-α/RIP1/RIP3/MLKL pathway.
More and more studies have shown that a variety of inflammatory cytokines, such as TNF-α, IL-1β, and IL-6, are released after cerebral ischemia, which can transform ischemic injury into inflammatory injury and promote the apoptosis and necrosis of nerve cells and the formation of brain edema (Fann et al., 2013). The inhibition of early inflammatory response can prevent further brain necrosis and improve cerebral nerve function (Duan et al., 2020). MPO is a marker of neutrophils activation, and the detection of MPO in brain tissue can reflect the degree of neutrophils infiltration and local inflammatory reaction (Zhang et al., 2017). It can effectively reduce the contents of TNF-α and MPO in brain tissue of rats with cerebral ischemia-reperfusion injury, which has a significant effect on brain protection (Lei et al., 2016). IBA-1 can specifically bind to microglia cells and is often used as a marker to identify microglia cells. When microglia cells are activated by chronic stress, their morphology changes and the expression of IBA-1 is upregulated (Waller et al., 2019). Consistent with these findings, THSWD reduced neutrophilic infiltration, microglia activation, and the amount of cell necrosis according to our results. Specifically, THSWD decreased and inhibited the protein expression of TNF-α (a marker of inflammation) and IBA-1 (a marker of microglia).
According to the literature, MCP-1 is a glycoprotein regulated by nuclear transcription factor-κB (NF-κB), which can be produced by mesangial cells stimulated by TNF-α (Sheryanna et al., 2007). Glycoprotein MCP-1 regulated by NF-κB not only promotes the migration, adhesion, and aggregation of inflammatory cells, but also has dual functions of inducing chemotaxis and activating monocytes to inflammatory sites (Chen et al., 2012). Chemokine ligand 1 (CXCL1), also known as GRO-α oncogene, is a member of the chemokine family (Cecchinato and Uguccioni, 2018). Originally found in melanoma, it is also expressed in macrophages, neutrophils, and epithelial cells. CXCL1 is mainly expressed and secreted by vascular endothelial cells, activated macrophages, and fibroblasts in peripheral tissues, while it is mainly expressed and secreted by neurons, microglia, oligodendrocytes, and activated astrocytes in brain tissues (Zhang et al., 2017). By recruiting neutrophils, CXCL1 activates neutrophils FGR and HCK (a member of the protein tyrosine kinase PTK family) and promotes the release of VEGF-A, thereby promoting angiogenesis in vivo (Scapini et al., 2004). The results of ELISA and qPCR showed that THSWD could inhibit the release of TNF-α, MCP-1, and IL-1β inflammatory factors.
Currently, there are many ways to induce programmed necrosis. Among these ways, the RIP1/RIP3/MLKL signaling pathway is the most typical one (Wang et al., 2019). RIP1/RIP3 is a key regulatory node of programmed necrosis, which is widely present in neurons (Zhou et al., 2017). Under the stimulation of death signal TNF and other stimulation, programmed cell death can be promoted through autophosphorylation (Xin et al., 2017; Huang et al., 2018). The expression level of RIP1/RIP3 was positively correlated with programmed cell necrosis. Inflammatory factors such as TNF-α and IL-6 are highly expressed in acute cerebral infarction, presenting as non-infectious inflammation (Fu et al., 2015). RIP1/RIP3 plays a key regulatory role in the radiation damage of central nervous tissue and inflammatory response of nervous tissue after vasospasm and ischemia (Das et al., 2016). MLKL is a key protein signaling molecule downstream of RIP3 in the TNF-α receptor-mediated programmed cell necrosis pathway. MLKL is a substrate for RIPK3 kinase in an inactive form. The C-terminal region of MLKL contains the kinase domain, and the N-terminal region contains the 4-helix domain. Activation of MLKL kinase depends on RIPK3-induced residual phosphorylation of the kinase domain (Qian and Sun, 2021). When phosphorylated, RIPK3 binds to MLKL’s 4HBD, thereby transforming MLKL from an inactive monomer structure to an active oligomer structure. Activated RIPK1 and RIPK3 recruit and phosphorylate MLKL and activate downstream signal transduction pathways to perform programmed cell necrosis (Zhao et al., 2012). Activation of MLKL kinase is considered to be an important link in the execution of programmed cell necrosis (Fang et al., 2019). According to the results of this paper, it can be obtained that THSWD can reduce brain injury after MCAO in rats by inhibiting the TNF-α/RIP1/RIP3/MLKL pathway and inhibiting programmed cell necrosis.
Our study showed that THSWD could reduce cell necrosis and neuroinflammation by inhibiting the expression levels of MCP-1, IL-1β, IBA-1, and MPO inflammatory factors as well as inhibiting the TNF-α/RIP1/RIP3/MLKL pathway. However, our results do not completely rule out the alternative pathways that regulate the inflammasome pathway. Therefore, further studies need to investigate the relationship of other inflammatory activators to rule out or include alternative pathways.
Conclusion
In conclusion, our results suggest that THSWD can inhibit neutrophil infiltration, microglia activation, and cell necrosis in the ischemic area after MCAO. Meanwhile, it can inhibit the expression levels of MCP-1, IL-1β, IBA-1, and MPO inflammatory factors, and inhibit the TNF-α/RIP1/RIP3/MLKL pathway. Finally, it can reduce cell necrosis and neuroinflammation. This study supports continued research where THSWD is used as a potential treatment for patients with ischemic stroke.
Data Availability Statement
The raw data supporting the conclusions of this article will be made available by the authors, without undue reservation.
Ethics Statement
The animal study was reviewed and approved by the Committee on the Ethics of Animal Experiments of Anhui University of Chinese medicine (Permit Number: AHUCM-Rats-2021023).
Author Contributions
XD and DP conceived and designed the study. NW, FC, and CF performed the in vitro experiments. NW wrote the manuscript, LP and SH provided ideas for the experimental design and modified the manuscripts to ensure the integrity of the entire experimental design. All authors read and approved the final manuscript.
Funding
This research was supported by the National Natural Science Fund Regional Innovation and Development Joint Fund Project (No.U19A2009), National Natural Science Foundation of China (Grant No. 82074059), Anhui University Collaborative Innovation Project (GXXT-2019-043), the Anhui Provincial College Natural Science Research Key Project (No.KJ 2019A0466, No.KJ 2020A0409), Excellent and Top Talents Program in Colleges and Universities (No. gxyq2019034) and Anhui Provincial Key Laboratory of Traditional Chinese Medicine Compounds (2019AKLCMF03).
Conflict of Interest
The authors declare that the research was conducted in the absence of any commercial or financial relationships that could be construed as a potential conflict of interest.
Publisher’s Note
All claims expressed in this article are solely those of the authors and do not necessarily represent those of their affiliated organizations, or those of the publisher, the editors and the reviewers. Any product that may be evaluated in this article, or claim that may be made by its manufacturer, is not guaranteed or endorsed by the publisher.
Supplementary Material
The Supplementary Material for this article can be found online at: https://www.frontiersin.org/articles/10.3389/fphar.2021.732358/full#supplementary-material
References
Cecchinato, V., and Uguccioni, M. (2018). Insight on the Regulation of Chemokine Activities. J. Leukoc. Biol. 104 (2), 295–300. doi:10.1002/jlb.3mr0118-014r
Chen, F.-F., Wang, M.-M., Xia, W.-W., Peng, D.-Y., and Han, L. (2020). Tao-Hong-Si-Wu Decoction Promotes Angiogenesis after Cerebral Ischaemia in Rats via Platelet Microparticles. Chin. J. Nat. Medicines 18 (8), 620–627. doi:10.1016/s1875-5364(20)30074-1
Chen, F., Wang, Q., Wei, G., Ma, X., Ma, D., Deng, W., et al. (2012). Effects of Mycophenolic Ester on the Expression of MCP-1 and FN in Human Mesangial Cells Cultured with High Glucose [J]. J. Cell Mol. Immunol. 28 (5), 455–457. doi:10.13423/j.cnki.cjcmi.006405
Chen, S., Zhao, L., Sherchan, P., Ding, Y., Yu, J., Nowrangi, D., et al. (2018). Activation of Melanocortin Receptor 4 with RO27-3225 Attenuates Neuroinflammation through AMPK/JNK/p38 MAPK Pathway after Intracerebral Hemorrhage in Mice. J. Neuroinflammation 15 (1), 106. doi:10.1186/s12974-018-1140-6
Chen, Y.-J., Nguyen, H. M., Maezawa, I., Grössinger, E. M., Garing, A. L., Köhler, R., et al. (2016). The Potassium Channel KCa3.1 Constitutes a Pharmacological Target for Neuroinflammation Associated with Ischemia/reperfusion Stroke. J. Cereb. Blood Flow Metab. 36 (12), 2146–2161. doi:10.1177/0271678x15611434
Das, A., Mcdonald, D. G., Dixon-Mah, Y. J., Jacqmin, D. J., Samant, V. N., Vandergrift, W. A., et al. (2016). RIP1 and RIP3 Complex Regulates Radiation-Induced Programmed Necrosis in Glioblastoma. Tumor Biol. 37 (6), 7525–7534. doi:10.1007/s13277-015-4621-6
Duan, X., Pan, L., Bao, Q., and Peng, D. (2020). UPLC-Q-TOF-MS Study of the Mechanism of THSWD for Breast Cancer Treatment. Front. Pharmacol. 10 (10), 1625. doi:10.3389/fphar.2019.01625
Fang, Z., Wei, Z., and Tao, Y. (2019). The Role of Programmed Necrosis in Cancer [J]. J. Zhejiang University-Science B(Biomedicine Biotechnology) 20 (05), 399–413.
Fann, D. Y.-W., Lee, S.-Y., Manzanero, S., Chunduri, P., Sobey, C. G., and Arumugam, T. V. (2013). Pathogenesis of Acute Stroke and the Role of Inflammasomes. Ageing Res. Rev. 12 (4), 941–966. doi:10.1016/j.arr.2013.09.004
Fu, C.-Y., He, X.-Y., Li, X.-F., Zhang, X., Huang, Z.-W., Li, J., et al. (2015). Nefiracetam Attenuates Pro-inflammatory Cytokines and GABA Transporter in Specific Brain Regions of Rats with Post-Ischemic Seizures. Cell Physiol Biochem. 37 (5), 2023–2031. doi:10.1159/000438562
Huang, L., Ma, Q., Li, Y., Li, B., and Zhang, L. (2018). Inhibition of microRNA-210 Suppresses Pro-inflammatory Response and Reduces Acute Brain Injury of Ischemic Stroke in Mice. Exp. Neurol. 300, 41–50. doi:10.1016/j.expneurol.2017.10.024
Lei, J., Tu, X., and Zhang, H. (2016). Cerebral Ischemia-Reperfusion Injury Induced by the Activation of PI3K/Akt Signaling Pathway in Rats with Resveratrol [J]. Chin. J. Clin. Neurosurg. 21 (7), 425–428. doi:10.13798/j.issn.1009-153X.2016.07.012
Longa, E. Z., Weinstein, P. R., Carlson, S., and Cummins, R. (1989). Reversible Middle Cerebral Artery Occlusion without Craniectomy in Rats. Stroke 20 (1), 84–91. doi:10.1161/01.str.20.1.84
Mao, H., Liu, X., and Shi, D. (2016). Research Progress in the Treatment of Coronary Heart Disease by Taohong Siwu Decoction [J]. Glob. Chin. Med. 9 (09), 1145–1148.
Qian, Y., and Sun, F. (2021). The Role of Programmed Cell Necrosis in Neuronal Death in Acute and Chronic Brain Injury [J]. J. Fudan Univ. (Medical Sciences) 48 (02), 240–247.
Roger, V. L., Go, A. S., Lloyd-Jones, D. M., Benjamin, E. J., Berry, J. D., Borden, W. B., et al. (2012). Executive Summary: Heart Disease and Stroke Statistics--2012 Update: a Report from the American Heart Association. Circulation 125 (1), 188–197. doi:10.1161/CIR.0b013e3182456d46
Scapini, P., Morini, M., Tecchio, C., Minghelli, S., Di Carlo, E., Tanghetti, E., et al. (2004). CXCL1/Macrophage Inflammatory Protein-2-Induced Angiogenesis In Vivo Is Mediated by Neutrophil-Derived Vascular Endothelial Growth Factor-A. J. Immunol. 172 (8), 5034–5040. doi:10.4049/jimmunol.172.8.5034
Shan, B., Pan, H., and Najafov, A. (2018). Necroptosis in Development and Diseases[J]. Genes Dev. 32 (5-6), 327–340. doi:10.1101/gad.312561.118
Sheryanna, A., Bhangal, G., Mcdaid, J., Smith, J., Manning, A., Foxwell, B. M. J., et al. (2007). Inhibition of P38 Mitogen-Activated Protein Kinase Is Effective in the Treatment of Experimental Crescentic Glomerulonephritis and Suppresses Monocyte Chemoattractant Protein-1 but Not IL-1β or IL-6. Jasn 18 (4), 1167–1179. doi:10.1681/asn.2006010050
Waller, R., Baxter, L., Fillingham, D. J., Coelho, S., Pozo, J. M., Mozumder, M., et al. (2019). Iba-1-/CD68+microglia Are a Prominent Feature of Age-Associated Deep Subcortical white Matter Lesions[J]. PLoS One 14 (1), e0210888. doi:10.1371/journal.pone.0210888,
Wang, B., Huang, N., and Wei, M. (2019). Research Progress of Programmed Necrosis and Tumor [J]. Chem. Life 39 (01), 75–80. doi:10.13488/j.smhx.20181265
Wang, M., Liu, Z., Hu, S., Duan, X., Zhang, Y., Peng, C., et al. (2020). Taohong Siwu Decoction Ameliorates Ischemic Stroke Injury via Suppressing Pyroptosis. Front. Pharmacol. 11, 590453. doi:10.3389/fphar.2020.590453
Wang, X., Li, Y., and Zhu, H. (2021). Effects of Glycyrrhitin on the Expression of Brain-Derived Neurotrophic Factor and Bax and Bcl-2 in the Prefrontal Cortex of Rats with post-stroke Depression [J]. Chin. J. Geriatr. Cardio-Cerebrovascular Dis. 23 (06), 647–650.
Wu, H. R. (2018). Study on the Regulation Effect of Taohong Siwu Decoction on Brain-Derived Neurotrophic Factor in Rats with Cerebral Ischemia Reperfusion[D]. PhD thesis. Anhui University of Chinese Medicine.
Xin, J., You, D., Breslin, P., Li, J., Zhang, J., Wei, W., et al. (2017). Sensitizing Acute Myeloid Leukemia Cells to Induced Differentiation by Inhibiting the RIP1/RIP3 Pathway. Leukemia 31 (5), 1154–1165. doi:10.1038/leu.2016.287
Xu, J., Wang, L., and Wang, Y. (2021). The Effect of Taohong SiWu Decoction on the Treatment of Postherpetic Neuralgia in Rats Based on EphrinBs/EphBs Pathway [J]. Tianjin Med. 49 (02), 147–152.
Yu, G., Liang, Y., Zheng, S., and Zhang, H. (2018). Inhibition of Myeloperoxidase by N-Acetyl Lysyltyrosylcysteine Amide Reduces Oxidative Stress-Mediated Inflammation, Neuronal Damage, and Neural Stem Cell Injury in a Murine Model of Stroke. J. Pharmacol. Exp. Ther. 364 (2), 311–322. doi:10.1124/jpet.117.245688
Zhang, B., Wang, L., and Po, Y. (2017a). Effects of Carbamylated Erythropoietin on Cerebral Ischemia Injury by PI3-K/Akt Signaling Pathway [J]. Stroke Neurol. Dis. 34 (6), 488–490. doi:10.19845/j.cnki.zfysjjbzz.2017.06.002
Zhang, D. (2019). Effect of Taohong Siwu Decoction on Inflammatory Factors and Neural Function in Patients with Ischemic Stroke[J]. Henan Traditional Chin. Med. 39 (12), 1854–1857. doi:10.16367/j.issn.1003-5028.2019.12.0455
Zhang, D. (2014). Research Progress in Clinical Application of Taohong Siwu Decoction[J]. J. Liaoning Univ. Traditional Chin. Med. 16 (11), 217–219. doi:10.13194/j.issn.1673-842x.2014.11.079
Zhang, L., Yao, Y., and Wang, Q. (2020). Effects of Total Flavonoids of Sphyllostachys Scl. On the RIP1/RIP3 Pathway in Rats with Acute Cerebral Infarction after Ischemia-Reperfusion [J]. J. traditional Chin. Med. 35 (07), 1476–1484. doi:10.16368/j.issn.1674-8999.2020.07.331
Zhang, Z.-J., Jiang, B.-C., and Gao, Y.-J. (2017b). Chemokines in Neuron-Glial Cell Interaction and Pathogenesis of Neuropathic Pain. Cell. Mol. Life Sci. 74 (18), 3275–3291. doi:10.1007/s00018-017-2513-1
Zhao, J., Jitkaew, S., Cai, Z., Choksi, S., Li, Q., Luo, J., et al. (2012). Mixed Lineage Kinase Domain-like Is a Key Receptor Interacting Protein 3 Downstream Component of TNF-Induced Necrosis. Proc. Natl. Acad. Sci. 109 (14), 5322–5327. doi:10.1073/pnas.1200012109
Zhao, J., and Zhang, X. (2021). Research Progress of TNF-α Inhibitor in Immunocheckpoint Therapy [J]. Cancer Prev. Treat. Res. 48 (04), 414–417.
Keywords: taohong siwu decoction, ischemic stroke, cell death, nerve inflammation, rats
Citation: Wang N, Fei C, Chu F, Huang S, Pan L, Peng D and Duan X (2021) Taohong Siwu Decoction Regulates Cell Necrosis and Neuroinflammation in the Rat Middle Cerebral Artery Occlusion Model. Front. Pharmacol. 12:732358. doi: 10.3389/fphar.2021.732358
Received: 29 June 2021; Accepted: 19 July 2021;
Published: 10 August 2021.
Edited by:
Jian Gao, Second Affiliated Hospital of Dalian Medical University, Dalian, ChinaReviewed by:
Yang Ming, Shanghai University of Traditional Chinese Medicine, Shanghai, ChinaQianru Zhang, Zunyi Medical College, China
Copyright © 2021 Wang, Fei, Chu, Huang, Pan, Peng and Duan. This is an open-access article distributed under the terms of the Creative Commons Attribution License (CC BY). The use, distribution or reproduction in other forums is permitted, provided the original author(s) and the copyright owner(s) are credited and that the original publication in this journal is cited, in accordance with accepted academic practice. No use, distribution or reproduction is permitted which does not comply with these terms.
*Correspondence: Xianchun Duan, Duanxc@ahtcm.edu.cn