- 1Julia Jones Matthews Department of Public Health, Texas Tech University Health Sciences Center, Abilene, TX, United States
- 2Edward E. Whitacre Jr. College of Engineering, Texas Tech University, Lubbock, TX, United States
- 3EPS Fragrances, Istanbul, Turkey
Reports of COVID-19 cases potentially attributed to fomite transmission led to the extensive use of various disinfectants to control viral spread. Alternative disinfectants, such as essential oils, have emerged as a potential antimicrobial. Four essential oil blends were tested on three different surfaces inoculated with a coronavirus surrogate, bacteriophage Phi 6, and a bacterial indicator, Staphylococcus aureus. Log10 concentration reductions were analyzed using GraphPad Prism software. Data collected in this study show that the application of dilute essential oil disinfectants using a spray delivery device is an effective way to reduce concentrations of bacterial and viral microorganisms on ceramic, stainless steel, and laminate surfaces. Surrogate viruses were reduced up to 6 log10 PFU and bacterial were reduced up to 4 log10 CFU. Although surfaces are no longer considered a high risk fomite for COVID-19 transmission, the disinfection of microorganisms on surfaces remains an important consideration for high touch areas in hospitals, waiting rooms, etc. The application of spray disinfectants, based on essential oil blends, provides a rapid and effective means to reduce microbial contamination on high-touched surfaces.
Introduction
During the 2020 outbreak of Severe Acute Respiratory Syndrome Coronavirus 2 (SARS-CoV-2), the causative agent of COVID-19, contaminated surfaces were proposed as a potential source for the dissemination of viral particles (1). Respiratory viruses are known to survive for hours to days on such surfaces (2–4), and viral nucleic acids have been detected on surfaces in health care, and community settings (4–7). Enhanced environmental cleaning was therefore recommended early in the pandemic as a component of outbreak control for SARS-CoV-2 (8).
As of May 2021, the United States Centers for Disease Control no longer states that SARS-CoV-2 is likely transmitted through surface contamination (8), but is more likely spread through aerosolization of viral particles (9). Despite this, much effort went into the cleaning of environmental surfaces during the pandemic and continues to be a major component of infection control protocols in areas of interaction both in hospital and community settings. Generally, surface disinfection is typically performed through the manual application of liquid or spray disinfectants. Here, we tested the effectiveness of an essential oil disinfectant on SARS-CoV-2 surrogate microorganisms. Phi 6, an RNA pseudomonas phage, has been previously evaluated as a surrogate for multiple virus pathogens including ebolavirus (10–12) and various human respiratory viruses (13, 14), including coronaviruses such as SARS-CoV-2 (3, 15, 16) on surfaces (16–18). We have also examined the use of these disinfectants against a bacterial target, Staphylococcus aureus, known to be important in health care and food service settings where the disinfection of pathogens on surfaces is important (19, 20).
Essential oil disinfectants have been proposed for a variety of uses, particularly in the inhibition or inactivation of influenza viruses in vitro, with cultured cells (21–26). Benefits of using essential oils as alternative disinfectants include their potential for application on porous surfaces that may not be effectively reached by traditional chemical disinfectants as well as the ability to combine essential oils in blends that affect microbes at different stages in the life cycle. In this study, we evaluate four different blends of essential oils on three different surface types using two SARS-CoV-2 microbial surrogates, a bacterial and a viral surrogate.
Materials and Methods
Test Organisms
Bacteriophage phi 6 and host Pseudomonas syringae were kindly supplied by the Water Institute laboratory of the Gillings School of Public Health at the University of North Carolina at Chapel Hill. Using prepared 18-h growth of P. syringae in 50 ml tryptic soy broth (TSB), bacteriophage phi 6 was propagated by reconstituting with 1 ml of prewarmed (37°C) TSB. Five hundred microliters of reconstituted phi 6 was transferred into 50 ml of fresh TSB with 100 ul of host P. syringae, and incubated at 22°C with gentle agitation (100 rpm) for 18 h. The phi 6 stock titer was ~109 plaque forming units (PFU)/ml.
Bacteria S. aureus was obtained from the American Type Culture Collection (ATCC 12600) and prepared as previously described (27). The S. aureus stock titer was approximately 108 colony forming units (CFU)/ml.
Essential Oil Disinfectants
Four essential oil blends produced and delivered free of charge by EPS Fragrances (Istanbul, Turkey) were examined. These essential oil blends were designed to target viruses, including SARS-CoV-2 and included a proprietary mix of essential oils at various concentrations (Table 1).
Disinfectant 1 (NewAnti FL) was a solution of 5% disinfectant 2 (AntiVir19ED) in 95% ethanol. Disinfectant 2 was undiluted AntiVir19ED. Disinfectant 3 was undiluted Anti-COV19VERS4. Disinfectant 4 was 5% disinfectant 3 (Anti-COV19VERS4) in 95% ethanol.
Disinfectants were provided as either sprays or liquid chemicals and redistributed into 2oz spray bottles for application on surfaces for microbial disinfection.
Surface Inoculation and Sampling
The effectiveness of the four disinfectants was examined on three different surface material: laminate, stainless steel, and ceramic tile. As the laminate surface was more porous than the other two surface material, it was not possible to decontaminate this surface between disinfectant trials; therefore, a new piece of laminate tile was used for each experiment. The stainless steel and ceramic tiles were cleaned and autoclaved between each use.
For each trial run, microbes were applied to the tile surface and allowed to dry for approximately two min. Then spray disinfectants were applied onto experimental surfaces and allowed to air dry. Cultures were collected from control surfaces before the addition of spray disinfectants. CultureSwabs (Becton Dickinson) premoistened with sterile Dey-Engley neutralizer were used to collect samples from each experimental surface. In addition to experimental surfaces, control surfaces were also sampled during the course of the experiment. Two control surfaces were prepared for each tile surface (1) a negative control surface inoculated with phosphate buffered saline, and (2) a non-spiked surface; both surfaces were sampled using the same methods as the experimental surfaces. Samples of bacteria were detected using a standard bacteriological spread plate method (27) and bacteriophage viruses were cultured using United States Environmental Protection Agency (US EPA) method 1602 (28).
Statistical Analysis
Data analysis and graphical representations were created in GraphPad Prism (Version 9). Log10 concentration values were calculated by taking the log10 of the concentration (N) of each microbe (phi 6 and S. aureus) detected at time (t), the end of the experiment, and subtracting the log10 concentration of each microbe respectively at time 0. Total log10 concentrations for each surface material are shown for phi 6 in Figure 1 and for Staphylococcus aureus in Figure 2. A one-way ANOVA (analysis of variance) test was used to determine if there was a difference among mean log10 reductions by tile and Tukey's multiple comparisons test was used to examine the relationship between means. All statistical significance was evaluated at an alpha level of 0.05.
Results
The log10 reductions for each microorganism by disinfectant and tile type are summarized in Figures 1, 2. On laminate tiles, disinfectant 1 reduced bacteriophage phi 6 an average of 2.4 log10 plaque forming units (PFU) and S. aureus 1.5 log10 colony forming units (CFU). The log10 reduction for disinfectant 2 on this surface for S. aureus was also close to two log10 (2.2 log10 CFU); however, the bacteriophages were decreased to a much lower degree at only 1.0 log10 PFU. Disinfectant 3 decreased the S. aureus and phi 6 1.3 and 0.2 log10 CFU and PFU respectively. On laminate tile, disinfectant 4 was the most effective disinfectant against phi 6 with a log10 reduction of 6.5 log10 PFU but the least effective against S. aureus with a 0.9 log10 CFU reduction.
On the stainless-steel surface, disinfectant 1 had the greatest overall effect on S. aureus with a log10 reduction of 3.9 log10 CFU. Disinfectant 1 was also very effective against phi 6 with a reduction of 3.4 log10 PFU. Disinfectants 2 and 3 were not very effective against either microbe with log10 reductions of 2.0 and 1.4 log10 CFU for S. aureus and 0.6 and 1.0 log10 PFU for phi 6 respectively. In contrast to disinfectant 1, disinfectant 4 was the most effective for phi 6 with a reduction of 4.2 log10 PFU but only moderately effective for S. aureus with a reduction of 2.2 log10 CFU.
On the ceramic surface, the bacterial log10 reductions were very similar for all four disinfectants, each falling within the rage of 1.8–2.7 log10 CFU (<1 log10). For the bacteriophage phi 6, disinfectant 4 was the most effective with a log10 reduction of 6.4 log10 PFU, followed by disinfectant 3 with a log10 reduction of 4.2 log10 PFU.
In order to compare the differences between mean log10 reduction across surface type by disinfectant, a one-way ANOVA was conducted followed by a Tukey's multiple Comparison Test (Table 2). Based on this analysis, there were no significant differences between disinfectants 1, 2, 3, or 4 on laminate or ceramic surfaces when disinfecting S. aureus. However, there was difference in the disinfection of S. aureus on stainless steel surfaces between disinfectants 1 and 3, which have average log10 reductions of 3.9 and 1.4 log10 CFU respectively.
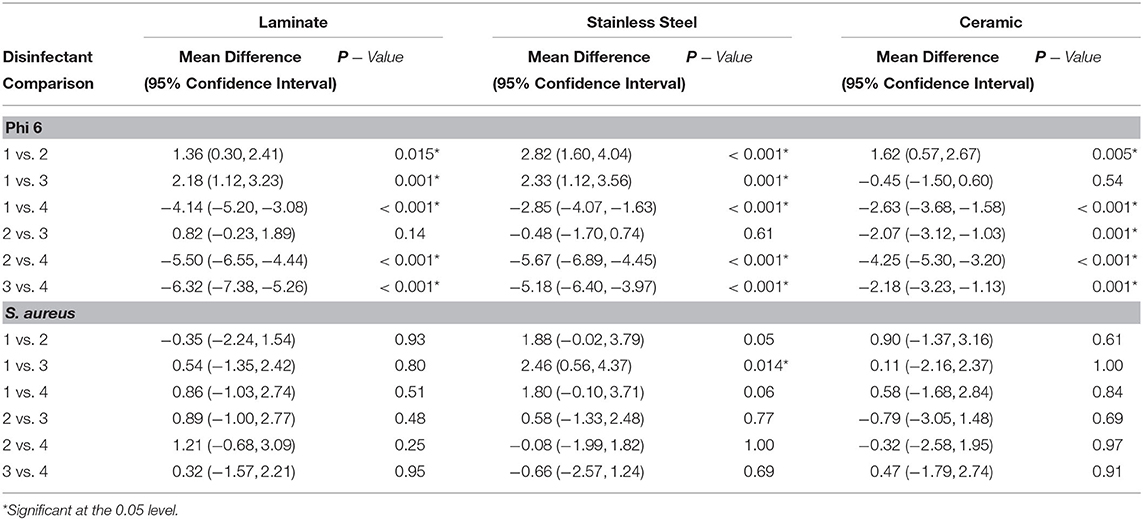
Table 2. Tukey's multiple comparison test of the relationship between log10 reduction means by disinfectant and surface type.
In the comparison of disinfectants used against bacteriophage phi 6, most were statistically significantly different from one another based on mean log10 reduction analysis. Disinfectants that were not different include disinfectant 2 and 3 on laminate and stainless-steel surfaces and disinfectants 1 and 3 on ceramic surfaces.
Discussion
In this study, we found that essential oil disinfectants were able to reduce bacterial and viral microorganisms up to 6 log10 PFU for bacteriophage phi 6 and up to 4 log10 CFU for S. aureus. There was a statistically significant difference by Tukey's Multiple Comparison Test between all disinfectants and disinfectant 4 for phi 6. This result indicates that disinfectant 4 (5% Anti-COV19VERS4 in 95% ethanol) was the most effective for reducing bacteriophage phi 6 on all surfaces considered. For S. aureus, there was not one disinfectant that was clearly the most effective. There was a statistically significant difference between disinfectants 1 and 3 on the stainless steel surface, but this is primary due to the high efficacy of disinfectant 1 on this surface. During the coronavirus pandemic, there has been increased attention to surface disinfection methods, particularly in common areas and highly trafficked surfaces. The application of spray disinfectants, such as those examined in this study, provide a rapid and effective means to reduce bacterial and viral contamination on these surfaces.
Previous studies have determined that human coronaviruses can survive on dry surfaces for up to 9 days (8, 10), and that SARS-CoV-2 in particular is viable on plastic and stainless steel for up to 72 h after aerosol contact (11). Despite this, SARS-CoV-2 is readily inactivated by lipid solvents; and multiple studies have evaluated the efficacy of ethanol at various concentrations (29) against viral agents. In suspension tests with ethanol, concentrations of SARS-CoV has been shown to be reduced >5.5 log10 tissue culture infectious doses (30). On surfaces, including porcelain and ceramic, spray applications ethanol have shown an inverse relationship between log10 reduction and ethanol concentration. A recent study has shown that 95% ethanol was only able to achieve ~2 log10 reductions in infectivity (31), indicating that the blend of essential oils examined in this study is more effective in combination with ethanol than the lipid solvent alone. Although surfaces are no longer considered a high risk fomite for COVID-19 transmission (8), the disinfection of microorganisms on surfaces remains an important consideration for high touch areas in hospitals, waiting rooms, etc. Recently detected and continually evolving variants of SARS-CoV-2, such as the delta variant, may have greater transmissibility. As transmission of SARS-CoV-2 variants on surfaces has not yet been examined and it has been proposed the viral load of the SARS-CoV-2 virus in individuals infected with these strains of the virus is higher than with previous variants (32), it will be important to continue with multilayered control measures and surface cleaning procedures. The use of essential oils as disinfectants and the results presented here provide important context in light of the current coronavirus pandemic. As variants continue to emerge, new technologies and methods of delivering disinfectants are important to preventing the spread of pathogens on surfaces and through contact with fomites. As our results show, the use of dilute essential oil blends on surfaces may be an alternative for high concentration lipid solvents in some situations, as we determined similar log10 reductions during spray application.
Antimicrobial properties of essential oils have been previously evaluated for both bacteria and viruses (33). Despite this, the mechanism of action of essential oil inactivation or disinfection is not fully understood. Although not the primary focus of our pilot study, previous research has suggested that potential mechanisms for viral inactivation with essential oils may be due to either damage to virus particles and the inhibition of virus adsorption to host cells (33). In work with influenza A, an enveloped virus, authors concluded that essential oils did not prevent adsorption of virus to host cells (23, 34); however, in work with herpes simplex virus, a non-enveloped virus, other researchers proposed that the mechanism of action was direct binding to the virus and inhibition of virus adsorption to the host cells (35–37). In our work with essential oil blends, it is clear that inactivation is occurring on surfaces spiked with microorganisms, but it is outside of the scope of this pilot study to elucidate mechanisms of action for the essential oil blends.
Limitations of our study include the use of a bacteriophage instead of the evaluation of a viral pathogen. However, there is evidence that bacteriophage phi 6 is a reliable model for the survival of coronaviruses under various conditions (38–42). Further research is recommended to include additional viruses, such as SARS-CoV-2 and influenza viruses. A second limitation includes the use of only one type of spray application of these disinfectants. It may be that there are other delivery methods that are more effective in applying or distributing these essential oil disinfectants. Our primary goal was to conduct a pilot study to evaluate the efficacy of the disinfectants on microorganisms, but future work should evaluate the delivery method.
Our results suggest that the application of dilute essential oil disinfectants by using a spray delivery device is an effective way to reduce concentrations of viral and bacterial microorganisms on ceramic, stainless steel, and laminate surfaces. Additional studies are needed to evaluate the utility of these sprays in community settings and to optimize the method of delivery in the decontamination of surfaces.
Data Availability Statement
The raw data supporting the conclusions of this article will be made available by the authors, without undue reservation.
Author Contributions
EB wrote the manuscript and conducted the data analysis. MC performed laboratory experiments. HE provided essential oil disinfectants. JB, NB, and AS conceived of the idea and developed the study. All authors have read, reviewed and agreed to the manuscript.
Funding
Funding for this work was provided by discretionary funds at Whitacre College of Engineering Dean's office at Texas Tech University and was supported by EB's discretionary funding in the Department of Public Health at Texas Tech Health Sciences Center.
Conflict of Interest
The authors declare that the research was conducted in the absence of any commercial or financial relationships that could be construed as a potential conflict of interest.
Publisher's Note
All claims expressed in this article are solely those of the authors and do not necessarily represent those of their affiliated organizations, or those of the publisher, the editors and the reviewers. Any product that may be evaluated in this article, or claim that may be made by its manufacturer, is not guaranteed or endorsed by the publisher.
Acknowledgments
We thank Alyssa Western and Miranda Rice for their help in the laboratory experiments.
Abbreviations
ANOVA, analysis of variance; ATCC, American Type Culture Collection; CFU, colony forming units; COVID-19, novel coronavirus 19 disease; PFU, plaque forming units; SARS-CoV-2, Severe Acute Respiratory Syndrome Coronavirus 2; TSB, tryptic soy broth; US EPA, United States Environmental Protection Agency.
References
1. Cadnum JL, Jencson AL, Livingston SH Li DF, Redmond SN, Pearlmutter B, et al. Evaluation of an electrostatic spray disinfectant technology for rapid decontamination of portable equipment and large open areas in the era of SARS-CoV-2. Am J Infect Control. (2020) 48:951–4. doi: 10.1016/j.ajic.2020.06.002
2. Otter JA, Donskey C, Yezli S, Douthwaite S, Goldenberg SD, Weber DJ. Transmission of SARS and MERS coronaviruses and influenza virus in healthcare settings: the possible role of dry surface contamination. J Hosp Infect. (2016) 92:235–50. doi: 10.1016/j.jhin.2015.08.027
3. van Doremalen N, Bushmaker T, Morris DH, Holbrook MG, Gamble A, Williamson BN, et al. Aerosol and surface stability of SARS-CoV-2 as compared with SARS-CoV-1. N Engl J Med. (2020) 382:1564–7. doi: 10.1056/NEJMc2004973
4. Boone SA, Gerba CP. Significance of fomites in the spread of respiratory and enteric viral disease. Appl Environ Microbiol. (2007) 73:1687–96. doi: 10.1128/AEM.02051-06
5. Boone SA, Gerba CP. The occurrence of influenza A virus on household and day care center fomites. J Infect. (2005) 51:103–9. doi: 10.1016/j.jinf.2004.09.011
6. Fong MW, Leung NHL, Xiao J, Chu DKW, Cheng SMS, So HC, et al. Presence of influenza virus on touch surfaces in Kindergartens and primary schools. J Infect Dis. (2020) 222:1329–33. doi: 10.1093/infdis/jiaa114
7. Memish ZA, Almasri M, Assirri A, Al-Shangiti AM, Gray GC, Lednicky JA, et al. Environmental sampling for respiratory pathogens in Jeddah airport during the 2013 Hajj season. Am J Infect Control. (2014) 42:1266–9. doi: 10.1016/j.ajic.2014.07.027
8. Prevention, CfDCa,. Infection Control Guidance for Healthcare Professionals About Coronavirus (COVID-19). Available online at: https://www.cdc.gov/coronavirus/2019-ncov/communication/guidance.html (accessed March 15, 2021).
9. Centers for Disease Control and Prevention. Infection Control Guidance for Healthcare Professionals About Coronavirus (COVID-19).
10. Fischer R, Judson S, Miazgowicz K, Bushmaker T, Prescott J, Munster VJ. Ebola virus stability on surfaces and in fluids in simulated outbreak environments. Emerg Infect Dis. (2015) 21:1243–6. doi: 10.3201/eid2107.150253
11. Piercy TJ, Smither SJ, Steward JA, Eastaugh L, Lever MS. The survival of filoviruses in liquids, on solid substrates and in a dynamic aerosol. J Appl Microbiol. (2010) 109:1531–9. doi: 10.1111/j.1365-2672.2010.04778.x
12. Bibby K, Fischer RJ, Casson LW, Stachler E, Haas CN, Munster VJ. Persistence of ebola virus in sterilized wastewater. Environ Sci Technol Lett. (2015) 2:245–9. doi: 10.1021/acs.estlett.5b00193
13. Adcock NJ, Rice EW, Sivaganesan M, Brown JD, Stallknecht DE, Swayne DE. The use of bacteriophages of the family Cystoviridae as surrogates for H5N1 highly pathogenic avian influenza viruses in persistence and inactivation studies. J Environ Sci Health A Tox Hazard Subst Environ Eng. (2009) 44:1362–6. doi: 10.1080/10934520903217054
14. Guan J, Chan M, VanderZaag A. Inactivation of Avian influenza viruses on porous and non-porous surfaces is enhanced by elevating absolute humidity. Transbound Emerg Dis. (2017) 64:1254–61. doi: 10.1111/tbed.12499
15. Casanova LM, Jeon S, Rutala WA, Weber DJ, Sobsey MD. Effects of air temperature and relative humidity on coronavirus survival on surfaces. Appl Environ Microbiol. (2010) 76:2712–7. doi: 10.1128/AEM.02291-09
16. Fedorenko A, Grinberg M, Orevi T, Kashtan N. Survival of the enveloped bacteriophage Phi6 (a surrogate for SARS-CoV-2) in evaporated saliva microdroplets deposited on glass surfaces. Sci Rep. (2020) 10:22419. doi: 10.1038/s41598-020-79625-z
17. Whitworth C, Mu Y, Houston H, Martinez-Smith M, Noble-Wang J, Coulliette-Salmond A, et al. Persistence of bacteriophage Phi 6 on porous and nonporous surfaces and the potential for its use as an ebola virus or coronavirus surrogate. Appl Environ Microbiol. (2020) 86:e01482–20. doi: 10.1128/AEM.01482-20
18. String GM, White MR, Gute DM, Mühlberger E, Lantagne DS. Selection of a SARS-CoV-2 surrogate for use in surface disinfection efficacy studies with chlorine and antimicrobial surfaces. Environ Sci Technol Lett. (2021) 8:995–1001. doi: 10.1021/acs.estlett.1c00593
19. Tassou CC, Nychas GJ. Inhibition of Salmonella enteritidis by oleuropein in broth and in a model food system. Lett Appl Microbiol. (1995) 20:120–4. doi: 10.1111/j.1472-765X.1995.tb01301.x
20. Tassou CC, Nychas GJE. Inhibition of Staphylococcus aureus by olive phenolics in broth and in a model food system. J Food Prot. (1994) 57:120–4. doi: 10.4315/0362-028X-57.2.120
21. Dai JP, Zhao XF, Zeng J, Wan QY, Yang JC Li WZ, et al. Drug screening for autophagy inhibitors based on the dissociation of Beclin1-Bcl2 complex using BiFC technique and mechanism of eugenol on anti-influenza A virus activity. PLoS One. (2013) 8:e61026. doi: 10.1371/journal.pone.0061026
22. Garozzo A, Timpanaro R, Bisignano B, Furneri PM, Bisignano G, Castro A. In vitro antiviral activity of Melaleuca alternifolia essential oil. Lett Appl Microbiol. (2009) 49:806–8. doi: 10.1111/j.1472-765X.2009.02740.x
23. Garozzo A, Timpanaro R, Stivala A, Bisignano G, Castro A. Activity of Melaleuca alternifolia (tea tree) oil on Influenza virus A/PR/8: study on the mechanism of action. Antiviral Res. (2011) 89:83–8. doi: 10.1016/j.antiviral.2010.11.010
24. Patne TMJ, Tomurke P. Inhalation of essential oils: could be adjuvant therapeutic strategy for COVID-19. Int J Pharm Sci Res. (2020) 11:4095–103. doi: 10.13040/IJPSR.0975-8232
25. Loizzo MR, Saab AM, Tundis R, Statti GA, Menichini F, Lampronti I, et al. Phytochemical analysis and in vitro antiviral activities of the essential oils of seven Lebanon species. Chem Biodivers. (2008) 5:461–70. doi: 10.1002/cbdv.200890045
26. Aydin AD, Altinel F, Erdogmus H, Son CD. Allergen fragrance molecules: a potential relief for COVID-19. BMC Complement Med Ther. (2021) 21:41. doi: 10.1186/s12906-021-03214-4
27. Standard Methods for the Examination of Water and Wastewater. Part 9000: Microbiological Examination. Washington, DC: American Public Health Association (1999).
28. United States Environmental Protection Agency. Method 1602: Male-specific (F+) and Somatic Coliphage in Water by Single Agar Layer (SAL) Procedure. EPA 821-R-01-029. Washington, DC: United States Environmental Protection Agency (2001).
29. Kampf G, Todt D, Pfaender S, Steinmann E. Persistence of coronaviruses on inanimate surfaces and their inactivation with biocidal agents. J Hosp Infect. (2020) 104:246–51. doi: 10.1016/j.jhin.2020.01.022
30. Rabenau HF, Kampf G, Cinatl J, Doerr HW. Efficacy of various disinfectants against SARS coronavirus. J Hosp Infect. (2005) 61:107–11. doi: 10.1016/j.jhin.2004.12.023
31. Meyers C, Kass R, Goldenberg D, Milici J, Alam S, Robison R. Ethanol and isopropanol inactivation of human coronavirus on hard surfaces. J Hosp Infect. (2021) 107:45–9. doi: 10.1016/j.jhin.2020.09.026
32. Onakpoya IJ, Heneghan CJ, Spencer EA, Brassey J, Pluddemann A, Evans DH, et al. SARS-CoV-2 and the role of fomite transmission: a systematic review. F1000Res. (2021) 10:233. doi: 10.12688/f1000research.51590.1
33. Gilling DH, Kitajima M, Torrey JR, Bright KR. Antiviral efficacy and mechanisms of action of oregano essential oil and its primary component carvacrol against murine norovirus. J Appl Microbiol. (2014) 116:1149–63. doi: 10.1111/jam.12453
34. Wu S, Patel KB, Booth LJ, Metcalf JP, Lin HK, Wu W. Protective essential oil attenuates influenza virus infection: an in vitro study in MDCK cells. BMC Complement Altern Med. (2010) 10:69. doi: 10.1186/1472-6882-10-69
35. Reichling J, Koch C, Stahl-Biskup E, Sojka C, Schnitzler P. Virucidal activity of a beta-triketone-rich essential oil of Leptospermum scoparium (manuka oil) against HSV-1 and HSV-2 in cell culture. Planta Med. (2005) 71:1123–7. doi: 10.1055/s-2005-873175
36. Koch C, Reichling J, Schneele J, Schnitzler P. Inhibitory effect of essential oils against herpes simplex virus type 2. Phytomedicine. (2008) 15:71–8. doi: 10.1016/j.phymed.2007.09.003
37. Astani A, Reichling J, Schnitzler P. Screening for antiviral activities of isolated compounds from essential oils. Evid Based Complement Alternat Med. (2011) 2011:253643. doi: 10.1093/ecam/nep187
38. Aquino de, Carvalho N, Stachler EN, Cimabue N, Bibby K. Evaluation of Phi6 persistence and suitability as an enveloped virus surrogate. Environ Sci Technol. (2017) 51:8692–700. doi: 10.1021/acs.est.7b01296
39. Kim K, Jothikumar N, Sen A, Murphy JL, Chellam S. Removal and inactivation of an enveloped virus surrogate by iron conventional coagulation and electrocoagulation. Environ Sci Technol. (2021) 55:2674–83. doi: 10.1021/acs.est.0c07697
40. Ma B, Linden YS, Gundy PM, Gerba CP, Sobsey MD, Linden KG. Inactivation of Coronaviruses and phage Phi6 from irradiation across UVC wavelengths. Environ Sci Technol Lett. (2021) 8:425–30. doi: 10.1021/acs.estlett.1c00178
41. Silverman AI, Boehm AB. Systematic review and meta-analysis of the persistence and disinfection of human coronaviruses and their viral surrogates in water and wastewater. Environ Sci Technol Lett. (2020) 7:544–33. doi: 10.1021/acs.estlett.0c00313
Keywords: coronavirus, SARS-CoV-2, essential oil, surrogate, surface
Citation: Bailey ES, Curcic M, Biros J, Erdogmuş H, Bac N and Sacco A Jr (2021) Essential Oil Disinfectant Efficacy Against SARS-CoV-2 Microbial Surrogates. Front. Public Health 9:783832. doi: 10.3389/fpubh.2021.783832
Received: 27 September 2021; Accepted: 22 November 2021;
Published: 14 December 2021.
Edited by:
Mohiuddin Md. Taimur Khan, Washington State University Tri-Cities, United StatesReviewed by:
Md. Asaduzzaman Shishir, Primeasia University, BangladeshVarsha Rani Gajamer, Darjeeling Government College, India
Kundlik Gadhave, Johns Hopkins University, United States
Copyright © 2021 Bailey, Curcic, Biros, Erdogmuş, Bac and Sacco. This is an open-access article distributed under the terms of the Creative Commons Attribution License (CC BY). The use, distribution or reproduction in other forums is permitted, provided the original author(s) and the copyright owner(s) are credited and that the original publication in this journal is cited, in accordance with accepted academic practice. No use, distribution or reproduction is permitted which does not comply with these terms.
*Correspondence: Emily S. Bailey, ZWJhaWxleUBjYW1wYmVsbC5lZHU=
†Present address: Jnev Biros, Department of Pediatrics, Texas Tech University Health Sciences Center, Lubbock, TX, United States
Emily S. Bailey, Department of Public Health, College of Pharmacy and Health Sciences, Campbell University, Buies Creek, NC, United States