- 1Standardization Research Center of Traditional Chinese Medicine Dispensing, School of Chinese Materia Medica, Beijing University of Chinese Medicine, Beijing, China
- 2Department of Cardiovascular Diseases, Guang'anmen Hospital, China Academy of Chinese Medical Sciences, Beijing, China
Background: The global community has been affected by the coronavirus disease 2019 (COVID-19), which emerged in December 2019. Since then, many studies have been conducted on cardiovascular diseases (CVDs) and COVID-19. The aim of this study was to perform a bibliometric and visual analysis of the published relationship between CVDs and COVID-19.
Methods: 1,890 publications were retrieved from the Web of Science Core Collection database on January 5, 2022. Microsoft Office Excel and CiteSpace were then used to carry out scientometric analysis on the relevant literature according to seven aspects: document type, countries/regions, institutions, authors, journals, references, and keywords.
Results: The research on CVDs and COVID-19 is currently in a period of rapid development, with China, USA, England, and Italy leading the field. There is active cooperation between most countries and institutions. Harvard Medical School stands out among the many institutions not only for the largest number of publications, but also for their high quality. Banerjee A, Solomon SD and Narula J are three representative authors in this field. Frontiers in Cardiovascular Medicine was the journal with the highest number of published studies, and The Lancet was the most cited journal. Two documents with a high degree of significance in this field were identified. Popular research topics in this field are specific diseases, such as acute coronary syndrome and heart failure; pathogenesis related to ACE2, insulin resistance and pericyte; the specific therapeutic drug chloroquine; and clinical characteristics, physical activity, and mental health. ACE2 and NF-κB will be the focus of future research.
Conclusions: This study provides useful information for the research of CVDs and COVID-19, including potential collaborators, popular research topics, and a reference for more extensive and in-depth research in the future.
Introduction
The coronavirus disease 2019 (COVID-19) is caused by the novel Severe Acute Respiratory Syndrome Coronavirus type 2 (SARS-CoV-2). Ever since the first case of COVID-19 was detected in Wuhan, China in December 2019, both the global health system and the whole world have faced severe challenges (1). In addition to Severe Acute Respiratory Syndrome Coronavirus (SARS-CoV) (2) in 2003 and Middle East Respiratory Syndrome Coronavirus (MERS-CoV) (3) in 2012, SARS-CoV-2 is the third high-risk virus to be found in humans. It is an enveloped positive single-stranded RNA virus (4), characterized by low pathogenicity and high transmissibility (5). On March 11, 2020, the World Health Organization (WHO) declared COVID-19 as a global pandemic. As of February 6, 2022, more than 392 million confirmed cases and over 5.7 million deaths have been reported globally (6).
As the COVID-19 pandemic enters its third year, although it is known that the lungs are the main organ involved in COVID-19 infections, SARS-CoV-2 also causes systemic diseases with multiple clinical manifestations (7). The core pathology of COVID-19 is the infection of respiratory cells by SARS-CoV-2, resulting in excessive inflammation and respiratory diseases. The most serious cases involve cytokine storm and acute respiratory distress syndrome (ARDS) (1). Angiotensin-converting enzyme 2 (ACE2) is the host cell receptor for the viral spike protein through which SARS-CoV-2 infects the heart, vascular tissues and circulating cells (8). Specific cardiovascular diseases (CVDs) may occur including acute myocardial injury and myocarditis (9, 10), arrhythmia (11, 12), cardiac fibrosis (13), heart failure (HF) (14, 15), vascular dysfunction (16), and thromboembolic diseases (17). Among the confirmed COVID-19 cases reported by the National Health Commission of China (NHC), some patients were initially admitted to the hospitals due to cardiovascular symptoms (11). Several studies have reported that the presence of CVDs and its risk factors are associated with severe COVID-19 and higher mortality (18–20). In addition, many antiviral drugs can also cause cardiac insufficiency, arrhythmias, or other CVD during COVID-19 treatment (21, 22). The COVID-19 pandemic has led to severe disruptions in health care service around the world, and the global reduction in cardiovascular diagnostic testing had a significant impact on overall CVDs morbidity and mortality (23, 24). Widespread social lockdowns and restructuring of healthcare systems can also lead to less-than-optimal treatment of patients with acute or established CVDs (25).
Bibliometrics is a discipline that studies the distribution structure, quantitative relationships, rules of change and quantitative management of document information using mathematical, statistical and other metrological research methods (26). Bibliometrics differs from traditional literature reviews by allowing for quantitative and qualitative analysis of the literature to assess the current status, trends, and frontiers of research activities (27, 28). In addition, this method compares the contributions of different countries/regions, institutions, journals, and scholars (29, 30). A unique feature of a high-level bibliometric study is that it will provide researchers with frontier areas of research to save time (31). Researchers commonly employ CiteSpace as a bibliometric visualization tool for data analysis and visualization (32, 33). Since the pandemic, researchers around the world have published many studies on CVDs and COVID-19. Previously, a bibliometric analysis on cardiac involvement in COVID-19 had used VOSviewer software for bibliometric analysis (34). In this study, the relevant CVDs and COVID-19 literature contained on the Web of Science Core Collection was reviewed and CiteSpace visualization software was used to perform bibliometric analysis on the relevant literature. Visual knowledge maps were drawn the research statuses were displayed and various popular research topics were identified, which will fill the gaps from previous papers and provide references for future research.
Methods
Data collection
The following search terms were used to retrieve literature from the Web of Science Core Collection (http://apps.webofknowledge.com/) on January 5, 2022: Topic = (“COVID-19” OR “COVID 19” OR “SARS-CoV-2 Infection” OR “Infection, SARS-CoV-2” OR “SARS CoV 2 Infection” OR “SARS-CoV-2 Infections” OR “2019 Novel Coronavirus Disease” OR “2019 Novel Coronavirus Infection” OR “2019-nCoV Disease” OR “2019 nCoV Disease” OR “2019-nCoV Diseases” OR “Disease, 2019-nCoV” OR “COVID-19 Virus Infection” OR “COVID 19 Virus Infection” OR “COVID-19 Virus Infections” OR “Infection, COVID-19 Virus” OR “Virus Infection, COVID-19” OR “Coronavirus Disease 2019” OR “Disease 2019, Coronavirus” OR “Coronavirus Disease-19” OR “Coronavirus Disease 19” OR “Severe Acute Respiratory Syndrome Coronavirus 2 Infection” OR “SARS Coronavirus 2 Infection” OR “COVID-19 Virus Disease” OR “COVID 19 Virus Disease” OR “COVID-19 Virus Diseases” OR “Disease, COVID-19 Virus” OR “Virus Disease, COVID-19” OR “2019-nCoV Infection” OR “2019 nCoV Infection” OR “2019-nCoV Infections” OR “Infection, 2019-nCoV” OR “COVID19” OR “COVID-19 Pandemic” OR “COVID 19 Pandemic” OR “Pandemic, COVID-19” OR “COVID-19 Pandemics”) AND (“Cardiovascular Diseases” OR “Cardiovascular Disease” OR “Disease, Cardiovascular” OR “Diseases, Cardiovascular”) AND Language = English. The retrieval time was set from 2020 to 2022. The retrieved data were collected within 1 day to avoid any potential deviations due to daily updates. A total of 1,890 publications were retrieved, of which 221 irrelevant publications were excluded, including editorial materials, early access, letters, meeting abstracts, book chapters, corrections, expression of concern, new items, proceedings papers, retracted publications, and retractions. A total of 1,669 publications were exported in the form of full records with cited references, saved as plain text files, and stored in download_.txt format. Figure 1 shows the flowchart for literature selection.
Data analysis
Microsoft Office Excel 2019 and CiteSpace (5.8.R3) were utilized to analyze all 1,669 publications and Microsoft Office Excel 2019 was used to construct the tables. CiteSpace (a web-based Java application) is a document visualization analysis software that was gradually developed for bibliometrics and data visualization (31). In this study, CiteSpace was employed to construct visual knowledge maps for the distribution of countries/regions, institutions, authors, journals, references, and keywords (35). The parameters of CiteSpace were set as follows: time slice (2020–2022), years per slice (1), term source (entire selection), node type (chosen one at a time), and selection criteria (top 100% objects). Other parameters were left at the default settings. Modularity is an index that is used to evaluate network modularity. The greater the modularity value of a network, the better the clustering of the network. The value space for Q was (0, 1), with Q > 0.3 indicating that the obtained network community structure is significant (36). The silhouette was evaluated by measuring the network homogeneity. The closer the silhouette value is to 1, the higher the network homogeneity. A clustering result with a value of 0.7 is highly reliable, while a result above 0.5 can be considered reasonable (36).
Results
Number of publications and distribution type
The number of publications within a period reflects the research development speed and trends in a discipline (37). A total of 1,890 publications related to CVDs and COVID-19 were identified in the Web of Science Core Collection database, reflecting the rapid development in CVDs and COVID-19 research during this period. Table 1 shows the types of publications that were analyzed, where 61.47% (1,026) of the documents were articles and 38.53% (643) were review articles. Among the publications, there were a total of 33,788 articles that were cited 54,277 times, with an h-index of 86, and an average per item of 32.52.
Distribution of countries/regions and institutions
A total of 1,669 publications were published from 111 different countries/regions and 3,715 institutions. As shown in Table 2, the greatest number of publications came from USA (496, 29.72%), followed by China (249, 14.92%), Italy (234, 14.02%) and England (175, 10.49%). These four countries accounted for more than half of the total reports, which shows that USA, China, Italy, and England are the leading countries in CVDs and COVID-19 research.
In CiteSpace, the cooperative scientific research networks of the different countries/regions, institutions and authors correspond to the macro, meso and micro cooperative networks, respectively. The visual knowledge maps can provide information to aid in the identification of influential research teams and potential collaborators, thus helping researchers establish cooperative relationships (38). As illustrated in Figures 2, 3, each circle represents a country/institution, and the size of the circle indicates the number of publications output by the country/institution. The lines between the circles denote cooperation between countries/institutions, with wider lines, closer cooperation. There was active cooperation between many countries and institutions, such as USA, China, India, Australia, Brazil, Harvard Medical School, Wuhan University, Huazhong University of Science and Technology, University College London, Zhejiang University, University of Sydney, and Milan Bicocca University.
In CiteSpace, the nodes with betweenness centrality > 0.1 are the key points (39). CiteSpace uses betweenness centrality to find and measure the importance of documents. A purple circle highlights such documents (or authors, journals, countries, and institutions) and indicates that the node betweenness centrality is > 0.1. Publications with high betweenness centrality are usually key hubs connecting two different fields, also known as turning points in CiteSpace (32). An analysis of the country distribution of these publications shows that Australia, England, and Germany have the highest betweenness centrality (Table 2), but none of them exceeded 0.1, indicating that influential countries have not yet been formed in this field. An analysis of the distribution of research institutions shows that Harvard Medical School is the most prominent research institution for co-authored papers, not only for the largest number of articles written in collaboration but also for the highest betweenness centrality (0.15), revealing that Harvard Medical School is highly outstanding in CVDs and COVID-19 research, both quantitatively and qualitatively.
Distribution of authors
A total of 12,823 authors were involved in the publication of literature on CVDs and COVID-19 research. From Table 3, we can see that Banerjee A and Narula J's betweenness centrality is 0.02, Solomon SD's betweenness centrality is 0.01, and other authors' betweenness centrality is 0, which indicates that there are no influential authors in CVDs and COVID-19 research. The reason for this situation is probably due to the short 2-year time span.
Figure 4 shows that there is a cooperative network between different authors. Each node represents an author, the connections represent the existence of a cooperative relationship between the authors, the color of the connections indicates the first instance of cooperation, and the thickness of the connection represents the intensity of cooperation between authors. As shown in Figure 4, Banerjee A, Narula J, Sliwa K, Pablo P, and Harry H worked closely together. Yonetsu T worked closely with Matsue Y, Kohsaka S, Takuya K, and Kitai T. In addition, Solomon SD, Leon A, Orly V, Stefan DA, and Marco M worked closely together.
Distribution of journals and co-cited journals
Figure 5 shows the network visualization maps for citation analysis of journals that are active in CVD and COVID-19 research. We found that 1,699 publications related to CVDs and COVID-19 research were published in 737 journals. Frontiers in Cardiovascular Medicine (33, 1.98%) had the highest number of publications, followed by Journal of Clinical Medicine (31, 1.86%). Among the top 10 journals, Journal of the American College of Cardiology (24.09) had the highest impact factor (IF).
Furthermore, Table 4 shows that 70% of journals belong to Q1. The influence of a journal is determined by the number of times the journal is co-cited, which reflects whether the journal has significant influence in a particular research field (40). Among the top 10 co-cited journals, three journals have been cited more than 1,000 times. As shown in Table 4, the journal with the highest number of citations is The Lancet (1,190), followed by New England Journal of Medicine (1,083). According to the 2020 Journal citation report (JCR), almost all of the co-cited journals in the top 10 journals were in the Q1 region, except for Plos One.
Co-cited references
Co-citation analysis involves tracking two or more references that are cited in the same literature (41). By analyzing the clusters and turning points in the co-citation network, the knowledge structure of a research area and its transformations can be revealed (42). From the 389 co-cited references retrieved, the top 10 co-cited references are shown in Table 5, of which “Clinical course and risk factors for mortality of adult inpatients with COVID-19 in Wuhan, China: a retrospective cohort study” (43) was the most frequently cited (523).
Figure 6 shows the co-citation network for CVDs and COVID-19 research. Two nodes can be clearly seen with a purple circle in the network. The betweenness centrality of “Clinical course and risk factors for mortality of adult inpatients with COVID-19 in Wuhan, China: a retrospective cohort study” is 0.16 (43). That paper reviewed the relevant literature and it was published online in the Lancet in March 2020. The study presented details of 191 laboratory-confirmed COVID-19 patients admitted to Wuhan Jinyintan Hospital and Wuhan Pulmonary Hospital before January 31, 2020. It was the largest retrospective cohort study conducted at that time among COVID-19 patients who experienced definitive symptoms (43). The results showed that the potential risk factors for early identification of patients with poor prognosis included old age, high sequential organ failure assessment (SOFA) score and D-dimer > 1 μg/L (43). Prolonged viral shedding provides a theoretical basis for future isolation of infected patients and optimal antiviral interventions (43). The study found, for the first time, that patients had developed sepsis without bacterial infection. It was revealed that the median time for detoxification of COVID-19 in surviving patients was 20 days, and the longest duration of virus detoxification was 37 days (43). This also partly explains why some patients have nucleic acid reactivation after discharge.
From the second node, the betweenness centrality of the article “Presenting Characteristics, Comorbidities, and outcomes among 5,700 patients hospitalized with COVID-19 in the New York City Area” is 0.19 (52). That article was published in JAMA-Journal of the American Medical Association in April 2020 and it was the first large-scale case study in USA to include COVID-19 inpatients (52). By analyzing the characteristics and early results of 5,700 inpatients with COVID-19 in New York, it was found that the most common complications were hypertension, obesity, and diabetes (52).
Research hotspots and frontiers analysis
Research hotspots analysis
Keywords are the core and essence of a paper. Co-word analysis uses the co-occurrence of word pairs or noun phrases in a collection to determine the relationships between topics in the discipline represented by the collection (53). Figure 7 displays the co-occurrence analysis results for keywords in CVD and COVID-19 research. A network was obtained with 172 nodes, 753 connections and a density of 0.0512, Modularity Q = 0.5234, and Silhouette S = 0.7579. After running the software, synonymous keywords were merged to obtain the top 20 keyword co-occurrence networks in the research literature, and the results are shown in Table 6. For example, COVID 19 was merged with coronavirus disease 2019 and coronavirus disease 2019 (COVID-19) to form COVID-19; and ace2, angiotensin converting enzyme 2, and angiotensin-converting enzyme 2 were merged into ACE2. Besides cardiovascular disease (441) and COVID-19 (253), other keywords with high frequency in this study include ACE2 (192), mortality (122), risk (118), coronavirus (111), infection (110), Wuhan (108), pneumonia (106), and risk factor (102). These keywords reflect the popular research hotspots in this field. As shown in Table 6, the specific diseases involved include cardiovascular disease, COVID-19, pneumonia, heart failure, myocardial infarction, and acute respiratory syndrome.
Based on keywords co-occurrence analysis, clusters can be formed in the network map to summarize the research hotspots and obtain the basic knowledge structure. Figure 8 shows nine clusters of keywords, with each cluster composed of multiple closely related words. These nine clusters are #0 ACE2, #1 acute coronary syndrome,#2 clinical characteristics, #3 insulin resistance, #4 physical activity, #5 heart failure, #6 pericyte, #7 chloroquine, and #8 mental health.
Identification of research frontiers
The burst detection provided by CiteSpace can extract emergent words among subject words in the literature to clearly show the research frontiers and development trends of a certain discipline (54). In this study, keywords burst detection was conducted based on the keywords co-occurrence network, and the top 20 keywords with the strongest citation bursts in CVDs and COVID-19 research were reported. As illustrated in Figure 9, the blue line denotes the time axis and the red segment on the blue time axis displays the burst detection, indicating the start year, end year, and burst duration. The top keywords with the strongest citation bursts can reflect emerging academic trends and new hotspots, predict research frontiers, and explore potential popular topics in CVDs and COVID-19 research (55). Notably, “functional receptor” had the strongest citation bursts, with a maximum burst strength of 6.02, followed by “pathogenesis” and “respiratory syndrome” at 4.18 and 3.65, respectively. The next 17 top keywords with the strongest citation bursts were: angiotensin converting enzyme 2, inhibition, chloroquine, myocarditis, model, blockade, SARS-CoV, MERS-CoV, c reactive protein, severity, death, coronary artery disease, NF-κB, endothelial cell, cancer, admission, and body mass index.
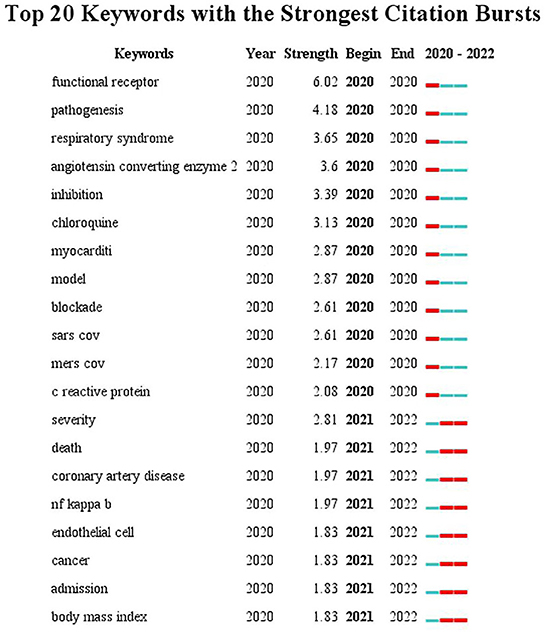
Figure 9. Top 20 Keywords with the Strongest Citation Bursts in the studies of CVDs and COVID-19 research.
Discussion
General information
Given the number of publications and distribution types in CVDs and COVID-19 research, the results show that there is greater on clinical practice and specific scientific research. However, in the wake of the COVID-19 pandemic, global attention is more focused on the impact of the disease on public health. Various countries have invested a lot of manpower and material resources to study the disease and address the impacts. Therefore, many high-quality studies have been published in a short period of time.
By analyzing the results of countries/regions and institutions, it was found that USA, China, Italy, and England are the countries with the largest number of publications in this field. In fact, this finding is related to the outbreak of the pandemic starting in Wuhan, China, and then spreading around the world, with England, Italy, USA, and other European countries becoming the hardest hit areas. America and European countries have developed economies, a high level of medical technology, and strong data management and academic systems. These factors have contributed to the high number of relevant studies from these countries. In addition, through the visual knowledge maps in Figures 2, 3, we can clearly see that the links and cooperation between various countries and institutions are relatively close, which is conducive to creating global strength to solve the COVID-19 pandemic and the problems it brings.
The visual analysis of institutions reveals that Harvard Medical School not only ranked first in terms of the number of published papers, but the quality of their CVDs and COVID-19 research was also very high. In fact, according to the relevant literature, Harvard Medical School was also investigating the relationship between CVDs and COVID-19, including summarizing the potential impacts of coronavirus on the cardiovascular system (55). The researchers at Harvard Medical School believe that COVID-19 is associated with a high inflammatory burden, and they are working to find specific vaccines and antiviral drugs against SARS-CoV-2 (56). Their specific projects include: participating in a study comparing the clinical status of patients with moderate COVID-19 on day 11 treated with Remdesivir vs. standard therapy (57); explaining why COVID-19 disproportionately affects the elderly from the perspective of molecular differences and examining treatment methods to improve the survival of the elderly (58); pharmacoepidemiologic studies to clarify whether renin-angiotensin system (RAS) inhibition mediates the relationship between CVDs and COVID-19 mortality (59); and an investigation of the role of cardiac injury and HF in COVID-19, its pathogenesis and potential therapeutic implications (60). Longitudinal trends in hospitalizations for acute cardiovascular disease in the tertiary health care system were studied and it was found that in the first phase of the COVID-19 pandemic, the rate of hospitalizations for acute cardiovascular disease decreased significantly and the length of hospital stay for hospitalized patients was shortened (61). These data confirm that acute care for CVDs may be delayed or shortened during the COVID-19 pandemic (61). A multicentre, observational cohort study evaluating the frequency, risk factors, prevention, and management patterns of arterial and venous thromboembolic disease in patients with COVID-19 concluded that despite high thromboprophylaxis use, major arterial or venous thromboembolism, major adverse cardiovascular events and symptomatic venous thromboembolism were more common in patients with COVID-19, especially in the intensive care setting (62). These have been important questions for CVDs patients and physicians since the beginning of the COVID-19 pandemic. The publication of these documents will help people better understand and solve the current problems.
The evaluation criteria for core authors include the number of published documents, the total citations, and h-index (63). Analysis of the core authors is helpful for exploring the distribution of the publications. Based on an analysis of the relevant literature, Professor Banerjee A from the University of London, UK is the author with the most frequent occurrence in this field. Banerjee A's main research direction is the clinical research of COVID-19 in conjunction with CVDs, including: management of patients with CVDs diagnosed or suspected of COVID-19 under limited resource conditions (64); description of cardiovascular manifestations and cardiovascular risk factors in hospitalized COVID-19 patients (65); modeling studies to estimate the number of individuals at increased risk of severe COVID-19 due to underlying health conditions globally, regionally and nationally during 2020 (66); a retrospective cohort study comparing the rate of organ-specific dysfunction in COVID-19 patients after discharge with a matched control group from the general population (67); assessing the impact of the COVID-19 pandemic on cancer care services and overall excess deaths among cancer patients (68); monitoring hospital CVDs presentation, diagnosis, and treatment activities during the COVID-19 pandemic to understand indirect effects (69); using electronic health records for primary care in England to investigate the prevalence, incidence, and outcomes of a range of specific CVDs among homeless people (70); as well as population-wide studies of COVID-19 and CVDs (71).
Professor Solomon SD from Harvard University is the author with the highest number of citations. Solomon SD mainly focuses on researching the mechanism of the disease, including some clinical studies. His specific studies include: the potential effects of coronavirus on the cardiovascular system (56); the cardiovascular effects of COVID-19 and influenza infection (72); description of the role of cardiac injury and HF in COVID-19, its pathogenesis and potential therapeutic significance (60); description of the impact of influenza vaccination on cardiovascular morbidity and mortality among COVID-19 patients (73) and longitudinal trends in tertiary health system hospitalizations for acute cardiovascular disease during the COVID-19 pandemic (61).
Professor Narula J from the Icahn School of Medicine at Mount Sinai has the highest h-index. Narula J's research directions are: clinical research, discussion of policy guidelines, and research on disease mechanisms. His specific studies include the incidence and impact of myocardial injury in patients hospitalized with COVID-19 infection (74), the international impact of COVID-19 on cardiac diagnosis (75), and the impact on cardiovascular testing in USA and the rest of the world (76). In addition, Narula J also elaborated on the clinical significance of the interactions between SARS-Cov2 and the renin-angiotensin system (77), and he conducted a special workshop on coronavirus and cardiometabolic syndrome (78).
Visualized knowledge maps can provide information on influential research teams and potential collaborators, and help researchers build collaborative relationships (38). In the context of the COVID-19 pandemic, these representative authors have made important contributions to the knowledge base and discussion of theoretical mechanisms, clinical case studies, and their impact on various countries and regions. These authors have a strong academic reputation in this field.
According to the top 10 journals shown in Table 4, the journal with the most articles on CVDs and COVID-19 is Frontiers in Cardiovascular Medicine, followed by Journal of Clinical Medicine and International Journal of Environmental Research and Public Health. According to the journal ranking, the main research focus in this field is currently the specific clinical problems and public health problems involved since the outbreak of COVID-19, which will also be the key research direction in this field in the future. As for co-cited journals, The Lancet was the most cited journal, followed by New England Journal of Medicine and the Journal of the American Medical Association. Analyzing the distribution of publication sources can be helpful for revealing the core journals. The co-cited journals are from high-impact journals, indicating that CVDs and COVID-19 research is highly valued in the global academic community.
Among the top 10 co-cited references, 8 articles were about the clinical characteristics and clinical course of COVID-19, which shows that people in this field are paying more attention to the actual clinical situations of patients, which is also in line with the specific situation we are currently facing. In addition, we also obtained two articles with betweenness centrality > 0.1. These two documents (43, 52) can be considered as landmark events in this field and they have far-reaching impact on the development of this field. In fact, these two articles have been cited more than 2,000 times, of which “Clinical course and risk factors for mortality of adult inpatients with COVID-19 in Wuhan, China: a retrospective cohort study” (43), ranked fifth in the world in 2020, with a cumulative citation frequency of 4,881.
The hotspots and frontiers
The hotspots in CVDs and COVID-19
Based on the results of keyword clustering and the top 20 keywords with the strongest citations, the current research hotspots in CVDs and COVID-19 are indicated by these nine clusters. From these nine clusters, we can see that the specific diseases involved in the basic knowledge structure for this field were #1 acute coronary syndrome and #5 heart failure. The pathogenesis of the disease was #0 ACE2, #3 insulin resistance, and #6 pericyte. The specific therapeutic drugs were #7 chloroquine and #2 clinical characteristics were #4 physical activity and #8 mental health. #0 ACE2 was the largest cluster and the main research hotspot for CVDs and COVID-19 research. In the next sections, we will analyze the content of these clusters in detail.
First, the specific disease involved was #1 acute coronary syndrome. According to the relevant literature, patients with acute coronary syndrome (ACS) infected with SARS-CoV-2 were generally in serious condition, with high mortality and poor prognosis (79). During the COVID-19 pandemic, the European Society of Cardiology Guidelines for the Diagnosis and Management of Cardiovascular Disease also noted that COVID-19 inpatients often showed signs of myocardial damage upon admission, possibly because many ACS patients did not receive medical care and hospitalization for fear of contracting COVID-19. In addition, several studies have suggested that plaque instability and supply-demand imbalance were key causes of COVID-19 induced ACS (80). #5 heart failure was identified as a risk factor for a more severe course of COVID-19 and increased mortality (80). In particular, acute HF may complicate the clinical course of COVID-19, especially in severe cases. Patients with chronic HF may be at higher risk for COVID-19 due to their older age, multiple comorbidities, and a significantly higher risk of adverse outcomes. A meta-analysis by Sentongo et al. concluded that COVID-19 patients with HF had a higher risk of death compared to those with coexisting conditions (81). Tomasoni et al. believe that patients with heart failure are more susceptible to COVID-19 and, once infected, have a more severe clinical course (60). Studies have shown that at least 10% of hospitalized COVID-19 patients developed HF and myocardial damage, and the proportion can be 25–35% or higher when critical patients or those with heart disease were considered (60).
Second, specific disease mechanisms of COVID-19 include #0 ACE2, which is a membrane-bound aminopeptidase that was discovered in 2000 (82, 83). ACE2 is a key molecular target for the occurrence and development of COVID-19. The surface spike protein of SARS-CoV-2 binds to ACE2 and enters human cells (4). On the one hand, it will cause a series of pathological reactions that support the expression and replication of SARS-CoV-2 and destroy the human body (84). On the other hand, by lowering the level of ACE2, the ACE2/Ang (1–7)/Mas receptor pathway will be inhibited, breaking the balance of renin angiotensin aldosterone system (RAAS), which is not conducive to the maintenance of hemodynamic stability and normal cardiorenal function, and may induce acute myocardial injury, ACS, arrhythmia, and HF, leading to increased mortality of COVID-19 patients (45, 85). Angiotensin converting enzyme inhibitor (ACEI) and angiotensin receptor antagonist (ARB) not only inhibit the classical RAAS pathway, but they also up-regulate the level of ACE2. Therefore, a large amount of pharmacoepidemiologic evidence is needed to support the use of ACEI/ARB during COVID-19 prevention and control (59, 77, 86). Gender and age differences in ACE2 gene expression and immune responses have also been suggested to be important reasons for reduced susceptibility to COVID-19 infection and improved survival in women (87). #3 insulin resistance can lead to neurodegeneration, HF and viral infection (88). Insulin resistance is an important pathophysiological cause of poor prognosis in COVID-19 patients (89). Studies have shown increased coagulopathy and ACE2 expression in adipose cells and pulmonary adipose fibroblasts in insulin-resistant patients. This process enhances SARS-CoV-2 binding and cell uptake and drives a high immune response, leading to pulmonary fibrosis and multi-organ system failure (90–92). #6 cardiac pericytes highly express ACE2, and therefore may be a direct target of SARS-CoV-2 (93). Studies have shown that pericytes may be a highly infectious cell population of SARS-CoV-2. They may promote the imbalance between ANGPT1/2 and TIE2, disrupt the integrity of the vascular barrier and increase vascular permeability, leading to endothelial dysfunction and participation in the occurrence and development of hypertension (93). They also induce dysfunction of myocardial microcirculation (94, 95). Pericytes play an important role in the microvascular system, and infection of pericytes may impair the ability of the myocardial supply to meet metabolic needs (96). Patients with underlying CVDs have higher ACE2 expression levels in pericytes and they experience more severe disease (95).
A specific drug involved in this study is #7 chloroquine. The antimalarial drugs chloroquine and hydroxychloroquine were originally authorized by the FDA for emergency use (EUA) and was used in many COVID-19 treatment regimens (96, 97). The mechanism of this drug is that it can induce antiviral activity against many RNA viruses, including SARS and SARS-CoV-2, by increasing endosomal pH and interfering with glycosylation processes (98). At the time of the COVID-19 outbreak, a clinical study demonstrated the clinical benefits of chloroquine in patients with COVID-19 compared to control treatments (99). However, after more clinical studies, it was found that chloroquine lacks clinical efficacy and has obvious side effects, especially adverse cardiac events (100). The FDA withdrew the EUA on May 28, 2021 (101). Hence, this cluster has attracted extensive attention of scholars and is a much-researched topic.
In addition, there are #2 clinical characteristics that describe the clinical characteristics of different types of COVID-19 patients from different regions, different comorbidities, different age groups, vaccinated with different types of vaccines or unvaccinated. These characteristics are of great value for early intervention and later improvement of COVID-19 treatment plans. For example, Huang C et al. introduced the epidemiological, clinical, laboratory diagnostic and imaging characteristics, treatment, and clinical prognosis of 41 COVID-19 patients (45). Guan WJ et al. systematically collected 1,099 laboratory-confirmed COVID-19 cases from 552 hospitals in 30 provinces from December 2019 to January 29, 2020, and described the clinical characteristics of all cases in detail (102). Wang D et al. described in detail the epidemiological, demographic, clinical, laboratory, and radiological characteristics and treatment of 138 hospitalized COVID-19 patients (11). Chen N et al. analyzed epidemiological, demographic, clinical, radiographic, and laboratory data from nine COVID-19 cases and reported the clinical outcomes at follow-up (48).
As for #4 physical activity, it is without doubt that the COVID-19 pandemic has changed our way of life, and the reduction in physical activity due to lockdown measures may also lead to failure to control cardiovascular risk factors (103). Therefore, experts suggest that people should engage more frequently in physical activities, whether at home or in an outdoor area, which not only improves people's level of happiness, but also reduces cardiovascular risk factors, such as maintaining physical activity to avoid venous thromboembolism (104). Studies have shown that physical activity trackers can significantly increase physical activity and may be a useful auxiliary tool for promoting a healthy lifestyle (105).
#8 mental health studies have shown that COVID-19 lockdowns cause significant psychological effects on people, including: greater psychological stress, post-traumatic stress disorder (PTSD) symptoms, depressive symptoms, more severe anxiety, insomnia, and irritability (106). Stress caused by COVID-19, economic depression and isolation are also likely to lead to a decline in mental health. Furthermore, lower socioeconomic status was associated with an increased risk of CVDs mortality and CVDs risk factors (107).
The frontiers in CVDs and COVID-19 research
Based on keyword emergence in the literature, we speculate that ACE2 and NF-κB are the research frontiers and potential hotspots in this field. ACE2 is a functional receptor of SARS-CoV-2 (86). SARS-CoV-2 uses ACE2 as a functional receptor to enter human cells (108), causing the endocytosis of the ligand/receptor complex, inducing fusion between the virus and host cells, and then ACE2 is degraded in the cells. NF-κB is a complex protein system that exists in the cytoplasm. NF-κB signaling pathway is a typical pro-inflammatory pathway that plays a key role in the functions of the immune system and mediates a variety of cellular processes, including immune response, inflammation, cell proliferation, and cell survival (109, 110). Studies have shown that strategies can be developed to mitigate the cytokine storm and reduce the pathology of severe COVID-19 by focusing on how the NF-κB signaling pathway modulates the inflammatory response (111). Furthermore, identifying potential therapeutic targets related to the NF-κB signaling pathway could help control the more severe spectrum and mortality associated with the pandemic (112). A study by Hamid T et al. showed that chronic activation of NF-κB can lead to vascular disturbance and cardiomyocyte remodeling in patients with metabolic syndrome, which predisposes them to cardiac complications (113). NF-κB signaling pathway also plays an important role in COVID-19 drug treatments. Studies have shown that remdesivir reduces cytokine storm and severe diseases by reducing dsRNA-related induction of the NF-κB signaling pathway (114). Hydroxychloroquine blocks NF-κB signaling pathway by reducing levels of TNF-α, TNF-1β, IgG, and IFN-γ (115). Monoclonal antibodies such as Infliximab and Adalimumab inhibit atypical activation of NF-κB pathway by reducing TNF-α levels, which has the potential to relieve symptoms in critically ill patients with COVID-19 (116). In summary, inhibition of NF-κB pathway has a potential therapeutic role in alleviating severe forms of COVID-19. NF-κB inhibition may be one of the mechanisms of action of currently effective in anti-COVID-19 drugs. More work is needed to identify direct NF-κB inhibition as a treatment for severe forms of this disease (112). Therefore, we believe that ACE2 and NF-κB will be the focus of future research.
Limitations
This study has some limitations. First, the analysis of this study is based on articles from the Web of Science Core Collection database, which is the most well-known database of scientific publications on many research topics, but other databases such as PubMed, Scopus may provide broader coverage. Second, this article only includes English articles, which reduces the number of articles that were retrieved. Finally, the literature on CVDs and COVID-19 is overwhelming. With the rapid updating of research hotspots and frontiers, we may miss some research hotspots. However, visual analysis based on literature lays a foundation for understanding the research topics and frontiers in CVDs and COVID-19 research.
Conclusion
This study provides useful information for the research of CVDs and COVID-19, including potential collaborators, popular research topics, and a reference for more extensive and in-depth research in the future. Currently, research on CVDs and COVID-19 is in a period of rapid development, which indicates that this field has attracted more and more attention. Our findings are a summary of the current state of CVDs and COVID-19 and have important implications for future research direction.
Data availability statement
The raw data supporting the conclusions of this article will be made available by the authors, without undue reservation.
Author contributions
NW and YX designed the study and wrote the manuscript. HW, QJ, and XS collected the data. HW and XZ re-examined the data. NW, NZ, and YL analyzed the data. HZ and YH reviewed and revised the manuscript. All authors contributed to the article and approved the submitted version.
Funding
This study was supported by sample study on toxicity effect evaluation method of traditional Chinese patent medicine containing toxic components (2020-ZXFZJJ-072).
Acknowledgments
The authors acknowledge Beijing University of Chinese Medicine for their support of this work.
Conflict of interest
The authors declare that the research was conducted in the absence of any commercial or financial relationships that could be construed as a potential conflict of interest.
Publisher's note
All claims expressed in this article are solely those of the authors and do not necessarily represent those of their affiliated organizations, or those of the publisher, the editors and the reviewers. Any product that may be evaluated in this article, or claim that may be made by its manufacturer, is not guaranteed or endorsed by the publisher.
References
1. Zhu N, Zhang D, Wang W, Li X, Yang B, Song J, et al. A novel coronavirus from patients with Pneumonia in China, 2019. N Engl J Med. (2020) 382:727–33. doi: 10.1056/NEJMoa2001017
2. Drosten C, Günther S, Preiser W, van der Werf S, Brodt HR, Becker S, et al. Identification of a novel coronavirus in patients with severe acute respiratory syndrome. N Engl J Med. (2003) 348:1967–76. doi: 10.1056/NEJMoa030747
3. Zaki AM, van Boheemen S, Bestebroer TM, Osterhaus AD, Fouchier RA. Isolation of a novel coronavirus from a man with pneumonia in Saudi Arabia. N Engl J Med. (2012) 367:1814–20. doi: 10.1056/NEJMoa1211721
4. Zhou P, Yang XL, Wang XG, Hu B, Zhang L, Zhang W, et al. A pneumonia outbreak associated with a new coronavirus of probable bat origin. Nature. (2020) 579:270–3. doi: 10.1038/s41586-020-2012-7
5. Wu Y, Ho W, Huang Y, Jin DY Li S, Liu SL, et al. SARS-CoV-2 is an appropriate name for the new coronavirus. Lancet. (2020) 395:949–50. doi: 10.1016/S0140-6736(20)30557-2
6. World Health Organization. E. coli. (2022). Available online at: https://www.who.int/publications/m/item/weekly-epidemiological-update-on-covid-19-8-february-2022 (accessed February 8, 2022).
7. Wang C, Horby PW, Hayden FG, Gao GF. A novel coronavirus outbreak of global health concern. Lancet. (2020) 395:470–3. doi: 10.1016/S0140-6736(20)30185-9
8. Guzik TJ, Mohiddin SA, Dimarco A, Patel V, Savvatis K, Marelli-Berg FM, et al. COVID-19 and the cardiovascular system: implications for risk assessment, diagnosis, and treatment options. Cardiovasc Res. (2020) 116:1666–87. doi: 10.1093/cvr/cvaa106
9. Beşler MS, Arslan H. Acute myocarditis associated with COVID-19 infection. Am J Emerg Med. (2020) 38:2489.e1. doi: 10.1016/j.ajem.2020.05.100
10. Kotecha T, Knight DS, Razvi Y, Kumar K, Vimalesvaran K, Thornton G, et al. Patterns of myocardial injury in recovered troponin-positive COVID-19 patients assessed by cardiovascular magnetic resonance. Eur Heart J. (2021) 42:1866–78. doi: 10.1093/eurheartj/ehab075
11. Wang D, Hu B, Hu C, Zhu F, Liu X, Zhang J, et al. Clinical characteristics of 138 hospitalized patients with 2019 novel coronavirus-infected pneumonia In Wuhan, China. JAMA. (2020) 323:1061–9. doi: 10.1001/jama.2020.1585
12. Giustino G, Pinney SP, Lala A, Reddy VY, Johnston-Cox HA, Mechanick JI, et al. Coronavirus and cardiovascular disease, myocardial injury, and arrhythmia: JACC focus seminar. J Am Coll Cardiol. (2020) 76:2011–23. doi: 10.1016/j.jacc.2020.08.059
13. Jagia P, Ojha V, Naik N, Sharma S. Myocardial fibrosis detected by cardiovascular magnetic resonance in absence of myocardial oedema in a patient recovered from COVID-19. BMJ Case Rep. (2020) 13:e240193. doi: 10.1136/bcr-2020-240193
14. Chen T, Wu D, Chen H, Yan W, Yang D, Chen G, et al. Clinical characteristics of 113 deceased patients with coronavirus disease 2019: retrospective study. BMJ. (2020) 368:m1091. doi: 10.1136/bmj.m1091
15. Freaney PM, Shah SJ, Khan SS. COVID-19 and heart failure with preserved ejection fraction. JAMA. (2020) 324:1499–500. doi: 10.1001/jama.2020.17445
16. Pellegrini D, Kawakami R, Guagliumi G, Sakamoto A, Kawai K, Gianatti A, et al. Microthrombi as a major cause of cardiac injury in COVID-19: a pathologic study. Circulation. (2021) 143:1031–42. doi: 10.1161/CIRCULATIONAHA.120.051828
17. Kollias A, Kyriakoulis KG, Dimakakos E, Poulakou G, Stergiou GS, Syrigos K. Thromboembolic risk and anticoagulant therapy in COVID-19 patients: emerging evidence and call for action. Br J Haematol. (2020) 189:846–7. doi: 10.1111/bjh.16727
18. Sandoval Y, Januzzi JL. Jr., Jaffe AS. Cardiac troponin for assessment of myocardial injury in COVID-19: JACC Review Topic of the Week. J Am Coll Cardiol. (2020) 76:1244–58. doi: 10.1016/j.jacc.2020.06.068
19. Giustino G, Croft LB, Stefanini GG, Bragato R, Silbiger JJ, Vicenzi M, et al. Characterization of Myocardial Injury in Patients With COVID-19. J Am Coll Cardiol. (2020) 76:2043–55. doi: 10.1016/j.jacc.2020.08.069
20. Guo T, Fan Y, Chen M, Wu X, Zhang L, He T, et al. Cardiovascular implications of fatal outcomes of patients with coronavirus disease 2019 (COVID-19). JAMA Cardiol. (2020) 5:811–8. doi: 10.1001/jamacardio.2020.1017
21. Sakabe M, Yoshioka R, Fujiki A. Sick sinus syndrome induced by interferon and ribavirin therapy in a patient with chronic hepatitis C. J Cardiol Cases. (2013) 8:173–5. doi: 10.1016/j.jccase2013.08.002
22. Li M, Chen S, Xiang X, Wang Q, Liu X. Effects of SARS-CoV-2 and its functional receptor ACE2 on the cardiovascular system. Herz. (2020) 45:659–62. doi: 10.1007/s00059-020-04989-x
23. Mafham MM, Spata E, Goldacre R, Gair D, Curnow P, Bray M, et al. COVID-19 pandemic and admission rates for and management of acute coronary syndromes in England. Lancet. (2020) 396:381–9. doi: 10.1016/S0140-6736(20)31356-8
24. Lantelme P, Couray Targe S, Metral P, Bochaton T, Ranc S, Le Bourhis Zaimi M, et al. Worrying decrease in hospital admissions for myocardial infarction during the COVID-19 pandemic. Arch Cardiovasc Dis. (2020) 113:443–7. doi: 10.1016/j.acvd.2020.06.001
25. Butt JH, Fosbøl EL, Gerds TA, Andersson C, Kragholm K, Biering-Sørensen T, et al. All-cause mortality and location of death in patients with established cardiovascular disease before, during, and after the COVID-19 lockdown: a Danish Nationwide Cohort Study. Eur Heart J. (2021) 42:1516–23. doi: 10.1093/eurheartj/ehab028
26. Smith DR. Bibliometrics, dermatology and contact dermatitis. Contact Dermatitis. (2008) 59:133–6. doi: 10.1111/j.1600-0536.2008.01405.x
27. Pu QH Lyu QJ, Su HY. Bibliometric analysis of scientific publications in transplantation journals from Mainland China, Japan, South Korea and Taiwan between 2006 and 2015. BMJ Open. (2016) 6:e011623. doi: 10.1136/bmjopen-2016-011623
28. Chandra SP, Singh A, Goyal N, Laythalling RK, Singh M, Kale SS, et al. Analysis of changing paradigms of management in 179 patients with spinal tuberculosis over a 12-year period and proposal of a new management algorithm. World Neurosurg. (2013) 80:190–203. doi: 10.1016/j.wneu.2012.12.019
29. Avcu G, Sahbudak Bal Z, Duyu M, Akkus E, Karapinar B, Vardar F. Thanks to trauma: a delayed diagnosis of pott disease. Pediatr Emerg Care. (2015) 31:e17–8. doi: 10.1097/PEC.0000000000000637
30. Chen C. CiteSpace II: Detecting and visualizing emerging trends and transient patterns in scientific literature. J Am Soc Inf Sci Technol. (2006) 57:359–77. doi: 10.1002/asi.20317
31. Zhou Y, Zhu XP, Shi JJ, Yuan GZ, Yao ZA, Chu YG, et al. Coronary heart disease and depression or anxiety: a bibliometric analysis. Front Psychol. (2021) 12:669000. doi: 10.3389/fpsyg.2021.669000
32. Chen C. Searching for intellectual turning points: progressive knowledge domain visualization. Proc Natl Acad Sci U S A. (2004) 101:5303–10. doi: 10.1073/pnas.0307513100
33. van Eck NJ, Waltman L. Software survey: VOSviewer, a computer program for bibliometric mapping. Scientometrics. (2010) 84:523–38. doi: 10.1007/s11192-009-0146-3
34. Xu SC, Zhao XY. Xing HP, Wu W, Zhang SY. Cardiac involvement in COVID-19: a global bibliometric and visualized analysis. Front Cardiovasc Med. (2022) 9:955237. doi: 10.3389/fcvm.2022.955237
35. Perianesrodriguez A, Waltman L, Eck NJV, Egghe L. Constructing bibliometric networks: A comparison between full and fractional counting. J Informetr. (2016) 10:1178–95. doi: 10.1016/j.joi.2016.10.006
36. Chen C, Hu Z, Liu S, Tseng H. Emerging trends in regenerative medicine: a scientometric analysis in CiteSpace. Expert Opin Biol Ther. (2012) 12:593–608. doi: 10.1517/14712598.2012.674507
37. Qin Y, Zhang Q, Liu Y. Analysis of knowledge bases and research focuses of cerebral ischemia-reperfusion from the perspective of mapping knowledge domain. Brain Res Bull. (2020) 156:15–24. doi: 10.1016/j.brainresbull.2019.12.004
38. Liang YD Li Y, Zhao J, Wang XY, Zhu HZ, Chen XH. Study of acupuncture for low back pain in recent 20 years: a bibliometric analysis via CiteSpace. J Pain Res. (2017) 10:951–64. doi: 10.2147/JPR.S132808
39. Schneider J W. Mapping scientific frontiers: the quest for knowledge visualization. J Am Soc Inf Sci Technol. (2004) 55:363–5. doi: 10.1108/00220410310472554
40. Ma D, Yang B, Guan B, Song L, Liu Q, Fan Y, et al. A bibliometric analysis of pyroptosis from 2001 to 2021. Front Immunol. (2021) 12:731933. doi: 10.3389/fimmu.2021.731933
41. Henry S. Co-citation in the scientific literature: a new measure of the relationship between two documents. J Am Soc Inf Sci Technol. (1973) 24:265–7. doi: 10.1002/asi.4630240406
42. Lai Y, Wang R, Chen X, Tang D, Hu Y, Cai J, et al. Emerging trends and new developments in monoclonal antibodies: a scientometric analysis (1980-2016). Hum Vaccin Immunother. (2017) 13:1–10. doi: 10.1080/21645515.2017.1286433
43. Zhou F, Yu T, Du R, Fan G, Liu Y, Liu Z, et al. Clinical course and risk factors for mortality of adult inpatients with COVID-19 in Wuhan, China: a retrospective cohort study. Lancet. (2020) 395:1054–62. doi: 10.1016/S0140-6736(20)30566-3
44. Guan WJ Ni ZY, Hu Y, Liang WH, Ou CQ, He JX, et al. Clinical characteristics of coronavirus disease 2019 in China. N Engl J Med. (2020) 382:1708–20. doi: 10.1056/NEJMoa2002032
45. Huang C, Wang Y, Li X, Ren L, Zhao J, Hu Y, et al. Clinical features of patients infected with 2019 novel coronavirus in Wuhan, China. Lancet. (2020) 395:497–506. doi: 10.1016/S0140-6736(20)30183-5
46. Shi S, Qin M, Shen B, Cai Y, Liu T, Yang F, et al. Association of cardiac injury with mortality in hospitalized patients with COVID-19 in Wuhan, China. JAMA Cardiol. (2020) 5:802–10. doi: 10.1001/jamacardio.2020.0950
47. Wu Z, McGoogan JM. Characteristics of and important lessons from the coronavirus disease 2019 (COVID-19) outbreak in china: summary of a report of 72 314 cases from the Chinese Center for disease control and prevention. JAMA. (2020) 323:1239–42. doi: 10.1001/jama.2020.2648
48. Chen N, Zhou M, Dong X, Qu J, Gong F, Han Y, et al. Epidemiological and clinical characteristics of 99 cases of 2019 novel coronavirus pneumonia in Wuhan, China: a descriptive study. Lancet. (2020) 395:507–13. doi: 10.1016/S0140-6736(20)30211-7
49. Hoffmann M, Kleine-Weber H, Schroeder S, Krüger N, Herrler T, Erichsen S, et al. SARS-CoV-2 cell entry depends on ACE2 and TMPRSS2 and is blocked by a clinically proven protease inhibitor. Cell. (2020) 181:271–80.e8. doi: 10.1016/j.cell.2020.02.052
50. Yang X, Yu Y, Xu J, Shu H, Xia J, Liu H, et al. Clinical course and outcomes of critically ill patients with SARS-CoV-2 pneumonia in Wuhan, China: a single-centered, retrospective, observational study. Lancet Respir Med. (2020) 8:475–81. doi: 10.1016/S2213-2600(20)30079-5
51. Zheng YY, Ma YT, Zhang JY, Xie X. COVID-19 and the cardiovascular system. Nat Rev Cardiol. (2020) 17:259–60. doi: 10.1038/s41569-020-0360-5
52. Richardson S, Hirsch JS, Narasimhan M, Crawford JM, McGinn T, Davidson KW, et al. Presenting characteristics, comorbidities, and outcomes among 5700 patients hospitalized with COVID-19 in the New York City Area. JAMA. (2020) 323:2052–9. doi: 10.1001/jama.2020.6775
53. Guo S, Wang L, Xie Y, Luo X, Zhang S, Xiong L, et al. Bibliometric and visualized analysis of stem cells therapy for spinal cord injury based on web of science and CiteSpace in the last 20 years. World Neurosurg. (2019) 132:e246–e58. doi: 10.1016/j.wneu.2019.08.191
54. Dong Q, Liang Q, Chen Y, Li J, Lu L, Huang X, et al. Bibliometric and visual analysis of vascular calcification research. Front Pharmacol. (2021) 12:690392. doi: 10.3389/fphar.2021.690392
55. Kleinberg J. Bursty and hierarchical structure in streams. Data Min Knowl Discov. (2003) 7:373–97. doi: 10.1145/775047.775061
56. Madjid M, Safavi-Naeini P, Solomon SD, Vardeny O. Potential effects of coronaviruses on the cardiovascular system: a review. JAMA Cardiol. (2020) 5:831–40. doi: 10.1001/jamacardio.2020.1286
57. Spinner CD, Gottlieb RL, Criner GJ, Arribas López JR, Cattelan AM, Soriano Viladomiu A, et al. Effect of remdesivir vs standard care on clinical status at 11 days in patients with moderate COVID-19: a randomized clinical trial. JAMA. (2020) 324:1048–57. doi: 10.1001/jama.2020.16349
58. Mueller AL, McNamara MS, Sinclair DA. Why does COVID-19 disproportionately affect older people? Aging (Albany NY). (2020) 12:9959–81. doi: 10.18632/aging.103344
59. Hanff TC, Harhay MO, Brown TS, Cohen JB, Mohareb AM. Is there an association between COVID-19 mortality and the renin-angiotensin system? a call for epidemiologic investigations. Clin Infect Dis. (2020) 71:870–4. doi: 10.1093/cid/ciaa329
60. Tomasoni D, Italia L, Adamo M, Inciardi RM, Lombardi CM, Solomon SD, et al. COVID-19 and heart failure: from infection to inflammation and angiotensin II stimulation. Searching for evidence from a new disease. Eur J Heart Fail. (2020) 22:957–66. doi: 10.1002/ejhf.1871
61. Bhatt AS, Moscone A, McElrath EE, Varshney AS, Claggett BL, Bhatt DL, et al. Fewer hospitalizations for acute cardiovascular conditions during the COVID-19 pandemic. J Am Coll Cardiol. (2020) 76:280–8. doi: 10.1016/j.jacc.2020.05.038
62. Piazza G, Campia U, Hurwitz S, Snyder JE, Rizzo SM, Pfeferman MB, et al. Registry of arterial and venous thromboembolic complications in patients with COVID-19. J Am Coll Cardiol. (2020) 76:2060–72. doi: 10.1016/j.jacc.2020.08.070
63. Chen Y, Li Y, Guo L, Hong J, Zhao W, Hu X, et al. Bibliometric analysis of the inflammasome and pyroptosis in brain. Front Pharmacol. (2020) 11:626502. doi: 10.3389/fphar.2020.626502
64. Prabhakaran D, Perel P, Roy A, Singh K, Raspail L, Faria-Neto JR, et al. Management of Cardiovascular Disease Patients With Confirmed or Suspected COVID-19 in Limited Resource Settings. Glob Heart. (2020) 15:44. doi: 10.5334/gh.823
65. Sliwa K, Singh K, Raspail L, Ojji D, Lam CSP, Thienemann F, et al. The world heart federation global study on COVID-19 and cardiovascular disease. Glob Heart. (2021) 16:22. doi: 10.5334/gh.950
66. Clark A, Jit M, Warren-Gash C, Guthrie B, Wang HHX, Mercer SW, et al. Global, regional, and national estimates of the population at increased risk of severe COVID-19 due to underlying health conditions in 2020: a modelling study. Lancet Glob Health. (2020) 8:e1003–e17. doi: 10.1016/S2214-109X(20)30264-3
67. Ayoubkhani D, Khunti K, Nafilyan V, Maddox T, Humberstone B, Diamond I, et al. Post-covid syndrome in individuals admitted to hospital with covid-19: retrospective cohort study. BMJ. (2021) 372:n693. doi: 10.1136/bmj.n693
68. Lai AG, Pasea L, Banerjee A, Hall G, Denaxas S, Chang WH, et al. Estimated impact of the COVID-19 pandemic on cancer services and excess 1-year mortality in people with cancer and multimorbidity: near real-time data on cancer care, cancer deaths and a population-based cohort study. BMJ Open. (2020) 10:e043828. doi: 10.1136/bmjopen-2020-043828
69. Ball S, Banerjee A, Berry C, Boyle JR, Bray B, Bradlow W, et al. Monitoring indirect impact of COVID-19 pandemic on services for cardiovascular diseases in the UK. Heart. (2020) 106:1890–7. doi: 10.1136/heartjnl-2020-317870
70. Nanjo A, Evans H, Direk K, Hayward AC, Story A, Banerjee A. Prevalence, incidence, and outcomes across cardiovascular diseases in homeless individuals using national linked electronic health records. Eur Heart J. (2020) 41:4011–20. doi: 10.1093/eurheartj/ehaa795
71. Wood A, Denholm R, Hollings S, Cooper J, Ip S, Walker V, et al. Linked electronic health records for research on a nationwide cohort of more than 54 million people in England: data resource. BMJ. (2021) 373:n826. doi: 10.1136/bmj.n826
72. Khan MS, Shahid I, Anker SD, Solomon SD, Vardeny O, Michos ED, et al. Cardiovascular implications of COVID-19 versus influenza infection: a review. BMC Med. (2020) 18:403. doi: 10.1186/s12916-020-01816-2
73. Behrouzi B, Araujo Campoverde MV, Liang K, Talbot HK. Bogoch, II, McGeer A, et al. Influenza vaccination to reduce cardiovascular morbidity and mortality in patients with COVID-19: JACC state-of-the-art review. J Am Coll Cardiol. (2020) 76:1777–94. doi: 10.1016/j.jacc.2020.08.028
74. Lala A, Johnson KW, Januzzi JL, Russak AJ, Paranjpe I, Richter F, et al. Prevalence and impact of myocardial injury in patients hospitalized with COVID-19 infection. J Am Coll Cardiol. (2020) 76:533–46. doi: 10.1016/j.jacc.2020.06.007
75. Einstein AJ, Shaw LJ, Hirschfeld C, Williams MC, Villines TC, Better N, et al. International impact of COVID-19 on the diagnosis of heart disease. J Am Coll Cardiol. (2021) 77:173–85. doi: 10.1016/j.jacc.2020.10.054
76. Hirschfeld CB, Shaw LJ, Williams MC, Lahey R, Villines TC, Dorbala S, et al. Impact of COVID-19 on cardiovascular testing in the united states versus the rest of the world. JACC Cardiovasc Imaging. (2021) 14:1787–99. doi: 10.1016/j.jcmg.2021.03.007
77. Brojakowska A, Narula J, Shimony R, Bander J. Clinical implications of SARS-Cov2 interaction with renin angiotensin system. J Am Coll Cardiol. (2020) 75:1–34. doi: 10.1016/j.jacc.2020.04.028
78. Mechanick JI, Rosenson RS, Pinney SP, Mancini DM, Narula J, Fuster V. Coronavirus and cardiometabolic syndrome: JACC focus seminar. J Am Coll Cardiol. (2020) 76:2024–35. doi: 10.1016/j.jacc.2020.07.069
79. Chan JF, Yuan S, Kok KH, To KK, Chu H, Yang J, et al. A familial cluster of pneumonia associated with the 2019 novel coronavirus indicating person-to-person transmission: a study of a family cluster. Lancet. (2020) 395:514–23. doi: 10.1016/S0140-6736(20)30154-9
80. Task Force for the management of COVID-19 of the European Society of Cardiology. European Society of Cardiology guidance for the diagnosis and management of cardiovascular disease during the COVID-19 pandemic: part 1-epidemiology, pathophysiology, and diagnosis. Eur Heart J. (2021) 43:1033–58. doi: 10.1093/eurheartj/ehab696
81. Ssentongo P, Ssentongo AE, Heilbrunn ES, Ba DM, Chinchilli VM. Association of cardiovascular disease and 10 other pre-existing comorbidities with COVID-19 mortality: A systematic review and meta-analysis. PLoS ONE. (2020) 15:e0238215. doi: 10.1371/journal.pone.0238215
82. Touyz R, Li H, Delles C. ACE2 the Janus-faced protein-from cardiovascular protection to severe acute respiratory syndrome-coronavirus and COVID-19. Clin Sci (Lond). (2020) 134:747–50. doi: 10.1042/CS20200363
83. Donoghue M, Hsieh F, Baronas E, Godbout K, Gosselin M, Stagliano N, et al. A novel angiotensin-converting enzyme-related carboxypeptidase (ACE2) converts angiotensin I to angiotensin 1-9. Circ Res. (2000) 87:E1–9. doi: 10.1161/01.res.87.5.e1
84. Ge XY Li JL, Yang XL, Chmura AA, Zhu G, Epstein JH, et al. Isolation and characterization of a bat SARS-like coronavirus that uses the ACE2 receptor. Nature. (2013) 503:535–8. doi: 10.1038/nature12711
85. Nicin L, Abplanalp WT, Mellentin H, Kattih B, Tombor L, John D, et al. Cell type-specific expression of the putative SARS-CoV-2 receptor ACE2 in human hearts. Eur Heart J. (2020) 41:1804–6. doi: 10.1093/eurheartj/ehaa311
86. Turner AJ, Hiscox JA, Hooper NM. ACE2: from vasopeptidase to SARS virus receptor. Trends Pharmacol Sci. (2004) 25:291–4. doi: 10.1016/j.tips.2004.04.001
87. Maas A. COVID-19, the wake-up call for implementing sex and gender in cardiovascular disease. Cardiovasc Res. (2021) 117:e39–40. doi: 10.1093/cvr/cvab023
88. Timmons JA, Anighoro A, Brogan RJ, Stahl J, Wahlestedt C, Farquhar DG, et al. A human-based multi-gene signature enables quantitative drug repurposing for metabolic disease. Elife. (2022) 11:e68832. doi: 10.7554/eLife.68832
89. Nadolsky KZ, Hurley DL, Garvey WT. Covid-19 & obesity: beyond bmi. Endocr Pract. (2020) 26:923–5. doi: 10.4158/EP-2020-0302
90. Drucker DJ. Coronavirus infections and type 2 diabetes-shared pathways with therapeutic implications. Endocr Rev. (2020) 41:bnaa011. doi: 10.1210/endrev/bnaa011
91. Carter SA-O, Baranauskas MN, Fly AD. Considerations for obesity, vitamin d, and physical activity amid the COVID-19 pandemic. Obesity (Silver Spring, Md). (2020) 28:1176–7. doi: 10.1002/oby.22838
92. Kruglikov IL SP. The role of adipocytes and adipocyte-like cells in the severity of COVID-19 infections. Obesity (Silver Spring, Md). (2020) 28:1187–90. doi: 10.1002/oby.22856
93. Ragia G, Manolopoulos VG. Inhibition of SARS-CoV-2 entry through the ACE2/TMPRSS2 pathway: a promising approach for uncovering early COVID-19 drug therapies. Eur J Clin Pharmacol. (2020) 76:1623–30. doi: 10.1007/s00228-020-02963-4
94. Roberts KA, Colley L, Agbaedeng TA, Ellison-Hughes GM, Ross MD. Vascular manifestations of COVID-19–thromboembolism and microvascular dysfunction. Front Cardiovasc Med. (2020) 26:7:598400. doi: 10.3389/fcvm.2020.598400
95. Chen L, Li X, Chen M, Feng Y, Xiong C. The ACE2 expression in human heart indicates new potential mechanism of heart injury among patients infected with SARS-CoV-2. Cardiovasc Res. (2020) 116:1097–100. doi: 10.1093/cvr/cvaa078
96. Robinson FA, Mihealsick RP, Wagener BM, Hanna P, Poston MD, Efimov IR, et al. Role of angiotensin-converting enzyme 2 and pericytes in cardiac complications of COVID-19 infection. Am J Physiol Heart Circ Physiol. (2020) 319:H1059–h68. doi: 10.1152/ajpheart.00681.2020
97. Shi CS, Nabar NR, Huang NN, Kehrl JH. SARS-coronavirus open reading frame-8b triggers intracellular stress pathways and activates NLRP3 inflammasomes. Cell Death Discov. (2019) 5:101. doi: 10.1038/s41420-019-0181-7
98. Liu J, Cao R, Xu M, Wang X, Zhang H, Hu H, et al. Hydroxychloroquine, a less toxic derivative of chloroquine, is effective in inhibiting SARS-CoV-2 infection in vitro. Cell Discov. (2020) 6:16. doi: 10.1038/s41421-020-0156-0
99. Gao J, Tian Z, Yang X. Breakthrough: chloroquine phosphate has shown apparent efficacy in treatment of COVID-19 associated pneumonia in clinical studies. Biosci Trends. (2020) 14:72–3. doi: 10.5582/bst.2020.01047
100. Chatre C, Roubille F, Vernhet H, Jorgensen C, Pers YM. Cardiac complications attributed to chloroquine and hydroxychloroquine: a systematic review of the literature. Drug Saf. (2018) 41:919–31. doi: 10.1007/s40264-018-0689-4
101. FDA. Coronavirus (COVID-19) Update: FDA Revokes Emergency Use Authorization for Chloroquine and Hydroxychloroquine. Available online at: https://www.fda.gov/news-events/press-announcements/coronavirus-covid-19-update-fda-revokes-emergency-use-authorization-chloroquine-and (accessed May 28, 2021).
102. Mo P, Xing Y, Xiao Y, Deng L, Zhao Q, Wang H, et al. Clinical characteristics of refractory coronavirus disease 2019 in Wuhan, China. Clin Infect Dis. (2021) 73:e4208–e13. doi: 10.1093/cid/ciaa270
103. Nishiga M, Wang DW, Han Y, Lewis DB, Wu JC. COVID-19 and cardiovascular disease: from basic mechanisms to clinical perspectives. Nat Rev Cardiol. (2020) 17:543–58. doi: 10.1038/s41569-020-0413-9
104. Task Force for the management of COVID-19 of the European Society of Cardiology. ESC guidance for the diagnosis and management of cardiovascular disease during the COVID-19 pandemic: part 2-care pathways, treatment, and follow-up. Cardiovasc Res. (2021) 118:1618–66. doi: 10.1093/cvr/cvab343
105. S Oliveira J, Sherrington C, R Y Zheng E, Franco MR, Tiedemann A. Effect of interventions using physical activity trackers on physical activity in people aged 60 years and over: a systematic review and meta-analysis. Br J Sports Med. (2020) 54:1188–94. doi: 10.1136/bjsports-2018-100324
106. González-Sanguino C, Ausín B, Castellanos M, Saiz J, López-Gómez A, Ugidos C, et al. Mental health consequences during the initial stage of the 2020 Coronavirus pandemic (COVID-19) in Spain. Brain Behav Immun. (2020) 87:172–6. doi: 10.1016/j.bbi.2020.05.040
107. Naylor-Wardle J, Rowland B, Kunadian V. Socioeconomic status and cardiovascular health in the COVID-19 pandemic. Heart. (2021) 107:358–65. doi: 10.1136/heartjnl-2020-318425
108. Wang H, Yang P, Liu K, Guo F, Zhang Y, Zhang G, et al. SARS coronavirus entry into host cells through a novel clathrin- and caveolae-independent endocytic pathway. Cell Res. (2008) 18:290–301. doi: 10.1038/cr.2008.15
109. Lawrence T. The nuclear factor NF-kappaB pathway in inflammation. Cold Spring Harb Perspect Biol. (2009) 1:a001651. doi: 10.1101/cshperspect.a001651
110. Oeckinghaus A, Ghosh S. The NF-kappaB family of transcription factors and its regulation. Cold Spring Harb Perspect Biol. (2009) 1:a000034. doi: 10.1101/cshperspect.a000034
111. Ryan S, Taylor CT, McNicholas WT. Predictors of elevated nuclear factor- kappaB-dependent genes in obstructive sleep apnea syndrome. Am J Respir Crit Care Med. (2006) 174:824–30. doi: 10.1164/rccm.200601-066OC
112. Hariharan A, Hakeem AR, Radhakrishnan S, Reddy MS, Rela M. The role and therapeutic potential of NF-kappa-B pathway in severe COVID-19 patients. Inflammopharmacology. (2020) 29:91–100. doi: 10.1007/s10787-020-00773-9
113. Hamid T, Guo SZ, Kingery JR, Xiang X, Dawn B, Prabhu SD. Cardiomyocyte NF-κB p65 promotes adverse remodelling, apoptosis, and endoplasmic reticulum stress in heart failure. Cardiovasc Res. (2011) 89:129–38. doi: 10.1093/cvr/cvq274
114. Olalla J. Remdesivir for the treatment of Covid-19-preliminary report. N Engl J Med. (2020) 383:993–4. doi: 10.1056/NEJMc2022236
115. Liang N, Zhong Y, Zhou J, Liu B, Lu R, Guan Y, et al. Immunosuppressive effects of hydroxychloroquine and artemisinin combination therapy via the nuclear factor-κB signaling pathway in lupus nephritis mice. Exp Ther Med. (2018) 15:2436–42. doi: 10.3892/etm.2018.5708
Keywords: cardiovascular diseases, COVID-19, CiteSpace, bibliometrics, visualization
Citation: Wei N, Xu Y, Wang H, Jia Q, Shou X, Zhang X, Zhang N, Li Y, Zhai H and Hu Y (2022) Bibliometric and visual analysis of cardiovascular diseases and COVID-19 research. Front. Public Health 10:1022810. doi: 10.3389/fpubh.2022.1022810
Received: 19 August 2022; Accepted: 23 November 2022;
Published: 08 December 2022.
Edited by:
Reza Lashgari, Shahid Beheshti University, IranReviewed by:
Adebayo Oluwafemi Adekunle, Xuzhou Medical University, ChinaJoseph Adu-Amankwaah, Xuzhou Medical University, China
Nnamdi Valbosco Ugwuoke, Khalifa University, United Arab Emirates
Copyright © 2022 Wei, Xu, Wang, Jia, Shou, Zhang, Zhang, Li, Zhai and Hu. This is an open-access article distributed under the terms of the Creative Commons Attribution License (CC BY). The use, distribution or reproduction in other forums is permitted, provided the original author(s) and the copyright owner(s) are credited and that the original publication in this journal is cited, in accordance with accepted academic practice. No use, distribution or reproduction is permitted which does not comply with these terms.
*Correspondence: Huaqiang Zhai, emhhaWhxQGJ1Y20uZWR1LmNu; Yuanhui Hu, aHVpeXVodWk1NUBzb2h1LmNvbQ==