- 1Advanced Technologies and Laboratories (ATL) International, Inc., Gaithersburg, MD, United States
- 2National Institute for Occupational Safety and Health, Centers for Disease Control and Prevention, Cincinnati, OH, United States
- 3National Institute for Occupational Safety and Health, Centers for Disease Control and Prevention, Washington, DC, United States
- 4University of California, San Diego, San Diego, CA, United States
Like nanotechnology, translational science is a relatively new and transdisciplinary field. Translational science in occupational safety and health (OSH) focuses on the process of taking scientific knowledge for the protection of workers from the lab to the field (i.e., the worksite/workplace) and back again. Translational science has been conceptualized as having multiple phases of research along a continuum, beyond scientific discovery (T0), to efficacy (T1), to effectiveness (T2), to dissemination and implementation (D&I) (T3), to outcomes and effectiveness research in populations (T4). The translational research process applied to occupational exposure to nanomaterials might involve similar phases. This builds on basic and efficacy research (T0 and T1) in the areas of toxicology, epidemiology, industrial hygiene, medicine and engineering. In T2, research and evidence syntheses and guidance and recommendations to protect workers may be developed and assessed for effectiveness. In T3, emphasis is needed on D&I research to explore the multilevel barriers and facilitators to nanotechnology risk control information/research adoption, use, and sustainment in workplaces. D&I research for nanomaterial exposures should focus on assessing sources of information and evidence to be disseminated /implemented in complex and dynamic workplaces, how policy-makers and employers use this information in diverse contexts to protect workers, how stakeholders inform these critical processes, and what barriers impede and facilitate multilevel decision-making for the protection of nanotechnology workers. The T4 phase focuses on how effective efforts to prevent occupational exposure to nanomaterials along the research continuum contribute to large-scale impact in terms of worker safety, health and wellbeing (T4). Stakeholder input and engagement is critical to all stages of the translational research process. This paper will provide: (1) an illustration of the translational research continuum for occupational exposure to nanomaterials; and (2) a discussion of opportunities for applying D&I science to increase the effectiveness, uptake, integration, sustainability, and impact of interventions to protect the health and wellbeing of workers in the nanotechnology field.
Introduction
Nanotechnology and the development of nanomaterials have yielded a large number of commercial products and exposures to workers (1–3). The diffusion of nanotechnology into commerce in the early 2000's raised concern about potential hazards to workers (4–6). The small particle size, novel characteristics of nanoparticles and hypotheses about interaction with biological molecules and structures prompted public health authorities to consider whether workers could be at risk of adverse effects from exposure to engineered nanomaterials (ENM). To prevent such effects, if they were to occur, occupational public health authorities promoted and conducted research and issued guidance for employers and workers on risk management (6–8). The overarching questions are identifying the extent to which guidance was used, and whether the guidance made a difference in the health and safety of workers. Unfortunately, these and related questions have not been the focus of much research. In fact, such research has rarely, with notable exceptions, been considered or implemented in the occupational health field, in general, let alone in the area of exposure of workers to ENM (9–11). To address this shortfall there has been an effort in recent years to promote research on the “downstream” part of a continuum beyond basic research to impact (Figure 1) (11). This research, which includes dissemination and implementation (D&I) research, can fall under the umbrella of “translational science.” Traditionally, translational science has focused largely on barriers to intervention development at the efficacy and effectiveness stages (T1 and T2). Once an evidence base is established, D&I science has focused on barriers to the adoption, use, and sustainment (T3 and T4) of evidence-based interventions (12). Table 1 illustrates key characteristics of dissemination and implementation research and hypothetical examples for the occupational safety and health field. Figure 1 depicts the translational science cycle, conceptualized as crossing all translational or “T” phases of the research continuum, from scientific discovery (T0) to efficacy (T1), effectiveness (T2), dissemination and implementation (T3), and the outcomes and effectiveness and impact of research in populations (T4). OSH interventions (such as those represented in Table 2) may be situated anywhere along the research continuum, and, in reality, do not necessarily pass through all the T stages. In the context of OSH research (and beyond), there is a dearth of research conducted in the T3 and T4 stages of the research continuum (14).
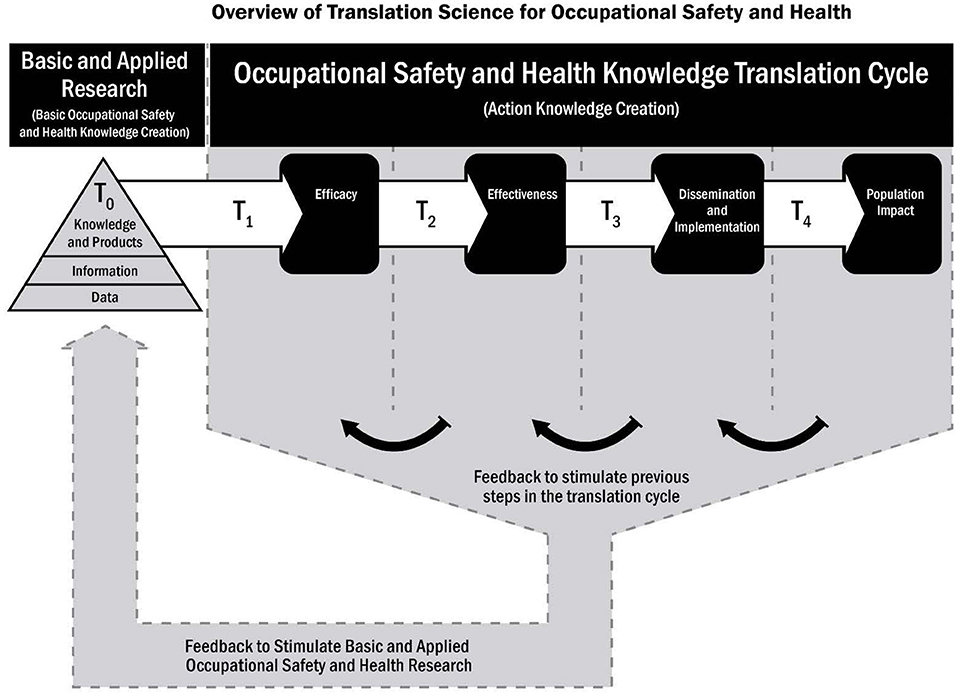
Figure 1. Overview of translational science for occupational safety and health [adapted from Schulte et al. (11)].
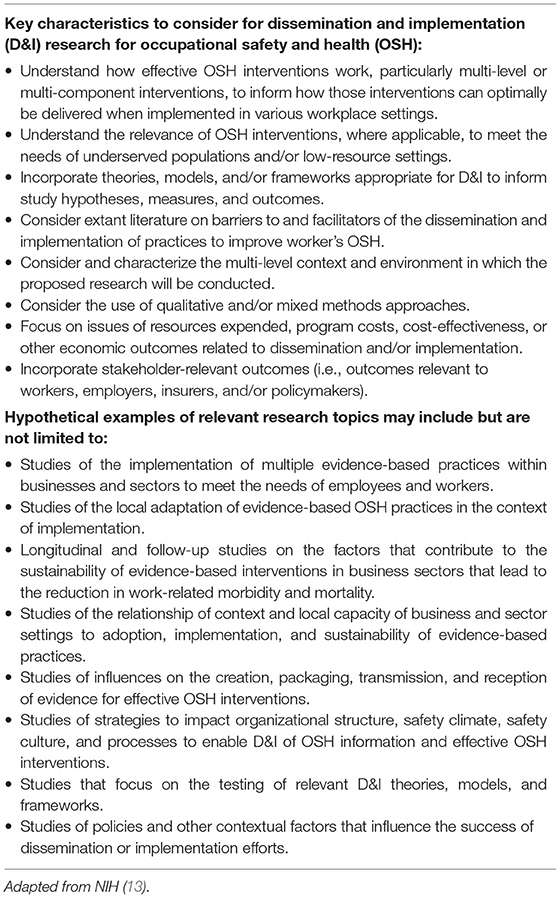
Table 1. Ideas for advancing dissemination and implementation science in occupational safety and health.
While closely connected, the primary focus of translational science is not to do translation or its synonymous activity, research-to-practice (R2P) [an approach to collaborations with partners on the adoption, use, and adaptation of knowledge, interventions and technologies (15)]. Rather, it is the study of how these activities and processes (i.e., uptake, implementation, sustained use) work, what their multilevel barriers and facilitators are, and what intermediate and ultimate impacts they have on diverse outcomes. It should be noted that the processes shown in Figure 1 are not linear but represent a dynamic and iterative interplay across stages and involve many factors that interact and provide feedback to previous and consecutive stages. The challenge is to study the main causal factors while accounting for and acknowledging the complex context within which they exist.
Ultimately, there is a need to investigate many casual pathways that influence worker safety and health outcomes. Sorensen et al. (16) developed a conceptual model “based on the premise that addressing multiple pathways in an integrated manner with a focus on the conditions of work will contribute to greater impairment in worker and enterprise outcomes than addressing each pathway separately” (16). This integrated approached can guide research and intervention designs and may be a framework for T1 and T2 efforts.
In this paper we examine how research and guidance on ENMs are situated within the translational science continuum, and identify gaps related to, and opportunities for, increasing research efforts particularly at the later stages (T3 and T4) of that continuum. To that end, the paper will provide: (1) an illustration of the translational research continuum for occupational exposure to ENMs; and (2) a discussion of opportunities for expanding efforts in D&I science to increase the effectiveness, integration and impact of interventions to protect the health and wellbeing of workers in the nanotechnology field.
For illustrative purposes, we present three examples of OSH interventions— broadly defined as programs, practices, policies, recommendations, and guidelines (17)—that can be considered relevant for occupational exposure to ENMs and could give rise to translational research questions: (1) National Institute for Occupational Safety and Health (NIOSH) recommended exposure limits for titanium dioxide (TiO2) and carbon nanotubes and nanofibers, (CNTs/CNFs) (18, 19); (2) World Health Organization (WHO) guidelines for protecting workers (20); and (3) The International Risk Governance Council (IRGC) guidance on nanotechnology risk governance (21, 22). These examples were selected because they are widely known by investigators and practitioners in the field of nanomaterial workers' health and illustrate how translational science can be applied to different situations.
What Is the Evidence and What Are Interventions for ENM?
Critical in translational science is understanding how evidence is defined. When is it decided that the science or assembled information is sound enough to transmit to end users? (23). Too much waiting for interventions to meet specific evidence standards may contribute to translational lag while also leading to the generation of interventions that cannot be replicated in real-world settings such as worksites (24, 25). Applying a translational science lens to the development and implementation of interventions related to ENMs might change our definition of evidence and what activities can be undertaken depending on the stage on the translational science continuum. While the evidence for basic science is well-established and is based on more traditional scientific experimentation randomized control trials (RCT), evidence at later stages of the continuum may vary and can be based on the synthesis of information and peer review of guidance or a critical review of the literature based on results from diverse study designs (e.g., cross-sectional, case control, or longitudinal studies). When establishing evidence for later stage translational work, traditional RCTs can become problematic. For example, the use of RCTs in occupational settings, arguably, could be seen as unethical if an intervention judged to be efficacious and safety or health-enhancing is withheld from some segment of the workforce exposed to a potential hazard (26, 27).
It is also important to identify what an intervention is with regard to ENMs. The interventions to protect workers from adverse effects may vary substantially. Generally, in translational science, interventions can be described in broad terms as one of the “7 P's”: programs, practices, principles, procedures, products, pills and policies (28). In the ENM arena most of these, except pills, would be considered as interventions to address occupational hazards (Table 2). Table 2 was developed to help the nanotechnology community understand that “intervention” can have a wide range of meanings. These interventions can fit at all stages of Figure 1. The target audiences for translational science are the organizations and decision-makers who develop, disseminate, or implement interventions. These audiences include government authorities, decision-makers, employers, trade associations, and unions. Ultimately the interventions will be implemented to protect workers but the pathway generally will be through employers.
Translational Science
It is useful to envision the continuum of translational science from beyond basic research through the impact of that research on populations. Translational science has been conceptualized as having multiple phases of research beyond scientific discovery (T0), to efficacy research (T1), to effectiveness research (T2), to dissemination and implementation research (D&I) (T3), to outcomes and effectiveness research in populations (T4) (12, 29–35). From an occupational safety and health perspective, for translational research findings to make a difference in the safety and health of workers it is necessary to determine the best ways to direct basic research findings and guidance information to employers, workers and authorities promotes the uptake of that information, and determines whether it made a positive impact.
The T0 stage is focused on scientific discovery driven by basic science. While there are important feedback loops between the T0 and later T1 through T4 stages, this paper will focus on T1 to T4 that is, how well the products of basic research can be utilized to affect and protect ENM workers and, specifically, how to study the stages leading to such protection. To better view the stages between T1 and T4 this paper reviews various examples of the continuum applied to hazard information and interventions related to ENMs. In the next sections and in Tables 3–5 we present three examples.
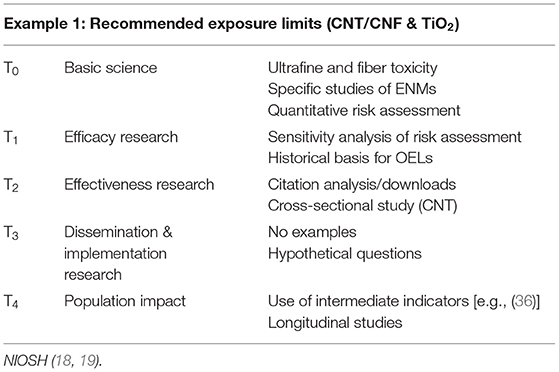
Table 3. Utilization of translational science pertaining to NIOSH recommended exposure limits for TiO2 and CNT/CNF.
Translational science is a field that merits support because it is a crucial that stakeholders receive and use recommendations and interventions as a means to responsible development of a technology, corporate responsibility, and worker and population wellbeing (37–42). The successful commercialization of nanotechnology depends on societal acceptance of it. Societal acceptance will be influenced by, among other things, whether workers are protected from nanotechnology hazards. When it is apparent that workers are at high risk of adverse health effects and not protected, the societal response toward acceptance of the technology is likely to be negative (37). Therefore, it is incumbent on employers and authorities to support the development of translational science as a means to support responsible development of nanotechnology. Supporting translational science will result in broad utilization of guidance and increase involvement of stakeholders in that process (37, 43–47). For example, Brownson (47), building on work of Curry (48) and Anderson et al. (49), described a push-pull model for strategic public health science that could be adopted to strategic transitional science for occupational safety and health: “This model posits that for science to effect practice there must be a combination of push (a basis in science and technology) and the pull (a market demand from practitioners), and the capacity (delivery ability of public health systems).” The adaption would be that practitioners would be considered as employers, workers, unions, trade associations, and other decision-makers that affect worker protection.
Example 1: NIOSH Recommended Exposure Limits for TiO2 and CNT
T0: Basic Science - Discovery Data
Table 3 illustrates the translational science continuum for this example. Titanium dioxide (TiO2) and CNT/CNF are ENMs that were studied early on in the commercialization of nanotechnology [see (18, 19) for review] and found to have adverse health effects in laboratory animals. Clearly, there are many types of TiO2, and CNT/CNF and the toxicity information only pertained to specific chemical and physical types. The type of toxicological assessments that is most relevant to occupational safety and health are rodent inhalation studies. TiO2 and other poorly soluble particles of low toxicity (PSLT) of fine and ultrafine sizes were found to show consistent dose-response relationships for adverse pulmonary inflammation and lung tumors when dose was expressed as particle surface area. Specifically, the chronic animal inhalation study of TiO2 (50) demonstrated the development of lung tumors (bronchioloalveolar adenomas) in response to exposure to a relatively large dose of 250 mg/m3.
Subsequently, Henrich et al. (51) showed a statistically significant lung cancer excess in rats exposed to ultrafine TiO2 at a concentration of 10 mg/m3.
Studies in rats and mice have shown that CNTs and CNFs can pose a respiratory hazard due to pulmonary inflammation and rapidly developing, persistent fibrosis (19, 52). Occupational exposure to CNTs and CNFs has been associated with biomarkers of fibrosis, inflammation, oxidative stress, and cardiovascular responses in workers (53).
T1: Efficacy Research
The translational science T1 phase refers to the development of an intervention and studies of its efficacy, which is a term to describe whether an intervention can work in an optimal and highly controlled laboratory setting. For controlling exposures, occupational exposure limits (OELs) [for example, NIOSH Recommended Exposure Limits (RELs)] are well-established interventions (7, 18, 19). The focus of this example are the NIOSH RELs for TiO2 and CNTs/CNFs. The RELs are generally based on quantitative risk assessments of appropriate toxicity data sets. In the realm of ENMs T1 can be thought of as whether a risk assessment is robust. For TiO2, animal data were analyzed in a dose-responsive quantitative risk assessment and two categories of TiO2 were assessed: fine (>100 nm) and ultrafine (≤100 nm), and respective RELs of 2.4 and 0.3 mg/m3, as a time-weighted average concentration for up to 10 h per day during a 40 h work week were determined. These RELs corresponded to lifetime risk estimates associated with <1/1,000 excess risk for lung cancer. To assess the efficacy of this risk determination a model averaging approach was utilized. This approach uses all the information from many exposure-response models and weights them by the Akaike information criteria for model fit and constructs an average dose-response model (54, 55). CNT animal studies indicated the CNT exposure may result in localized and systematic inflammation, cytotoxicity, pulmonary, interstitial fibrosis, mutagenesis, and the potential for lung cancer (19). NIOSH performed a quantitative risk assessment using dose-response data of adverse lung effects in rats following subchronic inhalation of CNTs and CNFs, and also evaluated rodent studies of lung effects by other routes of exposure. The NIOSH REL of 1 μg/m3 for CNTs/CNFs (as a respirable mass 8-h TWA concentration) was set at the limit of quantification (LOQ) of the analytical method for element carbon (NIOSH method 5040).
In addition to the RELs for TiO2 and CNT/CNFs, NIOSH provided guidance for hazard and risk management in the NIOSH Current Intelligence Bulletins for TiO2 and CNT/CNF that could be considered for expository purposes as part of the intervention (18, 19). The T1 efficacy research for this intervention included peer, stakeholder, and public reviews. In general, these reviews supported and validated the recommendations in the Current Intelligence Bulletins.
T2: Effectiveness Research
Studies conducted under the T2 stage of the translational continuum are focused on assessing the effectiveness of an intervention. This refers to whether the intervention “works” in the real world outside the laboratory where the conditions are less controlled. Can it protect workers and prevent disease from exposure to TiO2 and CNT/CNF? And can results from these studies be generalized to other ENM workers/settings?
While not specifically designed as a T2 study, a cross-sectional study showed that in 12 facilities handling CNT/CNFs that approximately 93% of averaged samples collected at the respirable fraction for EC mass were below the NIOSH REL of 1 μ/m3 (56). This indicates that the REL could be met in practice. No other studies were identified to assess the effectiveness of the NIOSH RELs.
T3: Dissemination and Implementation Research
Dissemination and implementation (D&I) science is defined as the study of methods and strategies for bridging the gap between public health research and practice (13). D&I scientists use a number of empirically tested theories, models and frameworks (TMFs) to plan, evaluate, or understand barriers and facilitators to D&I processes (57–62). Some commonly used TMFs among D&I researchers (63) include the Consolidated Framework for Implementation Research (CFIR) (57), the RE-AIM (Reach, Effectiveness, Adoption, Implementation, Maintenance) framework and its contextually expanded version the Practical Robust Implementation and Sustainability model (PRISM) (61, 64, 65), the EPIS (Exploration, Planning, Implementation, Sustainment) framework (66, 67), the Diffusion of Innovation theory (68), and the Theoretical Domains Framework (TDF) (69). However, more than 150 D&I TMFs have been identified (70).
In regard to nanotechnology and worker's health dissemination is the active and targeted distribution of information and intervention materials to a specific business audience. Critical to this is the involvement/engagement with stakeholders early on in the research process so the interventions and strategies for distribution are feasible, relevant, and equitable. Dissemination research is defined as the scientific study of these phenomena for the purpose of understanding how best to spread knowledge required to adopt or deploy an intervention. Implementation of the REL is the critical intermediate step in the continuum from research to prevention. Implementation can be defined as the adoption and integration of evidence-based health intervention (in this case RELs) into business practices.
Implementation research is the study of this process (12). Important translational research questions include what prompts employers to consider the uptake of, and implementation into routine practice and use of such guidance, and what are the key barriers and facilitators to the use of those interventions across diverse workplace settings with different resources (14). D&I science inquiry addresses what works, for who, how, why, in what settings, and how it is sustained over time (34).
Implementation strategies that might be relevant for study include engaging trusted intermediaries and developing a business case.
In the T3 D&I research stage, there are many rigorous study designs that have been used (28, 71). “These include both experimental (e.g., randomized controlled trial, cluster-randomized controlled trial, pragmatic trial, stepped wedge trial, dynamic wait-listed control trial) and quasi- experimental (e.g., nonequivalent groups, pre-/post-, regression discontinuity, interrupted time series), non-experimental or observational (including designs from epidemiology) designs as well as qualitative (e.g., focus groups, semi-structured interviews), mixed-methods (i.e., the collection and integration of qualitative and quantitative data), and system science (e.g., system dynamics, agent-based modeling) approaches” (71). Except for a few qualitative studies, rarely, have any of these study designs been applied to ENM interventions (36).
It appears that there are no published examples of T3 D&I studies related to ENM safety and health so we provide a hypothetical example of questions that could be investigated. Numerous law firms, trade associations, and labor groups served to amplify the REL information published by NIOSH. Translational research in the T3 stage could address what were the best strategies to get the REL information (i.e., intervention) to employers, what are the factors that hinder/facilitate adoption of REL information by employers, how REL information should be packaged and what additional information and resources are needed to facilitate broad dissemination, uptake, and initial implementation by organizations and what are key strategies and resources needed for the sustained use of REL information over time across diverse workplaces. It is critical at this stage and all stages of the research continuum, to consider questions around occupational health equity. These include: how diverse stakeholders including employers, employees labor groups, and professional organizations, will be engaged in the research process and the delivery and implementation of the intervention (i.e., REL information); and will the intervention be feasible for lower resource workplaces employing individuals with high vulnerability?
Measuring success in the T3 stage can take various forms. A possible indicator of success might be to assess the extent to which an intervention, in this case NIOSH guidance, was adopted by companies as the basis for risk management efforts for TiO2 exposure. To make this determination it would be necessary to survey employers on whether they based risk management decisions on NIOSH guidance. However, for such surveys it is difficult to get appropriate participation possibly because the information requested may be viewed as “business confidential”. It may be that tracking the uptake and use of specific guidance may be quite difficult and it might be better to attempt to track uptake of authoritative guidance in general.
Moreover, tracking use of guidance assumes a linear process that a certain authoritative report will influence an employer to take action when clearly employer decision-making is influenced by a large number of factors.
T4: Population Impact
Cross-sectional data shown under the T2 effectiveness stage indirectly demonstrates the population impact of REL information within the population of ENM workers and employers. Although due to the limitations of the design it is challenging to establish a causal pathway between the intervention (REL information) and the observed outcomes of REL usage and exposure control. More data are needed on potential impact of the REL intervention from workplaces where exposure could occur. Also needed are data on the prevalence of control use. There are few studies of the use of controls for ENM interventions in general. Iavicoli et al. (36) found that controls were used substantially but their results were based on a small response rate. This may in part be due to concerns about confidentiality of business information. Ultimately, there is need for studies of the extent of disease in workers over time. Ideally, these would be longitudinal studies or two point in time surveillance assessments (72) and would include diverse process and impact outcomes.
Example 2: WHO Guidance: Protecting From Potential Risks of Manufactured Nanomaterials
T0: Basic Science - Discovery Data
Table 4 illustrates the translational science continuum for this example. In addition to the type of toxicity data discussed earlier for TiO2 and CNT/CNFs, discovery data may be driven from stakeholders' concerns. For ENMs it was noted in 2007 that “Non-governmental organizations (NGOs) had been active in calling for worker protection in emerging nanotechnology industries (https://www.who.int/occupational_health/background_review_1.6.12.pdf; line 10).” In 2010 a European Trade Union Confederation recommended application of the precautionary principle because of potential hazards of ENMs. The focus of this example is the WHO guidance on protecting workers from potential risks of manufactured nanomaterials (20). Assessment of the health impacts of new technology is one of the activities of the Global Plan of Action on Worker's Health adopted in 2007. WHO was concerned that the increased production and use of ENM, meant that workers would be at the “…front line of exposures to these materials placing them at an increased of potential adverse health effects (20).” These guidelines were meant to prevent such effects.
T1: Efficacy Research
The determination of efficacy of control guidance (i.e., the intervention) was based on years of practice controlling ultrafine particles (6–8). This was supported by evidence-based literature. Specifically, for efficacy of the guidance information created by the WHO workgroup, they used the PECO (Population/Situation Exposure-Comparison-Outcome) approach and the GRADE (Grading of Recommendations Assessment, Development and Evaluation) framework for the judgement of the quality of the evidence (20). The target group for the guidance was in phase 1: policy makers from low- and medium-income countries and in phase 2, an implementation guide for employers and workers. The policy was designed to fill critical knowledge gaps such as: (1) Can an algorithm be developed to classify engineered nanoparticles by degree of potential hazards? (2) Which characteristics of particles and which measurement techniques should be used for the assessment of exposure to engineered nanoparticles? (3) What is the exposure to engineered nanoparticles in the workplace? (4) What are the limits of engineered controls and personal protective equipment (PPE) with regard to engineered nanoparticles? (5) What occupational health surveillance should be recommended for workers potentially exposed to engineered nanoparticles? (6) Should exposure registries be established for various groups of workers potentially exposed to engineered nanoparticles? (7) Should engineered nanoparticles be treated as “new” substances and evaluated for safety hazards? The guidance was developed by an international group of experts with the assistance of the WHO Global Network of Collaborating Centres who conducted systematic review studies. The guidelines were peer reviewed by external reviewers.
T2: Effectiveness Research
The effectiveness of the guidance can be assessed against past experience and is similar to the efficacy determination. As noted, one way to assess the effectiveness is through citation analysis. Translation research approaches could be used to determine the extent to which exposure data have been collected in locations where the guidance has been implemented. What was found? No studies on this aspect were identified.
T3: Dissemination and Implementation Research
The foundation of the D&I stage is the causal evidence that underlies the intervention and the organizational change envisioned. In the WHO guidelines the intervention is recommendations for the safe use of nanomaterials in the workplace.
The WHO Guideline Development Group (GDG) prepared an implementation plan, which included the following activities:
1. Translating to other languages;
2. Integrating the recommendations of the WHO guidelines into training courses on nanosafety (e.g., UNITAR);
3. Providing and disseminating information:
• web communication;
• conference presentations;
4. Providing information on the E.U. and U.S. regulations for nanomaterials in the workplace;
5. Developing Safety Data Sheets for nanomaterials in collaboration with the WHO/ILO International Chemical Safety Cards program and UN GHS: nanoscale titanium dioxide (TiO2) ICSC#1782 and nanoscale zinc oxide (ZnO);
6. Developing a corporate governance document in collaboration with OECD;
7. Developing a list of practical tools for guideline implementation;
8. Assessing the use of nanomaterials in countries;
9. Providing real-world examples on the use of hazard information and proposed OELs in countries and in workplaces.
The GDG started working on the first five activities but did not complete this plan due to competing demands and priorities. Various D&I research studies could examine aspects of the adoption, implementation and sustainment of this plan, however, no studies of the barriers or facilitators in the plan were conducted. This is what would be needed for T3 stage.
T4: Population Impact
There are no data to describe the population impact of the WHO report on intermediate indicators such as the use of recommended risk management practices or on ultimate indicators of morbidity or mortality.
Example 3: The International Risk Governance Council (IRGC) White Paper on Nanotechnology Risk
Table 5 illustrates the translational science continuum for this example. The International Risk Governance Council commissioned Renn and Roco to develop a white paper [distilled into a journal article (21)] on a risk governance framework for emerging nanotechnology.
T0: Basic Science - Discovery Data
The many facets of emerging nanotechnology coupled with many unknowns and extensive societal concerns in the early 2000's indicated that a comprehensive governance guidance was needed to protect citizens in general and workers in particular who might be at risk. The basic concern was that ENMs may potentially have toxic effects on people exposed to them. The purpose of the IRGC white paper on nanotechnology risk governance was to present decision makers with a systematic and integrated approach to analyzing and managing anticipated risks from exposure to ENMs (22). While the white paper was expected to have interest to governments, industry, academia, and NGOs, the prime focus was on governments' responsibility for developing and implementing policies.
There was also concern based on the history of dust explosions that nanoparticles could also be explosive. The white paper stated that, “There seems to be no lower particle size limits below which dust explosions could not occur. It may be possible that the increased surface area of nanoparticles could also include the likelihood that they become self-charged and ignite (22).”
T1: Efficacy Research
T1 research also involves assessing the potential of the IRGC to recommend risk governance guidance (intervention) (22). An initial scoping workshop involving a broad range of stakeholders and experts from academia, government, industry, insurance, law, and NGOs, was aimed at identifying large governance deficits for nanotechnology. Surveys of experts like those at the scoping workshop were also conducted. These surveys and workshops indicated that industry was highly aware of the potential impact on levels of innovation that could be caused by inadequate risk governance of human health and the environment, and the perception of the public. The major focus identified in the survey responses was research on Environment, Health and Safety and, in particular, the development of guidelines for worker health and safety and the establishment of an international methodology and nomenclature (22).
T2: Effectiveness Research
While many people and groups participated in the development of the IRGC risk guidance this is not necessarily evidence that it is effective. It does support, to some extent, its application potential and acceptance. Translational research could include: synthesis of evidence for risk governance and risk management of fine dust and powders, in particular, as well as efforts to explore applicability of governance guidelines for specific situations. In general, evidence gathering on effectiveness and value of governance could also be accomplished by multi-stakeholder evaluation (73).
T3: Dissemination and Implementation Research
T3 D&I studies would focus on research on the dissemination and implementation of the IRGC risk guidance. There were no examples in the published literature of T3 studies for the IRGC risk guidance. In the T3 stage, assessing the barriers, facilitators, and best practices for the dissemination and implementation of the IRGC risk guidance intervention could be undertaken. For dissemination, one might investigate the extent to which employers and other decision-makers received the governance guidance, what were the best strategies for dissemination, and was there variation in who did and did not receive the guidance (i.e., equity perspective). For implementation, research could focus on how employers adopted, used, and sustained practices based on the IRGC risk guidance.
T4: Population Impact
Like the other examples, the ultimate impact of an intervention is prevention of or decrease in morbidity and mortality associated with the ENM hazard potential. However, the ultimate impact of prevention may take a long time to obtain and the pathway between guidance and prevention may be indirect and involve diverse factors that are difficult to identify. Translational research may need to focus on intermediate outcomes, chiefly utilization of risk assessment and management practices included in the guidance. There were no published examples of T4 studies for the IRGC risk guidance.
Conclusion
If occupational safety and health research on ENMs is to lead to protection of workers exposed to ENMs, there is a need to assure that the full continuum from basic research to population impact is considered. Historically, there has not been sufficient focus on the stages that appear “downstream” from basic research. The types of evidence needed and factors and processes associated with these later stages of the continuum can substantially differ from those relevant in earlier stages. Furthermore, these later stages (i.e., understanding what will determine eventual dissemination, implementation, and broad impact of an intervention) can have an important impact on how earlier stages of research (i.e., basic science and efficacy) are done. This paper provides a framework for considering the application of translational science to protect workers exposed to ENMs. Three examples were provided to illustrate this application. The key issues in applying translational science to ENM exposures is the need to invest in such science and the ability to get the results of such research to decision-makers and employers. Translational science may also include explorations of the information uptake and use by employers and decision-makers and identification of barriers and facilitators to these functions.
Clearly, the uptake and use of translational science pertaining to ENMs occurs against a backdrop of societal rules, laws and norms with regard to worker protection. Nonetheless, the outputs of translational science can provide useful information that can lead to the protection of workers. This paper is a call for utilizing translational science on a regular basis. If that is to occur there is need for funding of a workforce with translational science skills. With limited funding for ENM research in general it may be necessary to shift funding for better balance along all phases of the research to impact continuum.
Author Contributions
PS wrote the first draft. RG and BR made major revisions. TC, LH, and VM provided useful comments and example materials. All authors contributed to the article and approved the submitted version.
Funding
This was developed as part of government work. PS initiated this paper as part of government work and subsequently under contract.
Author Disclaimer
The findings and conclusions in this report are those of the authors and do not necessarily represent the official position of the National Institute for Occupational Safety and Health (NIOSH), Centers for Disease Control and Prevention (CDC). Mention of any company or product does not constitute endorsement by NIOSH, CDC.
Conflict of Interest
PS was employed by the company Advanced Technologies and Laboratories (ATL) International, Inc., under contract with NIOSH.
The remaining authors declare that the research was conducted in the absence of any commercial or financial relationships that could be construed as a potential conflict of interest.
Publisher's Note
All claims expressed in this article are solely those of the authors and do not necessarily represent those of their affiliated organizations, or those of the publisher, the editors and the reviewers. Any product that may be evaluated in this article, or claim that may be made by its manufacturer, is not guaranteed or endorsed by the publisher.
References
1. Kuhlbusch TAJ, Winhoven SWP, Haase A. Nanomaterial exposures for workers, consumers and the general public. Nanoimpact. (2018) 10:11–25. doi: 10.1016/j.impact.2017.11.003
2. Oberbek P, Kozikowski P, Czarmeche K, Sobien P, Jakubiok S, Jankowski T. Inhalation exposure to various nanoparticles in work environment- contextual information and results of measurements. J Nanopart Res. (2019) 21:222. doi: 10.1007/s11051-019-4651-x
3. Schulte PA, Leso V, Niang M, Iavicoli I. Current state of knowledge of health effects of engineered nanomaterials in workers in a systemic review of human studies and epidemulogical investigation. SJWEH. (2019) 45:217–38. doi: 10.5271/sjweh.3800
4. SCENIHR. Request for a Scientific Opinion on the Appropriateness of Existing Methodologies to Assess the Potential Risks Associated With Engineered and Adventitious Nanotechnologies. Brussels: Scientific Committee on Emerging and Newly Identified Health Risks (2006).
6. BSI. Nanotechnologies Part 2: Guide to Safe Handling and Disposal of Manufactured Nanomaterials. London: British Standards Institution (2007). p. 6699.
7. Schulte P, Geraci C, Zumwalde R, Hoover M, Kuempel E. Occupational risk management of engineered nanoparticles. J Occup Environ Hyg. (2008) 5:239–49. doi: 10.1080/15459620801907840
8. Safe Work Australia. Engineered Nanomaterials: Feasibility of Establishing Exposure and Using Control Banding in Australia (2010).
9. Storm JF, LePrevost CE, Tutor-Marcom R, Cope WG. Adapting certified safe farm to North Carolina agriculture: an implementation study. J Agromed. (2016) 21:269–83. doi: 10.1080/1059924X.2016.1180273
10. Tinc PJ Gadomski A Sorensen JA Weinhall L and Jenkins P Lindvall K. Applying the consolidated framework for implementation research to agricultural safety and health: barriers, facilitators, and evaluation opportunities. Saf. Sci. (2018) 107:99–108. doi: 10.1016/j.ssci.2018.04.008
11. Schulte PA, Cunningham TR, Nickels L, Felknor S, Guerin R, Blosser F, et al. Translation research in occupational safety and health: a proposed framework. Am, J Ind Med. (2017) 60:1011–22. doi: 10.1002/ajim.22780
12. Leppin AL, Mahoney JE, Stevens KR, Bartels SJ, Baldwin L-M, Dolor RJ, et al. Situating dissemination and implementation sciences within and across the translational research spectrum. J Clin Transl Sci. (2019) 4:152–8. doi: 10.1017/cts.2019.392
13. NIH. Dissemination and Implementation Research in Health. PAR-19-274. (2019). Available online at: https://grants.nih.gov/grants/guide/pa-files/PAR-19-274.html (accessed May 03, 2022).
14. Guerin RJ, Glasgow RE, Tyler A, Rabin BA, Huebschmann AG. Methods to improve the translation of evidence-based interventions: a primer on dissemination and implementation science for occupational safety and health researchers and practitioners. Saf Sci. (2022) 152:105763. doi: 10.1016/j.ssci.2022.105763
15. Betit E, Bunting J, Fletcher MK, Barlet G, Tolentino-Gonzalez K. Summer Report: Research to Practice (r2p) in Construction: Science, Strategies and Partnerships to Advance Safety and Health. Silver Spring, MD: CPWR-The Center for Construction Research and Training (2019).
16. Sorensen G, McLellan DL, Dennerlein JT, Nagles EM, Sabbath EL, Pronti NP, et al. A conceptual model for guiding integrated interventions and research. Pathways through conditions of work. In: Hudson HL, Nigam JAS, Sauter SL, Chosewood LC, Schill AL, Howard J, editors. Total Worker Health. Washington: APA (2019). p. 91–106.
17. Brownson RC, Colditz GA, Proctor EK, (eds). Dissemintation and Implementation research in Health: Translating Science to Practice. New York, NY: Oxford University Press (2012) 49.
18. NIOSH. Current Intelligence Bulletin 63: Occupational Exposure to Titanium Dioxide. Cincinnati, OH: U.S. Department of Health and Human Services, Centers for Disease Control and Prevention, National Institute for Occupational Safety and Health, DHHS (NIOSH) Publication No. 2011–160 (2011).
19. NIOSH. Current Intelligence Bulletin 65: Occupational Exposure to Carbon Nanotubes and Nanofibers. Cincinnati, OH:, U.S. Department of Health and Human Services, Centers for Disease Control and Prevention, National Institute of Occupational Safety and Health, DHHS (NIOSH) Publication No. 2013–145 (2013).
20. WHO. WHO Guidelines on Protecting Workers from Potential Risks of Manufactured Nanomaterials. Geneva: World Health Organization (2017).
21. Renn O, Roco MC. Nanotechnology and the need for risk governance. J Nanopart Res. (2006) 8:153–91. doi: 10.1007/s11051-006-9092-7
22. IRGC. White Paper on Nanotechnology Risk Governance. Geneva: International Risk Governance Council (2006).
23. Howard J, Piacentino J, Schulte P, MacMahon K. Using systematic review in occupational safety and health. Am J Ind Med. (2017) 60:424–9. doi: 10.1002/ajim.22771
24. Chambers DA, Glasgow RE, Strange KC. The dynamic sustainability framework: addressing the paradox of sustainment amid ongoing change. Implement Sci. (2013) 8:117. doi: 10.1186/1748-5908-8-117
25. Keisler R, Glasgow RE. A proposal to speed translation of healthcare research into practice: dramatic change is needed. Am J Prev Med. (2011) 40:637–44. doi: 10.1016/j.amepre.2011.02.023
26. Allan RW, Barn PK, Lamphear BP. Randomized controlled trial in environmental health research: unethical or underutilized. PLoS Med. (2015) 12:e1001775. doi: 10.1371/journal.pmed.1001775
27. Yassi A, O'Hara LM, Engelbrecht MC, Uebel K, Nophal LE, Bryce E, et al. Considerations for preparing randomized population health intervention trials lessons from a South African-Canadian partnership to improve the health of workers. Glob Health Action. (2014) 7. doi: 10.3402/gha.v7.23594
28. Brown CH, Curran G, Palinkas LA, Aarons GA, Wells KB, Jones L, et al. An overview of research and evaluation designs for dissemination and implementation. Annu Rev Public Health. (2017) 38:1–22. doi: 10.1146/annurev-publhealth-031816-044215
29. Fort DG, Herr TM, Shaw PL, Gutzman KE, Starren JB. Mapping the evolving definitions of translational research. J Clin Transl Sci. (2017) 1:60–6. doi: 10.1017/cts.2016.10
30. Guerin RJ, Harden SM, Rabin BA, Rohlman DC, Cunningham TR, Te Poel MR, et al. Dissemination and implementation science approaches for advancing total worker health in the United States. Int J Environ Res Public Health. (2021) 18:11050. doi: 10.3390/ijerph182111050
31. Khoury MJ, Gwinn M, Yoon PW, Dowling N, Moore CA, Bradley L. The continuum of translation research in genomic medicine: how can we accelerate the appropriate integration of human genome discoveries into health care and disease prevention? Genet Med. (2007) 9:665–74. doi: 10.1097/GIM.0b013e31815699d0
32. Estabrook PA, Brownson RC, Pronk NP. Dissemination and implementation science for public health professionals: an overview and call to action. Prev Chronic Dis. (2018) 15:180525. doi: 10.5888/pcd15.180525
33. Fixsen DL Naoom SF Blas é KA Friedman RM and Wallace F. Implementation Research: A Synthesis of the Literature. Tampa, FL: University of South Florida, Louis de la Parte Florida Mental Health Institute, The National Implementation Research Network, FMHI Publication No. 231 (2005).
34. Gaglio B, Shoup JA, Glasgow RE. The RE-AIM and framework: a systematic review of use over time. Am J Public Health. (2013) 103:e38–46. doi: 10.2105/AJPH.2013.301299
35. Westfall JM, Mold J, Fagnan L. Practice-based research—“Blue Highways” on the NIH roadmap. JAMA. (2007) 297:403–6. doi: 10.1001/jama.297.4.403
36. Iavicoli I, Leso V, Piacci M, Cioffi DL, Canu IG, Schulte PA. An exploratory assessment of applying risk management practices to engineered nanomaterials. Int J Environ Res Public Health. (2019) 16:3290. doi: 10.3390/ijerph16183290
37. Schulte PA, Geraci CL, Murashov V, Kuempel ED, Zumwalde RD, Castranova V, et al. Occupational safety and health criteria for responsible development of nanotechnology. J Nanopart Res. (2014) 16:2153. doi: 10.1007/s11051-013-2153-9
38. Tomellini R, Giordani J. Third International Dialogue on Responsible Research and Development of Nanotechnology. Brussels: European Commission (2008).
39. Woolf SH. The meaning of translational research and why it matters. JAMA. (2008) 299:211–3. doi: 10.1001/jama.2007.26
40. Ponce Del Castillo A. Nanomaterials and Workplace Health and Safety: What Are the Issues for Workers? Brussels: European Trade Union Institute (2013).
41. Fleischer T, Jahnel J, Seitz SB. Technology assessment beyond toxicology – the case of nanomaterials, in responsibility in nanotechnology development. In: Arnaldi S, Ferrari A, Magaudda P, Marin F, editors. The International Library of Ethics, Law and Technology. Dordrecht: Springer (2014). p. 79–96.
42. Forloni G. Responsible nanotechnology development. J Nanopart Res. (2012) 14:1007. doi: 10.1007/s11051-012-1007-1
43. Berube DM. Nano-Hype: The Truth Behind the Nanotechnology Buzz. Amherst: Prometheus Books (2006).
44. Harthorn BH. Nano-buzz: societal dimensions of emerging technologies. Anthropol News. (2006) 47:26. doi: 10.1525/an.2006.47.7.26
45. Tannert C, Elvers H-D, Jandrig B. The ethics of uncertainty: in the light of possible dangers, research becomes a moral duty. EMBO Rep. (2007) 8:892–6. doi: 10.1038/sj.embor.7401072
46. Kurath M. Nanotechnology governance: accountability and democracy in new modes of regulation and deliberation. STI Stud. (2009) 5:87–110. Available online at: https://www.alexandria.unisg.ch/256028/
47. Brownson RC. Bridging research and practice to implement strategic public health science. Am J Public HEalth. (2021) 111:1389–91. doi: 10.2105/AJPH.2021.306393
48. Curry SJ. Organizational interventions to encourage guideline implementation. Chest. (2000). 118(suppl. 2):405–65. doi: 10.1378/chest.118.2_suppl.40S
49. Anderson NB, Orleans CT, Gruman J. Roadmaps for the next frontier; getting evidence-based behavioral medicine in practice. In: Paper presented at: 20th Annual Meetings of the Society of Behavioral Medicine March 3-6, 1999. San Diego, CA (1999).
50. Lee KP, Henry NWIII, Trochimowicz HJ, Reinhardt CF. Pulmonary response to impaired lung clearance in rats following excessive TiO[[sb]]2[[/s]] dust disposition. Environ Res. (1985) 41:144–69. doi: 10.1016/S0013-9351(86)80177-3
51. Heinrich U, Fuhst R, Rittenhausen S, Creutzenberg O, Bellman B, Koch W, et al. Chronic inhalation exposure of Wistar rats and two different strains of mice to diesel engine exhaust, carbon black, and titanium dioxide. Inal Toxicol. (1995) 74:533–56. doi: 10.3109/08958379509015211
52. Wang L Davidson DC Castranova V and Rojanasakul Y. Pulmonary effects of carbon nanomaterials. In: Chen C, Wang H, editors. Biomedical Applications and Toxicology of Carbon Nanomaterials. Weinheim: Wiley-VCH Verlag GmbH and Co. KGaA (2016). p. 163–193.
53. Beard JD, Erdely A, Dahm MM, de Perio MA, Birch ME, Evans DE, et al. Carbon nanotube and nanofiber exposure and sputum and blood biomarkers of early effect among US workers. Environ Int. (2018) 116:214–28. doi: 10.1016/j.envint.2018.04.004
54. Wheeler MW, Bailer AJ. Properties of model-averaged BMDs: a study of model averaging in dichotomus response risk estimation. Risk Anal. (2007) 27:659–70. doi: 10.1111/j.1539-6924.2007.00920.x
55. Akaike H. A Bayesian analysis of the minimum AIC procedure. Ann Inst Stat Math. (1978) 30:9–14. doi: 10.1007/BF02480194
56. Dahm MM, Schubauer-Berigan MK, Evans DE, Birch ME, Bertke S, Beard JD, et al. Exposure assessments for a cross-sectional epidemiological study of US carbon nanotube and nanofiber workers. Int, J Hyg Env Health. (2018) 221:429–40. doi: 10.1016/j.ijheh.2018.01.006
57. Damschroder LJ Reardon CM and Lowery JC. The Consolidated Framework for Implementation Research (CFIR). Handbook on Implementation Science CH 4. Edward Elgar Publishing (2020).
58. Nilson P. Making sense of implementation theories, models, frameworks. Implement Sci. (2015) 10:53. doi: 10.1186/s13012-015-0242-0
59. Nilson P, Bernhardsson S. Context matters in implementation science: a scoping review of determinant frameworks that describe contextual determinants for implementation outcome. BMC Health Serv Res. (2019) 19:189. doi: 10.1186/s12913-019-4015-3
60. Brownson RC, Colditz GA, Proctor EK. Dissemination and Implementation Research in Health. 2nd ed. New York, NY: Oxford Express (2017). p. 19–46.
61. McCreight M, Rabin B, Glasgow, Ayele R, Leonard C, Gilmartin H, et al. Using the Practical, Robust Implementation and Sustainability Model (PRISM) to qualitatively assess multilevel contextual factors to help plan, implement, evaluate, and disseminate health services programs, Transl Behav Med. (2019) 9:1002–11. doi: 10.1093/tbm/ibz085
62. Rabin BA, Glasgow RE, Kerner JF, Klump MP, Brownson RC. Dissemination and implementation research on community-based cancer prevention: a systematic review. Am J Prev Med. (2010) 38:443–56. doi: 10.1016/j.amepre.2009.12.035
63. Birken SA, Powell BJ, Presseau J, Kirk MA, Lorencatto F, Gould NJ, et al. Combined use of the consolidated framework for implementation research (CFIR) and the theoretical domains framework (TDF): a systematic review. Implement Sci. (2017) 12:2. doi: 10.1186/s13012-016-0534-z
64. Glasgow RE, Chambers D. Developing robust, sustainable implementation systems using rigorous, rapid and relevant science. Clin Transl Sci. (2012) 5:48–55. doi: 10.1111/j.1752-8062.2011.00383.x
65. Feldstein AC, Glasgow RE. A practical, robust implementation and sustainability model (PRISM) for integrating research findings into practice. Jt Comm J Qual Patient Saf. (2008) 34:228–43. doi: 10.1016/S1553-7250(08)34030-6
66. Aarons GA, Hurlburt M, Horwitz SM. Advancing a conceptual model of evidence-based practice implementation in public service sectors. Adn Policy Mental Health. (2011) 38:4–23. doi: 10.1007/s10488-010-0327-7
67. Moullin JC, Dickson KS, Stadnick NA, Rabin B, Aarons GA. Systematic review of the exploration, preparation, implementation, sustainment (EPIS) framework. Implement Sci. (2019) 14:1. doi: 10.1186/s13012-018-0842-6
69. Michie S, Richardson M, Johnston M, Abraham C, Francis J, Hardeman W, et al. The behavior change technique taxonomy (v1) of 93 hierarchically clustered techniques: building an international consensus for reporting behavior change interventions. Ann Behav Med. (2005) 46:81–95. doi: 10.1007/s12160-013-9486-6
70. Strifler L Cardoso R McGowan J Logo E Nincic V and Khan PA . Scoping review identifies significant number of knowledge translation theories, models, and frameworks with limited use. J Clin Epidemiol. (2018) 100:92–102. doi: 10.1016/j.jclinepi.2018.04.008
71. Neta G, Brownson RC, Chambers DA. Opportunities for epidemiologists in implementation science: a primer. Am J Edidemiol. (2018) 187:899–910. doi: 10.1093/aje/kwx323
72. Van Eerd D, Moser BA, Saunders R. A research impact model for work and health. AJIM. (2021) 64:3–12. doi: 10.1002/ajim.23201
Keywords: dissemination, implementation, nanotechnology, research, impact
Citation: Schulte PA, Guerin RJ, Cunningham TR, Hodson L, Murashov V and Rabin BA (2022) Applying Translational Science Approaches to Protect Workers Exposed to Nanomaterials. Front. Public Health 10:816578. doi: 10.3389/fpubh.2022.816578
Received: 16 November 2021; Accepted: 25 April 2022;
Published: 10 June 2022.
Edited by:
Robert McCunney, Brigham and Women's Hospital and Harvard Medical School, United StatesReviewed by:
Il Je Yu, Independent Researcher, Icheon, South KoreaDerk Henri Brouwer, University of the Witwatersrand, South Africa
Copyright © 2022 Schulte, Guerin, Cunningham, Hodson, Murashov and Rabin. This is an open-access article distributed under the terms of the Creative Commons Attribution License (CC BY). The use, distribution or reproduction in other forums is permitted, provided the original author(s) and the copyright owner(s) are credited and that the original publication in this journal is cited, in accordance with accepted academic practice. No use, distribution or reproduction is permitted which does not comply with these terms.
*Correspondence: Paul A. Schulte, cGFzNEBjZGMuZ292