- 1Department of Kinesiology, University of British Columbia, Kelowna, BC, Canada
- 2International Collaboration on Repair Discoveries, Vancouver Coastal Health Research Institute, Vancouver, BC, Canada
- 3The Healthcare Improvement Studies Institute, University of Cambridge, Cambridge, United Kingdom
- 4Department of Kinesiology, McMaster University, Hamilton, ON, Canada
- 5Department of Anesthesiology, Pharmacology, and Therapeutics, University of British Columbia, Vancouver, BC, Canada
- 6Department of Medicine, Division of Physical Medicine and Rehabilitation, University of British Columbia, Vancouver, BC, Canada
- 7Centre for Chronic Disease Prevention and Management, University of British Columbia, Kelowna, BC, Canada
Introduction: Persons with spinal cord injury (SCI) often report high levels of neuropathic pain (NP) and poor well-being, which may result from increased inflammation. This study examined the impact of sub-maximal aerobic exercise on NP, inflammation and psychological affect among adults with SCI.
Methods: Eight active adults with tetraplegia (n-4, AIS A-C) and paraplegia (n = 4, AIS A-C) performed 30-min of arm-crank aerobic exercise and reported their ratings of perceived exertion (RPE) each minute. Measures of NP, affect, and inflammatory cytokines (IL-6, IL-10, IL-1ra, TNF-α) were taken pre-(T0), immediately post-(T1), and 90-min post-exercise (T2).
Results: NP decreased between T0 and T1 for tetraplegics (−60%, d = 0.47; CI = −0.32, 2.02) and paraplegics (−16%, d = 0.15; CI = −0.30, 0.90). Correlations between change in cytokines and change in NP were medium-to large for tetraplegics (rs ranged from −0.820 to 0.965) and paraplegics (rs ranged from −0.598 to 0.833). However, the pattern of correlations between change in cytokines and affect was inconsistent between groups. Lower baseline levels of IL-1ra predicted greater decreases in NP immediately post-exercise (r = 0.83, p = 0.01).
Conclusion: Sub-maximal exercise can positively impact NP for some persons with SCI. Further experimental research should identify the optimal exercise intensity to reduce NP for persons with SCI, in addition to understanding biomarkers which may predict changes in NP.
Clinical Trial Registration: www.ClinicalTrials.gov, identifier NCT03955523.
Introduction
Neuropathic pain is caused by a lesion or disease of the somatosensory nervous system (1) and commonly manifests as allodynia (pain resulting from a non-noxious stimulus) and hyperalgesia [heightened response from a noxious stimulus; (2)]. Approximately 75% of persons with spinal cord injury (SCI) experience neuropathic pain (3), with many reporting pain to be more debilitating than the injury itself. Individuals with SCI who experience greater pain also report more negative affect [instantaneous displeasure that lacks cognitive appraisal; (4)] and susceptibility to developing mood disorders, such as depression or anxiety (5).
Current treatment options for neuropathic pain are primarily pharmaceutical; however, in addition to eliciting debilitating side effects, pharmaceuticals result in just 50% pain reduction for only 30% of individuals with SCI-related chronic neuropathic pain (6). Consequently, many individuals who experience neuropathic pain prefer non-pharmaceutical alternatives (7). In a survey of 90 adults with SCI, 79% of those who reported on their preference for neuropathic pain management, preferred non-pharmaceutical treatments (8). Together, these data demonstrate the need to identify alternative treatment options to treat neuropathic pain among individuals with SCI.
One potential treatment to alleviate SCI neuropathic pain, improve affect, and potentially reduce risk for mood disorders (9, 10) is exercise. Exercise has been recognized as having positive effects on neuropathic pain among persons with SCI, despite the limited quantity and quality of evidence (11). However, evidence is emerging which indicates that exercise induced hypoalgesia may exist for persons with SCI. For example, in a case series, participants with SCI reported decreased neuropathic pain sensations following at least one of two bouts of self-selected, community-based exercise performed within a single week (9). The neuropathic pain-modulating benefits of acute exercise appear to persist with exercise training, as 10 weeks of aerobic exercise training led to decreased neuropathic pain for adults with SCI upon completion of the intervention (12). Various biological mechanisms underlying exercise induced hypoalgesia have been explored among able-bodied individuals, with evidence suggesting an interplay between the opioid, endocannabinoid, serotonergic and immune systems (13–15). However, the explanations for why exercise modulates neuropathic pain among persons with SCI are currently unknown.
The mechanisms underpinning neuropathic pain development are not well-understood. Various hypotheses exist in attempts to explain neuropathic pain development including structural damage within the central nervous system, and somatosensory cortex reorganization (16). However, the nociceptive environment also impacts the presence and severity of neuropathic pain (17). Pro-inflammatory cytokines such as interleukin-6 (IL-6), and tumor necrosis factor-alpha (TNF-α) have been shown to induce hyperalgesia, thereby increasing levels of neuropathic pain (18). In contrast, a reduction in these pro-inflammatory cytokines has been shown to prompt analgesic effects (17, 18). This relationship has also been demonstrated among persons with SCI using a dietary intervention. Relative to a control group, participants who consumed an anti-inflammatory diet for 12 weeks experienced changes in sensory neuropathic pain as a function of the change in pro-inflammatory cytokines (19). It is not known whether exercise can elicit the same effects in people with SCI.
In persons without SCI, research consistently shows that exercise can lead to increased acute levels of circulating IL-6, and subsequent rises in plasma concentrations of anti-inflammatory cytokines IL-1 receptor antagonist (IL-1ra), IL-10, and soluble TNF receptors (20, 21). However, exercise needs to be performed at a minimum intensity (>50% VO2max) or duration (>30 min) for the anti-inflammatory response (21), analgesic effects (22) and improvements in affect (23) to occur. For persons with SCI above the 6th thoracic vertebrae (T6), the loss of somatic and autonomic control frequently results in a blunted cardiovascular response to exercise (24). Additionally, research evidence is inconclusive regarding the role and extent that the sympathetic nervous system plays in exercise-related changes in inflammation (25, 26) and subsequent analgesic effects. Although exercise can induce analgesia through upregulation of anti-inflammatory cytokines and reduced microglial activation in the central nervous system (27), it is unclear whether persons with SCI above T6 experience an attenuated analgesic response to exercise. Taken together, it is important to examine if any biomarkers can be used to predict who may experience exercise-related improvements in neuropathic pain following SCI.
The debilitating nature of neuropathic pain, coupled with the bidirectional relationship between pain and affect, highlight the need for understanding neuropathic pain as having both physiological and psychosocial contributors. Therefore, the primary purpose of this study was to test the effect of a single bout of sub-maximal aerobic exercise on inflammatory cytokines, neuropathic pain, and affect among individuals with SCI. Additionally, an exploratory aim of this study was to assess the relationship between baseline levels of cytokines and changes in neuropathic pain from pre- to post-exercise. Sub-maximal aerobic exercise was implemented in order to align with exercise guideline recommendations for people with SCI (28). It was hypothesized that a bout of sub-maximal aerobic exercise would lead to an acute increase in circulating levels of IL-6 and anti-inflammatory cytokines, decreased neuropathic pain, and improved affect from pre- to post-exercise among persons with SCI. Additionally, it was hypothesized that decreased neuropathic pain would be correlated with increased anti-inflammatory cytokines and improved affect.
Methods
Participants
To participate in this study, individuals were required to: (1) have incurred an SCI > 12 months ago with an injury at the third cervical level or below (as long as diaphragmatic control and arm functioning allowed upper-body exercise); (2) experience chronic below-level of SCI neuropathic pain (>3 months) (at-level of SCI pain was excluded to minimize the risk of pain misidentification). Chronic neuropathic pain was an inclusion criterion given that neuropathic pain typically persists >3 months for persons with SCI, and to ensure that pain did not spontaneously resolve between testing sessions; (3) individuals were required to have the ability to read/write in English; and (4) routinely achieve at least the lowest level of the SCI exercise guidelines consisting of 20 min of moderate-to-vigorous intensity aerobic activity two times per week, and strength training two times per week, consisting of three sets of 8–10 repetitions of each exercise for each major muscle group (28). The latter criterion was instated because acute exercise participation by chronically inactive individuals may induce pain “flare-ups” (29) and impact inflammatory profiles.
Participants were recruited January–March, 2019 through advertisements emailed from community organizations from across British Columbia, and through emails directed toward individuals who had previously expressed interest in participating in SCI-studies. After screening, 10 active individuals with SCI of >1-year duration participated in this study. Ten individuals were the desired sample size based on previous case series data (9). This study carried the approval of the UBC Clinical Research Ethics Board (CREB; H18-03191), whereby all experiments were performed in accordance with CREB guidelines and regulations. Participants provided written, informed consent prior to enrolling in this study.
Procedural Overview
This study is a secondary analysis of case series data (clinicaltrials.gov registered: NCT03955523; 20/05/2019).
Measures
Inflammatory Cytokines
Blood samples were drawn by a trained phlebotomist from participants' most accessible antecubital vein. Samples were collected in the same clinical laboratory room as the exercise bout. All biosafety hazard protocols were followed, and JL's laboratory held a UBC-approved biosafety permit for this research space. Samples were placed in EDTA tubes and centrifuged at 2,000 g for 15 min at 4°C (Eppendorf, Hamburg, Germany), followed by a subsequent centrifuge at 10,000 g for 10 min at 4°C to remove platelets. The resultant supernatant was subsequently aliquoted and stored at −80°C. IL-6, IL-1ra and TNF-α were analyzed and quantified using the U-PLEX metabolic group 1 assay kits (K151ACL-1, LOT 289109, Mesoscale, Maryland, USA) according to manufacturers' instructions, and read using an MSD QuickPlex SQ120 plate reader (Mesoscale, Maryland, USA). All samples were analyzed in duplicate. The plate-specific intra-assay coefficients of variation (CV) were as follows: IL-10 = 10.4%; IL-1ra = 5.91%; IL-6 = 13.73%; TNF-α = 17.84%. The combined CV was 11.97%.
Neuropathic Pain Scale
Participants' neuropathic pain was measured using a modified version of the Neuropathic Pain Scale [NPS; (30)]. This 10-item scale (0 = nothing at all, 10 = most intense sensation imaginable) measures pain sensations common to neuropathic pain (e.g., “burning,” “dull,” “deep”) in addition to measuring general pain qualities (i.e., “intensity” and “unpleasantness”). For each item on the NPS, participants were asked to verbally state how their pain sensation felt at that very instant. The first author (KRT) recorded each response. One question regarding the temporal nature of neuropathic pain was excluded, because it was not meaningful given the acute nature of the present study. Item scores were averaged to form a composite pain score at pre-, post- and 90-min post-exercise. The NPS has been shown to have sensitivity to detect acute treatment effects (30) and has been validated among people with various neuropathic pain syndromes (including SCI).
Affect
Hardy and Rejeski's (31) 11-point, single item Feeling Scale (FS) was used to measure participants' overall acute feeling of pleasure-displeasure (−5 = very bad, +5 = very good). In addition, Svebak and Murgatroyd's (32) Felt Arousal Scale (FAS) measured participants' perceived activation (1 = low arousal, 6 = high arousal), whereby “1” indicates feeling bored, relaxed or calm, and “6” indicates feeling excited, angry or frustrated. Using the FS alongside the FAS enhances construct validity by measuring activation in addition to affective valence [pleasure-displeasure; (33)]. Although the FS and FAS have not been validated in the SCI population, previous research has demonstrated the utility of these questionnaires for measuring affect in individuals with SCI participating in acute exercise (9, 10).
Protocol
Peak Power Output Graded Aerobic Exercise Test
Upon arriving at the lab, participants were given opportunity to use the toilet. After 5 min of seated rest, resting heart rate and blood pressure were measured. KRT explained how to use the Borg Rating of Perceived Exertion [RPE; (34)] (6–20) scale, adapted for persons with SCI (35). Participants then began the peak power output exercise test using a wall-mounted arm ergometer (Lode Angio, Groningen, Netherlands), with the height adjusted to align with the acromioclavicular joint. If required, participants' hands were bound to the handles of the arm ergometer with elastic tensor bandages. The test began with a 5-min warm-up at a self- selected cadence. Using a continuous graded exercise protocol (36), the resistance on the ergometer was adjusted such that power output was increased by 2 W/min for tetraplegics (37) and 10 W/min for paraplegics (38). Participants were asked to maintain a cadence of 55–65 revolutions per min (RPM) until volitional exhaustion. Within the last 10-s of each min of exercise, participants reported their RPE. The maximum wattage achieved during this exercise test was used to calculate the intensity (60% of maximum) of each participant's subsequent sub-maximal intensity aerobic exercise bout (operationalized as 60% peak power output).
Sub-maximal Aerobic Exercise Bout
Prior to arriving at the lab to complete the sub-maximal exercise bout, participants fasted for 12-h. Upon arrival, participants rested quietly for 10 min. Next, participants completed their baseline (T0) measurements which consisted of verbally reporting their NPS, FS, and FAS ratings. Blood samples (TNF- α, IL-6, IL-1ra, IL-10) were then collected by the trained phlebotomist. Prior to exercising, KRT reminded participants how to use the “6–20” RPE scale (34, 35). For the exercise bout, participants performed a 5-min warm-up at a self-selected pace and then performed 30-min of arm crank exercise at 60% of their maximum wattage, while maintaining a cadence of 55–65 RPM. Participants were prompted to report their RPE at the end of each minute during this exercise bout. If participants reported their RPE to be above “sub-maximal” (>16), the wattage was electronically lowered to 50% of participants maximum wattage, until their RPE recovered to “12–14,” Immediately following exercise (T1), participants verbally reported their NPS, FS, and FAS ratings, and provided another blood sample. Participants were then asked to quietly rest in a room separate from the testing room, and watch a video [“Planet Earth”; (BBC America)] for 90 min. After 90-min (T2), participants repeated the NPS, FS, and FAS measures, and their blood was drawn one final time. The order of administration of the NPS, FS, and FAS was systematically randomized at each measurement timepoint to control for presentation biases. See Figure 1 for graphical representation of study protocol.
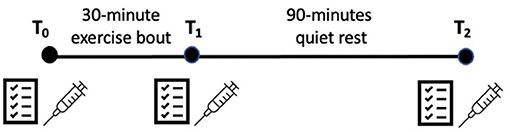
Figure 1. Graphical representation of sub-maximal exercise protocol. Questionnaire symbol = neuropathic pain scale, felt arousal scale and feeling scale; syringe symbol = blood draws.
Statistical Analyses
One-way repeated measures analysis of variance (ANOVA) with planned contrasts were conducted to assess change in neuropathic pain, cytokines and affect from pre- to post-exercise. Planned contrasts were the appropriate statistical method given that they allow for comparisons of just two means (of a set of means >2). The hypotheses of this study were based on changes in each dependent variable from pre- to post-exercise (i.e., not T1T2). Therefore, computing planned contrasts allowed for scientifically sensible comparisons and minimized the risk of a Type 1 error. Significance was set at p < 0.05. Effect sizes were calculated as Cohen's dav(average) with Hedge's gav correction applied (39) and interpreted according to Cohen's conventions [small = 0.20, medium = 0.50, large = 0.80; (40)].
Simple change scores (Δ) were calculated for change in cytokine levels between T0T1 and T0T2. To control for the correlation between baseline and subsequent measures of the self-reported variables, residualized change scores were computed to measure change in NPS, FS, and FA scores between T0T1 and T0T2. Pearson's correlation coefficients were then computed to determine: (a) the relationships between change in neuropathic pain, change in inflammatory cytokines, and change in affect and arousal at timepoints T0T1, and T0T2, and (b) whether baseline levels of inflammatory cytokines were related to change in neuropathic pain at timepoints T0T1 and T0T2. Two-tailed tests were used. Consistent with Widerstrom-Noga's recommendations (41), analyses were conducted separately for persons with tetraplegia and paraplegia. Cohen's conventions (40) were used for interpreting the magnitude of the correlations (small = 0.1, medium = 0.3, large = 0.5). SPSS version 22.0 was used for all analyses.
Results
One male participant with tetraplegia withdrew from the study due to a pressure sore (unrelated to the study protocol). Additionally, the phlebotomist was unable to obtain blood from one male participant; his data are not included in the analyses. Therefore, the following results include data from 8 participants [4 tetraplegics (all male), and 4 paraplegics (3 male, 1 female)]. The average age of participants was 37.9 ± 10.9 years, and ranged between 25 and 56 years. Participants' average years post-SCI was 17.8 ± 8.9, and ranged between 3 and 36 years. Three participants were consuming cannabis at the time of study participation, however, no participants were taking pharmaceuticals at the time of study participation. Participant demographics for the 8 people who completed the study are presented in Table 1.
Exercise Intensity Manipulation Check
Manipulation checks were used to verify that participants were exercising at the intended training intensity. MeanRPE during the exercise bout did not significantly differ between tetraplegic and paraplegic participants (MRPE tetra = 13.07 ± 1.10, MRPE para = 13.73 ± 0.69; t = −1.02, p = 0.35). Similarly, percent change in RPE between T0T1 did not significantly differ between tetraplegic and paraplegic participants [%change tetra = 47.6 ± 24.49, %change para = 47.48 ± 35.40; F(1, 6) = 0.702, p = 0.99]. MaxRPE (i.e., highest RPE reported during the exercise bout) for tetraplegic participants ranged from 12 to 15, indicating they were all exercising at a “somewhat hard to hard intensity” (32). For the paraplegic participants, MaxRPE ranged from 16 to 20, indicating that at certain points, they were exercising at “very hard intensity to exhaustion” (34). Results from an independent samples t-test indicated that average MaxRPE values for these two groups were significantly different (Tetra: M = 14.00 ± 1.4; Para: M = 17.25 ± 1.89; t = −2.71, p = 0.03). These data indicate that, consistent with the goal of the exercise manipulation, tetraplegic participants continuously exercised at a sub-maximal exercise intensity. However, paraplegic participants exceeded a sub-maximal intensity during their exercise bout (see 2-min average RPEs in Figure 2), and at points, may have exercised closer to maximal intensity.
Direct Effects of Exercise on NPS Scores, Affect, Arousal, and Inflammatory Cytokines
Given the small number of participants, results are reported and interpreted based on the magnitude of the effect sizes. Data are shown in Tables 2–5.
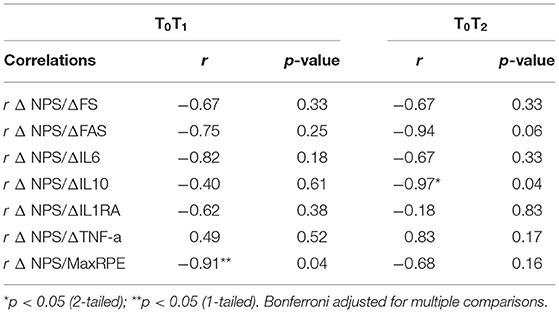
Table 3. Correlations between changes in pain, changes in affect, changes in arousal, and changes in cytokines among persons with tetraplegia (n = 4).
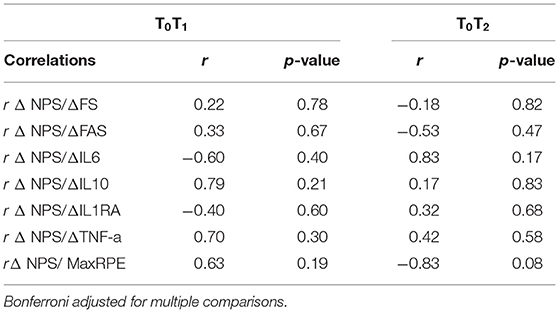
Table 5. Correlations between changes in pain, changes in affect, changes in arousal, and changes in cytokines among persons with paraplegia (n = 4).
For persons with tetraplegia, between T0T1 there was some evidence of clinically meaningful changes in neuropathic pain [i.e., reductions > 30%; (42)] and improvements in affect and arousal. Effect sizes for changes in NPS scores, affect, and arousal between T0T1 were medium-to large (gav = −0.47, 0.34, and 0.89, respectively). Changes in inflammatory cytokines between T0T1 were very small (gav range: 0.02–0.16). Between T0T2, effect sizes for changes in NPS scores and affect were very-small to small (0.07 and 0.17, respectively). Effect sizes for arousal did not change between T0T2. Effect sizes for changes in inflammatory cytokines between T0T2 were also very-small (gav range: 0.02–0.09).
For persons with paraplegia, calculation of the effect sizes provided support for some notable changes in pain, affect, and cytokine measures at both time points. Between T0T1, the effect size for change in NPS scores was small (−0.15), and medium for affect and arousal (−0.51, 0.43, respectively). Effect sizes for change in inflammatory cytokines between T0T1 were very-small (0.08–0.17), except for IL-6 which was medium-large (0.66). Between T0T2, change in NPS scores were small- to medium (−0.32), and change in arousal was medium (−0.51). Effect sizes for affect did not change between T0T2,. Effect sizes for change in T0T2 cytokines were very small (0.03–0.18), except for IL-6 which was medium-large (0.66).
Correlations Between Change in Inflammatory Cytokines and Change in Neuropathic Pain
For persons with tetraplegia, there were medium-to large, negative correlations between change in cytokine levels and change in NPS scores between T0T1 (rs ranged from −0.82 to 0.49), and T0T2 (rs > −0.67), except for IL-1ra (r = −0.18; Table 3).
For persons with paraplegia, correlations between change in T0T1 levels of inflammatory cytokines and change in NPS scores were medium to large (rs ranged from −0.60 to 0.79; Table 5) but were not in a consistent direction immediately post-exercise. However, correlations between change in NPS scores and change in levels of cytokines between T0T2 were small to medium (rs ranged from 0.17 to 0.42), except for IL-6 which was large (r = 0.83).
Correlations Between Change in Neuropathic Pain and Change in Affect
For persons with tetraplegia, as hypothesized, there were medium- to large, negative correlations between change in NPS scores, and change in FS and FA scores between T0T1, (rs > −0.67), and T0T2 (rs > −0.67).
For persons with paraplegia, between T0T1, small- to-medium positive correlations were observed between change in NPS scores and change in FS scores (r = 0.22), and FA scores (r = 0.33). In contrast, between T0T2, small-to medium, negative correlations were observed between change in NPS scores and FS scores (r = −0.18) and FA scores (r = −0.53).
Post-hoc Correlations Between Maximum Ratings of Perceived Exertion and Change in Neuropathic Pain
The hypotheses of the current study were based on acute responses to exercise at a sub-maximal intensity. However, the manipulation check of MaxRPE data revealed tetraplegic participants were indeed exercising at a sub-maximal intensity, but paraplegic participants may have surpassed this sub-maximal level at certain points in the exercise bout, and approached an exercise intensity near maximal. As previous research among chronic pain populations indicates that extremely high exercise intensities may acutely increase experimentally induced pain (43, 44), a post-hoc decision was made to compute Pearson's correlations between MaxRPE and NPS scores to determine if the relationship between MaxRPE is different for tetraplegic vs. paraplegic participants.
For persons with tetraplegia, there were medium-to-large, negative correlations between MaxRPE and change in NPS scores between T0T1 (r = −0.91) and T0T2 (r = −0.68).
In contrast, for persons with paraplegia, a medium-to-large positive correlation was found between MaxRPE and change in NPS scores between T0T1 (r = 0.63). However, between T0T2, a large, negative correlation was observed between MaxRPE and change in NPS scores (r = −0.83).
Correlations Between Baseline Levels of Cytokines and Change in Neuropathic Pain
A large-sized significant, positive correlation was observed between baseline levels of IL-1ra and change in NPS scores between T0T1 (r = 0.833; Table 6; Figure 3). However, this relationship was not sustained 90-min post-exercise (r = −0.17). Correlations between baseline levels of all other cytokines and change in NPS scores were inconsistent in size and direction at timepoints T0T1 and T0T2 (rs = −0.27–0.55; Table 6).
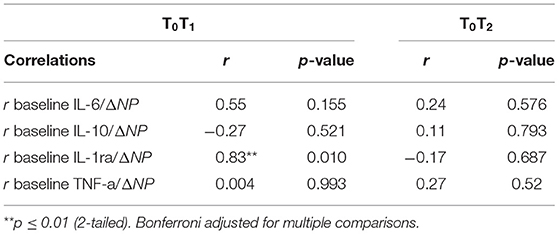
Table 6. Correlations between baseline levels of cytokines and changes in pain for all study participants (n = 8).
Discussion
The primary purpose of this study was to test the acute effects of sub-maximal aerobic exercise on inflammatory cytokines, neuropathic pain, affect and arousal among individuals with SCI. Additionally, this study aimed to explore if the baseline levels of cytokines may be related to exercise-related changes in neuropathic pain. Consistent with our hypotheses, exercise led to decreased levels of neuropathic pain, and improved affect, in both participants with tetraplegia and paraplegia. However, changes in inflammatory cytokines following exercise participation were inconsistent in direction. For example, a small-sized acute decrease in IL-6 was observed for persons with tetraplegia following exercise participation, whereas a medium-large acute increase in IL-6 was observed for persons with paraplegia. Interestingly, lower baseline levels of IL-1Ra were significantly related to greater acute decreases in neuropathic pain. To the best of our knowledge, this is the first SCI study to evaluate the effects of exercise on neuropathic pain, inflammatory cytokines and affect.
The observed positive effects of exercise on neuropathic pain align with previous SCI research (6, 7, 9–11). However, previous research suggests that exercise reduces neuropathic pain to a similar extent for paraplegics and tetraplegics. In our study, these effects were large for tetraplegics and small for paraplegics. These differences may be at least partly attributable to the significant differences in intensity at certain points of the exercise bout, as reported by tetraplegic vs. paraplegic participants.
Indeed, research in able-bodied individuals suggests that the analgesic effects of exercise are elicited once exercise exceeds a threshold of intensity. Moderate- to high-intensity exercise [but not maximal; (14, 45)], has been shown to lead to greater reductions in pain compared to lower intensity exercise in other chronic health populations. However, performing exercise that is perceived to be “very hard to exhaustive” may increase pain, at least in the short-term. Paraplegics reported exercising at a “very hard” subjective intensity at certain points during this exercise bout, and their neuropathic pain and MaxRPE were positively correlated immediately post-exercise. The correlation became negative at 90-min post-exercise, paralleling greater reductions in neuropathic pain at 90-min post-exercise for this group. Research suggests that exercise-induced hypoalgesia remains for ≤ 30 min after exercise, but may be impaired in individuals with chronic pain (14). Therefore, paraplegic participants may have highly exerted themselves during this bout of exercise, which inhibited exercise-induced hypoalgesia immediately after exercise completion. Exercising “near maximal” intensity, may have conflated paraplegic participants immediate post-exercise neuropathic pain reports, with muscle soreness. Ninety minutes of recovery may have allowed for sufficient rest, and for paraplegic participants to experience the benefits of high-intensity exercise, such as reduced neuropathic pain. Future research must continue to examine the impact of exercise intensity on neuropathic pain in persons with SCI, given the distinctly different relationships with neuropathic pain when participants met, vs. exceeded a “hard” (34) exercise intensity in this study.
Among the able-bodied population, research evidence indicates that exercise-related changes in IL-6 is intensity dependent (46), and IL-6 can be sizeably increased compared to baseline levels following high-intensity exercise (47). Results from this study support previous findings, given the large increase in IL-6 that occurred from pre- to post-exercise for persons with paraplegia (i.e., “very hard”) exercisers. IL-6 is an inflammation-controlling cytokine and stimulates the exercise-related anti-inflammatory cascade, which suggests that tetraplegic participants may not have been exercising at a high enough intensity to initiate increased levels of anti-inflammatory cytokines. Research evidence also indicates that exercise-related increases in IL-6, and the subsequent anti-inflammatory cascade is further responsible for eliciting analgesic effects (17, 18, 48). However, results from this study do not align with this evidence, given that a stronger correlation was observed between IL-6 and neuropathic pain for persons with tetraplegia. Therefore, conflicting correlations between neuropathic pain and inflammatory cytokines, coupled with the significant difference in MaxRPE reported between levels of injury, suggests the inflammatory etiology of exercise-related changes in neuropathic pain among persons with SCI may also be impacted by exercise intensity. Future research should test the mediating effects of inflammation, by identifying whether specific exercise intensities lead to decreases in neuropathic pain, through effects on the inflammatory cascade.
Although impaired autonomic nervous systems may contribute to the conflicting pattern of correlations observed for tetraplegic vs. paraplegic participants between neuropathic pain, affect and arousal, differences in exercise intensity reported between these groups may help further explain these correlations. The intensity of exercise required to stimulate optimal affective responses remains highly debated (43, 44, 49). For individuals with SCI, previous literature demonstrates that acute exercise-related decreases in pain are correlated with improvements in feeling states (9, 10). However, the intensity and type of exercise prescribed within these previous studies were inconsistent. For persons with tetraplegia, the large, negative correlations between neuropathic pain, affect and arousal align with previous research (9, 10). In contrast, for persons with paraplegia, exercise-related changes in neuropathic pain were positively correlated with changes in feeling states immediately post-exercise, and negatively correlated 90 min post-exercise. Indeed, moderate vs. high-intensity exercise has been shown to differentially impact affective responses and pain sensations in the general population (43). Future research should be directed toward understanding the impact of exercise intensity on these constructs among persons with SCI.
Further, the timepoint of assessments of affective responses may impact the interpretation of results. For participants with paraplegia, conflicting affective responses immediately post-exercise vs. 90-min post-exercise may be explained by the rebound model (49). High-intensity exercise often stimulates negative affective responses immediately post-exercise, whereas these responses become positive after a period of recovery. Paraplegic participants exercised near maximal intensity and may have experienced a disruption in physiological homeostasis immediately post-exercise. However, 90-min of rest may have been sufficient for paraplegics to recover and experience an affective “rebound.” While the intensity of exercise and timing of affective assessments are not presumed to fully explain participants' affective response to exercise nor its correlation with neuropathic pain, they are presented here as potential exercise protocol characteristics that may partially explain these relationships. Future psychophysiological SCI-exercise research should also investigate the impact of additional social-cognitive and physiological predictors of affective responses to exercise (e.g., exercise self-efficacy, thermoregulation, and ventilatory threshold) and their relationship with neuropathic pain.
In addition to its primary purpose, this study provided the opportunity to assess whether inflammatory cytokines may be used as predictive biomarkers to determine individuals likely to benefit from exercise. Lower levels of IL-1ra at baseline were associated with larger exercise- related reductions in neuropathic pain. IL-1ra is an anti-inflammatory cytokine which is stimulated by post-exercise increases in IL-6. Therefore, individuals who have lower baseline levels of IL-1ra may have a greater capacity for upregulation of IL-1ra in response to exercise, thereby inducing anti-inflammatory and subsequent analgesic effects. Results from this study support previous research among persons with pain (e.g., knee osteoarthritis), whereby levels of plasma IL-1ra have been shown to predict response to treatment (50). Future research, including the RCT associated with this pilot study (51), should continue to investigate IL-1ra as a potential predictive biomarker for exercise-related changes in neuropathic pain among persons with SCI. Better understanding predictive biomarkers will enhance our knowledge of who may experience improvements in neuropathic pain following exercise, and therefore inform patient care decisions (52).
Study Strengths and Limitations
This study has several strengths. First, collecting multiple measurements and observing possible pathways responsible for exercise-related changes in pain allowed for a deeper evaluation of the relationship between exercise and neuropathic pain among persons with SCI. Results of this study provide rationale to further examine potential mechanisms impacting exercise-related changes in neuropathic pain, such as whether the intensity of exercise leads to decreased neuropathic pain, through its effects on inflammation among individuals with SCI. Second, evaluating the effect of exercise on neuropathic pain among humans with SCI (rather than animals), allowed for use of clinically relevant measurement tools and the ability to measure spontaneous neuropathic pain. Evaluating animal models would have precluded evaluating psychosocial contributors to neuropathic pain, given the difficulty of assessing affective measures of neuropathic pain in pre-clinical research (53). Third, assessing the concomitant impact of exercise on neuropathic pain, inflammation and affect allowed for a greater understanding of the dynamic interaction among physiological (i.e., inflammation) and psychological (i.e., affect) contributors to neuropathic pain.
Despite these strengths, some limitations must be noted. First, participants' individual sub-maximal exercise intensity was based on their peak power output rather than their VO2peak. Although we intended for all participants to exercise at 60% peak power output, there was a significant difference in MaxRPE reported between tetraplegic and paraplegic participants at certain points, and paraplegic participants likely exceeded a sub-maximal exercise intensity. Further, paraplegic participants approached an “exhaustive” RPE (34) halfway through the exercise bout, which prompted the research team to decrease their wattage from 60 to 50% peak power output for 5 min to allow for a brief recovery period. Despite this limitation, the significantly different MaxRPE reported between tetraplegic vs. paraplegic participants provided valuable insight into the potential impact of exercise intensity on neuropathic pain, inflammatory cytokines, affect and arousal among individuals with SCI. Second, although International SCI Pain Data Sets have been introduced to measure neuropathic pain in adults with SCI (54), we employed the NPS due to its brevity, ease of comprehension, and ability to assess responses to treatment (30). Future research should incorporate the International SCI Pain Data Set in order to ensure proper identification of participants' pain, and to allow for comparison of results across studies (51). Third, the FS and FAS have been used extensively in exercise research to investigate individuals' affective responses to exercise. Additional research is needed to assess the validity of the FAS when used in exercise contexts with persons with SCI. Fourth, this study included a small number of participants, and only one female, which is limiting due to the research evidence supporting sex differences in post-exercise immune and pain responses (55, 56). While the ratio of male to female participants in this study is representative of the global SCI population (57), future SCI research must strengthen recruitment strategies to include more female participants and enhance the generalizability of findings. The small sample size may have influenced our results (in terms of both effect size and lack of statistical significance), and these results should be interpreted with caution as they were not all statistically significant. Fifth, this study evaluated only inflammation as a possible pathway responsible for exercise-related changes in neuropathic pain. It is understood that many additional mechanisms (e.g., microglial activation, cortisol levels, pain catastrophizing) may be responsible for exercise-related changes in neuropathic pain among adults with SCI. To progress toward mechanism-based treatment, future SCI research should investigate further mechanisms that may impact the relationship between exercise and neuropathic pain. And finally, we did not employ a control condition against which to evaluate the effects of the exercise bout. Designing a true control for tests of pain-reducing interventions is challenging, but should be considered in future studies to control for the effects of attention, distraction and other psychological variables on neuropathic pain and affect.
Conclusion
Taken together, the results of this study suggest that exercise may reduce neuropathic pain and improve affect in adults with SCI, and changes in inflammation may be related to these effects. Additionally, exercise intensity may play an important role in the exercise related changes in neuropathic pain, inflammatory profiles, and affect for adults with SCI. Last, levels of IL-1ra may help determine who experiences exercise-related reductions in neuropathic pain sensations. Future research should be directed toward understanding the ideal exercise intensity for decreasing neuropathic pain among adults with SCI, and the potential role of inflammatory cytokines and other possible mediators.
Data Availability Statement
The raw data supporting the conclusions of this article will be made available by the authors, upon reasonable request.
Ethics Statement
The studies involving human participants were reviewed and approved by University of British Columbia Clinical Research Ethics Board. The patients/participants provided their written informed consent to participate in this study.
Author Contributions
KT was responsible for designing the study protocol, writing the protocol and report, participant recruitment, data collection, analyzing data, interpreting results, writing manuscript, updating reference lists, and creating tables and figures. JV, JL, and JK were responsible for designing the study protocol, interpreting results, and manuscript revision. JW was responsible for designing the study protocol, assisting with data collection, interpreting results, and manuscript revision. GJ was responsible for designing the study protocol, analyzing data, and manuscript revision. GD was responsible for designing the study protocol, assisting with data collection and analysis, and revising the manuscript. KMG was responsible for designing the protocol, assisting with writing the protocol and report, analyzing data, interpreting results, manuscript revision, and designing tables and figures. All authors contributed to the conception and design of this study, in addition to drafting, or critically revising the article.
Funding
This work was supported by the Rick Hansen Foundation through the Blusson Integrated Cures Partnership (2019).
Conflict of Interest
The authors declare that the research was conducted in the absence of any commercial or financial relationships that could be construed as a potential conflict of interest.
Publisher's Note
All claims expressed in this article are solely those of the authors and do not necessarily represent those of their affiliated organizations, or those of the publisher, the editors and the reviewers. Any product that may be evaluated in this article, or claim that may be made by its manufacturer, is not guaranteed or endorsed by the publisher.
Supplementary Material
The Supplementary Material for this article can be found online at: https://www.frontiersin.org/articles/10.3389/fresc.2021.700780/full#supplementary-material
References
1. Merksey H, Bogduk N. Part III: pain terms, a current list with definitions and notes on usage. Classification of chronic pain, second edition. IASP Task Force. (1994) 3:15–20.
2. Jensen TS, Finnerup NB. Allodynia and hyperalgesia in neuropathic pain: Clinical manifestations and mechanisms. Lancet Neurol. (2014) 13:924–35. doi: 10.1016/S1474-4422(14)70102-4
3. Burke D, Fullen BM, Stokes D, Lennon O. Neuropathic pain prevalence following spinal cord injury: a systematic review and meta-analysis. Eur J Pain. (2017) 21:29–44. doi: 10.1002/ejp.905
4. Ekkekakis P. The Measurement of Affect, Mood, and Emotion. A Guide for Health Behavioural Research. 1st ed. New York, NY: Cambridge University Press (2013). p. 38.
5. Rodrigues D, Tran Y, Wijesuriya N, Guest R, Middleton J, Craig A. Pain intensity and its association with negative mood states in patients with spinal cord injury. Pain Ther. (2012) 2:113–9. doi: 10.1007/s40122-013-0017-8
6. Finnerup NB, Johannesen IL, Sindrup SH, Bach FW, Jensen TS. Pain and dysesthesia in patients with spinal cord injury: a postal survey. Spinal Cord. (2001) 39:256–62. doi: 10.1038/sj.sc.3101161
7. Löfgren M, Norrbrink C. “But I know what works” - patients' experience of spinal cord injury neuropathic pain management. Disabil Rehabil. (2012) 34:2139–47. doi: 10.3109/09638288.2012.676146
8. Budh CN, Lundeberg T. Non-pharmacological pain-relieving therapies in individuals with spinal cord injury: a patient perspective. Comp Therap Med. (2004) 12:189–97. doi: 10.1016/j.ctim.2004.10.003
9. Todd KR, Martin Ginis KA. An examination of diurnal variations in neuropathic pain and affect, on exercise and non-exercise days, in adults with spinal cord injury. Spinal Cord Ser Cases. (2018) 4:1. doi: 10.1038/s41394-018-0130-3
10. Martin Ginis KA, Latimer AE. The effects of single bouts of body-weight supported treadmill training on the feeling states of people with spinal cord injury. Spinal Cord. (2007) 45:112–5. doi: 10.1038/sj.sc.3101911
11. Guy SD, Mehta S, Casalino A, Côté I, Kras-Dupuis A, Moulin DE, et al. The canpain SCI clinical practice guidelines for rehabilitation management of neuropathic pain after spinal cord: recommendations for treatment. Spinal Cord. (2016) 54:S14–23. doi: 10.1038/sc.2016.90
12. Norrbrink C, Lindberg T, Wahman K, Bjerkefors A. Effects of an exercise programme on musculoskeletal and neuropathic pain after spinal cord injury - results from a seated double-poling ergometer study. Spinal Cord. (2012) 50:457–61. doi: 10.1038/sc.2011.160
13. Woolf CJ, Decosterd I. Implications of recent advances in the understanding of pain pathophysiology for the assessment of pain in patients. Pain. (1999) 82(Suppl. 1):S141–7. doi: 10.1016/S0304-3959(99)00148-7
14. Rice D, Nijs J, Kosek E, Wideman T, Hasenbring MI, Koltyn K, et al. Exercise-induced hypoalgesia in pain-free and chronic pain populations: state of the art and future directions. J Pain. (2019) 20:1249–66. doi: 10.1016/j.jpain.2019.03.005
15. Lima LV, Abner TS, Sluka KA. Does exercise increase or decrease pain? Central mechanisms underlying these two phenomena. J Physiol. (2017) 595:4141–50. doi: 10.1113/JP273355
16. Wrigley PJ, Press SR, Gustin SM, Macefield VG, Gandevia SC, Cousins MJ, et al. Neuropathic pain and primary somatosensory cortex reorganization following spinal cord injury. PAIN. (2009) 141:52–9. doi: 10.1016/j.pain.2008.10.007
17. Sommer C, Leinders M, Üçeyler N. Inflammation in the pathophysiology of neuropathic pain. PAIN. (2018) 159:595–602. doi: 10.1097/j.pain.0000000000001122
18. Sommer C, Kress M. Recent findings on how proinflammatory cytokines cause pain: peripheral mechanisms in inflammatory and neuropathic hyperalgesia. Neurosci Lett. (2004) 361:184–7. doi: 10.1016/j.neulet.2003.12.007
19. Allison DJ, Thomas A, Beaudry K, Ditor DS. Targeting inflammation as a treatment modality for neuropathic pain in spinal cord injury: a randomized clinical trial. J Neuroinflammation. (2016) 13:1. doi: 10.1186/s12974-016-0625-4
20. Ostrowski K, Rohde T, Asp S, Schjerling P, Pedersen BK. Pro- and anti-inflammatory cytokine balance in strenuous exercise in humans. J Physiol. (1999) 515:287–91. doi: 10.1111/j.1469-7793.1999.287ad.x
21. Brown WMC, Davison GW, McClean CM, Murphy MH. A systematic review of the acute effects of exercise on immune and inflammatory indices in untrained adults. Br J Sports Med. (2001) 1:1–10. doi: 10.1186/s40798-015-0032-x
22. Hoffman MD, Shepanski MA, Ruble SB, Valic Z, Buckwalter JB, Clifford PS. Intensity and duration threshold for aerobic exercise-induced analgesia to pressure pain. Arch Phys Med Rehabil. (2004) 85:1183–7. doi: 10.1016/j.apmr.2003.09.010
23. Ekkekakis P, Parfitt G, Petruzzello SJ. The pleasure and displeasure people feel when they exercise at different intensities. Sports Med. (2011) 41:641–71.
24. Teasell RW, Arnold JM, Krassioukov A, Delaney GA. Cardiovascular consequences of loss of supraspinal control of the sympathetic nervous system after spinal cord injury. Arch Phys Med Rehab. (2000) 81:506–16. doi: 10.1053/mr.2000.3848
25. Kouda K, Furusawa K, Sugiyama H, Sumiya T, Ito T, Tajima F, et al. Does 20-min arm crank ergometer exercise increase plasma interleukin-6 in individuals with cervical spinal cord injury? Eur J Appl Physiol. (2012) 112:597–604. doi: 10.1007/s00421-011-2004-2
26. Paulson TAW, Goosey-Tolfrey VL, Lenton JP, Leicht CA, Bishop NC. Spinal cord injury level and the circulating cytokine response to strenuous exercise. Med Sci Sports Exerc. (2013) 45:1649–55. doi: 10.1249/MSS.0b013e31828f9bbb
27. Mee-Inta O, Zhao ZW, Kuo YM. Physical exercise inhibits inflammation and microglial activation. Cells. (2019) 8:691. doi: 10.3390/cells8070691
28. Martin Ginis KA, Van Der Scheer JW, Latimer-Cheung AE, Barrow A, Bourne C, Carruthers P, et al. Evidence-based scientific exercise guidelines for adults with spinal cord injury: an update and a new guideline. Spinal Cord. (2018) 56:308–21. doi: 10.1038/s41393-017-0017-3
29. Thompson PD, Franklin BA, Balady GJ, Blair SN, Corrado D, Estes NAM, et al. Exercise and acute cardiovascular events: placing the risks into perspective a scientific statement from the American Heart Association Council on Nutrition, Physical Activity, and Metabolism and the Council on Clinical Cardiology. Circulation. (2007) 115:2358–68. doi: 10.1161/CIRCULATIONAHA.107.181485
30. Galer BS, Jensen MP. Development and preliminary validation of a pain measure specific to neuropathic pain: the neuropathic pain scale. Neurology. (1997) 48:332–8. doi: 10.1212/WNL.48.2.332
31. Hardy CJ, Rejeski WJ. Not what, but how one feels: the measurement of affect during exercise. J Sport Exerc Psychol. (1989) 11:304–17. doi: 10.1123/jsep.11.3.304
32. Svebak S, Murgatroyd S. Metamotivational dominance. A multimethod validation of reversal theory constructs. J Pers Soc Psychol. (1985) 48:107–16. doi: 10.1037/0022-3514.48.1.107
33. Watson D, Clark LA. Measurement and mismeasurement of mood: recurrent and emergent issues. J Pers Assess. (1997) 68:267–96. doi: 10.1207/s15327752jpa6802_4
34. Borg GAV. Psychophysical bases of perceived exertion. Med Sci Sport Exerc. (1982) 14:377. doi: 10.1249/00005768-198205000-00012
35. Hutchinson MJ, Valentino SE, Totosy de Zepetnek J, MacDonald MJ, Goosey-Tolfrey VL. Perceptually regulated training does not influence the differentiated RPE response following 16-weeks of aerobic exercise in adults with spinal cord injury. Appl Physiol Nutr Metab. (2020) 45:129–34. doi: 10.1139/apnm-2019-0062
36. Claydon VE, Hol AT, Eng JJ, Krassioukov AV. Cardiovascular responses and postexercise hypotension after arm cycling exercise in subjects with spinal cord injury. Arch Phys Med Rehabil. (2006) 87:1106–14. doi: 10.1016/j.apmr.2006.05.011
37. Lasko-McCarthey P, Davis JA. Protocol dependency of VO2max during arm cycle ergometry in males with quadriplegia. Med Sci Sports Exerc. (1991) 23:1097–101. doi: 10.1249/00005768-199109000-00016
38. Martel G, Noreau L, Jobin J. Physiological responses to maximal exercise on arm cranking and wheelchair ergometer with paraplegics. Paraplegia. (1991) 29:447–56. doi: 10.1038/sc.1991.61
39. Lakens D. Calculating and reporting effect sizes to facilitate cumulative science: a practical primer for t-tests and ANOVAs. Front Psychol. (2013) 4:863. doi: 10.3389/fpsyg.2013.00863
40. Cohen J. Statistical Power Analysis for the Behavioural Sciences. 2nd ed. New York, NY: Academic Press (1977). p. 8.
41. Widerström-Noga E. Neuropathic pain and spinal cord injury: phenotypes and pharmacological management. Drugs. (2017) 77:967–84. doi: 10.1007/s40265-017-0747-8
42. Celik EC, Erhan B, Lakse E. The clinical characteristics of neuropathic pain in patients with spinal cord injury. Spinal Cord. (2012) 50:585–9. doi: 10.1038/sc.2012.26
43. Lind E, Ekkekakis P, Vazou S. The affective impact of exercise intensity that slightly exceeds the preferred level. J Health Psychol. (2008) 13:464–8. doi: 10.1177/1359105308088517
44. Ekkekakis P, Zenko Z, Ladwig M, Hartman M. Affective Determinants of Health Behavior. New York, NY: Oxford University Press (2018). p. 237.
45. Whiteside A, Hansen S, Chaudhuri A. Exercise lowers pain threshold in chronic fatigue syndrome. Pain. (2004) 109:497–9. doi: 10.1016/j.pain.2004.02.029
46. Pedersen BK, Febbraio MA. Muscle as an endocrine organ: focus on muscle-derived interleukin-6. Physiol Rev. (2008) 88:1379–406. doi: 10.1152/physrev.90100.2007
47. Fischer CP. Interleukin-6 in acute exercise and training: what is the biological relevance? Exerc Immunol Rev. (2006) 41:6–33.
48. Mathur N, Pedersen BK. Exercise as a mean to control low-grade systemic inflammation. Mediators Inflamm. (2008) 2008:109502. doi: 10.1155/2008/109502
49. Bixby WR, Spalding TW, Hatfield BD. Temporal dynamics and dimensional specificity of the affective response to exercise of varying intensity: differing pathways to a common outcome. J Sport Exerc Psychol. (2001) 23:171–90. doi: 10.1123/jsep.23.3.171
50. Ma CA, Rajandran SN, Liu J, Wong SB, Leung YY. The association of plasma IL-1Ra and related cytokines with radiographic severity of early knee osteoarthritis. Osteoarthr Cartil. (2020) 2:100046. doi: 10.1016/j.ocarto.2020.100046
51. Martin Ginis KA, van der Scheer JW, Todd KR, Davis JC, Gaudet S, Hoekstra F, et al. A pragmatic randomized controlled trial testing the effects of the international scientific SCI exercise guidelines on SCI chronic pain: protocol for the EPIC-SCI trial. Spinal Cord. (2020) 58:746–54. doi: 10.1038/s41393-020-0519-2
52. Amur S, LaVange L, Zineh I, Buckman-Garner S, Woodcock J. Biomarker qualification: toward a multiple stakeholder framework for biomarker development, regulatory acceptance, and utilization. Curr Clin Pharmacol. (2015) 98:34–46. doi: 10.1002/cpt.136
53. Kramer JL, Minhas NK, Jutzeler CR, Erskine EL, Liu LJ, Ramer MS. Neuropathic pain following traumatic spinal cord injury: models, measurement, and mechanisms. J Neurosci Res. (2017) 95:1295–306. doi: 10.1002/jnr.23881
54. Widerström-Noga E, Biering-Sørensen F, Bryce TN, Cardenas DD, Finnerup NB, Jensen MP, et al. The international spinal cord injury pain basic data set (version 2.0). Spinal Cord. (2014) 52:282–6. doi: 10.1038/sc.2014.4
55. Gillum TL, Kuennen MR, Schneider S, Moseley P. A review of sex differences in immune function after aerobic exercise. Exerc Immunol Rev. (2011) 17:104–21.
56. Wiesenfeld-Hallin Z. Sex differences in pain perception. Gend Med. (2005) 2:137–45. doi: 10.1016/S1550-8579(05)80042-7
Keywords: neuropathic pain, exercise, disability, affect, inflammation
Citation: Todd KR, Van Der Scheer JW, Walsh JJ, Jackson GS, Dix GU, Little JP, Kramer JLK and Martin Ginis KA (2021) The Impact of Sub-maximal Exercise on Neuropathic Pain, Inflammation, and Affect Among Adults With Spinal Cord Injury: A Pilot Study. Front. Rehabilit. Sci. 2:700780. doi: 10.3389/fresc.2021.700780
Received: 26 April 2021; Accepted: 29 September 2021;
Published: 26 October 2021.
Edited by:
Mats Granlund, Jönköping University, SwedenReviewed by:
Zhuoying Qiu, China Rehabilitation Research Center/WHO Collaborating Center for Family International Classifications, ChinaVerna Stavric, Auckland University of Technology, New Zealand
Copyright © 2021 Todd, Van Der Scheer, Walsh, Jackson, Dix, Little, Kramer and Martin Ginis. This is an open-access article distributed under the terms of the Creative Commons Attribution License (CC BY). The use, distribution or reproduction in other forums is permitted, provided the original author(s) and the copyright owner(s) are credited and that the original publication in this journal is cited, in accordance with accepted academic practice. No use, distribution or reproduction is permitted which does not comply with these terms.
*Correspondence: Kendra R. Todd, a3RvZGQwM0BtYWlsLnViYy5jYQ==