- 1School of Chemical and Biomedical Engineering, Nanyang Technological University, Singapore, Singapore
- 2School of Engineering and Science, Tecnológico de Monterrey, Monterrey, Mexico
The search for natural and sustainable biopolymers is increasing. Biopolymers are used in applications, such as to encapsulate and stabilize probiotic bacteria. Currently, this process requires many steps, including the separation of bacteria from the culture media after fermentation, which is energy intensive. In this study, we developed a strategy to use a natural biopolymer from durian seed, durian seed gum (DSG), to address these issues. DSG was used to serve dual roles, firstly as a nutrient source, and secondly as an encapsulating agent. DSG was used in synergy with reconstituted skim milk (RSM), to be a complete nutrient source, for probiotic bacteria Lactobacillus plantarum (L. plantarum). Usually RSM requires supplementation, with costly yeast extract. DSG was characterized to be a suitable nutrient source as it contains polysaccharides, organic acids, amino acids and fatty acids. In the presence of DSG and RSM, the growth of L. plantarum increased from 8.73 log CFU/mL to 13.86 log CFU/mL, at the end of 72 h. In comparison, when grown with commercial gum arabic (GA) and RSM, growth of L. plantarum reached a lower 11.49 log CFU/mL at 72 h. Metabolomics revealed that several metabolites, including lactose, depleted after fermentation, in the DSG and RSM treatment group, as compared to GA and RSM treatment group. This suggested that glycolysis were up regulated. This correlated with the increased growth, lactic acid, malic acid and fatty acids production by L. plantarum when it was grown on DSG and RSM. Next, the entire culture of L. plantarum with DSG and RSM was taken for spray drying, without the need to separate the bacteria from the culture media. DSG and RSM was able to stabilize L. plantarum, to remain viable at 10 log CFU/g after storage for 10 weeks. On the other hand, the viability was 8.8 log CFU/g when L. plantarum was spray dried with GA and RSM. This study demonstrated an innovative process and low-cost strategy to produce spray dried probiotic powder. DSG could be a potential low cost and sustainable replacement for GA, to develop other functional foods.
Introduction
Biopolymers such as gums, have many versatile applications in the food industry. It can serve as a stabilizer, emulsifier, and as an encapsulating agent during spray drying (Desmond et al., 2002; Cornelia et al., 2015; Herlina et al., 2016). Gums are frequently used to encapsulate sensitive compounds, including probiotic bacteria. Lactobacillus plantarum (L. plantarum) is a well-known probiotic bacteria. Its ability to produce lactic acid can suppress the growth of pathogenic bacteria in the gut, such as Escherichia coli (Herías et al., 1999). Hence, probiotic bacteria are incorporated into food products, such as cereals and fruit juices (Sheehan et al., 2007; Ewe et al., 2010).
Spray drying probiotic bacteria is a widely used method to maintain its viability (Huang et al., 2017). The traditional process to produce spray dried probiotic powders, requires many steps. In the first step, fermentation of the probiotic bacteria is carried out. The most common nutrient media for probiotic Lactobacillus bacteria, is De Man Rogosa and Sharpe (MRS) media. The disadvantage of using MRS media however, is that it is expensive and non-food grade (Huang et al., 2017). Due to the non-food grade property of MRS media, a second step is needed to completely remove the entire MRS media, from the probiotic bacteria. Next, the probiotic bacteria are re-suspended in the encapsulating agent, such as gum biopolymers. Lastly, the bacteria are spray dried into powder form. The overall process is time consuming and energy intensive. It would be ideal to develop a strategy, whereby the nutrient medium used to support the growth of the probiotic bacteria is food grade and can also act as an encapsulating agent. This would remove the need to separate the probiotic bacteria from the nutrient medium after fermentation, hence saving energy and cost.
During the spray drying process, an encapsulating agent such as gums is required, to protect the probiotic bacteria (Shori, 2017). The gum entraps the bacteria in an external coating, protecting the bacteria from the heat generated during the spray drying process. This prolongs the viability of the bacteria, during the adverse storage conditions (Iravani et al., 2015; Shori, 2017). The most widely used and commercial gum, is gum arabic (GA). It is obtained from the acacia tree. The use of GA has its disadvantages due to its high cost, and fluctuations in supply and availability. Due to this, alternate natural biopolymers from other sources, such as biomass from agro resources, are being investigated (Valdés et al., 2014; Patel and Goyal, 2015). Furthermore, there is increasing demand for natural gum biopolymers, as they are considered more safe for human consumption as compared to synthetic gum biopolymers (Rana et al., 2011).
Recently, a polysaccharide-protein biopolymer has been extracted from the seasonal fruit, durian (Durio zibethinus), known as durian seed gum (DSG). Durian is a popular fruit consumed in South East Asia (Cheok et al., 2018). After consumption of the fruit, the durian seeds which makes up 20–25% of the entire fruit, is thrown away (Amid et al., 2012). Durian seed contains polysaccharides, fatty acids, proteins, amino acids and minerals (Amin et al., 2007). The biopolymer extracted from durian seed, DSG, contains glucose, galactose, rhamnose, in the ratio 9:1:3. Therefore it not a galactomannan. It has a molecular weight which can range from 1.08 to 1.44 × 105 (g/mol) (Amid et al., 2012; Mirhosseini and Tabatabaee Amid, 2012). Additionally, it has a 10% starch content, in dry weight (Taewee, 2008). Currently, few studies exist on the applications for DSG. It would be desirable to develop more applications, for this natural biopolymer, which is nutrient rich.
A growing number of studies have shown that reconstituted skim milk (RSM) can be used as a food grade culture medium, and also as an encapsulating agent for Lactobacillus. As a culture medium, RSM needs to be supplemented with a nitrogen source which is yeast extract (Gardiner et al., 2000; Akabanda et al., 2014). Yeast extract is costly (Zarei et al., 2016). Interestingly, a recent study showed that the use of gums, such as xanthan gum, worked in synergy with RSM, to enhance the growth of Lactobacillus, as compared to RSM alone (Karlton-Senaye and Ibrahim, 2013; Karlton-Senaye, 2015).
Hence, we hypothesized that a potential use of DSG, could be to replace yeast extract. As DSG is nutritionally rich, we expect that DSG as an added nutrient source to RSM, can form a complete nutrient medium for probiotic bacteria such as L. plantarum. In addition, as DSG and RSM are both food grade, the entire culture medium can be taken directly for spray drying. This removes the need to separate, and harvest the bacteria from culture medium, unlike with the use of non-food grade MRS medium. It is known that the nutrient source can affect the metabolic activity of the cell. Hence in this study, metabolomics will be used as a tool to investigate the metabolic activities of L. plantarum when grown on DSG with RSM, as a nutrient source. The stability of spray dried L. plantarum with DSG and RSM, after 10 weeks of refrigerated storage, will also be determined. In this study, commercial GA will be included as a comparison to DSG.
Materials and Methods
Durian Seed
Durian seeds were supplied by Four Seasons Durian, Singapore. The seeds were thoroughly washed. Then it were sliced and freeze dried using a LabConco freeze dryer. The freeze-dried seeds were then milled into powder, and stored in the dark at 4°C.
Preparation of Durian Seed Gum
DSG was extracted accordingly (Amid and Mirhosseini, 2012b). The gum was extracted using pure distilled water from milled durian seed powder. Next, this was centrifuged at 1,200 rpm, for 10 min. The supernatant containing the gum, was collected. Then, three volumes of 95% ethanol were added to precipitate the gum. The precipitated gum was washed three times with distilled water, and dried overnight at 40°C, using a vacuum oven.
Bacteria Strain and Culture Conditions
L. plantarum (DSM 12028) was maintained on MRS agar. A colony of L. plantarum was taken and grown overnight in MRS media. This was used as the inoculum for fermentation. The initial concentration used for fermentation was 8.73 log CFU/mL. The culture media contained 10% (w/v) RSM (from VWR 84615.0500). RSM contained ≤1% lipid, ≤4% moisture, and ≥34% protein. It was pasteurized at 90°C for 30 min. To that, an added nutrient source of 10% (w/v) of DSG, or commercial gum Arabic (GA) from acacia tree (Sigma G9752), was added. GA is a branched polymer of galactose, rhamnose, arabinose, and glucuronic acid. Fermentation was carried out in a total culture volume of 50 mL, using 250 ml Erlenmeyer flasks, for 72 h, at 30°C, shaking at 200 rpm.
Spray Drying of L. plantarum
After fermentation, the entire culture medium were taken for spray drying using a mini spray dryer (Buchi B-290, Switzerland) according to Desmond et al. (2002) The powders were collected, sealed in a polythene bag and stored at 4°C, for further analysis.
Evaluation of Microbial Viability
To determine the viability of L. plantarum cells spray dried with DSG and RSM or GA and RSM, 10 mg of spray dried powder were reconstituted in 1 ml of 0.5% sterile saline water. The appropriate serial dilutions were made, and colonies were enumerated using MRS agar. The plates were incubated at 30°C, overnight.
Scanning Electron Microscopy (SEM)
L. plantarum spray dried with either DSG or GA, was examined using SEM (JSM-6701F, JEOL Asia). The powders were spread thinly on a carbon tape and coated with gold. Images were taken at a voltage of 5 kV.
Characterization of DSG and the Effect of DSG as an Added Nutrient Source on the Metabolic Activity of L. plantarum
The metabolites present in DSG were characterized by gas chromatography-mass spectrophotometry (GC-MS). Also, metabolites after L. plantarum was grown on DSG and RSM, and GA with RSM, as nutrient source after fermentation, were analyzed. To do this, 800 μl of cold methanol was first added to extract the metabolites. 10 μl of 2 mg/mL ribitol was also added as an internal standard to correct for differences in extraction efficiency and sample loss during preparation. This solution was then left to dry completely on a heat block, set at 30°C, overnight. The next day, 50 μl of 20 mg/mL methoxyamine hydrochloride dissolved in pyridine was added, and incubated for 1 h at 37°C. Derivitization were carried out by adding 1 μl trimethylchlorosilane (TMCS) and 99 μl of N-methyl-N-(trimethylsilyl)-trifluoroacetamide (MSTFA), incubated for 30 min at 70°C. This was centrifuged at 10,000 rpm for 10 min at 4°C, and transferred to sample vials. Samples were run immediately using Shimadzu QP 2010 Plus GC-MS system according to (Lee et al., 2014). The Chemstation Data Analysis software was used together with the National Institute of Standards and Technology (NIST) '08 mass spectral library, for chromatogram acquisition and mass spectra identification. The quality of the compound had to be at least 90%, for its identity to be considered true.
Statistical Analysis
All the values are presented as mean, with error bars indicating the standard error (SD). Graph Pad Prism (GraphPad Software) was used for t-test. Values were considered significant if P < 0.05, and indicated as *.
Results
Characterization of DSG
This is a first study to use GC-MS to characterize the metabolites in DSG, to the best of our knowledge. In total, 14 metabolites were discovered (Table 1). The metabolites could be categorized into carbohydrates, amino acids, organic acids and fatty acids. The retention time and relative abundance of each compound are shown in Table 1. The presence of these compounds indicated that DSG is a potential nutrient source for L. plantarum.
Effect of DSG and GA as an Added Nutrient Source on the Growth of L. plantarum
As can be seen in Figure 1A, when DSG and RSM were used as the added nutrient source, growth of L. plantarum increased exponentially from the initial concentration of 8.73 log CFU/mL, to reach 13.86 log CFU/mL after 72 h. In comparison, when GA and RSM were used as the nutrient source, the growth of L. plantarum increased from 8.73 log CFU/mL, to reach only 11.49 log CFU/mL after 72 h (Figure 1A). This showed that DSG was a better added nutrient source for L. plantarum, as compared to GA.
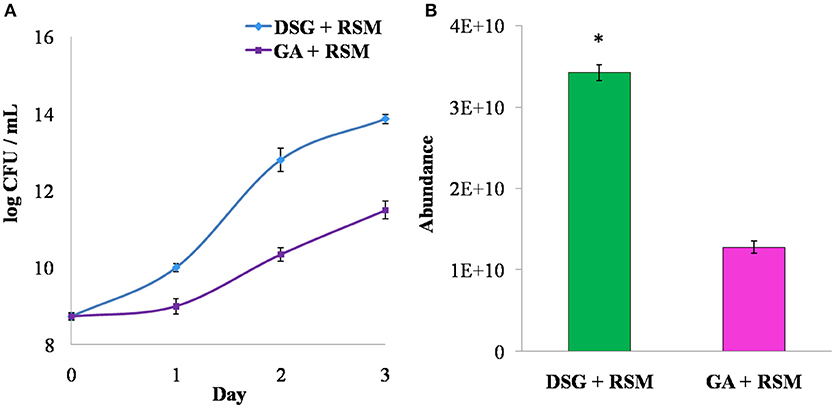
Figure 1. The effect of DSG and GA as an added nutrient source on the microbial count for L. plantarum after fermentation for 72 h (A). The level of lactic acid produced by L. plantarum after fermentation for 72 h (B). All values are expressed as mean ± SD (n = 3). *Denotes that the value is statistically significantly higher.
After fermentation, the amount of intracellular lactic acid produced by L. plantarum were analyzed by GC-MS. The abundance of intracellular lactic acid produced when L. plantarum was grown with DSG and RSM was 3.4 × 1010, after 72 h (Figure 1B). This was 2.6 times higher as compared to the lactic acid abundance of 1.3 × 1010 produced by L. plantarum when GA and RSM was used as the nutrient source, after 72 h.
Cost Analysis of Using DSG as an Added Nutrient Source and Encapsulating Agent for L. plantarum
Table 2 details the cost of using 20% RSM supplemented with 0.5% yeast extract, as the food grade culture medium, for Lactobacillus fermentation (Gardiner et al., 2000). In this typical process, after the growth of the bacteria, GA is the encapsulating agent used for spray drying of probiotic bacteria (Desmond et al., 2002). The total cost for this entire process to produce spray dried probiotic powder, is estimated at $59.93/L. In comparison, the strategy developed in this study which used 10% RSM supplemented with 10% DSG, as both nutrient source and encapsulating agent for L. plantarum, resulted in an overall cost at $13.30/L. This is approximately 4.5 times cheaper, as compared to the typical process.

Table 2. Cost of using DSG as added nutrient source and encapsulating agent for spray dried L. plantarum, as compared to the typical process.
Effect of DSG and GA on the Stability of L. plantarum
Encapsulating agents provide a wall material for the probiotic bacteria during spray drying, and serve to enhance the storage stability (Paim et al., 2016). After fermentation, the entire culture medium of L. plantarum grown either on DSG with RSM, or GA with RSM, was taken for spray drying.
L. plantarum spray dried with GA and RSM showed a cell viability of 9.5 log CFU/g, at 4 weeks after refrigerated storage (Figure 2). The viable count decreased to 9.3 log CFU/g at 8 weeks, followed by 8.8 log CFU/g after 10 weeks. In comparison, L. plantarum spray dried with DSG and RSM, had a higher viable count over time. The viability was 10.3 log CFU/g at 4 weeks, and were maintained at 10.0 log CFU/g at 8 weeks and 10 weeks. Hence, DSG with RSM preserved the storage viability of L. plantarum better than GA with RSM.
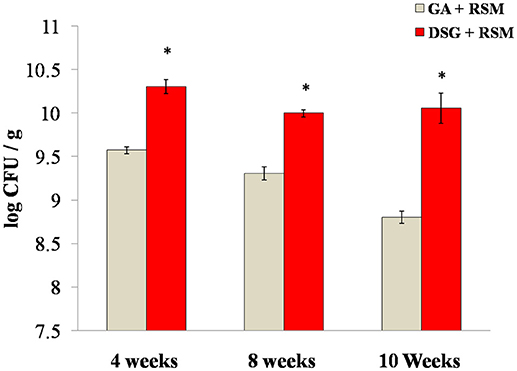
Figure 2. The viable counts of L. plantarum spray dried with either DSG with RSM (DSG), or GA with RSM (GA). All values are expressed as mean ± SD (n = 3). *Denotes that the value is statistically significantly higher.
SEM Images of L. plantarum Spray Dried With DSG and GA
SEM images of spray dried L. plantarum were taken. Figure 3 showed the SEM micrographs of L. plantarum spray dried with either DSG and RSM (Figure 3A), or GA and RSM (Figure 3B). In general, no cracks or fissures were observed on the surfaces of L. plantarum in Figures 3A,B. This is ideal, as it reduces the air permeability, reducing oxidation, and allowed a higher protection for L. plantarum cells (Jafari et al., 2008). In Figure 3A, the surface of L. plantarum spray dried with DSG and RSM were smoother, and more spherical. On the other hand, L. plantarum spray dried with GA and RSM had many wrinkles, dents and concavities on the surface (Figure 3B).
Utilization and Metabolism of L. plantarum Using DSG and GA as Added Nutrient Source
The potential use of DSG and GA as an added nutrient source with RSM, for the growth of L. plantarum was investigated. To shed light behind the increased growth of L. plantarum when grown on DSG and RSM, as compared to GA and RSM, metabolomics was carried out using GC-MS (Park et al., 2015). Metabolites in DSG before fermentation were also analyzed.
In total, 6 important metabolites were identified. Their retention times and abundance are shown in Table 3. The metabolites could be classified into carbohydrates, organic acids and fatty acids. Overall, there were a decrease in metabolites found in the DSG and RSM treatment group after fermentation, as compared to original DSG before fermentation. The carbohydrate metabolites included glycerol, glucose, galactose, glucitol, xylose. The organic acids metabolites included L-threonic acid, tartaric acid, and gluconic acid. The levels of these metabolites which were present in DSG, were found to be depleted after fermentation in the treatment group DSG and RSM (Table 1). In addition, the amino acids including L-proline and glycine, and the fatty acid oleic acid, were also not detected after fermentation, in the DSG and RSM treatment group. Interestingly, the amount of lactose, were also depleted after L. plantarum were fermented using DSG and RSM as the nutrient source. This suggested that L. plantarum had utilized and consumed these metabolites for growth, which might account for its high growth seen in Figure 1A. On the other hand, the metabolites which increased were malic acid, palmitic acid and stearic acid, in the DSG and RSM treatment group after L. plantarum fermentation, as compared to DSG (Table 1).
To better understand the changes in levels of each metabolite, we mapped the changes in relation to biochemical pathways, as shown in Figure 4. This gives an overall view of the metabolic changes happening during fermentation of L. plantarum grown with DSG and RSM, as compared to GA and RSM. This could help us understand the reason for higher growth of L. plantarum when DSG and RSM were the nutrient source.
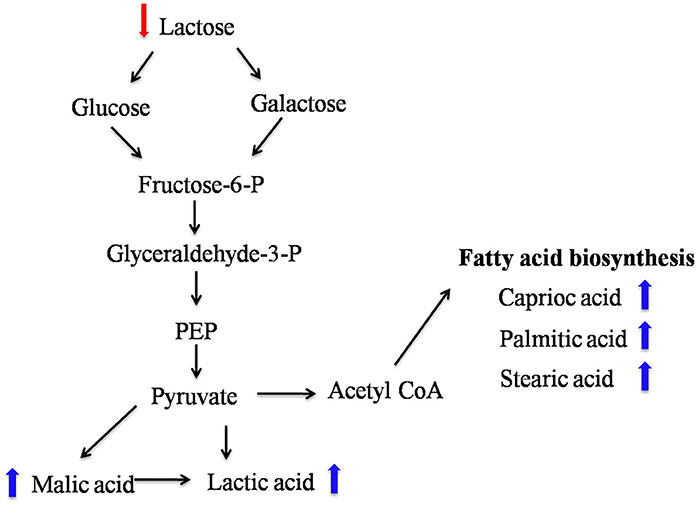
Figure 4. Metabolic changes in L. plantarum grown with DSG and RSM as compared to GA with RSM as the nutrient source, mapped onto metabolic pathways. Colored arrows represent an increase or decrease in the level of the metabolite.
Discussion
In this study, we evaluated the use of DSG and GA, as an added nutrient source with RSM, to serve as a food grade culture medium for L. plantarum. RSM mainly contains lactose and proteins. L. plantarum is well-known to be able to utilize lactose in RSM for growth. However, only some strains of L. plantarum are known to secrete proteases to utilize RSM (Akabanda et al., 2014). The L. plantarum strain used in our study was determined to be able to grow on RSM alone, without DSG (data not shown), which suggested a proteolytic ability for this strain. However, L. plantarum growth was higher, when DSG was used as an added nutrient source with RSM. It has been reported that a positive synergistic effect exists when gums were used in combination with RSM. This synergistic relationship resulted in an enhanced growth of the probiotic bacteria Lactobacillus rhamnosus GG, although the mechanism is not known (Karlton-Senaye and Ibrahim, 2013; Karlton-Senaye, 2015).
As can be seen in Figure 1A, DSG performed better as an added nutrient source for L. plantarum, as compared to GA. The reason for higher growth of DSG and RSM might be because durian seed contains fibers and minerals such as zinc, manganese, natrium, and calcium. (Amin et al., 2007; Amid and Mirhosseini, 2012a). These compounds could also be present in DSG, to act as a solid support for L. plantarum during fermentation, which enhanced its growth (Charalampopoulos et al., 2003). Fiber and minerals in fruit wastes, have been shown to be able to immobilize L. acidophilus cells during fermentation, which resulted in increased growth (Teh et al., 2009). In addition, many durian types are known to exist, which can be classified based on morphologic characteristics such as seed size and shape (Siew et al., 2018). We therefore extracted DSG from four durian types, with different seed shapes. When L. plantarum were grown on the four types of DSG and RSM as the nutrient source, growth was higher as compared to when grown on GA and RSM. Hence the variations in seed shape, did not appear to affect the use of DSG as a nutrient source for L. plantarum (Supplementary Materials).
Interestingly, the levels of lactic acid produced were approximately 2.6 times higher, when L. plantarum was grown with DSG and RSM, as compared to GA and RSM (Figure 1B). Lactic acid is an end product of Lactobacillus fermentation (Fu and Mathews, 1999; Bernardo et al., 2016). This correlated with the increased growth of L. plantarum seen when DSG with RSM were used as the nutrient source, as compared to GA and RSM. The increased lactic acid production by L. plantarum grown on DSG and RSM, is useful for human health. Lactic acid has been shown to inhibit the pathogen Helicobacter pylori, in the human gastrointestinal tract (Chen et al., 2012). Lactic acid also helps to lower the pH in the gut, inhibiting pathogenic bacteria growth (Chaikham et al., 2017). Hence this suggested that L. plantarum fermented with DSG and RSM, could be beneficial, as a functional food for human consumption.
As shown in Figure 2, the stability of L. plantarum was maintained at an average of 10.0 log CFU/g after 10 weeks. The DSG used in our study contained fatty acids (Table 1). Lipids have been shown to help to stabilize the cell wall of L. plantarum, maintaining its viability during storage (Bustos and Bórquez, 2013). This might be one of the reasons why DSG performed better than GA as an encapsulating material. Spray drying of L. plantarum with cheese whey, also helped to maintain a viable count of 9.98 log CFU/g, after 10 weeks (Bustos and Bórquez, 2013). In addition, the viable counts of L. plantarum spray dried with DSG with RSM after 10 weeks, far exceeded the minimum viable count required in a probiotic product, which is 7 log CFU/g according to the International Dairy Federation (Chávez and Ledeboer, 2007).
As shown in Figure 3A, spray dried L. plantarum with DSG and RSM had a smooth surface. The smoother, spherical micro particles of L. plantarum spray dried with DSG and RSM, might also have been due to the lipids in DSG (Table 1). Lipids can reduce moisture loss and shrinkage during the spray drying process (Eratte et al., 2015). Previously when black carrot anthocyanin pigment was spray dried with maltodextrin of different dextrose equivalents, a smooth surface was also observed (Ersus and Yurdagel, 2007). In contrast, when L. plantarum was spray dried with GA and RSM, a “flat ball” effect was observed (Figure 3B). This was due to the dehydration and shrinkage of the cell during spray drying (Lian et al., 2002).
Lactose is the main disaccharide found in RSM. Cells can uptake sugars such as lactose, through translocators (Barrangou et al., 2006). Lactose is then broken down into glucose and galactose, where it could enter the glycolysis pathway (Figure 4). During glycolysis, glucose, and galactose is converted into glyceraldehyde-3-phosphate, transformed into phosphoenolpyruvate (PEP), and converted into the important energy molecule, pyruvate (Figure 4; Plumed-Ferrer et al., 2008). This energy obtained from glycolysis could be used by cells for growth. No lactose was detected at the end of fermentation when L. plantarum was grown on DSG and RSM as the nutrient source. This suggested that L. plantarum cells had consumed lactose rapidly, and hydrolyzed it into glucose and galactose. These compounds could enter glycolysis. One of the product of the glycolysis pathway, is the important energy molecule, pyruvate, which is used to provide energy for cellular processes such as growth. This could explain the exponential increase in L. plantarum growth seen in Figure 1, when DSG was used as the added nutrient source, as compared to GA. On the other hand, lactose was still detected after fermentation, when L. plantarum was grown on GA and RSM (Table 3). This suggested that lactose was not completely utilized by L. plantarum cells. This suggested that glycolysis had taken place at a decreased rate, which resulted in less available energy for the cell. This could explain the lower growth shown in Figure 1. The reason for this is unclear, and requires further studies.
Pyruvate can also be transformed into malic acid, lactic acid, or acetyl CoA (Figure 4) (Plumed-Ferrer et al., 2008; Drissi et al., 2014). Malic acid was detected after fermentation, when L. plantarum was grown on DSG and RSM, but not when grown on GA and RSM. This might be due to the upregulation of glycolysis, resulting in an increased amount of available pyruvate, to be converted into malic acid. Malic acid can also be transformed into lactic acid, through the malolactic enzyme (Figure 4; Lonvaud-Funel, 1999). The decrease in lactose and increase in malic acid and lactic acid strongly supports the suggestion that glycolysis were upregulated.
As seen in Figure 4, pyruvate can also be transformed into the molecule acetyl CoA, which is the precursor for the fatty acid biosynthesis pathway. There were higher production of the fatty acids, caprioc acid, palmitic acid, and stearic acid (Table 3) when L. plantarum was grown on DSG and RSM (Veerkamp, 1971; Botta et al., 2017). Increased glycolysis, could result in higher amounts of the precursor acetyl-CoA which could enter the fatty acid biosynthesis pathway. Taken together, these results suggested that when L. plantarum was grown on DSG and RSM, it was able to rapidly consume lactose, leading to an up regulation of glycolysis. This provided increased amounts of the precursor acetyl-CoA and pyruvate, leading to increased production of malic acid, lactic acid and increased fatty acids production.
Conclusion
The biopolymer DSG was characterized to contain carbohydrates, organic acids, amino acids and fatty acids. The use of DSG as an added nutrient source with RSM were found to increase the growth of L. plantarum from 8.73 log CFU/mL to 13.86 log CFU/mL at the end of 72 h fermentation. This was much higher as compared to 11.49 log CFU/mL reached when L. plantarum was grown on GA with RSM. Further, L. plantarum spray dried with DSG and RSM was able to maintain stable storage viability at 10 log CFU/g, for 10 weeks, which was higher than GA with RSM. The presence of lipids in DSG, might have played in a beneficial role in enhancing the stability of L. plantarum. Metabolomics was a useful tool to shed light on the increased growth of L. plantarum on DSG with RSM. Many metabolites including lactose were depleted, with increased malic acid, lactic acid and fatty acids production. This suggested that glycolysis were upregulated, and could account for the increased growth seen when L. plantarum was grown on DSG and RSM. Our results showed that DSG can serve dual roles as an added nutrient source and encapsulating agent for spray dried L. plantarum. The strategy used in this study, is also more cost efficient than the typical process (Table 2).
Author Contributions
JL, RP-S, and W-NC designed the study and wrote the manuscript. JL, GZ, CC-Z, JK, and MR-A carried out the experimental procedures and analysis. All the authors reviewed and approved the final manuscript.
Funding
The study was conducted with funding from Nanyang Technological University (iFood Research grant).
Conflict of Interest Statement
The authors declare that the research was conducted in the absence of any commercial or financial relationships that could be construed as a potential conflict of interest.
Supplementary Material
The Supplementary Material for this article can be found online at: https://www.frontiersin.org/articles/10.3389/fsufs.2018.00053/full#supplementary-material
References
Akabanda, F., Owusu-Kwarteng, J., Tano-Debrah, K., Parkouda, C., and Jespersen, L. (2014). The use of lactic acid bacteria starter culture in the production of Nunu, a spontaneously fermented milk product in Ghana. Int. J. Food Sci. 2014:721067. doi: 10.1155/2014/721067
Amid, B. T., and Mirhosseini, H. (2012a). Effect of different purification techniques on the characteristics of heteropolysaccharide-protein biopolymer from durian (Durio zibethinus) seed. Molecules 17, 10875–10892. doi: 10.3390/molecules170910875
Amid, B. T., and Mirhosseini, H. (2012b). Optimisation of aqueous extraction of gum from durian (Durio zibethinus) seed: a potential, low cost source of hydrocolloid. Food Chem. 132, 1258–1268. doi: 10.1016/j.foodchem.2011.11.099
Amid, B. T., Mirhosseini, H., and Kostadinovic, S. (2012). Chemical composition and molecular structure of polysaccharide-protein biopolymer from Durio zibethinus seed: extraction and purification process. Chem. Cent. J. 6, 117. doi: 10.1186/1752-153x-6-117
Amin, A. M., Ahmad, A. S., Yap, Y. Y., Yahya, N., and Ibrahim, N. (2007). Extraction, purification and characterization of durian (Durio zibethinus) seed gum. Food Hydrocol. 21, 273–279. doi: 10.1016/j.foodhyd.2006.04.004
Barrangou, R., Azcarate-Peril, M. A., Duong, T., Conners, S. B., Kelly, R. M., and Klaenhammer, T. R. (2006). Global analysis of carbohydrate utilization by Lactobacillus acidophilus using cDNA microarrays. Proc. Natl. Acad. Sci. U S A. 103:3816–3821. doi: 10.1073/pnas.0511287103
Bernardo, M. P., Coelho, L. F., Sass, D. C., and Contiero, J. (2016). l-(+)-Lactic acid production by Lactobacillus rhamnosus B103 from dairy industry waste. Braz. J. Microbiol. 47, 640–646. doi: 10.1016/j.bjm.2015.12.001
Botta, C., Acquadro, A., Greppi, A., Barchi, L., Bertolino, M., Cocolin, L., et al. (2017). Genomic assessment in Lactobacillus plantarum links the butyrogenic pathway with glutamine metabolism. Sci. Rep. 7:15975. doi: 10.1038/s41598-017-16186-8
Bustos, P., and Bórquez, R. (2013). Influence of osmotic stress and encapsulating materials on the stability of autochthonous Lactobacillus plantarum after spray drying. Dry. Technol. 31, 57–66. doi: 10.1080/07373937.2012.717325
Chaikham, P., Kemsawasd, V., and Seesuriyachan, P. (2017). Spray drying probiotics along with maoluang juice plus Tiliacora triandra gum for exposure to the in vitro gastrointestinal environments. LWT 78, 31–40. doi: 10.1016/j.lwt.2016.12.013
Charalampopoulos, D., Pandiella, S. S., and Webb, C. (2003). Evaluation of the effect of malt, wheat and barley extracts on the viability of potentially probiotic lactic acid bacteria under acidic conditions. Int. J. Food Microbiol. 82, 133–141. doi: 10.1016/S0168-1605(02)00248-9
Chávez, B. E., and Ledeboer, A. M. (2007). Drying of probiotics: optimization of formulation and process to enhance storage survival. Dry. Technol. 25, 1193–1201. doi: 10.1080/07373930701438576
Chen, X., Liu, X. M., Tian, F., Zhang, Q., Zhang, H. P., Zhang, H., et al. (2012). Antagonistic activities of lactobacilli against Helicobacter pylori growth and infection in human gastric epithelial cells. J. Food Sci. 77, M9–14. doi: 10.1111/j.1750-3841.2011.02498.x
Cheok, C. Y., Mohd Adzahan, N., Abdul Rahman, R., Zainal Abedin, N. H., Hussain, N., Sulaiman, R., et al. (2018). Current trends of tropical fruit waste utilization. Crit. Rev. Food Sci. Nutr. 58, 335–361. doi: 10.1080/10408398.2016.1176009
Cornelia, M., Siratantri, T., and Prawita, R. (2015). The utilization of extract durian (Durio zibethinus L.) seed gum as an emulsifier in vegan mayonnaise. Proc. Food Sci. 3, 1–18. doi: 10.1016/j.profoo.2015.01.001
Desmond, C., Ross, R. P., O'Callaghan, E., Fitzgerald, G., and Stanton, C. (2002). Improved survival of Lactobacillus paracasei NFBC 338 in spray-dried powders containing gum acacia. J. Appl. Microbiol. 93, 1003–1011. doi: 10.1046/j.1365-2672.2002.01782.x
Drissi, F., Merhej, V., Angelakis, E., El Kaoutari, A., Carrière, F., Henrissat, B., et al. (2014). Comparative genomics analysis of Lactobacillus species associated with weight gain or weight protection. Nutr. Diabetes. 4:e109. doi: 10.1038/nutd.2014.6
Eratte, D., McKnight, S., Gengenbach, T. R., Dowling, K., Barrow, C. J., and Adhikari, B. P. (2015). Co-encapsulation and characterisation of omega-3 fatty acids and probiotic bacteria in whey protein isolate–gum Arabic complex coacervates. J. Funct. Foods 19, 882–892. doi: 10.1016/j.jff.2015.01.037
Ersus, S., and Yurdagel, U. (2007). Microencapsulation of anthocyanin pigments of black carrot (Daucus carota L.) by spray drier. J. Food Eng. 80, 805–812. doi: 10.1016/j.jfoodeng.2006.07.009
Ewe, J. A., Wan-Abdullah, W. N., and Liong, M. T. (2010). Viability and growth characteristics of Lactobacillus in soymilk supplemented with B-vitamins. Int. J. Food Sci. Nutr. 61, 87–107. doi: 10.3109/09637480903334163
Fu, W., and Mathews, A. P. (1999). Lactic acid production from lactose by Lactobacillus plantarum: kinetic model and effects of pH, substrate, and oxygen. Biochem. Eng. J. 3, 163–170. doi: 10.1016/S1369-703X(99)00014-5
Gardiner, G. E., O'Sullivan, E., Kelly, J., Auty, M. A., Fitzgerald, G. F., Collins, J. K., et al. (2000). Comparative survival rates of human-derived probiotic Lactobacillus paracasei and L. salivarius strains during heat treatment and spray drying. Appl. Environ. Microbiol. 66, 2605–2612.
Herías, M. V., Hessle, C., Telemo, E., Midtvedt, T., Hanson, L. Å., and Wold, A. E. (1999). Immunomodulatory effects of Lactobacillus plantarum colonizing the intestine of gnotobiotic rats. Clin. Exp. Immunol. 116, 283–290. doi: 10.1046/j.1365-2249.1999.00891.x
Herlina, Lindriati, T., Praptiningsih, Y., and Suciani, C.M. (2016). Use of crude extract water–soluble polysaccharides of durian (Durio zibethinus Murr) seeds as stabilizer for pineapple juice production. Agricult. Agricult. Sci. Proc. 9, 440–449. doi: 10.1016/j.aaspro.2016.02.161
Huang, S., Vignolles, M.-L., Chen, X. D., Le Loir, Y., Jan, G., Schuck, P., et al. (2017). Spray drying of probiotics and other food-grade bacteria: a review. Trends Food Sci. Technol. 63, 1–17. doi: 10.1016/j.tifs.2017.02.007
Iravani, S., Korbekandi, H., and Mirmohammadi, S. V. (2015). Technology and potential applications of probiotic encapsulation in fermented milk products. J. Food Sci. Technol. 52, 4679–4696. doi: 10.1007/s13197-014-1516-2
Jafari, S. M., Assadpoor, E., Bhandari, B., and He, Y. (2008). Nano-particle encapsulation of fish oil by spray drying. Food Res. Int. 41, 172–183. doi: 10.1016/j.foodres.2007.11.002
Karlton-Senaye, B. (2015). Comparing the effect of gums on the growth of lactobacillus species in laboratory medium and fluid milk. J Nutr Health Food Eng. 2:00054. doi: 10.15406/jnhfe.2015.02.00054
Karlton-Senaye, B. D., and Ibrahim, S. A. (2013). Impact of Gums on the Growth of Probiotic Microorganisms. Greensboro, NC: Springer International Publishing.
Lee, J. J., Chen, L., Shi, J., Trzcinski, A., and Chen, W. N. (2014). Metabolomic profiling of Rhodosporidium toruloides grown on glycerol for carotenoid production during different growth phases. J. Agric. Food Chem. 62, 10203–10209. doi: 10.1021/jf502987q
Lian, W. C., Hsiao, H. C., and Chou, C. C. (2002). Survival of bifidobacteria after spray-drying. Int. J. Food Microbiol. 74, 79–86. doi: 10.1016/S0168-1605(01)00733-4
Lonvaud-Funel, A. (1999). Lactic acid bacteria in the quality improvement and depreciation of wine. Antonie Van Leeuwenhoek 76, 317–331.
Mirhosseini, H., and Tabatabaee Amid, B. (2012). Influence of chemical extraction conditions on the physicochemical and functional properties of polysaccharide gum from durian (Durio zibethinus) seed. Molecules 17, 6465–64380. doi: 10.3390/molecules17066465
Paim, D. R. S. F., Costa, S. D. O., Walter, E. H. M., and Tonon, R. V. (2016). Microencapsulation of probiotic jussara (Euterpe edulis M.) juice by spray drying. LWT 74, 21–25. doi: 10.1016/j.lwt.2016.07.022
Park, S. E., Yoo, S. A., Seo, S. H., Lee, K. I., Na, C. S., and Son, H. S. (2015). GC-MS based metabolomics approach of Kimchi for the understanding of Lactobacillus plantarum fermentation characteristics. LWT 68, 313–321. doi: 10.1016/j.lwt.2015.12.046
Patel, S., and Goyal, A. (2015). Applications of natural polymer gum arabic: a review. Int. J. Food Prop. 18, 986–998. doi: 10.1080/10942912.2013.809541
Plumed-Ferrer, C., Koistinen, K. M., Tolonen, T. L., Lehesranta, S. J., Karenlampi, S. O., Makimattila, E., et al. (2008). Comparative study of sugar fermentation and protein expression patterns of two Lactobacillus plantarum strains grown in three different media. Appl. Environ. Microbiol. 74, 5349–5358. doi: 10.1128/aem.00324-08
Rana, V., Rai, P., Tiwary, A. K., Singh, R. S., Kennedy, J. F., and Knill, C. J. (2011). Modified gums: approaches and applications in drug delivery. Carbohydr. Polym. 83, 1031–1047. doi: 10.1016/j.carbpol.2010.09.010
Sheehan, V. M., Ross, P., and Fitzgerald, G. F. (2007). Assessing the acid tolerance and the technological robustness of probiotic cultures for fortification in fruit juices. Innov. Food Sci. Emerg. Technol. 8, 279–284. doi: 10.1016/j.ifset.2007.01.007
Shori, A. B. (2017). Microencapsulation improved probiotics survival during gastric transit. HAYATI J. Biosci. 24, 1–5. doi: 10.1016/j.hjb.2016.12.008
Siew, G. Y., Ng, W. L., Tan, S. W., Alitheen, N. B., Tan, S. G., and Yeap, S. K. (2018). Genetic variation and DNA fingerprinting of durian types in Malaysia using simple sequence repeat (SSR) markers. PeerJ 6:e4266. doi: 10.7717/peerj.4266
Taewee, T. (2008). Some properties of starch extracted from three thai aromatic fruit seeds. Starch Stärke 60, 199–207. doi: 10.1002/star.200800641
Teh, S. S., Ahmad, R., Wan-Abdullah, W. N., and Liong, M. T. (2009). Evaluation of agrowastes as immobilizers for probiotics in soy milk. J. Agric. Food Chem. 57, 10187–10198. doi: 10.1021/jf902003a
Valdés, A., Mellinas, A. C., Ramos, M., Garrigós, M. C., and Jiménez, A. (2014). Natural additives and agricultural wastes in biopolymer formulations for food packaging. Front. Chem. 2:6. doi: 10.3389/fchem.2014.00006
Veerkamp, J. H. (1971). Fatty acid composition of bifidobacterium and Lactobacillus strains. J. Bacteriol. 108, 861–867.
Keywords: spray drying, encapsulation, Lactobacillus plantarum, biopolymer, stability, sustainability, fermentation process, metabolomics
Citation: Lee JJL, Zhao G, Kim J, Castillo-Zacarias C, Ramirez-Arriaga MT, Parra-Saldivar R and Chen W-N (2018) Dual Use of a Biopolymer From Durian (Durio zibethinus) Seed as a Nutrient Source and Stabilizer for Spray Dried Lactobacillus Plantarum. Front. Sustain. Food Syst. 2:53. doi: 10.3389/fsufs.2018.00053
Received: 25 April 2018; Accepted: 06 August 2018;
Published: 03 September 2018.
Edited by:
Lorenzo Pastrana, Laboratório Ibérico Internacional de Nanotecnologia (INL), PortugalReviewed by:
Maria A. Longo, University of Vigo, SpainJosé Antonio Vázquez, Instituto de Investigaciones Marinas (IIM), Spain
Copyright © 2018 Lee, Zhao, Kim, Castillo-Zacarias, Ramirez-Arriaga, Parra-Saldivar and Chen. This is an open-access article distributed under the terms of the Creative Commons Attribution License (CC BY). The use, distribution or reproduction in other forums is permitted, provided the original author(s) and the copyright owner(s) are credited and that the original publication in this journal is cited, in accordance with accepted academic practice. No use, distribution or reproduction is permitted which does not comply with these terms.
*Correspondence: Jaslyn J. L. Lee, amFzbHlubGVlQG50dS5lZHUuc2c=
Wei-Ning Chen, d25jaGVuQG50dS5lZHUuc2c=