- 1UMR Metis, Sorbonne Université CNRS EPHE, Paris, France
- 2Department of Economics and Social Sciences, Institute of Social Ecology (SEC), University of Natural Resources and Life Sciences, Wien, Austria
France is a major agricultural power, characterized by a high degree of regional specialization, either in stockless cash crop farming, exporting most of its intensive cereal production, or in intensive livestock farming highly dependent on foreign feed imports. This agricultural model is characterized by wide nutrient and carbon cycle opening and severe environmental pollution. Based on the nutrient accounting GRAFS model, two contrasted scenarios for the French agricultural system at the 2050 horizon have recently been designed and evaluated for their capacity to meet both the national population's food demand and environmental standards in terms of water pollution. The first scenario (O/S, for opening and specialization) assumes the continuation of the current trends of intensification, specialization, and opening to international markets. The second one (A/R/D, for autonomy, reconnection, and demitarian diet) assumes a radical change toward organic farming with diversification of crop rotations, reconnection of crop and livestock farming, and reduction of the proportion of animal proteins in the human diet. Herein we calculate the budget of CO2 emissions and C sequestration in soils of these two scenarios compared with the current situation of the French agro-food system, by coupling the GRAFS and AMG models. These simulations reveal that the overall CO2 emissions balance of the O/S scenario is far higher than those of the A/R/D, namely because of the emissions associated with mineral fertilizer manufacture, and imported feed and mechanization of land management requiring a large amount of fossil fuel. As the organic carbon content of the soil is known to be highly path-dependent (in the sense that it is the inheritance of previous land use practices), we tested the effect of two rates of implementation of the two scenarios and evaluated the response time of the C soil store, which is of the order of two decades or more. This reveals that after about two-three decades following the implementation of a scenario, an equilibrium is reached with no more net soil C emission nor sequestration.
Introduction
The 4% initiative (http://www.4p1000.org/) launched by France at the COP 21 in 2015 stressed the fact that carbon (C) sequestration in agricultural soil could be part of a mitigation strategy of greenhouse gas emissions. Some authors even went so far as to say: “the concept that soils and agriculture are solutions to the global issue of climate change, food insecurity, and environmental pollution, is a major shift of historical significance” (Lal, 2016). This paper is an attempt to assess this statement for the paradigmatic case of French agriculture.
The behavior of agricultural soils as a sink or source of C to the atmosphere results from the equilibrium between inputs and mineralization of humified C to the soil (Jenkinson and Rayner, 1977; Poulton et al., 2018). The former depends on the quantity and nature of fresh organic material inputs (Kong et al., 2005; Autret et al., 2016) and the latter on the pedo-climatic context (Kätterer et al., 1998; Stockmann et al., 2013). These conditions are themselves constrained by the agricultural practices such as fertilization and motorization of agriculture, the production patterns of food and feed, and the historical trajectories of agro-food systems, which determine the current C dynamic in soils (Aguilera et al., 2018; Le Noë et al., 2019).
Whether the agricultural sector can be considered as having a potential for climate change mitigation through C storage in soil also depends on the C emissions generated by the use of fossil-fuel for agricultural production. Gingrich et al. (2007) and Erb et al. (2007) have indeed underlined that an increased soil C sequestration was only made possible in the mid-twentieth century owing to an unprecedented use of non-solar energy in agriculture, a phenomenon described as a “fossil-fuel-powered carbon sink.” However, Garnier et al. (2019) showed that CO2 emissions account for 22% of the French agricultural greenhouse gas emissions. Therefore, the future challenges of climate change mitigation and food safety needs to account for the systemic effects of the structure of the agro-food system and the legacies left out by the past. Accounting for the structural causes of C emission/sequestration in agricultural soil and challenging them echo the last IPCC (2018) stating that “limiting global warming to 1.5°C would require rapid, far-reaching and unprecedented changes in all aspects of society.”
Designing prospective scenarios for the future of the agro-food system as a whole makes it possible to consider the effects of a combination of factors on the environmental imprint of the agro-food system. Recently, several studies used this contrasted scenario building approach to investigate their consequences on the ability to feed humans while preserving ecosystems. At the global scale, Billen et al. (2015) and Erb et al. (2016) highlighted that a wide array of possibilities to feed the world at the 2050 horizon exists but only some of them can do it in a sustainable way for ecosystems. Other studies (Kastner et al., 2012; Lassaletta et al., 2016; Muller et al., 2017; Billen et al., 2018a,b) emphasized the role of human diet, agricultural practices and specialization of agro-food systems as important drivers of their environmental impact at the regional and global scales. To our knowledge, none of these studies investigated the impact of such scenarios on C soil sequestration together with C emissions from agriculture. Such a study would inform the issue of climate change mitigation through C sequestration in agricultural land. However, as C sequestration from agricultural land depends on local pedo-climatic conditions and C inputs to soils, the regional level is more suited than the national or global scale to investigate the effect of different scenarios on C sequestration. Furthermore, to account for the C budget of agriculture requires also considering the C emissions released by energy consumption in agriculture.
In this context, here we explore the impact of two contrasted prospective scenarios designed by Billen et al. (2018a) on the C emissions budget of the French agro-food systems at the regional scale at the 2050 horizon. The first scenario (O/S, for opening and specialization) assumes the continuation of the current trends of intensification, specialization and opening to international markets. The second one (A/R/D, for autonomy, reconnection and demitarian diet) assumes a radical change toward organic farming with diversification of crop rotations, reconnection of crop and livestock farming, and reduction of the proportion of animal proteins in the human diet. We coupled the GRAFS approach (Billen et al., 2014; Le Noë et al., 2018) and the AMG model (Saffih-Hdadi and Mary, 2008; Milesi Delaye et al., 2013) to calculate the C dynamic in agricultural soil in the two scenarios, prolonging the trajectory already described by Le Noë et al. (2019). We then estimated the C emissions related to energy consumption in agriculture accounting for direct (machinery and heating) and indirect (N and P fertilizer manufacture and feed importation) emissions using the coefficients provided by Doublet (2011) (see also Garnier et al., 2019). The balance between net sequestration of C in agricultural soils and C emissions by energy consumption provides a budget of net C emissions by the agricultural sector for the two scenarios. By doing so we also aimed at answering two related questions: (i) How could structural changes in agro-food systems influence C fluxes and CO2 emissions; (ii) Which paths could allow us to engage a transition in agro-food systems toward more sustainability regarding the C emissions budget?
Materials and Methods
Two Contrasted Scenarios for Agro-Food System at the 2050 Horizon
The same assumptions as those described by Billen et al. (2018a) are used to quantitatively assess the impact of two contrasted scenarios on the net C emissions budget in the 33 French agricultural regions as defined by Le Noë et al. (2016). Briefly summarizing, the O/S scenario assumes a pursuit of specialization with a disconnection of livestock and crop production, a current human diet with a proportion of animal protein reaching 60% of the protein ingested and a strong dependence on mineral fertilizer and feed import. By contrast the A/R/D scenario is characterized by a reconnection of crop and livestock production, the generalization of organic agriculture practices and the autonomy regarding livestock production with no feed importation to meet the demand of livestock nutrition in each region. Both scenarios are able to feed the French population; the O/S scenario exports large amounts of cereals and other vegetal as well as animal products but depends on large imports of protein feed, while the A/R/D scenario is self-sufficient for animal products and feed, but can still export cereals to the international market at more than 40% the rate of current cereal exports.
These prospective scenarios for French regional agro-food systems can be represented in terms of C fluxes with the nutrient-accounting GRAFS model (Billen et al., 2014; Le Noë et al., 2018, 2019) (Figure 1). It must be made clear from this figure that only the proportion of net primary production (NPP) (above- and below-ground) that is neither exported nor respired by livestock and humans is brought to the soil as either crop residues or manure; of these only the fraction which is not mineralized can be considered as sequestered in the soil, in the end comprising a very tiny share of NPP.
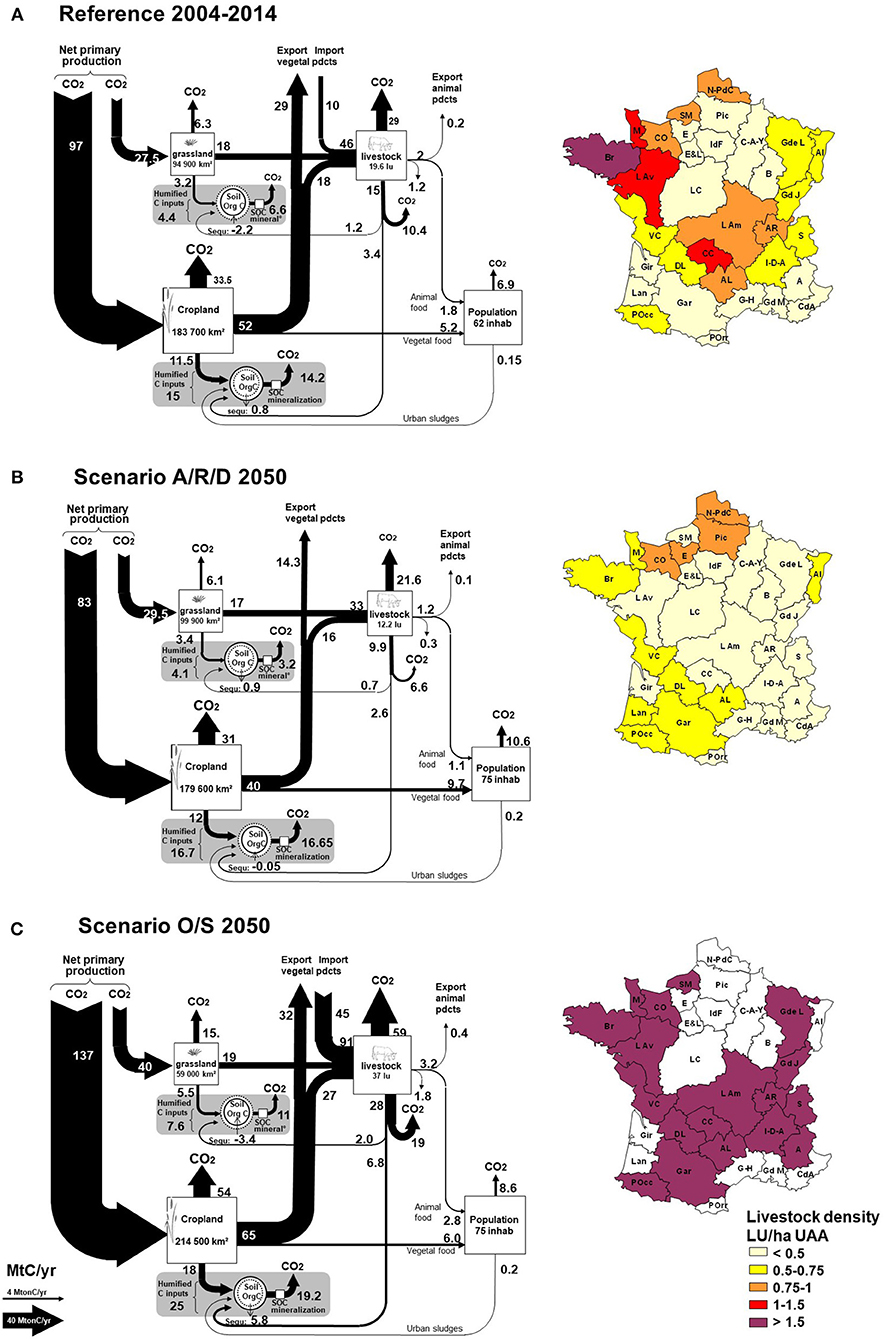
Figure 1. GRAFS representation of the C fluxes within the French agro-food system for the current (reference) situation in 2004–2014 (A) and for the A/R/D (B) and O/S (C) scenarios at the 2050 horizon. The corresponding geographical distribution of livestock density (LU ha−1) is also shown.
The O/S scenario uses the projections made by the National Institute for Statistics and Economic Studies (INSEE; www.insee.fr) to estimate population growth and its geographical distribution, which concentrates in large metropoles, while the A/R/D scenario hypothesizes a more homogeneous distribution of the new population in favor of small and medium cities. In the former scenario, an increased population leads to an increased urban area, which we assumed to be subtracted from cropland, while, in the latter, cities are assumed to densify instead of increasing their spatial extension, and the total agricultural area is kept constant at its current value (see Billen et al., 2018a).
Regarding the cropping system, the O/S scenario hypothesizes a strengthening of the current specialization of agricultural regions (Le Noë et al., 2017), with no changes in the crop rotation and farming practices, although the rate of chemical fertilization is adjusted up to a cropland N soil balance of 50 kgN ha−1 yr−1 according to strict environmental regulations. The corresponding yield is calculated using the relationship established by Anglade et al. (2015) between yields (Y, kgN ha−1 yr−1) and total N inputs to agricultural soils (F, kgN ha−1 yr−1), including biological N fixation, mineral fertilizer, seeds, atmospheric deposition and manure recycling. This is expressed as the following hyperbolic function with the value of the parameter Ymax depending on the pedo-climatic context of each region:
The A/R/D scenario assumes a generalization of organic farming systems. Cropping systems are estimated based on the current literature on organic farming in different French regions (ITAB, 2011; Billen et al., 2018a). The main changes are the increased share of fodder legume crops and the reduction of cereal production. Yields are calculated according to the relationship (1) considering legume N fixation, manure application and atmospheric deposition as sole N inputs. Furthermore, in the A/R/D scenario, a systematic use of cover crop has been assumed for organic farming practices, accounting for an additional input of fresh residues to the soil of ca. 0.11 tC ha−1 yr−1 (Constantin et al., 2010; Justes et al., 2012), estimating that 70% of cropland would be planted with cover crop.
Similarly, the production of permanent grassland systems is calculated from the total N input and the current Ymax value for grassland, considering the current rate of symbiotic atmospheric N fixation. The cropland and grassland productions are subsequently translated in terms of C using the coefficients gathered by Le Noë et al. (2018, 2019). The NPP can be derived from the calculated harvest production applying the approach reported by Bolinder et al. (2007). This approach considers C allocation between the harvested product, the above-ground residues and the root residues, the latter two being calculated as a fraction of the C in the harvested product. The fraction of C residues that is not exported represents the C input to cropland and grassland.
Ymax values for both crops and grassland in each region are considered constant over the whole period of simulation, thus neglecting possible effects of climate change on agricultural production.
Regarding livestock in the O/S scenario, its density increases up to 2 LU ha UAA−1 (livestock units per hectare utilized agricultural area) in all regions except the Paris Central Basin, Nord-Pas-de-Calais, Alsace, Gironde, Landes, and the regions of the extreme southeast, which were already specialized in an intensive cropland system in the 2004–2014 period and which are assumed to become completely devoid of livestock and grassland at the 2040–2050 horizon. If local cropland and grassland production is not sufficient to feed cattle, the unmet need is covered by feed importation from abroad. Conversely, in the A/R/D scenario, all regions are assumed to move toward an integrated crop and livestock farming mode of production. To that end, livestock densities are adjusted to the available grass and fodder production of each region, which implies complete regional self-sufficiency for animal production. Grassland areas are adjusted to meet at least one-third of livestock needs.
All these hypotheses regarding both scenarios bear a series of constraints that are compiled and applied to all regions using a simple Microsoft Excel VBA macro (Billen et al., 2018a). Output variables include livestock, crop and grass production, C inputs to cropland and grassland via manure and crop residues, cropland and grassland surfaces, and feed importation.
C Sequestration in Soils
Net emissions of C from cropland and grassland are calculated by coupling the GRAFS approach with the AMG model (Saffih-Hdadi and Mary, 2008). This method has been extensively described by Le Noë et al. (2019). Briefly summarizing, the AMG model considers three pools of C: fresh organic C inputs as well as soil labile organic C and inert soil organic C. Humified C inputs to the soil are estimated from the GRAFS data considering three main types of inputs: manure, above-ground residues, and below-ground residues. The balance between annual humified C input and output via labile SOC mineralization drives the change in the total SOC pool of cropland in the absence of land-use change. Although the AMG approach was initially developed for cropland, we also applied it to grassland in this study.
Because of the slow rate of change of soil organic carbon stock (SOC) and its resulting long inertia, it is necessary to know its past dynamics to simulate the trends of the prospective scenarios. Therefore, we used the estimation of SOC trajectories in agricultural soil of French regions from 1852 to 2014 already calculated by Le Noë et al. (2019) to initialize the calculation of the future scenarios. Data sources to estimate C inputs to cropland and grassland include the harvested production and number of livestock reported by Agreste (2017) for the 1970–2014 period and by non-digitized official registers (available from Gallica.bnf.fr) from 1852 to 1965, the coefficient of animal excretion rates for current and past periods (Le Noë et al., 2018) for the 39 livestock categories considered, humification rates of fresh C inputs (Justes et al., 2009; Clivot et al., 2017) and the distribution of manure application between cropland and grassland, which has been documented or estimated by Le Noë et al. (2018). The mineralization rate of the soil labile organic C is a function of several pedo-climatic parameters: clay content, soil pH, soil carbonate content, air temperature, humidity and the soil C:N ratio (Clivot et al., 2017). These data have been gathered for French regions by Le Noë et al. (2019), as well as temperature changes. Data sources include Ballabio et al. (2016) for clay content in the top 30 cm of cropland and grassland soil, Moisselin et al. (2002) and Raimonet et al. (2017) for air temperature, the HYDRO data bank for humidity and the Network of Measurements and Quality of Soil provided by the French National Institute of Agronomy (RMQS, INRA) for the C:N ratio, pH and carbonate content in the top 30 cm of cropland and grassland soil.
The coupling of the GRAFS description of the two scenarios to the AMG model makes it possible to calculate the dynamics of SOC in cropland and grassland, assuming a gradual implementation of the scenarios starting from 2014 and reaching its final state in 2050, following a linear trajectory. The extension by 10 years of the scenarios originally designed by Billen et al. (2018a) for 2040 allows one to better estimate soil C dynamics as a mean over a 10 year period, from 2040 to 2050. An average temperature increase of 1.5°C by 2050 in the continuity of the increase observed over the last decades is also assumed. Other pedo-climatic parameters were kept constant over the simulated period. The calculation provides the variations in the C content of the cropland's and grassland's upper 30 cm soil layer (tonC ha−1). The net C emissions from agricultural soils of each region over the last decade of the scenario (tonC ha−1 yr−1) is estimated from these results, as the average rate of change during 10 years of C stock in grassland and cropland, taking into account the changes in surface area as follows:
where net C emissions UAA is the annual average net C emissions from agricultural soil over the 2040–2050 period in region i (tonC-CO2 ha UAA−1 yr−1);
Stock C CL/GL yr i is the simulated SOC stock in cropland or grassland in any year (yr expressed in tonC yr−1), obtained as the product of SOC content (tonC ha−1) by the corresponding area of cropland or grassland of the region (ha);
Ha UAA2050 i is the surface of utilized agricultural area in 2050 (ha UAA). This calculation procedure accounts for the shifts in SOC stocks induced both by change in SOC content, which depends on C dynamics in soil, and changes in cropland and grassland areas as a result of the hypotheses for each scenario.
Carbon net emissions from agricultural soils were calculated for both scenarios (average over the 2040–2050 decade) and for the reference situation (average over the 2004–2014 period). Positive net emission indicates that agricultural soils are a source of C, either because soils release C or because of a reduction of agricultural surface leading to a decrease of the total C stock in soil. Negative net emission indicates that agricultural soils are a sink of C, either because soils accumulate C or because of an increase in agricultural surface leading to an increase of the total C stock in agricultural soil.
The details of the calculations are provided in the xls files appended as Supplementary Materials SM1–SM3 to this paper.
C Emissions Linked to Energy Consumption of the Agricultural Sector
The C-CO2 emissions related to energy consumption in agriculture includes mechanical and motorized work, building heating, mineral fertilizer manufacture, production, and transport of imported feed. We calculated these emissions based on the data provided by Doublet (2011) for the French agricultural sector in 2006, which are summarized in Table 1.
We used these figures to estimate C-CO2 emissions related to energy consumption in agriculture in both prospective scenarios and for the current situation, assuming that no significant technical evolution would occur in both scenarios so that coefficients remain valid and C-CO2 emissions only depend on the use of mineral fertilizers, importation of feed, and cultivated and grazed surfaces. Note also that emissions related to pesticide products, irrigation, mineral K fertilizer and lime inputs were not accounted here, as they represented <10% of agricultural emissions in 2006.
Budget of Net C Emissions in Agriculture
The budget of net C emissions of each French region was calculated as the sum of net emissions from agricultural soils and the net emissions related to energy consumption by agriculture. A positive budget indicates that the agriculture sector is a net emitter of C-CO2 while a negative budget indicates that it is a net sink for atmospheric C-CO2 through soil sequestration.
Sensitivity Analysis
Since the organic carbon content of the soil is known to be highly path-dependent (in the sense that it follows the inheritance of previous land use practices), we tested the effect of different rates and timing of implementation of the two scenarios and evaluated the response time of the C soil storage. To that end two alternative pathways to the same final scenarios were calculated: (i) the scenarios follow an early and rapid linear implementation for 10 years from 2014 and the systems then remain in the same configuration from 2024 to 2050; (ii) the current situation remains constant until 2040 and the scenarios are then rapidly implemented following a linear pathway from 2040 to 2050.
Results
At the National Level
Both prospective scenarios and the reference level for the 2004–2014 period reveal positive net C emission budgets in agriculture at the national level. However, the extent to which the agricultural sector is a contributor of net C emissions to the atmosphere varies greatly between the three situations. The carbon budget is the highest in the current reference system, with net emissions amounting to 7.4 MtC yr−1, but is very close to the C emissions budget of the O/S scenario (6.6 MtC yr−1). By contrast, the A/R/D scenario would enable agriculture to reduce its net emissions to 2.7 MtC yr−1, more than 2.5 times lower. These values result from the balance between net emissions from agricultural soil and from energy consumption in agriculture.
In the current reference system, net C emissions from agricultural soil result from both the long-term past SOC dynamics in cropland and grassland and recent changes in agricultural land surfaces. Although C concentrations in agricultural soils tend to increase due to a slow increase of humified C inputs, the reduction of agricultural areas, more specifically of grassland surfaces led agricultural soils to be net emitters of C over the 2004–2014 period (Figure 2; Table 2). These losses of C from agricultural soils are added to C emissions for energy consumption in agriculture, which reached 6 MtC yr−1 over the 2004–2014 period. Mechanized work for animal husbandry, crop- and grassland account for 56% of these emissions while mineral fertilizer manufacture and feed importation account for 37 and 8%, respectively.
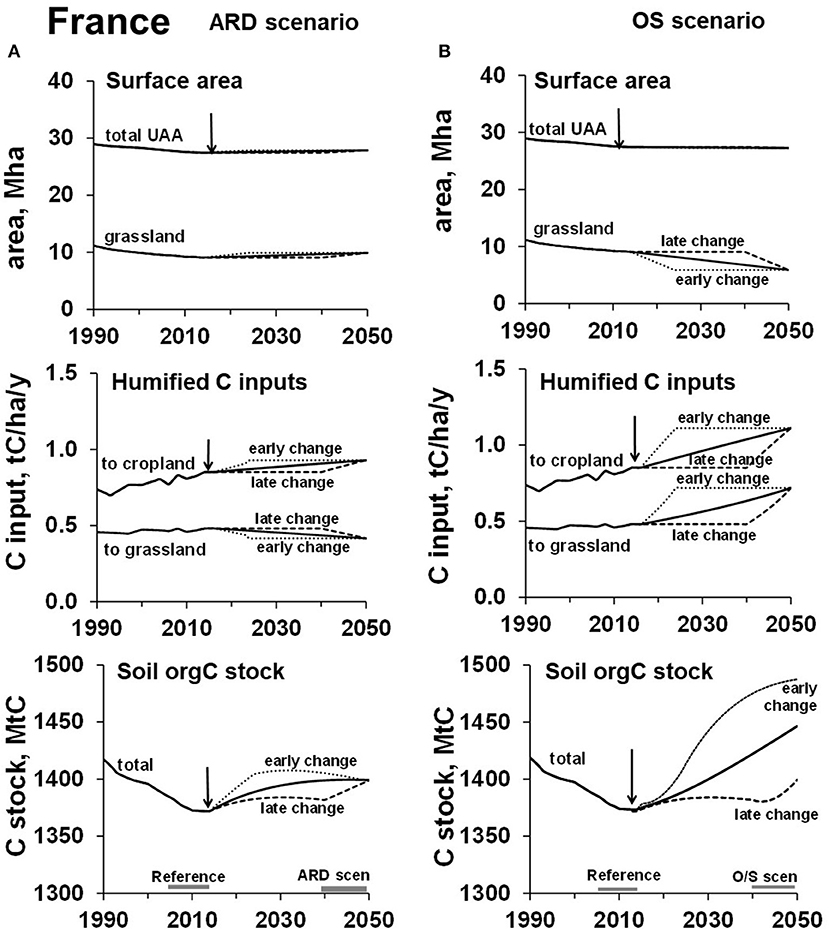
Figure 2. (A,B) Past and future trajectory of land use humified C inputs to cropland and grassland, and resulting evolution of the total SOC in agricultural (crop- and grassland) soils at the scale of France for the OS and ARD scenarios. The dotted and dashed lines, respectively, represent the effects of rapid early or late implementation of the scenario around the central hypothesis of a gradual, linear change from 2014 to 2050.
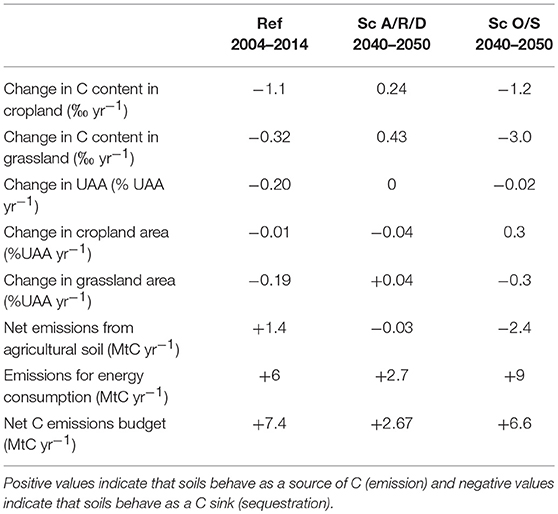
Table 2. Main variables involved in net C emissions of the French agricultural sector (national level) for the current reference situation (2004–2014) and the two contrasted scenarios.
In the A/R/D scenario (Figure 2), agricultural surfaces remain stable and humified C inputs to cropland increase slightly owing to the implementation of cover crops. Inputs to grassland slightly decrease however due to lower livestock density. Overall, these changes lead to a slight increase of soil organic C stock, which is offset however from 2040 on by enhanced C mineralization rates provoked by the temperature rise (Figure 2). At the end of the period, the net effect is a slight decrease of soil C content, with average rate of emission over the 2040–2050 period reaching 0.01 and 0.02 tC ha−1 yr−1 from cropland and grassland, respectively. However, as grassland surfaces increase due to de-specialization of intensive cropping systems and de-intensification of intensive livestock systems, agricultural soils would, on the whole, reach a nearly balanced net emission budget of −0.03 Mt C yr−1 (Figure 2; Table 2). However, in the absence of mineral N fertilizer and feed imports C emissions induced by direct and indirect energy consumption for agriculture would be much lower, reaching only 2.7 MtC yr−1. About 76% of these C emissions would be caused by mechanization and other machinery, P mineral fertilizer would account for another 24% of emissions.
By contrast, in the O/S scenario, despite losses of grassland surfaces, increased humified C inputs through manure and crop residues would lead to an average build-up of C stocks in agricultural soils as high as 2.4 MtC yr−1 (i.e., negative net emissions of −2.4 MtC yr−1) over the period from 2040 to 2050 (Figure 2; Table 2). The most significant losses of C from soil would arise from grassland change into cropland (average losses of 3.4 MtC yr−1). While C stocks per hectare of grassland would still accumulate at a rate of 3% yr−1 (0.15 tC ha−1 yr−1), grassland surface reduction is responsible for a net loss of C stock (Table 2). This is a result of the specialization hypothesis in which both intensive cropping systems and livestock systems require no or very little grassland area. However, in this scenario, increased stocks of C in cropland soils resulting from improved manure and residue inputs would be permitted by substantial recourse to mineral N fertilizer (in crop-dominated regions) and imported feed (in livestock farming regions). As a consequence, carbon emissions due to consumption of fossil fuel for agriculture would reach 9 MtC yr−1. About 25% of these C emissions would be caused by production and transport of imported feed, mineral fertilizer would account for another 26%, while mechanization and other machinery would contribute 49% of emissions.
At the Regional Level
Analyzing results only at the national level conceals significant disparities at the regional level, strongly linked to the current specialization of agricultural regions, into either intensive livestock farming (e.g., Brittany), stockless cash crop farming (e.g., Eure) or mixed crop and livestock farming (e.g., Loire Amont), as described by Le Noë et al. (2018). The application of the scenario hypotheses results in different dynamics in terms of land use change and humified C inputs to soils, as illustrated in Figure 3. Figures 4, 5 reveal the very different patterns at the regional scale in the changes of permanent grassland and cropland areas, as well as in the net C emission budgets.
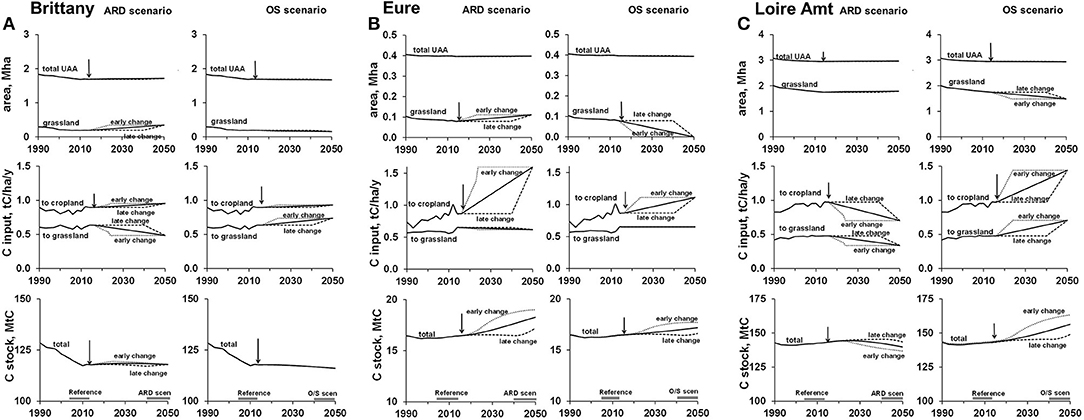
Figure 3. (A–C) Past and future trajectory of land use humified C inputs to cropland and grassland, and resulting evolution of the total SOC in agricultural (crop- and grassland) soils at the scale of three examples of agricultural regions in France for the OS and ARD scenarios. The dotted and dashed lines, respectively, represent the effects of rapid early or late implementation of the scenarios around the central hypothesis of a gradual, linear change from 2014 to 2050.
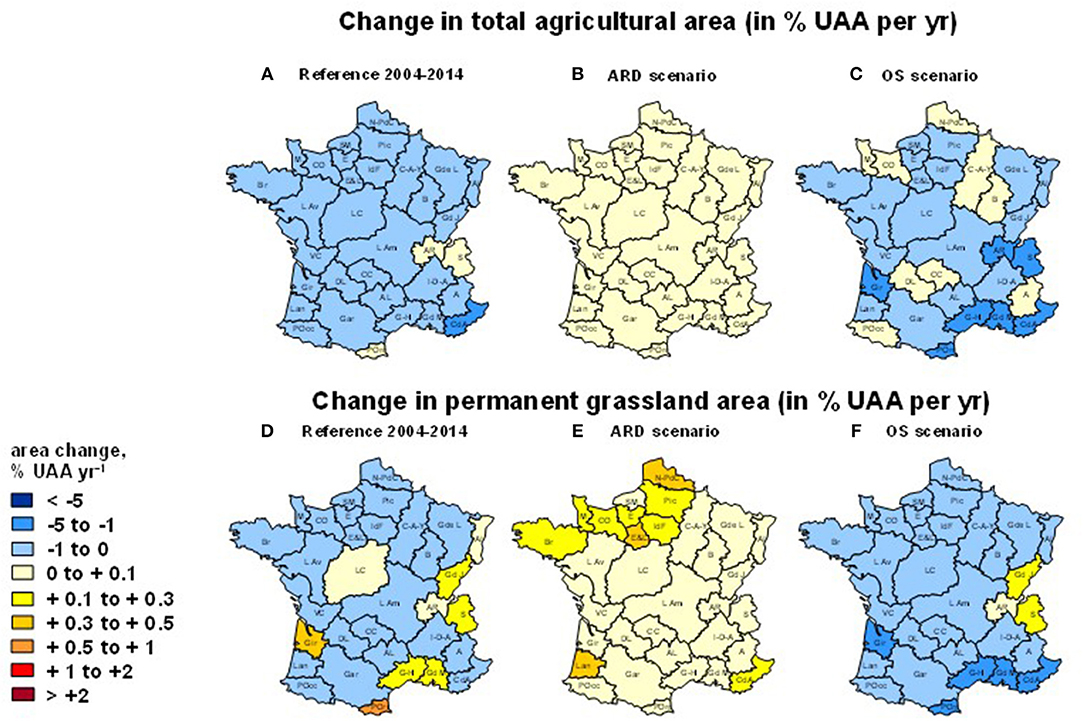
Figure 4. Relative change in total usable agricultural area (A–C) and permanent grassland area (D–F) (% of total usable agricultural area per year) in the reference case (A,D), the A/R/D scenario (B,E) and O/S scenario (C,F).
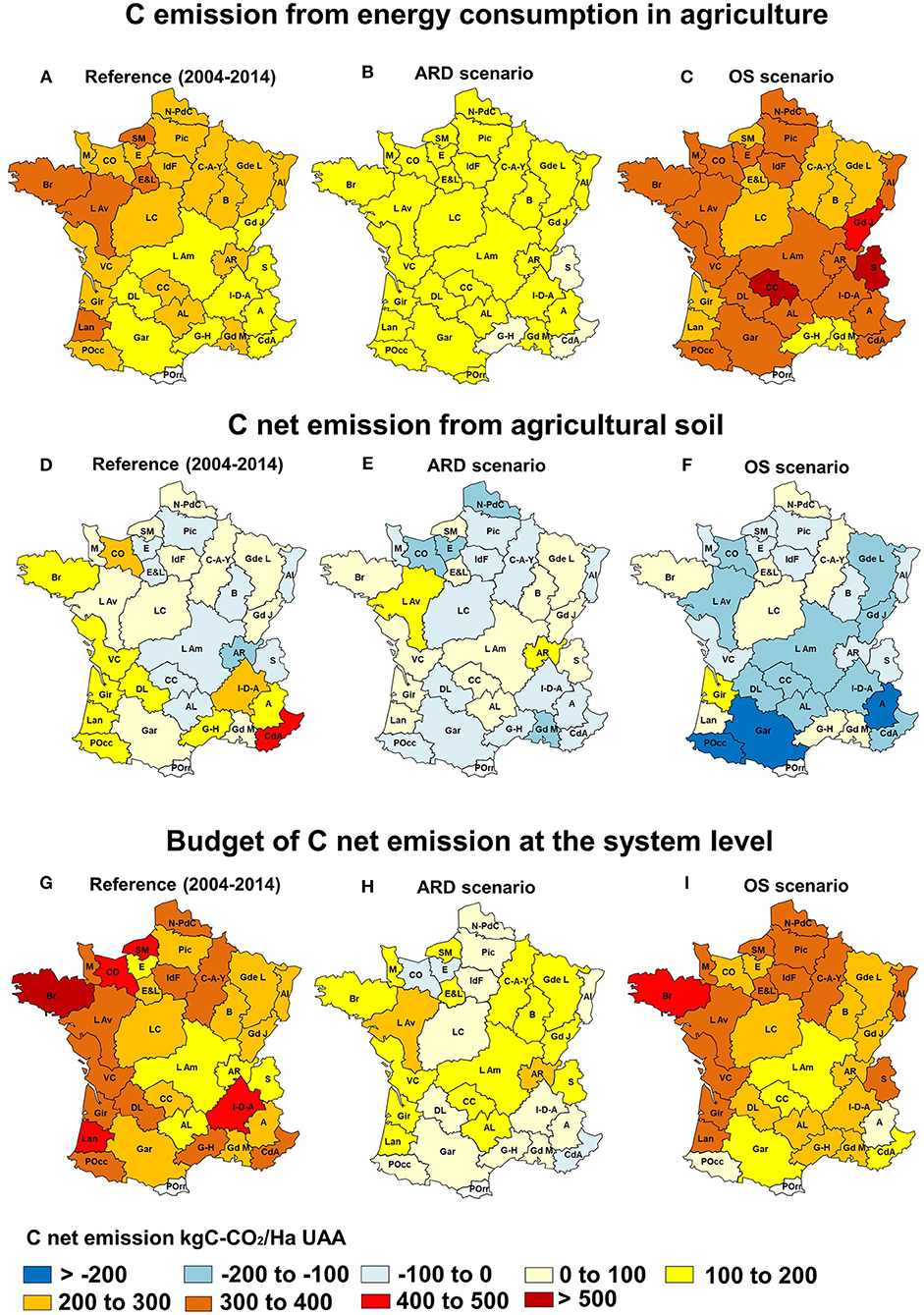
Figure 5. Net C emissions from energy consumption in agriculture for the reference situation (2014–2014) (A), A/R/D (B), and O/S (C) scenarios; net C emissions from agricultural soil for the reference situation (2014–2014) (D), A/R/D (E), and O/S (F) scenarios; budget of net C emissions of the agricultural sector (2014–2014) (G), A/R/D (H), and O/S (I) scenarios. All figures are expressed in kgC-CO2 ha UAA−1. Note that net C emissions from agricultural soils refer to the C balance from agricultural soils, with positive values indicating that soils behave as a source of C (emission) and negative values indicating that soils behave as a C sink (sequestration). Region names: A, Alpes; Al, Alsace; AL, Aveyron-Lozère; AR, Ain-Rhône; B, Bourgogne; Br, Bretagne; C-A-Y, Champagne-Ardennes-Yonne; CC, Cantal-Corrèze; CdA, Côte d'Azur; CO, Calvados-Orne; DL, Dordogne-Lot; E, Eure; EandL, Eure-et-Loire; Gar, Garonne; Gd J, Grand Jura; Gd M, Grand Marseille; Gde L, Grande Lorraine; G-H, Gard-Hérault; Gir, Gironde; I-D-A, Isère-Drôme-Ardèche; IdF, Ile de France; L Am, Loire Amont; L Av, Loire Aval; Lan, Landes; LC, Loire Centrale; M, Manche; N-PdC, Nord Pas-de-Calais; Pic, Picardie; Pocc, Pyrénées Occidentales; POr, Pyrénées Orientales; S, Savoie; VC, Vendée-Charentes.
In the reference situation, the highest net C-emitting region is Brittany followed by Calvados-Orne, Isère-Drôme-Ardèche, Landes, and Seine Maritime (Figure 4). These regions are characterized by C emissions for energy consumption above 200 kg C-CO2 ha UAA−1 (except for Isère-Drôme-Ardèche) and net positive C emissions from agricultural soils above 200 kg C-CO2 ha UAA−1 (except for Seine Maritime, see Figure 4). These high C emissions for energy consumption in agriculture are due to intensive livestock breeding (in the case of Brittany, livestock breeding contributes more than 50% of net C emissions for energy consumption) or to high inputs of mineral fertilizer (in the case of Landes, inputs of mineral fertilizer contribute more than 50% of net C emissions). Net C emissions from agricultural soils are mostly due to a reduction in agricultural surfaces (Figure 5) but also arise from SOC mineralization that is not offset by fresh inputs of C (as in Brittany and Calvados-Orne). Indeed we showed in a previous study that a reduction of SOC content was mostly due to a decrease of fresh C inputs and NPP, especially from fodder and temporary grassland (Le Noë et al., 2019). By contrast, regions with the lowest net C emissions budgets are Loire Amont, Ain-Rhône, Aveyron-Lozère, Eure, and Savoie. In these regions, C emissions for energy consumption varies from 100 to 300 kg C-CO2 ha UAA−1 (Figure 4) while agricultural soils always behave as a C sink, i.e. a negative net emitter of at least −100 kg C-CO2 ha UAA−1 (Figures 3, 4). The increase in SOC stocks in agricultural soils in these regions results from C accumulation in cropland and permanent grassland at rates between 0.24 and 4.4% yr−1 and between 0.14 and 0.74% yr−1, respectively.
In the A/R/D scenario, most regions have a net C emissions budget between 0 and 200 kg C-CO2 ha UAA−1 (Figure 3B). These values can be explained by relatively low C emissions for energy consumption in almost all regions (between 100 and 200 kg C-CO2 ha UAA−1; see Figure 5B), which are significantly reduced compared to the reference situation (Figure 5A). As mentioned in section At the National Level, such a reduction in energy consumption could be reached in this scenario due to the absence of mineral N fertilizer use for cropping and self-sufficiency for livestock production, allowing a drop in feed imports and associated C emissions. In a few regions where the expansion of grassland areas leads to increased SOC stocks (Calvados-Orne, Eure), the combined effect of both low energy consumption in agriculture and negativenet C emissions from agricultural soils results in an overall negative net emissions budget (Figure 2), indicating that agriculture as a whole is contributing to CO2 emissions mitigation by C sequestration in soil in these few regions.
In the O/S scenario, Brittany is still the most net C-emitting region with net emissions reaching 470 kg C-CO2 ha UAA−1 (Figure 5I). This high C budget results from the coincidence between high C emissions from energy consumption (Figure 5C) and net C emissions from agricultural soils (Figure 5F), the latter emissions being the outcome of both SOC mineralization from cropland and shrinking grassland surface. These evolutions result from the reinforcement of intensive livestock specialization in Brittany and the subsequent increase of animal feed and decrease of grassland surface. Several other regions have a net C-emitting budget above 300 kg C-CO2 ha UAA−1 yr−1. As agricultural soils of these regions often behave as a C sink, their relatively high net C emissions budget has to be attributed to increased C emissions for energy consumption, which arise from the increased use of mineral fertilizer and/or the increased livestock density and feed importation. The least emitting regions are those characterized by negative net C emissions from agricultural soil (e.g., Loire Amont, Cantal-Corèze, Garonne, etc.; see Figure 5F). In these regions, increased SOC stocks in agricultural soils can be attributed to C accumulation in cropland and permanent grassland due to rising C inputs to soils stemming from a boost of NPP and/or additional manure inputs.
Sensitivity to Scenario Development Over Time
A sensitivity analysis was carried out to assess the extent to which the design and hypothesis of the modeled scenarios regarding the timing and rate of the change from the current situation to one or the other scenario tested for 2050 affect the above results (Figures 2, 3).
At the national level, our analysis indicates that an early and rapid change starting in 2014 and completed in 2024 would lead to higher SOC stocks in 2050 in agricultural soils in the O/S scenario compared with the level of SOC stocks as calculated with the reference assumption of a slower linear evolution from 2014 to 2050 (Figure 4). The rate of C sequestration in the 2040–2050 period would be lower, however, as the SOC stock would be nearer equilibrium with respect to the rate of humified C input. The opposite is true for a delayed implementation of the scenario.
As far as the A/R/D scenario is concerned, in spite of deep changes in the agro-food system, the rate of humified C inputs remains relatively stable at the national level, so that the effect of the different transition timing is less visible and the SOC stock reached in 2050 is quite similar whatever the implementation pattern (Figure 2). At the regional level however, significant differences can result from different timing of scenario implementation, as for instance in the Eure region (Figure 3).
Overall, this test highlights the temporary nature of C sequestration in agricultural soils: an early rapid 10 year change leads the SOC stock to reach an equilibrium with respect to the rate of humified inputs at the 2050 horizon. This equilibrium means that neither sequestration nor soil net CO2 emissions continues to occur. C sequestration in agricultural soil is therefore only a transient characteristic of a given scenario.
Discussion and Conclusion
Limits of the Approach
A scenario is by no means a prediction of the future, but simply a representation of what it could be under certain constraints. Even so, representing such a projection of a possible future in an intelligible way requires simplifying the system under study and considering only certain parameters to model, leaving aside other aspects of the system, thus limiting the model.
In the present study, it must be stressed that a major restriction to our hypothesis is the non-inclusion of forest as part of the C budget. Losses or gains of C in cropland and grassland soils represent here the combined effects of changes in SOC content in soils and changes in agricultural land surfaces. When reduction in agricultural surfaces results from extension of urbanized areas, this indeed represents an effective loss of C from the agricultural system. However, if land-use change in agriculture is linked to change in the forest area, then the estimated change in SOC stocks of agricultural soils may not correspond to net emissions of C-CO2 to the atmosphere. Purposedly, in the scenarios tested here, no expansion of cropland or grassland at the expense of forest area and no expansion of forest over agricultural land were taken into account, forest area remaining thus strictly constant in both scenarios. A deeper prospective investigation, not only into the agro-food system, but also into the overall land-use system could articulate a vision of how all land uses are related to one another in a given socio-ecological scenario.
Another restriction of this study is that it is limited to the C emissions budget and does not take into consideration non-CO2 greenhouse gas emissions by agriculture, namely methane and nitrous oxide, which also contribute to global warming. This aspect is taken into account in another paper (Garnier et al., 2019).
Several other aspects have not been taken into account, as for instance the possible effect of soil erosion on the soil C budget. Also, by lack of robust models for predicting climate change in the next 50 years at the scale of the French regions as defined here, we only considered a gradual 1.5°C temperature increase from 2014 to 2050 with respect to the current seasonal temperature variations in each region, as estimated from the mere linear extrapolation of the trend observed in the last 20 year (Le Noë et al., 2019).
Impact of Two Contrasted Future Agro-Food Systems on CO2 Emissions
In spite of the above limitations, our analysis of the C emissions budget reveals that the current French agricultural sector is by no means a net sink of C, as C sequestration in agricultural soils is far from compensating the CO2 emissions linked to fossil fuel combustion for mechanization, fertilizer manufacture and import of feed. The dependence of modern agriculture on fossil fuels and industrial fertilization, and its openness to distant markets, are the structural causes of this largely CO2-emitting balance.
The O/S scenario depicting a future in which these trends of opening and specialization would be continued and reinforced, even while meeting the current environmental regulation regarding fertilization, would temporarily increase C sequestration in agricultural soil, owing to a larger NPP, hence an increased C input to the soil. It does not result in a very significant decrease of the net emitting CO2 budget of agriculture, however (Table 2).
The A/R/D scenario, on the other hand, represents a quite different vision of the future, based on the extension of some low-level trends already emerging today toward a more local connection between food consumption and production, and between crop and livestock farming, as well as a more frugal food regime (Billen et al., 2018b). This scenario implies a complete overhaul of the current structure of the agro-food system, including the generalization of agro-ecological practices. This scenario is able to strongly reduce the net CO2-emitting budget of the agricultural sector, not because of increased C sequestration in agricultural soils but because of a strong reduction in fossil fuel consumption. This A/R/D scenario implies a de-intensification of crop production, and hence would sequester less C than the O/S scenario.
We can therefore conclude that a deep redesign of the structure of the agro-food system, toward more autonomy and local connection and less dependency on fossil fuel would reduce CO2 emissions by the agriculture sector. Our results also show that the role of C sequestration in agricultural soils, although quite desirable in terms of improvement of their agronomical properties, has rather limited effects for mitigating C emissions. Moreover, this effect is limited in time because it accompanies the changing period but would disappear once the SOC pool has reached its equilibrium, as clearly seen in Figures 2, 3, and previously discussed by Aguilera and Lassaletta (2015). In terms of strategy for limiting CO2 emissions by agriculture, the priority target should therefore be placed on the reduction of transport of feed, synthetic fertilizer manufacture and fossil fuel consumption for mechanization. A drastic reduction of these requires a radical shift toward agro-food systems based on agro-ecology rather than measures that would aim at increasing efficiency without structural change.
The Role of Scenarios for Reality
The present study is based entirely on the biogeochemical analysis of the two contrasted, hypothetical scenarios of the future of the French agro-food system (Billen et al., 2018a). This approach sets both epistemological and political issues that we wish to discuss briefly here.
Unless adopting a completely deterministic view of both human and natural history in which the future could be known just as the past as far as we were able to model the systems studied (as in the famous metaphor of the Devil of Laplace, Bergson, 1905), there must always exist an irreducible part of unpredictability of the future. In environmental sciences, the unpredictability is reinforced by the fact that the future course of events does not only arise from the inevitability of biophysical constraints, but also from the conflict of differing human willing with various interests and a number of desires for the future, the results of which cannot a priori be determined. This doubt regarding the ability to predict drives us to first adopt a reflective stance regarding our scientific position. Environmental science cannot predict the future, nor can it decide for the future. Consequently, it must be acknowledged that designing future scenarios involves an inalienable degree of subjectivity. Our subjectivities are manifest in the choices made to develop one scenario rather than another, e.g., here, extreme scenarios of either opening and specialization or fully agro-ecological. Therefore, one must wonder how such choices can be justified: which intelligible logic is guiding our design of scenarios?
According to Wright (2010), the concept of an “emancipatory social science,” i.e., typically constructing scenarios must be assigned three fundamental tasks: (1) to elaborate a systematic criticism of the world as it is; (2) to imagine viable alternatives; (3) to understand the barriers faced by any project of social transformation but also its possibilities and dilemmas. If the word “social” is replaced by the word “environmental” (emancipatory environmental science), it means that the type of facts we try to understand are related to the interaction between societies (socio-ecological metabolism) and their biophysical environment (embedded regional and national scales). The two contrasted scenarios analyzed here highlight that the pursuit of opening and specialization in agriculture would be an alienation to unsustainable agro-food system, whereas a deep change toward a more self-sufficient, organic and demitarian agro-food system would portend socio-ecological emancipation.
Following the Weberian vision of the relation between science and politics (Weber, 1959), the challenge we addressed here for a future sustainable agro-food system must be separated from policy, but the results produced must be useful. The question is to know to whom and how? Any person or collective concerned by climate change and food sovereignty should be involved in the decision regarding their common future. Consequently, in order to fulfill the third requirement of an “emancipatory” science, further perspectives include a reflection on the possibility of our research to be appropriated as widely as possible.
Therefore, scientists may help advance toward that goal by conceiving transmittable and appropriable knowledge favoring an ascending transition toward more sustainability in agro-food systems and, more generally, in socio-ecological systems (Gonzalez de Molina and Toledo, 2014). A major issue for future research is probably to federate research projects with the aim of bringing together different scientific tools and approaches from various disciplinary backgrounds. Further, a non-prescriptive but also a non-neutral role, accounting for the current balance of forces that we are part of can be the basis for an ecological transition, necessarily driven, among others, by the hot debate on the sustainability of agro-food systems.
Author Contributions
JG and GB contributed to data processing and article redaction. JL designed the paper and contributed to data processing and article redaction.
Conflict of Interest Statement
The authors declare that the research was conducted in the absence of any commercial or financial relationships that could be construed as a potential conflict of interest.
Acknowledgments
This work has been carried out within the scope of the PIREN-Seine research projects (CNRS/AESN). Thanks are also due to the Fédération Ile-de-France de Recherche pour l'Environnement (FIRE) for providing an interdisciplinary framework that was beneficial for this work. We are very grateful to Manuel Martin (INRA, Infosol) for his valuable help and assistance in data collection from the RMQS network (www.gissol.fr/tag/rmqs), which were used to calibrate the inert cropland SOC stock (contract no. 2018-RMQS-UPMC-METIS). Thanks are also due to Mélanie Raimonet for helping collect temperature data at the departmental scale for the 1990–2010 period.
Supplementary Material
The Supplementary Material for this article can be found online at: https://www.frontiersin.org/articles/10.3389/fsufs.2019.00019/full#supplementary-material
References
Agreste. (2017). La Statistique Agricole. Ministère de l'Agriculture, de l'Agro-Alimentaire et de la Forêt. Available online at: http://agreste.agriculture.gouv.fr/la-statistique-agricole/ (accessed November 15, 2018).
Aguilera, E., Guzman, G. I., Álvaro-Fuentes, J., Infante-Amate, J., García-Ruiz, R., Carranza-Gallego, G., et al. (2018). A historical perspective on soil organic carbón in Mediterranean cropland (Spain, 1900-2008). Sci. Tot. Environ. 621, 634–648. doi: 10.1016/j.scitotenv.2017.11.243
Aguilera, E., and Lassaletta, L. (2015). Soil carbon sequestration is a climate stabilization wedge: comments on Sommer and Bossio (2014). J. Environ. Manage. 153, 48–49. doi: 10.1016/j.jenvman.2015.01.038
Anglade, J., Billen, G., Makridis, T., Garnier, J., Puech, T., and Tittel, C. (2015). Nitrogen soil surface balance of organic vs conventional cash crop farming in the Seine watershed. Agric. Syst. 139, 82–92. doi: 10.1016/j.agsy.2015.06.006
Autret, B., Mary, B., Chenu, C., Balabane, M., Girardin, C., Bertrand, M., et al. (2016). Alternative arable cropping systems: a key to increase soil organic carbon storage? Results from a 16 year field experiment. Agric. Ecosyst. Environ. 232, 150–164. doi: 10.1016/j.agee.2016.07.008
Ballabio, C., Panagos, P., and Monatanarella, L. (2016). Mapping topsoil physical properties at European scale using the LUCAS database. Geoderma 261, 110–123. doi: 10.1016/j.geoderma.2015.07.006
Billen, G., Lassaletta, L., and Garnier, J. (2014). A biogeochemical view of the global agro-food system: nitrogen flows associated with protein production, consumption and trade. Glob. Food Secur. 3, 209–219. doi: 10.1016/j.gfs.2014.08.003
Billen, G., Lassaletta, L., and Garnier, J. (2015). A vast range of opportunities for feeding the world in 2050: trade-off between diet, N contamination and international trade. Environ. Res. Lett. 10:025001. doi: 10.1088/1748-9326/10/2/025001
Billen, G., Lassaletta, L., Garnier, J., Le Noë, J, Aguilera, E., and Sanz-Cobena, A. (2018b). “Opening to distant markets or local reconnection of agro-food systems? Environmental consequences at regional and global scales. Chapter 25,” in Agroecosystem Diversity. Reconciling Contemporary Agriculture and Environment Quality, eds G. Lemaire, P. Carvalho, S. Kronberg, and S. Recous (London: Elsevier), 391–412.
Billen, G., Le Noë, J, and Garnier, J. (2018a). Two contrasted future scenarios for the French agro-food system. Sci. Tot. Environ. 637–638, 695–705. doi: 10.1016/j.scitotenv.2018.05.043
Bolinder, M. A., Janzen, H. H., Gregorich, E. G., Angers, D. A., and VandenBygaart, A. J. (2007). An approach for estimating net primary productivity and annual carbon inputs to soil for common agricultural crops in Canada. Agric. Ecosyst. Environ. 118, 29–42. doi: 10.1016/j.agee.2006.05.013
Clivot, H., Mary, B., Valé, M., Cohan, J.-P., Champolivier, L., Praux, F., et al. (2017). Quantifying in situ and modeling net nitrogen mineralization from soil organic matter in arable cropping systems. Soil Biol. Biochem. 111, 44–59. doi: 10.1016/j.soilbio.2017.03.010
Constantin, J., Mary, B., Laurent, F., Aubrion, G., Fontaine, A., Kerveillant, P., et al. (2010). Effects of catch crops, no till and reduced nitrogen fertilization on nitrogen leaching and balance in three lon-term experiments. Agric. Ecosyst. Environ. 135, 268–278. doi: 10.1016/j.agee.2009.10.005
Doublet, S. (2011). CLIMAGRI: Bilan Energies et GES des Territoires Ruraux, la Ferme France en 2006 et 4 Scénarios Pour 2030. Rapport ADEME. Available online at: https://www.ademe.fr/sites/default/files/assets/documents/climagri-la-ferme-france-en-2006-et-4-scenarios-pour-2030.pdf (accessed May, 2018).
Erb, K.-H., Haberl, H., and Krausmann, F. (2007). “The fossil fuel-powered carbon sink. Carbon flows and Austria's energetic metabolism in a long-term perspective,” in Socioecological Transitions and Global Change, eds M. Fischer-Kowalski and H. Haberl (Cheltenham: Edward Elgar), 60–82.
Erb, K. H., Lauk, C., Kastner, T., Mayer, A., Theurl, M. C., and Haberl, H. (2016). Exploring the biophysical option space for feeding the world without deforestation. Nat. Commun. 7:11382. doi: 10.1038/ncomms11382
Garnier, J., Le Noë, J, Marescaux, A., Sanz-Cobena, A., Lassaletta, L., Silvestre, M., et al. (2019). Long term changes in greenhouse gas emissions of French agriculture (1852-2014): from traditional agriculture to conventional intensive systems. Sci. Tot. Environ. 660, 1486–1501. doi: 10.1016/j.scitotenv.2019.01.048
Gingrich, S., Erb, K.-H., Krausmann, F., Gaube, V., and Haberl, H. (2007). Long-term dynamics of terrestrial carbon stocks in Austria: a comprehensive assessment of the time period from 1830 to 2000. Reg. Environ. Change 7, 37–47. doi: 10.1007/s10113-007-0024-6
Gonzalez de Molina, M., and Toledo, V. M. (2014). The Social Metabolism, A Socio-Ecological Theory of Historical Change. Cham; Heidelberg; New York, NY; Dordrecht; London: Springer. ISSN 2211–9027.
IPCC (2018). Global Warming of 1.5°C: an IPCC Special Report on the Impacts of Global Warming of 1.5°C Above Pre-industrial Levels and Related Global Greenhouse Gas Emission Pathways, in the Context of Strengthening the Global Response to the Threat of Climate Change, Sustainable Development, and Efforts to Eradicate Poverty. Available online at: http://www.ipcc.ch/report/sr15/ (accessed November, 2018).
ITAB (2011). Rotations en Grandes Cultures Biologiques Sans Elevage. Réseau Expérimental RotAB. Available online at: http://www.itab.asso.fr/downloads/rotab/rotab-broch-fertilite.pdf (accessed April, 2017).
Jenkinson, D. S., and Rayner, J. F. (1977). The turnover of soil organic matter in some of the Rothamsted classical experiment. Soil Sci. 123, 298–305. doi: 10.1097/00010694-197705000-00005
Justes, E., Beaudoin, N., Bertuzzi, P., Charles, R., Constantin, J., Dürr, C., et al. (2012). Réduire les Fuites de Nitrate au Moyen de Cultures Intermédiaires: Conséquences Sur les Bilans d'eau et D'azote, Autres Services Ecosystémiques, Chapter 10. Rapport d'étude. INRA.
Justes, E., Mary, B., and Nicolardot, B. (2009). Quantifying and modelling C and N mineralization kinetics of catch crop residues in soil: parameterization of the residue decomposition module of STICS model for mature and non mature residues. Plant Soil. 325, 171–185. doi: 10.1007/s11104-009-9966-4
Kastner, T., Rivas, M. J. I., Koch, W., and Nonhebel, S. (2012). Global changes in diets and the consequences for land requirements for food. Proc. Natl. Acad. Sci. U.S.A. 109, 6868–6872. doi: 10.1073/pnas.1117054109
Kätterer, T., Reichstein, M., Andrén, O., and Lomander, A. (1998). Temperature dependence of organic matter decomposition: a critical review using literature data analyzed with different models. Biol. Fertil. Soils. 27, 258–262. doi: 10.1007/s003740050430
Kong, A. Y. Y., Six, J., Bryant, D. C., Ford Denison, R., and van Kessel, C. (2005). The relationship between carbon input, aggregation, and soil organic carbon stabilization in sustainable cropping systems. Soil Sci. Soc. Am. J. 69, 1078–1085. doi: 10.2136/sssaj2004.0215
Lal, R. (2016). Beyond COP21: potential and challenges of the “4 per Thousand” initiative. J. Soil Water Conserv. 71:1. doi: 10.2489/jswc.71.1.20A
Lassaletta, L., Billen, G., Garnier, J., Bouwman, L., Velazquez, E., Mueller, N. D., et al. (2016). Nitrogen use in the global food system: historical trends and future trajectories of agronomic performance, pollution, trade, and dietary demand. Environ. Res. Lett. 11:095007. doi: 10.1088/1748-9326/11/9/095007
Le Noë, J., Billen, G., Esculier, F., and Garnier, J. (2018). Long-term socioecological trajectories of agro-food systems revealed by N and P flows in French regions from 1852 to 2014. Agric. Ecosyst. Environ. 265, 132–143. doi: 10.1016/j.agee.2018.06.006
Le Noë, J., Billen, G., and Garnier, J. (2017). How the structure of agro-food systems shapes nitrogen, phosphorus, and carbon fluxes: the Generalized Representation of Agro-Food System applied at the regional scale in France. Sci. Tot. Environ. 586, 42–55. doi: 10.1016/j.scitotenv.2017.02.040
Le Noë, J., Billen, G., Lassaletta, L., Silvestre, M., and Garnier, J. (2016). La place du transport de denrées agricoles dans le cycle biogéochimique de l'azote en France: un aspect de la spécialisation des territoires. Cahiers Agric. 25:15004. doi: 10.1051/cagri/2016002
Le Noë, J., Billen, G., Mary, B., and Garnier, J. (2019). Drivers of long-term carbon dynamics in cropland: a bio-political history (France, 1852-2014). Environ. Sci. Pollut. 93, 53–65. doi: 10.1016/j.envsci.2018.12.027
Milesi Delaye, L. A., Irizar, A. B., Andriulo, A. E., and Mary, B. (2013). Effects of continuous agriculture of grassland soils of the rolling pampa on soil organic carbon and nitrogen. Appl. Environ. Soil Sci. 2013:487865. doi: 10.1155/2013/487865
Moisselin, J. M., Schneider, M., Canellas, C., and Mestre, O. (2002). Les changements climatiques en France au XXe siècle. Étude des longues séries homogénéisées de données de température et de précipitations. La Météorol. 38, 45–56. doi: 10.4267/2042/36233
Muller, A., Schader, C., El-Hage Scialabba, N., Brüggemann, J., Isensee, A., Erb, K.-H., et al. (2017). Strategies for feeding the world more sustainably with organic agriculture. Nat. Commun. 8:1290. doi: 10.1038/s41467-017-01410-w
Poulton, P., Johnston, J., Macdonald, A., White, R., and Powlson, D. (2018). Major limitations to achieving “4 per 1000” increases in soil organic carbon stock in temperate regions: evidence from long-term experiments at Rothamsted Research, United Kingdom. Glob. Change Biol. 24:2563–2584. doi: 10.1111/gcb.14066
Raimonet, M., Oudin, L., Thieu, V., Silvestre, M., Vautard, R., Rabouille, C., et al. (2017). Evaluation of gridded meteorological datasets for hydrological modeling. Am. Meteorol. Soc. 18, 3027–3041. doi: 10.1175/JHM-D-17-0018.1
Saffih-Hdadi, K., and Mary, B. (2008). Modeling consequences of straw residues export on soil organic carbon. Soil Biol. Biochem. 40, 594–607. doi: 10.1016/j.soilbio.2007.08.022
Stockmann, U., Adams, M. A., Crawford, J. W., Field, D. J., Henakaarchchi, N., Jenkins, M., et al. (2013). The knowns, known unknowns and unknowns of sequestration of soil organic carbon. Agric. Ecosyst. Environ. 164, 80–99. doi: 10.1016/j.agee.2012.10.001
Weber, M. (1959). Le Savant et le Politique. Paris: Trad. Julien Freund. Plon ed. Available online at: http://classiques.uqac.ca/classiques/Weber/savant_politique/Le_savant_et_le_politique.pdf (accessed June, 2018).
Keywords: prospective scenarios, French agro-food systems, soil organic carbon, energy consumption, carbon emissions budget
Citation: Le Noë J, Billen G and Garnier J (2019) Carbon Dioxide Emission and Soil Sequestration for the French Agro-Food System: Present and Prospective Scenarios. Front. Sustain. Food Syst. 3:19. doi: 10.3389/fsufs.2019.00019
Received: 26 November 2018; Accepted: 13 March 2019;
Published: 04 April 2019.
Edited by:
Ngonidzashe Chirinda, International Center for Tropical Agriculture (CIAT), ColombiaReviewed by:
Justice Nyamangara, Marondera University of Agricultural Sciences and Technology (MUAST), ZimbabweJessica Davies, Lancaster Environment Centre, Lancaster University, United Kingdom
Copyright © 2019 Le Noë, Billen and Garnier. This is an open-access article distributed under the terms of the Creative Commons Attribution License (CC BY). The use, distribution or reproduction in other forums is permitted, provided the original author(s) and the copyright owner(s) are credited and that the original publication in this journal is cited, in accordance with accepted academic practice. No use, distribution or reproduction is permitted which does not comply with these terms.
*Correspondence: Julia Le Noë, anVsaWEubGVub2VAYm9rdS5hYy5hdA==