- Crop Improvement and Protection Unit, United States Department of Agriculture, Agricultural Research Service, Salinas, CA, United States
Agriculture in many regions of the world has reduced bird habitat and abundance, and altered avian community structure. A study was conducted on an organic research farm over two winters (Oct to Mar) in an intensive agricultural region of Salinas Valley, CA to determine how cover crop variety and planting density influenced birds. Cover crops were rye (Secale cereale), a mixture of rye and legumes (Vicia spp., Pisum sativum), and a mustard mixture (Brassica juncea, Sinapis alba). White-crowned Sparrows (Zonotrichia leucophyrs), Savannah Sparrows (Passerculus sandwichensis) and Song Sparrows (Melospiza melodia) were observed both years in the study field. Bird droppings in cover crops were quantified and dissected to determine dietary preferences, and sparrow movement when flushed was determined. Dropping number and weight per m2 were at least 10 times greater in mustard than in rye and in the legume-rye mixture. Droppings were dominated by leaf tissue in mustard vs. arthropod tissue in rye and legume-rye. Within cover crop variety, plant density did not have a clear or consistent effect on sparrows. Sparrows flushed from cover crops usually settled in mustard. The White-crowned Sparrow fed on mustard leaves and apparently on weed foliage under mustard. The arthropod-dominated droppings in rye and legume-rye cover crops were consistent with the food preferences of Song and Savannah Sparrows. The White-crowned Sparrow's clear preference for mustard cover crops is likely due in part to their high dietary needs for sulfur-rich amino acids during the prenuptual molt. This paper provides novel information to help farmers and others understand the cover crop preferences of sparrows, and ways that farmers might use mustard cover crops as trap crops to reduce White-crowned Sparrow feeding damage on winter and spring vegetable crops. It also provides evidence of ecosystems services that these sparrows provide by feeding on weed tissue in winter cover crops.
Introduction
Agricultural intensification during the past 70 years has increased world food supplies several fold through the use of synthetic chemicals, new technologies, and improved crop varieties, but has also dramatically altered the earth's ecosystems (Matson et al., 1997; Tilman et al., 2002). During this period, farmland bird community structure has been altered and populations have declined in many regions of the world (Chamberlain et al., 2000; Shutler et al., 2000; Donald et al., 2001; Murphy, 2003; Brennan and Kuvlesky, 2005; Butler et al., 2007; Rosenberg et al., 2019). These reductions are due to habitat loss (Green et al., 2005) and are associated with a reduction in insect and arable weed populations that are a food resource for higher trophic groups including birds (Benton et al., 2002; Storkey and Westbury, 2007). Bird population dynamics in some of the most intensive agricultural regions in the United States such as in Salinas valley in Monterey county, California, have not been well-documented but have likely declined as the agricultural landscape transitioned from rainfed dairy and agronomic crops (1890–1920) to irrigated sugar beets (1920–1930), followed by the current systems that focus on lettuce and other high value vegetables and strawberries. The intensity and economic importance of agriculture in this region are evident in the U.S. $ 4.3 billion production value derived mostly from vegetable production in 2018 (Monterey County Agricultural Commissioner, 2018); organic agriculture in Monterey county comprises ~10% of this production value and was grown by 185 producers in 2018.
Farm land lease prices in this region typically range from U.S. $3,000 to <6,000 ha−1 year−1. The presence of wildlife in these intensive systems and important conservation practices (i.e., cover cropping and hedgerows) has become increasingly suspect and scrutinized due to food safety issues with leafy greens (Beretti and Stuart, 2008), although the causes of fatal Escherichia coli 0157:H outbreaks are still poorly understood. Interestingly, there is evidence that pathogen prevalence increased on farms in this region where non-crop vegetation, that may provide habitat for birds, had been removed (Karp et al., 2015). Furthermore, recent research on strawberry systems in this region highlighted the benefits of maintaining seminatural habitat around farms to help reduce bird damage on strawberries (without increasing food safety risks) and also to increase bird diversity (Olimpi et al., 2020).
The least intensive vegetable production period in agricultural fields in Salinas occurs during the rainy winter period (Oct to Mar) when most conventional fields are maintained weed free with tillage and herbicides. These bare fallow fields and the lack of hedgerows and non-crop vegetation on most farms in this region provide poor habitat for birds and other wildlife. However, winter cover crops and native plant hedgerows (Brennan, 2015; Earnshaw, 2018) that typically occur more often on organic farms in the region may provide farmland birds with valuable habitat. Quantitative information on the prevalence of cover crops and hedgerows on farms in the Salinas Valley is not available, however, annual cover crops are a more common form of non-crop vegetation than are hedgerows.
Winter cover crops in this region can reduce nitrate leaching (Jackson et al., 1993; Wyland et al., 1996), suppress weeds (Brennan and Smith, 2005), and add organic matter to the soil (White et al., 2020). Common winter cover crops of cereals, legume-cereal mixtures, or mustards are incorporated into the soil in the spring prior to vegetable planting. The effects of winter cover crops on birds in these systems have not been studied, but such information could help farmers develop management practices that enhance on-farm biodiversity of birds and other beneficial wildlife and thus increase ecosystem services. The objective of this study was to determine how cover crop variety and planting density influence sparrows.
Study Area
The study occurred in a commercial scale, organic vegetable research field at the United States Department of Agriculture, Agriculture Research Service in Salinas, CA during the winter cover cropping period from Oct to Mar over 2 years. The 0.9 ha-experimental field where the study occurred was within 10 ha of certified organic research land (Figure 1). The soil series at the site is a Chualar loamy sand. The field where the study occurred contains an ongoing, long-term trial on organic vegetable production systems that has been described in several previous papers (Brennan and Boyd, 2012; Brennan, 2013; Maltais-Landry et al., 2016; White et al., 2020). This site included a hedgerow of native plants on the south-west and south-east including Baccharis pilularis, Sequoiadendron giganteum, Rhamnus californica, Atriplex lentiformis, Rhus ovata, Lavatera sp., Eriogonum sp., Heteromeles arbutifolia, Salvia sp., Ceanothus sp. Fremontodendron californicum, Quercus agrifolia, and Sambucus mexicana. The layout of the organic farm and hedgerows relative to the surrounding conventionally managed land is further illustrated in this video (Brennan, 2015). The inclusion of annual cover crops at the research farm every winter and hedgerows makes this site a model of best management practices for an organic farm in the Salinas Valley; however, relatively few farms in the Valley include hedgerows or cover crops every winter.
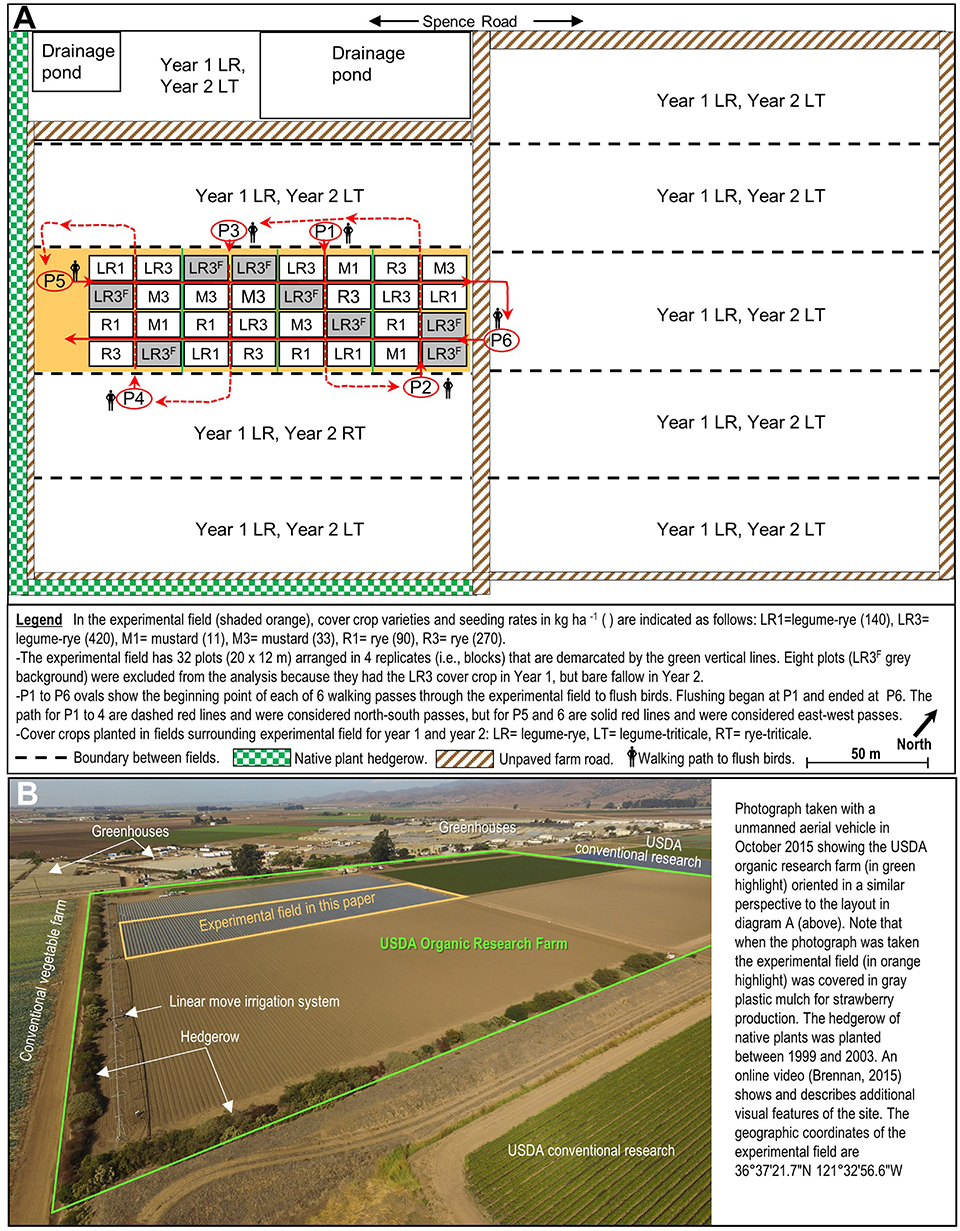
Figure 1. Layout of the agricultural fields (A) at the 10 ha USDA organic research farm along Spence Road in Salinas, California including the 0.9 ha field (shaded orange) where the effect of cover crops on sparrows was studied during two winter periods. The photograph (B) provides an aerial view of the organic research farm (in green highlight) and surrounding area.
Materials and Methods
The residue from the previous vegetable crop (broccoli) was incorporated into the soil with a disc or spader prior to planting winter cover crop treatments. Cover crops were planted with a grain drill with 15-cm spacing between rows at seeding rates that resulted in a range of plant densities (Table 1). Sprinkler irrigation was used after planting as necessary to stimulate cover crop germination before the onset of winter rainfall. Cover crop treatment plots were 20 m long by 12 m wide and arranged in a randomized complete block design with four replications (Figure 1A). Planting dates were 2 Nov 2006 and 15 Oct, 2007. Due to a problem with mustard emergence in Year 2, parts of the mustard plots were reseeded with a hand-push planter on 29 and 30 Oct, 2007. The plot layout was slightly different between years because during Year 1 all eight plots in each replicate were cover cropped, whereas in Year 2 two of the plots in each replicate were bare fallowed all winter.
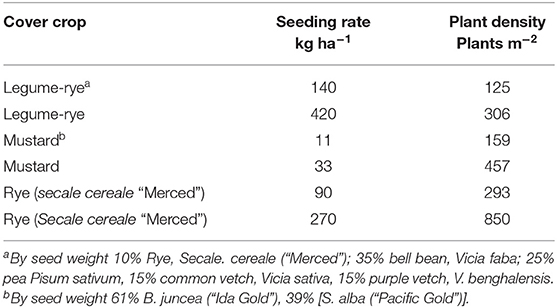
Table 1. Cover crop seeding rates and resulting mean cover crop densities during two years in Salinas CA, USA.
Bird Dropping Collection and Processing
Bird droppings were collected from the soil surface under the cover crop canopies (Figure 2A) and from the vegetation directly above a 45 × 100 cm quadrat (Year 1) and 50 × 100 cm quadrat (Year 2) in a 2 m−2 area in each plot in Year 1 (24 Feb, 2007) and Year 2 (13–15 Feb, 2008). The droppings were counted as they were collected and were then oven-dried at 65 C for at least 48 h. The droppings were then examined at 6–10× magnification under a dissecting microscope and attached particles of gravel or other debris were removed manually before weighing. The weight of five randomly chosen fully intact droppings from each plot were used to estimate the number of intact droppings in collected samples; this was necessary because a small portion of the droppings had broken during drying and cleaning. Fully intact droppings were obvious because they had tapered ends. Rodent droppings seldom occurred in the plots and were distinguishable from bird droppings by their appearance and composition; compared with bird droppings, rodent droppings were more tapered on the ends, were dominated by small fragments of monocot vegetative tissue, and tended to be denser than bird droppings. To minimize the potential effect of adjacent plots, the quadrats where bird droppings were collected were randomly chosen from an area at least 1 m or more away from the edge of each plot. A 0.5–1 mm piece from one randomly chosen bird dropping from each plot from each collection date was placed on a microscope slide, dissolved in a few drops of water, and was examined under a compound microscope at 25–100× for the presence of leaf, seed, and arthropod fragments. To understand bird diets, I compared unique anatomical features (i.e., leaf and fruit surface trichomes, stomatal characteristics, seed surface characteristics, and leaf margin spines) of plant fragments in the bird feces with those of cover crops and weeds from the field; this approach is often used to study animal diets (Baumgartner and Martin, 1939; Martin, 1955; Stewart, 1967; Vaughan, 1967; Jennings and Barkham, 1975; Stevens et al., 1987; Yamamoto-Ebina et al., 2016; Takatsuki and Morinaga, 2020). Signs of folivory on cover crops and weeds were noted when droppings were collected. Bird feeding on large leaves results in torn or relatively straight edged gaps in leaf tissue (Figure 2B), compared with that of insect herbivores that removed smaller, rounded areas of leaf tissue.
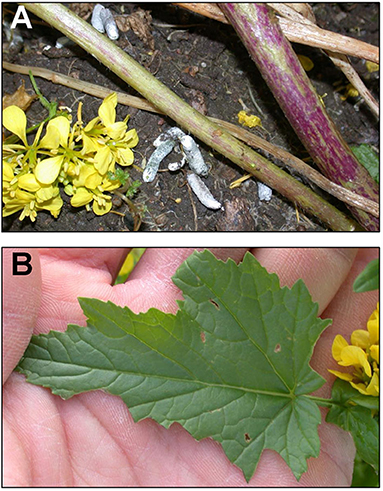
Figure 2. Soil surface under a mustard cover crop canopy (A) with foliage-rich sparrow droppings. Mustard (Sinapis alba) leaf (B) that has been fed on by a sparrow, presumable the White-crowned sparrow. Note the edge toward the top of the photo is mostly intact, whereas the edge toward the bottom has jagged edges indicating bird feeding.
Sparrow Movement Between Cover Crops
Sparrow behavior when flushed was determined between 10:45 a.m. and 14:00 p.m. on 3 dates in Year 1 (Feb 25, 26, Mar 8) and Year 2 (Feb 13, 15, 17). On each date, sparrow counts were made as I walked through the field in six consecutive passes including one northwest/southeast pass through the center of each of the four replicates, and two southwest/northeast passes across the four replicates at each date; this walking pattern ensured that I walked evenly between all eight plots in each replicate three times. The starting point and path for each pass through the field is illustrated in Figure 1. The walking speed was ~25 m min−1 and the six passes made on each date were completed in ~15 min. As I walked through the field I recorded the number of sparrows flushed from each plot, and the plot where the flushed sparrows resettled. The data were pooled over the three observation days and both walking directions to obtain the number of birds flushed from each plot, and the number of birds that entered each plot each year. The sparrow presence and movement data were not differentiated by species and thus potentially reflect the behavior of the three sparrow species observed in the cover crop including the White-crowned sparrow (Zonotrichia leucophyrs), the Savannah sparrow (Passerculus sandwichensis), and the Song sparrow (Melospiza melodia). Four subspecies of Z. leucophrys occur in California including the non-migratory nuttalli, the short distance migrant pugetensis, the medium distance migrant oriantha, and the long distance migrant gambelii (Lisovski et al., 2019; Chilton et al., 2020). The subspecies of Z. leucophyrs was not determined but was likely pugetensis or gambelii, rather than nuttalli which typically occurs in a narrow band near the ocean, and oriantha that occurs at higher elevations (Ramenofsky and Wingfield, 2006; Chilton et al., 2020).
The cover crops had not produced viable seed during the data collection periods each year, however, the taller S. alba mustard component was in full bloom, and the rye and legumes were in the early to mid stages of anthesis. During the data collection periods the legume-rye mix, rye and mustard (S. alba) were ~1.5–2 m tall.
Statistical Analyses
The data analysis was focused on the inference by eye method (Cumming and Finch, 2005) to visualize the general patterns in the raw data along with point and interval estimates that were used to make statistical inferences and determine the practical significance of the results. The point estimates used were means and mean paired differences (i.e., effect sizes) and their 95% confidence intervals (CI) (Cumming and Finch, 2005; Cumming and Fidler, 2009). CIs are reported in square brackets [] in the text and figure captions, and were calculated in SAS (ver. 9.4, SAS Institute, Cary, NC) and the Explanatory Software for Confidence Intervals (ESCI) (Cumming and Calin-Jageman, 2017). This analysis approach was used due to valid and ongoing criticisms of null-hypothesis significance testing (Anderson et al., 2000; Fidler et al., 2006; Nakagawa and Cuthill, 2007; Hubbard and Lindsay, 2008; Lambdin, 2012; Campbell et al., 2015; Smith, 2018, 2020) that can lead to dichotomous thinking and misinterpretation of results. Furthermore, when presenting and discussing the results I followed the recommendation of several authors (Sterne and Smith, 2001; Higgs, 2013; Smith, 2020) and avoided using “significant” or “statistically significant.” The CIs in the figures were made in the shape of cat's eyes to emphasize that the population mean (μ) is several times more plausible near the center or sample mean of the CI than at the upper or lower limit of the interval (Cumming, 2007, 2014). In other words, values at the fattest (i.e., horizontally widest) part of the CI are the most plausible values of the population mean. Supplementary Figures 1, 2 provide additional detailed information for readers that are not familiar with the relationship between p-values and the rule of eye method for making statistical inferences. Paired differences for comparisons between cover crops were calculated as the difference within a replicate. For example, if the average number of bird droppings per m2 in replicate one was ten droppings in the mustard plot and one in the legume-rye plot, then their paired difference for the mustard vs. legume-comparison in that replicate would be nine droppings m2 because 10–1 = 9. With this approach, if the CI of paired difference does not include zero P < 0.05 (i.e., a comparison-wise error rate of <5%), and P = 0.05 if one of the limits is just at zero. The relationship between P-values and CIs is mentioned as a point of reference that readers may be more familiar with, but I discourage readers from using CIs in a rigid or dichotomous way; for example, by concluding that a CI of a paired difference that includes zero indicates no difference. Rather, I suggest that readers consider: (1) the patterns in the raw data, (2) the direction and size of the mean effect and width of the CI, and (3) visualize CIs in the shape of a “cat's eye” as describe above. The response variables analyzed were the number of bird droppings per m2, and the oven-dry weight of bird droppings per m2. Years were analyzed separately due to differences between years in the presence of fallow plots. The analysis was focused primarily on the results averaged across seeding rates within each cover crop type due to the lack of evidence of a clear or consistent cover crop seeding rate effect (i.e., plant density effect) on the number or weight of bird droppings. For the analysis of the bird movement data, means and CIs are presented, however, it is important to highlight that some individual birds were undoubtedly counted more than once on each date as they flew between plots. Thus, in contrast to the bird dropping data, the bird flushing data does not meet the assumption of independence, and thus is less robust than the bird dropping data.
Results
Bird Dropping Number and Weight
The number of bird droppings in the cover crops ranged from 0 to 190 per m2 and were on average usually <10 per m2 in rye and the legume-rye mixture, but generally were much more numerous in the mustard (Figures 3A,B). Cover crop seeding rate affected the population density of the cover crops (Table 1) but did not have clear or consistent effects on the number of bird droppings in the cover crops. For example, in mustard, the number of droppings was higher on average at the 1× seeding rate in Year 1 (Figure 3A), but higher in the 3× rate in Year 2 (Figure 3B), primarily due to a single but different replicate each year that had more than 150 droppings per m2. Averaged across seeding rates and years, the number of dropping per m2 was at least ten times greater in mustard than in legume-rye or rye (Figures 4A,B). While the CIs of the paired differences in the number of droppings between the different cover crop types included 0, the scatter of the raw data and the distance of the most plausible parts of the CI from zero provides consistent evidence of more droppings in mustard than in rye or legume-rye cover crops (Figures 4C,D, Supplementary Figures 1, 2). In contrast, the paired comparison of the number of dropping in rye vs. legume-rye do not provide clear or consistent evidence of a difference between these cover crops. For example, during Year 1 the legume-rye cover crop had slightly more droppings than rye, but during Year 2 the opposite pattern occurred. The weight of droppings in the three cover crops averaged across seeding rates followed the same general patterns as occurred with the number of droppings (Figures 3C,D; Figure 5).
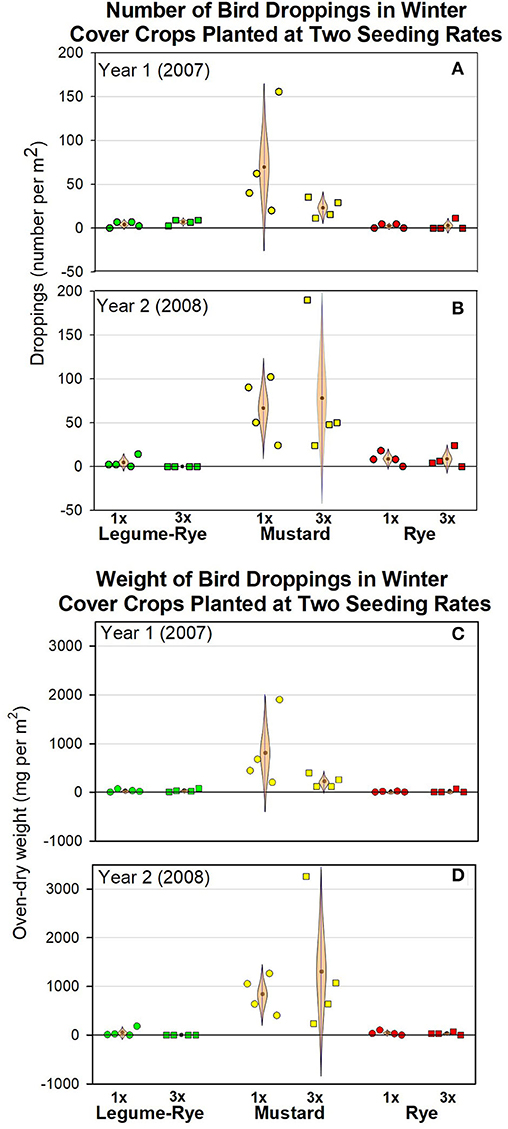
Figure 3. The number (A,B) and weight (C,D) of bird droppings under three winter cover crops planted at two seeding rates during two winters in Salinas, California. The data were collected in Feb 24 (Year 1) and Feb 13 to 15 (Year 2) that was ~16–17 weeks after planting. The standard (1x) and high (3x) seeding rates in kg per ha were 140 and 420 (legume-rye), 11 and 33 (mustard), and 90 and 270 (rye). The light brown, cat's eye shaped error bars are 95% confidence intervals (CIs) with the sample mean at the central point on the bar. The CIs are shown in this shape to help illustrate how plausibility varies along the CI with values at the fattest (i.e., widest horizontal) area near the mean being several times more plausible to be the population mean (μ) than values at the ends of the CI. Data points are clustered around the mean in order of replicates 1–4. The data in this figure are available in the Supplementary Materials.
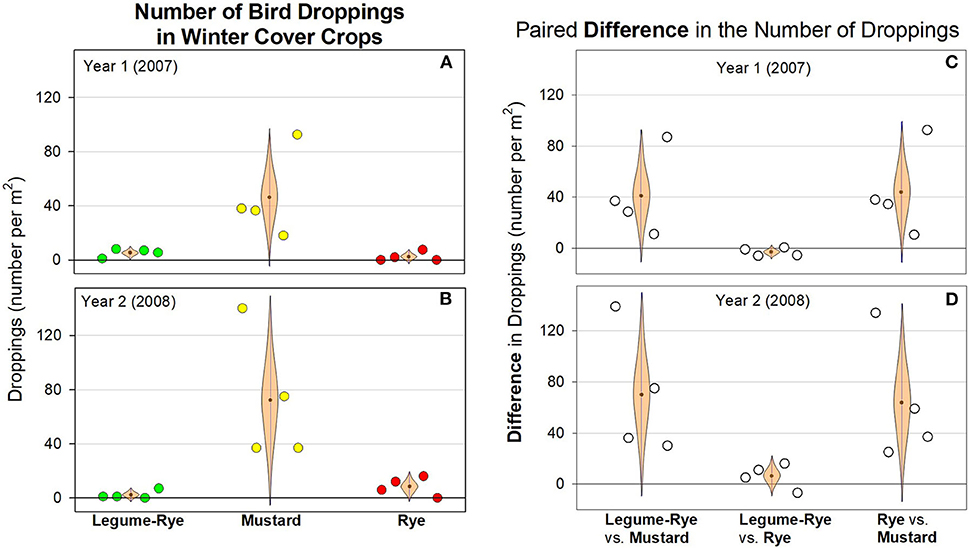
Figure 4. The number (A,B) of bird droppings under three winter cover crops averaged, and their paired differences (C,D), averaged across two seeding rates during two winters in Salinas, California. The data were collected in Feb 24 (Year 1) and Feb 13 to 15 (Year 2) that was ~16–17 weeks after planting. The light brown, cat's eye shaped error bars are 95% confidence intervals (CIs) with the sample mean at the central point on the bar. The CIs are shown in this shape to help illustrate how plausibility varies along the CI with the fattest (i.e., widest horizontal) areas near the mean being several times more plausible to be the population mean (μ) than the ends of the CI. Data points are clustered around the mean in order of replicates 1–4. The mean number of droppings per m2 and the 95% CI in [ ] are 5 [0, 10] and 2 [−3, 7] in legume-rye, 46 [−5, 97] and 72 [−5, 150] in mustard, 2 [−3, 8] and 9 [−3, 20] in rye for Year 1 and Year 2, respectively. The mean difference in the number of droppings per m2 for paired comparisons were 41 [−11, 93] and 70 [−10, 150] for legume-rye vs. mustard, −3 [−8, 2] and 6 [−10, 22] for legume-rye vs. rye, and 44 [−11, 99] and 64 [−14, 142] for rye vs. mustard, in Year 1 and Year 2, respectively. See Supplementary Figures 1, 2 for additional details on the analysis.
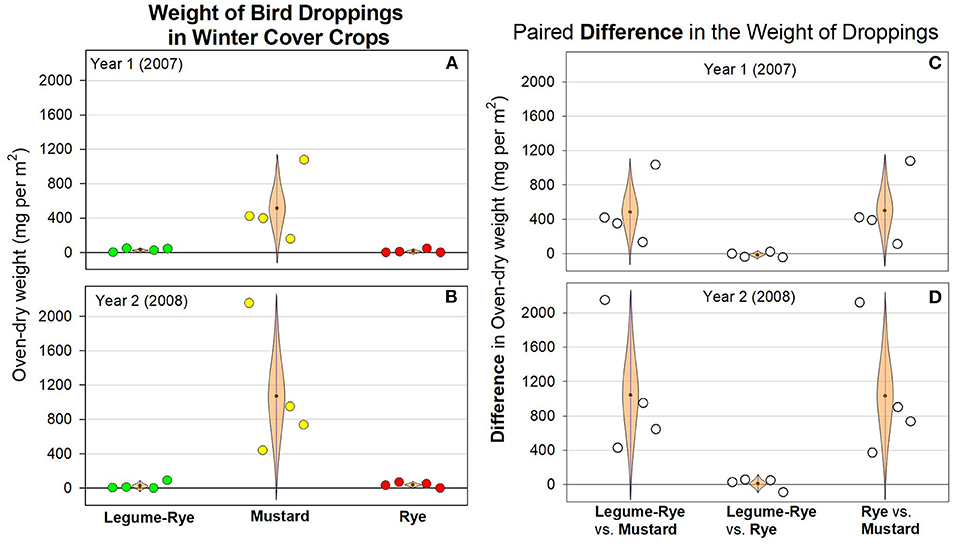
Figure 5. The weight (A,B) of bird droppings under three winter cover crops, and their paired differences (C,D), averaged across two seeding rates during two winters in Salinas, California. The data were collected in Feb 24 (Year 1) and Feb 13 to 15 (Year 2) that was ~16–17 weeks after planting. The light brown, cat's eye shaped error bars are 95% confidence intervals (CIs) with the sample mean at the central point on the bar. The CIs are shown in this shape to help illustrate how plausibility varies along the CI with values at the fattest (i.e., widest horizontal) area near the mean being several times more plausible to be the population mean (μ) than values at the ends of the CI. Data points are clustered around the mean in order of replicates 1–4. The mean weight of droppings in mg per m2 was 29 [−3, 62] and 27 [−41, 94] in legume-rye, 514 [−114, 1,141] and 1,071 [−127, 2,268] in mustard, 13 [−21, 48] and 38 [−8, 83] in rye for Year 1 and Year 2, respectively. The mean difference in the weight of droppings in mg per m2 for paired comparisons were 484 [−130, 1,099] and 1,044 [−178, 2,266] for legume-rye vs. mustard, −16 [−65, 33] and 11 [−98, 120] for legume-rye vs. rye, and 500 [−151, 1,151] and 1,033 [−175, 2,241] for rye vs. mustard, in Year 1 and Year 2, respectively.
Bird Dropping Composition
There were large differences in the composition of droppings in the three cover crop varieties (Table 2). For example, in mustard all sampled droppings from 16 plots had leaf tissue and most (i.e., 14 of 16) also had arthropod tissue. In contrast all sampled droppings in rye and legume-rye cover crops had arthropod tissue but they seldom had leaf tissue. Most leaf tissue was from dicotyledon plants that was most likely from the mustard cover crops or weeds. Leaf tissue of weeds that was observed in the droppings included, Sonchus oleraceus (based on leaf marginal spines), Capsella bursa-pastoris (based on the presence of star shaped epidermal trichomes) and Stellaria media (based on multicellular trichomes on the immature fruit and the peduncle) (Figure 6).
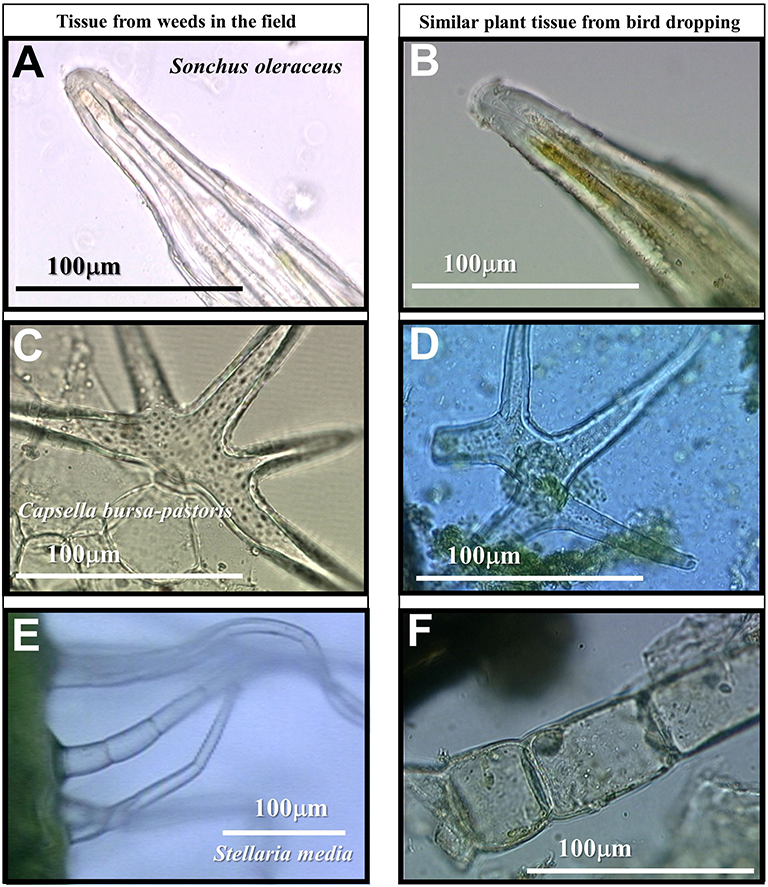
Figure 6. Tissue with trichomes of three weed species in the cover crops. All species had signs of bird folivory. The photographs in the left column (A,C,E) were collected from intact plants while those in the right column (B,D,F) were from bird droppings under the cover crops and had similar morphological characteristics to the weed samples in the left column. Note that the scale is the same for all photographs except for (E). Photograph (A) is a leaf edge trichome, (C) is a stellate (star shaped) leaf trichome, and (E) shows multicellular trichomes on the peduncle of immature fruit.
Weed seed and fruit tissue were less common than leaf and arthropod tissue, and was also more common in droppings in Year 2 than Year 1. For example, in Year 2, weed seed and fruit tissue was present in all sampled droppings in rye and legume-rye, but only occurred in 38% of the sampled droppings in the mustard plots.
Averaged across years, 88% of the sampled droppings in mustard were dominated by leaf tissue, whereas in rye 78% were dominated by arthropod tissue as also occurred in 70% of the sampled droppings under legume-rye. Weed seed and fruit tissue were also present in the bird droppings but were far less common than leaf and arthropod tissue. Across both years, Chickweed (S. media) seed and fruit tissue was the most common weed species found in the droppings followed by Burning Nettle (Urtica urens) and Annual Blue Grass (Poa annua).
Folivory
During the experimental period, the White-crowned Sparrow was observed feeding on leaves of Sinapsis alba mustard as they perched on top of the plant. In addition, leaves of several weeds in the understory of mustard had signs of bird folivory including C. bursa-pastoris, S. media, S. oleraceus, and P. annua (Figure 7). In contrast, weeds in the understory of the rye and the legume-rye cover crops lacked signs of bird folivory.
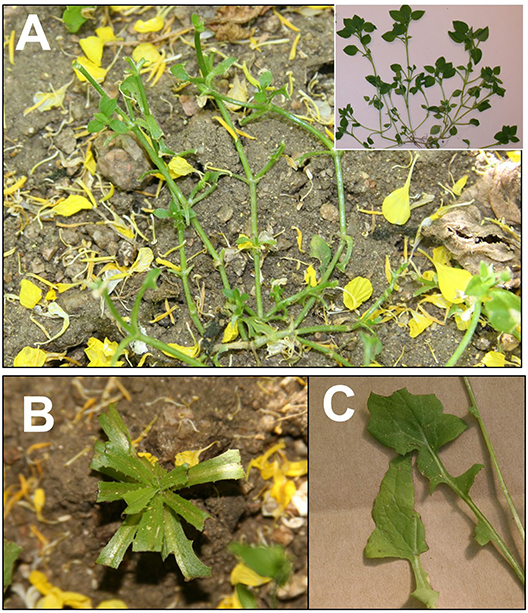
Figure 7. Evidence of weed folivory by sparrows in the understory of a mustard (S. alba and Brassica juncea) cover crop. (A) Common Chickweed (Stellaria media) with most leaves removed; the insert in the top right shows a plant that has not been defoliated. (B) Defoliated rosette of Annual Sowthistle (S. oleraceus). (C) Leaves of S. oleraceus with large areas with removed edges indicating bird feeding.
Sparrow Movement
Cover crop variety affected sparrow movement between cover crop plots when flushed (Table 3). Sparrows were seldom observed in the legume-rye or rye cover crops compared with the mustard plots where they occurred often. For example, during Year 2 an average of 30 sparrows were flushed from mustard plots compared to six that were flushed from legume-rye and rye plots. Flushed sparrows usually settled in mustard plots. In addition, sparrows that were flushed from the hedgerow on the edge of the field often relocated to mustard cover crop plots although this was not quantified. Cover crop density as affected by seeding rate had no apparent effect on sparrow movement (data not shown).
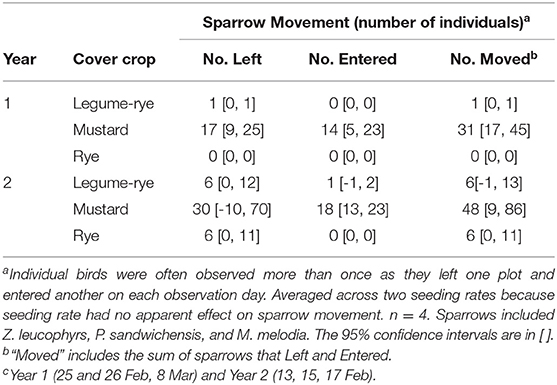
Table 3. Sparrow movement between cover crop plots at 1,045–1,400 h in Salinas, CA USA on 3 days each year at the end (Feb and Mar) of the cover cropping period.
Discussion
Using Bird Droppings to Study Habitat Preferences
Bird droppings have been used as a reliable and indirect measurement to estimate bird habitat preferences and population densities (Evans et al., 2007). As in Odderskaer et al. (1997), I assumed that dropping density was proportional to bird foraging time in a habitat. The dropping data suggest that bird species that fed primarily on leaves, arthropods, and weed seed/fruit tissue preferred the mustard habitat, whereas those that specialized on arthropods and weed seed/fruit preferred rye and the legume-rye cover crops. The White-crowned Sparrow was the only species observed in the study site that is known to feed on leaves and buds (Chilton et al., 2020) whereas the White-crowned, Song, and Savannah Sparrows all eat seeds and arthropods (Arcese et al., 2020; Chilton et al., 2020; Wheelwright and Rising, 2020). Thus, the leaf tissue-dominated droppings in the mustard cover crops most likely reflect the preference of the White-crowned Sparrow for mustard cover crops, whereas the arthropod-dominated droppings that only occurred in the legume-rye and rye plots were most likely produced by the Song or Savannah Sparrows.
What Factors Affect Sparrow Preferences for Cover Crops?
Cover Crop Canopy Structure
Crop structure is known to affect bird foraging efficiency, risk of predation, nesting, and exposure to weather (Wilson et al., 2005). There is little information on how crop structure affects foliage feeding birds like the White-crowned sparrow, but studies with invertebrate feeding birds found a positive relationship with bare ground and a negative relationship with sward height (Perkins et al., 2000; Atkinson et al., 2004; Butler and Gillings, 2004; McCracken and Tallowin, 2004; Whittingham and Evans, 2004; Wilson et al., 2005; Devereux et al., 2006). All three sparrow species observed in the present study are known to forage on the ground near cover (Pullman and Mills, 1977; Arcese et al., 2020; Chilton et al., 2020; Wheelwright and Rising, 2020). Compared with the other cover crops, mustard had a much more open understory that provided a relatively obstacle-free foraging habitat and greater visibility along the soil surface. This open structure may facilitate foraging by the White-crowned Sparrow under mustard and explain why herbivory by birds on weeds was only apparent under mustard. The understory of the legume-rye mixture was especially dense and tangled by pea and vetch vines that climbed between the cereals and bell beans. A dense and tangled canopy structure would likely restrict the movement of ground feeding birds and increase their vulnerability to predators. Potential predators of sparrows at this site included raptors and feral house cats. The occurrence of arthropod-dominated droppings only in the rye and legume-rye cover crops indicates that birds that specialize on arthropods did forage in these less open understories. This pattern may be due to differences in the abundance and type of arthropods in the various cover crops. The results from this study showed that within three distinctly different crop types and canopy structures, plant density did not consistently affect birds. Devereux et al. (2006) similarly found that sward density of ryegrass (Lolium perenne) did not affect foraging behavior or ability of an insectivorous bird. The rigid stems of S. alba mustard appeared to provide better perching sites for sparrows than those of rye or the legume-rye mix and may explain why all three sparrow species settled most often in the mustard plots when flushed. However, the absence of insect-dominated droppings in the mustard plots indicates that insect feeders such as the Song and Savannah Sparrows spent relatively little time in the mustard cover crops.
Food Availability
The apparent preference of the White-crowned Sparrow for mustard cover crops is undoubtedly due in part to its ability to use mustard leaves for food, but may also be influenced by its ability to forage on weed tissue (foliage, fruit, and seed) and insects in the more open understory of mustard (discussed below). In the highly tilled systems in this region, the availability of weed seed for birds is extremely low in the fall and winter until the weeds that germinated with fall-planted cover crops shed seed. Most fallow winter fields in this region are maintained relatively weed-free with herbicides or mechanical weed control to prevent weed seed production that would likely increase hand weeding cost in subsequent vegetable crops. However, weed seed production under winter cover crops in this region can be extremely high in some situations, ranging from several hundred to thousands of seed produced per m2 (Brennan and Smith, 2005; Boyd and Brennan, 2006). This is the primary reason that high seeding rates have been recommended for cover crops such as legume-cereal mixtures (Brennan et al., 2009).
The value of arable weed seed as a food source for birds during the winter is well-documented (Moorcroft et al., 2002; Marshall et al., 2003; Cederbaum et al., 2004; Gibbons et al., 2006; Chamberlain et al., 2007). However, the role of foliage as a food source has received little attention. The composition of the droppings from the mustard cover crop indicate that dicotyledon leaves were an important food source of the White-crowned Sparrows during the winter. Leaves of cereals are important in Skylark diets in arable fields when crop or weed seed were unavailable (Green, 1978), however, avian folivory is rare because leaves contain relatively low amounts of energy and thus may be an inefficient food source for birds (Montgomery, 1978). Morton (1967) reported that White-crowned sparrows (Z. leucophrys gambelii) consumed ~35–50 kcal per day during the prenuptial molt period (Mar to Apr) and speculated that feeding on high caloric foods like plant buds and insects could facilitate fat deposition during the period of premigratory hyperphagia. Ettinger and King (1981) found that ovarian growth of this species increased when green plant tissue was included in the diet of captive birds and speculated that this may affect reproductive success. The direct signs of bird folivory on several understory weeds (S. media, C. bursa-pastoris, S. oleraceus) and the presence of foliage or reproductive plant tissue of these in droppings indicates that these relatively common weed species may provide important winter food for sparrows. It would be useful to know if consumption of weed vegetative tissue by birds in the understory of cover crops reduces weed seed production and hence weed management costs in subsequent cash crops.
Dietary Needs
Plumage synthesis by the White-crowned Sparrow during molting utilizes more sulfur amino acids (cysteine, methionine) than are used during non-molting periods (Murphy and King, 1992). The feathers of this species contain substantially higher levels of sulfur amino acids than feathers of many other birds (King and Murphy, 1987). Glucosinolates are well-studied secondary metabolites in mustards and other Brassicaceae that play important roles in plant-insect and multitrophic interactions (Hopkins et al., 2009). Glucosinolate biosynthesis occurs in the leaves and utilizes sulfur amino acids (Halkier and Gershenzon, 2006). I speculate that feeding on sulfur-rich mustard leaves helps the White-crowned Sparrow to meet its essential dietary requirements for sulfur amino acids. Moreover, it seems likely that the White-crowned Sparrow's preference for mustard leaves may be because they can taste or smell glucosinolates as they feed on the leaves. The glucosinolate content of Brasicaceae is known to mediate interactions with other organisms (Glen et al., 1990; Giamoustaris and Mithen, 1995; Hopkins et al., 1998) but is not well-studied in birds. Selective feeding by wild birds to meet specific nutrient requirements is poorly understood, however, White-crowned Sparrows are known to discriminate between diets that differ in the amount of sulfur amino acids (Murphy and King, 1987). Similarly, Vireos can discriminate between diets differing in fatty acid composition (Pierce et al., 2004). Although the mustard cover crop was a mixture of two species (S. alba, B. juncea), S. alba was the taller, more productive and dominant species in the mixture (Brennan, unpublished data) and was thus the primary mustard that the White-crowned Sparrow fed on.
Management Implications and Future Research Needs
This paper provides the first information on interactions between winter cover crops and birds in the intensive agricultural systems of the central coast of California. The biodiversity and abundance of birds and other wildlife in intensive agricultural systems is complex and is affected by ecological heterogeneity at multiple temporal and spatial scales (Benton et al., 2003). Peterjohn (2003) noted that reverting to less intensive agricultural practices across North America is not realistic, and suggested the need for innovative solutions to farmland bird conservation based on current agricultural practices. In the intensive, high-value cropping systems in the central coast of California, growing winter cover crops increases habitat heterogeneity and could provide valuable food and foraging habitat for birds and augment resources provided by permanent non-crop habitats such as hedgerows. In turn, these birds may provide ecosystem services by eating weed foliage and weed seed under the cover crop and pest insects. While winter cover cropping in regions such as the Salinas Valley is still relatively uncommon, even on many organic farms, it seems likely to increase as farmers here are required to implement more sustainable soil management practices (Brennan, 2017).
More research is needed to understand the year-round habits and population dynamics of farmland birds and the role of cover crops vs. non-cropped areas on birds. Such research is critical to help farmers in the central coast region, who are under increasing scrutiny due to concerns about food safety (Beretti and Stuart, 2008; Karp et al., 2015; Hughes et al., 2019; Olimpi et al., 2020), to understand and appreciate the differences between bird species on their farms and develop management practices that minimize crop losses while enhancing ecosystem services that birds provide. While this issue applies to all farms that are working to improve their sustainability, it is particularly important on certified organic farms where USDA National Organic regulations require biodiversity and natural resource conservation (USDA National Organic Program, 2016; WFA, 2016).
The White-crowned Sparrow is an important species for future bird research in this region given its clear preference for mustard cover crops, and its ability to cause serious damage to vegetables like broccoli planted near hedgerows during the winter (Figure 8). Broccoli is grown in Salinas Valley year-round as one of the most economically important crops (annual crop value of U.S. $388 million, Monterey County Agricultural Commissioner, 2018) and for its ability to reduce soilborne diseases of other important crops (Subbarao et al., 1999, 2007; Hao et al., 2003). I remember the frustration expressed by my farmer neighbor who called me in the winter of 2012 to discuss his damaged broccoli (Figure 8), because he referred to the birds that damaged the broccoli as “your birds.” He considered the birds that damaged his broccoli to be my birds because they were often seen in the hedgerow on the organic research farm that I have managed since 2001. He wanted the hedgerow removed. While I understood his frustration, I did my best to explain that the hedgerow was an important and essential part of the biological control efforts on the organic farm because of all the beneficial insects that reside in it, as I later described in this video (Brennan, 2015). The 2012 broccoli incident was the first time in more than a decade that I had managed the organic research farm, that my conventional farmer neighbor had expressed frustration to me about birds in his field. This might be because most winters this field adjacent to the hedgerow had been bare fallowed, however, interestingly the winter of 2012 was also the first year in the previous 8 years that mustard cover crops were not grown on the long-term study in the organic field adjacent to the hedgerow. I speculate that growing mustard in the organic field adjacent to the hedgerow might have reduced or eliminated damage to the conventional broccoli crop. In other words, I believe that a mustard cover crop could be strategically planted adjacent to a hedgerow to act as a trap crop to help meet the dietary needs of the White-crown Sparrow during the relatively short winter period that they occur in the hedgerow. It is important to note that broccoli was grown and harvested on a commercial scale in this organic field adjacent to the hedgerow from Jul to Nov of 2005 to 2010 with no damage by the White-crowned Sparrow. This is likely because the White-crowned Sparrows that occur in hedgerows in the winter are a migratory subspecies (pugetensis or gambelii), although this is one of the important questions that needs to be answered in future research.
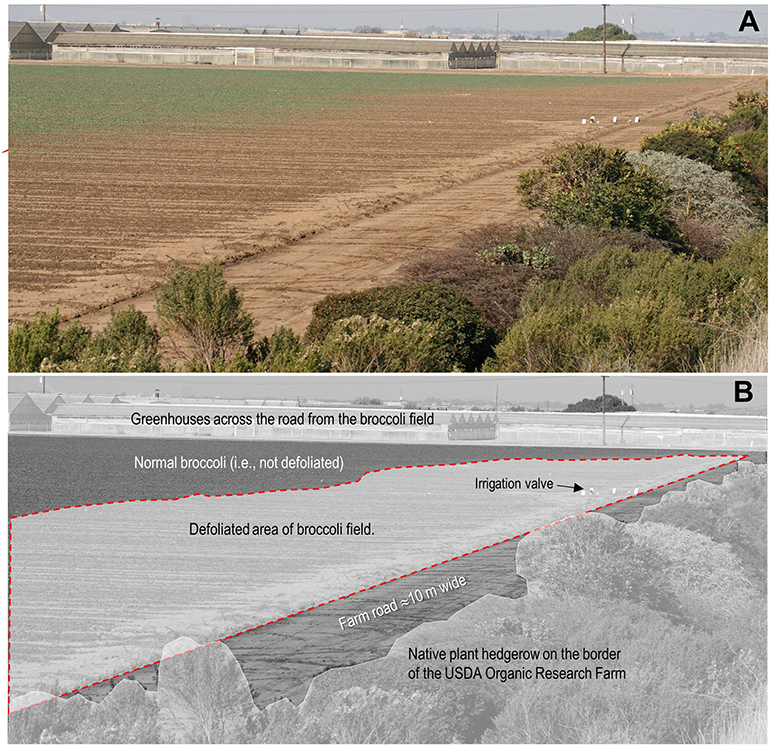
Figure 8. Defoliated broccoli in a conventional farm adjacent to the hedgerow on the USDA organic research farm in Salinas, CA. The color photograph (A) was taken Jan 31, 2012 and shows the defoliated area from feeding by the White-crowned Sparrow, that extending ~10 m into the broccoli field or ~20 m from the edge of the hedgerow. Key features of the photograph are labeled in the black and white version (B). The fields adjacent to the hedgerow on the organic farm had strawberries and a rye cover crop when the photograph was taken. See Figure 1B for an aerial view of this conventional broccoli field relative to the experimental field described in the paper. There is an asphalt road (Spence Road) between the broccoli field the greenhouses in the background. The geographic coordinates of the irrigation value in the field are 36°37'18.4 “N 121°32'59.1” W.
Conclusion
This paper provides the first data on sparrow preferences in three broad categories of winter cover crops that are used in high-value vegetable systems in the central coast region of California. Based on the abundance and composition of bird droppings, mustard cover crops are preferred by the foliage-eating White-crowned Sparrows, while the Song and Savannah Sparrows, that feed on insects and seeds, preferred rye and legume-rye cover crops. The White-crowned Sparrow's preference for mustard is likely due to the sulfur-rich amino acids in the leaves that may help to meet its dietary needs during the prenuptial molt. This clear preference by the White-crowned Sparrow suggests that farmers might be able to strategically plant mustard as a trap crop to help meet the dietary needs of this sparrow that can be challenging in winter vegetable plantings adjacent to hedgerows. Future research on this and other birds in the central coast region of California is urgently needed to help farmers gain a more nuanced understanding of birds in these agroecosystems and develop strategies to maximize the ecosystem benefits that birds provide while minimizing crop losses.
Data Availability Statement
The original contributions presented in the study are included in the article/Supplementary Material, further inquiries can be directed to the corresponding author.
Ethics Statement
This study only observed the behavior of wild birds in an agricultural field when the researcher walked through the field and thus did not require ethical review.
Author Contributions
EB designed the study, collected, analyzed the data, and wrote the manuscript.
Funding
United States Department of Agriculture.
Conflict of Interest
The author declares that the research was conducted in the absence of any commercial or financial relationships that could be construed as a potential conflict of interest.
Acknowledgments
I thank G. B. Ochoa and R. F. Smith for assistance with planting the cover crops. This manuscript was improved by comments from N. S. Boyd and R. C. Grube.
Supplementary Material
The Supplementary Material for this article can be found online at: https://www.frontiersin.org/articles/10.3389/fsufs.2020.567579/full#supplementary-material
References
Anderson, D. R., Burnham, K. P., and Thompson, W. L. (2000). Null hypothesis testing: problems, prevalence, and an alternative. J. Wildl. Manage. 64, 912–923. doi: 10.2307/3803199
Arcese, P., Sogge, M. K., Marr, A. B., and Patten, M. A. (2020). “Song sparrow (Melospiza melodia) version 1.0,” in The Birds of the World, ed A. Poole (Ithaca, NY: Cornell Lab of Ornithology). doi: 10.2173/bow.sonspa.01
Atkinson, P. W., Buckingham, D., and Morris, A. J. (2004). What factors determine where invertebrate-feeding birds forage in dry agricultural grasslands? Ibis 146, 99–107. doi: 10.1111/j.1474-919X.2004.00346.x
Baumgartner, L. L., and Martin, A. C. (1939). Plant histology as an aid in squirrel food-habit studies. J. Wildl. Manage. 3, 266–268. doi: 10.2307/3796113
Benton, T. G., Bryant, D. M., Cole, L., and Crick, H. Q. P. (2002). Linking agricultural practice to insect and bird populations: a historical study over three decades. J. Appl. Ecol. 39, 673–687. doi: 10.1046/j.1365-2664.2002.00745.x
Benton, T. G., Vickery, J. A., and Wilson, J. D. (2003). Farmland biodiversity: is habitat heterogeneity the key? Trends Ecol. Evol. 18, 182–188. doi: 10.1016/S0169-5347(03)00011-9
Beretti, M., and Stuart, D. (2008). Food safety and environmental quality impose conflicting demands on Central Coast growers. Calif. Agric. 62, 68–73. doi: 10.3733/ca.v062n02p68
Boyd, N. S., and Brennan, E. B. (2006). Weed management in a legume-cereal cover crop with the rotary hoe. Weed Technol. 20, 733–737. doi: 10.1614/WT-05-157R.1
Brennan, E. B. (2013). Agronomic aspects of strip intercropping lettuce with alyssum for biological control of aphids. Biol. Control 65, 302–311. doi: 10.1016/j.biocontrol.2013.03.017
Brennan, E. B. (2015). A Biological Control Buffet in the Salad Bowl of America. YouTube. Available online at: https://www.youtube.com/watch?v=zLvJLHERYJI (accessed 25 May 2020).
Brennan, E. B. (2017). Can we grow organic or conventional vegetables sustainably without cover crops? Horttechnology 27, 151–161. doi: 10.21273/HORTTECH03358-16
Brennan, E. B., and Boyd, N. S. (2012). Winter cover crop seeding rate and variety affects during eight years of organic vegetables: I. Cover crop biomass production. Agron. J. 104, 684–698. doi: 10.2134/agronj2011.0330
Brennan, E. B., Boyd, N. S., Smith, R. F., and Foster, P. (2009). Seeding rate and planting arrangement effects on growth and weed suppression of a legume-oat cover crop for organic vegetable systems. Agron. J. 101, 979–988. doi: 10.2134/agronj2008.0194x
Brennan, E. B., and Smith, R. F. (2005). Winter cover crop growth and weed suppression on the central coast of California. Weed Technol. 19, 1017–1024. doi: 10.1614/WT-04-246R1.1
Brennan, L. A., and Kuvlesky, W. P. (2005). North American grassland birds: an unfolding conservation crisis? J. Wildl. Manage. 69, 1–13. doi: 10.2193/0022-541X(2005)069<0001:NAGBAU>2.0.CO;2
Butler, S. J., and Gillings, S. (2004). Quantifying the effects of habitat structure on prey detectability and accessibility to farmland birds. Ibis 146, 123–130. doi: 10.1111/j.1474-919X.2004.00352.x
Butler, S. J., Vickery, J. A., and Norris, K. (2007). Farmland biodiversity and the footprint of agriculture. Science 315, 381–384. doi: 10.1126/science.1136607
Campbell, K. G., Thompson, Y. M., Guy, S. O., McIntosh, M., and Glaz, B. (2015). Is, or is not, the two great ends of fate: errors in agronomic research. Agron. J. 107, 718–729. doi: 10.2134/agronj14.0167
Cederbaum, S. B., Carroll, J. P., and Cooper, R. J. (2004). Effects of alternative cotton agriculture on avian and arthropod populations. Conserv. Biol. 18, 1272–1282. doi: 10.1111/j.1523-1739.2004.00385.x
Chamberlain, D. E., Freeman, S. N., and Vickery, J. A. (2007). The effects of GMHT crops on bird abundance in arable fields in the UK. Agric. Ecosyst. Environ. 118, 350–356. doi: 10.1016/j.agee.2006.05.012
Chamberlain, D. E., Fuller, R. J., Bunce, R. G. H., Duckworth, J. C., and Shrubb, M. (2000). Changes in the abundance of farmland birds in relation to the timing of agricultural intensification in England and Wales. J. Appl. Ecol. 37, 771–788. doi: 10.1046/j.1365-2664.2000.00548.x
Chilton, G., Baker, M. C., Barrentine, C. D., and Cunningham, M. A. (2020). “White-crowned sparrow (Zonotrichia leucophrys), version 1.0,” in The Birds of the World, ed A. Poole (Ithaca, NY: Cornell Lab of Ornithology). doi: 10.2173/bow.whcspa.01
Cumming, G. (2007). Inference by eye: pictures of confidence intervals and thinking about levels of confidence. Teach. Stat. 29, 89–93. doi: 10.1111/j.1467-9639.2007.00267.x
Cumming, G. (2014). The new statistics: why and how. Psychol. Sci. 25, 7–29. doi: 10.1177/0956797613504966
Cumming, G., and Calin-Jageman, R. (2017). Introduction to the New Statistics: Estimation, Open Science, and Beyond. New York, NY: Routledge. doi: 10.4324/9781315708607
Cumming, G., and Fidler, F. (2009). Confidence intervals better answers to better questions. Zeitschrift Psychol. J. Psychol. 217, 15–26. doi: 10.1027/0044-3409.217.1.15
Cumming, G., and Finch, S. (2005). Inference by eye-confidence intervals and how to read pictures of data. Am. Psychol. 60, 170–180. doi: 10.1037/0003-066X.60.2.170
Devereux, C. L., Vickery, J. A., Fernandez-Juricic, E., Krebs, J. R., and Whittingham, M. J. (2006). Does sward density affect prey availability for grassland birds? Agric. Ecosyst. Environ. 117, 57–62. doi: 10.1016/j.agee.2006.03.007
Donald, P. F., Green, R. E., and Heath, M. F. (2001). Agricultural intensification and the collapse of Europe's farmland bird populations. Proc. R. Soc. Lond. B Biol. Sci. 268, 25–29. doi: 10.1098/rspb.2000.1325
Earnshaw, S. (2018). Hedgerows for California Agriculture, Community Alliance with Family Farmers. Available online at: https://www.caff.org/wp-content/uploads/2020/08/CAFF_Hedgerow_Manual_2018.pdf (accessed September 24, 2020).
Ettinger, A. O., and King, J. R. (1981). Comsumption of green wheat enhances photostimulated ovarian growth in white-crowned sparrows. Auk 98, 832–834.
Evans, S. A., Mougeot, F., Redpath, S. M., and Leckie, F. (2007). Alternative methods for estimating density in an upland game bird: the red grouse Lagopus lagopus scotieus. Wildl. Biol. 13, 130–139. doi: 10.2981/0909-6396(2007)13[130:AMFEDI]2.0.CO;2
Fidler, F., Burgman, M. A., Cumming, G., Buttrose, R., and Thomason, N. (2006). Impact of criticism of null-hypothesis significance testing on statistical reporting practices in conservation biology. Conserv. Biol. 20, 1539–1544. doi: 10.1111/j.1523-1739.2006.00525.x
Giamoustaris, A., and Mithen, R. (1995). The effect of modifying the glucosinolate content of leaves of oilseed rape (Brassica napus ssp. oleifera) on its interaction with specialist and generalist pests. Ann. Appl. Biol. 126, 347–363. doi: 10.1111/j.1744-7348.1995.tb05371.x
Gibbons, D. W., Bohan, D. A., Rothery, P., Stuart, R. C., Haughton, A. J., Scott, R. J., et al. (2006). Weed seed resources for birds in fields with contrasting conventional and genetically modified herbicide-tolerant crops. Proc. R. Soc. Lond. B Biol. Sci. 273, 1921–1928. doi: 10.1098/rspb.2006.3522
Glen, D. M., Jones, H., and Fieldsend, J. K. (1990). Damage to oilseed rape seedlings by the field slug Deroceras reticulatum in relation to glucosinolate concentration. Ann. Appl. Biol. 117, 197–207. doi: 10.1111/j.1744-7348.1990.tb04207.x
Green, R. (1978). Factors affecting the diet of farmland skylarks, Alauda arvensis. J. Appl. Ecol. 47, 913–928. doi: 10.2307/3678
Green, R. E., Cornell, S. J., Scharlemann, J. P. W., and Balmford, A. (2005). Farming and the fate of wild nature. Science 307, 550–555. doi: 10.1126/science.1106049
Halkier, B. A., and Gershenzon, J. (2006). Biology and biochemistry of glucosinolates. Annu. Rev. Plant Biol. 57, 303–333. doi: 10.1146/annurev.arplant.57.032905.105228
Hao, J. J., Subbarao, K. V., and Koike, S. T. (2003). Effects of broccoli rotation on lettuce drop caused by Sclerotinia minor and on the population density of sclerotia in soil. Plant Dis. 87, 159–166. doi: 10.1094/PDIS.2003.87.2.159
Hopkins, R. J., Ekbom, B., and Henkow, L. (1998). Glucosinolate content and susceptibility for insect attack of three populations of Sinapis alba. J. Chem. Ecol. 24, 1203–1216. doi: 10.1023/A:1022498903175
Hopkins, R. J., van Dam, N. M., and van Loon, J. J. A. (2009). Role of glucosinolates in insect-plant relationships and multitrophic interactions. Annu. Rev. Entomol. 54, 57–83. doi: 10.1146/annurev.ento.54.110807.090623
Hubbard, R., and Lindsay, R. M. (2008). Why P values are not a useful measure of evidence in statistical significance testing. Theory Psychol. 18, 69–88. doi: 10.1177/0959354307086923
Hughes, D. F., Green, M. L., Warner, J. K., and Davidson, P. C. (2019). There's a frog in my salad! A review of online media coverage for wild vertebrates found in prepackaged produce in the United States. Sci. Total Environ. 675, 1–12. doi: 10.1016/j.scitotenv.2019.03.254
Jackson, L. E., Wyland, L. J., and Stivers, L. J. (1993). Winter cover crops to minimize nitrate losses in intensive lettuce production. J. Agric. Sci. 121, 55–62. doi: 10.1017/S0021859600076796
Jennings, T. J., and Barkham, J. P. (1975). Food of slugs in mixed deciduous woodland. Oikos 26, 211–221. doi: 10.2307/3543711
Karp, D. S., Gennet, S., Kilonzo, C., Partyka, M., Chaumont, N., Atwill, E. R., et al. (2015). Comanaging fresh produce for nature conservation and food safety. Proc. Natl. Acad. Sci. U.S.A. 112, 11126–11131. doi: 10.1073/pnas.1508435112
King, J. R., and Murphy, M. E. (1987). Amino-acid-composition of the calamus, rachis, and barbs of White-crowned Sparrow feathers. Condor 89, 436–439. doi: 10.2307/1368503
Lambdin, C. (2012). Significance tests as sorcery: science is empirical-significance tests are not. Theory Psychol. 22, 67–90. doi: 10.1177/0959354311429854
Lisovski, S., Nemeth, Z., Wingfield, J. C., Krause, J. S., Hobson, K. A., Seavy, N. E., et al. (2019). Migration pattern of gambel's white-crowned sparrow along the pacific flyway. J. Ornithol. 160, 1097–1107. doi: 10.1007/s10336-019-01685-4
Maltais-Landry, E., Scow, K., Brennan, E. B., Torbert, E., and Vitousek, P. (2016). Higher flexibility in input N:P ratios results in more balanced phosphorus budgets in two long-term experimental agroecosystems. Agric. Ecosyst. Environ. 223, 197–210. doi: 10.1016/j.agee.2016.03.007
Marshall, E. J. P., Brown, V. K., Boatman, N. D., Lutman, P. J. W., Squire, G. R., and Ward, L. K. (2003). The role of weeds in supporting biological diversity within crop fields. Weed Res. 43, 77–89. doi: 10.1046/j.1365-3180.2003.00326.x
Martin, D. J. (1955). Features on plant cuticle: an aid to the analysis of the natural diet of grazing animals, with special reference to scottish hill sheep. Trans Botan. Soc. Edinburgh 36, 278–288. doi: 10.1080/13594865509441615
Matson, P. A., Parton, W. J., Power, A. G., and Swift, M. J. (1997). Agricultural intensification and ecosystem properties. Science 277, 504–509. doi: 10.1126/science.277.5325.504
McCracken, D. I., and Tallowin, J. R. (2004). Swards and structure: the interactions between farming practices and bird food resources in lowland grasslands. Ibis 146, 108–114. doi: 10.1111/j.1474-919X.2004.00360.x
Monterey County Agricultural Commissioner (2018). Monterey County Crop Report 2018. Monterey County Agricultural Commissioner. Available online at: https://www.co.monterey.ca.us/home/showdocument?id=78579 Agricultural Commissioner. (accessed April 6, 2020).
Montgomery, G. G. (1978). “Avian folivores: why not?,” in The Ecology of Arboreal Folivores, ed G. G. Montgomery (Washington, DC: Smithsonian Insititution Press), 123–130.
Moorcroft, D., Whittingham, M. J., Bradbury, R. B., and Wilson, J. D. (2002). The selection of stubble fields by wintering granivorous birds reflects vegetation cover and food abundance. J. Appl. Ecol. 39, 535–547. doi: 10.1046/j.1365-2664.2002.00730.x
Morton, M. L. (1967). Diurnal feeding patterns in white-crowned sparrows, Zonotrichia leucophrys gambelii. Condor 69, 491–512. doi: 10.2307/1366149
Murphy, M. E., and King, J. R. (1987). Dietary discrimination by molting white-crowned sparrows given diets differing only in sulfur amino-acid concentration. Physiol. Zool. 60, 279–289. doi: 10.1086/physzool.60.2.30158652
Murphy, M. E., and King, J. R. (1992). Energy and nutrient use during molt by white-crowned sparrows zonotrichia-leucophrys-gambelii. Ornis Scandinavica 23, 304–313. doi: 10.2307/3676654
Murphy, M. T. (2003). Avian population trends within the evolving agricultural landscape of eastern and central United States. Auk 120, 20–34. doi: 10.1642/0004-8038(2003)120[0020:APTWTE]2.0.CO;2
Nakagawa, S., and Cuthill, I. C. (2007). Effect size, confidence interval and statistical significance: a practical guide for biologists. Biol. Rev. 82, 591–605. doi: 10.1111/j.1469-185X.2007.00027.x
Odderskaer, P., Prang, A., Poulsen, J. G., Andersen, P. N., and Elmegaard, N. (1997). Skylark (Alauda arvensis) utilisation of micro-habitats in spring barley fields. Agric. Ecosyst. Environ. 62, 21–29. doi: 10.1016/S0167-8809(96)01113-9
Olimpi, E. M., Garcia, K., Gonthier, D. J., De Master, K. T., Echeverri, A., Kremen, C., et al. (2020). Shifts in species interactions and farming contexts mediate net effects of birds in agroecosystems. Ecol. Appl. 30:e02115. doi: 10.1002/eap.2115
Perkins, A. J., Whittingham, M. J., Bradbury, R. B., Wilson, J. D., Morris, A. J., and Barnett, P. R. (2000). Habitat characteristics affecting use of lowland agricultural grassland by birds in winter. Biol. Conserv. 95, 279–294. doi: 10.1016/S0006-3207(00)00042-2
Peterjohn, B. G. (2003). Agricultural landscapes: can they support healthy bird populations as well as farm products? Auk 120, 14–19. doi: 10.1093/auk/120.1.14
Pierce, B. J., McWllliams, S. R., Place, A. R., and Huguenin, M. A. (2004). Diet preferences for specific fatty acids and their effect on composition of fat reserves in migratory Red-eyed Vireos (Vireo olivaceous). Comp. Biochem. Physiol. Mol. Integr. Physiol. 138, 503–514. doi: 10.1016/j.cbpb.2004.06.014
Pullman, H. R., and Mills, G. S. (1977). The use of space by wintering sparrows. Ecology 58, 1393–1399. doi: 10.2307/1935091
Ramenofsky, M., and Wingfield, J. C. (2006). Behavioral and physiological conflicts in migrants: the transition between migration and breeding. J. Ornithol. 147, 135–145. doi: 10.1007/s10336-005-0050-4
Rosenberg, K. V., Dokter, A. M., Blancher, P. J., Sauer, J. R., Smith, A. C., Smith, P. A., et al. (2019). Decline of the North American avifauna. Science 366:120. doi: 10.1126/science.aaw1313
Shutler, D., Mullie, A., and Clark, R. G. (2000). Bird communities of prairie uplands and wetlands in relation to farming practices in Saskatchewan. Conserv. Biol. 14, 1441–1451. doi: 10.1046/j.1523-1739.2000.98246.x
Smith, E. P. (2020). Ending reliance on statistical significance will improve environmental inference and communication. Estuaries Coasts 43, 1–6. doi: 10.1007/s12237-019-00679-y
Smith, R. J. (2018). The continuing misuse of null hypothesis significance testing in biological anthropology. Am. J. Phys. Anthropol. 166, 236–245. doi: 10.1002/ajpa.23399
Sterne, J. A. C., and Smith, G. D. (2001). Sifting the evidence-what's wrong with significance tests? Br. Med. J. 322, 226–231. doi: 10.1136/bmj.322.7280.226
Stevens, E. J., Stevens, S. J., Gates, R. N., Eskridge, K. M., and Waller, S. S. (1987). Procedure for fecal cuticle analysis of herbivore diets. J. Range Manage. 40, 187–189. doi: 10.2307/3899218
Stewart, D. R. M. (1967). Analysis of plant epidermis in faeces - a technique for studying food preferences of grazing herbivores. J. Appl. Ecol. 4, 83–111. doi: 10.2307/2401411
Storkey, J., and Westbury, D. B. (2007). Managing arable weeds for biodiversity. Pest Manag. Sci. 63, 517–523. doi: 10.1002/ps.1375
Subbarao, K. V., Hubbard, J. C., and Koike, S. T. (1999). Evaluation of broccoli residue incorporation into field soil for verticillium wilt control in cauliflower. Plant Dis. 83, 124–129. doi: 10.1094/PDIS.1999.83.2.124
Subbarao, K. V., Kabir, Z., Martin, F. N., and Koike, S. T. (2007). Management of soilborne diseases in strawberry using vegetable rotations. Plant Dis. 91, 964–972. doi: 10.1094/PDIS-91-8-0964
Takatsuki, S., and Morinaga, Y. (2020). Food habits of horses, cattle, and sheep-goats and food supply in the forest-steppe zone of Mongolia: A case study in Mogod sum (county) in Bulgan aimag (province). J. Arid Environ. 174, 7. doi: 10.1016/j.jaridenv.2019.104039
Tilman, D., Cassman, K. G., Matson, P. A., Naylor, R., and Polasky, S. (2002). Agricultural sustainability and intensive production practices. Nature 418, 671–677. doi: 10.1038/nature/01014
USDA National Organic Program (2016). Guidance: Natural Resources and Biodiversity Conservation A.M.S. United States Department of Agriculture. Available online at: https://www.ams.usda.gov/sites/default/files/media/NOP%205020%20Biodiversity%20Guidance%20Rev01%20%28Final%29.pdf (accessed May 28, 2020).
Vaughan, T. A. (1967). Food habits of northern pocket gopher on shortgrass prairie. Am. Mid. Natura. 77, 176–189. doi: 10.2307/2423437
WFA (2016). Biodiversity Conservation: An Organic Farmer's and Certifier's Guide. Wild Farm Alliance. Available online at: https://www.wildfarmalliance.org/biodiversity_guide (accessed 28 May, 2020).
Wheelwright, N. T., and Rising, J. D. (2020). “Savannah sparrow (Passerculus sandwichensis) version 1.0,” in The Birds of the World, ed A. Poole (Ithaca, NY: Cornell Lab of Ornithology). doi: 10.2173/bow.savspa.01
White, K. E., Brennan, E. B., Cavigelli, M. A., and Smith, R. F. (2020). Winter cover crops increase readily decomposable soil carbon, but compost drives total soil carbon during eight years of intensive, organic vegetable production in California. PLoS ONE 15:e0228677. doi: 10.1371/journal.pone.0228677
Whittingham, M. J., and Evans, K. L. (2004). The effects of habitat structure on predation risk of birds in agricultural landscapes. Ibis 146, 210–220. doi: 10.1111/j.1474-919X.2004.00370.x
Wilson, J. D., Whittingham, M. J., and Bradbury, R. B. (2005). The management of crop structure: a general approach to reversing the impacts of agricultural intensification on birds? Ibis 147, 453–463. doi: 10.1111/j.1474-919x.2005.00440.x
Wyland, L. J., Jackson, L. E., Chaney, W. E., Klonsky, K., Koike, S. T., and Kimple, B. (1996). Winter cover crops in a vegetable cropping system: impacts on nitrate leaching, soil water, crop yield, pests and management costs. Agric. Ecosyst. Environ. 59, 1–17. doi: 10.1016/0167-8809(96)01048-1
Keywords: bird droppings, rye, legume-rye mixtures, mustard, Salinas valley, Melospiza melodia, Passerculus sandwichensis, Zonotrichia leucophyrs
Citation: Brennan EB (2020) Sparrow Preferences for Winter Cover Crops in California's Central Coast. Front. Sustain. Food Syst. 4:567579. doi: 10.3389/fsufs.2020.567579
Received: 30 May 2020; Accepted: 10 September 2020;
Published: 17 November 2020.
Edited by:
Leslie George Firbank, University of Leeds, United KingdomReviewed by:
Dorcas Honora Franklin, University of Georgia, United StatesEmma Louise Burns, Australian National University, Australia
Copyright © 2020 Brennan. This is an open-access article distributed under the terms of the Creative Commons Attribution License (CC BY). The use, distribution or reproduction in other forums is permitted, provided the original author(s) and the copyright owner(s) are credited and that the original publication in this journal is cited, in accordance with accepted academic practice. No use, distribution or reproduction is permitted which does not comply with these terms.
*Correspondence: Eric B. Brennan, ZXJpYy5icmVubmFuQHVzZGEuZ292