- 1Agroecology, Environment and Systems Group, Instituto de Investigaciones Forestales y Agropecuarias de Bariloche (IFAB), Instituto Nacional de Tecnología Agropecuaria – Consejo Nacional de Investigaciones Científicas y Técnicas, San Carlos de Bariloche, Argentina
- 2Conservation Ecology Group, Groningen Institute of Evolutionary Life Sciences, Groningen University, Groningen, Netherlands
- 3Agroécologie et Intensification Durable (AïDA), Centre de Coopération Internationale en Recherche Agronomique pour le Développement (CIRAD), Université de Montpellier, Montpellier, France
- 4Facultad de Agronomía, Instituto de Investigaciones Fisiológicas y Ecológicas Vinculadas a la Agricultura, Consejo Nacional de Investigaciones Científicas y Técnicas (CONICET), Universidad de Buenos Aires, Buenos Aires, Argentina
- 5Departamento de Sistemas Ambientales, Facultad de Agronomía, Universidad de la República, Montevideo, Uruguay
- 6Universidad Nacional de Río Negro and Consejo Nacional de Investigaciones Científicas y Técnicas (UNRN-CONICET), Instituto de Investigaciones en Recursos Naturales, Agroecología y Desarrollo Rural, San Carlos de Bariloche, Argentina
- 7Instituto de Investigaciones en Recursos Naturales, Universidad Nacional de Río Negro, Agroecología y Desarrollo Rural, Río Negro, Argentina
- 8Departamento de Producción Vegetal, Facultad de Agronomía, Universidad de la República, Montevideo, Uruguay
- 9Grupo de Estudios Ambientales, Instituto de Matemática Aplicada San Luis, Consejo Nacional de Investigaciones Científicas y Técnicas (CONICET), San Luis, Argentina
- 10South American Institute for Resilience and Sustainability Studies, Maldonado, Uruguay
Agroecology promises a third way between common global agriculture tradeoffs such as food production and nature conservation, environmental sustainability and ecosystem services. However, most successful examples of mainstreaming agroecology come from smallholder, family agriculture, that represents only about 30% of the world agricultural area. Mainstreaming agroecology among large scale farmers is urgently needed, but it requires addressing specific questions in research, technology and policy development to support sustainable transitions. Here we take stock of the existing knowledge on some key aspects necessary to support agroecological transitions in large scale farming, considering two contrasting starting points: highly subsidized and heavily taxed agricultural contexts, represented here by the examples of Western Europe and temperate South America. We summarize existing knowledge and gaps around service crops, arthropod-mediated functions, landscape and watershed regulation, graze-based livestock, nature-inclusive landscapes, and policy mechanisms to support transitions. We propose a research agenda for agroecology in large scale farming organized in five domains: (i) Breeding for diversity, (ii) Scalable complexity, (iii) Managing cycles beyond fields and farms, (iv) Sharing the cultivated landscape, and (v) Co-innovation with farmers, value chains and policy makers. Agroecology may result in a renewed impetus in large scale farming, to attract the youth, foster clean technological innovation, and to promote a new generation of large-scale farmers that take pride in contributing to feeding the world while serving the planet and its people.
Introduction
As the extent and output of agriculture still grows to new global records, so does the awareness of societies on the multiple contributions of land ecosystems to human well-being. Agroecology, or the application of social and ecological principles for the design and management of sustainable agroecosystems, sits at the core of this tension promising one of the very few ways out from the current trade-offs between food production and security and the broader well-being of humans and nature. Over the last decades large scale farming led to increasing production but also caused substantial environmental degradation, as such increases were mostly based on its expansion onto natural areas and greater use of external inputs and other forms of intensified use (IPBES, 2019). Meanwhile agroecology developed and became most successful in small scale family farming, which contributes roughly more than half of the food consumed by humans by one estimate, farming on <30% of the agricultural land (Graeub et al., 2016; Lowder et al., 2019). The potential of agroecology to become a “third way” model, able to address the tradeoffs between food security and other ecosystem services has raised interest among governments, development agencies, scientists and farmers (e.g., HLPE, 2019; WWF, 2020 etc.). Yet, the necessary step for the expansion and global scaling of agroecological approaches needs to consider their adaptation to large-scale commercial contexts. While several enlightening examples exist, we think that an open but assertive research agenda is still needed.
Large scale farming, which occupies the majority of the global agricultural area, is defined here as the highly mechanized, commercial cropping and livestock keeping activities that take place in privately owned or rented land by an individual farmer, company or family enterprise. This sector is responsible for 70% of current deforestation, the largest share of agriculture-related GHG emissions and agricultural water use, and habitat disruption resulting in biodiversity loss (IPBES, 2019).
Although examples do exist of large-scale commercial farming that follows the principles of agroecology, both in the Americas and in Europe (Kleijn et al., 2019), a full transition toward agroecology in large scale farming entails yet a number of challenges. Among them, the lack of appropriate knowledge, management practices and technologies adapted to large field sizes, mechanized farming, several commercial and value chain impediments, and high dependence on external input technologies (often recommended by agronomic “advisors” provided by the companies that produce or commercialize those inputs, or profit from high outputs). An additional factor deterring a wide scale transition to agroecology among large scale farmers is the distortion created by financial measures such as production subsidies, mostly in the North and heavy taxation, mostly in the South. Western Europe, and the temperate regions of South America are conspicuous examples of these two end points, from heavily subsidized to heavily taxed agricultural systems.
Beyond market incentives, high input agriculture models in western Europe evolve and are shaped as the result of simultaneous top-down incentives and disincentives in the form of governmental subsidies, private credit loans, EU agri-environmental measures, regulations such as on nitrogen emissions or the EU Natura-2000 directive, or incentives for the maintenance of cultural heritage landscapes. However, high productivity per unit land or per animal is also often seen as a mark of “efficiency” by EU farmers and related sectorial actors, which has also deep-rooted bonds with traditional cultures and rural styles of living (van der Ploeg, 2013). This high productivity per animal or unit land is even frequently presented as being environmentally friendly, based on “land sparing” arguments, despite its very high environmental impact per unit area of farmland (Loconto et al., 2020). The pursue of high productivity results in elevated use of external inputs and consequent high environmental impacts per unit area in the production region, in spite of several EU regulations designed to mitigate them. A growing number of farmers and their associations, governments, and environmental NGOs tend to see agroecology as an opportunity to reduce environmental impacts (e.g., nitrogen emissions, biodiversity loss) while continuing to maintain current productivity levels.
In the Pampas and Campos natural regions of Argentina, Brazil and Uruguay large scale, export-oriented agriculture and livestock systems contribute substantially to the national economies and represent an important source of livelihood and incomes. As these systems operate without any form of subsidy, economic efficiency is a central attribute to their functioning and long-term sustainability. In the case of Argentina, for example, tax policies impose a heavy burden on farmers, with export retentions of up to 30% for the dominant crops like soybean. While agroecology tends to draw the attention of large-scale South American farmers as a means to reduce production costs or risks of failure, possible yield penalties during the initial phases of the transition deters farmers from undertaking agroecology. In the absence of subsidies or other governmental incentives, any strategy oriented to support the transition to agroecology in large scale farming needs to secure incomes and profits from the very onset of the transition.
Our objective is to take stock of the knowledge available and identify key open research questions critical for the transition of large-scale farming systems to agroecology, from the realm of agronomy, ecology and social sciences. To do that, we will first describe the space of recommendation domains for large scale farming defined by the wide gradient from subsidized to taxed agricultural contexts, and their consequences for the resulting agricultural systems. We will briefly summarize the state of knowledge on six key areas of research that need to be addressed to inform the agroecological redesign of large-scale systems, namely:
(i) Multifunctional service crops;
(ii) Arthropod-mediated ecosystem services (from plot to landscape);
(iii) Watershed regulation and soil conservation (and restoration);
(iv) Regenerative grazed-based livestock systems;
(v) Nature inclusive agricultural landscapes;
(vi) Policy and finance to foster agroecological transitions.
We end by proposing an agenda and discussing the socio-technical and political implications that promoting such changes entail. We are aware that agroecological transitions and the necessary innovation systems are complex, adaptive, and entail multiple dimensions, actors and levels (cf. Tittonell, 2019, 2020). But instead of diving into socio-technical transitions, upscaling and their inherent complexity—which comprise a number of research questions in themselves—here we chose to focus on the more practical, end-user-oriented research questions that we receive in our daily practice from farmers, advisors, policy makers, and third sector organizations engaged in biodiversity and environmental sustainability.
Intensification in Large-Scale Agriculture
The design of agroecosystems based on the principles of the green revolution coined in the 60's focused almost exclusively on obtaining products that can be harvested, without a systemic look or evaluation of the social and environmental impacts of agriculture. Specialization resulted in a simplification of the agricultural landscape, with a consequent decline in its ability to deliver ecosystem services due to a gradual loss or degradation of the natural capital (e.g., Tsiafouli et al., 2015). Economic productivity measured as income and profit has been a major driver of agricultural intensification, especially but not exclusively in terms of input intensification. Figure 1 presents a simplified model of the relationship between input intensification and (broad sense) economic productivity, measurable for example in terms of gross monetary income. The economically rational level of intensification would be, simplistically, that at which the maximum gross margin (GM = income minus costs) is attained. In many parts of the world, however, large scale intensive or industrial farming dominated by mono-cultures or intensive livestock husbandry has aimed at maximizing productivity (imax; Pmax), even at the expense of their gross margins, fueled by subsidies and other incentives, producing beyond the level of input intensification that an economic rationale would indicate (iopt; Popt—Figure 1A). High production costs and narrow margins lead to high risks of failure, which is normally absorbed by subsidies or other forms of economic compensation.
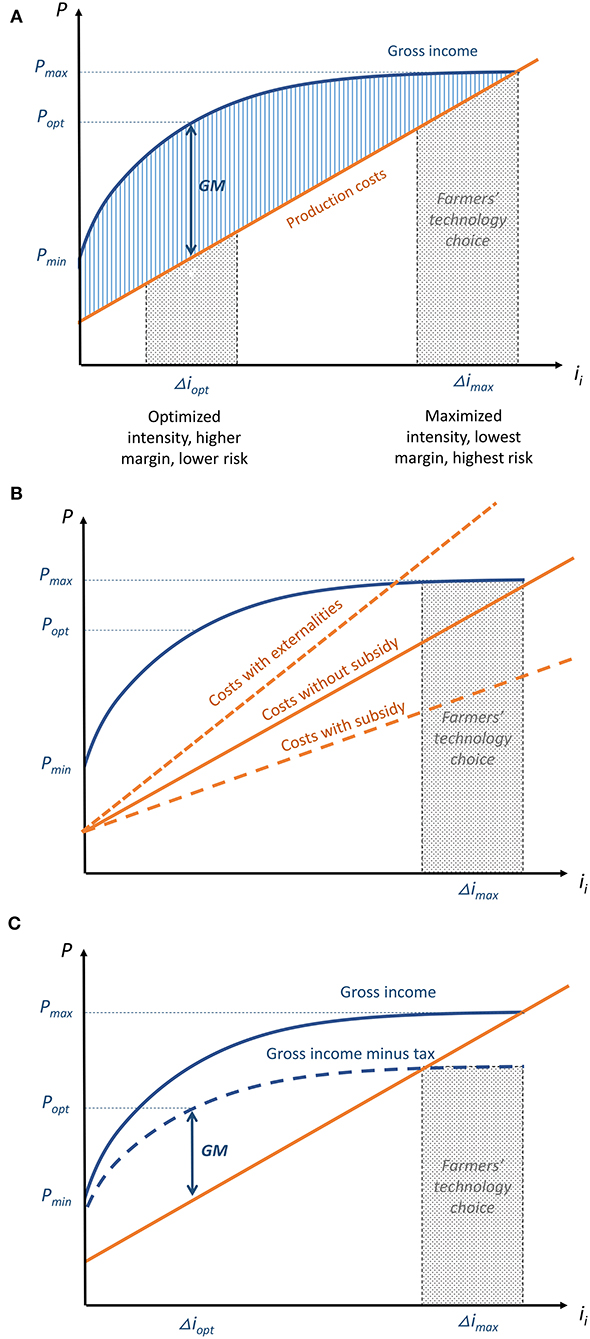
Figure 1. Schematic representation of the relationship between level of input intensification (i) and productivity (P), for example in terms of gross monetary income. (A) The widest gross margins (GM) can be attained at lower than maximum productivity levels (Popt), at a context-specific optimum level of intensification (iopt); current farmers' preferred intensification level (imax) however leads to high investment risks and narrower attainable margins, and increased intensification generally reduces ecosystem services and natural capital. (B) Countries or regions that subsidize their agriculture render imax intensification levels apparently profitable, but if subsidies were removed or externality costs (social, environmental) internalized current technological choices would render the system unprofitable. (C) Countries that tax agricultural exports render imax intensification levels totally unprofitable, leading to the misinformed perception that government tax policies are the main reason for the economic failure of such high input agriculture models (while maximum GM would still be attainable at iopt intensification levels).
Following this simple, archetypal model, the effect of subsidies can be seen as a modification in the relationship between costs and productivity that creates the false impression that these systems are “efficient” because they are highly productive (Figure 1B); or worse, that the only way of achieving efficiency is through attaining the highest possible yields per unit land area or animal head. On the other hand, if the true costs of this form of agriculture were computed, that is, including their environmental and social externalities, then the responsible levels of optimum and maximum productivities and input intensification would be much lower than those of nowadays. At the other end of the gradient (Figure 1C), countries that tax agricultural exports render imax intensification levels totally unprofitable, leading to the misinformed but common perception that government tax policies are the main reason for the economic failure of high input agriculture models. Under heavy taxation, however, the maximum GM would still be attainable at iopt intensification levels according to this simplified production function.
Yet, maximum gross margins are not necessarily attained at environmentally or socially sustainable intensification levels. The model in Figure 1 considers costs, income and margin but disregards the biophysical resource flows to and from the agricultural system, such as carbon, nutrients and water associated with the different levels of intensification. To do that, we added a new y-axis parallel to productivity that indicates increasing flows of these biophysical factors, necessary to maintain a balanced, sustainable use and long-term conservation of natural resources (Figure 2A). The ideal level of input intensification in this new model is indicated as isust, corresponding to a higher productivity (Psust). This point, arbitrarily depicted as a half-way productivity between Popt and Pmax in Figure 2A, represents a level of input intensification that ensures for example soil nutrient replenishment through fertilization, greater flows of carbon from plant litter to soil, more efficient use of rainfall water, or more diversified feeds and/or direct foraging resulting in greater animal welfare in livestock systems. Such a higher level of intensification may not necessarily result in the widest margins nor in the highest productivity, but can be seen as an investment in maintaining the natural capital. Subsidies or tax policies could be in some cases an instrument to regulate intensification levels following this logic (Figure 2A). However, there is a limit to the ability of these instruments to shape sustainable forms of agriculture, and virtually none to promote by themselves a transition to agroecological farming.
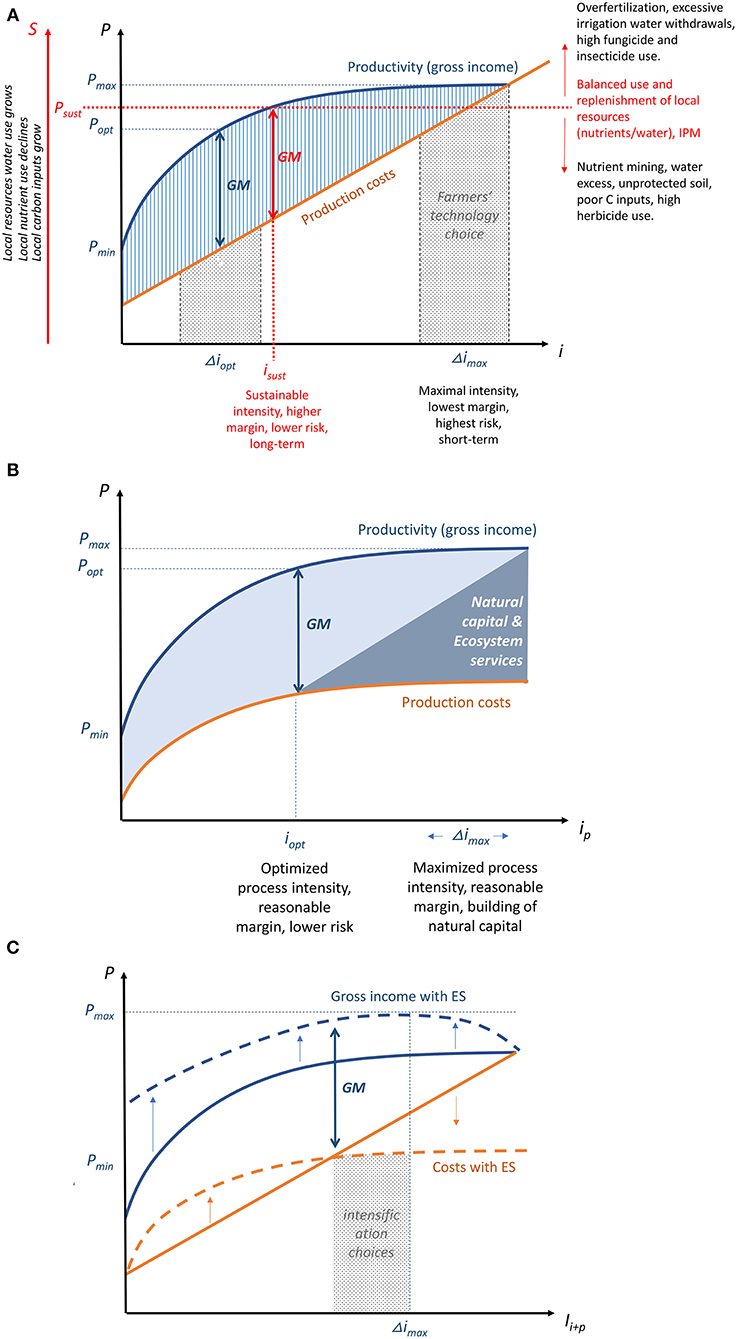
Figure 2. Steps in the agroecological transition of large-scale farming systems. (A) First step consists of regulating productivity to address short- and long-term goals. A new y-axis (S) is added to the model of Figure 1 that represents desirable shifts in terms of local resource use (water, nutrients, carbon) and a proposed ‘sustainable’ productivity level (Psust) compatible with balanced, efficient water use while ensuring soil fertility maintenance (isust); costs are higher than economically optimal, but can be seen as investments in long term productivity. (B) Second step consists of a thorough agroecological redesign of the farming system to favor process- in detriment of input-intensification. A new model is proposed replacing input intensity (ii) by process intensity (ip) in the x-axis; optimal productivity is closer to maximum productivity, which allows a reasonably gross margin (GM); and further intensification beyond iopt does not necessarily result in greater margins but in building of natural capital (i.e., long-term productivity) and greater provision of ecosystem services. (C) Combining input and process intensification using principles of agroecology to foster ecosystem services. Continuous lines indicate income and costs under current baseline (cf. Figure 1A) whereas dotted lines include the addition of ecosystem services to incomes and costs.
Figure 2B illustrates a theoretical, full transition to agroecology of large-scale farms, in which the productivity increases as a result of “process” intensification (ip), extending the notion of intensification to include the “intellectual” inputs that characterize agroecology, such as managing landscape complexity, diversity, synergies, natural regulation, and ecosystem services. Under process intensification, it is assumed that production costs do not grow linearly, as in the case of input-intensification, but they reach a plateau as the relative importance of processes in the system outgrows that of the minimum inputs. Optimal productivity would be closer to maximum productivity (NB: in a real agroecological system, productivity is measured for all different activities on the farm simultaneously, not just a single crop species, or a single activity such as annual cropping), providing a reasonable economic margin (GM) with low risk, and ideally with no financial debts. Intensifying beyond this point may not result in much higher overall productivity but in the long-term building of the natural capital of the system, in the form of fertile (often regenerated) soils, balanced water flows, greater biodiversity and positive biological interactions, quality habitats for wildlife species, carbon sequestration, etc. In other words, in a more efficient provision of ecosystem services of local and global importance, that may even result in lower production costs for farmers and/or in alternative sources of income in some cases, through e.g., financial incentives for nature conservation, agro-tourism, etc.
In reality, however, a limited number of large-scale farmers are or would eventually be able to fully transition to agroecology relying exclusively on process technologies. A most plausible scenario, represented in Figure 2C, is one in which large scale farmers would use agroecological principles for a combined input and process intensification (ii+p) of their system, making efficient use of ecosystem services to increase incomes and reduce costs. Under this model, there is a limit to intensification (imax) beyond which economic productivity, measured as gross income, starts decreasing due to resource degradation, excessive pesticide inputs, loss of natural enemies and pollinators, resistance to pesticides or antibiotics, or other pollutants.
We hypothesize that agroecology can provide the tools and knowledge to make efficient use of the natural functionalities that ecosystems offer, so that the reliance on external inputs of non-renewable resources or toxic molecules can gradually decrease along a so-called ecological intensification trajectory (cf. Tittonell, 2014). Although several factors preventing the transition to agroecology among large scale farmers are political, commercial or even cultural, we still consider that current agricultural research, from breeding to agronomy, ecology or social sciences, has a number of urgent calls in order to inform, sustain, and promote transitions. In the following section, we highlight selected areas of knowledge that are currently providing, but also require more, innovation to inform such transitions. They form the basis of the research agenda for agroecology in large scale farming that we propose toward the end of this manuscript.
Promising Avenues to Enhance Agroecological Transitions
Multifunctional Service Crops
Traditional, pre-twentieth century agricultural practices were largely based on growing multiple plant species, several of them not to be harvested. Cover crops, green manures, trap crops, green bridges, and a variety of other multipurpose species are able to provide regulation and support ecosystem services, which are important locally (ecosystem services that affect agricultural production), regionally (ecosystem services that are provided by rural landscapes), or globally (ecosystem services that benefit humanity). These associated, non-harvested species or service crops represent a valuable—necessary but not sufficient—tool within agro-ecologically managed systems.
The benefits of service crops in terms of the provision of ecosystem services is well-known and recognized nowadays in the agricultural research community (Schipanski et al., 2014; Pinto et al., 2017; Garcia et al., 2018), but less so among large scale farmers. Agricultural census data in the Americas show that service crops were adopted in <5% of the total agricultural land (e.g., 3.9% in the US in 2017: Zulauf and Brown, 2019). Data from Europe indicate that cover crops, plant residues and multi-annual plants occupy shares of 8, 7, and 8% respectively in arable land during winter (EUROSTAT: online data code: EF_MP_SOIL, updated 03/08/2020). In Argentina, the area of cover crops is increasing rapidly from 4 to 5% until 2015 to 13% in the 2019/2020 season (Bolsa de Cereales, 2020), largely associated with conservation tillage practices. Among the reasons that farmers put forward for the limited adoption of service crops are the perceived extra costs associated with the practice, and the limited availability (and high price) of seeds of several species used as service crops in local markets. Yet there are also farmers that consider service crops as an investment, since they help to regenerate the structure and functioning of degraded agroecosystems, reduce external inputs costs, contribute to increasing agricultural productivity and long-term sustainability (Schipanski et al., 2014).
Most species and their cultivars used nowadays as service crops have been bred or improved usually for fodder production (Scholberg et al., 2010), and hence their plant architecture, growth habit, phenology or functional traits do not always respond to the objectives for which they are grown in agroecological schemes. To integrate service crops in agroecological design and management, breeding programs for multipurpose traits are urgently needed, taking into account desirable morphological attributes that can contribute to multiple objectives, such as deep rooting, high leaf to stem ratios (or the opposite according to needs), promiscuity in terms of symbiotic N fixation, drought resistance or high water use efficiency, short or long cycles, propensity for mycorrhizal infestations, attractiveness to pests and/or to natural enemies, competitive ability against weeds, allelopathy, etc. (Kell, 2011; Wayman et al., 2017). A major challenge in this regard is the potential tension between private and public, non-profit plant breeding programs, that shape interests and determine asymmetrical investments in breeding programs (Brummer et al., 2011). Programs should include breeding for in-field diversity, and participatory breeding programs where farmers take action in the selection of plans leading to rapid local developments and adoption (Weltzien and Christinck, 2017). In addition, there is need for further collaboration between plant breeders and ecologists to develop future cultivars that fulfill both food production and ecosystem services restoration and maintenance, which will require stable and long-term funding sources (Brummer et al., 2011).
Research is needed to functionally link plant traits with ecosystem service provision in the specific context of agroecological design and management, which implies that service crop and their mixtures should provide multiple functions simultaneously according to variable designs of the mixtures. In agroecological systems, service crops will be incorporated in heterogeneous space-time combinations that will affect the expression of different traits in the service plants, hence plasticity is important. Several research questions arise also from practice, for example:
- How to regulate cover/companion crops vigor in order to avoid competition with the main cash crops for space, water and nutrients, reduce air moisture to avoid plant diseases, especially in wetter climates;
- How to reduce soil water depletion by cover crops during winter in drier climates;
- How to improve cover crop establishment in the dry conditions of late summer;
- How to terminate (vigorous) cover crops, maintaining soil cover, while ensuring viable seeding and germination conditions for the main cash crop:
- Under which conditions are perennial service crops (e.g., grass-legume mixtures) and biomass transfers for mulching a viable alternative in ecological and economic terms;
- How can service crops periods be used to replenish soil nutrients, control weed seed banks, and/or regulate pest populations so as to benefit the main cash crops in the rotation;
- What extra specific traits are needed when service crops are incorporated in crop-livestock, agroforestry, or agro-silvo-pastoral systems?
These are just a few examples of questions that arise from practice. Next to them, there is also the major challenge of mechanizing these activities, several of which cannot be efficiently accomplished with the machinery currently available on the market.
Arthropod-Mediated Ecosystem Services
In large scale agriculture, larger field sizes are usually associated with landscape simplification, a main driver of the loss of arthropod species providing biological pest control or pollination services (Dainese et al., 2019; Martin et al., 2019). Garibaldi et al. (2016) presented consistent evidence that larger fields reduce arthropod-mediated ecosystem services. This global study quantified to what degree enhancing pollinator density and richness improved yields on 344 fields from 33 pollinator-dependent crop systems in small and large farms from Africa, Asia, and Latin America. Worldwide, the benefits of flower-visitor density to crop yield were greater for smaller than larger holdings, and when flower-visitor richness was higher. For fields of <2 hectares, it was found that yield gaps could be closed by a median of 24% through higher flower-visitor density. For larger fields, such benefits only occurred at high flower-visitor richness. Improving flower-visitor richness in large fields is a challenge that will require a combination of practices, the effectiveness of which is context dependent, including sowing flower strips and planting hedgerows, providing nesting resources, more targeted use of pesticides, and/or restoration of semi-natural and natural areas adjacent to crops (Garibaldi et al., 2014). A recent synthesis of 89 studies with 1,475 locations showed that pollinator and enemy richness directly supported ecosystem services in complementary ways to abundance and dominance (Dainese et al., 2019). This review shows that negative effects of landscape simplification on ecosystem services were importantly mediated by richness losses of service-providing organisms, with negative consequences for crop yields. Given the positive influence of edge density on arthropod communities providing biological pest control or pollination services (Martin et al., 2019). A possible way to counteract the negative effects of large fields is to design them in strips to increase edge density without compromising the amount of area effectively cropped. Promising experiences in this direction are increasingly being documented (e.g., Ditzler et al., 2020). When agroecological designs imply using field hedges as uncultivated habitat/feeding environments for beneficial organisms, the tradeoffs with potential agricultural productivity losses need to be weighed also against overall costs and risks at farm scale, in the short and the long terms.
Watershed Regulation and Soil Conservation
Agriculture and its multiple management practices influences the way in which water and its load of sediments, nutrients, salts and pollutants are routed through the landscape and the hydrological system. As a result, it affects the long-term performance of production (e.g., modulating soil erosion rates and rainfall infiltration—Basche and DeLonge, 2017) as well as critical ecosystem services such as water provision (e.g., amount, timing, quality) or the regulation of downstream aquatic systems (e.g., their hydrological regime or pollution levels). Some of the most promising interventions in large-scale agricultural systems regarding these impacts involve landscape designs in which croplands coexist with other fixed or rotating vegetation types including cultivated pastures, tree plantations, and natural vegetation. Key decisions in this regard include the fraction, grain, and landscape position in which these non-agricultural patches are located, in order to mitigate the most concerning degradation processes.
In small watersheds of the North American corn-belt, 10% area of prairie filter strips either at foot slopes or contour strips were sufficient to cut run-off and sediment transport by 30 and 95%, respectively, in the dominant simplified farming systems, improving water availability for crops, protecting soils and water quality, and providing many additional services (Helmers et al., 2012; Schulte et al., 2017). In the drier part of the flat plains of Argentina, relatively low intensity farming systems with long fallows and extremely simplified landscapes, experience gradual water table level raises that end in massive waterlogging and salinization (Jobbágy et al., 2008; Nosetto et al., 2012; Kuppel et al., 2015). Sustaining evapotranspiration from waterlogged areas in wet years with dedicated natural wetland areas, or through the inclusion of flood-tolerant service crops, and “rescuing” deeply recharged water in dry years with native forest patches or rotating deep rooted grasslands are viable options to regulate a deleterious hydrological shift that damages farmland, infrastructure, and settlements (Mercau et al., 2013; Nosetto et al., 2015; Giménez et al., 2016). In more intensified settings in Europe and North America, ditch networks can be designed (e.g., density, topology) and managed (e.g., dredging, weeding/mowing regime) in a way that simultaneously regulates waterlogging and provides water cleaning, hydrological regulation, and habitat protection services (Dollinger et al., 2015). Plot to whole farm level designs that include patches of non-agricultural vegetation should be accompanied by whole watershed initiatives that include an explicit design (width/length/vegetation type) of riparian corridors (Cole et al., 2020).
As explicit landscape design becomes embraced by farmers and land and water policy makers, many questions will emerge. From a biophysical standpoint, developing reliable functions that depict how ecosystem services (e.g., water flow magnitude and timing; or sediment, nutrient, and pollutant loads) respond to the fraction of different types of non-agricultural land covers that are included in the landscape, is an ambitious but critical target, that can be approached gradually through adaptive management strategies in which interventions are used as experiments and modeling frameworks are used to integrate knowledge (e.g., Groot et al., 2016). For this reason, explicit monitoring and comparison plans are crucial in large-scale farming systems shifting toward agroecological management. These strategies need to consider the episodic nature of many disservices such as massive erosion and sediment transport, which can be assessed sporadically.
Landscape level non-linearities are among the most difficult but also promising aspects of watershed management. For example, pure forests and pure cropland may display a hydrological balance that is not maintained when they coexist as a patchwork in the landscape. Understanding aerial, surface and subsurface lateral transport of energy, water, and nutrients in increasingly complex farming landscapes is still an open challenge. From a human standpoint, knowledge on the perception and values of farmers and settlers regarding different land covers and landscape arrangements is also critical to achieve sound agreements or at least steer negotiations. Where watersheds are larger than individual farms, understanding how neighbors distribute the costs and benefits of agroecological landscape designs is a necessary step to develop sustainable incentives and agreements. Finally, a “fractal” approach to landscape diversification, in which tools from the realms of precision agriculture (within plot), landscape design (across plots), and watershed management (whole landscape) are integrated, is a promising avenue for further research to support agroecological transitions in large scale farming.
Regenerative Graze-Based Livestock Systems
Transition to agroecology in graze-based livestock systems may provide a way to increase productivity and income without increasing the use of external inputs and risks, to restore the productivity of degraded or overgrazed grasslands, and at the same time enhancing the provision of ecosystem services. For example, simply organizing the herd and the grazing regime to increase forage allowance, forage height, and plant biomass leads to enhanced energy consumption per animal and forage production by native grassland species (Carvalho et al., 2011; Carriquiry et al., 2012; Do Carmo et al., 2016). Other agroecological strategies include matching grassland growth rates during the year with forage demands by different animal categories, multi-species grazing designs to stimulate complementarity, matching spatial heterogeneity to different quantity and quality of forage requirements, respecting reseeding, and maintaining a permanent soil cover, all of which have proven successful at both experimental and real farm conditions, doubling current productivity levels (e.g., Albicette et al., 2017; Do Carmo et al., 2018). These transitions require also animal breeding support, an agenda that has been highlighted for ruminants, pigs and poultry (Phocas et al., 2016). Yet, as in the case of cropping systems, two broadly distinct approaches are needed for the agroecological transition of livestock systems in currently extensive vs. intensive production systems and contexts.
Livestock production based on native grasslands as the main source of animal nutrition is an example of large-scale farming producing meat, wool, and leather with low inputs of chemical fertilizers, fossil energy, and pesticides (Picasso et al., 2014). This form of privately-owned livestock operation is often referred to as ranching, and it is more common in the Americas than in Europe. Large areas of tropical forest are being cleared for the establishment of pasture lands, particularly in Brazil's Amazon and Mato Grosso regions (Pinillos et al., 2020). Here, however, we refer to native grasslands, areas that have not been forests since the last glacial period. Such is the case of the Rio de la Plata grasslands, i.e., the grassland biome that covers south Brazil, North East Argentina and the whole of Uruguay are a hotspot for biodiversity (Bilenca and Miñarro, 2004; Overbeck et al., 2007), and provide ecosystems services such as carbon sequestration, moderating regional climate, preserving the soil from erosion and maintaining water quality. However, the degree of ecosystem service provision across space and time and the tradeoffs between these and animal or economic productivity need to be more rigorously quantified (Tittonell et al., 2016). The sustainability of these grazing systems is threatened by overgrazing, which results in poor economic results, deterioration of the natural grassland, increased erosion rates, reduction of the carbon stock and increase in greenhouse gas emissions per unit area and product (Altesor et al., 2005; Overbeck et al., 2007; Modernel et al., 2018). This process has also increased the vulnerability of these systems to drought events (Modernel et al., 2019). While measures for their ecological intensification do exist and prove technically viable, their wide adoption by farmers is still challenging, no less due to the aging of this farming population and the gradual disappearance of this traditional lifestyle. When ranching farmers retire, the land is often leased out to agricultural enterprises that grow export crops (Albicette et al., 2017).
In intensive livestock systems and environments, where animal stocking rates are higher as sustained by external inputs such as in Europe, animal health and welfare considerations are central, as these affect production and product quality. Dumont et al. (2013) summarized key principles for the transition to agroecology in such animal production systems:
(i) Management practices that improve animal health;
(ii) Decrease the amount of inputs needed for production;
(iii) Optimize the metabolic functioning of farming systems;
(iv) Enhancing diversity to strengthen resilience;
(v) Preserving biological diversity in agroecosystems.
Even in intensively managed sown pastures, that are normally simple grass-clover mixtures, plant species diversification (forbs) can greatly improve efficiency of nutrient use by plants and grazing animals, soil quality and biological activity (de Haas et al., 2019; Hoogsteen et al., 2020). Yet many of the benefits of plant diversity are only evident in the long term (e.g., Cardinale et al., 2007), which is not always compatible with the current management of short-lived (3 years) pastures within intensive rotations. As in the case of extensive livestock on native grasslands, regenerative management of intensive grasslands includes their species diversification, water table/runoff management, balanced combination of direct grazing and mechanical mowing during the year, sometimes mechanical interventions to loosen compacted soil and/or inter-sow desired grassland species, inclusion of trees and fodder shrubs (also relevant to improve animal health), nutrient restitution (preferably together with organic matter through solid manure), watershed protection, increased permanent soil cover, natural re-seeding enclosures, multi-species grazing, selection of animal breeds adapted to agroecological management, etc. Yet, an agroecological transition cannot be described as a blueprint recipe or a set of predefined steps to be followed. Farmers are diverse not only in their resource endowment, socio-economic and bio-physical environments but also in their ability to experiment, modify, and adapt technologies to their conditions.
Nature Inclusive Agricultural Landscapes
The trade-off between increased productivity through increased inputs vs. declining ecosystem services and natural capital summarizes a complex underlying process of changing species-environment relations. Different species groups are differentially sensitive to agricultural intensification; some plants, invertebrates, and bird species can still find a (temporal) habitat in large-scale agriculture, while others cannot. These differences reflect underlying niche differences between species in their relations with environmental factors modified by agriculture, such as nutrient availability, soil pH, hydrology, and disturbance regimes. For example, plants or insects that have natural habitats with stable, low nutrient inputs as temperate peat bogs, and tropical upland forests, will be much more sensitive to agricultural intensification than species that evolved under more nutrient rich and dynamic conditions, such as riverine floodplains and shores. In such habitats, large-scale agricultural intensification can lead to complete species replacement of the local species pool, in many cases by invasive species from other continents (Mack et al., 2000).
Higher nutrient inputs generally lead to less coexistence opportunities for different plant species, as competition shifts from several belowground resources to mostly for light, often with a single-best competitor (Tilman, 1988). Reductions of inputs will therefore not only add species to the local species pool, but also cause turnover of species that are better adapted to the new conditions. Less diversity of plants is generally associated with less diversity of invertebrates due to less resource diversity for specialized species, and can lead to a decline of soil biodiversity and associated ecosystem services as soil fertility. Various recent studies show a strong decline of both insect biomass and insect diversity with a potential cause in agricultural intensification (Hallmann et al., 2017), as in increased inputs of nutrients and pesticides.
For birds that are mobile, large-scale agriculture can serve a key role during parts of their life cycle, such as a foraging ground during migration or as a breeding ground. But also for birds, agricultural intensification generally leads to a decrease in bird diversity, and their associated benefits as seed dispersal and pest control (Hendershot et al., 2020). Including agroecological principles in large-scale agriculture can potentially retain such benefits (Holland et al., 2016). In Northwestern Europe, meadow birds—associated with mostly dairy farming grasslands—have strongly declined, likely due to declining food availability for their mostly precocious young (independently foraging directly after hatching). While low-input flower-rich grasslands in spring provided a diversity of food for such species, high-input grasslands dominated by only a few productive grasses do not offer these opportunities anymore, where the combination of reduced food, increased disturbance frequency and enhanced predation due to changing food web structure are likely fatal (Kleijn et al., 2004; Kentie et al., 2016). Additionally, frequent mowing and often early in spring destroys the bird nesting habitats and offspring, resulting in severe population declines (Gill et al., 2007).
But too strong declines in nutrient availability is also not beneficial for such species as this leads to decreasing soil fauna as earthworms, an important food source; these species typically profit from low-input agricultural practices that may not be economically feasible anymore by generating too little net revenue. Efforts to generate new revenue models for combining payments for meadow bird protection with dairy farming (so payment for ecosystem services, see below) have until now show little success, as this species group is still in very strong decline (Howison et al., 2018).
Even large scale, high input agriculture can form a breeding habitat for some species of birds, even for highly endangered species such as Montagu's harrier or the short-eared owl (Koks et al., 2007). Such species can profit from the relative safety from predators of very large, uniform agricultural fields, given that suitable foraging habitat to feed the young is nearby. Especially when combined with active nest protection by volunteer groups, this can lead to the surprising return of nearly-extinct endangered raptors (Schlaich et al., 2015).
In summary, different species groups respond differently to the primary axis of intensification depicted in Figure 1, with generally a loss of diversity but also opportunities for particular endangered groups at different levels of agricultural inputs that still can be economically feasible. Protecting such species requires good knowledge of the life history and niche dimensions of these different species, and specific interventions aimed at protection of nests (delayed mowing, nest caging) have been deployed. However, this has not stabilized populations of these species until now, calling for alternative strategies for agroecological farming that is still compatible with preservation of some components of the biodiversity characteristics of historic agricultural landscapes. Examples do exist of nature-inclusive, yet high output farming in western Europe, such as in the intensive Dutch dairy sector on the Flevopolder (cf. Figure 3), combining diverse grasslands and vegetated waterways, solid manure applications, self-production of fodder and grain crops and adapted local breeds (Blaarkop × Holstein) of animals that live up to 15 years on the farm, with milk productivity levels in the order or 8 t animal−1 year−1. Such examples are common in practice but unfortunately still seldomly documented in the scientific literature, and hence the actual tradeoffs between their economic viability and the other ecosystem services they provide remains an important research question to explore further.
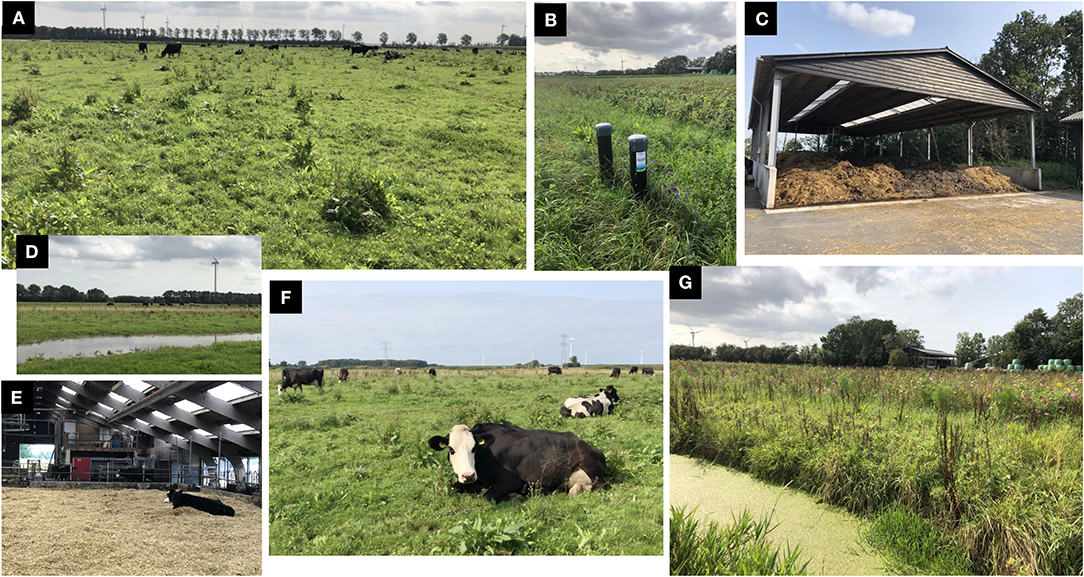
Figure 3. Images of a nature-inclusive farm in the intensive polder farming area of Flevoland, in The Netherlands. (A) Highly diversified grasslands, combining grasses, legumes and forbs and grazed directly; (B) Monitoring and intensive management of water table levels throughout the year to create spatial heterogeneity; (C) Storing and composting of solid manure; (D) small water bodies necessary for insect and bird diversity, creating better conditions also for animals; (E) Stable with deep bedding system (straw), free gates in and out and free access to a milking robot (in red, at the back); (F) Adapted, on farm bred herd combining Blaarkop (local breed) and Holstein lines, merging productivity, longevity and rusticity; (G) Interconnected biodiversity corridors throughout the farm. Pictures taken in September 2020 by P. Tittonell.
Policies and Financial Mechanism to Foster Agroecological Transitions
Several policies have been developed and implemented in the contexts of both heavily taxed to highly subsidized agricultural systems. The effect of such policies in terms of promoting a transition to agroecological farming has been variable, but generally weak (Figure 4). In heavily taxed contexts, such as in the flatland regions of South America, tax reduction per se will not result in a transition to agroecology but probably the opposite (e.g., tax reduction on fertilizers), unless tax cuts are tied to the provision of specific ecosystem services or to agroecological transitions. Incentives for good agricultural practices may result in less and more efficient use of external inputs, but is not enough to promote a thorough transition to agroecology. Access to soft credits to finance agroecological transitions may have positive impacts, although their effectiveness will also depend on farmer accountability (i.e., to what extent are credit beneficiaries using the credit to finance agroecological transitions), which is more easily ensured in situations with strong institutions and where farmers are used to reporting their activities to the government every year. Government financed advice and support on agroecology, on the other hand, may have a greater effect in heavily taxed contexts, where agroecology is seen also as an opportunity to reduce production costs.
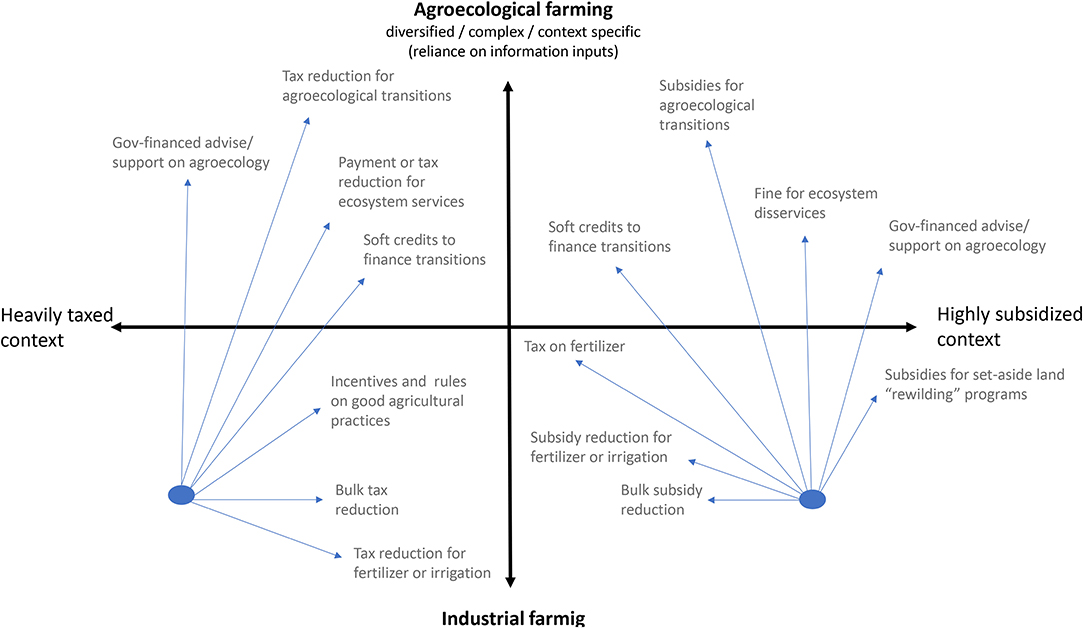
Figure 4. Possible net effects of several policy and financial mechanisms on the likelihood of large-scale farming systems to transition to agroecological farming from their current industrial farming practices. Blue circles indicate the approximate position of average farming systems nowadays, in both heavily taxed and highly subsidized contexts and blue arrows their expected trajectories toward agroecological farming.
In the context of highly subsidized agriculture, subsidies for set aside or rewilding programs (which are, in a way, also a form of payment for ecosystem services) have had a timid effect on promoting more sustainable agricultural practices (Batáry et al., 2015). Government financed support and advice on agroecological transitions are likely to have a limited effect as well (Figure 4), since there is no real incentive for the transition under the current policy, regulatory and market environments (e.g., Stassart et al., 2018). Fines and other forms of disincentives for ecosystem dis-services, such as water or air pollution, may be a mechanism to promote more agroecological practices, provided that they are applied in combination with the development of alternative practices and technologies available to farmers (e.g., Deverre and de Sainte-Marie, 2008; Elzen et al., 2017; Ministére de l'Agriculture and de l'Agroalimentaire et de la Forét, 2017). Perhaps the most daring hypothesis proposed in Figure 4 is that, in a context of heavily subsidized agriculture, an overall reduction of subsidies and the selective subsidy of agroecological practices and redesigns will have the greatest effect at fostering agroecological transitions.
Discussion
A research agenda to support the transition to agroecology among large scale farmers should contemplate societal goals beyond economic productivity. There is an increasing awareness that agricultural land benefits to society are not just about food production (IPBES, 2019). However, given the pressing financial situation in which most large-scale farmers operate, and the fact that economic considerations often come up as a top priority in discussions with farmers, policy makers, and other sectoral actors, we took economic productivity as the entry point for our reflection on intensification pathways (cf. Figures 1, 2). Also due to the increasing awareness among these actors that agroecology is able to reduce production costs and risks, while generating additional benefits to society as a whole (Wezel et al., 2018; WWF, 2018; Anderson et al., 2019). Our agenda goes however beyond simplified production functions as those used in Figures 1, 2, and considers five domains in which research is most urgently needed (Figure 5). Specific innovation pathways can be then identified within these major domains, which correspond to different levels of integration and spatio-temporal scales.
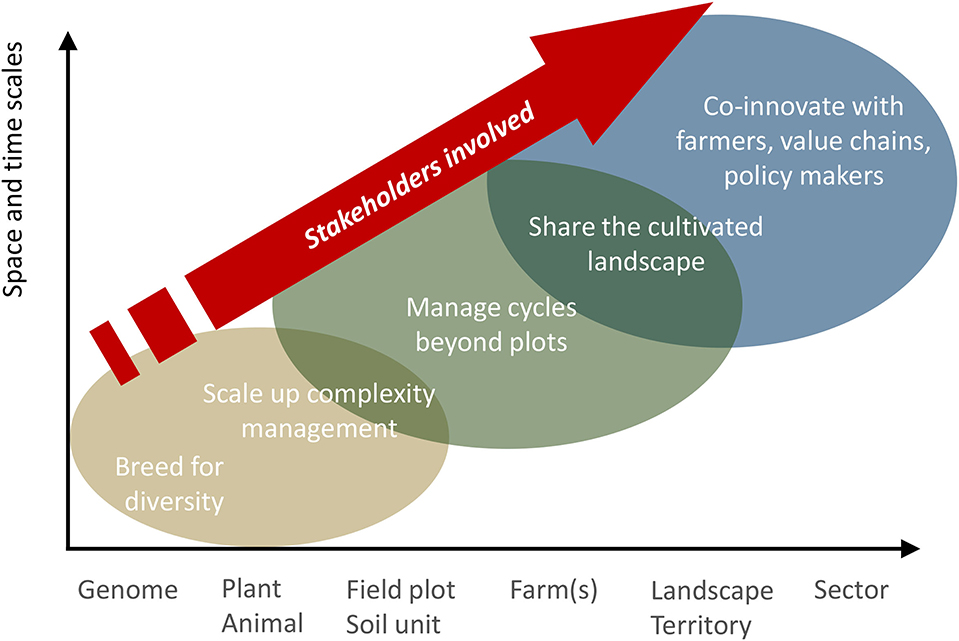
Figure 5. Five major domains defining a research agenda to support agroecological transitions at different levels of integration. At higher levels of integration from genome to sector the number and complexity of stakeholders involved in the process increase.
From an agronomic perspective (domains I and II in Figure 5), research to support agroecological transitions should focus on increasing the diversity of available—and economically viable—production crops or animal breeds, leading to more options to farmers for diversification. This has been indicated as breeding for diversity in Figure 5 but it entails also the smart use of existing genetic resources, such as native grass species as forage, natural fallows, locally adapted ancient cultivars as service plants, creole breeds of domestic animals and their backcrossings in marginal or low input environments, etc. Over the last decades, however, a large divergence of yield gains is seen in mainstream crops like dented maize vs. flint maize or sorghum that are left behind (Parra et al., 2020), not to mention non-soy legumes (Shunmugam et al., 2018). Breeding for diversity implies then including more crop species in the portfolio of the strongest breeding institutions (both public and private), breed more effective service crops adapted to local environments, and develop community breeding strategies in which multiple species are bred together (i.e., symbionts + plants, consociated crops; Denison, 2015).
When considering available knowledge and technologies currently at farmers' reach, service crops appear as one of the preferred tools because they are relatively easy to implement without a thorough structural reconfiguration of the farming system. Their benefits and the knowledge available on their management have already been highlighted, as have also the needs for breeding strategies aiming at increasing their adaptability and performance (cf. section Multifunctional Service Crops). But including service plants or multiple cropping designs such as strip- or intercropping requires new technologies, especially in terms of mechanization, able to cope with the greater complexity inherent to multi-species systems at higher scales.
A key pathway to support the transition to agroecology in large scale farming is the management of carbon, nutrient, and water cycles beyond the scale of the agricultural plot (Figure 5). This is nowadays referred to as circular agriculture (Hoes et al., 2018), or the integration of crop and livestock systems through biomass transfers at different scales, from single farms to landscapes and regions. The differences between circular agriculture, and integration of crop-livestock systems at farm scale proposed in agroecological systems (e.g., Botreau et al., 2014), are hard to see. Whenever possible, integrating crops and livestock within the same production systems has a number of advantages as it reduces the need for transport and/or processing of bulky biomass, facilitates the rotation between annual crops and pastures over time, opens opportunities for the economic utilization of multi-purpose service plants, incentivizing their adoption by farmers, promotes nutrient recycling within the farm and a diversification of land uses, with more opportunities for ecosystem service provision at landscape level, especially when wetland or forest patches are used as grazing units. The latter point takes us to the next domain in our research agenda, the identification of strategies and spatio-temporal arrangements to share the agricultural landscape with nature.
The interspacing of non-agricultural patches that are co-beneficial in terms of supporting production (e.g., pollinators, natural enemies, flow regulation, etc.) and multiple other ecosystem services can render agriculture more stable, less risky, and less dependent on external inputs (e.g., MacFadyen et al., 2012; Kristensen, 2016; Modernel et al., 2016; Douglas and Landis, 2017; Geneletti et al., 2018; Maldonado et al., 2019).
The last domain of research we see as fundamental to support agroecological transitions is the co-innovation with farmers, value chains and policy makers to develop new technologies, markets and policy environments that are more conducive for alternative farming. While co-innovation between farmers and researchers has seen much progress in the last decade (e.g., Lacombe et al., 2018), organizational innovations with higher scale actors have been less common (Duru et al., 2015). This domain may develop in parallel with a redirection of taxes and subsidies to stimulate all the above interventions. The level of complexity of the system changes required in agroecological transitions may be also a constraint for some farmers to be able to select and adapt the technologies just by themselves. Collective action, sharing, and co-innovation is often needed.
In innovation systems thinking, innovation is seen as an interactive, non-linear process resulting from the interaction between heterogeneous actors and emerging technological and institutional change (Fagerberg, 2009). Formal agronomic research is an important part of this interactive picture (Sumberg et al., 2012). In the context of innovation platforms (cf. learning centers, e.g., Tittonell et al., 2012) researchers, resource users such as farmers or herders, and other stakeholders come together not just to share their previous knowledge, but to design, experiment, discuss results, make decisions, engage in debate, raise new questions, etc., in a way that allows them to learn together and co-construct new knowledge. The new, emerging mental frames form the basis for more creative and collective solutions. We refer to this collective process as co-innovation or co-design (Dogliotti et al., 2014). Involvement of farmers, extension agents and researchers is essential to develop innovative agroecological systems as well as and innovative advice tools and decision support methods to foster shared learning (e.g., Luedeling and Shepherd, 2016).
Although not explicitly outlined in the rather agronomy-focused research agenda presented here, a number of research questions emerge on the most effective ways to design and organize participation, learning and communication within innovation platforms across different socio-cultural settings and production systems (e.g., Berthet et al., 2018). Context-specific system innovation tools may also be necessary to anchor local innovations with the potential to deliver global scale solutions, as a pre-requisite to their out-scaling (Tittonell et al., 2016). A long-lasting example of bottom-up innovation platforms in the context of large-scale agriculture are the CREA farmer groups in Argentina and Uruguay (CREA: Regional Consortia for Agricultural Experimentation). Emerged in 1957 as a spontaneous organization of a group of farmers (https://www.crea.org.ar/historia) to experiment and learn together, they soon disseminated the idea to other regions and created a national association of regional consortia. Today, they count some 2,000 private farmers organized in 208 regional co-innovation groups that support knowledge development, testing and evaluation of technologies and practices, relying on strong links with the academia. Their success and long-standing experience have been often the object of research studies from the domain of social science, knowledge systems and organizations (e.g., Peirano, 2010; Pacín and Oesterheld, 2014). Although the activities of the CREA groups have been to date far from promoting any form of agroecological transitions in large scale farming, their experience, their horizontal, and context-specific nature, plus their demonstrated adaptive capacity over time make them a potentially interesting platform to launch a wide agroecological transition program in large scale farming.
Down to Earth
Agroecology is defined by principles, not by a set of practices (Tittonell, 2020). This is why it becomes so difficult to decide to what extent any farming system is an agroecological system, or at which point in the transition it sits, or what set of practices needs to be implemented for a system to become agroecological, etc. (FAO, 2019). These are common questions among farmers and agronomists, but also among scientists and policy makers who often wonder what options are readily available to engage in a transition. Far from being universally applicable, specific practical recommendations for the transition to agroecology should be designed considering the type of farming system, its context, and the starting point in the transition. This is illustrated in Table 1 for arable agriculture, particularly large-scale grain production (cereal, pulses, and oil seed crops) in the two contrasting contexts of governmental interventions described earlier, taxes, and subsidies. Management measures for the transition to agroecology in these cases are largely identified in response to the currently most pressing problems associated with industrial agriculture in each context. The list is not exhaustive, and excludes more transformative measures such as integrating livestock in the system, switching from tillage to conservation agriculture, or integrating trees as part of an agroforestry strategy. Such transformative measures would require a thorough redesign of the agricultural system, even re-training farmers and advisors, in parallel with a redesign of associated value chains and sectoral policies (cf. the co-innovation domain in Figure 5). Let us not dream too wildly: in most industrial agricultural settings a transition to agroecology, if any, is likely to be gradual, partial, and generally slow.
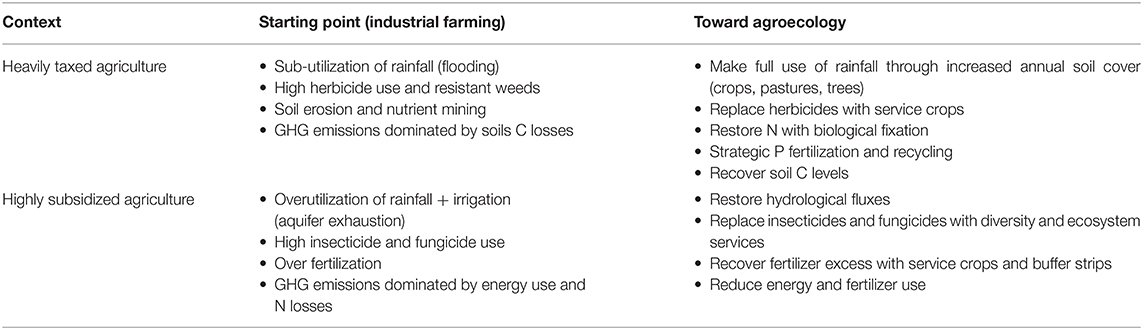
Table 1. An illustration of possible measures to transition from current industrial to agroecological intensification in arable agriculture (grain production).
From the general measures illustrated for arable farming in Table 1, a number of finer-grain agronomic and ecological questions remain to be addressed to support agroecology. For example, through ad-hoc consultation with farmers, fellow researches, and field agronomists we identified at least 20 fine-grain research questions that we present here:
1. How do above- and below-ground species interact to provide multiple ecosystem services?
2. How many supportive and regulating species are needed and which ones?
3. Is it possible to improve not only yields but services as well through breeding and selection?
4. Under what circumstances does biodiversity improve crop yield, crop quality and yield/quality stability?
5. To what degree can ecosystem services replace, complement, or interact synergistically with agricultural inputs to achieve resilient and productive farming?
6. What are the impacts of the reduction of feeding resources for beneficial organisms resulting from herbicide use?
7. What are the impacts on pests and beneficial arthropods of long-term exposures to sub-lethal concentrations of different agro-chemicals?
8. How much area of natural or semi-natural habitat is needed within a farm or a landscape?
9. How should these areas be distributed across the landscape? What is the minimum area required?
10. Which variables should be measured for habitat quality?
11. How much soil organic matter is needed in different cropping systems?
12. What fractions of soil organic matter are strategic to protect or restore in each case?
13. Can DNA-extraction based methods for soil biodiversity identification be used as decision support indicators in soil management?
14. How does plant diversity affect water cycles?
15. What mix of annual crops and perennials is needed?
16. How can local practices complement or interact synergistically when integrated into landscape design?
17. What is the potential productivity boundary of agroecological livestock system with different levels of external inputs?
18. How can ecosystem services and other externalities of farming systems be effectively incorporated into decision making?
19. How effective are different approaches to training and skills development in delivering agroecology?
20. How best to quantify tradeoffs between economic viability, biodiversity conservation and ecosystem service provision in agricultural landscapes?
Intensive agriculture is not only affecting biodiversity within its own fields itself, but also in neighboring nature areas, e.g., through nitrogen emissions, pesticide losses, lowering groundwater tables for agriculture (e.g., Hallmann et al., 2017). In the Netherlands, for example, export-oriented horticulture, agriculture, and livestock systems form an important land claim: 0.7% of all households in the country are farmers, but together they use 54% of the land surface (2019). The mounting evidence on such impacts are a strong argument against land sparing approaches for nature conservation. More insight is needed on the relation between intensive agriculture and neighboring nature areas, particularly in the context of Western Europe where both land uses coexist within single landscapes. At which scale can these be in a mosaic? What is the scale of the checkerboard necessary for biodiversity in nature areas to be spared from the impact of intensive farming and still survive? Also, if we were to include a third curve in Figure 1 to represent natural capital or ecosystem services, we would likely draw an exponential decline indicating loss of biodiversity as input intensification increases. A classical tradeoff. However, we think there is room for smart agroecological interventions that would render the relationship between profit and nature synergistic, allowing for high economic productivity and biodiversity conservation (e.g., Modernel et al., 2018; El Mujtar et al., 2019; Pinillos et al., 2020). In this sense, agroecology supports land sharing approaches to conservation, due to the co-benefits that can be expected from greater biodiversity and ecosystem services.
Yet addressing each one of these questions without embedding them into broader strategies that are context-aware (e.g., Table 1) and integrative across scales and levels of organization (e.g., Figure 5) would be of little use. In this regard, rooting convergent avenues of agroecological research and innovation at the core of each large-scale farming belt of the world is critical, since knowledge gains from one region are unlikely to get easily transferred to another as it may happen with many industrial agriculture technologies. Also critical is the development of knowledge co-production schemes that are based from their early stage on real life large-size farms. Otherwise, unrealistic promises derived from “boutique” demonstration plots may create more frustration than transformation. Agroforestry initiatives offer a reminder in this sense, with many ecological benefits well-documented in experimental settings for decades, but management systems and business models for large-scale, commercial implementation being still rare (but see Duru et al., 2015).
Beyond Profits
Although the models presented in Figures 1, 2 to illustrate intensification pathways assume that large scale farmers are generally gross income maximisers, this is obviously a simplification, as in reality farmers tend to also maximize gross margins and profits. However, economic profit is not the main driver of technology choice among large scale farmers. Socio-cultural factors, such as being perceived as modern farmers that attain high productivity per unit land or animal, or use the latest technologies available is also an important motivation to select intensification means and levels (Dessart et al., 2019). Particularly in highly subsidized systems, farmers tend to pursue the highest possible productivity levels fueled also by the processing sector and by an aggressive commercial push by input and technology suppliers (Levidow et al., 2014).
A similar picture can be seen in heavily taxed contexts, although the economic failure or risk of high input intensification schemes is often attributed, by farmers and other sectoral actors, to the tax burden (OECD, 2019). A comparison between Argentina and Uruguay, however, may indicate otherwise. While the agrarian structure, land use and agricultural practices are quite similar in both countries, public policies with regards to agriculture differ broadly. Tax policies impose a much heavier burden on Argentinian farmers (export tax of 30% for soybean), and although many claim that the economic failure of high input agriculture is due to the government tax policy, the experience in Uruguay where the taxes are much lower shows exactly the same pattern of economic risks, failure and consequent land concentration associated with the expansion of large scale agriculture.
A controversial point about agroecological transitions is their possible impact on labor requirements, especially when several practices for landscape restoration or agroecological management are not yet mechanizable to date. Progress in the fields of robotics, big data, sophisticated precision agriculture or automation is fast and promising, and represents an opportunity when combined with the other sources of knowledge mobilized in agroecology (HLPE, 2019). Labor is still seen as a high cost by farmers, and it may be even seen as rather prohibitive in certain contexts and production systems such as those of Western Europe. Current labor requirements in agriculture range widely across the world, from e.g., 0.004 h Tn−1 grain in Argentina, to 0.010 h Tn−1 in France or 0.060 h Tn−1 in Brazil. Yet unemployment is a major concern affecting livelihoods all over the world. Biodiversity-intensive landscapes can provide more employment in a real economy. A global study using country-level agricultural and socio-economic data showed that countries where crop diversity increased also supported more agricultural jobs (Garibaldi and Pérez-Méndez, 2019). Such effects were independent of differences among countries in the size of the agricultural sector, fertilizer use, crop yields, socio-economic development or economic growth. Moreover, the study found no evidence that the jobs lost in the rural areas were incorporated into other sectors of the economy. Thus, there is evidence for a positive link between two of the United Nations Sustainable Development Goals (SDGs), in the sense that enhancing crop diversity (SDG 2) can also contribute to improving employment rates (SDG 8).
Transforming this labor demand into attractive jobs and livelihoods in large-scale farming contexts will still remain as a challenge that calls for integrating agroecological innovation and social programs. Here again, co-innovation in policies, incentives and regulations are urgently needed to make this shift both viable and attractive to large scale farmers and society as a whole.
Conclusions
A broad transition to agroecology in large-scale farming across the diverse set of contexts in which it operates will generate returns on four capitals (www.commonland.com): on social capital, by creating jobs, education, business, and security; on natural capital, by restoring biodiversity, soil, water quality and carbon; on financial capital, by realizing long-term sustainable profit: and on inspiration (being a sort of emotional or psychological capital) by giving hope and a sense of purpose to people. Biodiverse farming, as proposed through agroecology, can create more jobs in rural areas, restore biodiversity and ecosystem services, and renew inspiration among large-scale farmers, reduce risks of economic failure in farm business models and provide long term stability (NB: the authors have not yet encounter cases in their practice in which farmers who transitioned to agroecology went bankrupt or failed economically; the opposite was often observed: bankrupt or fatigued industrial farmers that moved toward agroecology).
But knowledge gaps remain, at both practical and theoretical levels, to inform real life strategies for the transition of large-scale farming to agroecology. We identified five domains of research that need to be prioritized to foster this agenda:
- Breeding for diversity;
- Scaling up complexity management;
- Managing cycles beyond fields and farms;
- Sharing the cultivated landscape;
- Co-innovating with farmers, value chains and policy makers.
The figures and models used in this paper to describe the current situations of large-scale farming present unidirectional developments. In reality, however, large scale farming landscapes are shaped by several simultaneous drivers—topography being not a minor one—that result in rather homogeneous but not identical situations on each single farm or landscape. We argue that optimum economic productivity does not necessarily occur at the balanced resource use level of intensification, as it may be higher or lower depending on the context. Yet we do know that subsidies will push input intensification and overuse of local resources, while taxes tend to have the opposite effect. Thus, at some point, these instruments may contribute to tune optimum resource use, albeit in a poorly targeted way. Incentives that operate more directly on critical inputs and impacts would be more desirable. For example, by redirecting agricultural tax revenues to restoring soil phosphorus in South America, or through subsidies applied specifically to farmers that cut fertilization or irrigation inputs in Western Europe. A step further would be to tune taxes or subsidies based on the specific incorporation of agroecology's high “intellectual” inputs in the form of landscape complexity management, crop diversity, co-innovation, etc. Yet we see the tuning of taxes and subsidies as a necessary but not sufficient condition to promote transitions to agroecology, which will also require the engagement of other actors along a value chain, including consumers.
Large scale farmers are not necessarily happy in their current situation. They are seen as responsible for environmental degradation while often complaining that they have to spend more time filling in papers than running around on their tractors or in the field with their animals. No doubt that under such circumstances, in both taxed and subsidized agricultural contexts, the average age of large-scale farmers is reaching 60 years old. We hope agroecology can set the scene for a renewed impetus in large scale farming, to attract the youth and its innovative capacity, to foster clean technological innovation, and to promote a new generation of large-scale farmers that take pride in contributing to feeding the world while preserving the planet.
Data Availability Statement
The original contributions presented in the study are included in the article/supplementary material, further inquiries can be directed to the corresponding author/s.
Author Contributions
PT led the writing process. GP, LG, SD, HO, and EJ contributed text and discussed the different sections. All authors contributed to the article and approved the submitted version.
Conflict of Interest
The authors declare that the research was conducted in the absence of any commercial or financial relationships that could be construed as a potential conflict of interest.
The reviewer DB declared a shared affiliation with one of the authors, PT, to the handling editor at time of review.
Acknowledgments
PT wishes to thank the World Wildlife Fund for their financial support of his Chair on Resilient Landscapes for Nature and People at Groningen University.
References
Albicette, M. M., Leoni, C., Ruggia, A., Scarlato, S., Blumetto, O., Albín, A., et al. (2017). Co-innovation in family-farming livestock systems in Rocha, uruguay: a 3-year learning process. Outlook Agric. 46, 92–98. doi: 10.1177/0030727017707407
Altesor, A., Oesterheld, M., Leoni, E., Lezama, F., and Rodriguez, C. (2005). Effect of grazing n community structure and productivity of a uruguayan grassland. Plant Ecol. 179, 83–91. doi: 10.1007/s11258-004-5800-5
Anderson, C., Bruil, J., Chappell, M., Jahi, K., Csilla, P., and Michel, P. P. (2019). From transition to domains of transformation: getting to sustainable and just food systems through agroecology. Sustainability 11:5272. doi: 10.3390/su11195272
Basche, A., and DeLonge, M. (2017). The impact of continuous living cover on soil hydrologic properties: a meta-analysis. Soil Sci. Soc. Am. J. 81, 1179–1190. doi: 10.2136/sssaj2017.03.0077
Batáry, P., Dicks, L. V., Kleijn, D., and Sutherland, W. J. (2015). The role of agri-environment schemes in conservation and environmental management. Conserv. Biol. 29, 1006–1016. doi: 10.1111/cobi.12536
Berthet, E. T., Hickey, G. M., Klerkx, L. (2018). Opening design and innovation processes in agriculture: insights from design and management sciences and future directions. Agric. Syst. 165, 111–115 doi: 10.1016/j.agsy.2018.06.004
Bilenca, D., and Miñarro, F. (2004). Identificación de Áreas Valiosas de Pastizal (AVPs) en las Pampas y Cam- pos de Argentina, Uruguay y sur de Brasil. Buenos Aires: Fundación Vida Silvestre Argentina, 320.
Botreau, R., Farruggia, A., Martin, B., Pomiès, D., and Dumont, B. (2014). Towards an agroecological assessment of dairy systems: proposal for a set of criteria suited to mountain farming. Animal 8, 1349–1360. doi: 10.1017/S1751731114000925
Brummer, E. C., Barber, W. T., Collier, S. M., Cox, T. S., Johnson, R., Murray, S. C., et al. (2011). Plant breeding for harmony between agriculture and the environment. Front. Ecol. Environ. 9, 561–568. doi: 10.1890/100225
Cardinale, B. J., Wright, J. P., Cadotte, M. W., Carroll, I. T., Hector, A., Srivastava, D. S., et al. (2007). Impacts of plant diversity on biomass production increase through time because of species complementarity. Proc. Natl. Acad. Sci. U.S.A. 104, 18123–18128 doi: 10.1073/pnas.0709069104
Carriquiry, M., Espasandin, A., Astessiano, A., Casal, A., Claramunt, M., Do Carmo, M., et al. (2012). La cría vacuna sobre campo nativo: Un enfoque de investigación jerárquico para mejorar su productividad y sostenibilidad. Vet. 48, 41–48.
Carvalho, P., Nabinger, C., Lemaire, G., Genro, T. (2011). “Challenges and opportunities for livestock production in natural pastures: the case of Brazilian Pampa Biome,” in Diverse Rangelands for a Sustainable Society, IX International Rangeland Congress, eds S. Feldman, G. Oliva, and M. Sacido (Rosario), IX–XV.
Cole, L. J., Stockan, J., and Helliwell, R. (2020). Managing riparian buffer strips to optimise ecosystem services: a review. Agric. Ecosyst. Environ. 296:106891. doi: 10.1016/j.agee.2020.106891
Dainese, M., Martin, E. A., Aizen, M. A., Albrecht, M., Bartomeus, I., Bommarco, R., et al. (2019). A global synthesis reveals biodiversity-mediated benefits for crop production. Sci. Adv. 5:eaaax0121. doi: 10.1126/sciadv.aax0121
de Haas, B. R., Hoekstra, N. J., van der Schoot, J. R., Visser, E. J. W., de Kroon, H., and van Eekeren, N. (2019). Combining agro-ecological functions in grass-clover mixtures. AIMS Agric. Food. 4, 547–567. doi: 10.3934/agrfood.2019.3.547
Denison, R. F. (2015). “Chapter 9: A darwinian perspective on improving nitrogen-fixation efficiency of legume crops and forages,” in Crop Physiology, 2nd edn, eds V. O. Sadras and D. F. Calderini (Amsterdam: Academic Press), 207–222. doi: 10.1016/B978-0-12-417104-6.00009-1
Dessart, F. J., Barreiro-Hurlé, J., and van Bavel, R. (2019). Behavioural factors affecting the adoption of sustainable farming practices: a policy-oriented review. Eur. Rev. Agric. Econ. 46, 417–471. doi: 10.1093/erae/jbz019
Deverre, C., and de Sainte-Marie, C. (2008). L'écologisation de la politique agricole européenne. Verdissement ou refondation des systèmes agro-alimentaires? Anglais 89, 83–104. doi: 10.22004/ag.econ.207798
Ditzler, L. van Apeldoorn, D. F., Schulte, R. P. O., Tittonell, P., and Rossing, W. A. H. (2020). Redefining the field to mobilize three-dimensional diversity and ecosystem services on the arable farm. Euro. J. Agron. 177:126197. doi: 10.1016/j.eja.2020.126197
Do Carmo, M., Cardozo, G. A., Jaurena, M. A., and Soca, P. M. (2018). Demonstrating control of forage allowance for beef cattle grazing Campos grassland in Uruguay to improve system productivity. Trop. Grasslands Forrajes Trop. 7:35–47. doi: 10.17138/tgft(7)35-47
Do Carmo, M., Claramunt, M., and Soca, P. (2016). Animal energetics in extensive grazing systems: rationality and results of research models to improve energy efficiency of beef cow-calf grazing Campos systems. J. Anim. Sci. 94, 84–92. doi: 10.2527/jas2016-0596
Dogliotti, S., García, M. C., Peluffo, S., Dieste, J. P., Pedemonte, A. J., Bacigalupe, G. F., et al. (2014). Co-innovation of family farm systems: a systems approach to sustainable agriculture. Agric. Syst. 126, 76–86. doi: 10.1016/j.agsy.2013.02.009
Dollinger, J., Dagès, C., Bailly, J., Lagacherie, P., and Voltz, M. (2015). Managing ditches for agroecological engineering of landscape. A review. Agron. Sustain. Dev. 35, 999–1020. doi: 10.1007/s13593-015-0301-6
Douglas, A., and Landis, D. A. (2017). Designing agricultural landscapes for biodiversity-based ecosystem services. Basic Appl. Ecol. 18, 1–12. doi: 10.1016/j.baae.2016.07.005
Dumont, B., Fortun-Lamothe, L., Jouven, M., Thomas, M., and Tichit, M. (2013). Prospects from agroecology and industrial ecology for animal production in the 21st century. Animal 7, 1028–1043. doi: 10.1017/S1751731112002418
Duru, M., Therond, O., and Fares, M. (2015). Designing agroecological transitions a review. Agron. Sustain. Dev. 35, 1237–1257. doi: 10.1007/s13593-015-0318-x
El Mujtar, N., Muñoz, B., Prack Mc Cormick, M., Pulleman, M., and Tittonell, P. (2019). Role and management of soil biodiversity for food security and nutrition; where do we stand? Global Food Sec. 20, 132–144. doi: 10.1016/j.gfs.2019.01.007
Elzen, B., Augustyn, A. M., Barbier, M., and van Mierlo, B. (2017). Agroecological Transitions: Changes and Breakthroughs in the Making. Wageningen: Wageningen University & Research. doi: 10.18174/407609
Fagerberg, J. (2009). “Innovation: a guide to the literature,” in The Oxford Handbook of Innovation, eds J. Fagerberg and D. C. Mowery. doi: 10.1093/oxfordhb/9780199286805.003.0001
FAO (2019). TAPE Tool for Agroecology Performance Evaluation 2019 - Process of Development and Guidelines for Application, Test version. Rome: FAO.
Garcia, L., Celette, F., Gary, C., Ripoche, A., Valdés-Gómez, H., and Metay, A. (2018). Management of service crops for the provision of ecosystem services in vineyards: a review. Agric. Ecosyst. Environ. 251, 158–170. doi: 10.1016/j.agee.2017.09.030
Garibaldi, L. A., Carvalheiro, L. G., Leonhardt, S. D., Aizen, M. A., Blaauw, B. R., Isaacs, R., et al. (2014). From research to action: enhancing crop yield through wild pollinators. Front. Ecol. Environ. 12, 439–447. doi: 10.1890/130330
Garibaldi, L. A., Carvalheiro, L. G., Vaissiere, B. E., Gemmill-Herren, B., Hipolito, J., Freitas, B. M., et al. (2016). Mutually beneficial pollinator diversity and crop yield outcomes in small and large farms. Science 351, 388–391. doi: 10.1126/science.aac7287
Garibaldi, L. A., and Pérez-Méndez, N. (2019). Positive outcomes between crop diversity and agricultural employment worldwide. Ecol. Econ. 164:106358. doi: 10.1016/j.ecolecon.2019.106358
Geneletti, D., Rocco, S., and Blal Adem, E. (2018). Assessing ecosystem services and biodiversity tradeoffs across agricultural landscapes in a mountain region. Int. J. Biodiv. Sci. Ecosyst. Serv. Manag. 14, 188–208. doi: 10.1080/21513732.2018.1526214
Gill, J. A., Langston, R. H. W., Alves, J. A., Atkinson, P. W., Bocher, P., Cidraes Vieira, N., et al. (2007). Contrasting trends in two black-tailed godwit populations: a review of causes and recommendations. Wader Study Group Bull. 114, 43–50.
Giménez, R., Mercau, J., Nosetto, M., Páez, R., and Jobbágy, E. (2016). The ecohydrological imprint of deforestation in the semiarid Chaco: insights from the last forest remnants of a highly cultivated landscape. Hydrol. Process. 30, 2603–2616. doi: 10.1002/hyp.10901
Graeub, B. E., Chappell, M. J., Wittman, H., Ledermann, S., Kerr, R. B., and Gemmill-Herren, B., (2016). The state of family farms in the world. World Dev. 87, 1–15. doi: 10.1016/j.worlddev.2015.05.012
Groot, J. C. J., Cortez-Arriola, J., Rossing, W. A. H., Massiotti, R. D. A., and Tittonell, P. (2016). Capturing agroecosystem vulnerability and resilience. Sustainability 8:1206. doi: 10.3390/su8111206
Hallmann, C. A., Sorg, M., Jongejans, E., Siepel, H., Hofland, N., Schwan, H., et al. (2017). More than 75 percent decline over 27 years in total flying insect biomass in protected areas. PLoS ONE 12:e0185809. doi: 10.1371/journal.pone.0185809
Helmers, M. J., Zhou, X., Asbjornsen, H., Kolka, R., Tomer, M. D., and Cruse, R. M. (2012). Sediment removal by prairie filter strips in row?cropped ephemeral watersheds. J. Environ. Qual. 41, 1531–1539. doi: 10.2134/jeq2011.0473
Hendershot, J. N., Smith, J. R., Anderson, C. B., Letten, A. D., Frishkoff, L. O., Zook, J. R., et al. (2020). Intensive farming drives long-term shifts in avian community composition. Nature 579, 393–396. doi: 10.1038/s41586-020-2090-6
HLPE (2019). “Agroecological and other innovative approaches for sustainable agriculture and food systems that enhance food security and nutrition,” in A Report by the High Level Panel of Experts (HLPE) on Food Security and Nutrition of the Committee on World Food Security, Rome.
Hoes, A.-C., Roel, J., van Berkum, S., and Poppe, K. (2018). Towards Sustainable Food Systems. A Dutch Approach. Wageningen: Wageningen University.
Holland, J. M., Bianchi, F. J., Entling, M. H., Moonen, A. C., Smith, B. M., and Jeanneret, P., (2016). Structure, function and management of semi-natural habitats for conservation biological control: a review of European studies. Pest Manag. Sci. 72, 1638–1651. doi: 10.1002/ps.4318
Hoogsteen, M. J. J., Bakker, E.-J., van Eekeren, N., Tittonell, P. A., Groot, J. C. J., van Ittersum, M. K., et al. (2020). Do grazing systems and species composition affect root biomass and soil organic matter dynamics in temperate grassland swards? Sustainability 12:1260. doi: 10.3390/su12031260
Howison, R. A., Piersma, T., Kentie, R., Hooijmeijer, J. C. E. W., and Olff, H. (2018). Quantifying landscape-level land-use intensity patterns through radar-based remote sensing. J. Appl. Ecol. 55, 1276–1287. doi: 10.1111/1365-2664.13077
IPBES (2019). Global Assessment Report on Biodiversity and Ecosystem Services of the Intergovernmental Science-Policy Platform on Biodiversity and Ecosystem Services.
Jobbágy, E. G., Nosetto, M. D., Santoni, C. S., and Baldi, G. (2008). El desafío ecohidrológico de las transiciones entre sistemas leñosos y herbáceos en la llanura chaco - pampeana. Asoc. Argentina Ecol. AsAE. Buenos Aires 18, 305–322.
Kell, D. B. (2011). Breeding crop plants with deep roots: their role in sustainable carbon, nutrient and water sequestration. Ann. Bot. 108, 407–418. doi: 10.1093/aob/mcr175
Kentie, R., Senner, N. R., Hooijmeijer, J. C. E. W., Márquez-Ferrando, R., Figuerola, J., Masero, J. A., et al. (2016). Estimating the size of the dutch breeding population of continental black-tailed godwits from 2007–2015 using resighting data from spring staging sites. Ardea 104, 213–225. doi: 10.5253/arde.v104i3.a7
Kleijn, D., Berendse, F., Smit, R., Gilissen, N., Smit, J., Brak, B., et al. (2004). Ecological effectiveness of agri-environment schemes in different agricultural landscapes in the Netherlands. Conserv. Biol. 18, 775–786. doi: 10.1111/j.1523-1739.2004.00550.x
Kleijn, D., Bommarco, R., Fijen, T. P. M., Garibaldi, L. A., Potts, S. G., and van der Putten, W. H. (2019). Ecological Intensification: bridging the gap between science and practice. Trends Ecol. Evol. 34, 154–166. doi: 10.1016/j.tree.2018.11.002
Koks, B. J., Trierweiler, C., Visser, E. G., Dijkstra, C., and Komdeur, J. (2007). Do voles make agricultural habitat attractive to Montagu's Harrier Circus pygargus? Ibis 149, 575–586.
Kristensen, S. B. P. (2016). Agriculture and landscape interaction—landowners' decision-making and drivers of land use change in rural Europe. Land Use Policy 57, 759–763. doi: 10.1016/j.landusepol.2016.05.025
Kuppel, S., Houspanossian, J., Nosetto, M. D., and Jobbagy, E. G. (2015). What does it take to flood the pampas? Lessons from a decade of strong hydrological fluctuations. Water Resour. Res. 51, 2937–2950. doi: 10.1002/2015WR016966
Lacombe, C., Couix, N., and Hazard, L. (2018). Designing agroecological farming systems with farmers: a review. Agric. Syst. 165, 208–220. doi: 10.1016/j.agsy.2018.06.014
Levidow, L., Pimbert, M., and Vanloqueren, G. (2014). Agroecological research: con- forming—or transforming the dominant agro-food regime? Agroecol. Sustain. Food Syst. 38, 1127–1155. doi: 10.1080/21683565.2014.951459
Loconto, A., Marion, D., Moreau, T., Couvet, D., and Dorin, B. (2020). The land sparing – land sharing controversy: tracing the politics of knowledge. Land Use Policy 96:103610. doi: 10.1016/j.landusepol.2018.09.014
Lowder, S. K., Sánchez, M. V., and Bertini, R. (2019). Farms, family farms, farmland distribution and farm labour: What do we know today? FAO Agricultural Development Economics Working Paper 19–08. Rome: FAO.
MacFadyen, S., Cunningham, S. A., Costamagna, A. C., and Schellhorn, N. A. (2012). Managing ecosystem services and biodiversity conservation in agricultural landscapes: are the solutions the same? J. Appl. Ecol. 49, 690–694 doi: 10.1111/j.1365-2664.2012.02132.x
Mack, R. N., Simberloff, D., Lonsdale, W. M., Evans, H., Clout, M., and Bazzaz, F. A. (2000). Biotic invasions: causes, epidemiology, global consequences, and control. Ecol. Appl. 10, 689–710. doi: 10.1890/1051-0761(2000)010[0689:BICEGC]2.0.CO;2
Maldonado, A. D., Ramos-López, D., Aguilera, P. A. (2019). The role of cultural landscapes in the delivery of provisioning ecosystem services in protected. Areas Sustain. 11:2471. doi: 10.3390/su11092471
Martin, E. A., Dainese, M., Clough, Y., Báldi, A., Bommarco, R., Gagic, V., et al. (2019). The interplay of landscape composition and configuration: new pathways to manage functional biodiversity and agroecosystem services across Europe. Ecol. Lett. 22, 1083–1094. doi: 10.1111/ele.13265
Mercau, J. L., Jobbagy, E. G., Viglizzo, E., Menendez, A., Di Bella, C., Bert, F., et al. (2013). Sequía e inundación en la hiperllanura pampeana. Una mirada desde el lote al municipio. Rev. Agron. 33, 71–77.
Ministére de l'Agriculture and de l'Agroalimentaire et de la Forét (2017). Rapport Annuel: la Dynamique Agro-Écologique en 2016. Paris: Ministére de l'Agriculture and de l'Agroalimentaire et de la Forét, 52.
Modernel, P., Dogliotti, S., Alvarez, S., Corbeels, M., Picasso, V., Tittonell, P., et al. (2018). Identification of beef production farms in the Pampas and Campos area that stand out in economic and environmental performance. Ecol. Indicat. 89, 755–770. doi: 10.1016/j.ecolind.2018.01.038
Modernel, P., Picasso, V., Do Carmo, M., Rossing, W. A. H., Corbeels, M., Soca, P., et al. (2019). Grazing management for more resilient mixed livestock farming systems on native grasslands of southern South America. Grass Forage Sci. 74, 1–14. doi: 10.1111/gfs.12445
Modernel, P., Rossing, W. A. H., Corbeels, M., Dogliotti, S., Picasso, V., and Tittonell, P. (2016). Land use change and ecosystem service provision in Pampas and Campos grasslands of southern South America. Environ. Res. Lett. 11:113002. doi: 10.1088/1748-9326/11/11/113002
Nosetto, M. D., Jobbágy, E. G., Brizuela, A. B., Jackson, R. B. (2012). The hydrologic consequences of land cover change in central Argentina. Agric. Ecosyst. Environ. 154, 2–11. doi: 10.1016/j.agee.2011.01.008
Nosetto, M. D., Paez, R. A., Ballesteros, S. I., Jobbágy, E. G. (2015). Higher water-table levels and flooding risk under grain vs. livestock production systems in the subhumid plains of the pampas. Agric. Ecosyst. Environ. 206, 60–70. doi: 10.1016/j.agee.2015.03.009
OECD (2019). Agricultural Policies in Argentina. Trade and Agriculture Directorate Committee For Agriculture, Organisation for Economic Co-operation and Development, TAD/CA(2018)9/FINAL
Overbeck, G. E., Muller, S. C., Fidelis, A., Pfadenhauer, J., Pillar, V. D., Blanco, I. I., et al. (2007). Brazil's neglected biome: the South Brazilian campos perspect. Plant Ecol. Evol. Syst. 9, 101–116. doi: 10.1016/j.ppees.2007.07.005
Pacín, F., and Oesterheld, M. (2014). In farm diversity stabilizes return on capital. Agric. Syst. 124, 51–59. doi: 10.1016/j.agsy.2013.10.008
Parra, G., Borrás, L., and Gambin, B. L. (2020). Maize long-term genetic progress explains current dominance over sorghum in Argentina. Eur. J. Agron. 119:126122. doi: 10.1016/j.eja.2020.126122
Peirano, M. E. (2010). Sensemaking in Small Farming Stories: Knowledge Creation in Crea Groups in Argentina. Palmerston North: Massey University.
Phocas, F., Belloc, C., Bidanel, J., Delaby, L., Dourmad, J. Y., Dumont, B., et al. (2016). Review: towards the agroecological management of ruminants, pigs and poultry through the development of sustainable breeding programmes. II. Breeding strategies. Animal 10, 1760–1769. doi: 10.1017/S1751731116001051
Picasso, V. D., Modernel, P. D., Becoña, G., Salvo, L., Gutiérrez, L., and Astigarraga, L. (2014). Sustainability of meat production beyond carbon footprint: a synthesis of case studiesfrom grazing systems in Uruguay. Meat Sci. 98, 346–354. doi: 10.1016/j.meatsci.2014.07.005
Pinillos, D., Bianchi, F. J. J. A., Poccard-Chapuis, R., Corbeels, M., Tittonell, P., and Schulte, R. P. O. (2020). Understanding landscape multifunctionality in a post-forest frontier: supply and demand of ecosystem services in eastern Amazonia. Front. Environ. Sci. 7:206. doi: 10.3389/fenvs.2019.00206
Pinto, P., Long, M. E. F., and Piñeiro, G. (2017). Including cover crops during fallow periods for increasing ecosystem services: is it possible in croplands of Southern South America? Agric. Ecosyst. Environ. 248, 48–57. doi: 10.1016/j.agee.2017.07.028
Schipanski, M. E., Barbercheck, M., Douglas, M. R., Finney, D. M., Haider, K., Kaye, J. P., et al. (2014). A framework for evaluating ecosystem services provided by cover crops in agroecosystems. Agric. Syst. 125, 12–22. doi: 10.1016/j.agsy.2013.11.004
Schlaich, A. E., Klaassen, R. H. G., Bouten, W., Both, C., and Koks, B. J. (2015). Testing a novel agri-environment scheme based on the ecology of the target species, Montagu's Harrier Circus pygargus. Ibis 157, 713–721. doi: 10.1111/ibi.12299
Scholberg, J. M. S., Dogliotti, S., Leoni, C., Zotarelli, L., Cherr, C. M., and Rossing, W. A. H. (2010). “Chapter 2: Cover crops for sustainable agrosystems in the Americas,” in Genetic Engineering, Biofertilisation, Soil Quality and Organic Farming, ed E. Lichtfouse (New York, NY: Sustainable Agriculture Reviews; No. 4), 23–58. doi: 10.1007/978-90-481-8741-6_2
Schulte, L. A., Niemi, J., Helmers, M. J., Liebman, M., Arbuckle, J. G., James, D. E., et al. (2017). Prairie strips improve biodiversity and the delivery of multiple ecosystem services from corn-soybean croplands. Proc. Natl. Acad. Sci. 114, 11247–11252. doi: 10.1073/pnas.1620229114
Shunmugam, A. S. K., Kannan, U., Jiang, Y., Daba, K. A., Gorim, L. Y. (2018). Physiology based approaches for breeding of next-generation food legumes. Plants 7:72. doi: 10.3390/plants7030072
Stassart, P. M., Crivits, M., Hermesse, J., Tessier, L., Van Damme, J., and Dessein, J. (2018). The generative potential of tensions within belgian agroecology. Sustainability 10:2094. doi: 10.3390/su10062094
Sumberg, J., Thompson, J., and Woodhouse, P. (2012). Why agronomy in the developing world has become contentious. Agric. Hum. Values 1–13, doi: 10.1007/s10460-012-9376-8
Tilman, D. (1988). Plant Strategies and the Dynamics and Structure of Plant Communities. Princeton, NJ: Princeton University Press. doi: 10.1515/9780691209593
Tittonell, P. (2014). Ecological intensification – sustainable by nature. Curr. Opin. Environ. Sustain. 8, 53–61. doi: 10.1016/j.cosust.2014.08.006
Tittonell, P. (2020). Assessing resilience and adaptability in agroecological transitions. Agric. Syst. 184:102862. doi: 10.1016/j.agsy.2020.102862
Tittonell, P., Laurens, K., Frederic, B., Félix, G. F., Ruggia, A., van Apeldoorn, D., et al. (2016). Ecological intensification: local innovation to address global challenges. Sustain. Agric. Rev. 19, 1–34. doi: 10.1007/978-3-319-26777-7_1
Tittonell, P., Scopel, E., Andrieu, N., Posthumus, H., Mapfumo, P., Corbeels, M., et al. (2012). Agroecology-based aggradation-conservation agriculture (ABACO): targeting innovations to combat soil degradation and food insecurity in semi-arid Africa. Field Crop Res. 132, 168–174. doi: 10.1016/j.fcr.2011.12.011
Tittonell, P. (2019). Las transiciones agroecológicas: múltiples escalas, niveles y desafíos. Rev. FCA UNCUYO 51, 231–246.
Tsiafouli, M. A., Thébault, E., Sgardelis, S. P., de Ruiter, P. C., van der Putten, W. H., Birkhofer, K., et al. (2015). Intensive agriculture reduces soil biodiversity across Europe. Global Change Biol. 21, 973–985. doi: 10.1111/gcb.12752
van der Ploeg, J. (2013). The genesis and further unfolding of farming styles research. Historische Anthropol. 20, 427–439. doi: 10.7788/ha.2012.20.3.427
Wayman, S., Kucek, L. K., Mirsky, S. B., Ackroyd, V., Cordeau, S., and Ryan, M. R. (2017). Organic and conventional farmers differ in their perspectives on cover crop use and breeding. Renew. Agric. Food Syst. 32, 376–385. doi: 10.1017/S1742170516000338
Weltzien, E., and Christinck, A. (2017). “Chapter 8 - participatory breeding: developing improved and relevant crop varieties with farmers,” in Agricultural Systems, 2nd edn, eds S. Snapp and B. Pound (Amsterdam: Academic Press), 259–301.
Wezel, A., Goris, M., Bruil, J., Félix, G. F., Peeters, A., Bàrberi, P., et al. (2018). Challenges and actions points to amplify agroecology in Europe. Sustainability 10:1598. doi: 10.3390/su10051598
WWF (2020). Living Planet Report 2020 - Bending the Curve of Biodiversity Loss, eds R. E. A. Almond, M. Grooten, and T. Petersen.
Keywords: sustainability, intensification, agriculture, landscape, ecosystem services, South America, Europe
Citation: Tittonell P, Piñeiro G, Garibaldi LA, Dogliotti S, Olff H and Jobbagy EG (2020) Agroecology in Large Scale Farming—A Research Agenda. Front. Sustain. Food Syst. 4:584605. doi: 10.3389/fsufs.2020.584605
Received: 17 July 2020; Accepted: 12 October 2020;
Published: 18 December 2020.
Edited by:
Hans Rudolf Herren, Independent Researcher, Washington, DC, United StatesReviewed by:
Didier Bazile, Centre de Coopération Internationale en Recherche Agronomique pour le Développement (CIRAD), FranceFlorian Wichern, Rhine-Waal University of Applied Sciences, Germany
Copyright © 2020 Tittonell, Piñeiro, Garibaldi, Dogliotti, Olff and Jobbagy. This is an open-access article distributed under the terms of the Creative Commons Attribution License (CC BY). The use, distribution or reproduction in other forums is permitted, provided the original author(s) and the copyright owner(s) are credited and that the original publication in this journal is cited, in accordance with accepted academic practice. No use, distribution or reproduction is permitted which does not comply with these terms.
*Correspondence: Pablo Tittonell, dGl0dG9uZWxsLnBhYmxvQGludGEuZ29iLmFy