Linking Coleopteran Diversity With Agricultural Management of Maize-Based Agroecosystems in Oaxaca, Mexico
- 1Laboratorio Nacional de Ciencias de la Sostenibilidad, Instituto de Ecología, Universidad Nacional Autónoma de Mexico, Mexico City, Mexico
- 2Posgrado en Ciencias Biológicas, Universidad Nacional Autónoma de Mexico, Mexico City, Mexico
- 3Facultad de Ciencias, Universidad Nacional Autónoma de Mexico, Mexico City, Mexico
- 4Centro de Investigaciones Interdisciplinarias en Ciencias y Humanidades, Universidad Nacional Autónoma de Mexico, Mexico City, Mexico
- 5C3, Centro de Ciencias de la Complejidad, Universidad Nacional Autónoma de Mexico, Mexico City, Mexico
Biodiversity is known to be influenced by agricultural practices in many ways. However, it is necessary to understand how this relation takes place in particular agroecosystems, sociocultural contexts and for specific biological groups, especially in highly biodiverse places. Also, in order to systematically study and track how biodiversity responds or changes with agricultural practices, it is necessary to find groups that can be used as practical indicators. We conduct a study of beetle (Coleoptera) diversity in maize-based agricultural plots with heterogeneous management practices in the Central Valleys of Oaxaca, Mexico, a region with outstanding biodiversity and a long agricultural history. We use a mixture of local knowledge and multivariate statistics to group the plots into two broad and contrasting management categories (traditional vs. industrialized). Then, we present an analysis of Coleopteran diversity for each category, showing higher levels across different diversity indexes for the traditional plots. Specifically, Coleopteran guilds associated with natural pest control and soil conservation are more common in traditional plots than in industrialized ones, while herbivorous beetles are more abundant in the second. Also, our results let us postulate the Curculionidae family as an indicator of both management type and overall Coleopteran diversity in the agricultural lands of the study site. We discuss our results in terms of the agricultural matrix quality and its role in strategies that favor the coexistence of culturally meaningful agricultural systems and local biodiversity.
Introduction
Agriculture is a diverse and dynamic process that involves a large variety of production practices. These practices are not assembled at random, but occur as sets of land management activities, “management types,” that are interdependent, adapted to each other and that function as a system with specific goals (Andow and Hidaka, 1989; Vandermeer, 2011). Depending on management type, agriculture can affect in different ways the maintenance of biodiversity at the local and regional level. Indeed, it has been suggested that some types of agricultural management can coexist and even favor biodiversity conservation if it allows for the temporary or permanent establishment of local species in agroecosystems (Perfecto et al., 2009; Fahrig et al., 2011; Kremen and Merenlender, 2018).
However, the relationship between specific agricultural management types with biodiversity and ecosystem services, like pest control and pollination, is not straightforward. On the one hand, agricultural landscapes can rarely be associated with a single and clear management type. In the tropics and subtropics, for example, one regularly finds patchy landscapes with mixed management types that incorporate some industrial characteristics but keep many features of traditional agriculture (Perfecto et al., 2009; Álvarez et al., 2014; Ramos, 2020; Urrutia et al., 2020). It is thus a challenge to organize such heterogeneity into typologies that allow us to test the role of management, as well as of specific agricultural practices, on the maintenance of biodiversity and its associated ecosystem services (Álvarez et al., 2014). On the other hand, the response of biodiversity to agricultural management depends on numerous factors, like the species under study, landscape structure, type of crop, climatological conditions, etc. (Lindenmayer et al., 2012; Wilson et al., 2017; Winter et al., 2018; Qiu et al., 2019). In order to overcome a simplistic view that conceives agriculture as an absolute antagonist to biodiversity, it is thus necessary to assess the effect of agricultural management in different contexts and case studies, and to identify, favor or adapt strategies that allow for its coexistence with biodiversity.
In ecological terms, agricultural managements with high planned diversity and low input dependence are usually associated with heterogeneous landscapes that enable diverse ecosystem services (Perfecto, 2003; Fahrig et al., 2011; Kremen and Merenlender, 2018; Urrutia et al., 2020). Under certain conditions, these types of agroecosystems have been shown to maintain or even increment the biodiversity at a landscape or plot level, exhibiting a high diversity of organisms that are, in turn, good indicators of overall biodiversity and ecosystem functioning (Perfecto, 2003; Gallé et al., 2019; Happe et al., 2019; Qiu, 2019). In turn, it has been suggested that the reduction of plant species in some agroecosystems leads to a decrease in the number and type of habitats suitable for associated biodiversity, including arthropods (Tylianakis et al., 2007; Letourneau et al., 2011; Fabian et al., 2013; Isbell et al., 2017; Liere et al., 2017). Such reduction in plant diversity, and the concomitant reduction in potential habitats, is often driven by industrialized managements in which plants other than the main crop are not grown and are usually not tolerated and landscape structure is simplified (Ekroos et al., 2010; Cizek et al., 2012; Karp et al., 2018).
In particular, the loss of arthropod diversity has been reported to result in the loss of key ecosystemic services, like natural pest control, pollination, or soil conservation (Bianchi et al., 2006; Cizek et al., 2012; Poveda et al., 2012; Wan et al., 2019; Flores-Gutierrez et al., 2020). Within arthropods, in this work we will focus on Coleoptera (beetles), since their diversity and abundance has been found to correlate with other vertebrate and invertebrate taxa (Pearson and Cassola, 1992; Holland, 2002; Ohsawa, 2010), as well as with with key environmental variables (Lassau et al., 2005; Campanelli and Canali, 2012) and agricultural management practices (Kromp, 1999; Holland and Luff, 2000; Burgio et al., 2015; Flores-Gutierrez et al., 2020). Beetles are cosmopolitan, they define a great variety of niches, exhibit diverse life strategies and they take part in several ecological processes making them important matter and energy flux regulators (Hunt et al., 2007; Bouchard et al., 2011). Moreover, beetles have been postulated as bioindicators of agroecosystem functioning (Pizzolotto et al., 2018), as they include both potential pests and natural pest enemies, as well as pollinators and detritivorous species, among others (Hunt et al., 2007; Brooks et al., 2012).
In spite of the key role of beetles within agroecosystems, it remains to be tested if their overall diversity and abundance, as well as that of guilds potentially involved in agroecosystem services or disservices, exhibit differences between different types of management in widely distributed maize-based agroecosystems in the tropics. Addressing this issue may also lead to the postulation of specific groups of beetles as indicators of management, overall coleopteran diversity and agroecosystem functioning in this type of agroecosystem. Maize-based agroecosystems like the Mesoamerinca milpa, which includes maize, beans and other plants, are fundamental in terms of: (i) food production and nutrition (e.g., Bellon et al., 2018; GIAHS-FAO, 2020), (ii) the vast cultural diversity and knowledge associated to their reproduction, as illustrated for instance in their uncountable gastronomic expressions (CEMDA, 2017; Mora Van Cauwelaert, 2017; Lyver et al., 2019; GIAHS-FAO, 2020), and (iii) their potential role in conservation in the ample and highly biodiverse areas in which they have been historically practiced (Myers et al., 2000; Oviedo, 2002; Bellon et al., 2018).
In this work, we sampled the beetle community in plots belonging to a highly heterogeneous, peasant-driven agricultural landscape (Urrutia et al., 2020). We worked in the Central Valleys of Oaxaca, Mexico, a region recognized for its extraordinary biological and cultural diversity, and that is in many ways representative of small-scale agriculture landscapes in southern Mexico (Mora Van Cauwelaert, 2017; Ramos, 2020; Urrutia et al., 2020). In the area where this study was conducted, a relatively diverse scarab beetle community has been previously described, and was found to be as rich in croplands as in contiguous forests (Ramírez-Ponce et al., 2019). Nevertheless, the relationship between agricultural management and the diversity of beetle taxons and guilds, as well as their potential ecosystemic services, had not been assessed prior to our study. Given the evidence summarized above, we expected to find a higher diversity of Coleoptera in plots with traditional practices involving relatively high plant diversity (and habitats) and little use of external inputs, such as pesticides. Within this diversity, we expected to find a better representation of guilds associated to ecosystemic services in more diversified and traditionally managed agroecosystems. We also expected to find specific taxons that could function as practical biological indicators of the management type and the overall coleoptera diversity.
Methods
Study Area
This study was conducted at Villa de Zaachila (Zaachila hereafter), in the Central Valleys of Oaxaca, Mexico. It is a semi-urban population located 17 km southeast of the state's capital. The history of landscape management of Zaachila begins with the Zapotec peoples, about 3,500 years ago. Today, agricultural plots are mostly managed by small landholders for family or local consumption. Many of these plots represent a state-given usufruct land, the so-called ejidos, in which variable degrees of collective management occurs (INEGI, 2007; Mora Van Cauwelaert, 2017). The most represented land use types are agriculture, which covers 48% of its total area, secondary forest with 23% and urban zones with 19% (Urrutia et al., 2020). According to government data, the municipality is composed of 1,669 ha which are distributed among 1,521 small landholders (heads of family), which is to say every family has an average of 1 ha of land. This small-scale agricultural scheme combines very diverse practices in a rather fine-scale mosaic (INEGI, 2007; Figure 1). In order to organize this heterogeneous management context into types or categories that were amenable to further analyses, we used a previously reported method to generate management typologies (Álvarez et al., 2014; see Plot Categorization section below).
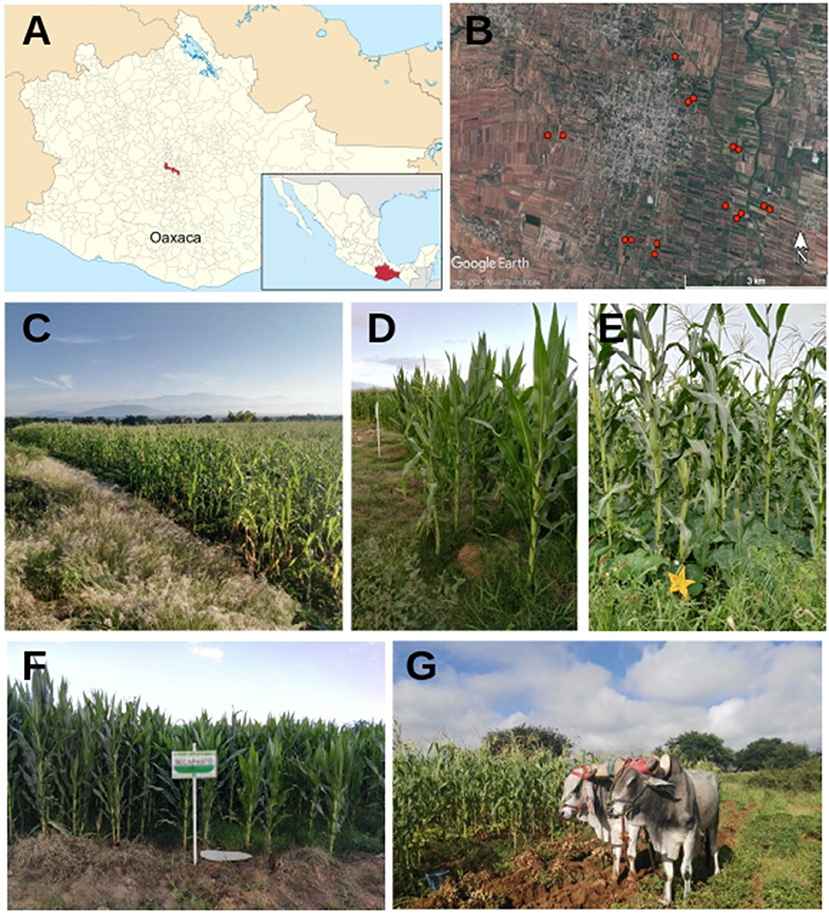
Figure 1. Maize-based agricultural systems in the study site. The plots were selected in the municipality of Zaachila, Oaxaca (A) as neighboring pairs with contrasting types of management, as suggested by our informers (B). The management practices were diverse and ranged from low-input polycultures to high-input monocultures (C–G). (D,F) illustrates more industrialized plots in which maize is grown in monoculture and with the use of external and commercial inputs. (C,E,G) illustrate more traditional plots exhibiting a maize-based polyculture called milpa, as well as animal traction. Sources: Wikimedia commons ID 360701525 and 284784087 (A), Google Earth (B) and personal photographs (C,D).
Plot selection
Using the methodology developed by Álvarez et al. (2014), we looked for 16 plots belonging to contrasting types of management. The methodology seeks to group heterogeneous plots based upon management information provided by local key informants in a reproducible way. It starts with an initial hypothesis, in which plots that are thought to belong to qualitatively different management categories are selected by key informants. Then, thorough information about their management is obtained through interviews and, finally, a statistical multivariate test is performed in order to confirm or refute the hypothesized categorization. In the following we elaborate these steps.
During September 2016 we worked with a group of small landholders, key informants in the community who provided us information about the study site (Marshall, 1996a,b). Together, we set out to find plots belonging to two qualitatively different management types. First, a traditional type, characterized by a low use of commercial inputs and the planting of diverse, local seeds. Even though the exact combination of agricultural practices in each plot actually varied, we postulated that by sharing those two features they would constitute an identifiable category. Second, we looked for the industrialized type, which would be formed by plots with a relatively high use of machinery (for the oaxacan Central Valleys context), agrochemicals and few types of hybrid seeds. With this in mind, and according to their knowledge of local practices, the local small landholders led us to eight pairs of plots composed of what they judged was one plot under traditional management and one industrialized plot. In order to make the sample as representative as possible and to reduce biases stemming from possible landscape heterogeneities, we required plots in each pair to be as close to one another as possible (when possible even side to side) and for them to cover the North, South, East and West parts of the locality (Figure 1). This assignment of plots to management type represented our initial hypothesis and was later tested with a statistical analysis performed over a set of variables that were obtained from detailed interviews to the owners of each plot, as we will explain in the subsequent sections.
Plot Typology Validation
Still following the methodology by Álvarez et al. (2014), we conducted semi-structured interviews with the owners of each plot in order to collect information on their agricultural management. We asked for information about the planted crops in the current and previous years, use of industrial inputs along the year (fertilizer, pesticides, herbicides, and machinery), rotation techniques, attitude toward non-planted herbs and trees, irrigation use, purpose of the crops (such as family use or market sales), relative economic importance for the family, and so forth. The complete list of variables, definitions and data type can be read in in the Supplementary Material (Supplementary Table 2).
Interviews lasted between 1 and 2 h and they were recorded in audio and summarized in written questionnaires. Some of the variables were also systematically verified by sight in the plots, such as the presence of trees or herbs and the number of crops planted at the current time, but most were only obtainable through the interviews since they involved actions taken in the previous months or other non-observable aspects. Prior to this process, an informative letter was given to each of the participants and all of them gave their informed consent to participate in the study. Because many plots were not managed by single individuals but by families, we interviewed more than one person by plot when it was possible, mainly couples who were together in charge of the plots and households. In total, we interviewed 21 people, with ages ranging from 39 to 78 years old (average age was 60, standard deviation 10.9 years), composed of 16 men and 5 women.
Out of all the information obtained, we chose those management-related variables for which we had clear and unequivocal responses from all interviewees. Two variables (number of crops and number of crop varieties) were recorded as absolute richness values and the rest were recorded as presence/absence values. We are aware of the loss of information that this process implied but we hold it necessary for having a comparable set of data for all plots and to proceed as suggested by Álvarez et al. (2014). This gave a result of 22 variables, which were poured into a quantitative table that can be found in the Supplementary Material (Supplementary Table 3). We used this table to test the initial categorization of plots proposed jointly with key informants (traditional and industrialized). We created a correlogram of Spearman coefficients in order to look for relationships among management practices. Then, due to the type of variables in our study, we used a Factor Analysis of Mixed Data (FAMD) for organizing the plots according to their management practices. This allowed us to confirm or refute the categorization hypothesized by our key informants in order to form the groups that were later used as treatments to compare beetle diversity. All tests were done using the FactoMineR and corrplot packages from the coding language R 1.1.383 (R Core Team, 2014). All scripts used for this investigation are publicly available at: https://github.com/laparcela/ColeopteraZaachila.
Diversity of Coleoptera
Plot extension was around 50 × 200 m, and we established five quadrants measuring 1.5 × 1.5 m in each one. In order to randomly locate the quadrants within plots, we first established a random point in each of the long sides of the plot and drew an imaginary line connecting them. At this line's center, we established the first sampling quadrant. Then we established another two quadrants halfway between this center and each of the line's extremes. Afterwards, a second imaginary line was drawn perpendicularly to the first line, crossing its center. The fourth and fifth quadrants were established at the extremes of this second line. Following this procedure, we obtained five quadrants within each plot, three at the plot interior and two at the plot borders (A diagram can be found in the Supplementary Figure 1). Sampling was made this way because it was done as part of a broader study and this design fitted all sampling purposes (work in preparation). Beetle collection was made before any other sampling in order to avoid disturbing individuals.
During September 2016, toward the end of the rainy season and when crops were almost ready for harvesting, all plots were sampled through the use of sweep nets in the five quadrants. Two plots were sampled each day, starting at 7:00 a.m. and until ~11:00 a.m. This was kept constant in order to avoid extra noise from a day's normal temperature variation. Due to time limitations, we only had one sampling date per site, which represents a shortcoming for our study. Likewise, it is important to note that the use of sweep nets biases the sampling toward beetles that are active in the above-ground part of the agroecosystem, mainly on stems, leaves, flowers, fruits or sometimes flying in the air. Collected specimens were fixed with 70% ethanol and specimens from the five quadrants were pooled together in a single sample for each plot and then taken to a laboratory for identification. Individuals were identified to family level using identification keys (White, 1983; Tripplehorn and Johnson, 2005) and to morph level afterwards. Diversity was quantified as abundance and richness at both family and morph levels. The complete dataset for this investigation is publicly available at: https://knb.ecoinformatics.org/view/urn%3Auuid%3A0b5a89ef-93f8-493c-8bff-e4843b9a45b9.
Diversity in the industrialized and the traditional plots was compared using one-way ANOVAs, Renyi diversity analysis [using the vegan and BiodiversityR packages from the coding language R 1.1.383 (R Core Team, 2014)] and direct quantifications at several taxonomic levels. Following the ANOVA tests, which were performed using each plot as a separate sample, we pooled all plots belonging to the industrialized group in one, and all plots belonging to the traditional group in another sample, and compared their overall richness. A Renyi diversity analysis was performed on these compound samples. Renyi's analysis relates in a graphical way many widespread ecological indexes, which are located along the horizontal axis while the vertical axis shows the corresponding value for Renyi's index (H-alpha)1. Afterwards, we grouped the families into four trophic guilds in order to assess functional differences between types of management. The guilds comprised herbivores, predators, detritivores, and polyphagues, the latter including cases when individuals are able to exploit a wide variety of feeding resources and cases when juveniles and adults belong to different categories. The complete table of families belonging to each guild can be found in Supplementary Table 4 (based on the public dataset mentioned above). We also performed a Canonical Correlation Analysis (CCA) in order to test the relation between a chosen set of management variables and a set of beetle families. In order to reduce the number of variables and meet the test's requirements, we chose those management practices whose contributions to our PCA's first two dimensions were above a reference value that corresponds to the expected value if the contributions were uniform. Variance-inflation factors were calculated to eliminate variables causing multicollinearity. Additionally, we focused only on the most abundant families inside each trophic guild. This procedure left us with 8 management variables: number of crops, irrigation, quelites, trees, compost, and industrial fertilizer, pesticide and herbicide. These were tested for correlation with 9 beetle families: Cleridae, Coccinellidae, Carabidae, Chrysomellidae, Curculionidae, Nitidulidae, Anobiidae, Cantharidae, and Phalacridae.
Finally, we looked for a beetle family that could be a useful indicator of the agricultural management type. We required it to be non-exclusive of a particular type of management, but to show a clear and different response to the management categories. In order to test its potential as an indicator, we analyzed the relation between its abundance and (i) plot management and (ii) overall beetle diversity (Figure 8B). For this, we adjusted a second degree polynomial to its abundance in each plot (we took the natural logarithm of this measure in order to comply with the analysis assumptions) against its position along the management gradient (the coordinate each plot occupied in the horizontal axis of Figure 3A). We also analyzed the relation between such family's abundance and the total richness of beetles in each plot.
Results
Plot Categorization
The correlogram showed relations among sets of management practices, as expected from the theoretical postulation of management types (Vandermeer, 2011; Álvarez et al., 2014). We found a positive correlation (Figure 2, groups of blue circles) between practices commonly associated to a traditional management, such as managed border, use of manure, animal traction, number of crops, quelites (non-planned herbs that are frequently useful to small landholders' families in different ways), number of varieties, presence of trees and use of organic fertilizers. Likewise, there is a strong positive correlation between practices associated with an industrialized management, such as use or industrial fertilizers, herbicides and pesticides, irrigation and monoculture. We also found negative correlations (Figure 2, groups of red circles) between irrigation and fallow time, and subsistence agriculture and monoculture.
The FAMD we conducted explained 46.2% of the total variance in its two first dimensions (27.4 and 18.8%, respectively). Figure 3B corresponds to the variables factor map, where we show that the most correlated variables with the first dimension were the number of varieties within crops (0.896 correlation), number of crops (0.872 correlation), and the monoculture planting scheme (−0.722 correlation); while the second dimension was most correlated with the presence of a fallow period (0.9 correlation), irrigation (−0.84 correlation) and use of industrial pesticides (−0.62 correlation) (see Supplementary Table 2 for a more detailed definition of each variable). Moreover, all the variables with a positive correlation to the first dimension were practices associated to a traditional management (Altieri et al., 1997): use of local seeds, crop rotation, fallow period, animal traction, presence of quelites, managed border, presence of trees, use of green manure, compost regular manure, and organic inputs. On the other hand, the variables with a negative correlation were all associated with an industrial model of agriculture (Perfecto et al., 2009): a monoculture scheme, irrigation and use of industrial inputs (fertilizers, herbicides, and pesticides).
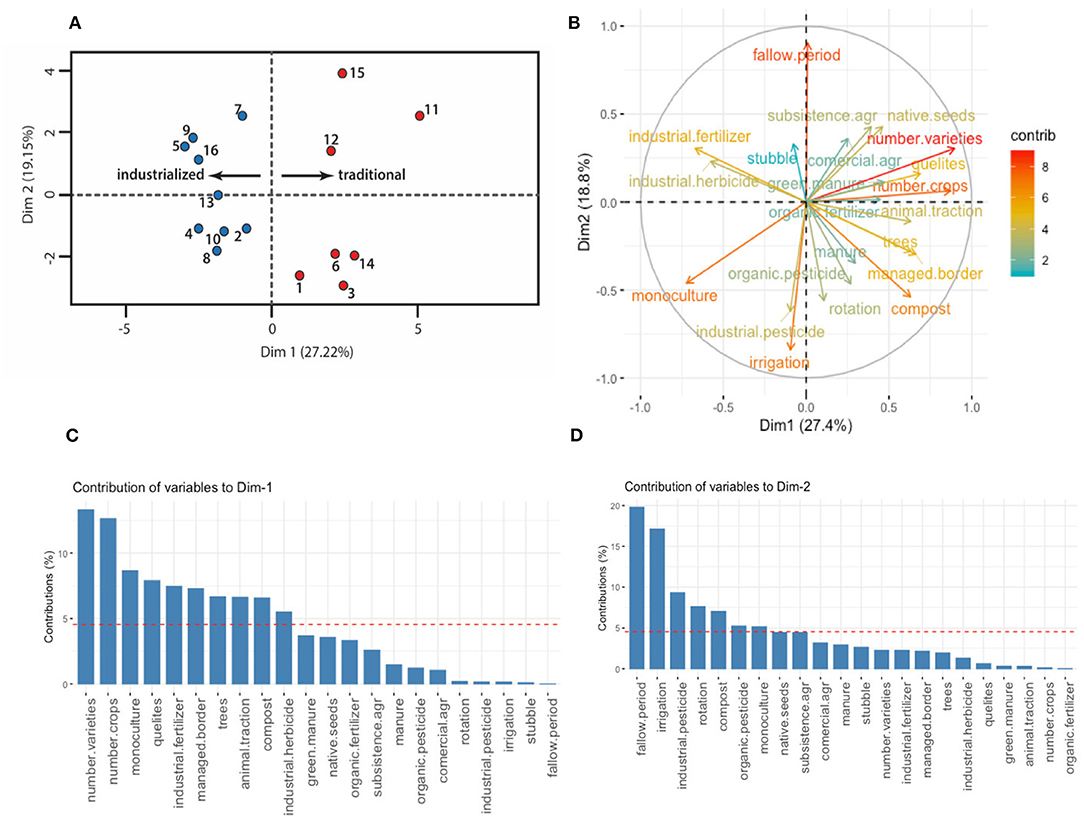
Figure 3. FAMD analysis. (A) FAMD output for the 16 plots under study, plots on the left side correspond to the industrial management type and plots to the right correspond to the traditional management; (B) variables factor map, colors are given by each variable's contribution to the first and second dimensions; (C) contribution of variables to the first dimension; (D) contribution of variables to the second dimension. The reference line in (C) and (D) corresponds to the expected value if the contributions were uniform.
The first dimension of the FAMD thus depicts a management gradient that goes from the most industrialized on its negative side to the most traditional on its positive side (Figure 3A). Based upon this result, we separated the plots falling to the right and left side of the first dimension's origin, thus discretizing the management gradient into two separate categories (industrial and traditional). By doing this, we found that 15 out of 16 plots fell in the expected side from the management category initially hypothesized by key informants. Thus, we sampled and compared coleopteran diversity among these two groups, leaving aside the one plot that did not match the original hypothesis. Note that the vertical axis was not used to further divide the plots in more groups, nevertheless it is worth saying that the variables with higher coefficients in this dimension were fallow period with a positive sign, and irrigation and pesticide use with a negative sign.
Beetle Diversity
In Figure 4 we present an overview of the sampled diversity of families in the order Coleoptera. In total, we found 1,168 individuals belonging to 25 families, which we then identified as 80 different morphs. The most abundant families were Chrysomelidae, Cantharidae and Curculionidae, with the first two being well-represented in both management types and the last mainly in the traditional plots. We found one exclusive family to the industrialized management: Trogossitidae. Likewise, there were four families exclusively found in the traditional plots: Bostrichidae, Buprestidae, Languriidae, and Tenebrionidae.
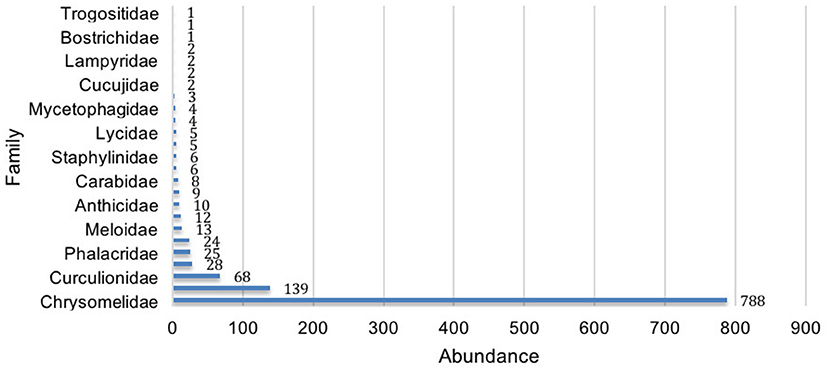
Figure 4. Total abundance of every beetle family identified in the traditional and industrialized management types of maize-based agroecosystems.
Overall, coleopteran abundance did not show significant differences among plots from the industrialized and the traditional groups, but both family and morph richnesses were significantly higher in the traditional plots (p = 0.0265 and p = 0.0203, respectively) (Figure 5). Figure 6A depicts the results of pooling together all plots belonging to the same management category. We present morph richness inside each family in these two compound samples. Renyi diversity was higher for the traditional category, as it was significantly more diverse across all the Renyi's spectra of indexes (confidence intervals at 95%) (Figure 6B).
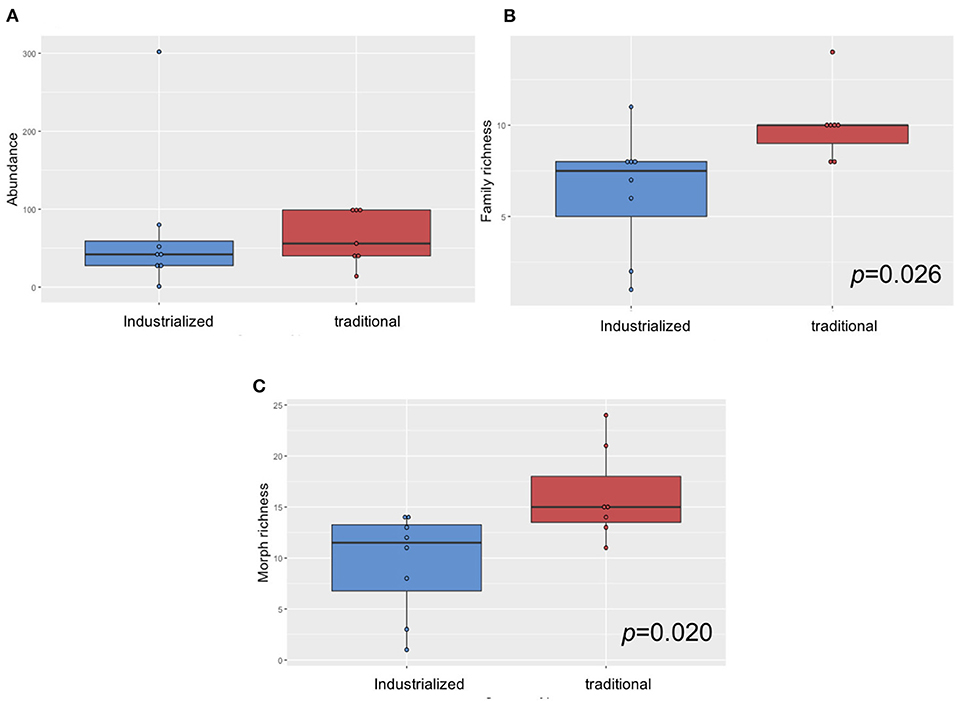
Figure 5. Boxplot and ANOVA tests for (A) individual abundances, (B) family richness, and (C) morph richness in each management type. The median is represented by the horizontal line inside the boxes, lower and upper hinges correspond to the first and third quartiles, whiskers extend to the largest value no further than 1.5 times the interquartile range, data points are represented by circles and data beyond the whiskers are outliers. The ANOVA assumptions of equality of variances and residual normality were covered by the data: Bartlett test p = 0.1551 and Shapiro test p = 0.4861 for family richness data and Bartlett test p = 0.6805 and Shapiro test p = 0.9387 for morph richness.
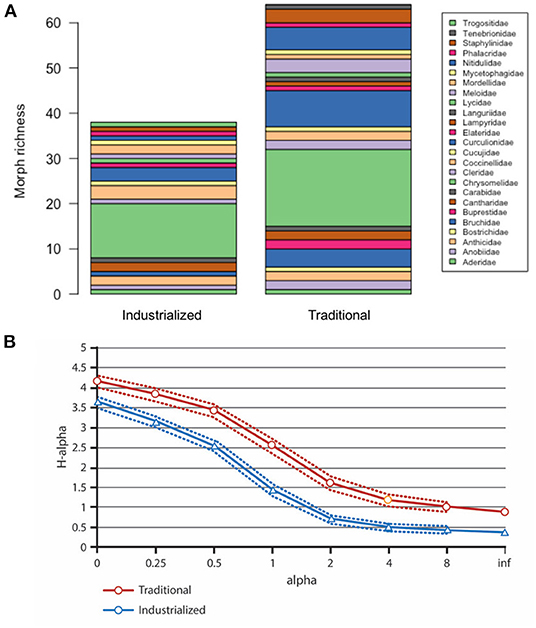
Figure 6. Comparison of the industrialized vs. traditional compound samples. (A) Morph richness is strikingly higher for most of the families in the traditional group; (B) Renyi diversity is consistently higher in the traditional group.
Functional groups varied strongly between management types. Traditional plots had 30% less abundance of herbivorous families, 27% more predators, 78% more detritivores, and 7% less polyphages. Herbivores were dominated by Chrysomelidae and Curculionidae; predators were mainly Cleridae, Coccinellidae and Carabidae; detritivores were dominated by Nitidulidae and Anobiidae, and polyphagues were mainly Cantharidae and Phalacridae.
The CCA showed that management variables accounted for 73% of the variance in beetle community composition, with the strongest predictor variables being: industrial herbicide, followed by industrial pesticide, quelites, industrial fertilizer, and number of crops (p < 0.05). Figure 7 shows that the most correlated pairs are Curculionidae with number of crops, and Cantharidae with the use of industrial fertilizer and herbicide, while families closer to the center of the graph show weaker correlations. The most abundant predators, Cleridae, correlate the most with the presence of quelites; while the most abundant herbivores, Chrysomellidae, correlate the most with industrial herbicide and fertilizer. Nitidulidae, the most abundant detritivores, correlate better with both quelites and number of crops. The full model was statistically significant (p < 0.001).
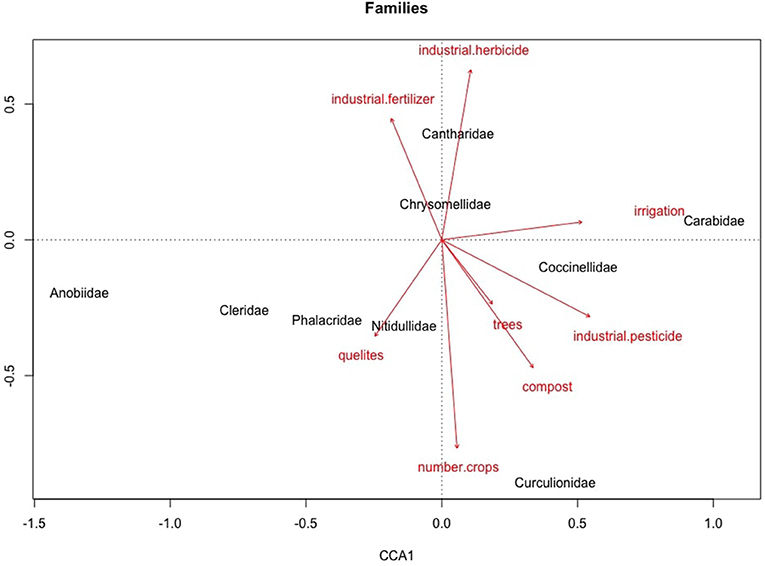
Figure 7. Canonical correlation analysis of management practices and the best represented beetle families.
Curculionidae As An Indicator of Management Type and Diversity
Curculionidae was the only family that complied with the requirements for working as an indicator: it was non-exclusive of any management type and showed a strong and different response to each one, it was also abundant and relatively easy to identify (Figure 8A), so we investigated its potential as an indicator of management and overall beetle diversity. The adjustment of a second degree polynomial between Cucrculionid abundance in each plot and its position along the management gradient gave a positive relation (R = 0.50428, p < 0.05), suggesting that plots with a more traditional management tend to have a higher abundance of Curculionidae (Figure 8B). Additionally, in Figure 8C we show the relation between curculionid abundance and the total richness of beetles in each plot. We first took richness at a family level but found no significant results, nevertheless, the same comparison at a morph level did show a significant positive relation between the two variables (R = 0.70715, p < 0.05).
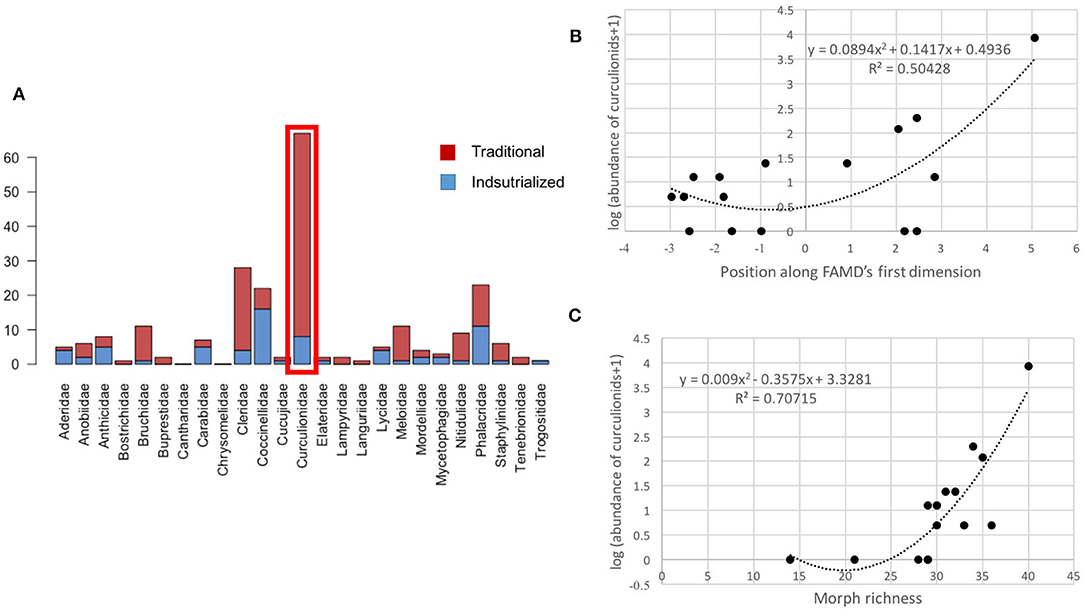
Figure 8. Curculionidae as indicators of management type and coleopteran diversity. (A) abundance of each family in traditional and industrialized plots, (B) polynomial regression of curculionid abundance against management gradient, (C) polynomial regression of curculionid abundance against richness of beetle morphs.
Discussion
In the first part of our study, we used the method by Álvarez et al. (2014) to define two broad management categories, traditional and industrialized, based upon several agricultural practices. Through the multivariate statistical approach we followed, we identified two variables to be most important for the organization of plots into these categories: total number of crops and total number of varieties grown. From an ecological point of view, crop diversity is involved in many processes both below or above ground. It is also associated with structural diversity (Del Río et al., 2003) and therefore, to the set of habitats available for the biota (feeding, refuge, reproduction spots, etc.) inhabiting or passing through the plots (Tylianakis et al., 2007; Letourneau et al., 2011; Fabian et al., 2013; Isbell et al., 2017; Liere et al., 2017). In a practical sense, this result is important given the complexity of defining different types of management in heterogeneous landscapes. Indeed, both variables can be measured with relative simplicity by academics, technicians or small landholders, and they seem to work as “umbrella” variables for many other practices associated with agricultural management, making them useful guidelines for future work that seeks to study different types of management in the region.
Another remarkable aspect is the difference in the grouping patterns of the industrialized and the traditional plots. As seen in Figure 4, the industrialized plots fall much closer to each other than the traditional plots. This means that even though both categories have a relatively high internal variation, the industrialized plots are far more similar to each other than the traditional ones, reflecting the tendency to homogenization that is characteristic of industrialized agriculture, in contrast with the heterogeneity and context-specificity of traditional agriculture (Gliessman, 2015). This homogenization tendency is clear, even for our study area, where the level and complexity of the machinery and inputs used in the industrialized plots is not as high as in other regions of Mexico, like El Bajio and the North of Mexico. The high variability found inside the traditional group of plots is surprising given the small scale of this work (Figure 1), which further illustrates the heterogeneity that can be found even in this type of landscape (Urrutia et al., 2020). This shows that the traditional management is not static or “archaic,” as there is significant variation in the practices that small landholders adopt through time and space.
As for coleopteran diversity, the traditional plots showed higher values at both family and morph level, regardless of the diversity measure used, and in spite of the fact that many of the industrialized plots were close to them (which could have otherwise brought diversity to industrialized plots). This indicates that coleopterans are significantly sensible to agricultural management even at small scales, a fact worth noting given that local communities are influenced by their surroundings and that their diversity depends on the species pool at a broad scale and on many landscape variables (Duelli et al., 1999; Gabriel et al., 2010). Moreover, management variability inside traditional and industrialized categories was also large, as we have discussed above, which makes significant inter-category differences more striking. In addition to having a higher diversity of beetles in general, the traditional plots had more exclusive species, which points to their importance as reservoirs of rare species.
In terms of trophic guilds, the traditional plots had less herbivores and more predators and detritivores, which results in a more functionally rich community with possible positive repercussions for agriculture. Namely, the bigger number of predators could be controlling the herbivorous populations and preventing potential pests, while detritivorous species could be sustaining healthier nutrient cycling processes. This may play a critical role for production, since it is estimated that 18–40% of crop losses can be attributed to herbivore arthropods (Oerke, 2006; FAO, 2017; Sharma et al., 2017), and predatory arthropods have proven important drivers of autonomous pest control mechanisms (Zhang et al., 2007; Vandermeer et al., 2010; Letourneau et al., 2011; Rusch et al., 2016). Our results are consistent with other studies that have found more natural enemies in diversified agroecosystems than in those with an industrialized management (Tooker and Frank, 2012; Torcat-Fuentes et al., 2018; Wan et al., 2019; Flores-Gutierrez et al., 2020). The reasons for this may include that natural enemies are more dependent on secondary sources of food and refuges or nesting sites, which are available in diverse environments (Bianchi et al., 2006; Chaplin-Kramer et al., 2011; Karp et al., 2018). Additionally, natural enemies appear to be more vulnerable to pesticides than herbivores, on the one hand because they too are killed by them (Theiling and Croft, 1988), but additionally, because their food sources (namely, other arthropods) are periodically depleted by pesticides while herbivores' food sources remain unaltered (Perfecto et al., 2009). The combination of predators and detritivorous beetles may be crucial in providing the ecosystemic services of pest regulation and soil fertility (Power, 2010; Tscharntke et al., 2012), which can in turn translate into a decrease in the need for agricultural inputs (Nabhan and Buchmann, 1997; Naylor and Erlich, 1997).
Moreover, the bigger amount of herbivores that we found in industrialized plots further suggests that this combination of practices is not associated to the alleviation of pest related problems. This has been documented before, with herbivore communities being sometimes unresponsive to management industrialization (Flores-Gutierrez et al., 2020) and other times being actually increased by it (Knight and Norton, 1989; Krauss et al., 2011; Meehan et al., 2011). Indeed, our CCA showed that the most abundant herbivores, the Chrysomellidae, were positively correlated to the use of industrial fertilizers and herbicides and negatively correlated to the number of crops, characteristics that together describe an industrialized management. On the other hand, the most abundant predators and detritivores, Cleridae and Nitidullidae, correlated positively with the presence of quelites and number of crops, while relating negatively to industrial inputs and irrigation, again strongly suggesting that a traditional management benefits this guild. Finally, little can be said of the polyphague guild in our analysis since it comprises families with too wide a variety of eating strategies; identification to genre or species levels would be required in order to refine the categorization of these taxa.
Regarding the family Curculionidae, we found that the abundance of this family increases as management tends to be more traditional and that it also reflects the diversity of coleopterans in general. Nevertheless, this should be taken with caution because it was the case for most, but not all the sampled plots (Figure 3). This said, curculionids have an easily recognizable morphology (mainly because of the anterior projection in their head) and in fact it is safe to say that all small landholders in Mexico are well-familiarized with them, so they can indeed be widely used as indicators of overall coleopteran diversity or agricultural management type. The family is abundant in most of the world, and more markedly in the tropics. They mainly feed on vegetal tissue, though they can also eat lichens, algae and fungi (Morrone, 2014). According to Zimmerman (1994), all angiosperms are probably consumed by at least one curculionid species. However, they are not just herbivores, for various plants depend on curculionids for pollination (Morrone, 2014). This family has been used as an indicator of coleopteran diversity before (see Ohsawa, 2010), but we have no knowledge of it being used in Mexico or in maize-based agroecosystems. Because of its cosmopolitan nature, it would probably be found to be a good estimator of coleopteran diversity in a wide arrangement of environments. On the other hand, as an indicator of agricultural management, Silva et al. (2002) also found this family to be more diverse under a management that would fit into our traditional category.
In the agricultural context, curculionids are often thought of as a pest, because they feed on a wide range of crops (maize, beans, avocado, cotton, rice, etc.) (Morrone, 2014). However, it is worth noting that none of the small landholders interviewed in this study regarded them as an important problem (see Silva Aparicio et al., 2003 for a similar case). In general, they mentioned that curculionids bit some of the stored grains, but the amount was not considered significant and these grains were generally used to feed fowl. Also, many of them said to avoid damage by storing the grains with local herbs and flowers which repel curculionids, while only a few of the more industrialized or market-oriented producers declared the use of industrial pesticides as part of their storage practices. In any case, none of the interviewed small landholders reported significant damage by curculionids in the field.
While our work provides significant insights into the relationship between agricultural management and beetle diversity, it has limitations that should be kept in mind. We only conducted one sampling (during the rainy season), which is restricted considering the high inter-annual, inter-geographic and inter-crop variability found in coleopterans (Finn et al., 1999; Andresen, 2003). For example, in another agroecosystem, Flores-Gutierrez et al. (2020) reported that arthropod abundance of herbivores was more than 100-times greater during the rainy season than during the dry season, so our results must be taken cautiously. Also, the use of sweep nets biases samples toward foliage-dwelling insects, so it would be advisable to use other sampling techniques in further studies, as they all have shortcomings and can complement each other (Doxon et al., 2011). Our sample size was relatively small (16 plots), although the collaboration of key informants and the recollection of a large amount of beetles seemed to compensate for this, as shown by the clear differences between management types and beetle diversity (see Blanco and Castro, 2007 and Álvarez et al., 2014 for discussions on sampling for qualitative information). Given that this was a field study, we had little control over environmental variables, which made statistical replicates impossible and limited the analysis possibilities. As a counterpart, those results that were statistically significant even under the great amount of environmental noise are probably a reflection of strong tendencies.
A previous work in Zaachila, Oaxaca, has shown that patches with different land use types, such as agricultural patches, grasslands and forests, all harbor a high beetle diversity but that the species arrangement is markedly different in each of them (Ramírez-Ponce et al., 2019). In this study we were able to further differentiate agricultural land uses by organizing the large heterogeneity of management practices into two broad types. From these types, the one characterized by the use of local landraces and little external inputs harbored a larger coleopteran diversity than its more industrialized counterpart. In another work, we showed that Zaachila's landscape is characterized by a high spatial heterogeneity given by small and multiple patches, a feature that is probably shared by other areas in the tropics with similar historic and physical characteristics, and that differentiates them from the more commonly studied landscapes of the temperate regions of the world (Urrutia et al., 2020). We also showed that agricultural patches, especially rainfed-agriculture patches, dominate this rural landscape and that, given their atomized pattern and large connectivity, they play a central role in the potential migration and recolonization among the adjacent forest patches (Urrutia et al., 2020). Together, these two studies suggest that a traditional management of the agricultural patches of Zaachila contributes to a high quality matrix for biodiversity conservation. We believe that the results of this work are relevant for the conservation of biodiversity in other contexts where small landholders are still the principal actors of agriculture and where traditional practices still survive. These and other works support the key role of traditional campesino agriculture in the conservation of biodiversity, including agrobiodiversity and its cultural expressions in landscapes driven by small landholders (Perfecto et al., 2009; Mora Van Cauwelaert, 2017; Bellon et al., 2018), a matter that is crucial since most of the world's biodiversity lies in these regions.
Data Availability Statement
The datasets presented in this study can be found in online repositories. The names of the repository/repositories and accession number(s) can be found in the article/Supplementary Material.
Ethics Statement
The studies involving human participants were reviewed and approved by Ethics Committee of the Ecology Institute at UNAM. Written informed consent for participation was not required for this study in accordance with the national legislation and the institutional requirements.
Author Contributions
MB, LJ-B, CG and TL conceived the ideas and designed methodology. CG and TL collected the data. CG analyzed the data. CG and MB led the writing of the manuscript. All authors contributed to the article and approved the submitted version.
Funding
CG acknowledged the graduate program Posgrado en Ciencias Biológicas, Universidad Nacional Autónoma de México and CONACyT scholarship. MB acknowledged financial support from UNAM-DGAPA-PAPIIT (IN207819). LJ-B acknowledged financial support form UNAM-DGAPA-PAPIIT (IA202515) and was grateful for NOT being part of the Sistema Nacional de Investigadores.
Conflict of Interest
The authors declare that the research was conducted in the absence of any commercial or financial relationships that could be construed as a potential conflict of interest.
Acknowledgments
The authors thank members of La Parcela Laboratory, Karen I. Carrasco Espinosa, Julieta Rosell, Ek del Val and the two reviewers for their valuable comments and suggestions. Raymundo Aguilar, Alexandre Beaupré, Maria de Guadalupe León, members of El Molote collective and the Regiduría de agricultura del Municipio de Villa de Zaachila helped in the field trips and recognition of the plots. This manuscript has been released as a pre-print at Biorxiv (González González et al., 2020).
Supplementary Material
The Supplementary Material for this article can be found online at: https://www.frontiersin.org/articles/10.3389/fsufs.2020.590720/full#supplementary-material
Footnotes
1. ^The equation that relates alpha and H-alpha is H-alpha=1/(1-alpha)*log(sum(pi∧alpha)). For more details, look at the work by Tóthmérész (1995) and Jost (2006).
References
Altieri, M., Hecht, S., Liebman, M., Magdoff, R., Norgaard, R., and Sikor, T. O. (1997). Agroecología: Bases científicas para una agricultura sustentable (No. F08 A48). Lima (Peru): Centro de Investigación, Educación y Desarrollo. La Paz (Bolivia): Secretariado Rural Peru-Bolivia.
Álvarez, S., Paas, W., Descheemaeker, K., Tittonell, P., and Groot, J. C. J. (2014). Construcción de tipologías, una forma de manejar la diversidad de las fincas: directrices generales para Humidtropics. Informe para el Programa de Investigación de CGIAR sobre Sistemas de los Trópicos Húmedos. Países Bajos: Grupo de Ciencias de las Plantas, Universidad de Wageningen, Paìses Bajos.
Andow, D. A., and Hidaka, K. (1989). Experimental natural history of sustainable agriculture: syndromes of production. Agric. Ecosyst. Environ. 27, 447–462. doi: 10.1016/0167-8809(89)90105-9
Andresen, E. (2003). Effect of forest fragmentation on dung beetle communities and functional consequences for plant regeneration. Ecography 26, 87–97. doi: 10.1034/j.1600-0587.2003.03362.x
Bellon, M. R., Mastretta-Yanes, A., Ponce-Mendoza, A., Ortiz-Santamaría, D., Oliveros-Galindo, O., Perales, H., et al. (2018). Evolutionary and food supply implications of ongoing maize domestication by Mexican campesinos. Proc. R. Soc. B Biol. Sci. 285:20181049. doi: 10.1098/rspb.2018.1049
Bianchi, F. J. J. A., Booij, C. J. H., and Tscharntke, T. (2006). Sustainable pest regulation in agricultural land- scapes: a review on landscape composition, biodiversity and natural pest control. Proc. R. Soc. Ser. B 273, 1715–1727. doi: 10.1098/rspb.2006.3530
Blanco, M. C. M. C., and Castro, A. B. S. (2007). El muestreo en la investigación cualitativa. Madrid: Nure Investigación, 27.
Bouchard, P., Bousquet, Y., Davies, A. E., Alonso-Zarazaga, M. A., Lawrence, J. F., Lyal, C. H., et al. (2011). Family-group names in Coleoptera (Insecta). ZooKeys 88, 1–972. doi: 10.3897/zookeys.88.807
Brooks, D. R. J. E., Bater, S. J., Clark, D. T., Monteith, C., Andrews, S. J., Corbett, D. A., et al. (2012). Large carabid beetle declines in a United Kingdom monitoring network increases evidence for a widespread loss in insect biodiversity. J. Appl. Ecol. 49, 1009–1019. doi: 10.1111/j.1365-2664.2012.02194.x
Burgio, G., Sommaggio, D., Marini, M., Puppi, G., Chiarucci, A., Landi, S., et al. (2015). The influence of vegetation and landscape structural connectivity on butterflies (Lepidoptera: Papilionoidea and Hesperiidae), carabids (Coleoptera: Carabidae), syrphids (Diptera: Syrphidae), and sawflies (Hymenoptera: Symphyta) in Northern Italy farmland. Environ. Entomol. 44, 1299–1307. doi: 10.1093/ee/nvv105
Campanelli, G., and Canali, S. (2012). Crop Production and environmental effects in conventional and organic vegetable farming systems: tThe case of a long-term experiment in Mediterranean conditions (Central Italy). J. Sustain. Agric. 36:599–619. doi: 10.1080/10440046.2011.646351
CEMDA (2017). Report on the biocultural relevance of Mexico's legislation and public policy on agriculture: the case of the “sustainable modernization of traditional agriculture” (MasAgro) program. Centro Mexicano de Derecho Ambiental, Mexico City, Mexico, 146.
Chaplin-Kramer, R., O'Rourke, M. E., Blitzer, E. J., and Kremen, C. (2011). A meta-analysis of crop pest and natural enemy response to landscape complexity. Ecol. Lett. 14, 922–932. doi: 10.1111/j.1461-0248.2011.01642.x
Cizek, O., Zamecnik, J., Tropek, R., Kokarek, P., and Konvicka, M. (2012). Diversification of mowing regime increases arthropods diversity in species-poor cultural hay meadows. J. Insect. Conserv. 16, 215–226 doi: 10.1007/s10841-011-9407-6
Del Río, M., Montes, F., Cañellas, I., and Montero, G. (2003). Revisión: Índices de diversidad estructural en masas forestales. Investigación agraria: Sistemas y recursos forestales 12, 159–176. doi: 10.1016/j.tree.2019.04.002
Doxon, E. D., Davis, C. A., and Fuhlendorf, S. D. (2011). Comparison of two methods for sampling invertebrates: vacuum and sweep-net sampling. J. Field Ornithol. 82, 60–67. doi: 10.1111/j.1557-9263.2010.00308.x
Duelli, P., Obrist, M. K., and Schmatz, D. R. (1999). “Biodiversity evaluation in agricultural landscapes: above-ground insects,” in En Invertebrate Biodiversity as Bioindicators of Sustainable Landscapes, ed M. G. Paoletti (Amsterdam: Elsevier), 33–64. doi: 10.1016/B978-0-444-50019-9.50006-6
Ekroos, J., Heliölä, J., and Kuussaari, M. (2010), Homogenization of lepidopteran communities in intensively cultivated agricultural landscapes. J. Appl. Ecol. 47, 459–467. doi: 10.1111/j.1365-2664.2009.01767.x
Fabian, Y., Sandau, N., Bruggisser, O. T., Aebi, A., Kehrli, P., Rohr, R. P., et al. (2013). The importance of landscape and spatial structure for hymenopteran-based food webs in an agro-ecosystem. J. Anim. Ecol. 82, 1203–1214. doi: 10.1111/1365-2656.12103
Fahrig, L., Baudry, J., Brotons, L., Burel, F. G., Crist, T. O., Fuller, R. J., et al. (2011). Functional landscape heterogeneity and animal biodiversity in agricultural landscapes. Ecol. Lett. 14, 101–112. doi: 10.1111/j.1461-0248.2010.01559.x
Finn, J., Gittings, T., and Giller, P. (1999). Spatial and temporal variation in species composition of dung beetle assemblages in southern Ireland. Ecol. Entomol. 24, 24–36. doi: 10.1046/j.1365-2311.1999.00169.x
Flores-Gutierrez, A. M., Mora, F., Avila-Cabadilla, L. D., Boege, K., and del-Val, E. (2020). Assessing the cascading effects of management and landscape on the arthropod guilds occurring in papaya plantations. Agricul. Ecosyst. Environ. 293:106836. doi: 10.1016/j.agee.2020.106836
Gabriel, D., Sait, S. M., Hodgson, J. A., Schmutz, U., Kunin, W. E., and Benton, T. G. (2010). Scale matters: the impact of organic farming on biodiversity at different spatial scales. Ecol. Lett. 13, 858–869. doi: 10.1111/j.1461-0248.2010.01481.x
Gallé, R., Happe, A. K., Baillod, A. B., Tscharntke, T., and Batáry, P. (2019). Landscape configuration, organic management, and within-field position drive functional diversity of spiders and carabids. J. Appl. Ecol. 56, 63–72. doi: 10.1111/1365-2664.13257
GIAHS-FAO (2020). Globally Important Agricultural Heritage Systems (GIAHS). Food and Agriculture Organization of the United Nations. Available online at: http://www.fao.org/giahs/en/
Gliessman, S. R. (2015). Agroecology: The Ecology of Sustainable Food Systems. Boca Raton, FL: CRC Press. doi: 10.1201/b17881
González González, C., Lara García, T., Jardón-Barbolla, L., and Mariana, B. (2020). Linking coleopteran diversity with agricultural management of maize agroecosystems in Oaxaca, Mexico. bioRxiv. bioRxiv 2020.01.07.897744. doi: 10.1101/2020.01.07.897744
Happe, A. K., Alins, G., Blüthgen, N., Boreux, V., Bosch, J., García, D., et al. (2019). Predatory arthropods in apple orchards across Europe: responses to agricultural management, adjacent habitat, landscape composition and country. Agric. Ecosyst. Environ. 273, 141–150. doi: 10.1016/j.agee.2018.12.012
Holland, J. M., and Luff, M. L. (2000). The effects of agricultural practices on Carabidae in temperate agroecosystems. Integr. Pest Manage. Rev. 5, 109–129. doi: 10.1023/A:1009619309424
Hunt, T., Bergsten, J., Levkanicova, Z., Papadopoulou, A., John, O. S., Wild, R., et al. (2007). A comprehensive phylogeny of beetles reveals the evolutionary origins of a superradiation. Science 318, 1913–1916. doi: 10.1126/science,.1146954
INEGI, V. (2007). Censo Agrícola, Ganadero y Forestal 2007. Aguascalientes: Instituto Nacional de Estadística, Geografía e Informática.
Isbell, F., Adler, P. R., Eisenhauer, N., Fornara, D., Kimmel, K., Kremen, C., et al. (2017). Benefits of increasing plant diversity in sustainable agroecosystems. J. Ecol. 105, 871–879. doi: 10.1111/1365-2745.12789
Karp, D. S., Chaplin-Kramer, R., Meehan, T. D., Martin, E. A., DeClerck, F., Grab, H., et al. (2018). Crop pests and predators exhibit inconsistent responses to surrounding landscape composition. Proc. Nat. Acad. Sci. 115, 7863–7870. doi: 10.1073/pnas.1800042115
Knight, A. L., and Norton, G. W. (1989). Economics of agricultural pesticide resistance in arthropods. Annu. Rev. Entomol. 34, 293–313. doi: 10.1146/annurev.en.34.010189.001453
Krauss, J., Gallenberger, I., and Steffan-Dewenter, I. (2011). Decreased functional diversity and biological pest control in conventional compared to organic crop fields. PLoS ONE 6:e0019502. doi: 10.1371/journal.pone.0019502
Kremen, C., and Merenlender, A. M. (2018). Landscapes that work for biodiversity and people. Science 362:eaau6020. doi: 10.1126/science.aau6020
Kromp, B. (1999). “Carabid beetle in sustainable agriculture: a review on pest control efficacy, cultivation impacts and enhancement,” in En Invertebrate biodiversity as bioindicators of sustainable landscapes, ed. M. G. Paoletti (Amsterdam: Elsevier), 187–228. doi: 10.1016/B978-0-444-50019-9.50014-5
Lassau, S. A., Hochuli, D. F., Cassis, G., and Reid, C. A. (2005). Effects of habitat complexity on forest beetle diversity: do functional groups respond consistently?. Divers. Distribut. 11, 73–82. doi: 10.1111/j.1366-9516.2005.00124.x
Letourneau, D. K., Armbrecht, I., Salguero-Rivera, B., Montoya-Lerma, J., Jiménez-Carmona, E., Constanza-Daza, M., et al. (2011). Does plant diversity benefit agroecosystems? A synthetic review. Ecol. Appl. 21, 9–21. doi: 10.1890/09-2026.1
Liere, H., Jha, S., and Philpott, S. M. (2017). Intersection between biodiversity conservation, agroecology, and ecosystem services. Agroecol. Sustain. Food Syst. 41, 723–760. doi: 10.1080/21683565.2017.1330796
Lindenmayer, D., Cunningham, S., and Young, A., (eds.). (2012). Land Use Intensification: Effects on Agriculture, Biodiversity and Ecological Processes. Clayton: CSIRO publishing. doi: 10.1071/9780643104082
Lyver, P.O., Timoti, P., Davis, T., and Tylianakis, J. M. (2019). Biocultural histeresis inhibits adaptation to environmental change. Trends Ecol. Evol. 34, 771–780. Available online at: https://recyt.fecyt.es/index.php/IA/article/view/2490?fbclid=IwAR303ElbBaxZHxqQUdjt4AlAIKmanIrgHOLHBeS4SNwDzBnDkWPmh07jdSE
Marshall, M. N. (1996a). The key informant technique. Fam. Pract. 13, 92–97. doi: 10.1093/fampra/13.1.92
Marshall, M. N. (1996b). Sampling for qualitative research. Fam. Pract. 13, 522–526. doi: 10.1093/fampra/13.6.522
Meehan, T. D., Werling, B. P., Landis, D. A., and Gratton, C. (2011). Agricultural landscape simplification and insecticide use in the Midwestern United States. Proc. Natl. Acad. Sci. U.S.A. 108, 11500–11505. doi: 10.1073/pnas.1100751108
Mora Van Cauwelaert, E. (2017). Diagnóstico del movimiento comercial del maíz y de las relaciones económicas y culturales-simbólicas para la siembra del maíz criollo en la Villa de Zaachila, Oaxaca. Un enfoque desde las familias campesinas. Masters thesis. Universidad Internacional de Andalucía, España.
Morrone, J. J. (2014). Biodiversidad de Curculionoidea (Coleoptera) en México. Rev. Mex. Biodivers 85, 312–324. doi: 10.7550/rmb.30038
Myers, N., Mittermeier, R. A., Mittermeier, C. G., Da Fonseca, G. A., and Kent, J. (2000). Biodiversity hotspots for conservation priorities. Nature 403:853. doi: 10.1038/35002501
Nabhan, G. P., and Buchmann, S. L. (1997). “Services provided by pollinators,” in Nature's Services; Societal Dependence on Natural Ecosystems, ed G. C. Daily (Washington, DC: Island Press), 133–150.
Naylor, R. L., and Erlich, P. (1997). “Natural pest control services and agriculture,” in Nature's Services; Societal Dependence on Natural Ecosystems, ed G. C. Daily (Washington, DC: Island Press), 151–174.
Ohsawa, M. (2010). Beetle families as indicators of Coleopteran diversity in forests: a study using Malaise traps in the central mountainous region of Japan. J. Insect Conserv. 14, 479–484. doi: 10.1007/s10841-010-9276-4
Oviedo, G. (ed). (2002). The Community Protected Natural Areas in the State of Oaxaca, Mexico. Gland: WWF.
Pearson, D. L., and Cassola, F. (1992). World-wide species richness patterns of tiger beetles (Coleoptera: Cicindelidae): indicator taxon for biodiversity and conservation studies. Conserv. Biol. 6, 376–391. doi: 10.1046/j.1523-1739.1992.06030376.x
Perfecto, I. (2003). Conservation Biology and Agroecology: De un Pájaro las dos Alas. Endangered Species Update 20, 4–5. Available online at: https://go.gale.com/ps/anonymous?id=GALE%7CA114168537&sid=googleScholar&v=2.1&it=r&linkaccess=abs&issn=10813705&p=AONE&sw=w
Perfecto, I., Vandermeer, J. H., and Wright, A. L. (2009). Nature's Matrix: Linking Agriculture, Conservation and Food Sovereignty. Boca Raton, FL: Routledge. doi: 10.4324/9781849770132
Pizzolotto, R., Mazzei, A., Bonacci, T., Scalercio, S., Iannotta, N., and Brandmayr, P. (2018). Ground beetles in Mediterranean olive agroecosystems: their significance and functional role as bioindicators (Coleoptera, Carabidae). PLoS ONE 13:e0194551. doi: 10.1371/journal.pone.0194551
Poveda, K., Martínez, E., Kersch-Becker, M. F., Bonilla, M. A., and Tscharntke, T. (2012). Landscape simplification and altitude affect biodiversity, herbivory and Andean potato yield. J. Appl. Ecol. 49, 513–522. doi: 10.1111/j.1365-2664.2012.02120.x
Power, A. G. (2010). Ecosystem services and agriculture: tradeoffs and synergies. Phil. Trans. R. Soc. B 365, 2959–2971. doi: 10.1098/rstb.2010.0143
Qiu, J. (2019). Effects of landscape pattern on pollination, pest control, water quality, flood regulation, and cultural ecosystem services: a literature review and future research prospects. Curr. Landsc. Ecol. Rep. 4, 113–124. doi: 10.1007/s40823-019-00045-5
Qiu, Z., Kennen, J. G., Giri, S., Walter, T., Kang, Y., and Zhang, Z. (2019). Reassessing the relationship between landscape alteration and aquatic ecosystem degradation from a hydrologically sensitive area perspective. Sci. Total Environ. 650, 2850–2862. doi: 10.1016/j.scitotenv.2018.10.036
R Core Team (2014). R: A Language and Environment for Statistical Computing. Vienna: R Foundation for Statistical Computing. Available online at: http://www.R-project.org/ (accessed December 2, 2020).
Ramírez-Ponce, A., Calderón-Patrón, J. M., Vásquez, H. M. G., and Moreno, C. E. (2019). Biotic heterogeneity among scarab beetle communities in an anthropized landscape in the Central Valleys of Oaxaca, Mexico. J. Insect Conserv. 23, 765–776. doi: 10.1007/s10841-019-00169-3
Ramos, I. (2020). La heterogeneidad espacial en regiones de México con distitnas estrategias de producción y conservación: tendencias en el tiempo y su relación con la conservación de la biodiversidad (Tesis de maestría). Universidad Nacional Autónoma de México, México.
Rusch, A., Chaplin-Kramer, R., Gardiner, M. M., Hawro, V., Holland, J., Landis, D., et al. (2016). Agricultural landscape simplifica- tion reduces natural pest control: a quantitative synthesis. Agric. Ecosyst. Environ. 221, 198–204. doi: 10.1016/j.agee.2016.01.039
Sharma, S., Kooner, R., and Arora, R. (2017). “Insect pests and crop losses,” in Breeding Insect Resistant Crops for Sustainable Agriculture, eds R. Arora, and S. Sandhu (Singapore: Springer), 45–66. doi: 10.1007/978-981-10-6056-4_2
Silva Aparicio, M., Castro Ramírez, A. E., León Cortés, J. L., and Ishiki Ishihara, M. (2003). Entomofauna asociada a maíz de temporal con diferentes manejos de malezas en Chiapas, México.
Theiling, K. M., and Croft, B. A. (1988). Pesticide side-effects on arthropod natural enemies: a database summary. Agric. Ecosyst. Environ. 21, 191–218. doi: 10.1016/0167-8809(88)90088-6
Tooker, J. F., and Frank, S. D. (2012). Genotypically diverse cultivar mixtures for insect pest management and increased crop yields. J. Appl. Ecol. 49, 974–985. doi: 10.1111/j.1365-2664.2012.02173.x
Torcat-Fuentes, M., Lenardis, A., and de la Fuente, E. (2018). Insect assemblies related to volatile sig- nals emitted by different soybean – weeds – herbivory combinations. Agric. Ecosyst. Environ. 255, 20–26. doi: 10.1016/j.agee.2017.12.007
Tóthmérész, B. (1995). Comparison of different methods for diversity ordering. J. Veget. Sci. 6, 283–290. doi: 10.2307/3236223
Tripplehorn, C. A., and Johnson, N. F. (2005). Borror and DeLong's Introduction to the Study of Insects. Belmont, CA: Thomson Brooks/Cole.
Tscharntke, T., Clough, Y., Wanger, T. C., Jackson, L., Motzke, I., Perfecto, I., et al. (2012). Global food security, biodiversity conservation and the future of agricultural intensifica- tion. Biol. Conserv. 151, 53–59. doi: 10.1016/j.biocon.2012.01.068
Tylianakis, J. M., Tscharntke, T., and y Lewis, O. T. (2007). Habitat modification alters the structure of tropical host-parasitoid food webs. Nat. Lett. 441, 202–205. doi: 10.1038/nature05429
Urrutia, A. L., González-González, C., Van Cauwelaert, E. M., Rosell, J. A., Barrios, L. G., and Benítez, M. (2020). Landscape heterogeneity of peasant-managed agricultural matrices. Agric. Ecosyst. Environ. 292:106797. doi: 10.1016/j.agee.2019.106797
Vandermeer, J., Perfecto, I., and Philpott, S. (2010). Ecological complexity and pest control in organic coffee production: uncovering an autonomous ecosystem service. Bioscience 60, 527–537. doi: 10.1525/bio.2010.60.7.8
Vandermeer, J. H. (2011). The Ecology of Agroecosystems. Burlington, MA : Jones and Bartlett Learning.
Wan, N. F., Ji, X. Y., Deng, J. Y., Podenphant Kiaer, L., Cai, Y. M., and Jiang, J. X. (2019). Plant diversification promotes biocontrol services in peach orchards by shaping the ecological niches of insect her- bivores and their natural enemies. Ecol. Indic. 99, 387–392. doi: 10.1016/j.ecolind.2017.11.047
Wilson, S., Mitchell, G. W., Pasher, J., McGovern, M., Hudson, M. A. R., and Fahrig, L. (2017). Influence of crop type, heterogeneity and woody structure on avian biodiversity in agricultural landscapes. Ecol. Indic. 83, 218–226. doi: 10.1016/j.ecolind.2017.07.059
Winter, S., Bauer, T., Strauss, P., Kratschmer, S., Paredes, D., Popescu, D., et al. (2018). Effects of vegetation management intensity on biodiversity and ecosystem services in vineyards: a meta-analysis. J. Appl. Ecol. 55, 2484–2495. doi: 10.1111/1365-2664.13124
Zhang, W., Ricketts, T. H., Kremen, C., Carney, K., and Swinton, S. M. (2007). Ecosystem services and dis- services to agriculture. Ecol. Econ. 64, 253–260. doi: 10.1016/j.ecolecon.2007.02.024
Keywords: agroecological matrix, agricultural management, typology, Oaxaca Mexico, Coleoptera (beetles)
Citation: González González C, Lara García T, Jardón-Barbolla L and Benítez M (2020) Linking Coleopteran Diversity With Agricultural Management of Maize-Based Agroecosystems in Oaxaca, Mexico. Front. Sustain. Food Syst. 4:590720. doi: 10.3389/fsufs.2020.590720
Received: 02 August 2020; Accepted: 24 November 2020;
Published: 22 December 2020.
Edited by:
Klaus Birkhofer, Brandenburg University of Technology Cottbus-Senftenberg, GermanyReviewed by:
Luciana Iannuzzi, Federal University of Pernambuco, BrazilAdrien Rusch, Institut National de la Recherche Agronomique (INRA), France
Copyright © 2020 González González, Lara García, Jardón-Barbolla and Benítez. This is an open-access article distributed under the terms of the Creative Commons Attribution License (CC BY). The use, distribution or reproduction in other forums is permitted, provided the original author(s) and the copyright owner(s) are credited and that the original publication in this journal is cited, in accordance with accepted academic practice. No use, distribution or reproduction is permitted which does not comply with these terms.
*Correspondence: Mariana Benítez, mbenitez@iecologia.unam.mx