Bioinoculants as Promising Complement of Chemical Fertilizers for a More Sustainable Agricultural Practice
- 1Department of Soil, Plant and Food Sciences, University of Bari Aldo Moro, Bari, Italy
- 2Faculty of Science and Technology, Free University of Bolzano-Bozen, Bolzano, Italy
- 3Competence Centre of Plant Health, Free University of Bolzano-Bozen, Bolzano, Italy
Plant Growth Promoting Rhizobacteria (PGPR) represent a heterogeneous group of bacteria, which have been characterized for their ability to influence the growth and the fitness of agricultural plants. In the quest of more sustainable practices, PGPR have been suggested as a valid complement for the agronomical practices, since they can influence several biochemical and molecular mechanisms related to the mineral nutrients uptake, the plant pathogens suppression, and the phytohormones production. Within the present work, three bacterial strains, namely Enterobacter asburiae BFD160, Pseudomonas koreensis TFD26, and Pseudomonas lini BFS112, previously characterized on the basis of distinctive PGPR traits, were tested to evaluate: (i) their persistence in soil microcosms; (ii) their effects on seeds germination; (iii) their possible influence on biochemical and physiological parameters related to plant growth, fruit quality, and plant nutrient acquisition and allocation. To these aims, two microcosms experiments featuring different complexities, i.e., namely a growth chamber and a tunnel, were used to compare the effects of the microbial inoculum to those of chemical fertilization on Cucumis sativus L. plants. In the growth experiment, the Pseudomonas spp. induced positive effects on both growth and physiological parameters; TFD26, in particular, induced an enhanced accumulation of mineral nutrients (Fe, Ca, Mn, Ni, Zn) in plant tissues. In the tunnel experiment, only P. koreensis TFD26 was selected as inoculum for cucumber plants used in combination or in alternative to a chemical fertilizer. Interestingly, the inoculation with TFD26 alone or in combination with half-strength chemical fertilizer could induce similar (e.g., Ca accumulation) or enhanced (e.g., micronutrients concentration in plant tissues and fruits) effects as compared to plants treated with full-strength chemical fertilizers. Overall, the results hereby presented show that the use of PGPR can lead to comparable, and in some cases improved, effects on biochemical and physiological parameters of cucumber plants and fruits. Although these data are referred to experiments carried out in controlled condition, though different from an open filed cultivation, our observations suggest that the application of PGPR and fertilizers mixtures might help shrinking the use of chemical fertilization and potentially leading to a more sustainable agricultural practice.
Introduction
The excessive and continuous application of chemical fertilizers has often compromised soil chemical and biological properties causing pollution of soil ecosystems and detrimental effects on human health (Zhang et al., 2018). Quite often, agricultural soil suffers chemical and biological degradation due to nutrient and biodiversity depletion (Kopittke et al., 2019). Thus, such inappropriate soil fertility often leads to the compulsory use of pesticides and chemical fertilizers as well as to high costs of agricultural management in order to ensure an adequate crop productivity. In the presented scenario, the quest of more sustainable agricultural practices has drawn the attention to the biological potential of the interactions between crops and microorganisms inhabiting the rhizosphere (Pii et al., 2015b; Mimmo et al., 2018). It is well-known that microorganisms have a key role in many biotic mechanisms essential to preserve the biological and physiochemical balance in soil (Alegria-Terrazas et al., 2016). Thus, the investigation on the dynamics underlying the plant-microbiome interactions are crucial to achieve sustainable innovations in agriculture and has recently become a main scientific Research Topic. In particular, research efforts have been focused on that group of bacteria commonly termed Plant Growth-Promoting Rhizobacteria (PGPR) that naturally inhabits in close association with roots and benefits their host improving their growth by several mechanisms (Saharan and Nehra, 2011). The growth enhancements brought about by PGPR can be the result of either indirect actions (e.g., preventing the harmful effects of phytopathogenic organisms via the production of antagonistic substances or thanks to the stimulation of the plant systemic resistance), or direct mechanisms, such as the production of bioactive molecules (e.g., phytohormones) and/or enhancing the nutrient bioavailability at the rhizosphere (Crecchio et al., 2018). Several pieces of research have also highlighted that PGPR might contribute to improve plants growth by increasing their tolerance to abiotic stressors (Jetiyanon and Kloepper, 2002; Zhang et al., 2008a, 2010; Dimkpa et al., 2009; Chithrashree et al., 2011; Marastoni et al., 2019) and by modulating the physiological and biochemical activities of plants, especially those underlying the acquisition of mineral nutrient (Zhang et al., 2008b; Xie et al., 2009; Pii et al., 2015b, 2016b, 2018; Scagliola et al., 2016; Kolega et al., 2020). In this context, the employment of PGPR, alone or in combination with synthetic fertilizers, with the aim of enhancing nutrients acquisition in plants and, more in general, of increasing the use efficiency of endogenous soil sources of nutrients, might help to shrink the use of agrochemicals, as also foreseen by the green deal concept for an increasingly sustainable agriculture. In this sense, several studies have demonstrated that the inoculation of PGPR, in complete or partial replacement of chemical fertilizers, increases growth and yield of many commercial plants such as tomato (Hernandez and Chailloux, 2004), strawberry (Bona et al., 2015), curcuma (Kumar et al., 2016), cucumber (Saeed et al., 2015), sunflower (Arif et al., 2016), and cotton (Gomathy et al., 2008; Dhale et al., 2010; Nalayini et al., 2010).
It is also worth mentioning that PGPR inoculation in crops has been shown to impact the production of secondary metabolites, thus affecting the overall nutraceutical value of the primary agricultural products. Indeed, several authors assessed that PGPR inoculation positively affected the quality of both fruit (e.g., mulberry, strawberry, citrus, apricot sweet cherry, and raspberry) (Eşitken et al., 2002, 2003, 2005, 2006; Orhan et al., 2006; Pii et al., 2018) and aromatic herbs (Banchio et al., 2009; Erika et al., 2010; Santoro et al., 2011; Heidari and Golpayegani, 2012; Cappellari et al., 2013, 2019a,b; Kolega et al., 2020) in terms of health-promoting compounds. However, very often the effects produced by PGPR inoculation on plant growth as well as on the qualitative and quantitative profile of secondary metabolites have been observed to be strongly dependent on the specific combination of plant species, microbial strain, and cultivation conditions. This possibly indicates the existence of specific interaction mechanisms, which can eventually lead to different outcomes in crops (Cappellari et al., 2019a).
On the basis of these premises, the aim of the present research was to assess the impact of different PGPR strains on cucumber plants grown in soil-based microcosms. In a previous work (Pii et al., 2016a; Scagliola et al., 2016), 80 bacterial colonies have been isolated from the rhizosphere of barley (Hordeum vulgare L.) and tomato (Solanum lycopersicon L.) plants grown in a calcareous soil, naturally featuring a low Fe availability. The bacterial strains were characterized for their PGPR traits, namely solubilization of phosphate, production of siderophores, and indole-3-acetic acid (Scagliola et al., 2016). Very often, it is reported in the literature that the outcomes of the microbe-plants interaction are strongly dependent on both the microbial strain and the plant species. With the aim of investigating whether the growth promotion properties of the isolated strains were restricted to barley and tomato or not, we decided to carry out further experiments on a different horticultural plant. Among the 80 bacterial colonies, 12 selected isolates, concurrently showing the best PGPR traits, were tested in vivo on hydroponically grown cucumber (Cucumis sativus L.) plants, in order to evaluate their ability to influence the biochemical and molecular mechanisms underpinning the Fe acquisition mechanisms at root level (Scagliola et al., 2016), as previously demonstrated for the model PGPR Azospirillum brasilense (Pii et al., 2015c, 2016b). On the base of the results presented by Scagliola et al. (2016), the three strains showing the best PGP features in the in vivo experiment were chosen to inoculate soil-grown cucumber plants. Therefore, the present experiments represent the natural prosecution of the research undertaken, from hydroponic growth system to soil-based systems.
Materials and Methods
Plant Growth-Promoting Rhizobacteria Growth Conditions and Inoculation
The three strains used in this work, identified as described below, are BFD160 E. asburiae, TFD26 P. koreensis, and BFS112 P. linii. They were routinely grown at 28°C in Nutrient Broth (Oxoid) for 24 h at 120 rpm. The strains, able to solubilize phosphates and to produce siderophores and indole-3-acetic acid, had been previously isolated from the rhizosphere of barley and tomato plants, grown in a calcareous soil by the RHIZOtest system (Bravin et al., 2010), and characterized on the basis of PGP features (Scagliola et al., 2016).
16S rDNA Gene Sequencing
DNA extraction was performed by thermal shock, heating the PGPR colonies in 50 μL of distilled water at 95°C for 15 min (Maniatis et al., 1982). The 16S rDNA gene PCR amplifications were carried out using two couples of universal primers E8F-E926R e E786R-E1541R (Baker et al., 2003). The PCR mix (50 μL) contained 50 pmol of each primer, 10 nmol of each 2′-deoxynucleoside 5′-triphosfate, 3 U of Taq DNA polymerase (EuroTaq; EuroClone), 2.5 mM MgCl2, and 25–50 ng of template DNA. All PCR amplifications were performed using a MyCycler™ thermal cycler (Bio-Rad Laboratories Inc.). Each PCR product was analyzed by electrophoresis in a 1.5% (w/v) TEAE agarose gel. Bands of interest, 918 and 755 bp long, were also excised from the gel and were purified using the Wizard® SV Gel and PCR Clean-Up System kit (Promega). The amplicons were sequenced from both ends by Eurofins Genomics (Milan, Italy). 16S rDNA sequences were aligned using the BLASTn tool (Camacho et al., 2009) against the NCBI database (www.ncbi.nlm.nih.gov) to identify the species of bacterial strains.
Phytotoxicity Test
Seed germination tests were used to assess the phytotoxicity of the three selected strains. Cucumber seeds (Cucumis sativus L. cv Chinese Long) were surface sterilized by washing with 3% (v/v) sodium hypochlorite and then with sterilized distilled water. One hundred seeds were incubated in a bacterial suspension of each PGPR strains (108 cfu mL−1) for 4 h at 28°C. Control seeds were incubated in sterile Nutrient Broth (Oxoid). The germination was carried out for 5 days in the dark at 22°C, placing the seeds between two layers of filter paper moistened with 0.5 mM CaSO4 (Stefan et al., 2008). The results were expressed as the ratio of the number of germinated seeds to the total number of tested seeds.
Persistence Assay in Soil
The PGPR strains were made competent chemically (Cohen et al., 1972) and labeled with the Green Fluorescent Protein (gfp) by in vitro transformation (Zhao et al., 2013) using the pGLOTM plasmid (BIO-RAD). The transformants were selected on Nutrient Broth (Oxoid) supplemented with 0.5% (w/v) L-arabinose and 0.01% (w/v) ampicillin and preserved at −80°C in 20% (v/v) glycerol. The stability of plasmid maintenance was evaluated by growing the transformed clones for 40 days under non-selective conditions at 28°C. Every 3 days the presence of the plasmid was ascertained by plating aliquots of the clones on selective and differential media (Nutrient Broth (Oxoid) supplemented with 0.5% (w/v) L-arabinose and 0.01% (w/v) ampicillin), then the liquid colture was centrifuged and the growth medium renewed. Having verified the long-term stability of the plasmid (40 days), the tagged strains were used in soil microcosms by inoculating fresh soil with 108 cfu g−1DW incubated for 30 days at 28°C. To evaluate and quantify the presence of the tagged strains, the bacterial communities were desorbed from soil by sonication and bacteria were isolated and counted by the standard dilution plating technique on Nutrient Agar (Oxoid) supplemented with 0.5% (w/v) L-arabinose and 0.01% (w/v) ampicillin after 24 h from inoculation and hereafter every 7 days. Three independent replicates were performed for each sampling time, in order not to alter the soil-microorganism system through sampling of soil aliquots.
Trial 1: Pot Experiment in Climatic Chamber
Cucumber seeds (Cucumis sativus L. cv Chinese Long), previously surface sterilized as before described, were germinated for 5 days in the dark at 22°C onto moist filter paper in 0.5 mM CaSO4 solution. Homogenous seedlings were then transferred in pots contained 70 g of a growth medium consisting of soil, sterilized peat and sterilized perlite at the ratio of 60:30:10. The soil was a sandy loam, classified as a Haplic Calcisol (FAO, 1998) collected from the South of Italy and had the following chemical-physical characteristic: pHH2O 7.4, Corg 7.0 g kg−1, Ntot 0.8 g kg−1, P 11.7 mg kg−1, and K 104 mg kg−1 (Sofo et al., 2010).
The experiment was performed in a climatic chamber with the following conditions: 18/6 h light/dark, 22°C and 44 μmol m−2 s−1 light intensity. The pots were inoculated with 7 mL of broth culture of each bacteria (BFD160 E. asburiae, TFD26 P. koreensis, and BFS112 P. linii) at 108 cfu mL−1 in the day of the transplant and after 15 days. The control pots were inoculated with the same amounts of sterile Nutrient Broth. The experiment was performed in six replicates in a complete randomized block design. After 30 days of cultivation, physiological parameters, such as photosynthetic rate, transpiration rate and stomatal conductance, were measured using the Li-6400 XT Portable Photosynthesis System (Licor Inc. Lincoln, NE, USA). Plant height (cm), root length (cm), above ground and root biomass (g), above ground and root water content (%), and the leaf chlorophyll content were recorded (Hiscox and Israelstam, 1979). To quantify the content of macro (P, S, K, Ca) and micro-nutrients (Fe, Zn, Mn, Ni, Cu, Br, Sr) of roots and leaves, the Total Reflection X-Ray Fluorescence Spectrometer (TXRF, Bruker S2 PICOFOX) was used.
Trial 2: Pot Experiment in Tunnel
On the basis of the results obtained in Trial 1, the best performing PGPR was used for further inoculation studies, together with a commercial fertilizer. Cucumber seeds (Cucumis sativus L. cv Chinese Long) were surface sterilized and germinated as described above. Homogenous seedlings were then transferred in 19 cm diameter pots, containing about 2 kg of soil, sterilized peat and sterilized perlite mixture at the ratio of 60:30:10 and arranged in a complete randomized block with six replicates for each treatment. Four treatments were performed: inoculation (Inoculated), half-strength fertigation (0.5x Fert Not-Inoculated), inoculation supplemented with half-strength fertigation (0.5x Fert Inoculated), and full-strength fertigation (Fert). The fertigation treatments were carried out using the commercial fertilizer Green-GO® 12:8:24 (N:P:K) + 10 CaO (Biolchim) (Supplementary Table 1) at the recommended rate of 5–15 Kg 1,000 m−2 In our study each pot received 0.06 g of fertilizer in 100 mL tap water, twice a week. The inoculum was performed once per week the day after the fertigation using 100 mL of 108 cfu mL−1 of TFD26 P. koreensis suspension. The fruits, collected during the cultivation, were freeze-dried using Laborota 4001 efficient (Heidolph) and preserved at −20°C for further analysis. After 60 days of cultivation the morphological and physiological assessments, as described for Trial 1, were carried out. In addition, C and N content of root and shoots were evaluated using elemental analyzer Flash EA 1112 and microbic biomass content of soil was determined by the standard chloroform fumigation extraction method (Brookes, 1995).
Nutraceuticals and Elemental Analyses of Fruits
Freeze-dried cucumber fruits samples were homogenized, and 100 mg of cucumber powder were extracted with 1 mL methanol (HPLC grade, Merck, Darmstadt, Germany). The mixtures were subjected to sonication for 30 min at 4°C and, afterwards, they were centrifuged at 14,000 xg for 30 min at 0°C; the supernatants were then collected and filtered through a 0.2 μm nylon filter, as previously described (Valentinuzzi et al., 2015).
Organic acids and sugars were separated simultaneously by HPLC equipped with a cation exchange column Aminex 87-H column (300 × 7.8 mm, 9 μm, Bio-Rad) using an isocratic elution with 10 mM H2SO4 as carrier solution at a flow rate of 0.6 mL min−1. Organic acids were detected at 210 nm using a Waters 2998 photodiode array detector (Waters Spa, Italy), whilst sugars were detected by a Waters 2414 refractive index detector (Waters Spa, Italy).
Lyophilized and ground cucumber tissues samples were mineralized with ultra-pure 68% HNO3 (Carlo Erba) using a Single Reaction Chamber Microwave (SRC, UltraWAVE, Milestone Inc, Shelton, CT, USA). Mineralized samples were diluted with distilled water, filtered, and elements concentration determined by inductively-coupled plasma - optical emission spectrometry – ICP-OES (Arcos Ametek, Spectro, Germany), using tomato leaves (SRM 1573a) and spinach leaves (SRM 1547) as external certified reference material, as previously described (Pii et al., 2015a).
Statistical Analyses
The results are reported as mean ± standard error (SE) of six independent biological replicates. The significance of differences among means was calculated by One-way ANOVA with post-hoc Tukey HSD with α = 0.05 using R software (version 3.6.0). The Principal Component Analysis (PCA) has been carried out by applying the prcomp function of the R software package ggfortify (Tang et al., 2016). The following R packages were used for data visualization and statistical analyses: ggplot2 v.3.2.0 (Wickham, 2016), Agricolae v.1.3-1 (de Mendiburu, 2019), and ggfortify (Tang et al., 2016).
Results
Strain Identification
The analyses of 16S rDNA sequences allowed to establish the species of BFD160, TFD26, and BFS112 clones as E. asburiae, P. koreensis, and P. linii, respectively (Table 1).

Table 1. Identification of bacterial strains used in the inoculation experiments on the base of 16S rDNA sequencing.
Phytotoxicity Test
The cucumber seeds incubated with the selected PGPR showed an inhibition of the germination rate by about 11, 15, and 9% for BFD160 E. asburiae, TFD26 P. koreensis, and BFS112 P. linii, respectively. However, these values resulted not statistically significant, when compared with the control seeds incubated in the sterile media (Supplementary Table 2).
Persistence Assay in Soil
The three clones, labeled using the method of plasmid transformation reported by Zhao et al. (2013), had maximum growth after 18 h of incubation at 28°C, similarly to the wild type strains. The stability assay of plasmid demonstrated that gfp-tagged strains completely maintained their marker genes up to 40 days, in absence of the selective medium, as all the colonies for each of the three stains grew on the selective and differential plates and appeared fluorescent under UV light at each assay up to 40 days of incubation (data not shown).
The numbers of PGPR cfu in the soil were counted after 24 h from the inoculation and then every week for 30 days on selective and differential medium. The results of PGPR persistence test showed that the number of bacteria in soil decreased gradually during the experiment until their complete absence. In detail, results showed that the initial density of 108 cfu g−1DW of PGPR decreased to 104 cfu g−1DW after 15 days and to 103 cfu g−1DW after 20 days with a trend perfectly comparable among all strains (data not shown). Consequently, the PGPR strains were repeatedly inoculated, depending on the duration and the complexity of the trials.
Trial 1
Effects of PGPR on Roots and Shoots of Plants Grown in Climatic Chamber
Growth parameters
Plant height, root length, above ground and root biomass, above ground and root water content of cucumber plants grown in inoculated soil microcosms were positively affected by the treatment, when compared to the control plants (Supplementary Table 2). In particular, the inoculation with TFD26 P. koreensis and BFS112 P. linii significantly enhanced the water content either of the above ground and of the root (Supplementary Table 2). Likewise, the chlorophyll a and b content resulted higher in the leaves of the plants grown in soil microcosms inoculated with the same strains (Supplementary Table 2). On the contrary, the effects of BFD160 E. asburiae were variable. Plants grown in soil microcosms treated with this PGPR exhibited an increment in plant height (+48, +27, and +17% with BFD160 E. asburiae, TFD26 P. koreensis, and BFS112 P. linii, respectively), water content (+2% with all the inoculants), and biomass (+50, +17, and +27% with BFD160 E. asburiae, TFD26 P. koreensis, and BFS112 P. linii, respectively), but showed slight decrease of leaf chlorophyll content. Interestingly, the same clone significantly reduced the root length of about 54% (Supplementary Table 2).
Physiological parameters
All the physiological activities were markedly enhanced by the employment of the three PGPR. In particular, the photosynthetic rate was incremented 1.7, 2.4, and 2.0 times in the plants grown in soil microcosms inoculated with BFD160 E. asburiae, TFD26 P. koreensis, and BFS112 P. linii, respectively, as compared with the non-treated plants. Furthermore, the treatment with TFD26 P. koreensis had a significant positive effect on the transpiration rate and stomatal conductance, too, which were increased 3.2 and 3.4 times, respectively (Supplementary Table 2).
Micro and macronutrient content
The data of the micro and macronutrient content are summarized in Supplementary Table 3. The most considerable result was the positive effect determined by the treatment of the soil microcosms with TFD26 P. koreensis, which increased the content of about all elements, either in leaves or in roots, with particular emphasis for Fe, whose concentration was enhanced by about 37.5 and 54% in leaves and in roots, respectively.
Trial 2
Effects of PGPR on Roots and Shoots of Plants Grown in Tunnel
Growth parameters
The chemical fertilization at both doses, with and without the inoculum, decreased the water content of roots; no statistically significant variation among treatments was observed in shoots (Figure 1). Shoots length and thickness were not significantly affected by fertilization and/or inoculum, too (Supplementary Table 4).
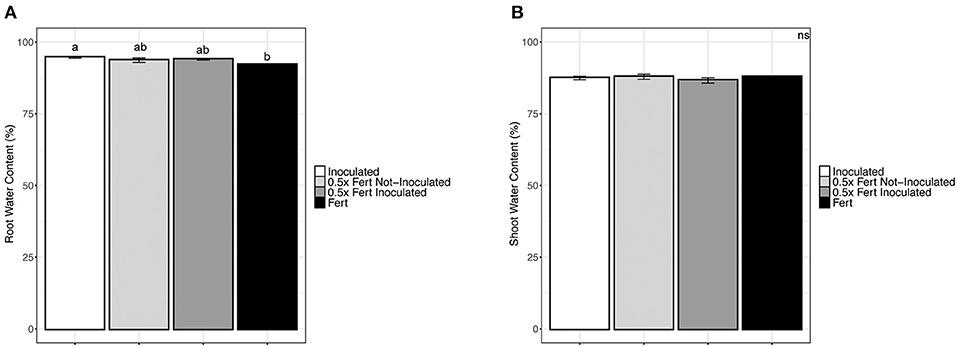
Figure 1. Water content in cucumber plants. (A) Water content in roots of cucumber plants harvested after 60 days of cultivation in greenhouse and subjected to the different fertilization treatments (i.e., Inoculated, 0.5x Fert Not-Inoculated, 0.5x Fert Inoculated, and Fert). (B) Water content in shoots of cucumber plants harvested after 60 days of cultivation in greenhouse and subjected to the different fertilization treatments (i.e., Inoculated, 0.5x Fert Not-Inoculated, 0.5x Fert Inoculated, and Fert). Data are reported as means ± SE. Different letters indicate significant differences according to one-way ANOVA with Tukey post-hoc tests (p < 0.05). Letters were omitted when no significant differences were found.
Plant physiological parameters and fruit production
Among the physiological parameters investigated, only the chlorophyll a content was significantly affected by the treatments, being the values of plants under half dose of chemical fertilizer implemented by the inoculum significantly higher than those with under half dose without inoculum and comparable to the values of plants under the commercial dose of fertirrigation. On the other hand, it is worth noting that the simple inoculum with PGPR was not enough to guarantee a comparable content of chlorophyll a (Figure 2). Other parameters such as chlorophyll b content, photosynthetic capacity, stomatal conductance, and transpiration capacity did not statistically change among plants under two dosed of fertilizers and with or without the inoculum (Supplementary Table 4). In addition, the different fertilization practices did not show any effects on the fruits production trait, which was evaluated as the number of fruits (data not shown), fruit weight and fruit length (Supplementary Table 4).
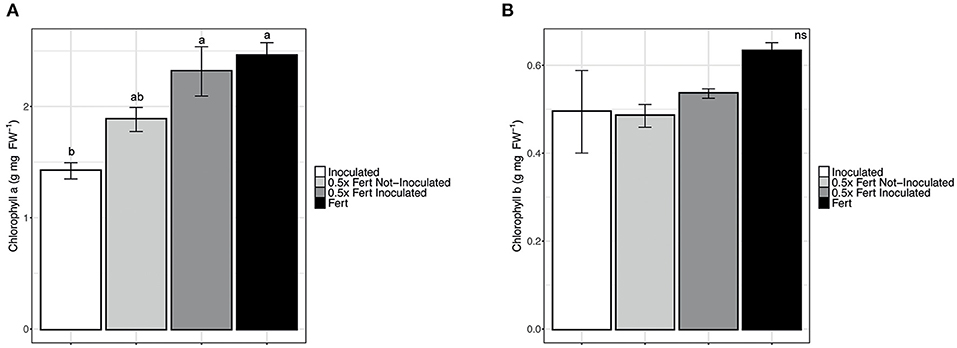
Figure 2. Chlorophylls concentration in cucumber leaves. (A) Chlorophyll a concentration in leaves of cucumber plants harvested after 60 days of cultivation in greenhouse and subjected to the different fertilization treatments (i.e., Inoculated, 0.5x Fert Not-Inoculated, 0.5x Fert Inoculated, and Fert). (B) Chlorophyll b concentration in leaves of cucumber plants harvested after 60 days of cultivation in greenhouse and subjected to the different fertilization treatments (i.e., Inoculated, 0.5x Fert Not-Inoculated, 0.5x Fert Inoculated, and Fert). Data are reported as means ± SE. Different letters indicate significant differences according to one-way ANOVA with Tukey post-hoc tests (p < 0.05). Letters were omitted when no significant differences were found.
Soil microbial biomass
Data reported in Figure 3 clearly indicate that the chemical fertilization negatively affects the soil microbial biomass that is, quite obviously, increased by the inoculum. Interestingly, the use of half dose of fertilizer in combination with the inoculum allows to reach almost the same high values without subtracting the important mineral nutrients to the plants.
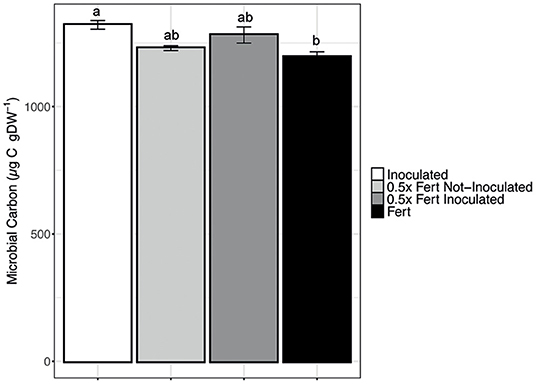
Figure 3. Microbial Carbon concentration in the rhizosphere. Data are reported as means ± SE. Different letters indicate significant differences according to one-way ANOVA with Tukey post-hoc tests (p < 0.05).
Ionomic Profiling of Roots, Shoots, and Fruits
The ionomic profile of roots, shoots, and fruits has been analyzed in order to unravel whether the different agronomic practices imposed might have affected the uptake and allocation of mineral nutrients in cucumber plants. The Principal Components Analysis carried out on the root ionome (Figure 4A) generated a model, in which the sum of Principal Component 1 (PC1) and PC2 accounted for 82% of the total variance. The scatter plot reported in Figure 4A showed the clustering of samples according with the different treatments imposed; the distribution along the PC1 was mainly influenced in the positive direction by the concentration of micronutrients, namely Fe, manganese (Mn), and zinc (Zn). Indeed, the concentrations of these elements were significantly higher in both 0.5x Fert Inoculated and 0.5x Fert Not-Inoculated samples, as compared to both Inoculated and Fert samples (Supplementary Table 5). On the other hand, the distribution of samples along the PC2 showed a separate clustering of Inoculated and Fert samples, being this distribution mainly driven by copper (Cu) in the positive direction and sulfur (S) in the negative one (Figure 4A). As predicted by PCA, the concentration of S was significantly higher in the Inoculated samples as compared to the Fert ones (Supplementary Table 5), whereas, unexpectedly, the concentration of Cu just showed an increasing trend in the Fert samples, yet not statistically different from that detected in the Inoculated roots (Supplementary Table 5).
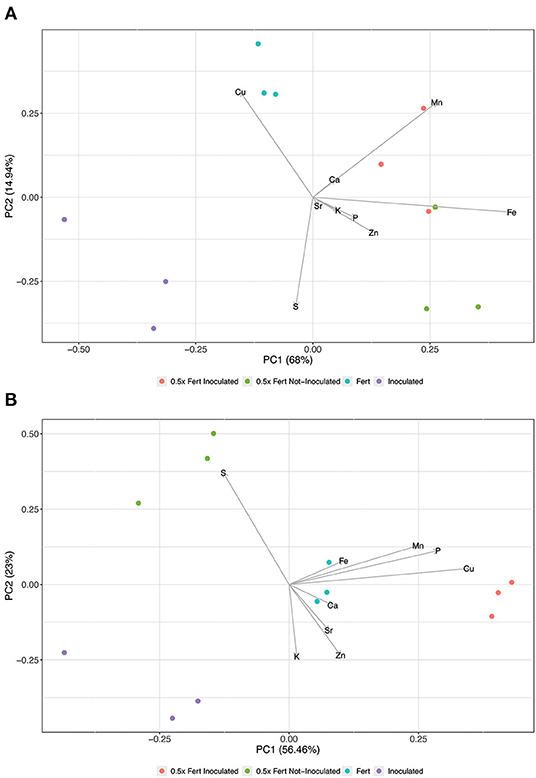
Figure 4. Principal Component Analyses of ionomic datasets. (A) Scatterplot representing the modification of root ionome in cucumber plants harvested after 60 days of cultivation in greenhouse and subjected to the different fertilization treatments (i.e., Inoculated, 0.5x Fert Not-Inoculated, 0.5x Fert Inoculated, and Fert). (B) Scatterplot representing the modification of shoot ionome in cucumber plants harvested after 60 days of cultivation in greenhouse and subjected to the different fertilization treatments (i.e., Inoculated, 0.5x Fert Not-Inoculated, 0.5x Fert Inoculated, and Fert).
At shoot level, the multivariate analyses produced a model, whose PC1 and PC2 described ~79.5% of the total variance (Figure 4B). As already observed in roots, the samples separated according with the treatments imposed, forming four independent clusters. The distribution along the PC1 (56.5% of the total variance) was predominantly determined by the concentration of both micro- (i.e., Cu, Mn, and Fe) and macronutrients [i.e., phosphorus (P), and calcium (Ca)]; in fact, Fe, Cu, and Ca displayed a significantly higher concentration in 0.5x Fert Inoculated and Fert samples as compared to the other two treatments. On the other hand, Mn and P were more concentrated in 0.5x Fert Inoculated plants as compared to the other plants analyzed (Supplementary Table 6). The PC2 (23% of the total variance) highlighted the separation between Inoculated and 0.5x Fert Not-Inoculated plants (Figure 4B), being this distribution mainly driven by S in the positive direction and by potassium (K) and Zn in the negative one. Indeed, the 0.5x Fert Not-Inoculated showed the highest concentration of S as compared to the other samples, whilst the concentrations of K and Zn were significantly higher in the shoot of Inoculated plants as compared to those detected in 0.5x Fert Not-Inoculated plants (Supplementary Table 6).
The scatter plot obtained from the fruit ionome PCA accounted for ~77% of the total dataset variance and highlighted a clear separation along PC1 of the Inoculated samples respect to the others (Figure 5). This clustering is mainly determined by the concentrations of Cu, Fe, molybdenum (Mo), and S, which, in fact, resulted significantly higher in cucumbers produced by inoculated plants (Supplementary Table 7). On the other hand, the other samples, namely 0.5x Fert Not-Inoculated, 0.5x Fert Inoculated and Fert, displayed a separation along PC2 that was mainly driven by Mg in the positive direction and by Ca, Zn, and sodium (Na) in the negative one (Figure 5). The concentration of Mg resulted in fact higher in 0.5x Fert Not-Inoculated samples with respect to both 0.5x Fert Inoculated and Fert samples (Supplementary Table 7). On the other hand, Ca and Zn were highly significantly accumulated in both 0.5x Fert Inoculated and Fert samples compared to 0.5x Fert Not-Inoculated, whilst Na displayed the highest concentration in 0.5x Fert Inoculated (Supplementary Table 7).
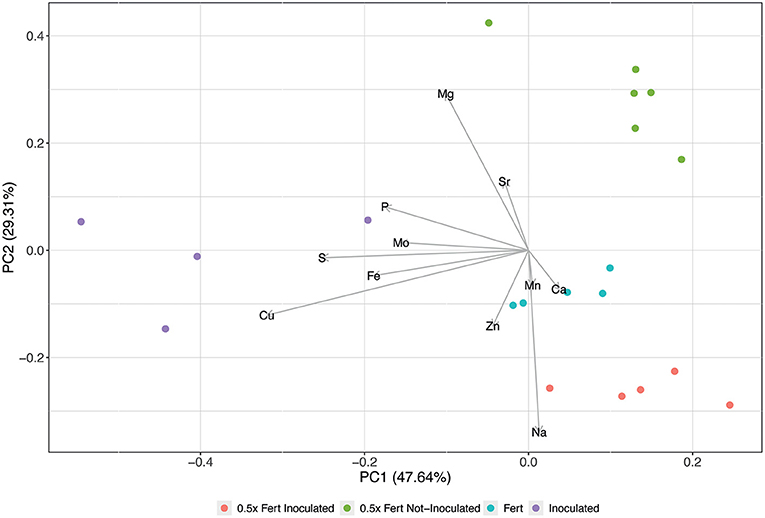
Figure 5. Principal Component Analyses of fruit ionome signature. Scatterplot representing the modification of cucumber fruit ionome harvested from plants cultivated for 60 days in greenhouse and subjected to the different fertilization treatments (i.e., Inoculated, 0.5x Fert Not-Inoculated, 0.5x Fert Inoculated, and Fert).
Nutraceuticals Content
In order to assess whether the different fertilization practices have affected the content of nutraceutical compounds in cucumber fruits, a targeted metabolomic approach has been undertaken. The quantification of antioxidant molecules, as for instance flavonoids and flavonols, did not reveal any significant variation according with the treatment (Supplementary Table 8). Cucumber fruits sugar content was determined by HPLC and the results showed that both glucose and fructose did not display significant alterations depending on the fertilization practices (Supplementary Table 8). On the other hand, sucrose was significantly accumulated in fruits of Fert plants, whereas the lowest concentrations were observed in cucumber fruits produced by Inoculated and 0.5x Fert Not-Inoculated plants (Figure 6A).
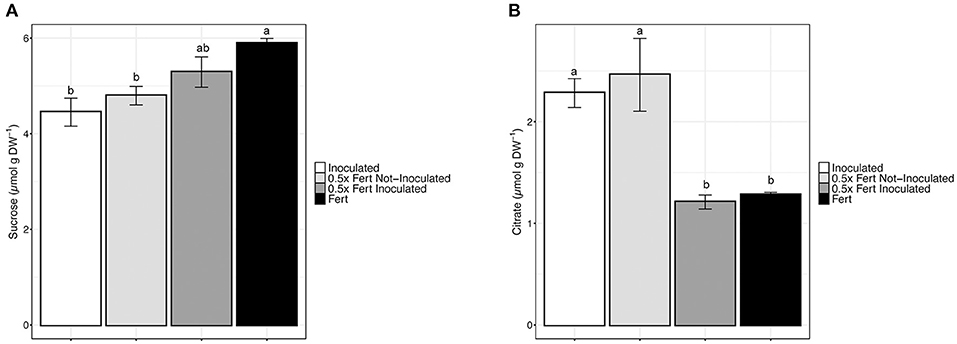
Figure 6. Nutraceuticals content in cucumber fruits. (A) Concentration of sucrose in cucumber fruit harvested from plants cultivated for 60 days in greenhouse and subjected to the different fertilization treatments (i.e., Inoculated, 0.5x Fert Not-Inoculated, 0.5x Fert Inoculated, and Fert). (B) Concentration of citrate in cucumber fruit harvested from plants cultivated for 60 days in greenhouse and subjected to the different fertilization treatments (i.e., Inoculated, 0.5x Fert Not-Inoculated, 0.5x Fert Inoculated, and Fert). Data are reported as means ± SE. Different letters indicate significant differences according to one-way ANOVA with Tukey post-hoc tests (p < 0.05). Letters were omitted when no significant differences were found.
The analysis of organic acids (OA) content highlighted that only citrate, among the other OA detected (i.e., malate, α-ketoglutarate, fumarate, and succinate), displayed a variation in the concentration depending on the treatment imposed (Figure 6B and Supplementary Table 8). In particular, fruits produced by Inoculated and 0.5x Fert Not-Inoculated plants had citrate concentrations that were almost doubled as compared to those detected in 0.5x Fert Inoculated and Fert samples (Figure 6B).
Discussion
Plant Growth Promoting Bacteria (PGPR), inhabiting rhizosphere and colonizing the surface of roots, play beneficial roles that directly or indirectly affect plant health. Thus, PGPR offer a sustainable alternative to conventional agricultural practices, stimulating plant growth and considerably helping to limit the input of fertilizers necessary in growing substrates (Bashan, 1998).
In the present investigation, three different PGPR, BFD160 E. asburiae, TFD26 P. koreensis, and BFS112 P. linii, isolated and characterized for their PGP activities (phosphates solubilization, production of siderophores, and IAA) in a previous study (Scagliola et al., 2016), were tested in a controlled soil-plant system (climatic chamber, 30 days plant growth) to select only one strain (TFD26 P. koreensis) with the best performances, to be further used in a tunnel experiment with a longer cultivation period, up to fruits production.
The effect of the three PGPR on the germination processes has been evaluated in vitro and the results showed a slightly decrease in the germination rate, yet not statistically significant, likely ascribable to the competition for nutrient resources between the cucumber seeds and the microorganisms (Stefan et al., 2008). These observations allowed to conclude that the strains hereby used do not exert toxic actions on seed germination. Furthermore, as suggested by Zhou et al. (2013), the observed effects could disappear during the seedling's development.
To facilitate the monitoring of PGPR and determine their survival in agricultural soil experiments, the strains, BFD160 E. asburiae, TFD26 P. koreensis, and BFS112 P. linii, were tagged with the gfp and liquid broth cultivation was carried out to assess the effect of the marker on the growth and on the labeling stability. The data indicated that the growth of the gfp-tagged PGPR, and likely the whole metabolism, were not affected by the labeling, obtained through the introgression of the recombinant plasmid. Indeed, the plasmid was maintained at least until 40 days of cultivation. On the other hand, the inoculation of agricultural soil with the labeled bacterial strains resulted in a gradual decrease of BFD160gfp E. asburiae, TFD26gfp P. koreensis, and BFS112gfp P. linii, most likely because of the competition with autochthonous microorganisms. In fact, it is well-known that the bacterial species decline after the inoculation in soil, mainly because of the competition with native communities and the hostility of either biotic or abiotic interactions (van Veen et al., 1997), whilst the survival of inocula is higher in the rhizosphere than in the bulk soil (Fischer et al., 2010). It is noteworthy that the declining trend was the same for BFD160gfp E. asburiae, TFD26gfp P. koreensis, and BFS112gfp P. linii, independently of the genera they belong to. These observations were fundamental for the setting up of the experimental plan for trial 2, in which repeated inoculations were carried out weekly, in order to maintain the bacterial density at a constant level during the 60 days of cultivation.
As expected, the inoculation of the two strains belonging to Pseudomonas spp. had positive effects on growth and physiological parameters. It is well-known that some strains of Pseudomonas spp. have different features that positively affect plant health, making them well suit as PGPR (Saharan and Nehra, 2011). In particular, according to the growth chamber trial, the plants grown in soil treated with TFD26 P. koreensis exhibited the most evident improvements. As previously reported (Scagliola et al., 2016), TFD26 P. koreensis was able to secrete low amounts of IAA, a phytohormones, which in low concentration (from 10−10 to 10−9 M) enhances the root extension (Murphy, 2006), fostering plant mineral uptake and, indirectly, plant development (Spaepen et al., 2008). Consistently, the PGPR traits of P. koreensis have been also recently demonstrated with the isolate S150 through in vitro tests on Arabidopsis thaliana plants (Gu et al., 2020). In addition, our findings showed that the presence in soil of TFD26 P. koreensis increased the plant content of several nutrients in either leaves or roots, such as Fe, Ca, Mn, Ni, Zn. Besides, the inoculation possibly improved the translocation of S and K, whose amounts resulted higher in leaves than in roots, when compared to the control. Indeed, related P. koreensis strains was recently shown to feature relevant K solubilizing ability in soil, thus increasing the K bioavailability to plants (Reyes-Castillo et al., 2019; Adhikari et al., 2020). Very interestingly, the Fe content was significantly increased, and this effect might be correlated to the capacity of the same strain to produce siderophores, organic compounds which increase the Fe bioavailability (Scagliola et al., 2016). Furthermore, among the three strains used in the growth chamber test, the inoculum of TFD26 P. koreensis was responsible of a relevant increase of all physiological parameters assessed (photosynthetic rate, transpiration rate, and stomatal conductance). Accordingly, it was used to determine its effects on growth and physiological parameters, as well as on fruit quality, when used in combination or in alternative to a chemical fertilization management (Trial 2).
It has been clearly demonstrated that the mineral elements concentration in the tissues of agricultural plants can be influenced by the growing conditions, as for instance the chemical and physical characteristics of the growth substrate, the fertilization practice, and the inoculation with PGPR. These alterations in the ionomic signature sometimes give rise to the increase in the concentration of essential and/or non-essential mineral elements, which can result in health-promoting effects for consumers (Tomasi et al., 2009; Pii et al., 2015a; Astolfi et al., 2018).
At leaf level, the treatment with the commercial fertilizer caused the highest accumulation of Ca, whose structural and biochemical (e.g., in the signaling process) role in both plants and animals is well-demonstrated (Marschner, 2012). Interestingly, the inoculated samples, independently of the treatment with the fertilizers (i.e., Inoculated and 0.5x Fert Inoculated), presented a Ca concentration that was comparable with the Fert samples. At fruit level, the 0.5x Fert Inoculated samples also showed the highest concentration of Ca, comparable to the values measured in fruits produced by Fert plants. A higher Ca concentration in the edible part of agricultural product might have a particular relevance to human health promotion, since the lack of this macronutrient has been epidemiologically related to several diseases (Sharma et al., 2017). The treatment with bacteria also caused a higher accumulation of S in the root tissues, albeit the concentration of S in the shoots of the same plants was lower as compared to the not-inoculated counterparts (i.e., Fert and 0.5x Fert Not-Inoculated). Indeed, the higher accumulation in the leaves of not-inoculated samples, regardless of the fertilization regime, might suggest a possible ongoing stress response. In fact, S has been demonstrated to be involved in several metabolic pathways that lead to the production of different metabolites, like hydrogen sulfide, cysteine, methionine and glutathione, that are usually produced to cope with both biotic and abiotic stressors (Abdalla and Mühling, 2019; Kopriva et al., 2019). Nevertheless, the higher S concentration detected in cucumber fruits produced by inoculated plants might represent a positive nutraceutical feature, since it might hint the higher accumulation of bioactive phytochemicals related with the scavenging action of oxidative stress (González-Morales et al., 2017). Concerning micronutrients (i.e., Fe, Mn, and Zn), the inoculation with the PGPR showed positive effects on the concentrations at the root level only when plants were also supplemented with half strength fertilizer. The same trend was also shown at leaf level. Indeed, the promoting effect of TFD26 P. koreensis inoculation on the Fe acquisition mechanisms (i.e., Fe chelate reductase activity) in cucumber plants has already been demonstrated (Scagliola et al., 2016). Consistently, Murgese et al. (2020) demonstrated that the inoculation of C. melo L. plants with a mixed microbial community, also featuring TFD26 P. koreensis, induced the upregulation of genes (i.e., CmFRO and CmIRT1) involved in the reduction and uptake of Fe2+ in dicot plants. In this context, the enhanced activity of Fe uptake system upon PGPR inoculation (Pii et al., 2016b) could also account for the higher concentration of other bivalent cations in roots, namely Mn and Zn, observed at root level. In fact, Fe, in its reduced form, is transported across the plasma membrane of root cells through the IRT1-like protein (Connolly et al., 2003) which is also able to catalyze the transmembrane fluxes of Mn, Zn, Cu, and Cd (Korshunova et al., 1999). On the other hand, consistently with the results obtained in PGPR-inoculated strawberries (Pii et al., 2018), the fruits produced by inoculated plants showed the highest concentration of micronutrients, like Cu, Fe, and Mo. These results might suggest a more efficient root-shoot translocation as compared to the other samples considered, thus resulting in a micronutrient fortification of cucumber fruits. Beside the concentration of mineral elements, the inoculation of cucumber plants with the sole TFD26 P. koreensis resulted in the lowest concentration of sucrose and the highest concentration of citrate in fruits. These results were not in agreement with the increase in the sugar content and the decrease of detected titratable acidity, for instance, in cherry tomatoes and strawberries after the inoculation with PGPR (Pii et al., 2018; Aini et al., 2019). This evidence further highlights that plants response to PGPR inoculation can be highly specific and depending on the two interacting partners and the soil characteristics.
In general, the results of the second trial, performed in conditions closer to the field scale including the fruit production step, are in accordance with those achieved in very controlled short-term growth (Trial 1). In some cases, the physiological and biochemical determinations in the presence of the inoculum are just comparable and not significantly higher than those measured in controls, very likely because a shorter trial makes the effects more evident. It is also worth noting that the first trial did not plan a fertilization treatment, just comparing plant watering, with or without the inoculum, a condition that is really far from the real agronomic practices. Overall, our results indicate that the inoculum, when added to a limited amount of chemical fertilizer, albeit still in partially controlled conditions, allows the plant to reach at least the same performances as in presence of the commercial dose of the fertilizer. This can highlight that bioinoculants might be considered as a valid complement of chemical fertilizers for a more sustainable agricultural scenario; nonetheless, in order to obtain more comprehensive knowledge about the possible activities of the investigated microbial strain in realistic conditions, field trials will be required.
Data Availability Statement
The datasets generated for this study can be found in online repositories. The names of the repository/repositories and accession number(s) can be found in the article/Supplementary Material.
Author Contributions
MS, SC, CC, and YP: experimental design and manuscript writing and critical revision. MS, FV, TM, and YP: experiments execution and data collection. MS and YP: data analyses and visualization. MS, TM, SC, CC, and YP: data interpretation. CC, TM, and YP: financial support. All authors contributed to the article and approved the submitted version.
Funding
The research was supported by grants from the Free University of Bolzano (TN2071) and by Italian MIUR (Programma PRIN 2017 - PHOBOS).
Conflict of Interest
The authors declare that the research was conducted in the absence of any commercial or financial relationships that could be construed as a potential conflict of interest.
Supplementary Material
The Supplementary Material for this article can be found online at: https://www.frontiersin.org/articles/10.3389/fsufs.2020.622169/full#supplementary-material
References
Abdalla, A. M., and Mühling, K. H. (2019). Plant-derived sulfur containing natural products produced as a response to biotic and abiotic stresses: a review of their structural diversity and medicinal importance. J. Appl. Bot. Food Qual. 92, 204–215. doi: 10.5073/JABFQ.2019.092.029
Adhikari, A., Khan, M. A., Lee, K. E., Kang, S. M., Dhungana, S. K., Bhusal, N., et al. (2020). The halotolerant rhizobacterium—Pseudomonas koreensis MU2 enhances inorganic silicon and phosphorus use efficiency and augments salt stress tolerance in soybean (Glycine max L.). Microorganisms 8:1256. doi: 10.3390/microorganisms8091256
Aini, N., Rizqi, P., and Wiwin, Y. (2019). The effect of nutrient concentration and inoculation of PGPR and AMF on the yield and fruit quality of hydroponic cherry tomatoes (Lycopersicum esculentum Mill. var. cerasiforme). J. Appl. Hortic. 21, 116–122. doi: 10.37855/jah.2019.v21i02.20
Alegria-Terrazas, R., Giles, C., Paterson, E., Robertson-Albertyn, S., Cesco, S., Mimmo, T., et al. (2016). Plant–microbiota interactions as a driver of the mineral turnover in the rhizosphere. Adv. Appl. Microbiol. 95, 1–67. doi: 10.1016/bs.aambs.2016.03.001
Arif, M. S., Riaz, M., Shahzad, S. M., Yasmeen, T., Akhtar, M. J., Riaz, M. A., et al. (2016). Associative interplay of plant growth promoting rhizobacteria (Pseudomonas aeruginosa QS40) with nitrogen fertilizers improves sunflower (Helianthus annuus L.) productivity and fertility of aridisol. Appl. Soil Ecol. 108, 238–247. doi: 10.1016/j.apsoil.2016.08.016
Astolfi, S., Pii, Y., Terzano, R., Mimmo, T., Celletti, S., Allegretta, I., et al. (2018). Does Fe accumulation in durum wheat seeds benefit from improved whole-plant sulfur nutrition? J. Cereal Sci. 83, 74–82. doi: 10.1016/j.jcs.2018.07.010
Baker, G. C., Smith, J. J., and Cowan, D. A. (2003). Review and re-analysis of domain-specific 16S primers. J. Microbiol. Methods 55, 541–555. doi: 10.1016/j.mimet.2003.08.009
Banchio, E., Xie, X., Zhang, H., and Paré, P. W. (2009). Soil bacteria elevate essential oil accumulation and emissions in sweet basil. J. Agric. Food Chem. 57, 653–657. doi: 10.1021/jf8020305
Bashan, Y. (1998). Inoculants of plant growth-promoting bacteria for use in agriculture. Biotechnol. Adv. 16, 729–770. doi: 10.1016/S0734-9750(98)00003-2
Bona, E., Lingua, G., Manassero, P., Cantamessa, S., Marsano, F., Todeschini, V., et al. (2015). AM fungi and PGP pseudomonads increase flowering, fruit production, and vitamin content in strawberry grown at low nitrogen and phosphorus levels. Mycorrhiza 25, 181–193. doi: 10.1007/s00572-014-0599-y
Bravin, M. N., Michaud, A. M., Larabi, B., and Hinsinger, P. (2010). RHIZOtest: a plant-based biotest to account for rhizosphere processes when assessing copper bioavailability. Environ. Pollut. 158, 3330–3337. doi: 10.1016/j.envpol.2010.07.029
Brookes, P. C. (1995). The use of microbial parameters in monitoring soil pollution by heavy metals. Biol. Fertil. Soils 19, 269–279. doi: 10.1007/BF00336094
Camacho, C., Coulouris, G., Avagyan, V., Ma, N., Papadopoulos, J., Bealer, K., et al. (2009). BLAST+: architecture and applications. BMC Bioinform. 10:421. doi: 10.1186/1471-2105-10-421
Cappellari, L. D. R., Chiappero, J., and Banchio, E. (2019a). Invisible signals from the underground: a practical method to investigate the effect of microbial volatile organic compounds emitted by rhizobacteria on plant growth. Biochem. Mol. Biol. Educ. 47, 388–393. doi: 10.1002/bmb.21243
Cappellari, L. D. R., Santoro, M. V., Nievas, F., Giordano, W., and Banchio, E. (2013). Increase of secondary metabolite content in marigold by inoculation with plant growth-promoting rhizobacteria. Appl. Soil Ecol. 70, 16–22. doi: 10.1016/j.apsoil.2013.04.001
Cappellari, L. D. R., Santoro, M. V., Schmidt, A., Gershenzon, J., and Banchio, E. (2019b). Induction of essential oil production in Mentha x piperita by plant growth promoting bacteria was correlated with an increase in jasmonate and salicylate levels and a higher density of glandular trichomes. Plant Physiol. Biochem. 141, 142–153. doi: 10.1016/j.plaphy.2019.05.030
Chithrashree, U. A. C., Nayaka, S. C., Reddy, M. S., and Srinivas, C. (2011). Plant growth-promoting rhizobacteria mediate induced systemic resistance in rice against bacterial leaf blight caused by Xanthomonas oryzae pv. oryzae. Biol. Control 59, 114–122. doi: 10.1016/j.biocontrol.2011.06.010
Cohen, S. N., Chang, A. C. Y., and Hsu, L. (1972). Nonchromosomal antibiotic resistance in bacteria: genetic transformation of Escherichia coli by R-factor DNA. Proc. Natl. Acad. Sci. U.S.A. 69, 2110–2114. doi: 10.1073/pnas.69.8.2110
Connolly, E. L., Campbell, N. H., Grotz, N., Prichard, C. L., and Guerinot, M. L. (2003). Overexpression of the FRO2 Ferric Chelate Reductase confers tolerance to growth on low iron and uncovers posttranscriptional control. Plant Physiol. 133, 1102–1110. doi: 10.1104/pp.103.025122
Crecchio, C., Mimmo, T., Bulgarelli, D., Pertot, I., Pii, Y., Perazzolli, M., et al. (2018). “Beneficial soil microbiome for sustainable agricultural production,” in Biocontrol; Sustainable Agriculture Reviews, ed E. Lichtfouse (Dordrecht: Springer), 443–481. doi: 10.1007/978-3-319-94232-2_9
Dhale, D. A., Chatte, S. N., and Jadhav, V. T. (2010). Effect of bioinoculants on growth, yield and fibre quality of cotton under irrigation. Res. J. Agric. Biol. Sci. 6, 542–547.
Dimkpa, C., Weinand, T., and Asch, F. (2009). Plant–rhizobacteria interactions alleviate abiotic stress conditions. Plant Cell Environ. 32, 1682–1694. doi: 10.1111/j.1365-3040.2009.02028.x
Erika, B., Bogino, P. C., Santoro, M., Torres, L., Zygadlo, J., and Giordano, W. (2010). Systemic induction of monoterpene biosynthesis in Origanum × majoricum by soil bacteria. J. Agric. Food Chem. 58, 650–654. doi: 10.1021/jf9030629
Esitken, A., Ercisli, S., and Eken, C. (2005). Effects of mycorrhiza isolates on symbiotic germination of terrestrial orchids (Orchis palustris Jacq. and Serapias vomeracea subsp. vomeracea (Burm.f.) Briq.) in Turkey. Symbiosis. 38, 59–68.
Eşitken, A., Karlidağ, H, Ercişli, S., and Şahin, F. (2002). Effects of foliar application of Bacillus subtilis OSU-142 on the yield, growth and control of shot-hole disease (Coryneum blight) of apricot. Gartenbauwissenschaft 67, 139–142.
Esitken, A., Karlidag, H., Ercisli, S., Turan, M., and Sahin, F. (2003). The effect of spraying a growth promoting bacterium on the yield, growth and nutrient element composition of leaves of apricot (Prunus armeniaca L. cv. Hacihaliloglu). Aust. J. Agric. Res. 54, 377–380. doi: 10.1071/AR02098
Esitken, A., Pirlak, L., Turan, M., and Sahin, F. (2006). Effects of floral and foliar application of plant growth promoting rhizobacteria (PGPR) on yield, growth and nutrition of sweet cherry. Sci. Hortic. 110, 324–327. doi: 10.1016/j.scienta.2006.07.023
Fischer, S. E., Jofr, é E. C, Cordero, P. V., Gutierrez Manero, F. J., and Mori, G. B. (2010). Survival of native Pseudomonas in soil and wheat rhizosphere and antagonist activity against plant pathogenic fungi. Antonie Van Leeuwenhoek 97, 241–251. doi: 10.1007/s10482-009-9405-9
Gomathy, M., Prakesh, D., Thangaraju, M., Sundaram, S. P., and Sundaram, P. M. (2008). Impact of biofertigation of Azophosmet on cotton yield under drip irrigation. Res. J. Agric. Biol. Sci. 4, 695–699.
González-Morales, S., Pérez-Labrada, F., García-Enciso, E. L., Leija-Martínez, P., Medrano-Macías, J., Dávila-Rangel, I. E., et al. (2017). Selenium and sulfur to produce allium functional crops. Molecules 22:558. doi: 10.3390/molecules22040558
Gu, Y., Ma, Y. N., Wang, J., Xia, Z., and Wei, H. L. (2020). Genomic insights into a plant growth-promoting Pseudomonas koreensis strain with cyclic lipopeptide-mediated antifungal activity. Microbiologyopen 9:e1092. doi: 10.1002/mbo3.1092
Heidari, M., and Golpayegani, A. (2012). Effects of water stress and inoculation with plant growth promoting rhizobacteria (PGPR) on antioxidant status and photosynthetic pigments in basil (Ocimum basilicum L.). J. Saudi Soc. Agric. Sci. 11, 57–61. doi: 10.1016/j.jssas.2011.09.001
Hernandez, M., and Chailloux, M. (2004). Las micorrizas arbusculares y las bacterias rizosfericas como alternativa a la nutricion mineral del tomate. Cult. Trop. 25, 5–16.
Hiscox, J. D., and Israelstam, G. F. (1979). A method for the extraction of chlorophyll without maceration from leaf tissue. Can. J. Bot. 57, 1332–1334. doi: 10.1139/b79-163
Jetiyanon, K., and Kloepper, J. W. (2002). Mixtures of plant growth-promoting rhizobacteria for induction of systemic resistance against multiple plant diseases. Biol. Control 24, 285–291. doi: 10.1016/S1049-9644(02)00022-1
Kolega, S., Moreno, B. M., Buffagni, V., Lucini, L., Valentinuzzi, F., Maver, M., et al. (2020). Nutraceutical profiles of two hydroponically grown sweet basil cultivars as affected by the composition of the nutrient solution and the inoculation with Azospirillum brasilense. Front. Plant Sci. 11:1683. doi: 10.3389/FPLS.2020.596000
Kopittke, P. M., Menzies, N. W., Wang, P., McKenna, B. A., and Lombi, E. (2019). Soil and the intensification of agriculture for global food security. Environ. Int. 132:105078. doi: 10.1016/j.envint.2019.105078
Kopriva, S., Malagoli, M., and Takahashi, H. (2019). Sulfur nutrition: impacts on plant development, metabolism, and stress responses. J. Exp. Bot. 70, 4069–4073. doi: 10.1093/jxb/erz319
Korshunova, Y. O., Eide, D., Gregg Clark, W., Guerinot, M. L., and Pakrasi, H. B. (1999). The IRT1 protein from Arabidopsis thaliana is a metal transporter with a broad substrate range. Plant Mol. Biol. 40, 37–44 doi: 10.1023/A:1026438615520
Kumar, A., Vandana Singh, M., Singh, P. P., Singh, S. K., Singh, P. K., et al. (2016). Isolation of plant growth promoting rhizobacteria and their impact on growth and curcumin content in Curcuma longa L. Biocatal. Agric. Biotechnol. 8, 1–7. doi: 10.1016/j.bcab.2016.07.002
Maniatis, T., Sambrook, J., and Fritsch, E. F., (eds). (1982). Molecular Cloning: A Laboratory Manual. Cold Spring Harbor, NY: Cold Spring Harbor Laboratory. doi: 10.1016/0307-4412(83)90068-7
Marastoni, L., Pii, Y., Maver, M., Valentinuzzi, F., Cesco, S., and Mimmo, T. (2019). Role of Azospirillum brasilense in triggering different Fe chelate reductase enzymes in cucumber plants subjected to both nutrient deficiency and toxicity. Plant Physiol. Biochem. 136, 118–126. doi: 10.1016/j.plaphy.2019.01.013
Marschner, P. (2012). Marschner's Mineral Nutrition of Higher Plants. London: Academic Press, 651. doi: 10.1016/C2009-0-63043-9
Mimmo, T., Pii, Y., Valentinuzzi, F., Astolfi, S., Lehto, N., Robinson, B., et al. (2018). Nutrient availability in the rhizosphere: a review. Acta Hortic. 1217, 13–27. doi: 10.17660/ActaHortic.2018.1217.2
Murgese, P., Santamaria, P., Leoni, B., and Crecchio, C. (2020). Ameliorative effects of PGPB on yield, physiological parameters, and nutrient transporter genes expression in Barattiere (Cucumis melo L.). J. Soil Sci. Plant Nutr. 20, 784–793. doi: 10.1007/s42729-019-00165-1
Murphy, A. S. (2006). “Auxin: the Growth hormone,” in Plant Physiology, eds L. Taiz and E. Zeiger (Sunderland MA: Sinauer Associates). doi: 10.1093/aob/mcg079
Nalayini, P., Sankaranarayanan, K., and Anandham, R. (2010). Bio inoculants for enhancing the productivity and nutrient uptake of winter irrigated cotton (Gossypium hirsutum) under graded levels of nitrogen and phosphatic fertilizers. Indian J. Agron. 55, 64–67.
Orhan, E., Esitken, A., Ercisli, S., Turan, M., and Sahin, F. (2006). Effects of plant growth promoting rhizobacteria (PGPR) on yield, growth and nutrient contents in organically growing raspberry. Sci. Hortic. 111, 38–43. doi: 10.1016/j.scienta.2006.09.002
Pii, Y., Borruso, L., Brusetti, L., Crecchio, C., Cesco, S., and Mimmo, T. (2016a). The interaction between iron nutrition, plant species and soil type shapes the rhizosphere microbiome. Plant Physiol. Biochem. 99, 39–48. doi: 10.1016/j.plaphy.2015.12.002
Pii, Y., Cesco, S., and Mimmo, T. (2015a). Shoot ionome to predict the synergism and antagonism between nutrients as affected by substrate and physiological status. Plant Physiol. Biochem. 94, 48–56. doi: 10.1016/j.plaphy.2015.05.002
Pii, Y., Graf, H., Valentinuzzi, F., Cesco, S., and Mimmo, T. (2018). The effects of plant growth-promoting rhizobacteria (PGPR) on the growth and quality of strawberries. Acta Hortic. 1217, 231–238. doi: 10.17660/ActaHortic.2018.1217.29
Pii, Y., Marastoni, L., Springeth, C., Fontanella, M. C., Beone, G. M., Cesco, S., et al. (2016b). Modulation of Fe acquisition process by Azospirillum brasilense in cucumber plants. Environ. Exp. Bot. 130, 216–225. doi: 10.1016/j.envexpbot.2016.06.011
Pii, Y., Mimmo, T., Tomasi, N., Terzano, R., Cesco, S., and Crecchio, C. (2015b). Microbial interactions in the rhizosphere: beneficial influences of plant growth-promoting rhizobacteria on nutrient acquisition process. A review. Biol. Fertil. Soils 51, 403–415. doi: 10.1007/s00374-015-0996-1
Pii, Y., Penn, A., Terzano, R., Crecchio, C., Mimmo, T., and Cesco, S. (2015c). Plant-microorganism-soil interactions influence the Fe availability in the rhizosphere of cucumber plants. Plant Physiol. Biochem. 87, 45–52. doi: 10.1016/j.plaphy.2014.12.014
Reyes-Castillo, A., Gerding, M., Oyarzúa, P., Zagal, E., Gerding, J., and Fischer, S. (2019). Plant growth-promoting rhizobacteria able to improve NPK availability: selection, identification and effects on tomato growth. Chil. J. Agric. Res. 79, 473–485. doi: 10.4067/S0718-58392019000300473
Saeed, K. S., Ahmed, S. A., Hassan, I. A., and Ahmed, P. H. (2015). Effect of bio-fertilizer and chemical fertilizer on growth and yield in cucumber (Cucumis sativus L.) in green house condition. Am. Euras. J. Agric. Environ. Sci. 15, 353–358. doi: 10.3923/pjbs.2015.129.134
Saharan, B. S., and Nehra, V. (2011). Plant Growth Promoting Rhizobacteria: a critical review. Life Sci. Med. Res. 21.
Santoro, M. V., Zygadlo, J., Giordano, W., and Banchio, E. (2011). Volatile organic compounds from rhizobacteria increase biosynthesis of essential oils and growth parameters in peppermint (Mentha piperita). Plant Physiol. Biochem. 49, 1177–1182. doi: 10.1016/j.plaphy.2011.07.016
Scagliola, M., Pii, Y., Mimmo, T., Cesco, S., Ricciuti, P., and Crecchio, C. (2016). Characterization of plant growth promoting traits of bacterial isolates from the rhizosphere of barley (Hordeum vulgare L.) and tomato (Solanum lycopersicon L.) grown under Fe sufficiency and deficiency. Plant Physiol. Biochem. 107, 187–196. doi: 10.1016/j.plaphy.2016.06.002
Sharma, D., Jamra, G., Singh, U. M., Sood, S., and Kumar, A. (2017). Calcium biofortification: three pronged molecular approaches for dissecting complex trait of calcium nutrition in finger millet (Eleusine coracana) for devising strategies of enrichment of food crops. Front. Plant Sci. 7:2028. doi: 10.3389/fpls.2016.02028
Sofo, A., Palese, A. M., Casacchia, T., Celano, G., Ricciuti, P., Curci, M., et al. (2010). Genetic, functional, and metabolic responses of soil microbiota in a sustainable olive orchard. Soil Sci. 175, 81–88. doi: 10.1097/SS.0b013e3181ce8a27
Spaepen, S., Dobbelaere, S., Croonenborghs, A., and Vanderleyden, J. (2008). Effects of Azospirillum brasilense indole-3-acetic acid production on inoculated wheat plants. Plant Soil 312, 15–23. doi: 10.1007/s11104-008-9560-1
Stefan, M., Mihasan, M., and Dunca, S. (2008). Plant growth promoting rhizobacteria can inhibit the in vitro germination of Glycine Max L. Seeds. Analele Ştiinţifice Ale Universităţii “Alexandru Ioan Cuza”, Secţiunea Genetică şi Biologie Moleculară TOM IX, 105–110.
Tang, Y., Horikoshi, M., and Li, W. (2016). Ggfortify: Unified interface to visualize statistical results of popular r packages. R J. 8, 478–489. doi: 10.32614/rj-2016-060
Tomasi, N., Kretzschmar, T., Espen, L., Weisskopf, L., Fuglsang, A. T., Palmgren, M. G., et al. (2009). Plasma membrane H+-ATPase-dependent citrate exudation from cluster roots of phosphate-deficient white lupin. Plant Cell Environ. 32, 465–475. doi: 10.1111/j.1365-3040.2009.01938.x
Valentinuzzi, F., Mason, M., Scampicchio, M., Andreotti, C., Cesco, S., and Mimmo, T. (2015). Enhancement of the bioactive compound content in strawberry fruits grown under iron and phosphorus deficiency. J. Sci. Food Agric. 95, 2088–2094. doi: 10.1002/jsfa.6924
van Veen, J., van Overbeek, L., and van Elsas, J. (1997). Fate and activity of microorganisms introduced into soil. Microbiol. Mol. Biol. Rev. 61, 121–135.
Wickham, H. (2016). Ggplot2 : Elegant Graphics for Data Analysis. New York, NY: Springer. doi: 10.1007/978-3-319-24277-4
Xie, X., Zhang, H., and Paré, P. W. (2009). Sustained growth promotion in Arabidopsis with long-term exposure to the beneficial soil bacterium Bacillus subtilis (GB03). Plant Signal. Behav. 4, 948–953. doi: 10.4161/psb.4.10.9709
Zhang, H., Kim, M. S., Sun, Y., Dowd, S. E., Shi, H., and Pare, P. W. (2008a). Soil bacteria confer plant salt tolerance by tissue-specific regulation of the sodium transporter HKT1. Mol. Plant Microbe Int. 21, 737–744. doi: 10.1094/MPMI-21-6-0737
Zhang, H., Murzello, C., Sun, Y., Kim, M.-S., Xie, X., Jeter, R. M., et al. (2010). Choline and osmotic-stress tolerance induced in Arabidopsis by the soil microbe Bacillus subtilis (GB03). Mol. Plant Microbe Int. 23, 1097–1104. doi: 10.1094/MPMI-23-8-1097
Zhang, H., Xie, X., Kim, M. S., Kornyeyev, D. A., Holaday, S., and Pare, P. W. (2008b). Soil bacteria augment Arabidopsis photosynthesis by decreasing glucose sensing and abscisic acid levels in planta. Plant J. 56, 264–273. doi: 10.1111/j.1365-313X.2008.03593.x
Zhang, L., Yan, C., Guo, Q., Zhang, J., and Ruiz-Menjivar, J. (2018). The impact of agricultural chemical inputs on environment: global evidence from informetrics analysis and visualization. Int. J. Low Carbon Technol. 13, 338–352. doi: 10.1093/ijlct/cty039
Zhao, F., Zhang, Y., Li, H., Shi, R., and Han, S. (2013). CaCl2-heat shock preparation of competent cells of three Pseudomonas strains and related transformation conditions. Ying Yong Shengtai Xuebao 24, 788–794.
Zhou, G. C., Wang, Y., Zhai, S., Ge, F., Liu, Z. H., Dai, Y. J., et al. (2013). Biodegradation of the neonicotinoid insecticide thiamethoxam by the nitrogen-fixing and plant-growth-promoting rhizobacterium Ensifer adhaerens strain TMX-23. Appl. Microbiol. Biotechnol. 97, 4065–4074. doi: 10.1007/s00253-012-4
Keywords: PGPR - plant growth-promoting rhizobacteria, Cucumis salivus L., fertilization practices, ionomic analysis, sustainable agriculture, fruit quality
Citation: Scagliola M, Valentinuzzi F, Mimmo T, Cesco S, Crecchio C and Pii Y (2021) Bioinoculants as Promising Complement of Chemical Fertilizers for a More Sustainable Agricultural Practice. Front. Sustain. Food Syst. 4:622169. doi: 10.3389/fsufs.2020.622169
Received: 27 October 2020; Accepted: 22 December 2020;
Published: 20 January 2021.
Edited by:
Everlon Cid Rigobelo, Universidade Estadual Paulista, BrazilReviewed by:
Lamine Baba-Moussa, University of Abomey-Calavi, BeninIftikhar Ahmad, COMSATS University Islamabad, Pakistan
Copyright © 2021 Scagliola, Valentinuzzi, Mimmo, Cesco, Crecchio and Pii. This is an open-access article distributed under the terms of the Creative Commons Attribution License (CC BY). The use, distribution or reproduction in other forums is permitted, provided the original author(s) and the copyright owner(s) are credited and that the original publication in this journal is cited, in accordance with accepted academic practice. No use, distribution or reproduction is permitted which does not comply with these terms.
*Correspondence: Youry Pii, youry.pii@unibz.it