- 1International Potato Center (CIP), Hanoi, Vietnam
- 2International Potato Center (CIP), Lima, Peru
- 3International Rice Research Institute (IRRI), Los Baños, Philippines
- 4International Center for Tropical Agriculture (CIAT), Cali, Colombia
Crop species and varietal diversity on farm have the potential to trigger multiple regulating and provisioning ecosystem services. The latter is commonly assessed through targeted studies covering a select number of geographies and crop species, precluding comparisons across crops and at scale. This study draws on a large dataset on the varietal release dynamics for 11 major food crops in 44 countries of Asia and Africa to assess trends in diversity across crops and regions with a 50-year perspective. Our results show an increasing reduction of crop varietal diversity linked to the spatial displacement of traditional landraces. This trend occurs at a faster rate in Asia than in Africa. So-called mega varieties tend to increasingly dominate agricultural landscapes, adding to spatial homogeneity. We further found a negative association between varietal richness and its relative abundance, challenging the relationship between crop improvement and varietal diversity. Our results show that among cereal, pulse, and root and tuber crops, varietal diversity is lowest for cereals in Asia and highest for root and tubers in Africa. The analysis contributes new information useful to prioritize crops for which increasing varietal diversity may lead to more sustainable food systems.
Introduction
Agricultural diversity can be beneficial for environmental health, resilience, and food production (Wolfe, 2000; Frison et al., 2011). In this context, crop species diversity and intraspecific diversity are often identified as resulting in both nutrition security and ecological resilience (De Haan et al., 2010; Lin, 2011; Jones, 2017; Reiss and Drinkwater, 2018). Biodiverse cropping systems can suppress pests more effectively than single species or varietal stands because they harbor populations of natural enemies that protect crops (Bianchi et al., 2006; He et al., 2019). In addition, genetic diversity within crops can suppress diseases by blocking the ability of pathogens to freely transmit diseases and evolve new pathotypes (Lin, 2011). In this context, cropping systems with mixed varietal arrangements are found to be more resilient, particularly under biotic stresses (Yang et al., 2019), and have been shown under favorable conditions to produce 2.2% higher yields than monocultures (Reiss and Drinkwater, 2018). Positive effects of diversity have also been reported for coping with abiotic stressors (Condori et al., 2014). Climate change will drive extreme weather events and the range expansion of infectious plant diseases and pests (Anderson et al., 2004; Elad and Pertot, 2014; Bebber, 2015), which suggests that managing genetic diversity within crop species and keeping crop varietal diversity a part of the agricultural landscape can be an increasingly important focus to enhance food system resilience, i.e., the capacity to respond and recover from shocks (Frison et al., 2011).
Reduced levels of varietal diversity within and among fields result in increased vulnerability to biotic and abiotic stressors (Ceccarelli et al., 2013), posing a threat to food security. Examples of the devastating effects of low crop varietal diversity over the past century have been extensively reviewed (Keneni et al., 2012). Diseases such as a new race of stem rust of wheat are spreading rapidly on a global scale (Singh et al., 2015). In developing countries, crop losses to diseases may have devastating effects on an already alarming food security situation. For example, wheat blast reached Bangladesh for the first time in 2016, causing major production losses (Callaway, 2016). The fall armyworm has damaged maize crops especially in Africa and is now wreaking havoc in Asia (Silver, 2019). The developed world is not spared as seen from a recent outbreak of stem rust reported in Italy (Bhattacharya, 2017). On a global scale, approximately 10–16% of annual yields are lost to plant diseases (Chakraborty and Newton, 2011; Savary et al., 2019), and current trends project diseases will continue to spread (Bebber et al., 2014). Meanwhile, higher levels of climate variability will increasingly impact crop performance and food security (Xu et al., 2017).
A few so-called mega varieties of major crops have come to dominate agricultural landscapes globally. Mega varieties are generally widely adaptable, exhibit preferred quality characteristics, and are traded in established marketing channels (Pandey et al., 2012). Structural evidence of the degree to which mega varieties of major food crops dominate national agricultural landscapes, especially in developing countries, is lacking. A study reported that only four varieties are planted on 65% of the world's total rice area and six varieties on 71% of maize area (Ceccarelli et al., 2013). Another strand of literature focuses on the interplay between the displacement of landraces and the resulting genetic erosion (Gao, 2003; Perales and Golicher, 2014; Thormann and Engels, 2015). Along with their implications for increased food security, factors such as seed delivery systems (Lipper et al., 2010), crop improvement practices (Fu, 2015), industry preferences, consumer behavior, ecological diversity, and capacity of extension services may all influence varietal diversity.
The importance of varietal and seed turnover has been analyzed in depth in the context of Africa (Walker and Alwang, 2015), including policy options for smallholders (Spielman and Smale, 2017). However, data are scarce as only a few analyses have been conducted in a handful of countries, and these at different aggregate levels (Launio et al., 2008; Ahuja and Mohan Jain, 2015). Major gaps in crop varietal release registries and unavailability of varietal adoption estimates have prevented a structural overview of the varietal diversity in current cropping systems. In addition, the strong emphasis on landraces and their importance for resilience has not been met by parallel literature on how improved varieties are shaping diversity portfolios and resilience (Fu, 2015). A lack of robust baseline data on spatial and temporal diversity is the key factor underlying this gap.
The objective of this study is to explore and unravel the relationship between modern crop improvement and varietal diversity of major staple food crops: cereals, pulses, and root and tuber crops for Africa and Asia. Specifically, we (i) describe crop variety release dynamics between the 1960s and 2010s, (ii) update knowledge on current agricultural diversity (i.e., spatial and temporal varietal diversity), (iii) analyze the relationship between varietal releases and mega varieties, and (iv) investigate the relationship between varietal richness and evenness with implications for resilience. In doing so, we presume a positive relationship between varietal diversity and agricultural systems' resilience, building on insights from intrafield diversity and resilience analyses. We utilize a large dataset for 137 crop–country combinations (CCCs) including 11 food crops in 44 countries in Asia and Africa.
A list of all CCCs studied here can be found in Supplementary Data Sheet 1. Our results are based on three distinct varietal release and adoption databases for South, East, and Southeast Asia and Sub-Saharan Africa, which are explained in more detail in section Materials and Methods. Countries were selected based on importance of a specific crop for a country in terms of production and food security. Thus, our results are representative of the major production areas of food crops in the regions studied.
Materials and Methods
Data
We used three publicly available databases covering a total of 137 CCCs corresponding to 11 food crops in 44 countries in South, East, and Southeast Asia (14 countries) and Sub-Saharan Africa (30 countries). Databases were developed under three projects for different regions and years. These were as follows: “Diffusion and Impacts of Improved Varieties in Africa” (DIIVA; 2009–2013) with a focus on Africa; “Tracking Improved Varieties in South Asia” (TRIVSA; 2010–2013) with a focus on rainfed areas of South Asia; and “Strengthening Impact Assessment in the CGIAR” (SIAC; 2013–2017) complementing TRIVSA with a larger scope in Asia. All data are publicly available from the Agricultural Science and Technology Indicators platform (https://www.asti.cgiar.org/; accessed October 2020). All three datasets build on one another, having similar objectives and following similar sampling and data collection protocols. Generally, the importance of a crop in terms of total area planted, food security, and economic development resulted in the selection of priority countries sampled for the three projects (Walker and Alwang, 2015; Turner and Bishaw, 2016). In most cases, the relative amount of agricultural land planted to a specific crop was used as a basis for inclusion. As a result, our sample may have high relevance for the major production areas of major crops and, at the same time, may underrepresent agricultural areas where certain crops are relatively less abundant. The combination of all three datasets results in a somewhat unbalanced sample. Whereas the numbers of cereal and root and tuber CCCs are similar within and across regions, the number of pulse–country combinations is relatively small, particularly for Asia (see Supplementary Table 1), and thus, we advise caution in comparing absolute varietal releases.
It is important to note that systematic records of adoption in most countries relevant for our study are missing, and existing ones vary in terms of representativeness and time of measurement. Nevertheless, our study leverages a consistent body of data that allow comparison of many countries and crops using similar methodologies and time references. More of such efforts are warranted.
All database development followed the same standardized methodology for data collection (Maredia and Reyes, 2014; Walker and Alwang, 2015) based on the Delphi method (Dalkey and Helmer, 1963). Expert elicitation workshops were conducted to estimate perceived adoption rates at varietal, national, and subnational levels. The methodology approaches the national estimates by first estimating varietal adoption at lower aggregates in subgroups. Final adoption estimates were achieved after discussion and by consensus. Published national crop production and area statistics were generally important inputs to each workshop, guiding expert opinions. For each CCC, an average of 15 key experts working in the respective crop-specific sector or value chain were invited to a 1-day workshop. The attendance of various experts, such as breeders, extension agents, processors, government officials, and non-governmental organization workers, was ensured. Jointly, more than 2,000 experts were consulted.
Calculation of Area-Weighted Varietal Release
In calculating annual estimated area-weighted varietal releases, we faced a data constraint because we only have crop-specific area data for 2014 (for Asia) and 2009 (for Africa) rather than for the entire period between 1960 and 2009/2014. We bypassed this data constraint by calculating the annual percentage crop-area changes for all CCCs studied between 1960 and 2009/2014 using FAO data (FAO, 2018). Formally, the calculation included various steps and was calculated as:
where Area Changecy is the percentage area change of crop c in year y; FAO areacy is the total land area taken from the FAO database of crop c in year y; and FAO areac2009/2014 is the total land area taken from the FAO database of crop c in year 2009 (for crops in Africa) or 2014 (for crops in Asia).
Annual crop-specific land area relevant for our study was calculated as follows:
where Study Areacy is the total annual study land area of crop c in year y; Study Areac2009/2014 is the total study land area of crop c in year 2009 (for crops in Africa) or 2014 (for crops in Asia).
To arrive at area-weighted varietal releases, we calculated:
where AWVRcy is the area-weighted varietal release of crop c in year y; Releasescy is the total number of varietal releases of crop c in year y.
The annual data were then averaged to arrive at decennial time intervals. Note here that for Asia, 2010s data refer to data for 2010–2014. Because of data limitations, for Africa the cutoff point was 2009, so the 2000s data refer to 2000–2009.
Calculation of Area Planted to Mega Varieties
Definitions of mega varieties generally are of qualitative nature (see Pandey et al., 2012), which limits regional comparisons. To allow for comparisons across countries and crops, we define mega variety as covering at least 5% of the crop-specific national area. More formally, a mega variety is calculated as:
where MV1cl is the most dominant mega variety in terms of area of crop c in country l; Var Area1cl is the area planted to the most dominant variety in terms of area of crop c in country l; Tot Areacl is the total area of a specific crop c in country l; all CCCs used in this calculation are listed in Supplementary Data Sheet 1. The calculations are continued for the second, third, and i's most dominant variety until MVicl is smaller than 0.05 (Equation 5), which means that MVicl is planted to less than 5% of the total crop-specific area.
Measurement of Spatial Diversity
In the fields of ecology and conservation biology, the terms richness and evenness are used to describe genetic diversity at different scales, whereby richness refers to the total number of species, and evenness to the relative abundance of species in a community (Zhang et al., 2012). Analyzing the relationship between these two indices can provide additional insights into the spatial diversity and linked resilience of agricultural landscapes (Smale, 1998). Whereas previous research focused on landraces (Jarvis et al., 2008), we apply the analysis of these components and their relationship to assess and describe spatial and temporal diversity of improved crop varieties.
Richness is the simplest measure of diversity. It is calculated here as the total number of improved varieties grown in a given year. Evenness is a measure of dominance and calculated as the inverted Simpson E index. We calculate evenness as proposed by Jarvis et al., 2008):
where Evennessc refers to the CCC c; pi is the proportion of total area cultivated to improved varieties planted to variety i; and n is the total number of adopted improved varieties for a given crop and country. A limitation of the research is that varietal adoption data were collected for the 10 most important varieties only. In cases where experts identified more varieties, these were lumped together in the “Others” category. The inclusion of additional minor varieties, however, will change the evenness score only marginally and is thus unlikely to bias our results.
Measurement of Temporal Diversity
We calculated area-weighted average varietal age (WAVA) as a proxy for temporal diversity, adopting Brennan and Byerlee (1991) definition:
where pi is the proportion of total area cultivated to improved varieties planted to variety i; and Ri is the number of years since the release of variety i.
The temporal diversity of crop varieties is measured only for a given year and does not reflect how varieties or crops are changing over time. This would require extensive knowledge of annual national varietal adoption estimates, which are unfortunately unavailable.
The validity of the indicator depends on accuracy of the data and on data availability (Walker and Alwang, 2015). The databases we used have some missing data, especially for the year of release of some generally older varieties. Because varieties for which the year of release is missing account for approximately 25% of the total area, actual varietal age is most likely higher than our estimates. In Supplementary Table 2, we show how representative the WAVA estimates are by crop and region.
Crop Diversity Framework
The relationship between richness and evenness was assessed to determine crop diversity at national scale. In this study, we propose a framework of four quadrants partly drawing on Yachi and Loreau (1999), Jarvis et al. (2008), and Borrell et al. (2020). The proposed Crop Diversity Framework is depicted in Figure 1. Assignment to one or another quadrant is determined by the combination of evenness and richness values of each CCC. The thresholds are calculated by the total sample mean values of area-weighted richness (calculated as total area of a given crop divided by total number of adopted varieties per crop) and evenness, which are 1.43 and 0.45, respectively.
Results
Release Dynamics of Improved Varieties
Absolute rates of varietal release increased dramatically between the 1960s and 2000s in Africa and between 1960s and 2010s in Asia, with most new releases for cereal crops. In Asia, average annual varietal releases for all crop groups are higher than in Africa between the 1960s and early 2000s (Figure 2). To partly control for an unbalanced sample of CCCs (see section Materials and Methods), we compared varietal releases in both regions by weighting number of crop-specific releases by total crop area. Until the 2000s, cumulative total varietal releases for all crops amounted to 98 varieties per 100,000 hectares in Asia and 70 varieties per 100,000 hectares in Africa (Figure 3). In Asia, intercrop comparison reveals that the rates of release of cereal and root and tuber crop varieties increased considerably since the 1990s, whereas pulse crop varieties also increased, but at a much slower rate. Between the 2000s and 2010s, cereal crop releases were declining, which may be explained by a decline in the number of annual varietal releases coincident with increasing area (Figures 2, 3). For pulses, the total crop area expanded faster than varietal release (Figures 2, 3). This may represent a lag time in breeding and varietal release, which have slowed down over the study period. For both cereals and pulses, this suggests that area expansion occurred by using already established and adopted rather than newly released varieties.
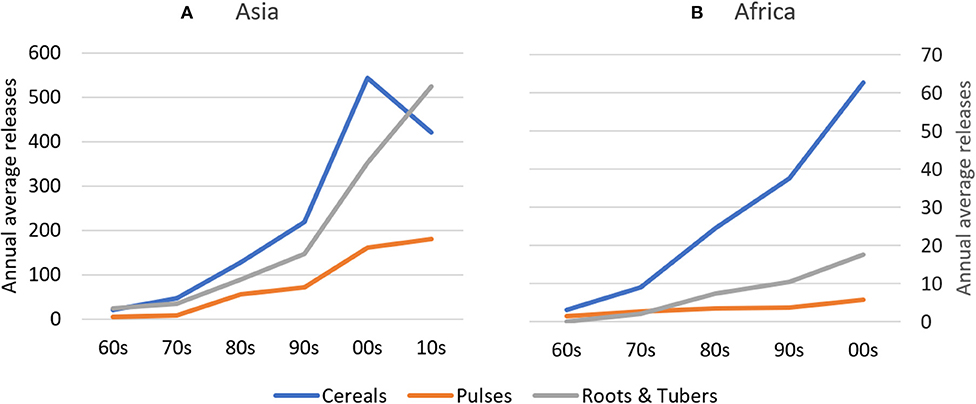
Figure 2. Annual Average Releases of Cereal, Pulse, and Root & Tuber Crop Varieties in Asia and Africa between the 1960s and 2010s. In (A), cereal values refer to the left axis; pulses and roots & tubers values refer to the right axis of (B). Given the slightly unbalanced dataset, inter-crop comparison should be made with caution. (A) Asia, (B) Africa.
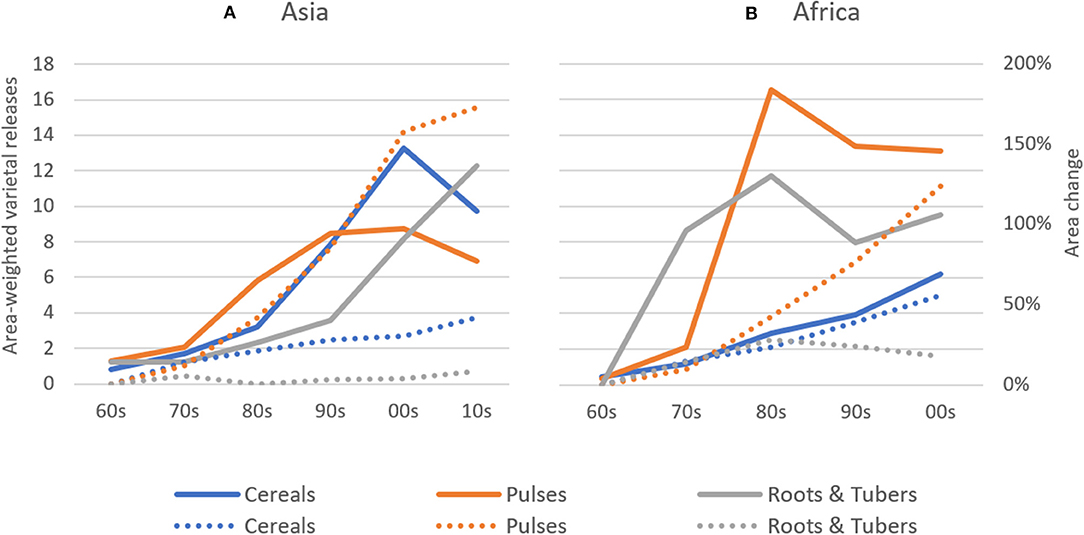
Figure 3. Area-weighted Varietal Releases in Asia and Africa between 1960–2014. Area-weighted varietal releases for cereal, pulse, and root & tuber crops are shown on a 1 release per 100 thousand hectares basis. Explanation on how area-weighted varietal releases were calculated can be found in the Materials and Methods section. Percentage land area changes for crop groups are also depicted (dotted trend lines) taking the 1960s' observations as base. (A) Asia, (B) Africa.
In Africa, the rates of cereal crop variety release increased during the past three to four decades, whereas the rates of root and tuber crop variety release stagnated. In contrast, the rates of release of pulse crop varieties experienced a drastic increase in the 1970s and 1980s and, despite a slight decreasing trend, remain relatively high in Africa (Figure 3).
Significant agricultural area expansion occurred in both regions between 1970 and 2014, resulting in an increasing area planted to improved varieties, while reducing the area planted to landraces. In Asia, the agricultural land coverage increased by 34%, from 177 to 237 million hectares, and in Africa, the rate of expansion was even higher, increasing by 59%, from 32 to 51 million hectares (Table 1). During the same period, area planted to improved varieties increased manifold (i.e., in Asia by 990%, in Africa by 15,614%), and much of the observed total area expansion is associated with this development.
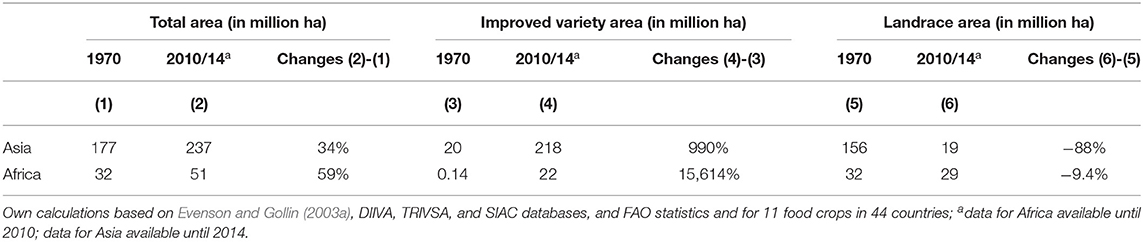
Table 1. Total area, area planted to improved varieties and landraces in Asia and Africa from 1970 to 2010/14.
At the same time, the area planted to landraces has drastically decreased in Asia by 88%, from 156 to 19 million hectares, and has seen a slight decrease in Africa by 9.4%, from 32 to 29 million hectares (Table 1). For both regions, the data suggest that improved varieties have replaced landraces for many food crops, as area expansion of improved varieties occurs at higher rates than total area expansion (Supplementary Figures 1, 2). Asia is experiencing this trend to a greater degree than is Africa.
Prevalence of Mega Varieties
For this study, we define mega variety as a variety that covers a minimum of 5% of the crop-specific national area (see section Materials and Methods for more details). Our analysis reveals that in Asia (in 2014) 35% and in Africa (in 2010) 18% of the total agricultural area were planted to mega varieties (Figure 4). Generally, the presence of mega varieties is more evident in Asia than in Africa, which is in line with the overall dominance of improved varieties in Asia and the marginal area planted to landraces. Dominance of mega varieties in Asia differs by crop group. For instance, for roots and tubers, 53% of total area is cultivated to four mega varieties; for pulses, four mega varieties cover 45%; and for cereals, two mega varieties cover 29% of the total area. Also, in Africa, root and tuber mega varieties are the most concentrated: three cover 29% of total area.
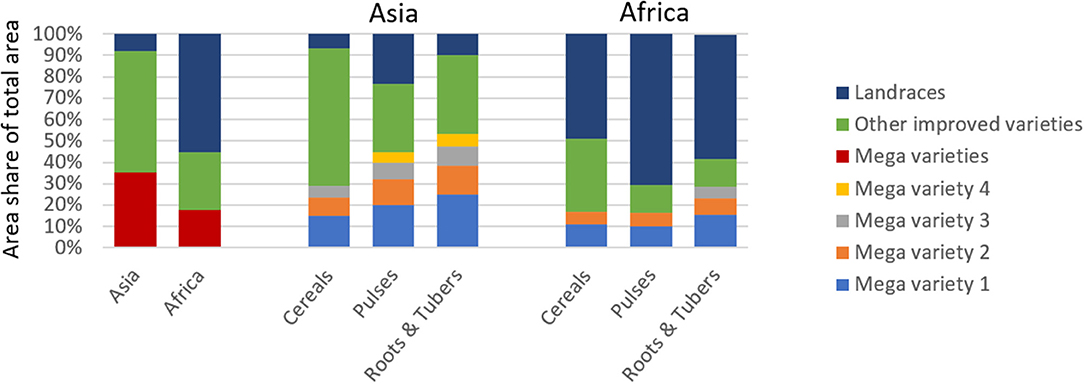
Figure 4. Share of Mega and other Improved Varieties and Landraces of Total Agricultural Area in Asia and Africa. Comparison of mega varieties in terms of their relative share of total area by region and crop group. Variety is defined as mega if variety covers a minimum of 5% of total crop-specific area in a given country.
In both regions, the area planted to other improved varieties is largest for cereal crops, suggesting that relatively more cereal varieties were adopted. Detailed crop-region–specific estimates are presented in Supplementary Figure 3. We further found that area planted to other improved varieties is substantial (57%) in Asia and much smaller in Africa (27%). In contrast, in Africa, two mega varieties jointly cover 17% of area under cereals and 16% of area under pulses, respectively.
Temporal Diversity
Low spatial diversity may—at least partly—be compensated for in cases where agricultural landscapes exhibit high temporal diversity, i.e., if varieties (and seed) were replaced frequently, allowing for higher varietal dynamics. We use the WAVA to describe temporal diversity of improved varieties in cropping systems at the national level (see section Materials and Methods for more details). We found temporal diversity to be low for all countries studied: in Asia, the average adopted improved variety was 17.8 years old and in Africa 18.4 years (Table 2). Available adoption data for Asia and Africa cover different time periods, complicating regional WAVA comparison. Intraregional comparisons show that root and tuber crop varieties have similar WAVA scores as cereals and pulses in both regions. These results point to relatively slow varietal turnover for all crops and regions studied, showing that mega varieties have not been replaced in almost 20 years on average—and even longer for some crops, especially in Asia.
Relationship Between Varietal Richness and Evenness
We calculated varietal richness (i.e., number of adopted varieties) and varietal evenness (i.e., relative varietal abundance) to examine varietal diversity, dominance, uniformity, and their relationship. Details on how richness and evenness were calculated can be found in section Materials and Methods.
We found significant differences between regions and crop groups, using simple t tests. Mean richness values were significantly higher in Asia than in Africa for all crop groups: i.e., 45.5 vs. 16.5 for cereals (P = 0.0042), 24.7 vs. 6.7 for pulses (P = 0.0005), and 20.1 vs. 8.7 for roots and tubers (P = 0.017). In contrast, mean evenness values for pulse and root and tuber crops were higher in Africa than in Asia: 0.59 vs. 0.27 (P = 0.003) and 0.55 vs. 0.39 (P = 0.0015). For cereals, mean evenness values were 0.43 in Africa and 0.38 in Asia and not significantly different (P = 0.187) from each other.
Our data confirm a significant negative correlation between richness and evenness for both regions (Figure 5). This means that with a larger stock of released and thus adopted improved varieties at the national level, agricultural landscapes still tend to be dominated by mega varieties while potentially keeping the large number of minor improved varieties on marginal areas.
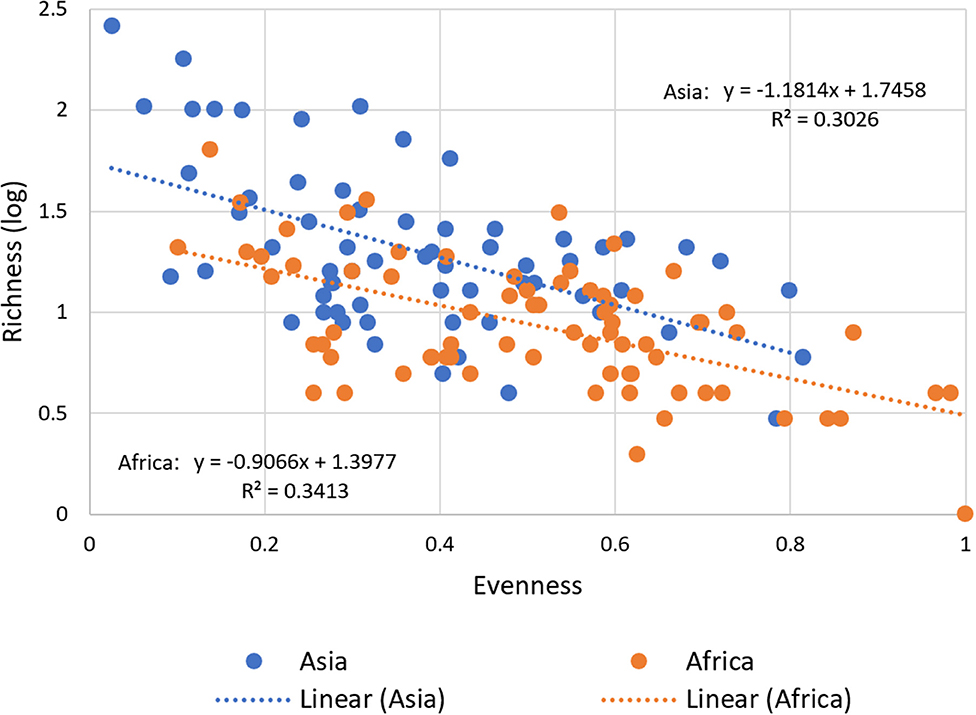
Figure 5. Relationship between Varietal Richness and Evenness in Asia and Africa. For all 137 CCCs, varietal richness, calculated as the number of adopted varieties (in log), is plotted against varietal evenness and calculated as inverse Simpson E index for diversity (see Section Materials and Methods). The correlation between varietal richness and evenness is negative for the total sample (−0.62, P < 0.00) and for the regional sub-sample for Asia (−0.51, P < 0.00) and Africa (−0.52, P < 0.00). An inverse relationship, as predicted, is obtained using a variety-area curve (see Supplementary Figure 4).
We further explored the relationship between varietal richness and evenness by area-weighting the richness index building on research by Jarvis et al. (2008). This results in a crop diversity framework that distinguishes four different diversity categories, as explained in Figure 6, thus further breaking down the immediate use and insurance diversity categories of Jarvis et al. (2008). The quadrants are a combination of high/low richness and high/low evenness values. We interpret high richness as a strategy to spread risk and confront pest, disease, and/or abiotic stresses—and attend to different end-uses—by utilizing a large portfolio of varieties. Evenness is seen as an indicator to equally spread risk or demand across different varieties (Figure 1).
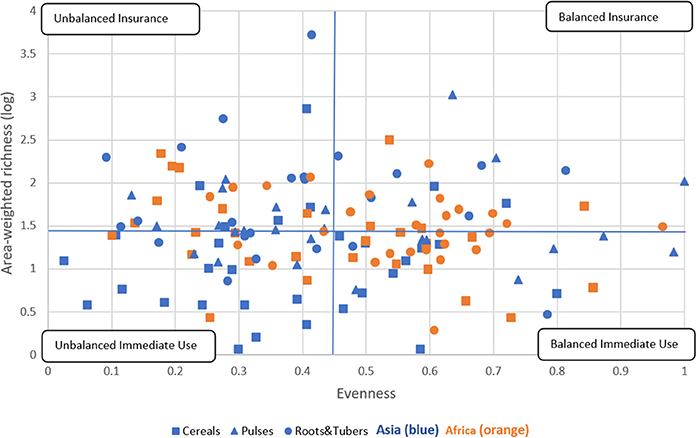
Figure 6. Crop diversity framework—an application. This framework allows comparision of the relative diversity of cereal, pulse, and root and tuber crop varieties in Asia and Africa using all 137 crop-country-combiations. Four quadrants are established using the combination of total mean values of area-weighted richness (1.43) and evenness (0.45). Diversity categories are described in Figure 1. The cropping systems are independently distributed, as the Chi-square test reveals across regions and diversity category (P < 0.005) and across crop group and diversity category (P < 0.009) (see Supplementary Tables 3, 4).
We found that 26 CCCs (19% of total) fall into the balanced insurance quadrant, 38 CCCs (28% of total) into the unbalanced insurance quadrant, 39 CCCs (28% of total) into the balanced immediate use quadrant, and 34 (25% of total) into the unbalanced immediate use quadrant (Supplementary Tables 3, 4). In more detail, cropping systems in the balanced insurance category are relatively more frequent in Africa and for roots and tubers systems, especially cassava. In contrast, cropping systems in the unbalanced immediate use category can mainly be found in Asia, such as cereals, in particular rice systems. Rice systems of all major Asian rice producers, such as China, India, Indonesia, and Bangladesh, fall into this category. In the unbalanced insurance category, all crop groups are included, making it the most equal diversity category. Finally, the balanced immediate use category includes many cereal systems in Asia, many of which are major cereal exporting countries, such as Cambodia, Myanmar, Vietnam (for rice), and Thailand (for maize). In Africa, only pulses, most of which are groundnuts, fall into this category. Data points for all CCCs are listed in Supplementary Data Sheet 1.
Discussion
Crop Improvement and Diversity
While breeding inevitably alters genetic diversity in farming systems, the trends that drive mega varieties challenge diversity enhancement. Variety release procedures, processing requirements, and established consumer preferences are among the multiple factors that tend to favor uniformity. Meanwhile, the impact of crop improvement programs is commonly assessed by acreage or market share, often of a single variety and alternatively as the economic or quantitative footprint of a particular breeding program on a region or a commodity. Genetic diversity is not generally among the values accounted for in the assessment of the impact of crop improvement, and indeed the achievement of mega variety status is highly regarded (Eriksson et al., 2018).
Modern breeding methods can tend to minimize diversity in cropping systems. Especially for inbred crops such as rice, successful modern, high-yielding varieties can cover millions of hectares and serve as homozygous lines for the introduction of single new resistance genes by back crossbreeding. Even as their acreage may decline with time, successors of these mega varieties are mostly their progenies or relatives (Mackill and Khush, 2018). This simplifies variety replacement, whereas overall diversity is minimally altered, leading to the discrepancy between number of varieties and genetic diversity in a system.
Regional Trend Differences in Varietal Releases (1960s−2010s)
A striking difference in the absolute number of total releases of improved varieties between the 1960s−2010s, especially for cereal crops, is evident from our regional comparison. This is the assumed outcome of the Green Revolution of the 1960s when major investments in crop improvement accelerated breeding of cereals in Asia (Evenson and Gollin, 2003a). The considerable regional differences in absolute terms can further be explained by a longer history of investment in breeding in Asia and a comparatively strong support for the dissemination of improved varieties through extension since the beginning of the Green Revolution. In Africa, where a lack of improved varieties responding to farmers' needs and more marginal conditions is evident (Evenson and Gollin, 2003b; Walker and Alwang, 2015), modern breeding for most food crops has only begun to catch up in the past three decades.
Regional differences in the scale of varietal releases are considerably reduced when weighted by total area, with differences across crops and countries. These differences are likely a reflection of the relative investment priorities given to different crop groups. For example, cassava—a key food security crop—has attracted significant recent investment from international and national breeding programs in Africa (Alene et al., 2018).
Varietal Diversity Is Decreasing in Africa and Asia
The land mass dedicated to agriculture and the share of improved varieties increased steadily in both regions between 1970 and 2010. We showed that the land share dedicated to landraces decreased drastically, particularly in Asia, which inevitably led to the loss of on-farm diversity and genetic erosion. This tendency has led to investments in gene banks to safeguard landraces under the common notion that varietal displacement is inevitable (Frankel and Hawkes, 1975; Brush, 2004). Yet, also in Asia, there are still many regions where landrace diversity remains valued for local diets, high-value niche markets, culinary quality, suitability to marginal environments, or cultural preferences (Schiller et al., 2006; Roy et al., 2015; Umakanth et al., 2017). Areal displacement does not always result in varietal replacement and loss.
The above is also true for the African continent where on-farm diversity has been shown to hold continued value for climate change adaptation, markets, and culture (Mwongera et al., 2014; Bellon et al., 2016). Smallholders frequently grow both landraces and improved varieties for different end uses. Even though areal displacement is occurring, the intensification and simplification of agriculture may not necessarily lead to a one-on-one wipeout of landrace diversity (Van de Wouw et al., 2009; Zimmerer and De Haan, 2018). However, there is clearly a knowledge gap as far as the contemporary observation status of cereal, pulse, and root and tuber landrace diversity under smallholder farmer management is concerned (Mercer et al., 2019). In addition, while landraces were being displaced, the share of improved varieties drastically increased between 1970 and 2010. In particular in Africa, the area share of improved varieties increased from 25 to 44% between 1998 and 2010. Despite this drastic jump, given not only data limitations but also context specificity, it is difficult to predict if Africa will follow a similar trend as observed in Asia, if the share of improved varieties will stagnate or even start to decrease. Only novel primary data will be able to confirm this trend.
The Emergence and Dominance of Mega Varieties
The large share of mega varieties in both Asia and Africa, with a comparatively higher dominance among roots and tubers compared to cereals and pulses, leads to questions about underlying drivers. What makes a variety become a mega variety? And why is it so difficult to replace them or diversify varietal portfolios? Clearly, mega varieties are characterized by wide environmental adaptability and consumer acceptability, desirable quality characteristics, and well-established market channels (Pandey et al., 2012). However, additional factors drive their comparative dominance. First, a founder effect can be recognized. Mega varieties are often early generation releases, and after their establishment in the 1960s−1980s, it has become proportionally more difficult to replace them. This is possibly related to the perception that new benefits and proportion gains from new varieties are generally not as high when compared to the initial benefits of mega varieties. A clear example relates to the rates of genetic gains for yield, which have proportionally slowed down during recent decades (Li et al., 2018; Schauberger et al., 2018). Second, a process alignment effect can firmly anchor mega varieties, especially if these varieties are strongly linked to seed systems or postharvest practices requiring standardized processes for selection, processing, or other forms of value addition. Third, a conformity effect can maintain the status quo. All types of preferences ranging from familiarity, handling, taste, to quality aspects are known to fix the market share for established varieties and add to the challenge of reshaping market demand for new varieties. Once a mega variety becomes dominant, there is a decline in the overall area of other varieties grown, assuming area is not expanding. Inertia to adopt new varieties will likely remain high in the absence of a strong demand from the producers, the industry, and consumers along with necessary support from seed systems (Mackill, 2018).
We found low temporal varietal diversity across all crops and regions, suggesting a low varietal turnover for the last two to three decades. Intraregional comparisons show that root and tuber crop varieties have similar WAVA scores as cereals and pulses in both regions. This is surprising, given the generally longer time required to fully adopt new root and tuber crop varieties due to slow rates and high cost of seed multiplication (Mateus-Rodriguez et al., 2013; Scott, 2020). Comparison of WAVA clearly suggests that temporal diversity cannot offset the trend of decreasing spatial diversity found for cereals, pulses, or roots and tubers. On the contrary, this highlights that the spatial dominance of mega varieties is linked to the longevity of these same varieties, despite the increased investment in breeding and number of varietal releases. Again, this may partially be explained by demand, preferences, and processes inherent to the effects outlined above (i.e., founder, process alignment, and conformity). It should be noted that the low decadal varietal turnover sensed from a crop improvement perspective may be relatively high from the crop evolutionary and genetic point of view, with a few exceptions (Vigouroux et al., 2011). In addition, we refrain from interpretations of interregional comparisons due to the differences in the years of observation. Nevertheless, we conjecture that varietal and seed turnover in African countries are lower compared to Asia, considering the comparative importance of the formal sector and farmer access to seed. To make substantiated claims of this nature, however, varietal release and adoption data need to be updated.
Negative Tradeoff Between Varietal Richness and Relative Abundance
We found a negative relationship between varietal richness and evenness, suggesting that for both regions, a higher number of adopted improved varieties are associated with larger areas covered by fewer varieties. This represents a clear negative tradeoff between varietal richness and relative abundance and also suggests that new additions from active breeding programs do not necessarily impact overall varietal evenness. It confirms the relative dominance of a few mega varieties in most countries, despite higher levels of adoption of improved varieties. Vice versa, CCCs with few adopted varieties may show higher levels of evenness just because of the relative dominance of mega varieties in combination with low richness. Modern crop improvement followed by varietal release and adoption can thus still lead to important effects at the margins, for example, serving areas characterized by multiple agroecological zones or for smaller market segments.
Toward Better Understanding Varietal Diversity and Resilience
We developed a crop diversity framework to better understand the implications of spatial varietal diversity for agricultural resilience comparing CCCs. We found that many root and tuber systems in Africa, especially for cassava, fall into the balanced insurance category, providing a degree of resilience contributing to regional food security. The relatively high diversity of landraces in Africa likely positively enforces this.
Our research shows that increased varietal richness from breeding may not actually affect the relative varietal distribution and turnover in the field. Investment in crop improvement under climate change can arguably affect a country's capacity to quickly respond to stresses and shocks, but harnessing the resilience from varietal diversity—rather than resistance and robustness alone—is likely undermined by the dependence on a few dominant mega varieties. The results of the crop diversity framework are useful for policymakers to assess the relative degree by which cropping systems benefit from varietal diversity and provide additional resilience to mitigate climate-related shocks.
Our overall findings, which point to an increasing varietal homogenization of key food cropping systems, are cause for concern because food production is jeopardized, especially in developing countries in Africa and Asia. At the same time, varietal uniformity—as cause or effect—is in line with the increasing global homogeneity in food supply and consumption, which, in turn, have been reported to cause serious threats to human health and food systems (Khoury et al., 2014). Our findings also contribute to the evidence base that shows that key components of agricultural biodiversity, such as genetic or species diversity, are declining globally (FAO, 2019), further threatening food systems through homogenization.
Our findings further suggest that markets and policies favor mega varieties or fail to provide the incentives for diversification and the rapid uptake of newly released varieties. Founder, process alignment, and conformity effects favor established varieties and provide a barrier for new releases. Furthermore, factors such as conservative breeding strategies, bureaucratic variety release procedures, and poor articulation with seeds systems can slow down diversification through breeding (Louwaars and de Boef, 2012; Turner and Bishaw, 2016). As a result, cropping systems have become increasingly uniform, as far as their varietal composition is concerned, and arguably more vulnerable to biotic and abiotic stresses and shocks.
Toward Enhancing Diversity in Food Systems
Effective varietal diversity on farm requires agile variety turnover based on rapid cycle breeding for agronomically adapted and end-user–preferred or otherwise beneficial traits and well-articulated variety testing and release procedures. Breeding must be accompanied by the active dissemination of new varieties. Meanwhile, the complementary practices, policy, and multidisciplinary partnership approaches and demand creation that may be needed to stimulate scaling of new technologies must be researched and built (Low and Thiele, 2020).
Breeders may explicitly seek to increase within field diversity by the development of multilines or cultivar mixtures. A main objective of “multiline cultivars” (i.e., mixtures of inbred lines bred for phenotypic uniformity of agronomic traits) is to resist dynamic pathogen populations, but it concerns diversity of a particular gene or locus in varieties with otherwise uniform genetic backgrounds, and thus does little to broaden the genetic base of crops. Multiline development is similar to replacement by back crossbreeding—a method used to incorporate one or a few genes into an elite or adapted variety—except that the resulting multilines are grown together in the same field. The uniform genetic background confers homogeneity for most farmer- and consumer- or process-relevant traits, which can simplify variety change. On the other hand, “cultivar mixtures” are mixtures of agronomically compatible cultivars developed without additional breeding for phenotypic uniformity (Mundt, 2002), and these can be expected to enhance diversity more than multiline varieties do.
Atlin et al. (2017) recommend that the objective of breeding and seed systems serving smallholder farmers should be to ensure that they use varieties developed in the last 10 years. Advocating for increased rates of variety turnover, these authors point out that the second wave of Green Revolution varieties released in the late 1970s was developed in a climate different from todays, leaving farmers vulnerable to climate change. Among key requirements for breeding programs to help accelerate variety change, they emphasize access to elite germplasm from other regions, shortened breeding cycles, and multilocation testing systems that adequately sample the target population of environments. Depending on the genetic base and stochastic release and lifespan of improved varieties, such turnover would contribute to enhance temporal and spatial diversity.
Unlike cereal crops for which elite cultivars may be available from regions already experiencing the climate expected for developing country regions, much of the genetic diversity needed to improve root and tuber crops remains with their landraces and wild relatives because of shorter breeding histories and clonal propagation that limits opportunity for recombination. This expands the imperative for the conservation and use of broad genetic resources including landraces, crop wild relatives, and so-called prebred germplasm, as well as encouraging the exchange and use of new elite cultivars. Public domain breeding programs such as those of the CGIAR and its networks that test and make improved germplasm available for small farmers merit increased awareness and continued policy and financial support. Of particular relevance are initiatives that link small farmers and their varietal diversity with markets by developing added-value uses and facilitating access to seed of diverse, traditional, and new, improved varieties (Tobin et al., 2018). Indeed, modern varieties and landraces typically complement each other in annual production cycles and food systems (De Haan et al., 2019).
Breeding efforts to deploy diversity at the landscape level are less precisely defined than those aimed at the field level, but no less influenced by practical considerations such as management and processing that may favor uniformity. The use of a wide base of germplasm, recurrent selection in genetically diverse populations, and open back crossbreeding are some methods used to generate crop varieties that differ significantly from one another. In heterozygous, outbreeding crops such as root and tubers that are bred by such methods, a high number of varieties in the landscape are more likely to imply genetic diversity than would be the case through back crossbreeding of inbred lines. Emerging types of resources such as multiparent advanced generation intercross populations that help dissect the genetic structure of traits and improve breeding populations can also be recommended for broadening the genetic base of crops (Huang et al., 2015).
To increase varietal uptake, breeders need to better understand what varietal traits and attributes targeted users' value. Greater emphasis needs to be placed on methods such as participatory varietal selection (Singh et al., 2014) that include farmers—especially women whose preferences are often overlooked—in feedback loops. On-farm conservation projects are also able to incentivize farmers to keep and benefit from diversity (Bellon et al., 2015). A range of actors in agricultural value chains, such as the private sector, need clear profit incentives to pull diverse varietal portfolios through seed systems and use them in product development. In enhancing varietal and crop diversity, inclusive value chain development by means such as the Participatory Market Chain Approach (Devaux et al., 2009; Bernet et al., 2011) deserves more attention.
Conclusions
We used a large varietal dataset on 137 CCCs including 11 food crops in 44 countries in Asia and Africa to analyze the relationship between modern crop improvement and varietal diversity at national levels. Breeding and the regular release of new varieties are important factors contributing to the transformation of cropping systems and food supply. After the Green Revolution of the 1960s, investment in and intensity of crop improvement accelerated considerably, especially in Asia where yields of major crops increased consistently (Khush, 2001). For example, yields of rice in Thailand, potato in China, and lentils in India increased by 48, 45, and 39%, respectively, between 1970 and 2010 (FAOSTAT, 2019). There is increased global recognition that breeding for environmental sustainability, climate resilience, and nutritional quality should transcend the still predominant focus on yield maximization (Berners-Lee et al., 2018). Varietal diversity can lend itself to achieve sustainability, climate adaptation, and nutrition security. Yet, as shown by our results, it has been negatively impacted in Asia and Africa by the advancement, longevity, and persistence of a few mega varieties.
The results of this study show an increasing reduction of crop varietal diversity linked to the spatial displacement of traditional landraces. This trend occurs at a faster rate in Asia than in Africa. In addition, mega varieties tend to increasingly dominate agricultural landscapes, adding to spatial homogeneity. The results further revealed a negative association between varietal richness and its relative abundance, which challenges the relationship between crop improvement and varietal diversity. In different words, a higher number of adopted varieties are positively associated with the incidence of mega varieties. Whereas crop improvement will remain of critical importance to equip smallholder farmers with new technologies to improve incomes and nutritional status, questions addressing how mega varieties emerge, remain “mega,” and can be replaced are important avenues of future research.
We acknowledge that there are several limitations. Despite the streamlined methodology of the databases used, three common biases exist. First, the importance of crops differs across countries resulting in a crop-importance bias. Experts' knowledge of varietal release and adoption estimates is likely greater and more accurate for more dominant crops. In DIIVA, this bias infrequently led to difficulties identifying experts and thus low expert workshop attendance. Second, the number of dominating varieties also largely varies across our sampled countries resulting in a crop-dominance bias. Estimating varietal adoption of more evenly adopted varieties resulted in much less bias compared with estimations of unevenly (i.e., many varieties adopted on small areas) adopted varieties. Third, country size and number of agro-ecologies differ largely across our sample, which makes it more difficult to estimate adoption rates. This country-size bias was generally minimized by inviting more experts and by organizing several expert elicitation workshops in very large countries, such as China or India.
In addition to challenges associated with data collection, our article is an application of the use of big data, which has its own challenges. To smoothen any future analyses of release and adoption data, the use of survey instruments that are standardized in terms of format, units, language, etc., is recommended. The previously mentioned Agricultural Science and Technology Indicators platform could serve as an ideal host.
We acknowledge that data constraints limit the analysis for comparisons of CCCs and varietal diversity among fields and that the quadrants are based on the internal distribution of our data rather than external reference points. Whereas solving the latter limitation will be challenging, in future applications of the crop diversity framework, the spatial diversity could be based on comparisons of intrafield genetic diversity and using lower aggregates. In addition to this avenue, future research may investigate total varietal diversity (and link to resilience) by including landraces in the analysis. Especially for Africa where more than 50% of agricultural area is planted to landraces, this may result in interesting findings. More research is needed to better understand threshold levels and dimensions of cropping systems that support resilience, and more insights are needed into the resilience of agricultural systems that are composed of various cropping systems that each has its own spatial and temporal diversity levels. Combined analysis of data on pest and disease levels and dynamics and our spatial diversity data would also be an interesting avenue for future research, enabling a test of the relationship between crop diversity and agricultural resilience that our study assumed.
In addition, future research is warranted to collect better data on the varietal category “other varieties.” The objective of the three datasets we accessed was to collect data for the most dominant varieties. Those varieties not considered and captured in the “other varieties” category may thus affect the diversity indices. To get a more accurate picture of varietal diversity, an exhaustive varietal list/database is required, which will remain a challenge for future research.
A further limitation of our study is that national-level analyses may not reflect varietal diversity at lower aggregate levels. However, as a tradeoff, the national-level lens is relevant because pests and diseases may spread effectively and at a high pace with increasing levels of seed system connectivity, better transportation infrastructure, and climate change (Bebber et al., 2013, 2014).
An increasing body of literature has emerged using varietal adoption estimates based on expert opinions (Tsusaka et al., 2015; Rashid and Hossain, 2016; Ochieng et al., 2019; Pradel et al., 2019; Schreinemachers et al., 2019; Gatto et al., 2020), which acknowledge the reliability of perceived adoption in relation to household survey estimates. While expert survey provides only indirect estimates, it is important to note that household surveys may also overestimate or underestimate true adoption rates (Walker and Alwang, 2015; Stevenson et al., 2018). Methods such as DNA fingerprinting will allow for high precision and accuracy in determining genetic identity and unique varieties [e.g., Rabbi et al. (2015)] but require significant additional resources.
Nevertheless, surveys based on expert opinion of the extent to which varieties are grown may fail to correlate with actual diversity because different varieties may be given the same name; the same variety may be given different names, or as mentioned previously, varieties may be very similar to each other. Additional efforts are needed to precisely measure genetic diversity within and among fields dedicated to crop production by, for instance, using DNA fingerprinting methods. Finally, we acknowledge that providing a clear definition of a mega variety is challenging, in particular because of its context specificity. Defining and quantifying mega varieties deserve more attention in future research.
There is no single solution for enhancing crop diversity. We need to acknowledge that what works for one crop and context, or for the same crop in a different situation, may not work as well for another. The crops analyzed in this study are of different nature (i.e., grains, vegetatively propagated) and of different use (e.g., food crop, cash crop) and thrive in different agroecological conditions. Rather than focusing on single solutions, crop diversity will likely only be successfully increased by identifying a range of innovations and incentives suitable for different sectors and crops, at different aggregation levels, and under varying agroecological conditions. In the end, food system sustainability depends on enhancing and maintaining species and varietal diversity.
Data Availability Statement
The datasets presented in this study can be found in online repositories. The names of the repository/repositories and accession number(s) can be found at: http://www.asti.cgiar.org.
Author Contributions
MG, AL, RL, and GH contributed to data collection. MG and SH developed the theoretical framework. MG conducted data analysis. All authors contributed to interpreting the data. MG and SH wrote the manuscript. AL, MB, RL, and GH provided suggestions and input to the text. All authors contributed to the article and approved the submitted version.
Funding
The authors wish to acknowledge financial support from the Standing Panel on Impact Assessment (SPIA) of the Independent Science and Partnership Council under the Strengthening Impact Assessment in the CGIAR (SIAC) program funded by the Bill and Melinda Gates Foundation and other donors. This research was undertaken as part of, and partly funded by, the CGIAR Research Programs on Roots, Tubers, and Bananas (RTB) and on Rice, and supported by CGIAR Fund Donors.
Conflict of Interest
The authors declare that the research was conducted in the absence of any commercial or financial relationships that could be construed as a potential conflict of interest.
Acknowledgments
We are grateful to Julian Bonierbale who copyedited our manuscript.
Supplementary Material
The Supplementary Material for this article can be found online at: https://www.frontiersin.org/articles/10.3389/fsufs.2021.626714/full#supplementary-material
References
Ahuja, M. R., and Mohan Jain, S. (2015). Genetic Diversity and Erosion in Plants. Cham: Springer, 323. doi: 10.1007/978-3-319-25637-5
Alene, A. D., Abdoulaye, T., Rusike, J., Labarta, R., Creamer, B., del Rio, M., et al. (2018). Identifying crop research priorities based on potential economic and poverty reduction impacts: the case of cassava in Africa, Asia, and Latin America. PLoS ONE 13:e0201803. doi: 10.1371/journal.pone.0201803
Anderson, P. K., Cunningham, A. A., Patel, N. G., Morales, F. J., Epstein, P. R., and Daszak, P. (2004). Emerging infectious diseases of plants: pathogen pollution, climate change and agrotechnology drivers. Trends Ecol. Evol. 19, 535–544. doi: 10.1016/j.tree.2004.07.021
Atlin, G. N., Cairns, J. E., and Das, B. (2017). Rapid breeding and varietal replacement are critical to adaptation of cropping systems in the developing world to climate change. Glob. Food Secur. 12, 31–37. doi: 10.1016/j.gfs.2017.01.008
Bebber, D. P. (2015). Range-expanding pest and pathogens in a warming world. Annu. Rev. Phytopathol. 53, 335–356. doi: 10.1146/annurev-phyto-080614-120207
Bebber, D. P., Holmes, T., and Gurr, S. J. (2014). The global spread of crop pests and pathogens. Glob. Ecol. Biogeogr. 23, 1398–1407. doi: 10.1111/geb.12214
Bebber, D. P., Ramotowski, M. A. T., and Gurr, S. J. (2013). Crop pests and pathogens move polewards in a warming world. Nat. Clim. Change 3, 985–988. doi: 10.1038/nclimate1990
Bellon, M. R., Gotor, E., and Caracciolo, F. (2015). Assessing the effectiveness of projects supporting on-farm conservation of native crops: evidence from the high Andes of South America. World Dev. 70, 162–176. doi: 10.1016/j.worlddev.2015.01.014
Bellon, M. R., Ntandou-Bouzitou, G. D., and Caracciolo, F. (2016). On-farm diversity and market participation are positively associated with dietary diversity of rural mothers in Southern Benin, West Africa. PLoS ONE 11:e0162535. doi: 10.1371/journal.pone.0162535
Berners-Lee, M., Kennelly, C., Watson, R., and Hewitt, C. N. (2018). Current global food production is sufficient to meet human nutritional needs in 2050 provided there is radical societal adaptation. Elem. Sci. Anth. 6:52. doi: 10.1525/elementa.310
Bernet, T., Devaux, A., Ortiz, O., and Thiele, G. (2011). “Participatory market chain approach,” in Innovation for Development: The Papa Andina Experience, eds M. O. Devaux, and D. Horton (International Potato Center (CIP)), 133–141.
Bhattacharya, S. (2017). Deadly new wheat disease threatens Europe's crops. Nature 542, 145–146. doi: 10.1038/nature.2017.21424
Bianchi, F. J., Booij, C. J., and Tscharntke, T. (2006). Sustainable pest regulation in agricultural landscapes: a review on landscape composition, biodiversity and natural pest control. Proc. R. Soc. B 273, 1715–1727. doi: 10.1098/rspb.2006.3530
Borrell, J. S., Dodsworth, S., Forest, F., Pérez-Escobar, O. A., Lee, M. A., Mattana, E., et al. (2020). The climatic challenge: which plants will people use in the next century? Environ. Exp. Bot. 170:103872. doi: 10.1016/j.envexpbot.2019.103872
Brennan, J. P., and Byerlee, D. (1991). The rate of crop varietal replacement on farms: measures and empirical results for wheat. Plant Varieties Seeds 4, 99–106.
Brush, S. B. (2004). Farmers Bounty: Locating Crop Diversity in the Contemporary World. London: Yale University Press. doi: 10.12987/yale/9780300100495.001.0001
Callaway, E. (2016). Devastating wheat fungus appears in Asia for first time. Nature 532, 421–422. doi: 10.1038/532421a
Ceccarelli, S., Galie, A., and Grando, S. (2013). “Participatory breeding for climate change-related traits,” in Genomics and Breeding for Climate-Resilient Crops, ed K. Chittaranjan (Heidelberg: Springer), 331–376. doi: 10.1007/978-3-642-37045-8_8
Chakraborty, S., and Newton, A. C. (2011). Climate change, plant diseases and food security: an overview. Plant Pathol. 60, 2–14. doi: 10.1111/j.1365-3059.2010.02411.x
Condori, B., Hijmans, R. J., Ledent, J. F., and Quiroz, R. (2014). Managing potato biodiversity to cope with frost risk in the high Andes: a modeling perspective. PLoS ONE 9:e81510. doi: 10.1371/journal.pone.0081510
Dalkey, N., and Helmer, O. (1963). An experimental application of the DELPHI method to the use of experts. Manage. Sci. 9, 458–467. doi: 10.1287/mnsc.9.3.458
De Haan, S., Burgos, G., Liria, R., Rodriguez, F., Creed-Kanashiro, H. M., and Bonierbale, M. (2019). The nutritional contribution of potato varietal diversity in Andean food systems: a case study. Am. J. Potato Res. 96, 151–163. doi: 10.1007/s12230-018-09707-2
De Haan, S., Nuñez, J., Bonierbale, M., and Ghislain, M. (2010). Multilevel agrobiodiversity and conservation of Andean potatoes in the central Andes: species, morphological, genetic and spatial diversity. Mt. Res. Dev. 30, 222–231. doi: 10.1659/MRD-JOURNAL-D-10-00020.1
Devaux, A., Horton, D., Velasco, C., Thiele, G., López, G., Bernet, T., et al. (2009). Collective action for market chain innovation in the Andes. Food Policy 34, 31–38. doi: 10.1016/j.foodpol.2008.10.007
Elad, Y., and Pertot, I. (2014). Climate change impacts on plant pathogens and plant diseases. J. Crop Improv. 28, 99–139. doi: 10.1080/15427528.2014.865412
Eriksson, D., Akoroda, M., Azmach, G., Labuschagne, M., Mahungu, N., and Ortiz, R. (2018). Measuring the impact of plant breeding on sub-Saharan African staple crops. Outlook Agric. 47, 163–180. doi: 10.1177/0030727018800723
Evenson, R. E., and Gollin, D. (2003a). Assessing the impact of the green revolution, 1960 to 2000. Science 300, 758–762. doi: 10.1126/science.1078710
Evenson, R. E., and Gollin, D. (2003b). “Crop genetic improvement in developing countries: overview and summary,” in Crop Variety Improvement and Its Effect on Productivity: The Impact of International Agricultural Research (Cambridge, MA: CABI Publishing), 7–38. doi: 10.1079/9780851995496.0007
FAO (2019). “The state of the world's biodiversity for food and agriculture,” in FAO Commission on Genetic Resources for Food and Agriculture Assessments, eds J. Bélanger, and D. Pilling (Rome: FAO), 572. Available online at: http://www.fao.org/3/CA3129EN/CA3129EN.pdf (accessed September 2, 2019).
FAOSTAT (2019). Food and Agriculture Organization of the United Nations Database Collections. Rome: FAO (accessed June 11, 2018).
Frankel, O. H., and Hawkes, J. G. (1975). Crop Genetic Resources for Today and Tomorrow (Vol. 2). CUP Archive.
Frison, E. A., Cherfas, J., and Hodgkin, T. (2011). Agricultural biodiversity is essential for a sustainable improvement in food and nutrition security. Sustainability 3, 238–253. doi: 10.3390/su3010238
Fu, Y. B. (2015). Understanding crop genetic diversity under modern plant breeding. Theor. Appl. Genet. 128, 2131–2142. doi: 10.1007/s00122-015-2585-y
Gao, L. Z. (2003). The conservation of Chinese rice biodiversity: genetic erosion, ethnobotany and prospects. Genet. Res. Crop Evol. 50, 17–32. doi: 10.1023/A:1022933230689
Gatto, M., Petsakos, A., and Hareau, G. (2020). Sustainable intensification of rice-based systems with potato in eastern indo-gangetic plains. Am. J. Potato Res. 97, 162–174. doi: 10.1007/s12230-020-09764-6
He, H. M., Liu, L. N., Munir, S., Bashir, N. H., Yi, W., Jing, Y., et al. (2019). Crop diversity and pest management in sustainable agriculture. J. Integr. Agric. 18, 1945–1952. doi: 10.1016/S2095-3119(19)62689-4
Huang, B. E., Verbyla, K. L., Verbyla, A. P., Raghavan, C., Singh, V. K., Gaur, P., et al. (2015). MAGIC populations in crops: current status and future prospects. Theor. Appl. Genet. 128, 999–1017. doi: 10.1007/s00122-015-2506-0
Jarvis, D. I., Brown, A. H. D., Cuong, P. H., Collado-Panduro, L., Latournerie-Moreno, L., Gyawali, S., et al. (2008). A Global perspective of the richness and evenness of traditional crop-variety diversity maintained by farming communities. Proc. Natl. Acad. Sci. U.S.A. 105, 5326–5331. doi: 10.1073/pnas.0800607105
Jones, A. (2017). Critical review of the emerging research evidence on agricultural biodiversity, diet diversity, and nutritional status in low- and middle-income countries. Nutr. Rev. 75, 769–782. doi: 10.1093/nutrit/nux040
Keneni, G., Bekele, E., Imtiaz, M., and Dagne, K. (2012). Genetic vulnerability of modern crop cultivars: causes, mechanism and remedies. Int. J. Plant Res. 2, 69–79. doi: 10.5923/j.plant.20120203.05
Khoury, C. K., Bjorkman, A. D., Dempewolf, H., Ramirez-Villegas, J., Guarino, L., Jarvis, A., et al. (2014). Increasing homogeneity in global food supplies and the implications for food security. Proc. Natl. Acad. Sci. U.S.A. 11, 4001–4006. doi: 10.1073/pnas.1313490111
Khush, G. S. (2001). Green revolution: the way forward. Nat. Rev. Genet. 2, 815–822. doi: 10.1038/35093585
Launio, C. C., Redondo, G. O., Beltran, J. C., and Morooka, Y. (2008). Adoption and spatial diversity of later generation modern rice varieties in the Philippines. Agron. J. 100, 1380–1389. doi: 10.2134/agronj2007.0297
Li, H., Rasheed, A., Hickey, L. T., and He, Z. (2018). Fast-forwarding genetic gain. Trends Plant Sci. 23, 184–186.
Lin, B. B. (2011). Resilience in agriculture through crop diversification: adaptive management for environmental change. BioScience 61, 183–193. doi: 10.1525/bio.2011.61.3.4
Lipper, L., Anderson, L., and Dalton, T. J. (2010). Seed Trade in Local Markets: Implications for Crop Diversity and Agricultural Development. London; Sterling, VA: Food and Agriculture Organization of the United Nations and Earthscan, 232.
Louwaars, N. P., and de Boef, W. S. (2012). Integrated seed sector development in Africa: a conceptual framework for creating coherence between practices, programs, and policies. J. Crop Improv. 26, 39–59. doi: 10.1080/15427528.2011.611277
Low, J. W., and Thiele, G. (2020). Understanding innovation: the development and scaling of orange-fleshed sweetpotato in major African food systems. Agric. Syst. 179:102770. doi: 10.1016/j.agsy.2019.102770
Mackill, D. J. (2018). Special issue: iconic rice varieties. Rice 11:16. doi: 10.1186/s12284-018-0214-5
Mackill, D. J., and Khush, G. S. (2018). IR64: a high-quality and high-yielding mega variety. Rice 11, 1–11. doi: 10.1186/s12284-018-0208-3
Maredia, M., and Reyes, B. (2014). Guidelines for collecting varietal release and adoption data. Objective 2.1. “Organize the collection of crop germplasm improvement research related direct outcomes”, Strengthening Impact Assessment in CGIAR (SIAC) Project. Available online at: https://ispc.cgiar.org/sites/default/files/docs/Guidelines-SIAC21-Activity_v7-4-25-14.pdf (accessed January 2021).
Mateus-Rodriguez, J. R., de Haan, S., Andrade-Piedra, J. L., Maldonado, L., Hareau, G., Barker, I., et al. (2013). Technical and economic analysis of aeroponics and other systems for potato mini-tuber production in Latin America. Am. J. Potato Res. 90, 357–368. doi: 10.1007/s12230-013-9312-5
Mercer, K. L., Vigouroux, Y., Castañeda-Álvarez, N. P., De Haan, S., Hijmans, H. J., Leclerc, C., et al. (2019). “Crop evolutionary agroecology: genetic and functional dimensions of agrobiodiversity and associated knowledge,” in Agrobiodiversity: Integrating Knowledge for a Sustainable Future, eds K. S. Zimmerer, and S. de Haan (Cambridge: The MIT Press), 21–62.
Mundt, C. C. (2002). Use of multiline cultivars and cultivar mixtures for disease management. Annu. Rev. Phytopathol. 40, 381–410. doi: 10.1146/annurev.phyto.40.011402.113723
Mwongera, C., Boyard-Micheau, J., Baron, C., and Leclerc, C. (2014). Social process of adaptation to environmental changes: how Eastern African societies intervene between crops and climate. Weather Climate Soc. 6, 341–353. doi: 10.1175/WCAS-D-13-00034.1
Ochieng, J., Schreinemachers, P., Ogada, M., Dinssa, F. F., Barnos, W., and Mndiga, H. (2019). Adoption of improved amaranth varieties and good agricultural practices in East Africa. Land Use Policy 83, 187–194. doi: 10.1016/j.landusepol.2019.02.002
Pandey, S., Gauchan, D., Malabayabas, M., Bool-Emerick, M., and Hardy, B. (2012) Patterns of Adoption of Improved Rice Varieties Farm-Level Impacts in Stress-Prone Rainfed Areas in South Asia. Los Banos: International Rice Research Institute, 318.
Perales, H., and Golicher, D. (2014). Mapping the diversity of maize races in Mexico. PLoS ONE 9:e114657. doi: 10.1371/journal.pone.0114657
Pradel, W., Gatto, M., Hareau, G., Pandey, S. K., and Bhardway, V. (2019). Adoption of potato varieties and their role for climate change adaptation in India. Clim. Risk Manage. 23, 114–123. doi: 10.1016/j.crm.2019.01.001
Rabbi, I. Y., Kulakow, P. A., Manu-Aduening, J. A., Dankyi, A. A., Asibuo, J. Y., Parkes, E. Y., et al. (2015). Tracking crop varieties using genotyping-by-sequencing markers: a case study using cassava (Manihot esculenta Crantz). BMC Genet. 16:115. doi: 10.1186/s12863-015-0273-1
Rashid, M. A., and Hossain, T. M. B. (2016). Adoption of wheat varieties in Bangladesh: expert elicitation approach. Bangladesh J. Agric. Res. 41, 491–505. doi: 10.3329/bjar.v41i3.29721
Reiss, E. R., and Drinkwater, L. E. (2018). Cultivar mixtures: a meta-analysis of the effect of intraspecific diversity on crop yield. Ecol. Appl. 28, 62–77. doi: 10.1002/eap.1629
Roy, S., Banerjee, A., Mawkhlieng, B., Misra, A. K., Pattanayak, A., Harish, G. D., et al. (2015). Genetic diversity and population structure in aromatic and quality rice (Oryza sativa L.) landraces from North-Eastern India. PLoS ONE 10:e0141405. doi: 10.1371/journal.pone.0141405
Savary, S., Willocquet, L., Pethybridge, S. J., Esker, P., McRoberts, N., and Nelson, A. (2019). The global burden of pathogens and pests on major food crops. Nat. Ecol. Evol. 3, 430–439. doi: 10.1038/s41559-018-0793-y
Schauberger, B., Ben Ari, T., Makowski, D., Kato, T., Kato, D., and Ciais, P. (2018). Yield trends, variability and stagnation analysis of major crops in France over more than a century. Sci. Rep. 8:16865. doi: 10.1038/s41598-018-35351-1
Schiller, J. M., Chanphengxay, M. B., Linquist, B., and Appa Rao, S. (2006). Rice in Laos. Los Baños: International Rice Research Institute (IRRI).
Schreinemachers, P., Sequeros, T., Rani, S., Md Rashid, A., Gowdru, N. V., Rahman, M. S., et al. (2019). Counting the beans: quantifying the adoption of improved mungbean varieties in South Asia and Myanmar. Food Sec. 11, 623–634. doi: 10.1007/s12571-019-00926-x
Scott, G. J. (2020). A review of root, tuber and banana crops in developing countries: past, present and future. Int. J. Food Sci. Technol. 1–22. doi: 10.1111/ijfs.14778
Silver, E. (2019). Caterpillar's devastating march across China spurs hunt for native predator. Nature 570, 286–287. doi: 10.1038/d41586-019-01867-3
Singh, R. P., Hodson, D. P., Jin, Y., Lagudah, E. S., Ayliffe, M. A., Bhavani, S., Rouse, M. N., et al. (2015). Emergence and spread of new races of wheat stem rust fungus: continued threat to food security and prospects of genetic control. Phytopathology 105, 872–884. doi: 10.1094/PHYTO-01-15-0030-FI
Singh, Y. P., Nayak, A. K., Sharma, D. K., Gautam, R. K., Singh, R. K., Singh, R., et al. (2014). Farmers' participatory varietal selection: a sustainable crop improvement approach for the 21st century. Agroecol. Sust. Food Syst. 38, 427–444. doi: 10.1080/21683565.2013.870101
Smale, M. (1998). Farmers, Gene Banks and Crop Breeding: Economic Analyses of Diversity in Wheat, Maize, and Rice. Boston, MA: Kluwer Academic Publisher, 270. doi: 10.1007/978-94-009-0011-0_1
Spielman, D., and Smale, M. (2017). Policy Options to Accelerate Variety Change Among Smallholder Farmers in South Asia and Africa South of the Sahara. IFPRI Discussion Paper 01666.
Stevenson, J., Macours, K., and Gollin, D. (2018). The Rigor Revolution in Impact Assessment: Implications for CGIAR. Rome: Independent Science and Partnership Council (ISPC).
Thormann, I., and Engels, J. M. M. (2015). “Genetic diversity and erosion – a global perspective,” in Genetic Diversity and Erosion in Plants, eds M. R. Ahuja, and S. Mohan Jain (Cham: Springer), 263–294. doi: 10.1007/978-3-319-25637-5_10
Tobin, D., Bates, R., Brennan, M., and Gill, T. (2018). Peru potato potential: biodiversity conservation and value chain development. Renew. Agric. Food Syst. 33, 19–32. doi: 10.1017/S1742170516000284
Tsusaka, T., Velasco, L., Yamano, T., and Pandey, S. (2015). Expert elicitation for assessing agricultural technology adoption: the case of improved rice varieties in South Asian countries. Asian J. Agric. Dev. 12, 19–33. Available online at: https://ajad.searca.org/read-articles/13-view-article?aid=490
Turner, M. R., and Bishaw, Z. (2016). A Review of Variety Release Procedures and Related Issues with Recommendations for Good Practices. ICARDA Working Paper 32, Beirut. Available online at: https://hdl.handle.net/20.500.11766/6362 (accessed January 2021).
Umakanth, B., Vishalakshi, B., Kumar, P. S., Rama Devi, S. J. S., Bhadana, V. P., Senguttuvel, P., et al. (2017). Diverse rice landraces of North-East India enables the identification of novel genetic resources for magnaporthe resistance. Front. Plant Sci. 8:1500. doi: 10.3389/fpls.2017.01500
Van de Wouw, M., Kik, C., Van Hintum, T., Van Treuren, R., and Visser, B. (2009). Genetic erosion in crops: concept, research results and challenges. Plant Genetic Resour. Char. Util. 8, 1–15. doi: 10.1017/S1479262109990062
Vigouroux, Y., Mariac, C., De Mita, S., Pham, J. L., Gérard, B., Kapran, I., et al. (2011). Selection for earlier flowering crop associated with climatic variations in the Sahel. PLoS ONE 6:e19563. doi: 10.1371/journal.pone.0019563
Walker, T. S., and Alwang, J. (2015). Crop Improvement, Adoption and Impact of Improved Varieties in Food Crops in Sub-Saharan Africa. Wallingford: CGIAR and CAB International, 450. doi: 10.1079/9781780644011.0000
Xu, Z., Tang, Y., Connor, T., Li, D., and Liu, J. (2017). Climate variability and trends at a national scale. Sci. Rep. 7:3258. doi: 10.1038/s41598-017-03297-5
Yachi, S., and Loreau, M. (1999). Biodiversity and ecosystem productivity in a fluctuating environment: the insurance hypothesis. Proc. Natl. Acad. Sci. U.S.A. 96, 1463–1468. doi: 10.1073/pnas.96.4.1463
Yang, L. N., Pan, Z. C., Zhu, W., Wu, E. J., He, D. C., Yuan, X., et al. (2019). Enhanced agricultural sustainability through within-species diversification. Nat. Sust. 2, 46–52. doi: 10.1038/s41893-018-0201-2
Zhang, H., John, R., Peng, Z., Yuan, J., Chu, C., Du, G., et al. (2012). The relationship between species richness and evenness in plant communities along a succession gradient: a study from sub-alpine meadows of the Eastern Qinghai-Tibetan Plateau, China. PLoS ONE 11:e49024. doi: 10.1371/journal.pone.0049024
Keywords: crop improvement, varietal diversity, richness, evenness, resilience, Africa, Asia
Citation: Gatto M, de Haan S, Laborte A, Bonierbale M, Labarta R and Hareau G (2021) Trends in Varietal Diversity of Main Staple Crops in Asia and Africa and Implications for Sustainable Food Systems. Front. Sustain. Food Syst. 5:626714. doi: 10.3389/fsufs.2021.626714
Received: 06 November 2020; Accepted: 07 January 2021;
Published: 23 February 2021.
Edited by:
Matteo Balderacchi, Independent Researcher, Piacenza, ItalyReviewed by:
James S. Borrell, Royal Botanic Gardens, Kew, United KingdomMatteo Dainese, Eurac Research, Italy
Copyright © 2021 Gatto, de Haan, Laborte, Bonierbale, Labarta and Hareau. This is an open-access article distributed under the terms of the Creative Commons Attribution License (CC BY). The use, distribution or reproduction in other forums is permitted, provided the original author(s) and the copyright owner(s) are credited and that the original publication in this journal is cited, in accordance with accepted academic practice. No use, distribution or reproduction is permitted which does not comply with these terms.
*Correspondence: Marcel Gatto, bS5nYXR0b0BjZ2lhci5vcmc=