- 1Department of Entomology, Michigan State University, East Lansing, MI, United States
- 2George Perkins Marsh Institute, Clark University, Worcester, MA, United States
- 3Department of Entomology, Purdue University, West Lafayette, IN, United States
While research suggests that pollinator decline is linked with agricultural practices, it is unclear whether farmers share this view and adapt management to promote pollinators based on their understanding of these threats. To address these issues, we surveyed farmers of pollinator-dependent cucurbit crops across four states in the Midwest, USA. We grouped farmers by their perceptions of pollinator declines and routes of pesticide exposure and used statistical models to evaluate if farmers manage pests and pollinators based on these perceptions. Out of 93 completed surveys, 39% of farmers believed pollinators were in decline. When grouped, 17% of farmers were classified as proponents, ranking (on a 1–5 Likert scale) the factors mediating pesticide exposure and pollinator declines as important or highly important. For comparison, 44 and 39% of farmers were classified as neutral or skeptical, respectively, of these same factors. Compared to the neutral and skeptic groups, proponents were on average younger, had fewer years farming but more years in family farming, and were more dependent on income from outside the farming system. Proponents also on average reported smaller farms, higher pest richness, more land in cucurbit production, and greater richness of crops that are not pollinator dependent, when compared to the neutrals and skeptics. We did not find pest and pollinator management to be related to farmer perceptions of pollinator decline or routes of pesticide exposure, but farmers classified as pollinator “proponents” were more likely to indicate participation in future pollinator habitat restoration programs. Rather, management strategies were better explained by on-farm environmental conditions (e.g., pest richness, farm size, number of pollinator dependent crops) and economic factors (e.g., sources of income). Generally, our research shows that farmers who perceive pollinator threats may not be using pollinator supportive practices. Thus, while some farmers believe in pollinator declines, there remains a need to connect this knowledge with on-farm practices.
Introduction
The decline of invertebrate biodiversity is due, in part, to industrialized farming practices used at a global scale (Hall and Steiner, 2019; Hall and Martins, 2020). More specifically, the decline of bees (Hymenoptera: Apoidea) due to farming practices (e.g., pesticide use) are concerning, given that fruit and vegetable crops rely on healthy bee communities to promote pollination (Klein et al., 2007) and the loss of bees may reduce crop productivity and human health (Potts et al., 2016). While practices that support pollinators on farms have been identified, their adoption is limited (Kleijn et al., 2018). Thus, understanding the barriers to farmer adoption of pollinator-supporting practices is important for sustaining agroecosystems and long-term food security.
Farmers of pollinator-dependent crops rely on two general approaches for enhancing pollination visitation to crop flowers: (1) the creation or management of pollinator nesting and foraging habitat, and (2) the reduction or alteration of pesticide use. Most farmers of pollinator-dependent crops in developed countries understand the importance of pollinators for crop production (Hanes et al., 2013; Gaines-Day and Gratton, 2017; Hevia et al., 2020; Park et al., 2020), while this knowledge is less predominant in developing countries (Kasina et al., 2009; Munyuli, 2011; Sawe et al., 2020). In addition, many farmers have positive attitudes toward pollinator conservation and perceive declines in on-farm pollinator biodiversity, but these perceptions do not imply conservation actions (Hanes et al., 2013; Westlake, 2019; Hevia et al., 2020). Thus, there appears to be a disconnect between farmer knowledge of pollinator importance and investment in pollinator habitat conservation (Hoshide et al., 2018), indicating contemporary approaches to promote pollinators may not align with the beliefs and production practices of farmers (Melathopoulos, 2015). Moreover, farmers historically (and erroneously) have been treated as a homogenous group in terms of their beliefs. But rather, farmer beliefs, demographics, and economics vary, and these factors all mediate the adoption of pollinator supportive production practices (Kaine and Bewsell, 2008; Wilson et al., 2009; Dicks et al., 2016).
Pesticide use on farms is a contributor to pollinator decline, though, the cause of pollinator decline is diffuse and generally attributed to a combination of factors including pesticides (Goulson et al., 2015; Wagner et al., 2021). Farmers use pesticides to reduce insect damage to crops, however, they have non-target impacts on insect pollinator health (Biddinger and Rajotte, 2015; Egan et al., 2020). Thus, farmers growing pollinator-dependent crops are faced with a potential tradeoff among two key production inputs, pollination and pesticides, and co-management of pests and pollinators is needed to promote yields (Ternest et al., 2020). In developed countries, farmers are generally aware of the ramifications of direct spraying of insecticides on pollinators (Dicks et al., 2013; Bhattacharyya et al., 2017) and often refrain from doing so (Hevia et al., 2020; Nalepa et al., 2020; Park et al., 2020). However, the literature is silent on farmers' understanding of other potential routes of pesticide exposure (e.g., residues left in the soil and planting dust). While greater awareness of farming practices that promote biodiversity (e.g., integrated pest management [IPM]) can reduce pesticide use and pollinator exposure (Blake et al., 2006; Schreinemachers et al., 2017), the factors mediating the adoption of practices that reduce the full spectrum of pollinator pesticide threats remain unknown.
To address this complex issue, we surveyed farmers of pollinator-dependent crops across four states in the Midwest, USA. We then grouped farmers by their perceptions of pollinator declines and routes of pesticide exposure and used statistical models to evaluate if farmers manage pests and pollinators based on their pollinator perceptions, farm characteristics, and demographics. Our findings show wide variation in the level of importance that farmers attribute to threats to pollinators and demonstrate a need to promote connections between pollinator knowledge and the practices used within farms.
Methods
Study System
Our study was conducted in pollinator-dependent cucurbit (Cucurbitaceae) (cucumber [Cucumis sativus], melon [Citrullus lanatus], squash [Cucurbita moschara], pumpkin [Cucurbita pepo]) crops of the Midwest, USA (Michigan, Ohio, Indiana, Illinois) (Figure 1). Midwest farms are dominated by row crops such as corn and soybean (Meehan and Gratton, 2016) with pollinator-dependent specialty crops geographically restricted to match suitable abiotic conditions for crop production (MWVG, 2020). Illinois, Indiana, and Ohio are part of the “corn belt” with ~35% of the landscape in corn and soybean production and <0.05% of land in cucurbit crops (USDA NASS, 2019). Farm size is variable across cucurbit crops and the states within our study region (Figure 1). For example, >50% of pumpkins across all states in our study region are grown on small farms with <15 acres in production. Overall, though, large scale producers (>100 acres), ~3% of cucurbit farmers, grow cucurbits on approximately two-thirds of all acres in production (USDA NASS, 2017). Cucurbit farmers rely on pesticides and IPM measures (e.g., crop rotation) to manage pests that reduce yields (Sánchez et al., 2018; MWVG, 2020). However, cucurbits also rely on bees for fruit production (McGregor, 1976) and these pollinators are at risk from pesticide exposure.
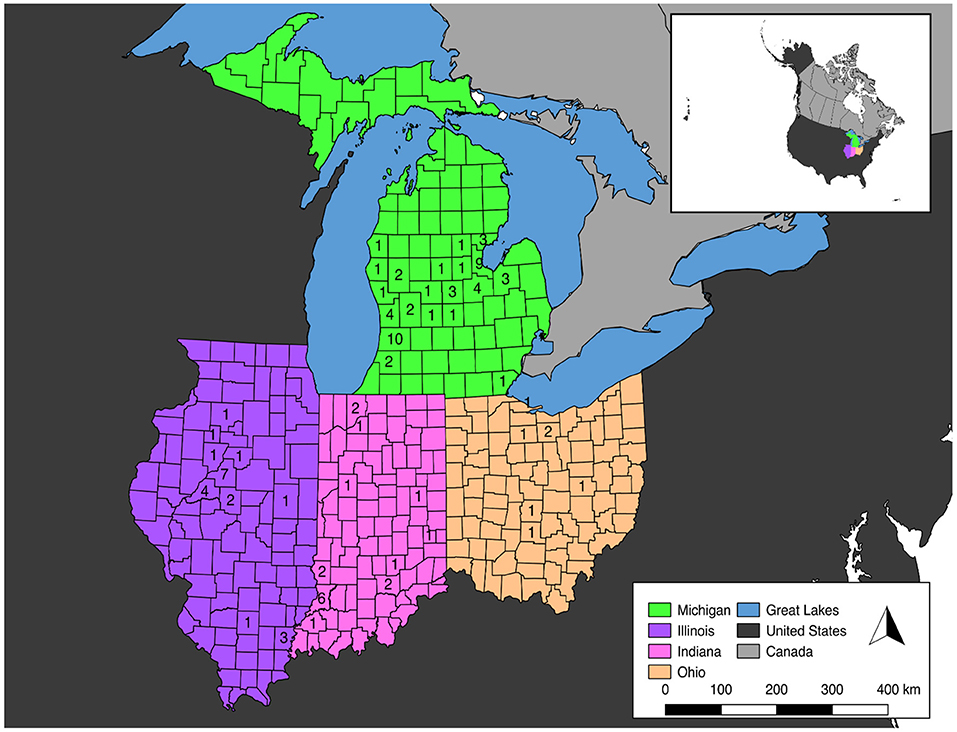
Figure 1. Map of study region showing distribution of surveys across the Midwest, USA. Farmers from 47 counties responded to our survey. Numbers on counties represent the total number of surveys from the given county.
Farmer Survey
A survey of cucurbit farmers was developed to elicit information regarding farmers' knowledge, perceptions, and behaviors regarding pest management, pollination strategies, and potential tradeoffs among these two key inputs to pollinator-dependent crop production (see Supplementary Tables 1–4 for survey questions). The multi-step design process included individual interviews, focus groups, and survey instrument pre-tests with farmers, extension personnel, and members of the research team (Mitchell and Carson, 1989). The final survey instrument had multiple sections with questions related to farm and farmer characteristics, current pest and pollination management practices, views on pollinator health and risks, and willingness to adopt pollinator best management practices. This paper reports on a subset of the survey (see Supplementary Tables 1–4); other questions are analyzed elsewhere.
The survey was mailed in early 2019 to a list of 2,543 cucurbit farmers across our study area (Figure 1) purchased from FarmMarketID (www.farmmarketid.com), an agri-business market research firm. We followed a multi-step mailing process (January through March) that included an introductory letter, a survey instrument, a reminder postcard, a second survey instrument to non-respondents, and a final reminder postcard to non-respondents (Dillman et al., 2008). By the end of May, we were contacted (via phone, email, or written correspondence) by 978 survey recipients (38% of the mailing sample), most frequently via a prepaid postcard included in the survey package to maintain respondent anonymity. Only 74 postcards specified that growers had returned a completed survey, although we ultimately received 106 mostly complete and 15 partially complete surveys. We subsequently removed 900 names from our potential respondent pool of 2,543 because the survey was undeliverable or, after hearing directly from the mail recipient, they did not meet our sampling criteria (i.e., the recipient was retired or deceased, had never grown cucurbits, had not grown cucurbits in the previous 5 years, only grew cucurbits in a home garden for personal use, or had never farmed [e.g., resided in a new residential subdivision on former farmland]). It is impossible for us to calculate a legitimate response rate, because more than 68% of the survey recipients that we heard from did not grow cucurbit crops. We ultimately received 106 complete or mostly complete, usable surveys from cucurbit farmers.
Farmer Perceptions
Farmers who perceive severe threats to pollinator populations are more likely to engage in pollinator-supporting practices (Nalepa et al., 2020). To assess farmers perceptions of the status of pollinators, we first asked farmers if they thought wild pollinator populations were in decline (yes, no, not sure). We then questioned farmers perceptions of changes to the availability and quality of managed bees over the past 5 years (increased, decreased, no change, or not sure). Declines in pollinators are thought to be driven by diverse and numerous stressors including habitat loss and degradation, agricultural and residential pesticide use, increases in pathogens, changes in weather patterns such as increases in extreme weather events, and pollution, among others (Winfree et al., 2009; Potts et al., 2010; Cameron et al., 2011; Goulson et al., 2015). Agricultural pesticides, in particular, are a major stressor on pollinator populations and pollinators can be exposed to pesticides directly, through exposure during spraying or contact with contaminated planting dust, or indirectly, via exposure to residues in pollen, translocation through plants, and drinking water contaminated with pesticide runoff (van der Sluijs et al., 2013; Sanchez-Bayo and Goka, 2014). Farmers who perceive multiple stressors and multiple pesticide exposure pathways as important may be more likely to engage in pollinator supporting practices. To assess farmers views about factors potentially impacting pollinator health and potential pesticide exposure pathways, farmers were asked to rank the importance of 10 factors contributing to pollinator health and eight factors contributing to pesticide exposure (Supplementary Tables 1, 2). Responses were recorded by the farmer on a Likert scale, with scores ranging from 1 to 5 (1 = Not at all important; 2 = Slightly important; 3 = Somewhat important; 4 = Very important; 5 = Extremely important).
Farmer classifications can be useful for targeting those farmers most likely to adopt new technologies or participate in conservation programs (Daloglu et al., 2014). We classified farmers by their responses to the aforementioned perception questions using a two-step process, a principal component analysis (PCA) followed by a cluster analysis. To begin, we examined correlations between our variables (i.e., question responses) using the generic cor function in R (R Core Team, 2020). No strong correlations were observed. We then used Bartlett's test to inspect the correlation matrix using the cortest.barlett function in the psych package (Revelle, 2020). Barlett's test was highly significant (χ2 = 959.06, p < 0.00001, df = 153) indicating that PCA was appropriate for our dataset. We also evaluated the determinant of the correlation matrix, which did not indicate that PCA would be a problematic approach (Field et al., 2012). PCA was then conducted on the variables using the principal function in the psych package (Revelle, 2020). Scree plots of eigenvalues were visually examined to find the point of inflection. This approach suggested a three-component solution. We then reran the PCA with the three-component solution with varimax rotation and examined the component loading matrix using the print.psych function in the psych package (Revelle, 2020). Variables with component loadings above 0.6 were retained for the cluster analysis (Balukas et al., 2019).
To classify farmers, we then used the 14 variables retained from the PCA in a k-means cluster analysis. We used the generic kmeans function in R and varied the number of cluster solutions (R Core Team, 2020). A three-cluster solution was found to maximize within-cluster homogeneity. We used the generic kruskal.test and pairwise.wilcox.test functions in R to conduct a Kruskal-Wallis rank sum test followed by pairwise comparisons using Wilcoxon rank sum tests with continuity correction to confirm that input variable values differed between the farmer clusters (R Core Team, 2020). We also used this series of tests (Kruskal-Wallis rank sum followed by Wilcoxon rank sum tests) to evaluate a subset of input variables that were not retained by the cluster analysis (see Results for variables tested). We used descriptive statistics of the perception variables for each cluster to better understand the heterogeneity of views of pollinator health and pesticide exposure risks among the different farmer classes and additional descriptive statistics on farm and farmer characteristics to better understand the common attributes of farmers in each class (Balukas et al., 2019).
Pest and Pollination Management Strategies
To evaluate the impact farm and farmer characteristics have on the adoption of strategies that farmers can use to manage pests and pollinators, we quantified 12 agricultural production practices using survey data. To determine the frequency of pesticide use, farmers were asked to record how many applications of insecticides, herbicides, and fungicides they applied per year, on average, over the last 5 years to their cucurbit fields. We also totaled across pesticide groupings to evaluate overall pesticide use. To quantify the use of IPM, farmers were asked if they engaged in 20 different IPM practices within the past 5 years. Practices were grouped in one of four categories (prevention, avoidance, monitoring, and suppression) and multiple selections from each category were allowed. For example, prevention IPM practices included: cultivated for pest control, removed or burned crop residues, cleaned equipment after field work, and chopped, sprayed, mowed, plowed or burned field edges, ditches or fence lines. Selected practices were totaled for each category (e.g., across the four options for prevention) and summed across all 20 practices. Lastly, to quantify pollination management practices, we asked farmers if they used managed bees to pollinate their crops (Yes or No) or installed pollinator habitat on their farm (Yes or No). We also asked how likely they were to participate in a pollinator habitat conservation incentive program (e.g., cost share programs), administered at five levels (federal, state, county, regional, non-governmental). This question was implemented using a Likert scale, and the values reported for each administration level were summed. Additional details on the survey questions used to quantify these 12 agricultural practices can be found in the Supplementary Table 3.
Farmer Demographics and Farm Characteristics
Farmers were asked a series of questions that characterized their farming system and demographics. To characterize their farming systems, we asked for the number of field and pollinator dependent crops grown within the previous 5 years. Farmers could select multiple options, which were given, and allowed room to detail other field crops that they grew. We also asked farmers to estimate the number of acres in cucurbit crops and total acres of cropland, managed in the year of the survey. To assess insect pests, farmers were given a list of common arthropod pest species, from which they could select multiple options, and also allowed to state other pests that they manage within their farm. To characterize farmers, we asked questions regarding farm ownership, if the farm was the main source of household income, the number of years farming, the length of time in family farming, and farmer age. Additional demographical information was collected (e.g., gender), though, these variables were highly skewed or sparse and could not be included in our statistical analysis (see below). Information sources regarding pest management were collected across nine categories (crop scout, university extension resource, National Resource Conservation Service staff, neighbor or friend, session at a grower meeting, newsletter [paper], email listserv, text message [automatic alert], social networking site [Twitter, Facebook, etc.]) and four response levels (used in past, use now, would use, never have, and never will use) from which farmers could select one option. For our analysis we summed the number of responses for “use now” across the nine categories to determine the number of current information sources for each farmer (e.g., a value of 9 would indicate the farmer used all sources of information). Additional details on the questions used to evaluate farmer and farm characteristics can be found in the Supplementary Table 4; see also Table 1 for summary statistics.
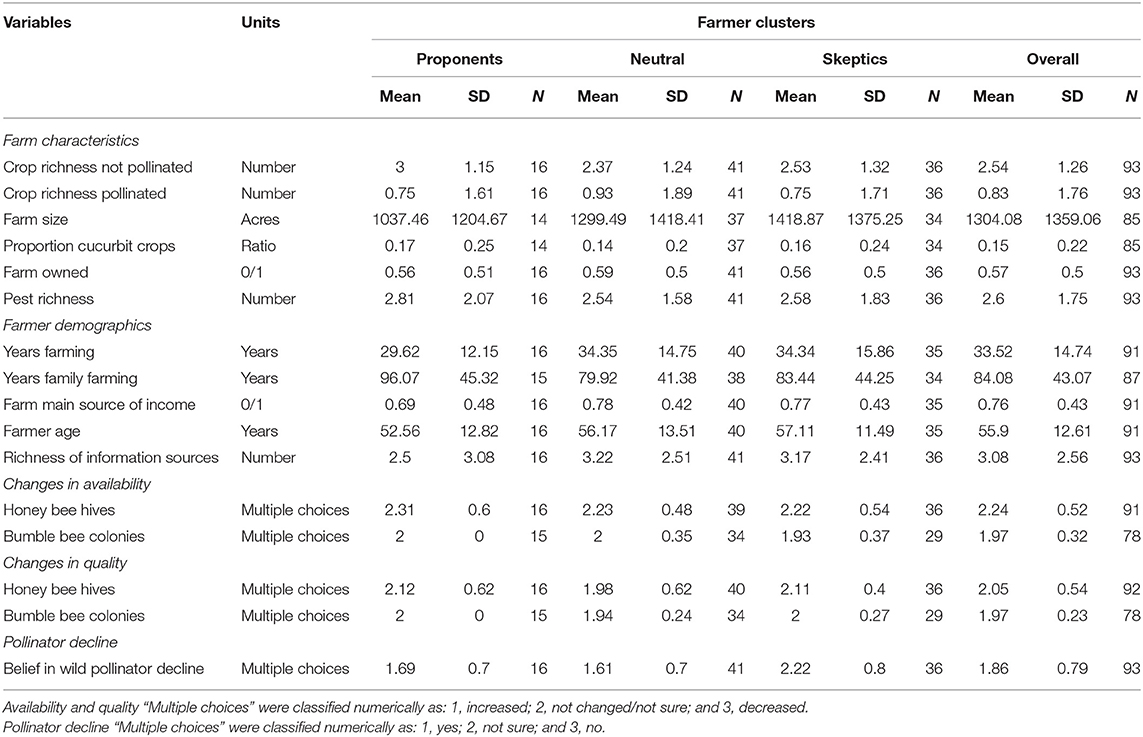
Table 1. Qualitative assessment of farmer classes including their farm characteristics, demographics, perceptions of the changes in quality and availability of managed honey bee hives and bumble bee colonies, and belief in pollinator declines.
Exploratory Statistical Analysis
To evaluate factors influencing pesticide use, IPM, and pollination management, we constructed generalized linear models using the 12 agricultural practices described earlier as dependent variables: sprays per year for insecticides, herbicides, fungicides, and all pesticide sprays summed together; number of IPM practices for prevention, avoidance, monitoring, suppression, and all practices summed together; use of managed bees; creation of pollinator habitat; and future participation in pollinator habitat conservation programs. Each model used the same set of explanatory variables, including the farmer classifications generated by the cluster analysis described earlier, as well as farm and farmer characteristics (Table 1). The assumed error distributions varied by model depending on the response variable (gaussian for continuous variables [e.g., the number of pesticide sprays] or binomial for dichotomous variables [e.g., a Yes or No response]).
We used an exploratory statistical analysis approach starting with a global model constructed for each dependent variable with the same set of explanatory variables:
where β0 is the model intercept, Yi is the estimated value for the dependent variable being modeled (e.g., number of pesticide applications), Xi are the explanatory variables (farm characteristics, farmer demographics, and clusters), βi are the partial regression coefficients for each explanatory variable, and εi is the error term which varied by the dependent variable being modeled (see above) (Zuur et al., 2009). The global model was constructed with the generic glm function in R (R Core Team, 2020). We used the dredge function in the MuMIn package to find model permutations (4,096 models for each dependent variable) (Bartón, 2020). Thus, dredge was used to generate models using all additive combinations of the explanatory variables from the global model, including a null model (intercept only). We then used the get.models function to extract a list of fitted model objects which was passed to the model.avg function to find estimates for all parameters (Bartón, 2020). In other terms, we used a model averaging approach (multi model inference) where parameter estimates were averaged across competing models to generate estimates for all explanatory variables. Therefore, we drew inferences from multiple models, rather than conducting comparisons of models. This approach was exploratory because it was not hypothesis driven. That is, we were interested in evaluating the relationship between all explanatory and response variables. By using model averaging, we found estimates for all explanatory variables and avoided uncertainty common to information theoretic (model selection) approaches (Burnham and Anderson, 2002). We considered explanatory variables important when the model averaged p-value estimates were below an α = 0.05. All statistical analyses were conducted in R 4.0.2 (R Core Team, 2020).
Results
Farmers who responded to our survey managed on average 2.6 pest species and cucurbits were grown on ~15% of their land. Approximately 60% of farmers owned their land, with the average area of production >1,300 acres. Farmers grew a variety of crops, though on average farmers grew more crops that were not pollinator dependent and ~90% of farmers grew at least one crop that did not depend on pollinators (Table 1). The average farmer age was 56 years old with 34 years of farming experience, and ~76% of respondents farmed as their main source of income (Table 1).
Farmer Views on Pollinator Health
Farmers, on average, perceived no change or were not sure regarding the availability and quality of honey bee hives and bumble bee colonies (Table 1). The result regarding managed bumble bee colonies is not surprising as only six survey respondents actually buy bumble bees for pollination and the remaining respondents would likely be unsure. On average, farmers also tended to be not sure about whether pollinators were in decline (Table 1). However, as described below, there are differences among some farmer classes regarding pollinator status.
The principal component analysis identified a three-component solution which we further ascribed as variables associated with: (1) pesticide exposure, (2) bee diet/diseases and habitat loss, and (3) changes in weather (Figure 2; Table 2; Supplementary Tables 1, 2). Three observations are worth noting. First, some variables—most notably, the pollinator status (i.e., wild pollinator declines, changes in availability, and quality of managed bees) and the agricultural and residential pesticide use variables—were dropped from the cluster analysis by the PCA process either because they didn't contribute significantly to the first three principal components or because of missing data. However, as described below, some of these variables differ among the farmer classes. Second, while the survey presented the pollinator health and pesticide exposure ranking questions in two groups (Supplementary Tables 1, 2), the questions related to weather changes resulted in their own principal component indicating different views regarding weather vs. other potentially more controllable factors. Third, even though the question regarding pesticide residues remaining in the soil after a previous crop rotation was grouped with the set of questions on pollinator health (see Supplementary Table 1), the PCA included that question in the component with the group of questions related to pesticide exposure routes (Table 2). In hindsight, that grouping makes more sense than the original survey design and survey respondents' views confirmed this.
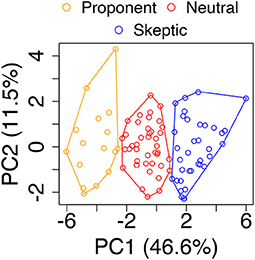
Figure 2. Cluster analysis identified three classes of farmers according to how they rated statements (on a 1–5 Likert scale) regarding factors impacting pollinator health and routes of pesticide exposure on their farms (Table 2). Principal component analysis identified a three-component solution with the first two components given here. Percentage of variance explained are given for each component.
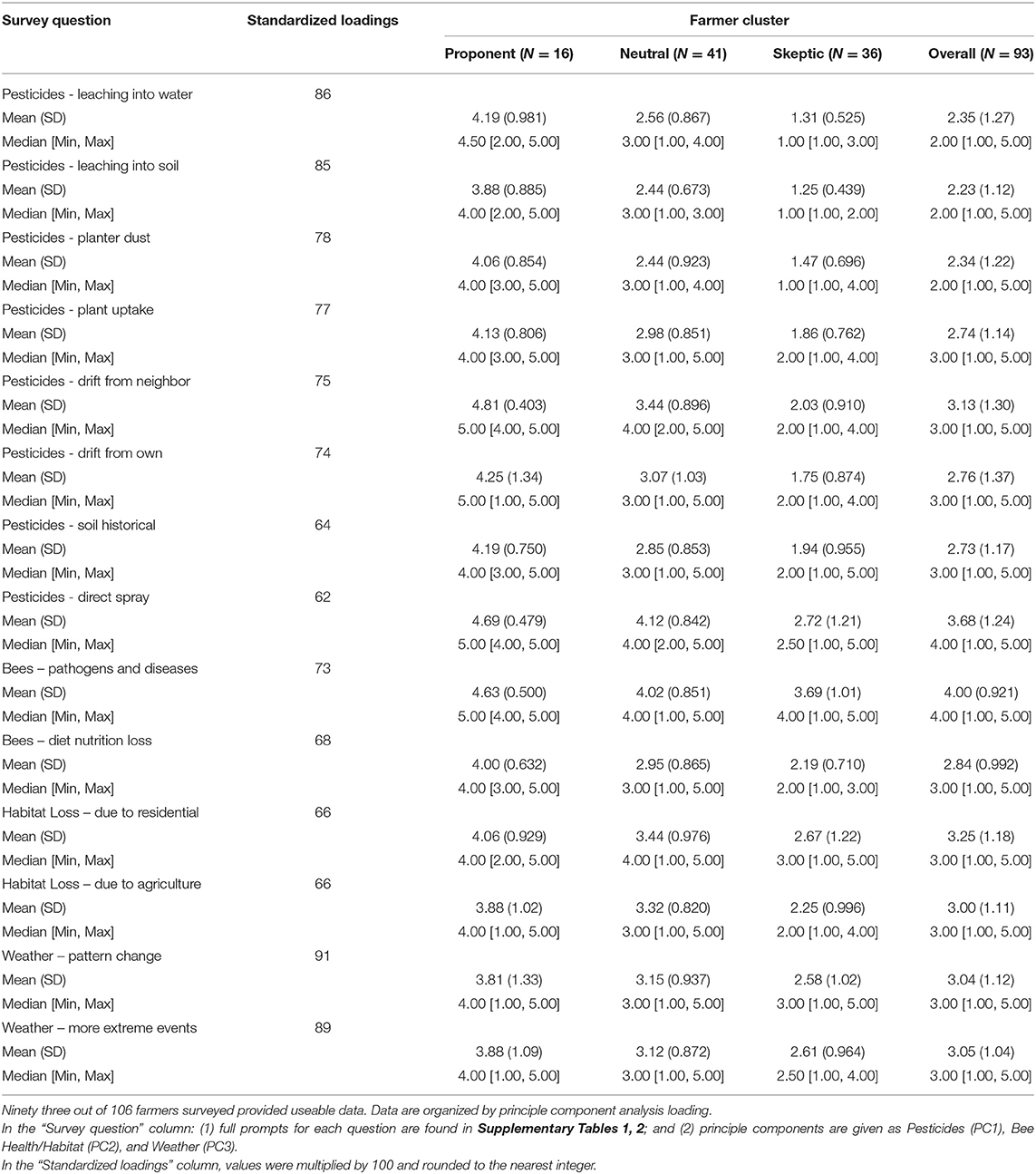
Table 2. Farmer classes based on views of factors contributing to declines in pollinator health and the routes of pesticide exposure for pollinators on their farms.
Using cluster analysis, we identified three classes of farmers according to how they rated the importance of the factors potentially impacting pollinator health including potential routes of pesticide exposure on their farms (Figure 2; Table 2). We found that farmer classes represented a spectrum of the Likert scale. Farmers classified as “proponents” typically responded with Likert scores ranging from 3 to 5 (n = 16), farmers classified as “neutral” occupied the middle of the scale with scores ranging from 2 to 4 (n = 41), and farmers classified as “skeptics” responded with Likert scale scores ranging from 1 to 3 (n = 36) (Figure 2; Table 2). Via Kruskal-Wallis rank sum test (χ2 = 423.79, p < 0.001, df = 2) and pairwise comparisons of the Wilcoxon rank sum test (neutral-proponent: p < 0.001; neutral-skeptic: p < 0.001; proponent-skeptic: p < 0.001) we confirmed that scores for the statements regarding the factors impacting pollinator health and routes of pesticide exposure differed between the three farmer classes, as qualitatively described above (Supplementary Figure 1).
Our additional tests on the subset of variables dropped in the cluster analysis (see above) suggested skeptics were less likely to think wild pollinator populations were declining than the proponent and neutral classes (Kruskal-Wallis rank sum test [χ2 = 12.04, p = 0.00243, df = 2], Wilcoxon rank sum test [neutral-proponent: p = 0.67; neutral-skeptic: p = 0.0029; proponent-skeptic: p = 0.039]). That is, skeptics were less likely to perceive pollinator populations as threatened (Supplementary Figure 2). Skeptics were also less likely to rate agricultural and residential pesticide use as a very or extremely important factor impacting pollinator health than the proponent and neutral classes (agricultural: Kruskal-Wallis rank sum test [χ2 = 20.12, p < 0.001, df = 2], Wilcoxon rank sum test [neutral-proponent: p = 0.26; neutral-skeptic: p < 0.001; proponent-skeptic: p < 0.001]; residential: Kruskal-Wallis rank sum test [χ2 = 22.76, p < 0.001, df = 2], Wilcoxon rank sum test [neutral-proponent: p = 0.009; neutral-skeptic: p = 0.0045; proponent-skeptic: p < 0.001]), despite there being substantial evidence of the impacts of these pesticides on pollinators (Supplementary Table 1; Supplementary Figures 3, 4).
Regarding the variables retained in the cluster analysis, proponents were more likely to rate (on a 1–5 Likert scale) factors impacting pollinator health as extremely important or very important (means from 3.81 to 4.63), while skeptics were more likely to rate these factors as slightly or not important (means from 2.19 to 3.69) (Table 2). On average, neutral farmers rated pollinator health factors as somewhat important (means from 2.95 to 4.02). Despite the differences in overall ratings, all three farmer classes ranked “the spread of pathogens and parasites harmful to bees” the most important factor for pollinator health (means of 4.6, 4.0, and 3.7 for the proponent, neutral, and skeptic classes, respectively; Table 2).
Proponents were also more likely to rate the different routes of pollinator exposure to pesticides as extremely important or very important (means from 3.88 to 4.81), while skeptics were more likely to rate the different routes of exposure as slightly or not important (means from 1.25 to 2.72) (Table 2). Neutral farmers on average rated the different pesticide exposure routes as somewhat important (means from 2.44 to 4.12; Table 2). Of the possible routes, proponents on average ranked “pesticide drift from neighboring fields” as the most important contributor to pollinator pesticide exposure (mean of 4.81; Table 2). In comparison, skeptics and neutral farmers on average ranked “direct spraying” as the most important contributor of pollinator pesticide exposure (means of 2.72 and 4.12 for the skeptic and neutral classes, respectively; Table 2).
Qualitatively, the three farmer classes were similar in their demographics and farm characteristics (Table 1). However, compared to the neutrals and skeptics, proponents were younger (mean of 52.6 years old), had fewer years farming (mean of 29.6 years) but more years in family farming (mean of 96.1 years), and had sources of income outside the farming system (31% had off-farm income) (Table 1). Proponents also reported smaller farms (mean farm size of 1037.5 acres), higher pest richness (mean of 3.0 pests), a greater proportion of their farm in cucurbits (17% of land in cucurbit production), and greater richness of crops that were not pollinator dependent (mean of 3.0 crops) (Table 1).
Pest and Pollination Management Strategies
Pest management was mediated by the physical environment and economic conditions of the farming system (Figures 3, 4; Tables 3, 4). The use of all pesticides summed together, and insecticides, herbicides, and fungicides individually, were positively correlated with the pest richness of the farming system (all pesticides: estimate = 1.4, SE = 0.23, z-value = 5.9, p-value <0.001; insecticides: estimate = 0.51, SE = 0.098, z-value = 5.1, p-value <0.001; herbicides: estimate = 0.12, SE = 0.053, z-value = 2.2, p-value = 0.026; fungicides: estimate = 0.79, SE = 0.17, z-value = 4.7, p-value <0.001; Table 3; Figures 3A,C–E). All pesticide use, and particularly, fungicide use, was higher when farming was the main source of household income (all pesticides: estimate = 2.5, SE = 0.94, z-value = 2.7, p-value = 0.0079; fungicides: estimate = 2.1, SE = 0.67, z-value = 3.00, p-value = 0.0026) (Figures 3B,F). No explanatory variables predicted individual IPM practice categories (e.g., prevention) (Table 4). However, when selections for all 20 IPM practices were summed, there was a positive correlation with pest richness (estimate = 0.75, SE = 0.23, z-value = 3.2, p-value = 0.0015) and farm size (estimate = 0.00071, SE = 0.00033, z-value = 2.2, p-value = 0.031) (Figures 4A,B; Table 4).
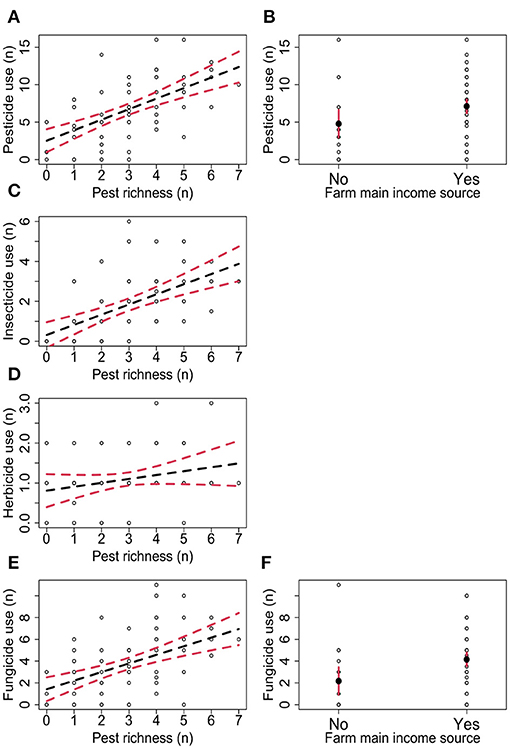
Figure 3. Model averaged predictions plotted with raw survey data for variables mediating the use of (A,B) all pesticides, (C) insecticides, (D) herbicides, and (E,F) fungicides. (A) Pesticide use was positively related to pest richness, particularly for (C) insecticides and (E) fungicides. (D) A weak positive relationship was observed between herbicide use and pest richness. Farming as the main source of income was positively related to (B) overall pesticide use, and (F) the use of fungicides.
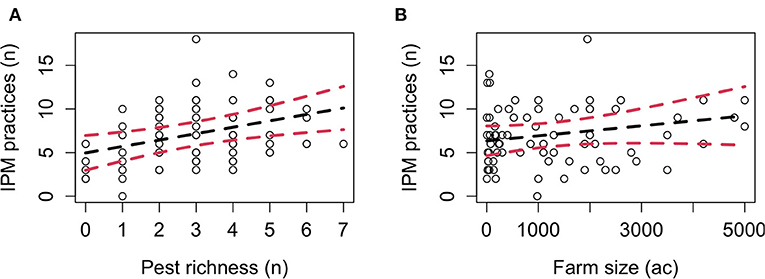
Figure 4. Model averaged predictions plotted with raw survey data for variables mediating the use of IPM practices. (A) The use of IPM was positively related to pest richness. (B) A weak positive relationship was also observed between IPM practices and farm size.
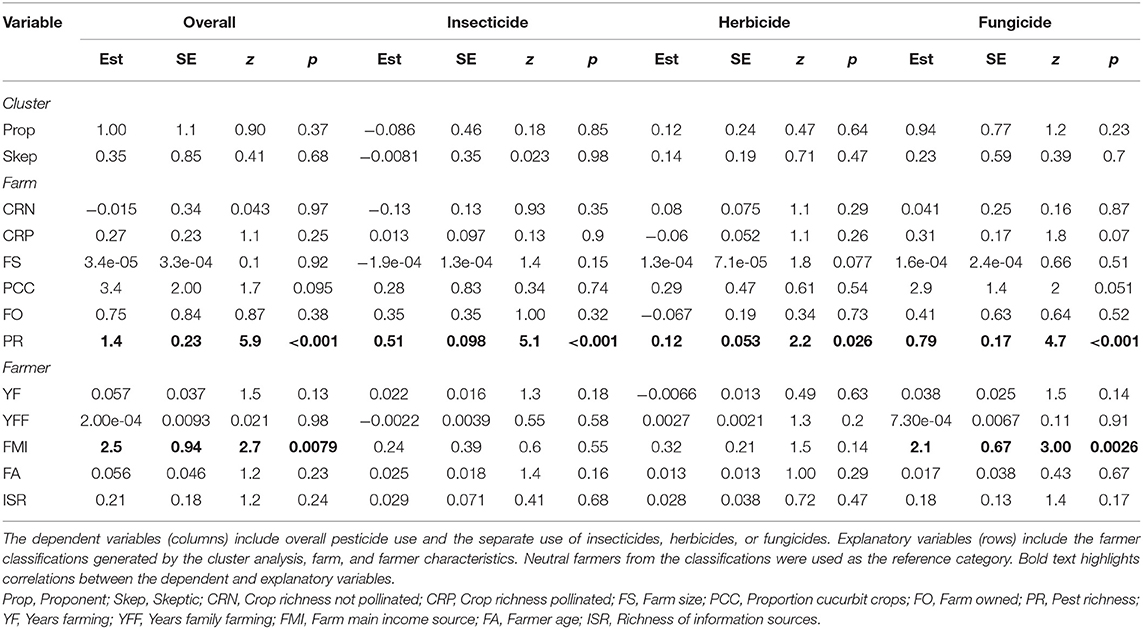
Table 3. Model averaged parameter estimates, standard errors, z-, and p-values for the relationship between pesticide use and explanatory variables (rows).
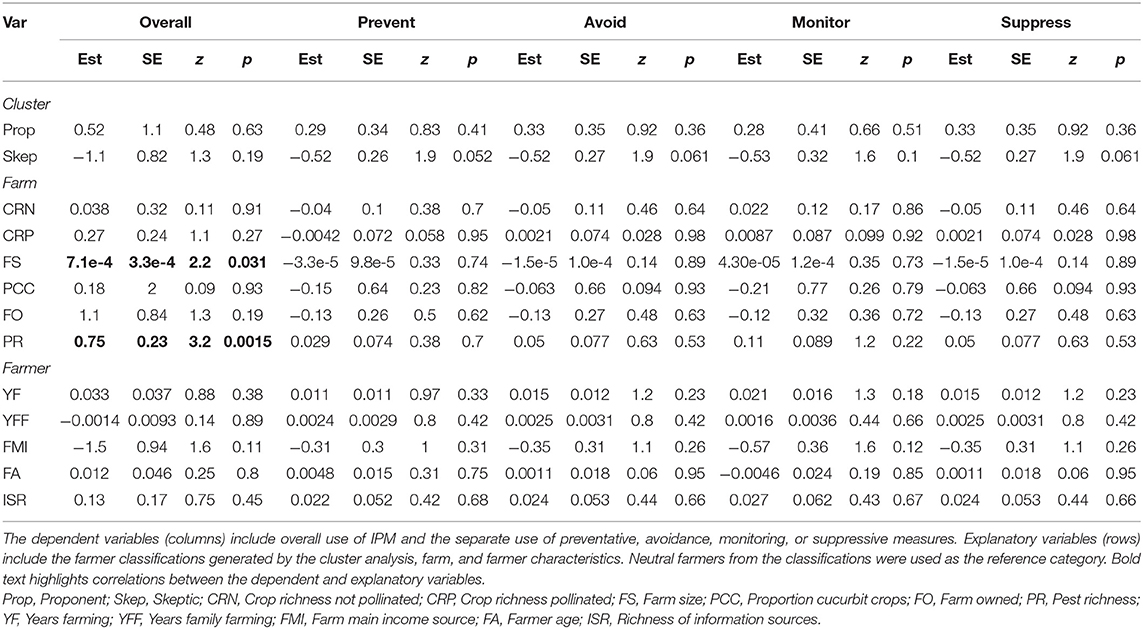
Table 4. Model averaged parameter estimates, standard errors, z-, and p-values for the relationship between IPM and explantory variables (rows).
The use of managed bee colonies was positively associated with farming as the main source of household income (estimate = 1.90, SE = 0.77, z-value = 2.40, p-value = 0.017) (Figure 5A; Table 5). The creation of pollinator habitat was positively related to the richness of pollinator-dependent crops grown by the farmer (estimate = 0.39, SE = 0.19, z-value = 2.00, p-value = 0.044) and negatively associated with farming as the main source of household income (estimate = −1.80, SE = 0.73, z-value = 2.40, p-value = 0.017) (Figures 5B,C; Table 5).
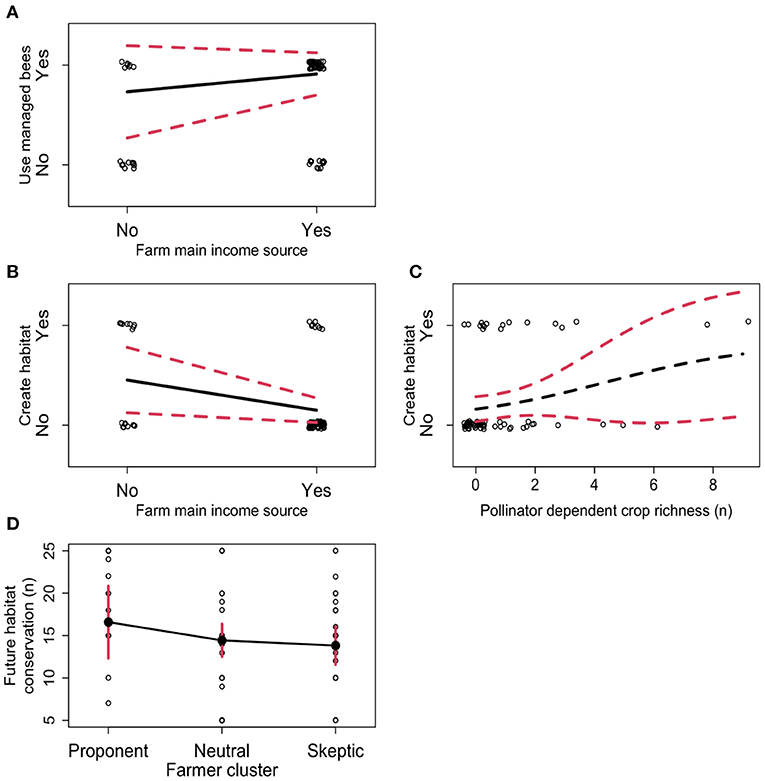
Figure 5. Model averaged predictions plotted with raw survey data for variables mediating (A) the use of managed bees, (B,C) the creation of pollinator habitat, and (D) future participation in pollinator habitat conservation programs. (A) The use of managed bees increased when farming was the main household income source. (B) The creation of pollinator habitat decreased when farming was the main household income source and (C) was positively correlated with the richness of pollinator-dependent crops. (D) Future participation in programs to create pollinator habitat had a weak positive relationship with farmers who viewed factors influencing pollinator health and pesticide exposure as very important (the proponent farmer class).
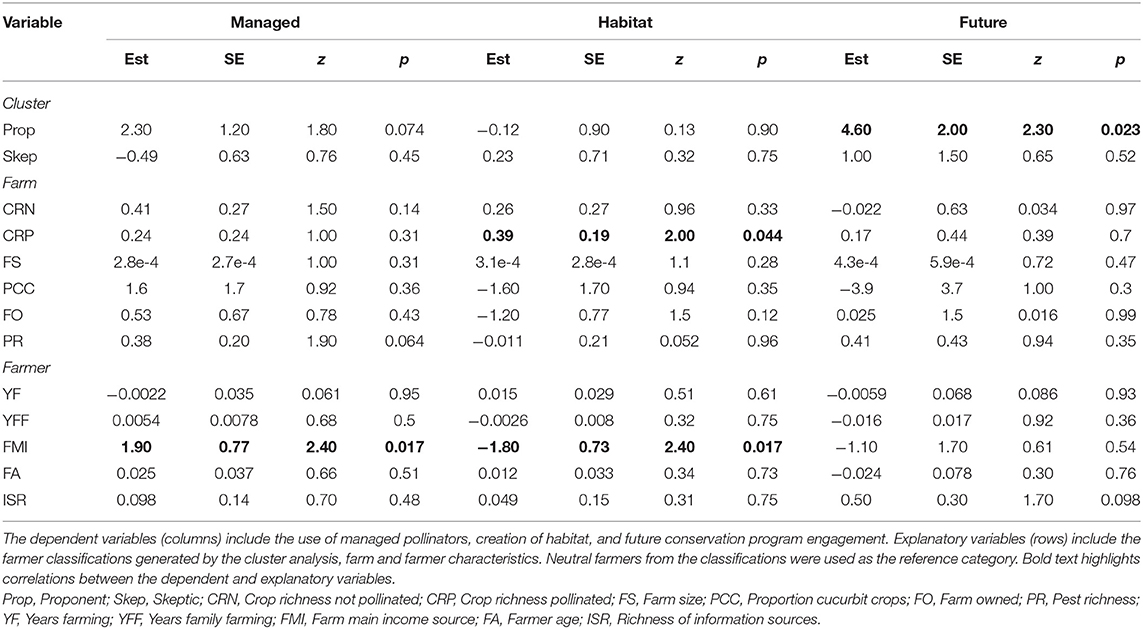
Table 5. Model averaged parameter estimates, standard errors, z- and p-values for the relationship between pollinator management strategies and explantory variables (rows).
Farmer classes (proponents, neutrals, and skeptics; Table 2) were not related to pesticide use, IPM, the use of managed bees, or the installation of pollinator habitat. However, proponents were more likely than neutrals and skeptics to indicate future participation in pollinator habitat conservation incentive programs (e.g., cost-share programs) when Likert scores were summed across all administrative levels (federal; state; county; regional; non-governmental) (proponent: estimate = 4.60, SE = 2.00, z-value = 2.30, p-value = 0.023) (Figure 5D; Table 5).
Discussion
Industrialized farming is a leading cause of insect decline, indicating the need to increase the use of farming practices that support pollinators (Kleijn et al., 2018; Wagner et al., 2021). Indeed, while ample public enthusiasm for pollinators exists, there remains a need to promote knowledge transfer on, and adoption of, pollinator supportive farming practices (Maderson and Wynne-Jones, 2016; Breeze et al., 2019; Hall and Steiner, 2019; Hall and Martins, 2020). We expected that farmers who thought pollinator populations were in decline and who perceive multiple stressors and multiple pesticide exposure pathways as very important would be more likely to implement on-farm practices that promote pollinators (e.g., create pollinator habitat, use IPM, and/or reduce pesticide use). However, we did not find evidence to support this expectation. Rather, we found that pest and pollinator management were related to on-farm environmental and economic conditions.
Farmer classifications are commonly used to explain adoption of technology or conservation practices (e.g., Pouta et al., 2011; Daloglu et al., 2014). We identified three distinct classes of farmers based on their views regarding pollinator decline, the importance of factors impacting pollinator health in general, and the importance of different routes of exposure to pesticides on their farm. “Proponents” were more likely to rate pollinator health and pesticide exposure as extremely important or very important, while “neutrals” were more likely to rate these items as somewhat important and “skeptics” were more likely to rate these items as slightly important or not important. Despite the different ratings (i.e., degree of importance), the three classes of farmers agreed on which factors were most and least important. All three farmer classes ranked “the spread of pathogens and parasites harmful to bees” the most important factor impacting pollinator health and ranked “pesticide drift from neighboring fields” and “direct spraying” the two most important contributors of pollinator exposure to pesticides on their farm, which may indicate widespread availability and acceptance of information on these items. The three farmer classes also ranked “pesticides leaching into soil” the least important contributor to pesticide exposure, potentially indicating a need for further education on this route of pesticide exposure particularly in agricultural landscapes where ground-nesting pollinators are important. This result could further indicate that relatively new advances regarding the consequence of soil-borne pesticides for pollinators has not yet been communicated to farmers (Anderson and Harmon-Threatt, 2019; Chan et al., 2019; Main et al., 2020). While none of the farmer classes explained pesticide or pollination management strategies, our findings show that farmers identified as “proponents” were more likely to indicate a willingness to participate in future habitat restoration programs.
Farmer perceptions of insect pest populations mediates pesticide use on farms (Obopile et al., 2008). However, damage caused by pest populations may be overestimated by farmers, suggesting that the use of pesticides on farms could be reduced, yielding benefits for pollinators (Yang et al., 2005). Our findings suggest that pesticide use, including fungicides, herbicides, and insecticides, increased with the richness of pest communities perceived by farmers. We suggest that insecticide use may increase in response to pest richness due to a need to manage an array of insect species, though, reverse causality is also possible. Moreover, these response variables (insecticide, fungicide, and herbicide use) are likely correlated, and it is unclear why pest richness is related to fungicide use, other than indicating that farmers who use more insecticides may also be more likely to use other pesticides (fungicides and herbicides). Broadly, though, there is a need to evaluate the relationship between farmer's perceptions of pest pressures and pesticide use. Pesticides are often overused on farms and wild and managed pollinator populations will likely benefit if these pest management interventions can be relaxed (Egan et al., 2020; Ternest et al., 2020).
Integrated pest management increased with both pest richness and farm size. Similar to our results regarding pesticide use, we expect that IPM increases with pest richness due to the need for additional tactics to manage a diversity of pest insect species. The use of IPM can benefit pollinators on farms (Egan et al., 2020). However, our findings suggest that farms using more IPM tactics also increased pesticide use, suggesting the benefits of IPM could be limited by this collinearity. This result further underscores the need to develop integrated pest and pollinator management (IPPM) programs that quantify the balance between pesticide use and IPM tactics to promote profits and yields in agroecosystems (Biddinger and Rajotte, 2015). Farmers who manage more land are also known to use higher levels of inputs for insect control and IPM (Blake et al., 2006). As proposed by Moser et al. (2008), the positive relationship in our data between farm size and the use of IPM may be due to increased access to financial capital on larger farms, which is necessary for implementing practices related to sustainability in agriculture. Indeed, the method of pest control (balance between IPM and pesticide use) has links to greater financial flexibility which is common to larger farms (Zhang et al., 2018). Therefore, we suggest larger farms may indirectly promote pollinator populations, not only through their size (e.g., species-area relationships) but also through flexibility in the practices they use, including the implementation of IPM.
Global crop pollination occurs through a combination of wild and managed (e.g., commercial) pollinators, although the contribution of each type of pollinator varies by crop type and the surrounding agroecosystem (Reilly et al., 2020). For example, a recent study showed almonds (Prunus dulcis) being pollinated almost exclusively by managed honey bees, while pumpkins (Curcurbita pepo) were mostly pollinated by wild bees (Reilly et al., 2020). In agroecosystems containing natural lands, wild pollinators may be sufficient to fully meet pollination needs (Petersen et al., 2013). However, even in landscapes with abundant wild pollinators, farmers may choose to lease managed bees as a form of insurance or risk avoidance (Park et al., 2020). In other cases, where crop pollination is dominated by managed bees, creating pollinator habitat for wild bees is another form of insurance (Hanes et al., 2013). Thus, the choice among pollination strategies will depend upon farm, farmer, and landscape characteristics. We found that farmers whose main source of income comes from farming were more likely to use managed bees, a purchased input. This same group of farmers was also more likely to use pesticides, another set of purchased inputs. Because these farmers are more reliant on farm income, it is possible that they are engaging in pollination and pest control risk aversion strategies (although we did not specifically ask this question).
The creation of pollinator habitat on farms has proven benefits for increasing wild bee biodiversity and managed pollinator health (Nicholson et al., 2020). Less clear, however, is the benefit of these habitats for increasing pollination and, more importantly, yields in adjacent crops, though these benefits may accrue over time (Blaauw and Isaacs, 2014; Egan et al., 2020; Nicholson et al., 2020). Our findings show that farmers whose main source of income comes from farming are less likely to create pollinator habitat on their farm. This may be due to the uncertainty regarding links between habitat and actual yields, which coincides with our risk aversion scenario described above. In contrast, farmers who grow a more diverse set of pollinator-dependent crops were more likely to create pollinator habitat. This suggests that at least some farmers value links between pollinator habitat and pollination services, which confirms the results of Nalepa et al. (2020) who found a positive relationship between the use of pollinator-supporting practices and farmer awareness of wild bees, perceptions of threats to wild bees, and perceived benefits from wild bees. In contrast, Park et al. (2020) found that 20% of apple growers did not understand the relationship between wild pollinators and pollination services. It is also possible that some farmers plant pollinator habitat as part of a larger conservation effort (Gaines-Day and Gratton, 2017). Although profit is important for all farmers, many farmers also note the importance of stewardship and conservation motivations (Chouinard et al., 2008; Timmons, 2014). Farmers' decisions to adopt a new conservation approach is influenced by farmer and farm characteristics as well as the opportunities to learn about new practices (e.g., Karali et al., 2014; Wauters and Mathijs, 2014). Influential factors include access to and quality of information, financial capacity, environmental attitudes, and connections to other stakeholders including agency personnel, extension staff, other farmers, or watershed groups (Baumgart-Getz et al., 2012; Reimer et al., 2012). While we do not know why cucurbit farmers in our study created pollinator habitat (e.g., to increase pollination to adjacent crops or as part of a larger conservation effort), it would be useful to further study these results to better understand the motivations behind the creation of pollinator habitat on farms.
A number of factors we included in our modeling approach did not relate to the use of pollinator conservation practices (e.g., reduced pesticide use, IPM, or pollinator management). Of those, farm ownership is known to mediate the use of conservation practices (e.g., IPM) (Ulrich-Schad et al., 2016; Ranjan et al., 2019; Nalepa et al., 2020). Approximately twice as many participants in our study owned, versus rented, land. Agricultural land rental is common in the US, indicating that our sample may be biased toward farm owners. Nevertheless, due to the extent of rented land, this factor will likely influence outcomes for pollinator conservation on farms and should receive additional consideration in future studies (Ulrich-Schad et al., 2016; Ranjan et al., 2019). For example, owners can include pollinator conservation provisions in their leases. Another potential limitation of our study is related to farm size. Statistically, the farms in our sample were larger than the national average. Most cucurbit farms have <15 acres in production, whereas the average area in cucurbit production in our sample was ~200 acres. Again, this may indicate that small farms were less likely to respond to our survey and could bias our findings. Indeed, the pest and pollinator management practices used on small farms often vary considerably from larger operations (Grasswitz, 2019; Headrick, 2021) and analyzing these differences may yield insights for pollinator conservation. However, because large farms control >50% of land in cucurbit production, our study highlights the views and practices of farmers that control the greatest proportion of land and thus could have the most impact on pollinator populations via engaging the fewest number of stakeholders (USDA NASS, 2017).
Understanding the barriers to farmer adoption of pollinator-supporting practices is important for sustaining agroecosystems and long-term food security. Overall, we found that pest and pollinator management were not related to farmers views on the importance of factors impacting pollinator health or routes of pesticide exposure. Indeed, we found that some farmers were neutral and skeptical regarding these factors, indicating a potential need for additional outreach and education (Bhattacharyya et al., 2017; Hevia et al., 2020). However, even if all farmers become “proponents” this does not mean they will take action to prevent threats to pollinators. For example, we found that only future participation in conservation programs was related to farmers we identified as proponents, but not their current actions. We are not the first to find a potential disconnect between knowledge and pollinator conservation action (Hoshide et al., 2018). Indeed, Breeze et al. (2019) found that ~50% of farmers believed they had pollination services deficits, and most used agri-environment schemes, though few used these schemes to promote pollinators, further highlighting how perceptions of pollinators may not spur pollinator-supportive actions by farmers. Nevertheless, it is important to recognize that there are multiple levels of knowledge, one recognizing the importance of pollinators and others understanding the complex relationship between pollinator habitat and ultimately crop pollination. Both Nalepa et al. (2020) and Hevia et al. (2020) found that farmers with higher awareness of pollinator contributions to crop pollination and greater understanding of the threats to pollinators were more likely to adopt pollinator-supporting practices, but that not all farmers possessed this depth of knowledge. Thus, there remains a need to educate farmers on pollinator declines, including the factors impacting pollinator health and the multiple routes of pesticide exposure, and connect this knowledge with the practices used within farming systems (Gaines-Day and Gratton, 2017). However, it is unclear whether the lower importance ratings of our skeptics are indicative of a lack of knowledge or a true assessment of their values. Skeptics were less likely to think wild pollinator populations were declining and were less likely to rate agricultural pesticide use as a very important contributor to impacts on pollinator health. This may be an accurate reflection of their actual on-farm experiences. That is, there may be farm specific differences that account for different views on importance (geographic heterogeneity in the beliefs of farmers). We were able to identify farm and farmer characteristics (e.g., crop richness, farm main income) related to pesticide use and IPPM practices, indicating a greater complexity of factors driving farmer decision making. That is, farmers can recognize the importance of pollinators but not be capable of translating reductions in pesticide use or increases in pollinator habitat to increases in yields and profits. Thus, there is a need for further research on understanding the integrated biophysical and socioeconomic relationships among pesticides, pollinators, and crop yields. Researchers will need to work in interdisciplinary teams and account for the heterogeneity of perceptions, perhaps using site specific extensions approaches, to further untangle these variables and support pollinator populations in the future.
Data Availability Statement
Data available via the Figshare Digital Repository doi: 10.6084/m9.figshare.14980104.v1 (Bloom et al., 2021).
Ethics Statement
The studies involving human participants were reviewed and approved by Clark University IRB. Written informed consent was obtained from focus group participants. The mail survey did not require written consent as it was implemented in an anonymous and voluntary manner. This is in accordance with the national legislation and the institutional requirements.
Author Contributions
DB and AK conceived the farmer survey. EB led manuscript preparation and statistical analysis with feedback from DB. EB, DB, IK, and ZS edited the manuscript. All authors contributed to the article and approved the submitted version.
Funding
This project was funded by USDA NIFA grant numbers 2016-51181-25410 and 2017-68004-26323.
Conflict of Interest
The authors declare that the research was conducted in the absence of any commercial or financial relationships that could be construed as a potential conflict of interest.
Publisher's Note
All claims expressed in this article are solely those of the authors and do not necessarily represent those of their affiliated organizations, or those of the publisher, the editors and the reviewers. Any product that may be evaluated in this article, or claim that may be made by its manufacturer, is not guaranteed or endorsed by the publisher.
Acknowledgments
We would like to thank the farmers who participated in this study.
Supplementary Material
The Supplementary Material for this article can be found online at: https://www.frontiersin.org/articles/10.3389/fsufs.2021.672981/full#supplementary-material
References
Anderson, N. L., and Harmon-Threatt, A. N. (2019). Chronic contact with realistic soil concentrations of imidacloprid affects the mass, immature development speed, and adult longevity of solitary bees. Sci. Rep. 9:3724. doi: 10.1038/s41598-019-40031-9
Balukas, J. A., Bell, K. P., and Bauer, D. M. (2019). Classifying private landowners to improve understanding of management decisions and conservation opportunities in urbanizing forested landscapes. J. Environ. Manage. 232, 751–758. doi: 10.1016/j.jenvman.2018.11.128
Bartón, K. (2020). MuMIn: Multi-model inference. R package version 1.43.17. Available online at: https://CRAN.R-project.org/package=MuMIn (accessed July 6, 2021).
Baumgart-Getz, A., Prokopy, L. S., and Floress, K. (2012). Why farmers adopt best management practices in the United States: a meta-analysis of the adoption literature. J. Environ. Manage. 96, 17–25. doi: 10.1016/j.jenvman.2011.10.006
Bhattacharyya, M., Acharya, S. K. R., and Chakraborty, S. K. (2017). Pollinators unknown: people's perception of native bees in an agrarian district of West Bengal, India, and its implication in conservation. Trop. Conserv. Sci. 10, 1–14. doi: 10.1177/1940082917725440
Biddinger, D. J., and Rajotte, E. G. (2015). Integrated pest and pollinator management—adding a new dimension to an accepted paradigm. Curr. Opin. Insect Sci. 10, 204–209. doi: 10.1016/j.cois.2015.05.012
Blaauw, B. R., and Isaacs, R. (2014). Flower plantings increase wild bee abundance and the pollination services provided to a pollination-dependent crop. J. Appl. Ecol. 51, 890–898. doi: 10.1111/1365-2664.12257
Blake, G., Sandler, H. A., Coli, W., Pober, D. M., and Coggins, C. (2006). An assessment of grower perceptions and factors influencing adoption of IPM in commercial cranberry production. Renew. Agric. Food Syst. 22, 134–144. doi: 10.1017/S1742170507001664
Bloom, E., Bauer, D. M., Kaminski, A., Kaplan, I., and Szendrei, Z. (2021). Data associated with manuscript. figshare. Dataset. doi: 10.6084/m9.figshare.14980104.v1
Breeze, T. D., Boreux, V., Cole, L., Dicks, L., Klein, A.-M., et al. (2019). Linking farmer and beekeeper preferences with ecological knowledge to improve crop pollination. People Nature 1, 562–572. doi: 10.1002/pan3.10055
Burnham, K. P., and Anderson, D. R. (2002). Model Selection and Multimodel Inference: A Practical Information-Theoretic Approach. New York, NY: Springer.
Cameron, S. A., Lozier, J. D., Strange, J. P., Koch, J. B., Cordes, N., Solter, L. F., et al. (2011). Patterns of widespread decline in North American bumble bees. Proc. Natl. Acad. Sci. U. S. A. 108, 662–667. doi: 10.1073/pnas.1014743108
Chan, D. S. W., Prosser, R. S., Rodríguez-Gil, J. L., and Raine, N. E. (2019). Assessment of risk to hoary squash bees (Peponapis pruinosa) and other ground-nesting bees from systemic insecticides in agricultural soil. Sci. Rep. 9:11870. doi: 10.1038/s41598-019-47805-1
Chouinard, H. H., Paterson, T, Wandschneider, P. R., and Ohler, A.M. (2008). Will farmers trade profits for stewardship? Heterogeneous motivations for farm practice selection. Land Econ. 84, 66–82. doi: 10.3368/le.84.1.66
Daloglu, I., Nassauer, J. I., Riolo, R. L., and Scavia, D. (2014). Development of a farmer typology of agricultural conservation behavior in the American Corn Belt. Agric. Syst. 129, 93–102. doi: 10.1016/j.agsy.2014.05.007
Dicks, L. V., Abrahams, A., Atkinson, J., Biesmeijer, J., Bourn, N., Brown, C., et al. (2013). Identifying key knowledge needs for evidence-based conservation of wild insect pollinators: a collaborative cross-sectoral exercise. Insect Conserv. Divers. 6, 435–446. doi: 10.1111/j.1752-4598.2012.00221.x
Dicks, L. V., Viana, B., Bommarco, R., Brosi, B., Arizmendi, M. d. C., Cunningham, S. A., et al. (2016). Ten policies for pollinators. Science 354, 975–976. doi: 10.1126/science.aai9226
Dillman, D. A., Christian, L. M., and Smith, J. D. (2008). Internet, Mail, and Mixed-Mode Surveys: The Tailored Design Method, 3rd Edn. Hoboken, NJ: John Wiley and Sons.
Egan, P. A., Dicks, L. V., Hokkanen, H. M. T., et al. (2020). Delivering integrated pest and pollinator management (IPPM). Trends Plant Sci. 25, 577–589. doi: 10.1016/j.tplants.2020.01.006
Field, A., Miles, J., and Field, Z. (2012). “Exploratory factor analysis,” in Discovering Statistics Using R, eds F. Andy, M. Jeremy, and F. Zoe (London: SAGE Publications Ltd), 749–811.
Gaines-Day, H. R., and Gratton, C. (2017). Understanding barriers to participation in cost-share programs for pollinator conservation by Wisconsin (USA) cranberry growers. Insects 8:79. doi: 10.3390/insects8030079
Goulson, D., Nicholls, E., Botías, C., and Rotheray, E. L. (2015). Bee declines driven by combined stress from parasites, pesticides, and lack of flowers. Science 347:1255957. doi: 10.1126/science.1255957
Grasswitz, T. R. (2019). Integrated pest management (IPM) for small-scale farms in developed economies: challenges and opportunities. Insects 10:179. doi: 10.3390/insects10060179
Hall, D. M., and Martins, D. J. (2020). Human dimensions of insect pollinator conservation. Curr. Opin. Insect Sci. 38, 107–114. doi: 10.1016/j.cois.2020.04.001
Hall, D. M., and Steiner, R. (2019). Insect pollinator conservation policy innovations at subnational levels: lessons for lawmakers. Environ. Sci. Policy 93, 118–128. doi: 10.1016/j.envsci.2018.12.026
Hanes, S. P., Collum, K. K., Hoshide, A. K., and Asare, E. (2013). Grower perceptions of native pollinators and pollination strategies in the lowbush blueberry industry. Renew. Agric. Food Syst. 30, 124–131. doi: 10.1017/S1742170513000331
Headrick, D. (2021). The future of organic insect pest management: be a better entomologist or pay for someone who is. Insects 12:140. doi: 10.3390/insects12020140
Hevia, V., García-Llorente, M., Martínez-Sastre, R., Palomo, S., García, D., et al. (2020). Do farmers care about pollinators? A cross-site comparison of farmers' perceptions, knowledge, and management practices for pollinator- dependent crops. Int. J. Agric. Sustain. 19, 1–15. doi: 10.1080/14735903.2020.1807892
Hoshide, A., Drummond, F., Stevens, T. H., Venturini, E. M., Hanes, S. P., Sylvia, M. M., et al. (2018). What is the value of wild bee pollination for wild blueberries and cranberries, and who values it? Environments 5:98. doi: 10.3390/environments5090098
Kaine, G., and Bewsell, D. (2008). Adoption of integrated pest management by apple growers: the role of context. Int. J. Pest Manage. 54, 255–265. doi: 10.1080/09670870802065256
Karali, E., Brunner, B., Doherty, R., Hersperger, A., and Rounsevell, M. (2014). Identifying the factors that influence farmer participation in environmental management practices in Switzerland. Hum. Ecol. 42, 951–963. doi: 10.1007/s10745-014-9701-5
Kasina, M., Kraemer, M., Martius, C., and Wittmann, D. (2009). Farmers' knowledge of bees and their natural history in Kakamega district, Kenya. J. Apic. Res. 48, 126–133. doi: 10.3896/IBRA.1.48.2.07
Kleijn, D., Bommarco, R., Fijen, T. P. M., Garibaldi, L. A., Potts, S. G., and van der Putten, W. H. (2018). Ecological intensification: bridging the gap between science and practice. Trends Ecol. Evol. 34, 154–166. doi: 10.1016/j.tree.2018.11.002
Klein, A., Vaissière, B.E, Cane, J.H., Steffan-Dewenter, I, Cunningham, S.A, Kremen, C., et al. (2007). Importance of pollinators in changing landscapes for world crops. Proc. Royal Soc. London Biol. Sci. 274, 303–313. doi: 10.1098/rspb.2006.3721
Maderson, S., and Wynne-Jones, S. (2016). Beekeepers' knowledges and participation in pollinator conservation policy. J. Rural Stud. 45, 88–98. doi: 10.1016/j.jrurstud.2016.02.015
Main, A. R., Webb, E. B., Goyne, K. W., and Mengel, D. (2020). Reduced species richness of native bees in field margins associated with neonicotinoid concentrations in non-target soils. Agric. Ecosyst. Environ. 287:106693. doi: 10.1016/j.agee.2019.106693
McGregor, S. E. (1976). Insect pollination of cultivated crop plants. Available online at: https://www.ars.usda.gov/ARSUserFiles/20220500/OnlinePollinationHandbook.pdf (accessed July 6, 2021).
Meehan, T. D., and Gratton, C. (2016). A landscape view of agricultural insecticide use across the conterminous US from 1997 through 2012. PLoS ONE 11:e0166724. doi: 10.1371/journal.pone.0166724
Melathopoulos, A. P. (2015). Ecosystem Services, Context and the Social Basis for Conservation: The Case of Insect Pollination Benefits to Lowbush Blueberry. Dissertation. Dalhousie University, Halifax, Nova Scotia.
Mitchell, R., and Carson, R. (1989). Using Surveys to Value Public Goods. Washington, DC: Resources for the Future.
Moser, R., Pertot, I., Elad, Y., and Raffaelli, R. (2008). Farmers' attitudes toward the use of biocontrol agents in IPM strawberry production in three countries. Biol. Control 47, 125–132. doi: 10.1016/j.biocontrol.2008.07.012
Munyuli, T. (2011). Farmers' perceptions of pollinators' importance in coffee production in Uganda. Agric. Sci. 2, 318–333. doi: 10.4236/as.2011.23043
MWVG (2020). Midwest Vegetable Production Guide for Commercial Growers. Lafayette, IN: Purdue Extension.
Nalepa, R. A., Epstein, G., Pittman, J., and Colla, S. R. (2020). Perceptions of wild bees and farm characteristics associated with the uptake of pollinatorsupporting land management practices among Canadian apple growers. Renew. Agric. Food Syst. 1–10. doi: 10.1017/S1742170520000344
Nicholson, C. C., Ward, K. L., Williams, N. M., Isaacs, R., Mason, K. S., Wilson, J. K., et al. (2020). Mismatched outcomes for biodiversity and ecosystem services: testing the responses of crop pollinators and wild bee biodiversity to habitat enhancement. Ecol. Lett. 23, 326–335. doi: 10.1111/ele.13435
Obopile, M., Munthali, D. C., and Matilo, B. (2008). Farmers' knowledge, perceptions and management of vegetable pests and diseases in Botswana. Crop Protect. 27, 1220–1224. doi: 10.1016/j.cropro.2008.03.003
Park, M. G., Joshi, N. K., Rajotte, E. G., Biddinger, D. J., Losey, J. E., and Danforth, B. N. (2020). Apple grower pollination practices and perceptions of alternative pollinators in New York and Pennsylvania. Renew. Agric. Food Syst. 35, 1–14. doi: 10.1017/S1742170518000145
Petersen, J. D., Reiners, S., and Nault, B. A. (2013). Pollination services provided by bees in pumpkin fields supplemented with either Apis mellifera or Bombus impatiens or not supplemented. PLoS ONE 8:e69819. doi: 10.1371/journal.pone.0069819
Potts, S. G., Biesmeijer, J. C., Kremen, C., Neumann, P., Schweiger, O., and Kunin, W. E. (2010). Global pollinator declines: trends, impacts, and drivers. Trends Ecol. Evol. 25, 345–353. doi: 10.1016/j.tree.2010.01.007
Potts, S. G., Imperatriz-Fonseca, V., Ngo, H. T., Aizen, M. A., Biesmeijer, J. C., Breeze, T. D., et al. (2016). Safeguarding pollinators and their values to human well-being. Nature 540, 221–229. doi: 10.1038/nature20588
Pouta, E., Myyra, S., and Hanninen, H. (2011). Farm landowners' objectives in Finland: two approaches for owner classifications. Soc. Nat. Resour. 24, 1042–1062. doi: 10.1080/08941920.2010.513698
Ranjan, P., Wardropper, C. B., Eanes, F. R., Reddy, S. M. W., Harden, S. C., Masuda, Y. J., et al. (2019). Understanding barriers and opportunities for adoption of conservation practices on rented farmland in the US. Land Use Policy 80, 214–223. doi: 10.1016/j.landusepol.2018.09.039
Reilly, J. R., Artz, D. R., Biddinger, D., Bobiwash, K., Boyle, N. K., Brittain, C., et al. (2020). Crop production in the USA is frequently limited by a lack of pollinators. Proc. Biol. Sci. 287:20200922. doi: 10.1098/rspb.2020.0922
Reimer, A. P., Thompson, A. W., and Prokopy, L. S. (2012). The multi-dimensional nature of environmental attitudes among farmers in Indiana: implications for conservation adoption. Agric. Human Values 29, 29–40. doi: 10.1007/s10460-011-9308-z
Revelle, W. (2020). psych: Procedures for Psychological, Psychometric, and Personality Research. Northwest University, Evanston, IL, United States.
Sánchez, E., De Gioia, F., Kime, L. F., and Harper, J. K. (2018). Agricultural Alternatives: Cumber Production. Penn State Extension. University Park, Pennsylvania, United States.
Sanchez-Bayo, F., and Goka, K. (2014). Pesticide residues and bees - a risk assessment. PLoS ONE 9:e94482. doi: 10.1371/journal.pone.0094482
Sawe, T., Nielsen, A., and Eldegard, K. (2020). Crop pollination in small-scale agriculture in Tanzania: household dependence, awareness and conservation. Sustainability 12:2228. doi: 10.3390/su12062228
Schreinemachers, P., Chen, H.-p., Nguyen, T. T. L., Buntong, B., Bouapao, L., Gautam, S., et al. (2017). Too much to handle? Pesticide dependence of smallholder vegetable farmers in Southeast Asia. Sci. Tot. Environ. 593–594, 470–477. doi: 10.1016/j.scitotenv.2017.03.181
Ternest, J. J., Ingwell, L. L., Foster, R. E., and Kaplan, I. (2020). Comparing prophylactic versus threshold-based insecticide programs for striped cucumber beetle (Coleoptera: Chrysomelidae) management in watermelon. J. Econ. Entomol. 113, 872–881. doi: 10.1093/jee/toz346
Timmons, D. (2014). Using former farmland for biomass crops: Massachusetts landowner motivations and willingness to plant. Agric. Resour. Econ. Rev. 42, 419–437. doi: 10.1017/S1068280500005529
Ulrich-Schad, J. D., Babin, N., Ma, Z., and Prokopy, L. S. (2016). Out-of-state, out of mind? Non-operating farmland owners and conservation decision making. Land Use Policy 54, 602–613. doi: 10.1016/j.landusepol.2016.02.031
USDA NASS (2019). USDA National Agricultural Statistics Service Cropland Data Layer. Washington, DC, United States.
van der Sluijs, J. P., Simon-Delso, N., Goulson, D., Maxim, L., Bonmatin, J-M., and Belzunces, L. P. (2013). Neonicotinoids, bee disorders and the sustainability of pollinator services. Curr. Opin. Environ. Sustain. 3–4, 293–305. doi: 10.1016/j.cosust.2013.05.007
Wagner, D. L., Grames, E. M., Forister, M. L., Berenbaum, M. R., and Stopak, D. (2021). Insect decline in the Anthropocene: death by a thousand cuts. Proc. Natl. Acad. Sci. U. S. A. 118:e2023989118. doi: 10.1073/pnas.2023989118
Wauters, E., and Mathijs, E. (2014). The adoption of farm level soil conservation practices in developed countries: a meta-analytic review. Int. J. Agric. Resour. Govern. Ecol. 10, 78–102. doi: 10.1504/IJARGE.2014.061058
Westlake, S. M. (2019). Attributes Affecting Adoption of Pollinator Conservation Practices. Dissertation. Mississippi State University, Starkville, MS, United States.
Wilson, R. S., Hooker, N., Tucker, M., LeJeune, J., and Doohan, D. (2009). Targeting the farmer decision making process: a pathway to increased adoption of integrated weed management. Crop Protect. 28, 756–764. doi: 10.1016/j.cropro.2009.05.013
Winfree, R., Aguilar, R., Vázquez, D. P., LeBuhn, G., and Aizen, M. A. (2009). A meta-analysis of bees' responses to anthropogenic disturbance. Ecology 90, 2068–2076. doi: 10.1890/08-1245.1
Yang, P. Y., Iles, M., Yan, S., and Joliffe, F. (2005). Farmers' knowledge, perceptions and practices in transgenic Bt cotton in small producer systems in Northern China. Crop Protect. 24, 229–239. doi: 10.1016/j.cropro.2004.07.012
Zhang, H., Potts, S. G., Breeze, T., and Bailey, A. (2018). European farmers' incentives to promote natural pest control service in arable fields. Land Use Policy 78, 682–690. doi: 10.1016/j.landusepol.2018.07.017
Keywords: integrated pest management, pesticide use, pollinator-dependent crops, perceptions of pollinators, pollination strategies, university extension, wild bee pollinators
Citation: Bloom EH, Bauer DM, Kaminski A, Kaplan I and Szendrei Z (2021) Socioecological Factors and Farmer Perceptions Impacting Pesticide Use and Pollinator Conservation on Cucurbit Farms. Front. Sustain. Food Syst. 5:672981. doi: 10.3389/fsufs.2021.672981
Received: 26 February 2021; Accepted: 28 June 2021;
Published: 02 August 2021.
Edited by:
Helda Morales, The South Border College (ECOSUR), MexicoReviewed by:
Neelendra K. Joshi, University of Arkansas, United StatesMenelaos Stavrinides, Cyprus University of Technology, Cyprus
Copyright © 2021 Bloom, Bauer, Kaminski, Kaplan and Szendrei. This is an open-access article distributed under the terms of the Creative Commons Attribution License (CC BY). The use, distribution or reproduction in other forums is permitted, provided the original author(s) and the copyright owner(s) are credited and that the original publication in this journal is cited, in accordance with accepted academic practice. No use, distribution or reproduction is permitted which does not comply with these terms.
*Correspondence: Elias H. Bloom, Ymxvb21lbDFAbXN1LmVkdQ==