- Civil and Environmental Engineering Department, University of California, Los Angeles, Los Angeles, CA, United States
While it is widely acknowledged that shifts in diet could play a large role in mitigating climate change with important health co-benefits, knowledge on how to accomplish these shifts is lacking. Our previous study showed a statistically significant reduction in the dietary carbon footprint of students who had completed a college course on the connections between food and the environment compared to a control group enrolled in an unrelated course. An extension of the previous study, this research evaluates the sustainability of female and male diets in both the intervention and control groups from baseline to follow up with respect to the following planetary boundaries: greenhouse gases, land use, water use, nitrogen loss, and phosphorus use. In addition, a 50-point modified Alternative Healthy Eating Index was calculated at baseline and follow up for all students. Female students enrolled in the intervention course reported diets with statistically significant reductions in their footprints from baseline to follow up for greenhouse gases (p = 0.011), land use (p = 0.012), and phosphorus (p = 0.045), and the female diets were statistically different from the control groups for those three boundaries. For water use, female diets increased in footprint from baseline to follow up due to an increase in vegetable intake. Males enrolled in the intervention showed similar trends (reductions in footprints for greenhouse gases, land use, and phosphorus use and an increase in blue water use), but differences were not statistically significant, partially due to the smaller number of male respondents. Student dietary footprints are compared to a per capita limit allowable for food according to the planetary boundaries concept. For all of the planetary boundaries except blue water use, the student dietary footprints were well above the per capita boundary for food-related sources.
Introduction
Currently, society is facing both critical environmental challenges and increases in non-communicable diseases that are already diminishing the quality of life for much of the global population and are on track to worsen. These issues are woven together such that solutions to each one offer numerous and substantial co-benefits for the other (Springmann et al., 2016, 2018a,b; Hallström et al., 2017; Godfray et al., 2018). In particular, diets are an important determinant in both of these issues (Tilman and Clark, 2014; Cleveland and Gee, 2017; Clark et al., 2018), and indeed, food systems need to be changed dramatically in order to mitigate climate change and its adverse impacts on human health and well-being globally (Clark et al., 2020). However, much work is needed to address both the relationships between personal dietary choices and the planetary boundaries and how to accomplish needed behavioral and societal changes.
The planetary boundaries concept offers a framework for understanding the limits on anthropogenic changes in order to avoid destabilization of the biosphere (Rockstrom et al., 2009; Steffen et al., 2015). The environmental processes for which boundaries were developed include climate change, nitrogen and phosphorus cycling, water use, and land use, among others. Boundaries represent a “safe operating space for humanity” (Rockstrom et al., 2009), suggesting that disruptions in each process beyond the sustainable limit may lead to non-linear changes in ecosystem services upon which human society depends. Notably, food systems are important drivers in all of the planetary boundaries. They account for a large fraction of anthropogenic greenhouse gas emissions, freshwater, and nutrient use, and land conversion for crops and pasture is the major driver of biodiversity loss (Tilman and Clark, 2014; Springmann et al., 2018a; Willett et al., 2019). A recent paper found that even if all fossil fuel emissions were halted immediately, food-related greenhouse gas emissions only would exceed the allowable limit if we are to avoid disastrous climate change (Clark et al., 2020). This is in accord with other work demonstrating that dramatic changes in food demand are necessary for climate stability (Hedenus et al., 2014; Bryngelsson et al., 2016).
The EAT Lancet Commission recently presented targets for food-related greenhouse gas emissions and land, water, N, and P use (assuming a population of 10 billion in 2050) exclusively for the food-system at a global level (see Table 1) (Willett et al., 2019). For example, in order for total CO2−eq emissions not to exceed 13 Gtons/year, the emissions from the agricultural sector alone should not exceed 5 Gton/year. Springmann et al. (2018a) investigated how 1) reductions in food waste, 2) improvements in technology, and 3) shifts in diet can bring our food system footprints more in line with the planetary boundaries. Modeling the impacts of the food system under various scenarios made clear that advancements are essential in all three of these areas in order to say within sustainable limits. Specifically, only the scenario of involving a 75% reduction in food loss and waste, more ambitious technology improvements, and global shift to flexitarian diets resulted in sustainable footprint for all the boundaries studied.
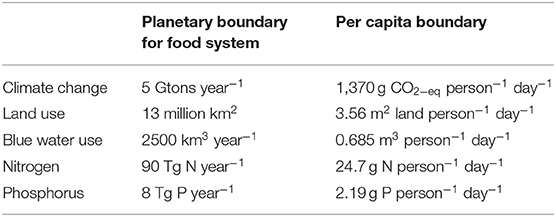
Table 1. Global planetary boundaries for the food system alone (Willett et al., 2019), and per capita boundaries calculated assuming a population of 10 billion people in 2050.
Typically, environmental footprints for various foods are calculated using Life Cycle Analysis (LCA), which is an estimate of the resources required for the item. However, LCA typically does not incorporate actual limits based on how much disruption can occur in ecosystems before undesirable outcomes occur (Ryberg et al., 2016). Even though environmental footprint data are available for a multitude of food items, information on how those data relate to allowable limits on a per capita basis is lacking and there is a need to express impact in the context of the planetary boundaries (Ryberg et al., 2018). While more theoretical work is needed to develop a new LCA framework for food, as a first approximation for connecting individual diets to the planetary boundaries, we used the EAT Lancet Commission targets for the allowable contribution from the agricultural sector's contribution in 2050 to calculate a per capita boundary (based on a population of 10 billion in 2050) as a first estimate of an “allowable” per capita dietary footprint for greenhouse gases and land, water, N, and P use. For carbon dioxide equivalents, the boundary is 5 Gt/year (1,370 g CO2−eq/cap-day); for cropland use, the boundary is 13M km2 (3.56 m2/cap-day); for blue water use, the boundary is 2,500 km3blue water/year (0.685 m3/cap-day); for nitrogen, the boundary is 90 Tg N/year (24.7 g N/cap-day); and for phosphorus, the boundary is 8 Tg P/cap-year (see Table 1). These values provide context for environmental footprint values and a target for defining a sustainable diet in terms of the planetary boundaries framework.
There is very little information currently available on the effectiveness of educational interventions concerning for promoting more sustainable diets at the college levels. See the Supplementary Material for the method and results from a systematic literature review for educational interventions conducted at colleges and universities. Among studies addressing information-only interventions, results were mixed. While traffic light labeling reduced meat sales in one study, it did not for a second study (Slapo and Karevold, 2019). Two studies showed that informational messaging reduced food waste by 15% (Whitehair, 2013; Pinto et al., 2018), while another study showed simply providing information didn't significantly impact the support for initiatives to reduce meat (De Groeve and Bleys, 2017). Shorter class-based interventions in the literature include a 50 min lecture showing a 4% increase in probability of purchasing a plant-based meal (Jalil et al., 2020), a two-week online intervention showing increased food knowledge (Mehta et al., 2020), a three-week course on water footprints that didn't significantly change consumption patterns (Godfrey and Feng, 2017), and a series of four class activities that increased student interest (Txurruka et al., 2016).
There are few studies of dietary impacts from environmental educational interventions spanning at least a quarter. A one-unit seminar course showed a dietary carbon footprint reduction at three universities (Malan et al., 2020), while a one-year intensive course showed decreased dietary carbon footprint even five years after the course (Cordero et al., 2020). An ecological footprint calculator used throughout a course showed increased awareness of the importance of food and about half of the students reported feeling ready to make changes to their diet (Collins et al., 2018). Our previous study described a significant dietary carbon footprint reduction associated with a two-quarter course at University of California Los Angeles (UCLA), indicating important climate mitigation potential for education in the connection between food and the environment (Jay et al., 2019). If the carbon footprint reduction observed in the course were extrapolated across the U.S. population, the emissions savings would amount to a third of the greenhouse gas reduction called for by the Paris Climate Accord. However, changes in the other planetary boundaries were not quantified, and further, the footprints were not compared to sustainability guidelines. Health improvements were also not assessed.
Using the same dietary survey dataset in Jay et al. (2019), we extend the previous work by: 1) comparing the carbon, nitrogen, phosphorus, water, and land use footprints for the students in the intervention and control groups at baseline and follow up; 2) comparing the dietary environmental footprints to per capita planetary boundaries; 3) quantifying impacts of the course on a modified 50 point Alternative Healthy Eating Index (modified AHEI); and 4) investigating the relationships between the modified AHEI and the environmental footprints for various groups of students.
Materials and Methods
Assessment of Environmental Footprints of Students Before and After Course
This work is an extension of a previous study describing the impacts of a two-quarter environmental science course on the carbon footprint of student diets (Jay et al., 2019). Briefly, first year undergraduate students enrolled in two STEM-field general education two-quarter classes were surveyed about their diets at baseline (the start of the class) and follow up (six months later at the end of the second quarter). While both courses fulfilled general education credits, they were both electives and categorized as Science, Technology, Engineering, and Mathematics (STEM) courses. The intervention course, titled, “Food: A Lens for Environment and Sustainability,” focused on the impact of food on the environment (experimental group, referred to hereafter as “Food Cluster”). The comparison course, titled, “Evolution of the Cosmos and Life,” which did not cover the impact of food has on the environment (control group, referred to hereafter as “Cosmos Cluster”). Please see the Supplementary Materials for the syllabi for both courses and the survey used to assess diets at baseline and follow up. A total of 162 freshmen students completed the survey, with 73 students in the Cosmos Cluster and 89 students in the Food Cluster. In both courses, there are a total of 40 males and 122 females. Because the students in both courses were exclusively freshmen, the baseline represents their diet upon arriving at college, and the trend for the comparison group should be representative of typical changes occurring for students beginning college. Details for both of the courses as well as the results for the carbon footprint calculations are presented in Jay et al. (2019). For this study, we applied published values for blue water use, land use, nitrogen and phosphorus footprints for various foods to the survey data that was previously analyzed for carbon footprint (Meier and Christen, 2013; Heller and Keoleian, 2014; Clune et al., 2017; Springmann et al., 2018a; Waite et al., 2019). All conversion factors along with sources can be found in SI. To downscale the planetary boundaries, we used an egalitarian per capita approach (Ryberg et al., 2020).
Categorization of Student Diets Based on Meat Consumption
Due to the relatively high carbon footprints for animal products compared to plant-based foods, student diets in both courses were categorized according to meat consumption in order to study the environmental footprints in terms of greenhouse gases and land, N, P, and blue water use of actual student diets containing varying levels of meat. The goal of this analysis was to investigate how the distribution of students between the various diet categories differed between the two courses and between baseline and follow up. The dietary categories used in the paper are drawn from Scarborough et al. (2014) and are as follows: high meat eaters, medium meat eaters, low meat eaters, fish eaters (pescitarians), vegetarians, and vegans. The diet types were determined by combining data from all eight data sets, standardizing the diets to a 2,000 kcal per day diet, and then grouping the participants by their meat and animal product consumption based on the cutoffs provided in Scarborough et al. (2014). Diets were normalized to 2,000 kcal per day so that the environmental footprints calculated here can be compared with literature values, which are typically reported for 2,000 kcal per day diets (Meier and Christen, 2013; Heller and Keoleian, 2014; Scarborough et al., 2014). In addition, the normalization partially addresses the student diets that were clearly underreported or overreported based on total calories reported. In our survey, meat includes chicken, beef, and pork. High meat eaters are identified as those who eat 100 g/d or more of meat, medium meat eaters are identified as those who eat 50 to 99 g/d of meat, and low meat eaters are identified as those who eat less than 50 g/d of meat but more than 0 g/d (Scarborough et al., 2014). Fish eaters were categorized as those who did not consume any meat (0 g/d) but did consume any amount of fish (>0 g/day). Vegetarians were categorized as those who did not consume any meat or fish but did consume any amount (>0 g/day) of eggs or dairy. Vegans consumed 0 g/day of all meat, fish, eggs, and dairy.
Healthy Eating Index for Student Diets Before and After Course
In order to measure the impact of diet on individual health, an Alternative Healthy Eating Index (AHEI) has been presented in the literature and shown to be correlated with decreased rates of chronic illness and premature death (Chiuve et al., 2012; Wang et al., 2014, 2019). The AHEI has a potential range of 0–100, with 0 being the least healthy and 100 being the most healthy. In 2017, the average global AHEI was 49.5 for males and 50.5 for females (~50 overall), inclusive of all diet types. A global shift to a healthier (plant-based) diet has the potential to prevent over 11 million premature deaths per year in the future (Wang et al., 2019).
A 50-point modified Alternative Healthy Eating Index (modified AHEI) was determined by considering consumption from fruits; vegetables; legumes, nuts and seeds; red meat; and sugar-sweetened beverages, each on a 10-point scale to demonstrate their overall contributions to the participants' health. This index took into account five of the ten categories in the original papers, and the conversion factors for the five categories analyzed were derived from these papers (Chiuve et al., 2012; Wang et al., 2014, 2019). Type of produce (organic versus non-organic) or meat (pasture raised versus conventional) is not considered in this analysis. Unfortunately, our dietary survey (see the Supplementary Materials) did not allow calculation of all ten categories, as has been done previously for the AHEI. For example, while the consumption of wheat was quantified in the student diets, the survey did not distinguish between whole grain and refined wheat. The omega-3 category of the AHEI was not used because it does not include plant-based sources of omega-3's, which were plentiful in some of the student diets. For each of the five categories, a score of 10 was considered as healthiest, while 0 was least healthy. For the first three categories, the weight (in grams) of the food reportedly consumed by a participant per day was multiplied by a conversion factor, with all values falling over the maximum of 10 being redefined as 10.
In order to calculate the fruit score, a conversion factor of 0.038 was multiplied by the normalized daily total fruit consumption in grams (including both temperate and tropical fruits) for both men and women. For example, a student consuming an average of one serving of temperate fruit such as apples, grapes, blueberries, strawberries per day (145 g per serving) would have a score of 5.5 (145*0.038 = 5.5) for the fruit category. A student consuming three servings of temperature fruit per day (435 g per day) would have the maximum score of 10 for the fruit category (435*0.038 = 16.5). For tropical fruits such as mangos, oranges, kiwis, and avocados, an average of 177 g per serving was used. Both categories of fruits were summed for the AHEI score.
A similar approach was used for vegetables, including all vegetables (114 g per serving), potatoes (without french fries) (213 g per serving), and corn (170 g per serving), and multiplied by a conversion factor of 0.030 for both men and women. One serving per day of vegetables would result in a score of 3.4 for the vegetable category. Legumes (117 g per serving), nuts (30 g per serving), and seeds (8.6 g per serving) included all legumes, peanuts, almonds, walnuts, chia seeds, and flax seeds, with conversion factors of 0.163 and 0.149 for women and men, respectively. The converted values were left as multiplied for these foods so that higher consumption was correlated to a higher health score, with a maximum of 10.
For the final two categories, the weight (in grams) of the food consumed per participant per day was multiplied by a conversion factor and then that value was subtracted from 10. Values that fell under the minimum of 0 were redefined as 0.
Red meat was calculated by multiplying the normalized daily grams consumed by conversion factors of 0.066 and 0.067 for women and men, respectively. For soda, the conversion factors were 0.041 for women and 0.043 for men. The converted values were subtracted from 10 for these foods so that higher consumption was correlated to a lower health score. One 12-oz can of soda every week (369 g per week, or 52.7 g per day) consumed by female student would result in a health score of 7.8 out of a possible 10 (e.g. 10–(52.7*0.041) = 7.8). One can consumed on a daily basis would result in a score of 0 for that category, since 369 times the conversion factor is greater than 10 for both females and males.
These scores were then summed in order to holistically rank the students' diets on a scale of 0 to 50. The individual modified AHEI scores were then averaged within their respective dietary categories in order to provide insight into the correlation between the student diet and health. This analysis was conducted for the various groups of students (males and females in the intervention and comparison groups) and for the various dietary categories.
Statistics
As in the previous study (Jay et al., 2019), output from Survey Monkey was downloaded as a Microsoft Excel sheet, and Excel was used to convert the reported servings of each food category to calories, grams, and environmental footprints in terms of greenhouse gas emissions, land use, blue water use, and nitrogen and phosphorus loss for every student. For assessment of the educational intervention, student diets were then grouped by gender, course taken, and time point for statistical analysis. For the diet categorization part of the study, student diets were grouped by level of meat consumption, as described above. R was used to assess normality of each group environmental footprints using visual inspection of histograms and the Shapiro test. The significance of difference between groups was assessed with t-tests only when both groups being compared were normally distributed; if one or both groups was not normally distributed, non-parametric analogs were used. To compare baseline and follow up responses from the same groups of students, paired t-tests and the Wilcoxon signed-rank test were used. For differences between groups of students, unpaired t-tests and the Mann Whitney Wilcoxon test were used.
Results and Discussion
Impacts of Course on Student Dietary Environmental Footprints
To test the hypothesis that education in food and sustainability resulted in lower dietary environmental footprints, student surveys from before and after the Food (intervention) and Cosmos (comparison) classes were used to calculate CO2−eq, water use, N, P, and land use footprints of the student diet (Figures 1–5). Each footprint can be compared to the per capita dietary footprint for each planetary boundary process consistent with sustainably feeding a population of 10 billion in 2050, as derived from the planetary boundaries for the agricultural sector as presented in the EAT Lancet Commission report on a Planetary Health Diet (Willett et al., 2019).
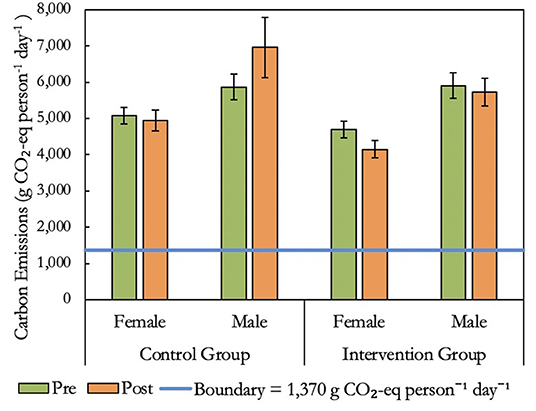
Figure 1. Changes in dietary carbon footprint from baseline to follow up for female and male students in the intervention and control group. The per capita food-related planetary greenhouse gas boundary of 1,370 g CO2−eq person−1 day−1 (for a population of 10 billion in 2050) is shown by the blue line.
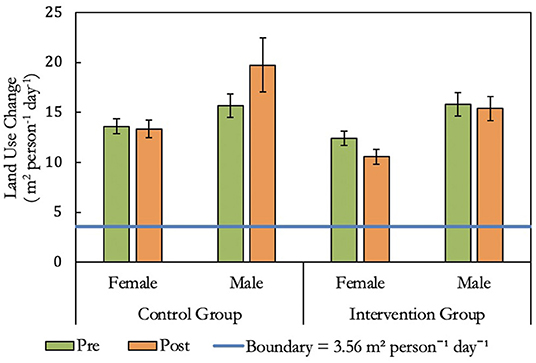
Figure 2. Changes in dietary land use footprint from baseline to follow up for female and male students in the intervention and control group. The per capita food-related planetary boundary of 3.56 m2 land person−1 day−1 (for a population of 10 billion in 2050) is shown by the blue line.
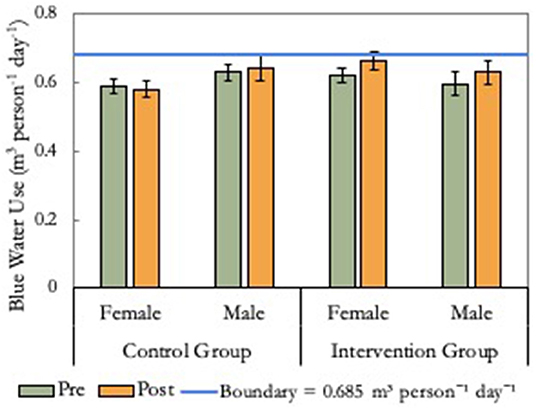
Figure 3. Changes in dietary water use footprint from baseline to follow up for female and male students in the intervention and control group. The per capita food-related planetary boundary of 0.685 m3 person−1 day−1 (for a population of 10 billion in 2050) is shown by the blue line.
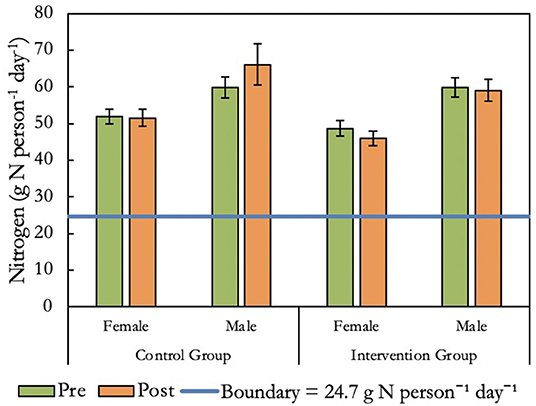
Figure 4. Changes in dietary nitrogen footprint from baseline to follow up for female and male students in the intervention and control group. The per capita food-related planetary boundary of 24.7 g N person−1 day−1 (for a population of 10 billion in 2050) is shown by the blue line.
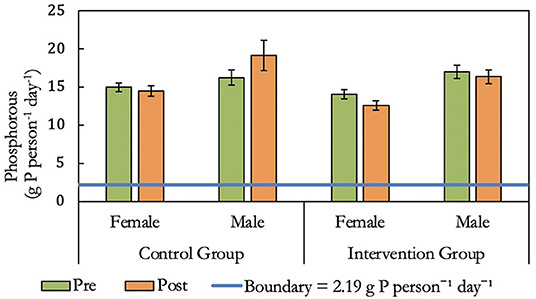
Figure 5. Changes in dietary phosphorus footprint from baseline to follow up for female and male students in the intervention and control group. The per capita food-related planetary boundary of 2.19 g P person−1 day−1 (for a population of 10 billion in 2050) is shown by the blue line.
Carbon Footprint
The carbon footprint of the 2,000 kcal-normalized student diets were reported in detail in Jay et al. (2019) and are compared here to the per capita boundary (Figure 1). See Jay et al. (2019) for an in-depth analysis of the impact of the course on dietary carbon footprint. Briefly, for female students, while the dietary carbon footprints were similar for the students in the intervention and control groups at baseline, there was a statistically significant drop (p = 0.011) in the intervention group's average footprint from 4,702 g CO2−eq person−1 day−1 baseline at 4,150 g CO2−eq person−1 day−1 to follow up and consequently the control and intervention groups differed by 798 kg CO2−eq per day (p = 0.032) at follow up. For male students, while the dietary footprints were similar at the baseline, the intervention group's carbon footprint at follow up was 1,232 kg CO2−eq per day lower than that for the control group, but due to small n the difference wasn't statistically significant. For the intervention group, female diets were statistically significantly lower than male diets at both baseline (p = 0.015) and follow up (p < 0.001).
Notably, all sets of student dietary footprints were above the per capita planetary boundary for carbon from the agricultural sector of 1,370 g CO2−eq/cap-day. While the idea of carbon footprints has become more well-known in recent years, generally people have difficulty understanding what level of greenhouse gas emissions would be allowable for a sustainable diet. The units of CO2−eq are unfamiliar to many people; thus, carbon footprints are often contextualized by comparing them to a footprint for driving a certain number of miles or leaving a light on for an amount of time. The analysis presented here could be extended to help the public understand how dietary carbon footprints relate to sustainability targets.
Land Use
See Figure 2 for land use footprints for female and male students in the intervention and control groups. For females, the control group's land footprint stayed at approximately 13.5 m2 land person−1 day−1 from baseline to follow up. For the intervention group, the female's land use footprint decreased (p = 0.012) from baseline (12.4 m2 land person−1 day−1) to follow up (10.6 m2 land person−1 day−1), and was lower (p = 0.016) than the control group at follow up. Statistically significant changes were not observed between intervention and control groups for male students at baseline or follow up. Females taking the course had statistically significantly lower land use footprints than males at both baseline (p = 0.0098) and follow up (p < 0.001). For both genders at both time points, dietary land use footprints were above the per capita food-related planetary N boundary of 3.56 m2 land person−1 day−1 (for a population of 10 billion in 2050).
Blue Water Use
The water footprint of foods can be thought of as having three components. Green water is the rain and soil moisture consumed in the production of the food, blue water is the irrigation from surface and ground water sources required for the food, and gray water is the amount of water needed to dilute pollution from production down to background levels. Because the planetary boundary is given for blue water, and because the literature presents clear conversion factors for blue water, this study focuses on just this part of the water footprint. See Figure 3 for blue water use footprints for female and male students in the intervention and control groups. Female students in the intervention group showed an increase (p = 0.023) in blue water footprint from 0.62 to 0.67 m3 land person−1 day−1, which resulted in an increase (p = 0.016) relative to the control group. Blue water footprints for males in both groups ranged from 0.60 to 0.65 m3 land person−1 day−1 and did not change significantly from baseline to follow up. For both genders at both time points, dietary water use footprints were just below the per capita food-related planetary boundary for blue water use of 0.685 m3 land person−1 day−1 (for a population of 10 billion in 2050).
Nitrogen Footprint
For both genders at both time points, dietary N footprints (Figure 4) were above the per capita food-related planetary N boundary of 24.7 g person−1 day−1 (for a population of 10 billion in 2050), with N footprints of roughly 50 and 60 g N person−1 day−1 for female and males, respectively. Statistically significant changes were not observed between intervention and control groups for either gender at baseline or follow up. Male dietary N footprints were greater than female footprints at both baseline (p = 0.011 and p = 0.049) and follow up (p < 0.001 and p = 0.027) for the intervention and control groups, respectively. Since the normalized calorie total was 2,000 kcal per day for each gender, the differences stem from the higher consumption of meat products in males.
Phosphorus Use
See Figure 5 for P footprints for female and male students in the intervention and control groups. Female students in the intervention group showed a decrease (p = 0.045) in P footprint from 14.5 to 12.5 g P person−1 day−1, which resulted in a decrease (p = 0.039) relative to the control group at baseline. P footprints for males in both groups ranged from 16.2 to 19.2 g P land person−1 day−1 and did not change significantly from baseline to follow up. For both genders at both time points, dietary P footprints were above the per capita food-related planetary boundary for phosphorus use of 2.19 g P person−1 day−1 (for a population of 10 billion in 2050).
Impacts of Course on Student Health Index and Relationship With Carbon Footprint
See Figure 6 for the 50-point modified AHEI for female and male students in the intervention and control groups. Female students in the intervention group showed an increase (p < 0.001) in modified AHEI from 35.2 to 39.2, which resulted in an increase (p = 0.029) relative to the control group at follow up. This was due to higher fruit and vegetable and lower red meat consumption. Modified AHEI for males in both groups ranged from 32.6 to 34.9 and did not change significantly from baseline to follow up.
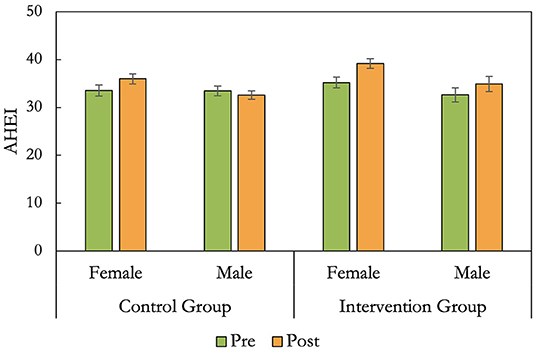
Figure 6. A 50 point modified Alternative Healthy Eating Index (AHEI) at baseline and follow up for female and male students in the intervention and control group.
Figure 7 depicts the relationship between the carbon footprint and the modified AHEI for all students in both intervention and control groups at baseline (Figure 7A) and follow up (Figure 7B). A negative slope is what is expected based given the co-benefits for health and the environment resulting from shifts toward diets with more plant foods. Indeed, a negative slope is observed in all four groups of students at both timepoints. A large increase in meat consumption in several of the control group males can be seen by comparing baseline to follow up for that category. This may be attributable to increased access to meat compared to when the students first arrive at college due to its constant presence in the dining halls.
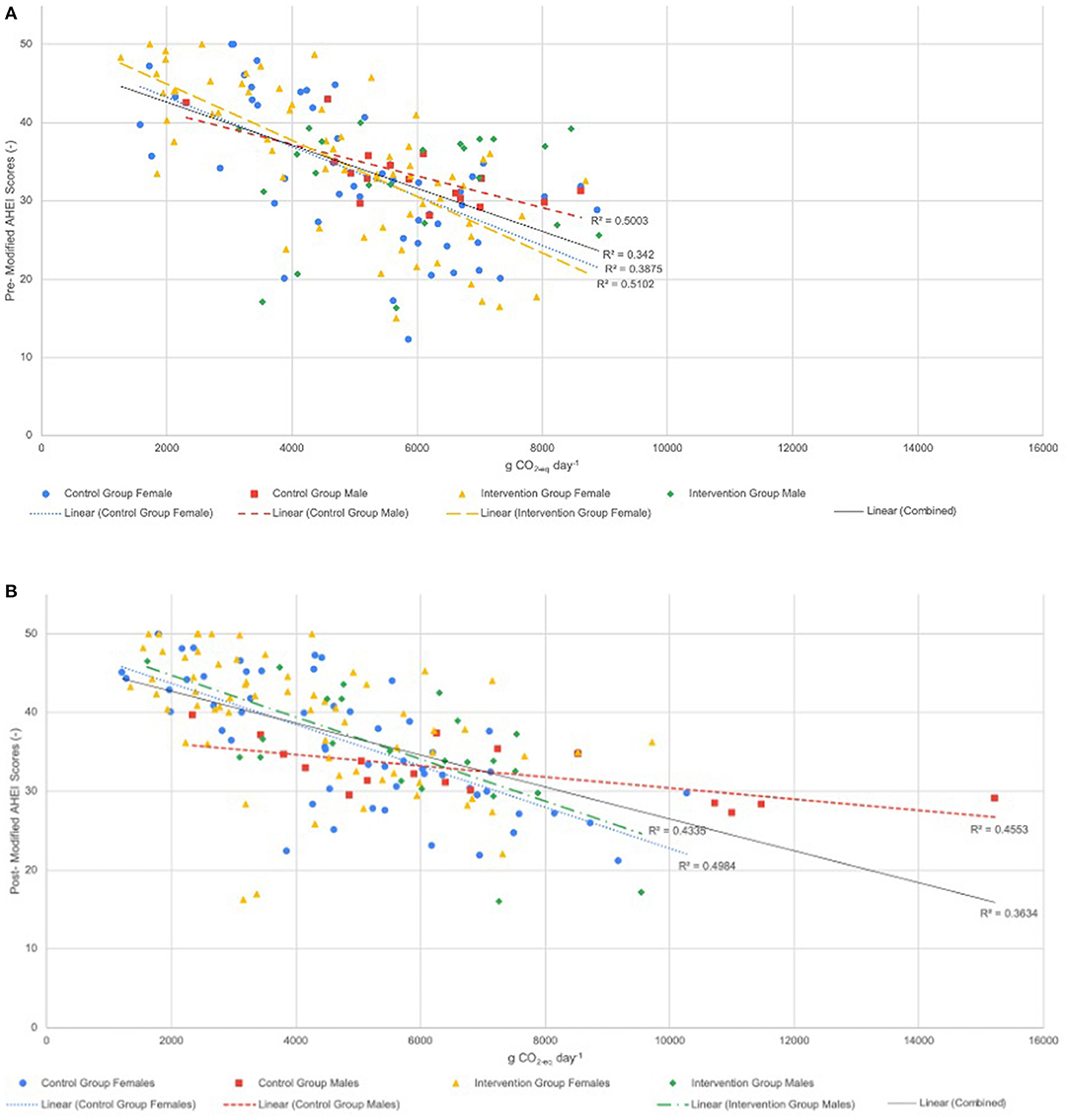
Figure 7. Relationship between a 50-point modified Alternative Healthy Eating Index and greenhouse gases for student diets at baseline (A) and follow up (B).
Categorization of Student Diets at Baseline and Follow Up
Before either course, the diets of the students in the Food Cluster (Supplementary Figure 1) had already consisted of less meat consumption than the Cosmos Cluster (Supplementary Figure 2). In the Food Cluster, 20% students were categorized as heavy meat eaters and 45% were categorized as average meat eaters, while the Cosmos Cluster had 26% students categorized as heavy meat eaters and 47% categorized as average meat eaters. See Supplementary Materials for figures. These differences might be attributed to the fact that students enrolled in a food-related class are more likely to be conscious of the health and environmental impacts of their dietary choices compared to students who are enrolled in a non-food related class. It should be noted that the average carbon footprints were not significantly different between the intervention and control groups for either females or males.
After the Cosmos Cluster, there was minimal change in animal product consumption (Supplementary Figures 2, 4). Diets of the students after two quarters in the Food Cluster moderately shifted to ones with less meat consumption (see Supplementary Figures). Although the heavy meat eater category decreased more in the Cosmo Cluster than it did in the Food Cluster, the net change in meat consumption was larger in the Food Cluster. This is because the flexitarian and vegetarian categories had increases, respectively, after the Food Cluster. No change was recorded in vegan diets.
Limitations
The limitations of the study include inaccuracies in the reported student diet that are inherent in dietary recall surveys. Also, while we used widely-accepted conversion factors for environmental footprints, these values are averages. A more refined calculation taking into account production methods was beyond the scope of the study. Finally, the per capita targets are based on the planetary boundary estimates for agricultural emission in 2050 by the EAT Lancet Commission, which are derived from extremely complex calculations. Research is needed on an ongoing basis to update and refine the targets.
Conclusions
This work is the first to our knowledge to connect individual dietary footprint calculations to targets based on the planetary boundaries framework. The per capita targets used in this analysis provide a first approximation of a sustainable diet's environmental footprints for greenhouse gases, and water, land, N, and P use. These targets could be used to contextualize environmental footprint values (currently given in absolute terms) by presenting them as a fraction or multiple of the daily target. In a recent report, the IPCC summarized the climate change mitigation potential of various diets. The same diet shifts that can result in a lower carbon footprint can help address other environmental challenges and result in improved health through higher levels of fruit and vegetable consumption. This study, as well as Jay et al. (2019) show that an intensive college course in environmental science taught through the lens of food resulted in shifts toward more sustainable diets in terms of greenhouse gases, phosphorus, and land use, particularly for female students. Due to increased produce consumption, the water footprint of female students enrolled in the course increased. Notably, though, in the case of greenhouse gases and land, N, and P use, the student dietary footprints were well above sustainable limits derived in accordance with the planetary boundaries concept for 2050. This study provides further evidence for the effectiveness of environmental education in promoting shifts toward sustainable diets.
Data Availability Statement
The data analyzed in this study is subject to the following licenses/restrictions: Data are publicly available at jaylabucla.org. Requests to access these datasets should be directed to Jennifer A. Jay, jennyjay@ucla.edu.
Ethics Statement
The studies involving human participants were reviewed and approved by UCLA Institutional Review Board. Written informed consent from the participants' legal guardian/next of kin was not required to participate in this study in accordance with the national legislation and the institutional requirements.
Author Contributions
VW, BC, JJ, IS, and PN conducted data analysis and writing. JJ conceived of the study, co-taught the course, and advised the project. All authors contributed to the article and approved the submitted version.
Conflict of Interest
The authors declare that the research was conducted in the absence of any commercial or financial relationships that could be construed as a potential conflict of interest.
Acknowledgments
The authors are grateful to the co-instructors of the Food Cluster course, Dr. J. Cully Nordby, Dr. Raffaella D'Auria, and Dr. D. Andy Rice, as well as the instructor of the Cosmos class, Dr. Anthony Friscia. We are also thankful to Dr. May Wang and Dr. Wendelin Slusser for guidance with the survey and to David Cleveland and Hannah Malan for helpful discussions. We thank our reviewers and the editor for substantially improving the manuscript.
Supplementary Material
The Supplementary Material for this article can be found online at: https://www.frontiersin.org/articles/10.3389/fsufs.2021.677002/full#supplementary-material
References
Bryngelsson, D., Wirsenius, S., Hedenus, F., and Sonesson, U. (2016). How can the EU climate targets be met? a combined analysis of technological and demand-side changes in food and agriculture. Food Policy 59, 152–164. doi: 10.1016/j.foodpol.2015.12.012
Chiuve, S. E., Fung, T. T., Rimm, E. B., Hu, F. B., McCullough, M. L., Wang, M., et al. (2012). Alternative dietary indices both strongly predict risk of chronic disease. J. Nutr. 142, 1009–1018. doi: 10.3945/jn.111.157222
Clark, M., Hill, J., and Tilman, D. (2018). The Diet, health, and environment trilemma. Ann. Rev. Environ. Res. 43, 109–134. doi: 10.1146/annurev-environ-102017-025957
Clark, M. A., Domingo, N. G. G., Colgan, K., Thakrar, S. K., Tilman, D., Lynch, J., et al. (2020). Global food system emissions could preclude achieving the 1.5° and 2°C climate change targets. Science 370, 705–708. doi: 10.1126/science.aba7357
Cleveland, D., and Gee, Q. (2017). “Plant-based diets for mitigating climate change,” in Vegetarian and Plant-Based Diets in Health and Disease Prevention, ed F. Mariotti (Cambridge, MA: Academic Press), 135–156. doi: 10.1016/B978-0-12-803968-7.00009-5
Clune, S., Crossin, E., and Verghese, K. (2017). Systematic review of greenhouse gas emissions for different fresh food categories. J. Cleaner Prod. 140, 766–783. doi: 10.1016/j.jclepro.2016.04.082
Collins, A., Galli, A., Patrizi, N., and Pulselli, F. M. (2018). Learning and teaching sustainability: the contribution of ecological footprint calculators. J. Cleaner Prod. 174, 1000–1010. doi: 10.1016/j.jclepro.2017.11.024
Cordero, E. C., Centeno, D., and Todd, A. M. (2020). The role of climate change education on individual lifetime carbon emissions. PLoS ONE 15, 1–24. doi: 10.1371/journal.pone.0206266
De Groeve, B., and Bleys, B. (2017). Less meat initiatives at Ghent University: assessing the support among students and how to increase it. Sustainability 9, 1–13. doi: 10.3390/su9091550
Godfray, H. C. J., Aveyard, P., Garnett, T., Hall, J. W., Key, T. J., Lorimer, J., et al. (2018). Meat consumption, health, and the environment. Science 361:eaam5324. doi: 10.1126/science.aam5324
Godfrey, D., and Feng, P. (2017). Communicating sustainability: student perceptions of a behavior change campaign. Int. J. Sustainability High. Edu. 18, 2–22. doi: 10.1108/IJSHE-01-2015-0009
Hallström, E., Gee, Q., Scarborough, P., and Cleveland, D. A. (2017). A healthier US diet could reduce greenhouse gas emissions from both the food and health care systems. Climatic Change 142, 199–212. doi: 10.1007/s10584-017-1912-5
Hedenus, F., Wirsenius, S., and Johansson, D. J. A. (2014). The importance of reduced meat and dairy consumption for meeting stringent climate change targets. Climatic Change 124, 79–91. doi: 10.1007/s10584-014-1104-5
Heller, M. C., and Keoleian, G. A. (2014). Greenhouse gas emission estimates of of U.S. Dietary choices and food loss. J. Ind. Ecol. 19, 391–401. doi: 10.1111/jiec.12174
Jalil, A. J., Tasoff, J., and Bustamante, A. V. (2020). Eating to save the planet: evidence from a randomized controlled trial using individual-level food purchase data. Food Policy 95:101950. doi: 10.1016/j.foodpol.2020.101950
Jay, J. A., D'Auria, R., Nordby, J. C., Rice, D. A., Cleveland, D. A., Friscia, A., et al. (2019). Reduction of the carbon footprint of college freshman diets after a food-based environmental science course. Climatic Change 154, 547–564. doi: 10.1007/s10584-019-02407-8
Malan, H., Challamel, G. A., Silverstein, D., Ho, C., Spang, E., Pace, S. A., et al. (2020). Impact of a scalable, multi-campus “foodprint” seminar on college student's dietary carbon footprint. Nutrients 12, 1–17. doi: 10.3390/nu12092890
Mehta, K., Rohrlach, H., Woodman, R., Coveney, J., Ward, P., and Booth, S. (2020). A critical food system program in South Australia and the effects on consumer knowledge and attitudes. Health Promot. J. Austr. doi: 10.1002/hpja.370. [Epub ahead of print].
Meier, T., and Christen, O. (2013). Environmental impacts of dietary recommendations and dietary styles: Germany as an example. Environ. Sci. Technol. 47, 877–888. doi: 10.1021/es302152v
Pinto, R. S., Pinto, R. M., dos, S., Melo, F. F. S., Campos, S. S., and Cordovil, C. M. dos S. (2018). A simple awareness campaign to promote food waste reduction in a University canteen. Waste Manage. 76, 28–38. doi: 10.1016/j.wasman.2018.02.044
Rockstrom, J., Steffen, W., Noone, K., Persson, A., Chapin, F. S., Lambin, E., et al. (2009). A safe operating space for humanity. Nature 461, 472–475. doi: 10.1038/461472a
Ryberg, M. W., Andersen, M. M., Owsianiak, M., and Hauschild, M. Z. (2020). Downscaling the planetary boundaries in absolute environmental sustainability assessments — A review. J. Clean. Prod. 276:123287. doi: 10.1016/j.jclepro.2020.123287
Ryberg, M. W., Owsianiak, M., Richardson, K., and Hauschild, M. Z. (2016). Challenges in implementing a planetary boundaries based lifecycle impact assessment methodology. J. Cleaner Prod. 139, 450–459. doi: 10.1016/j.jclepro.2016.08.074
Ryberg, M. W., Owsianiak, M., Richardson, K., and Hauschild, M. Z. (2018). Development of a life-cycle impact assessment methodology linked to the planetary boundaries framework. Ecol. Indic. 88, 250–262. doi: 10.1016/j.ecolind.2017.12.065
Scarborough, P., Appleby, P. N., Mizdrak, A., Briggs, A. D. M., Travis, R. C., Bradbury, K. E., et al. (2014). Dietary greenhouse gas emissions of meat-eaters, fish-eaters, vegetarians and vegans in the UK. 125, 179–192. doi: 10.1007/s10584-014-1169-1
Slapo, H. B., and Karevold, K. I. (2019). Simple eco-labels to nudge customers toward the most environmentally friendly warm dishes: an empirical study in a cafeteria setting. Front. Sustainable Food Syst. 3, 1–9. doi: 10.3389/fsufs.2019.00040
Springmann, M., Clark, M., Mason-D'Croz, D., Wiebe, K., Bodirsky, B. L., Lassaletta, L., et al. (2018a). Options for keeping the food system within environmental limits. Nature 562, 519–525. doi: 10.1038/s41586-018-0594-0
Springmann, M., Godfray, H. C. J., Rayner, M., and Scarborough, P. (2016). Analysis and valuation of the health and climate change cobenefits of dietary change. Proc. Natl. Acad. Sci. U.S.A. 113, 4146–4151. doi: 10.1073/pnas.1523119113
Springmann, M., Wiebe, K., Mason-D'Croz, D., Sulser, T. B., Rayner, M., and Scarborough, P. (2018b). Health and nutritional aspects of sustainable diet strategies and their association with environmental impacts: a global modelling analysis with country-level detail. Lancet Planet. Health 2, e451–e461. doi: 10.1016/S2542-5196(18)30206-7
Steffen, W., Richardson, K., Rockström, J., Cornell, S. E., Fetzer, I., Bennett, E. M., et al. (2015). Planetary boundaries: guiding human development on a changing planet. Science 347:1259855. doi: 10.1126/science.1259855
Tilman, D., and Clark, M. (2014). Global diets link environmental sustainability and human health. Nature 515, 518–522. doi: 10.1038/nature13959
Txurruka, I., Larretxi, I., Miranda, J., Bustamante, M., Simon, E., and Lasa, A. (2016). “Development of “sustainability and social responsibility” as a cross curricular skill in the subject general and applied dietetics in the degree of human nutrition and dietetics,” in EDULEARN Proceedings (Barcelona), 6808–6814. doi: 10.21125/edulearn.2016.0470
Waite, R., Vennard, D., and Pozzi, G. (2019). Tracking Progress Toward the Cool Food Pledge: Setting Climate Targets, Tracking Metrics, Using the Cool Food Calculator, and Related Guidance for Pledge Signatories. Technical Note. Washington, DC: World Resources Institute. Available online at: www.coolfoodpledge.org
Wang, D. D., Leung, C. W., Li, Y., Ding, E. L., Chiuve, S. E., Hu, F. B., et al. (2014). Trends in dietary quality among adults in the United States, 1999 through 2010. JAMA Int. Med. 174, 1587–1595. doi: 10.1001/jamainternmed.2014.3422
Wang, D. D., Li, Y., Afshin, A., Springmann, M., Mozaffarian, D., Stampfer, M. J., et al. (2019). Global improvement in dietary quality could lead to substantial reduction in premature death. J. Nutr. 149, 1065–1074. doi: 10.1093/jn/nxz010
Whitehair, K. (2013). Written messages improve edible food waste behavior in a University dining facility. J. Acad. Nutr. Diet. 113, 63–69. doi: 10.1016/j.jand.2012.09.015
Keywords: education, climate change, planetary boundaries, dietary footprint, food sustainability, environmental science course
Citation: Whitener VA, Cook B, Spielbauer I, Nguyen PK and Jay JA (2021) Impact of a College Course on the Sustainability of Student Diets in Terms of the Planetary Boundaries for Climate Change and Land, Water, Nitrogen and Phosphorus Use. Front. Sustain. Food Syst. 5:677002. doi: 10.3389/fsufs.2021.677002
Received: 07 March 2021; Accepted: 09 June 2021;
Published: 15 July 2021.
Edited by:
Rakesh Bhardwaj, National Bureau of Plant Genetic Resources (ICAR), IndiaReviewed by:
Marisa Porrini, University of Milan, ItalyGuobao Song, Dalian University of Technology, China
Copyright © 2021 Whitener, Cook, Spielbauer, Nguyen and Jay. This is an open-access article distributed under the terms of the Creative Commons Attribution License (CC BY). The use, distribution or reproduction in other forums is permitted, provided the original author(s) and the copyright owner(s) are credited and that the original publication in this journal is cited, in accordance with accepted academic practice. No use, distribution or reproduction is permitted which does not comply with these terms.
*Correspondence: Jennifer A. Jay, jennyjay@ucla.edu