- 1Grupo de Bioquímica Vegetal, Instituto de Biología Agrícola de Mendoza, CONICET-Universidad Nacional de Cuyo, Facultad de Ciencias Agrarias, Mendoza, Argentina
- 2Catena Institute of Wine, Bodega Catena Zapata, Mendoza, Argentina
- 3School of Agriculture Ingenieers, Unilasalle, Mont-Saint-Aignan, France
The chemical and sensory characteristics of the wines are related to the geographical origin of the grape, as a result of the interplay between the plant material (G), its acclimatization to the environment (E) and the human factor that influences both the vineyard and the winery. The range of phenotypes that a single genotype can express depending on its environment is known as phenotypic plasticity and is the result of G × E interaction. The present study evaluated the independent and interactive effects of Cabernet Sauvignon plant materials (G: Clone 7 and Mount Eden) implanted in different geographical indications of Mendoza, Argentina (E: Agrelo, Pampa El Cepillo, Altamira and Gualtallary) according to fruit yield and phenolic profiles of wines. The experiment was carried out during 2018 and 2019 vintages using a multifactorial design. When berries reached 24 °Brix, the clusters were harvested, analyzed and wines elaborated by a standardized procedure. Then, the anthocyanin and non-anthocyanin phenolic profiles of wines were determined by high-performance liquid chromatography with diode array and fluorescence detection (HPLC-DAD–FLD). The results revealed significant G × E interactions for yield traits, including the number of clusters per plant. Differential chemical composition and quality parameters of the resulting wines, markedly affected by E, were observed; that is the geographical location of the vineyards. There were similarities in the phenolic composition between Pampa El Cepillo and Altamira, while larger differences between Agrelo and Gualtallary were observed. Gualtallary presented the highest levels of anthocyanins, quercetin and trans-resveratrol. The increased amount of these compounds in Gualtallary was associated with an increased UV-B exposure of plants at this high altitude environment. This is the first report that characterizes the effects of plant material and environment for Cabernet Sauvignon. These results are of oenological and viticulture interest for the wine industry demonstrating that the selection of the plant material and the vineyard location for Cabernet Sauvignon can considerably affect the quality attributes of wines.
Introduction
Cabernet Sauvignon is one of the best-known and most widespread red wine varieties in the world, with a high tannins concentration, within the existing red varieties (Togores, 2003). In Argentina there are planted 14,279 ha of Cabernet Sauvignon and the 76.3% of the total surface is located in the province of Mendoza (Instituto Nacional de Vitivinicultura, 2019). Within Mendoza, the largest areas of Cabernet Sauvignon are distributed in Luján de Cuyo (19.8%) and the Uco Valley (27.4%); both regions reputed for their high-quality wines. The Uco Valley is characterized by high-altitude vineyards, high ultraviolet-B solar radiation, rocky and permeable soils, and high thermal amplitudes. Meanwhile, Luján de Cuyo has vineyards at lower altitudes with deeper soils and slightly warmer temperatures.
Grapevine adapt to a wide range of environments (Van Leeuwen and Seguin, 2006; Gianoli and Valladares, 2012) with a broad phenotypic plasticity (Keller, 2010; Dal Santo et al., 2016). The phenotypic plasticity is the capacity of a genotype to express different phenotypes (including differential wine sensory attributes) when exposed to different environments (Pigliucci, 2005; Nicotra and Davidson, 2010); and plays an important role in the acclimatization and adaptation to climate change (Weiner, 2004; Van Kleunen and Fischer, 2005). Furthermore, it is known that the responses to the environment vary depending on the plant material (Schultz, 2003), i.e., some genotypes might be more suitable for a particular environment than others.
In viticulture and oenology, it is expected that the combination of genotype (plant material), microbiome (fungi and bacteria), environmental (soil and climate) and human (vineyard management and winemaking) factors affect the quality of grapes and their corresponding wines. This interaction is usually referred as the “terroir” and is finally expressed in the wine (Organización Internacional de la Viña y el Vino, 2010; Senyard et al., 2011; Anesi et al., 2015; Marlowe and Lee, 2018).
From the consumer point of view, there is much research that reveals the importance of the terroir concept when choosing a wine (Cross et al., 2011; Versari et al., 2014). Wine consumers have learned to trust provenance to predict wine quality and are willing to pay extra for it (Urvieta et al., 2021). In fact, different wine regions have exploited this concept of exclusive parcels capable of giving unique wines as an approach to add economic value to their wines. In this way, understanding phenotypic plasticity can also play an important role in the attempt to obtain differentiated wines as a commercial strategy. Phenolic compounds (PCs) are of great importance in the production of red wines, being secondary metabolites present in the vacuoles of berry skin and extracted during the winemaking process. PCs are originated in the grape berries and are essential for wines sensory characteristics, such as color, astringency, bitterness and aging capacity (Jaffré et al., 2009). These compounds are also important to evaluate the effect of the grapes environment in the wines. For example, Urvieta et al. (2021) evaluated phenolic composition of Malbec wines across multiple sites in Mendoza and was able to discriminate different GIs.
There are some studies that have shown the relevance of the grapes origin in the chemical composition of the Cabernet Sauvignon wines. Ranaweera et al. (2021) classified Australian Cabernet Sauvignon wines from three wine regions based on spectrofluorometric and multi-element analyses. Others authors evaluated Cabernet Sauvignon wines from China, and found that PCs profiles and concentrations were dependent of the vineyards locations (Li et al., 2011; Jin et al., 2017; Jiang and Sun, 2019). Another study carried out on 5 different varieties, including Cabernet Sauvignon and cultured with the same environmental conditions, demonstrated the effect of the genotype in the grape phenotype (Aleixandre et al., 2015). Pajović et al. (2014) performed a varietal differentiation of grapes from Montenegro, including Cabernet Sauvignon according to their phenolic composition. Regarding Cabernet Sauvignon wines from Argentina, there is only one study that evaluated the chemical differences. Di Paola-Naranjo et al. (2011) analyzed wines from three provinces (Mendoza, San Juan and Córdoba) and concluded that both elemental and isotopic composition allow a good differentiation among these wide regions. It should be taken into account that the wines used in the mentioned study were from different wineries, elaborated without standardized winemaking conditions, and also with different plant material (within the Cabernet Sauvignon variety).
In the present study, two Cabernet Sauvignon plant materials, implanted in four different GIs, were evaluated during two growing seasons. The PC profiles (anthocyanins and non-anthocyanins) of wines were determined by high performance liquid chromatography with a photodiode array and fluorescence detection (HPLC-DAD-FLD) with the aim to evaluate the independent and interactive effects of Cabernet Sauvignon plant materials (G: Clone 7 and Mount Eden).
Materials and Methods
Standards Preparation and Solvents
Standards of gallic acid (99%), 3-hydroxytyrosol (≥99.5%), (–)-gallocatechin gallate (≥99%), caftaric acid (≥97%), (–)-epigallocatechin (≥95%), (+)-procyanidin B1 (≥90%), (+)-catechin (≥99%), procyanidin B2 (≥90%), (–)-epicatechin (≥95%), caffeic acid (99%), syringic acid (≥95%), p-coumaric acid (99%), trans-resveratrol (≥99%), quercetin hydrate (95%), kaempferol-3-glucoside (≥99%), myricetin (≥96%) and malvidin-3-O-glucoside chloride (≥95%) used for identification and quantification of PCs were purchased from Sigma-Aldrich (St. Louis, MO, USA). The standard of 2-(4-hydroxyphenyl) ethanol (tyrosol) (≥99.5%) was obtained from Fluka (Buchs, Switzerland). Individual stock solutions of compounds were prepared in MeOH at concentration levels ranging from 400 to 2,000 mg L−1. Further dilutions and mixtures of compounds were prepared monthly in MeOH and stored in dark-glass bottles at −20°C. Calibration standards and mixtures of analytes used for calibration purposes were prepared in the initial mobile phase of each chromatographic method (anthocyanins and non-anthocyanins) and used within 3 days after their preparation. HPLC-grade FA and MeCN were acquired from Mallinckrodt Baker Inc. (Pillispsburg, NJ, USA). Ultrapure water was obtained from a Milli-Q system (Millipore, Billerica, MA, USA).
Plant Material and Experimental Design
The experiment was carried out during the 2017–2018 (2018) and 2018–2019 (2019) growing seasons with vines from two selected plant materials of Vitis vinifera L. cv. Cabernet Sauvignon. Genotypes (G) were Clone 7 (C7) and Mount Eden (ME). The Cabernet Sauvignon Mount Eden originated at Mount Eden in Santa Cruz Mountains, CA, USA (Foundation Plant Services Grape, 2011) and Clone 7 from De Latour nursery in Beaulieu, CA, USA (Caldwell, 2002). Both genotypes were chosen based on availability, fruit quatlilty and yield characteristics. Four environments (E) as parcels containing both G were selected in Mendoza province, distributed in four GIs at the main viticulture zones; First Zone (GI: Agrelo) and Uco Valley (GIs: Pampa El Cepillo, Altamira and Gualtallary) (see map of the studied area in Figure 1). Table 1 shows the vineyard location, soil classification, planting year, and density of each parcel. In common for all four sites, the vines were planted on their own roots, formed in a vertical trellis system, arranged in north-south oriented rows, and drip-watered. The irrigation criteria were based on the soil characteristics and evapotranspiration measurements within each parcel. The plants were subjected to a weak water stress level after veraison, maintaining stem water potentials (Ψs) at midday between −0.6 and −0.9 Mpa. These four locations were selected because are the most representative in terms of production and quality of wines for the Cabernet Sauvignon in Mendoza. In addition, because of the possibility to have the two vegetative materials in places with different climatic conditions. All the vineyards have anti-hail nets, except for Agrelo GI.
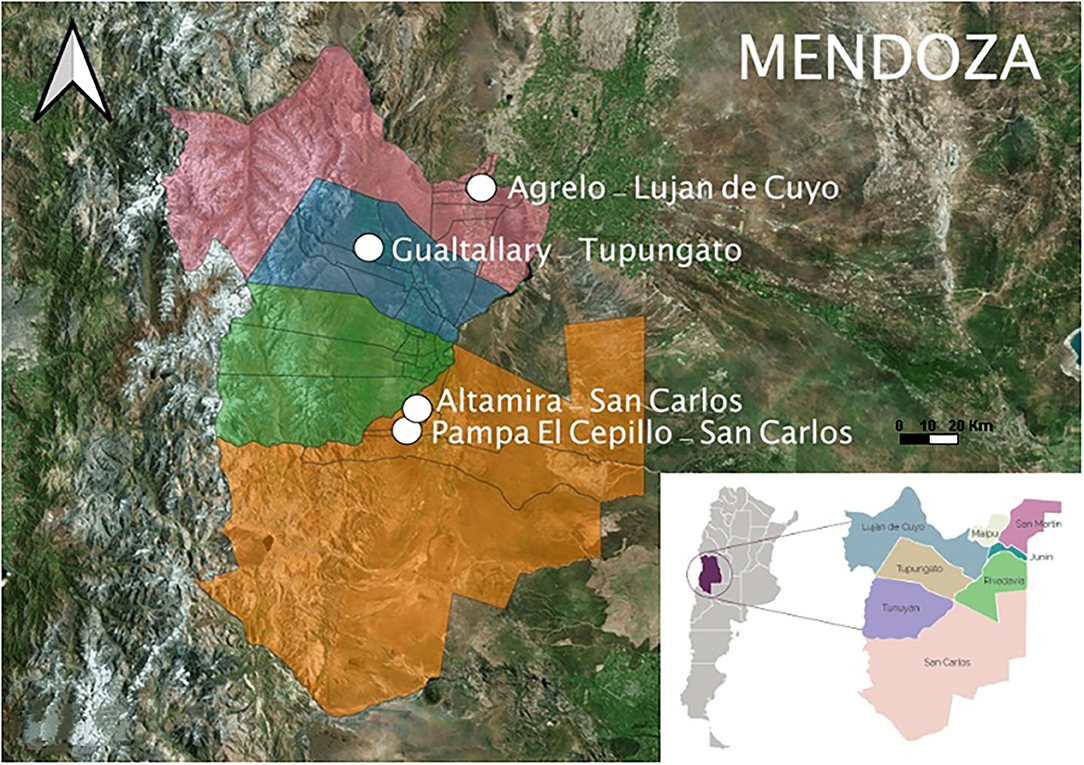
Figure 1. Geographical location of individual parcels in the study area. The images were created using QGIS.org, 2021. QGIS Geographic Information System. QGIS Association. http://www.qgis.org.
A multifactorial experimental design with 9 replicates was used. Within each G × E combination treatment, nine representative rows were selected (each one containing 100 plants). After veraison, berry maturation was followed by weekly determinations of total soluble substances in 200 randomly sampled berries with a Pocket PAL-1 digital hand-held refractometer (Atago Co., Ltd., Tokyo, Japan).
Meteorological Data
The air temperatures (daily maximum, minimum and thermal amplitude) and rainfall data for each site were registered during the 2018 and 2019 growing seasons, between September and April, i.e., from grapevine budburst to harvest. Data were obtained from the database of the Catena Institute of Wine (CIW) and the Department of Agriculture and Climate Contingencies of Mendoza, with an automatic weather station (iMetos ag, model IMT 300; Pessl Instruments, Weiz, Austria), located close to the experimental sites. Measurements were registered every 15 min for 24 h a day and the daily means were calculated. Supplementary Table 1 summarize the meteorological data of the studied parcels.
Measurements of Fruit Yield and Sampling of Clusters
At commercial maturity, when the berries of each G × E combination reached 24 ± 1 ° Brix, one plant was randomly selected per experimental row (n = 9). The first GI to be harvested was Agrelo, then Pampa El Cepillo and Altamira (1 week later) and finally Gualtallary with a difference of 3 weeks with respect to Agrelo. Two clusters were sampled from the same shoot, and all remaining clusters from the plant were harvested and weighed in the field as fruit yield. Furthermore, the number of berries per cluster, the fresh weight of the berries in the sampled clusters and the dry weight of the berry skin were determined (dried at 60°C in an oven to a constant weight).
Winemaking Procedure and Wine Analysis
A total of 24 individual wines were elaborated according to a standardized procedure (Urvieta et al., 2018) in order to obtain three replicates for each G × E combination. At commercial maturity, all the grapes from the experimental rows were manually harvested, transported to the winery, macerated for 24 h and microfermented at 25°C in 800 L plastic vessels with the addition of active dry yeast (20 g L−1; Lavin EC-1118, Lallemand Inc., Montréal, Canada). After alcoholic fermentation and 10 days of maceration (post-fermentation), 50 L of drained wine were transferred to stainless steel tanks and inoculated. with malolactic bacteria (1 g L−1; Lavin VP41, Lallemand Inc., Montreal, Canada). When the malic acid content was below 0.2 g L−1 as assessed by Oeno Foss (FOSS Analytical A/S, Hillerod, Denmark), decantation was carried out to remove thick lees. Afterwards, SO2 was added as K2S2O5 (Laffort Oenologie, France) at a final concentration of 35 mg L−1 free SO2. Then, wines were filtered with a nominal filter with a 0.2–0.4 μm cellulose plate. Wines were stored for 3 months in 50 L stainless steel tanks at 13–15°C. No tartaric stabilization was carried out during aging. Finally, wines were fractionated in green-glass bottles (750 mL volume) of each replicate (three per parcel) and stored at 15 °C until analysis.
Phenolic Compounds Profile of Wines
An HPLC-DAD-FLD (Dionex Ultimate 3000 system, Dionex Softron GmbH, Thermo Fisher Scientific Inc., Germering, Germany) and a reversed-phase Kinetex C18 column (3.0 × 100 mm, 2.6 mm; Phenomenex, Torrance, CA, USA) was used. The determination of anthocyanins was performed according to Urvieta et al. (2018), with minor modifications. Briefly, 1 mL aliquot of wine samples was evaporated to dryness by vacuum centrifugation and dissolved with 1 mL of initial mobile phase prior HPLC-DAD-FLD analysis. The different anthocyanins were separated in a reversed-phase Kinetex C18 column (3.0 × 100 mm, 2.6 μm) Phenomenex (Torrance, CA, USA). The mobile phase consisted of ultrapure H2O:FA:MeCN (87:10:3, v/v/v; eluent A) and ultrapure H2O:FA:MeCN (40:10:50, v/v/v; eluent B). The separation gradient was: 0 min, 10% B; 06 min, 25% B; 610 min, 31% B; 1,011 min, 40% B; 11–14 min, 50% B; 14–15 min, 100% B; 15–17 min, 10% B; 17–21 min, 10% B. The mobile phase flow was 1 mL min−1, column temperature 25°C and injection volume 5 μL. Quantification was carried out by measuring peak area at 520 nm and the content of each anthocyanin was expressed as malvidin-3-glucoside equivalents using an external standard calibration curve (1–250 mg L−1, r2= 0.997). The identity of detected anthocyanins was confirmed by comparison with the elution profile and identification of analytes realized in our previous research (Antoniolli et al., 2015). The compounds that were determined were the following: delphinidin 3-O-glucoside, cyanidin 3-O-glucoside, petunidin 3-O-glucoside, peonidin 3-O-glucoside, malvidin 3-O-glucoside, delphinidin 3-O-acetylglucoside, petunidin 3-O-acetylglucoside, peonidin 3-O-acetylglucoside, malvidin 3-O-acetylglucoside, cyanidin 3-O-p-coumaroylglucoside, petunidin 3-O-p-coumaroylglucoside, peonidin 3-O-p-coumaroylglucoside and malvidin 3-O-p-coumaroylglucoside.
For non-anthocyanins compounds, wine samples were analyzed according to Ferreyra et al. (2021). A reversed-phase Kinetex C18 column (3.0 × 100 mm, 2.6 μm; Phenomenex, Torrance, CA, USA) was used for the chromatographic separation. The mobile phases were an aqueous solution of 0.1% FA (solvent A) and MeCN (solvent B). The gradient was as follows: 0–1.7 min, 5% B; 1.7–10 min, 30% B; 10–13.5 min, 95% B; 13.5–15 min, 95% B; 15–16 min, 5% B; 16–19, 5% B. The total flow rate was set at 0.8 mL min−1. The column temperature was 35°C and the injection volume was 5 μL for calibration standards and wine samples. The analytical flow cell for DAD was set to scan from 200 nm to 400 nm. A data collection rate of 5 Hz, a band width of 4 nm and a response time of 1.000 s were used. Wavelengths of 254, 280, 320 and 370 nm were used depending on the analytes maximum absorbance for DAD, while an excitation wavelength (Ex) of 290 nm and a monitored emission (Em) responses of 315, 360 and 400 nm were used depending on the targeted analytes for FLD. A data collection rate of 10 Hz (peak width of 0.04 min corresponding to a response time of 0.8 s and a photomultiplier gain of 5 units) was used for FLD. The identification of non-anthocyanins in studied wines were based on the comparison of the retention times of samples with those of authentic standards. In addition, some wine samples were spiked with known concentrations of standards in order to ensure a correct quantification and identification to finally select an external calibration with pure standards as quantification technique. Linear ranges between 0.05 and 40 mg L−1 with coefficient of determination (r2) higher than 0.993 were obtained for quantified compounds.
Statistical Analysis
Multifactorial analyses and Fisher's least significant difference (LSD) test, with a significance level of P ≤ 0.05 were performed to evaluate the effect of genotype (G), environment (E) and the G × E interaction. Principal component analysis (PCA) throughout biplot graphics and standardized (centered and variance-scaled) were used to find association of the variables with the classification patterns. For anthocyanin and non-anthocyanin compounds, the matrix for the PCA consisted of 8 cases (G × E combinations) as classification criteria, with the wine phenolic compounds as variables. For meteorological data, the PCA matrix consisted of 8 cases (vineyards and growing seasons) as classification criteria. All the statistical analyses were performed with InfoStat Software (InfoStat version 2020; Grupo InfoStat, Córdoba, Argentina).
Results
Meteorological Data
Figure 2 shows that the vineyard in Agrelo (AGR) had higher maximum and minimum temperatures during both growing seasons (September–April), while the environment in Gualtallary (GUA) was the coldest as compared to the other GIs. The vineyard in Pampa El Cepillo (PEC) had the maximum accumulated rainfall for 2019 growing season and the thermal amplitude increased in AGR and Altamira (ALT) for 2018. In general, the air temperatures were similar for both growing seasons, while the 2019 was rainier than the 2018.
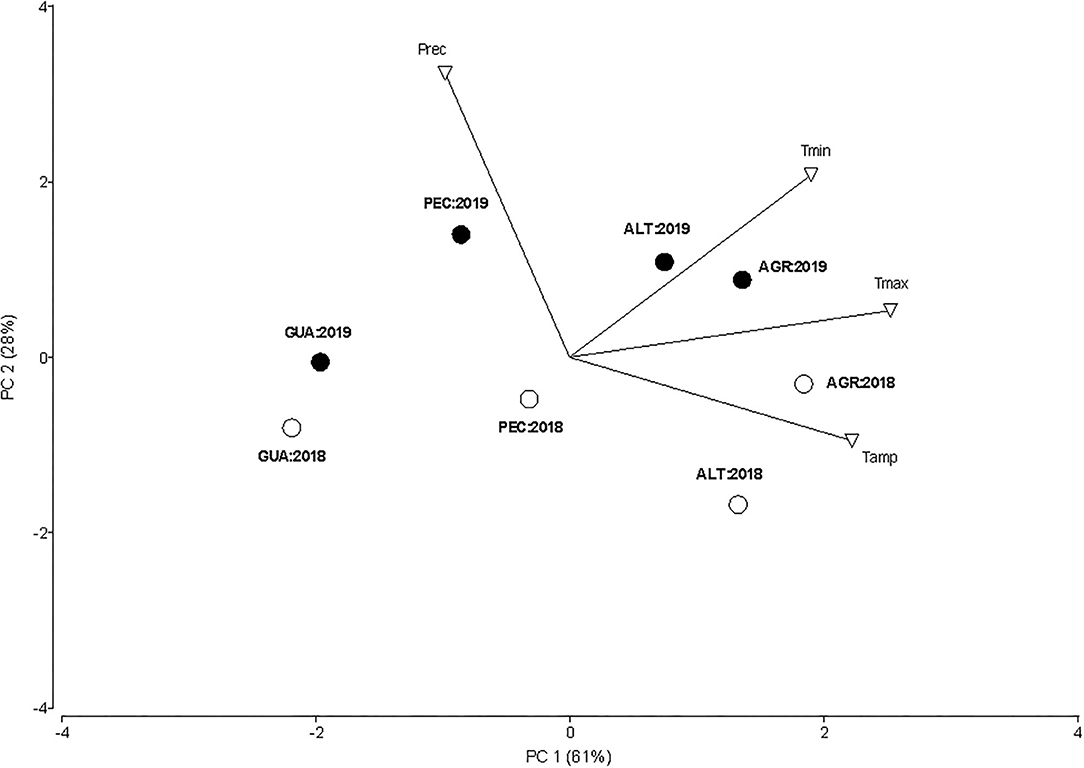
Figure 2. PCA of minimum and maximum daily air temperatures, thermal amplitude and precipitation measured in the AGR, PEC, ALT and GUA vineyards during the 2018 and 2019 growing seasons (September–April). daily maximum (Tmax; °C), minimum (Tmin), thermal amplitude (Tamp) and precipitation (Prec).
Fruit Yield
All the variables measured were affected by E, with significant G × E interactions for yield and the number of clusters per plant during the 2018 season (Figure 3A). The fruit yield in 2018 was higher in AGR, with differences between G (3 kg plant−1 for Clone 7 and 4 kg plant−1 for Mount Eden), and it was lower for PEC, ALT and GUA, respectively (significant G × E interaction; Figure 3A). The number of clusters per plant for 2018 was affected by E and G × E interaction, being higher in AGR for Mount Eden (Figure 3B). For the 2019 growing season, the AGR vineyard was affected by a hail storm that affected the plants canopy near the harvest date. In AGR, the yield (weight of the clusters and number of clusters per plant) was reduced for both G in 2019 (Figures 3A,B).
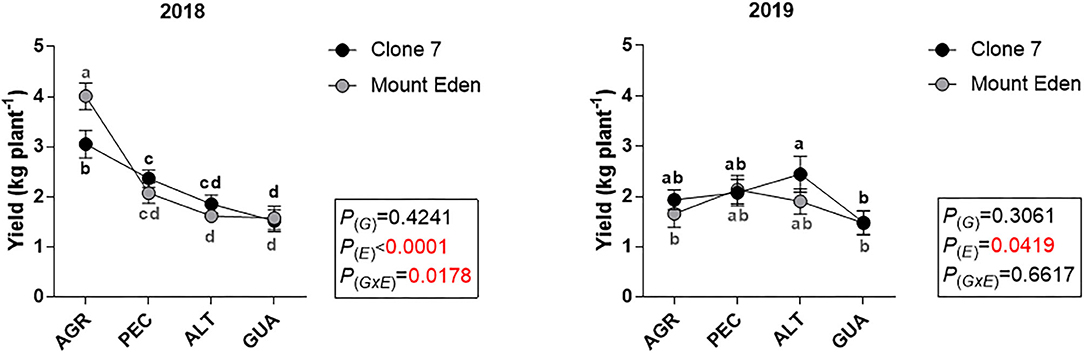
Figure 3. Fruit yield indicators, measured during 2018 and 2019 growing season for two Cabernet Sauvignon clones (Clone 7 and Mount Eden) in four vineyards (AGR, PEC, ALT and GUA). Fruit yield (A), number of clusters per plant (B), Berry fresh weight (C) and berry skin dry weight (D). Values are means (n = 9) and different letters indicate statistically significant differences (LSD Fisher; P ≤ 0.05). P(E), P(G) and P(GxE): effects of environment, genotype and their interaction, respectively. For the 2019 growing season, the AGR vineyard was affected by a hail storm that affected the plants canopy near the harvest date. Supplementary data in Supplementary Tables 2–4.
Significant G × E interactions were found for berries fresh weight in both seasons (Figure 3C). During 2018, the dry weight of the berry skin increased in GUA for both genotypes and more markedly for Clone 7 [P(G × E) = 0.0935; Figure 3D].
PCs Characterization of Wines
The anthocyanins detected were the same in all GIs, regardless of the year and genotype and their concentrations were mainly affected by E (Table 3). The predominant anthocyanin was malvidin-3-O-glucoside (386 mg L−1) for all GIs, followed by peonidin-3-O-glucoside (250 mg L−1) and petunidine-3-O-glucoside (187 mg L−1). These maximum concentrations belong to GUA for both G. Di-hydroxylated (cyanidin and peonidin) and tri-hydroxylated (delphinidin, petunidin and malvidin) anthocyanins were higher in GUA with 873 mg L−1 and decreased to 756, 765 and 300 mg L−1 for ALT, PEC and AGR, respectively (Table 2).
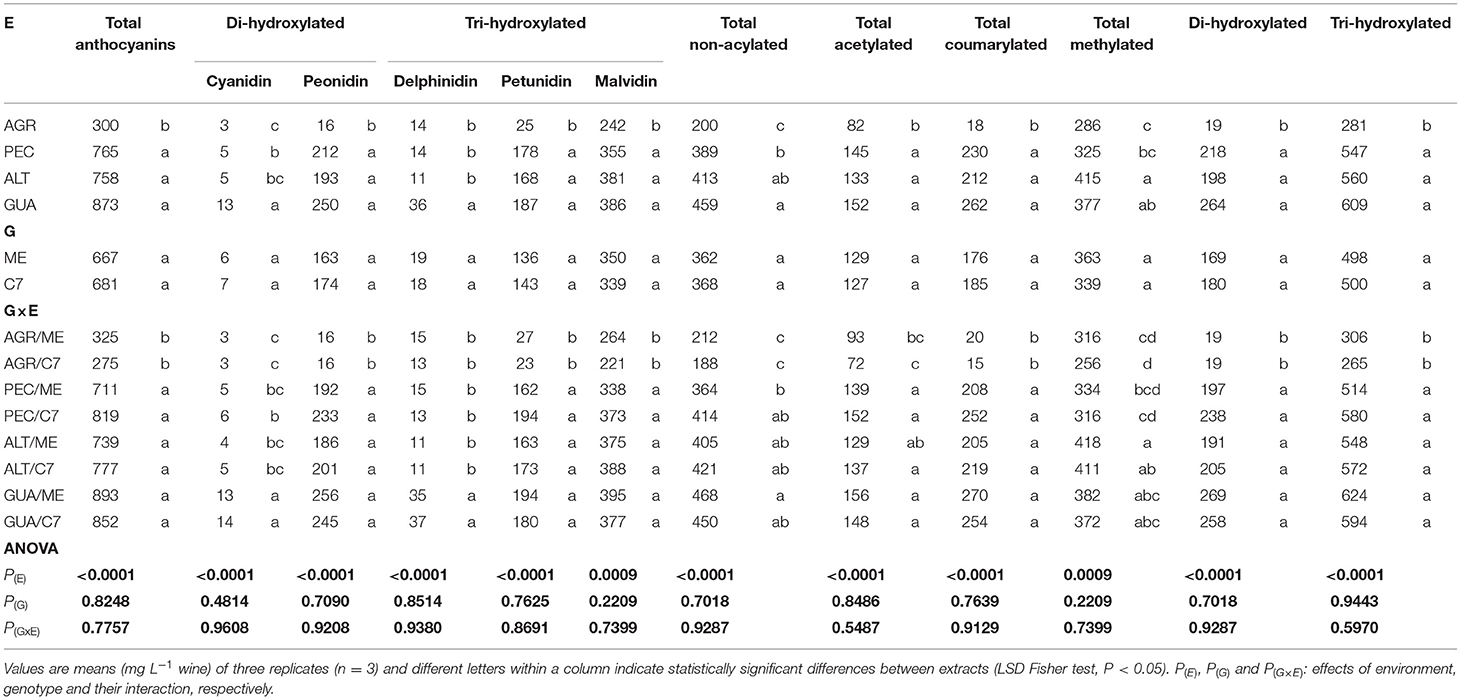
Table 2. Levels of anthocyanin PCs in wines of Cabernet Sauvignon Clone 7 (C7) and Mount Eden (ME) from four vineyards (AGR, PEC, ALT and GUA).
For non-acylated anthocyanins, the GUA interact with Mount Eden and increased the total concentration with respect to the other interactions (468 mg L−1; Table 2). AGR showed low concentration of total acetylated (82 mg L−1) and total coumarylated (17 mg L−1) anthocyanins, irrespectively of the G (Table 2). Methylated anthocyanins increased in ALT (415 mg L−1), especially for ME plant material.
The PCA with anthocyanins shows that PC1 explain 84.9% of the variance and PC2 10.6% (Figure 4). Also, that the main differences for anthocyanin concentration are between AGR and GUA, being the concentrations higher for GUA (AGR was associated with tri/di-hydroxylated anthocyanins ratio). It can be also observed from Table 2 that both ALT and PEC have similarities in the anthocyanin profile as peonidin-3-O-glucoside (193 mg L−1 for ALT and 212 mg L−1 for PEC), petunidin-3-O-glucoside (168 mg L−1 for ALT and 178 mg L−1 for PEC) and cyanidin-3-O-glucoside (5 mg L−1 for ALT and 5 mg L−1 for PEC).
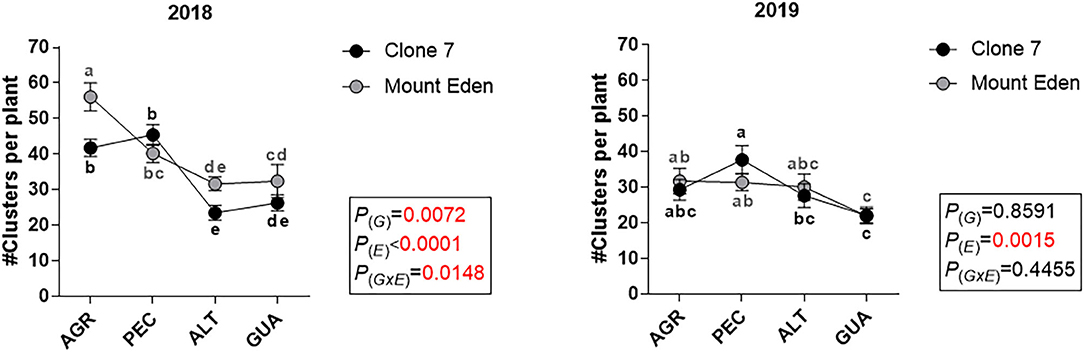
Figure 4. PCA of main anthocyanins in Cabernet Sauvignon Clone 7 (C7) and Mount Eden (ME) wines, from vineyards in AGR, PEC, ALT and GUA.
Tables 3, 4 shows the non-anthocyanins phenolic profiles of the wines and that the most abundant compounds were gallic acid, caftaric acid and epigallocatechin gallate with concentrations of 22, 7 and 22 mg L−1, respectively. These compounds were significantly higher in AGR. The highest accumulation of total non-anthocyanins was found for AGR/Clone 7 [P(G × E) = 0.3536], mainly through the effect of procyanidins (significant G × E interaction). Gallic acid, caffeic acid, epigallocatechin gallate and tyrosol stood out in the AGR/ME interaction at concentrations of 25, 8, 24 and 70 mg L−1, respectively.
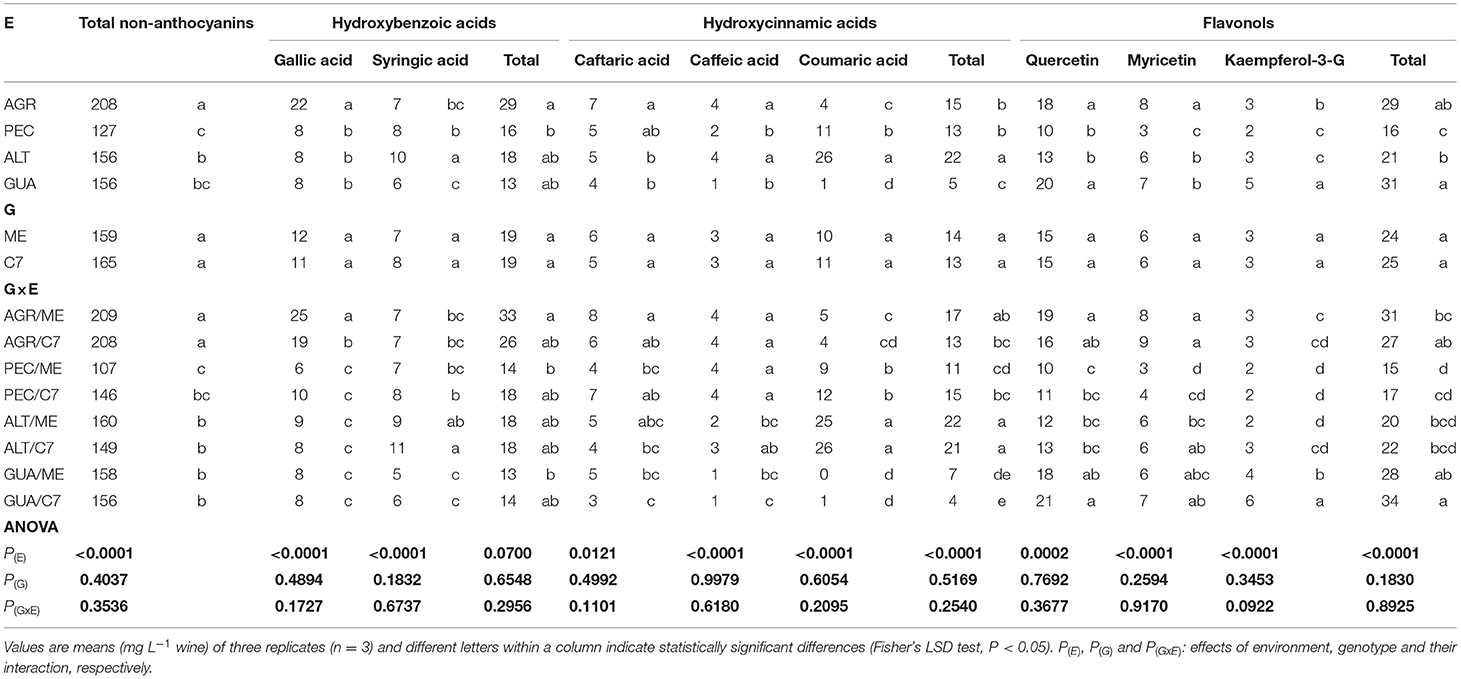
Table 3. Levels of non-anthocyanin PCs in wines of Cabernet Sauvignon Clone 7 (C7) and Mount Eden (ME) from four vineyards (AGR, PEC, ALT and GUA).
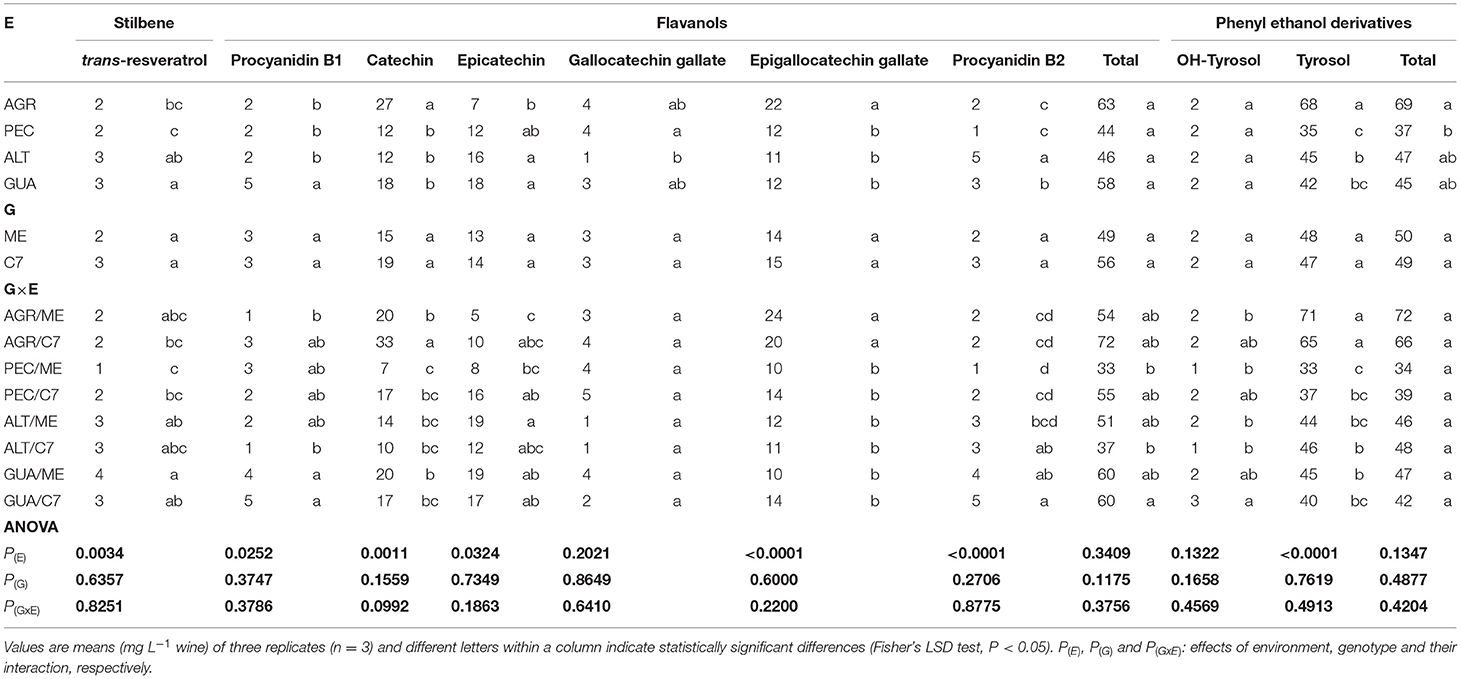
Table 4. Levels of non-anthocyanin PCs in wines of Cabernet Sauvignon Clone 7 (C7) and Mount Eden (ME) from four vineyards (AGR, PEC, ALT and GUA).
The stilbene trans-resveratrol increased markedly in GUA (3 mg L−1), followed by ALT (2 mg L−1). The trans-resveratrol levels in GUA were between 32 and 55% higher than those of the other environments. The GUA/C7 interaction stood out from the other G × E with a concentration of 3 mg L−1. In comparison with the other GIs, the flavanol quercetin was also found in higher concentration in GUA (20 mg L−1).
The PCA for non-anthocyanins PCs (Figure 5) explains 74.80 % of the data variability, where there is a differentiation of non-anthocyanin compounds based on G × E interactions. GUA grouped together trans-resveratrol (3 mg L−1), quercetin (20 mg L−1), procyanidin B1 (5 mg L−1). For AGR the associated compounds were epigallocatechin gallate (22 mg L−1), caftaric acid (7 mg L−1) and tyrosol (67 mg L−1), among others. With respect to C7 in ALT and PEC, there are some common compounds such as p-coumaric acid (25 mg L−1 for ALT and 11 mg L−1 for PEC) and syringic acid (11 mg L−1 for ALT and 8 mg L−1 for PEC).
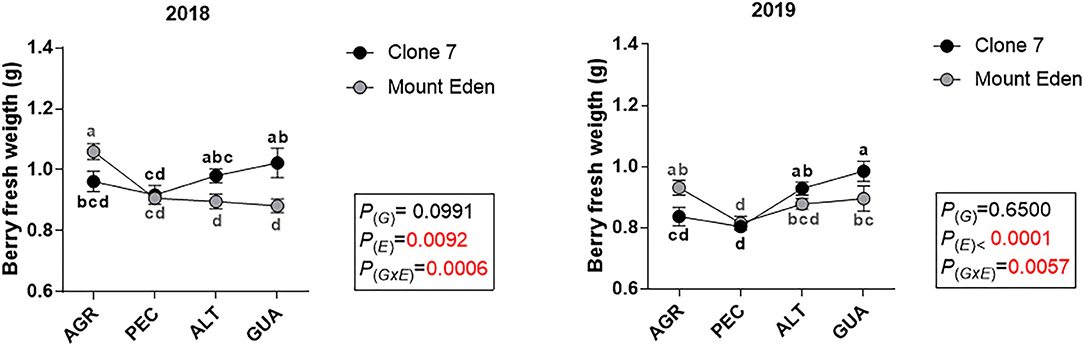
Figure 5. PCA of non-anthocyanin compounds in Cabernet Sauvignon Clone 7 (C7) and Mount Eden (ME) wines, from vineyards in AGR, PEC, ALT and GUA.
Discussion
Mendoza has a temperate arid continental climate with scarce rainfall (200–300 mm per year), so it is necessary to irrigate for the normal growth and development of the vines. Also, the vineyards are located in an altitudinal gradient that markedly modify the environmental conditions. The year of implantation and density of the Cabernet Sauvignon Clone 7 and Mount Eden at AGR and GUA are the same, but the parcels are located at different altitudes (see Table 1). Between the two parcels, there is a linear distance of 41 km and an altitude difference of 400 m, maximizing the differences in environmental conditions. There are differences in the soil types, being sandy loam with clay at 0.3–0.6 m depth in AGR and sandy in GUA.
Also, higher levels of ultraviolet-B (UV-B) radiation are registered in GUA in relation with AGR (Berli et al., 2010), with lower average air temperature (Varela et al., 2021). Previous data support that a lower temperature tends to increase color in red wines, while warmer temperatures during the day and night tend to reduce the content of anthocyanins (Drappier et al., 2019). Additionally, other research has shown that lower nighttime temperatures do not reverse the effects of higher daytime temperatures. Optimal anthocyanin accumulation occurs when grapes are exposed to cool nights (15°C) and moderate daytime temperatures (25°C) during ripening (Schultz, 2000). Low anthocyanin concentrations were correlated with warmer regions, which is climatically consistent with some studies revealing that high temperatures decreased the total anthocyanin content in Cabernet Sauvignon berries by 50% (Mori et al., 2007). These results agree with our results where the low levels of anthocyanins were associated to the lower altitude AGR. GUA presented nearly three times higher levels of total anthocyanins than AGR.
The classification of anthocyanins used is based on their biosynthetic pathway, where tri-hydroxylated anthocyanins derived from flavonoid 3′,5′-hydroxylase (F3′5′H), i.e., delphinidin-, petunidin- and malvidin-derived. Whereas, di-hydroxylated anthocyanins derived from flavonoid 3′-hydroxylase (F3′H), being cyanidin- and peonidin-derived (Castellarin et al., 2007). Within this classification criteria, we can separate methylated anthocyanins (malvidin-, petunidin- and peonidin-derived) from non-methylated anthocyanins (delphinidin- and cyanidin-derived). Also, we can group the anthocyanins by acylation as non-acylated, acetylated or coumarylated.
Although the anthocyanin profile is primarily a genetic characteristic unique to each grapevine cultivar, we previously found that stressful environmental conditions as high UV-B levels reduced the proportion of tri-hydroxylated anthocyanins, as acclimation shift in berry skin anthocyanin composition (Berli et al., 2011; Alonso et al., 2016), possibly since the most oxidized anthocyanins are less antioxidant (Wang et al., 1997).
Considering the different UV-B irradiance at different altitudes for several of the studied GIs, particularly those of the Uco Valley, this is an additional factor related to the differential composition of PCs that is observed in the present study. In China, a similar behavior was found with respect to PCs for Cabernet Sauvignon wines made with grapes grown at high altitudes and that had large differences between day and night temperatures (Li et al., 2011). In Argentina, Urvieta et al. (2021) also highlighted the importance of these “terroir” related conditions (elevation and temperatures of the places where vineyards are located) on the PCs composition of Malbec variety. Particularly, anthocyanins and quercetin showed high levels in GUA, in correspondence with the present work.
In relation to the anthocyanins of wines, higher differences between AGR and GUA were also found, while ALT and PEC showed similarities, particularly for petunidin, malvidin and methylated derivatives.
Particularly for the di-hydroxylated anthocyanins, they increased in the GI of high altitude and colder temperatures, in agreement with previous works for other varieties (Yamane et al., 2006; Berli et al., 2011; Gil et al., 2013; Alonso et al., 2016). Two effects could be associated to these results observed for the Uco Valley GIs. Previous research showed that anthocyanin accumulation in grapes depends on both, low temperatures and light (Azuma et al., 2012). The high altitude climatic characteristics could be responsible of the stimulation of the increased carbon flow to the F3′H branch pathway (Li et al., 2011). Therefore, cyanidin-derived (di-hydroxylated) anthocyanins might increase in the berries of GIs located at the high altitudes of Uco Valley, and related to the high levels of those compounds in GUA elaborated wines. We previously found that the high solar UV-B irradiances registered in GUA increased total anthocyanins and the relative abundance of di-hydroxylated anthocyanins (i.e., reducing the proportion of tri-hydroxylated relatives) in Malbec berry skins (Berli et al., 2011; Alonso et al., 2016). On the other side, AGR wines showed a lower concentration of anthocyanins, and associated with a higher ratio of tri/di-hydroxylated anthocyanins, that is, the proportion of tri-substituted anthocyanins (particularly malvidin derivatives) was more abundant than their di-substituted counterparts. These results are in agreement with previous reports in Sangiovese grapes where the effects of two different temperature regimes on the accumulation of mRNAs and enzymes controlling berry skin anthocyanins was evaluated (Movahed et al., 2016). They observed an anthocyanins biosynthesis reduction at both, transcriptional and enzymatic levels. Besides, the peroxidase activity was higher in berries ripened under high temperatures (36°C), a similar condition as AGR. In fact, the low anthocyanin levels were the result of a combined reduction of biosynthesis and an increase of their degradation by the direct action of peroxidases (Movahed et al., 2016). This may contribute to the contrasting results observed between AGR and GUA. The PEC and ALT GIs presented similar concentrations of individual anthocyanins which could be related to the geographical proximity and very similar climatic condition i.e., both GIs are located at similar altitudes (1073–1085 m a.s.l.).
ALT had the highest Tamp during the 2018 and 2019 seasons. The effects on berry PCs were low (data not shown), but after analyzing the wines, ALT increased the total anthocyanin content, particularly derivatives of malvidin, syringic acid and trans-resveratrol. These data are in agreement with the results observed for the other high-altitude GIs.
Among the non-anthocyanins, the ALT and PEC wines did not show significant differences, denoting the syringic and p-coumaric acids as the most representative compounds. AGR had higher amounts of caftaric and gallic acids, while GUA had higher amounts of quercetin, trans-resveratrol, OH-tyrosol and tannin derivatives. The concentration of these particular non-anthocyanins PCs depends on the elevation of vineyards, because at higher altitudes GIs, such as GUA, the levels of UV-B radiation increase and the average temperatures decrease. This stressful condition for plants stimulates the synthesis of high antioxidant compounds such as those found in GUA at higher concentrations. These compounds were those responsible of the separation of this GI from others in Figure 5. The increased synthesis and accumulation of these high antioxidant PCs has been also previously correlated with the increase of altitude of vineyards in different regions of the world (Tao et al., 2009; Jin et al., 2017; Urvieta et al., 2018, 2021).
In the anthocyanin profile of wines, the relative amounts of di-hydroxylated and tri-hydroxylated anthocyanins increased in GUA in comparison to the other studied GIs, possibly due to GUA lower air temperatures and higher solar UV-B levels (Berli et al., 2010). In addition, trans-resveratrol, an antioxidant compound known to increase with UV-B exposure in plants located at high altitudes (Haselgrove et al., 2000; Berli et al., 2008), increased significantly in GUA wines when compared to other GIs. Another compound that increased in this GI was quercetin, in correspondence with previous reports for Malbec leaves (Berli et al., 2010), berries (Berli et al., 2008, 2011) and wines (Urvieta et al., 2018, 2021). Price et al. (1995), determined that the concentration of flavonols such as quercetin-3-glucoside can play a fundamental role in co-pigmentation, helping to stabilize the color of the wine. Previous research showed that solar irradiation, water deficits, and higher temperature differences between day and night could positively regulate gene expression related to metabolism of flavonoids and, therefore, significantly increase the flavonoid content (Gollop et al., 2002; Yamane et al., 2006).
The presented and discussed results indicate that the fruit yields (number of clusters per plant) and the phenolic composition of wines are influenced by environment, plant material and their interaction. The environment has a direct relation with the climatic conditions in the studied GIs, highlighting the higher concentration of some specific PCs or families at lower temperatures and higher altitude GIs. The results suggest the possibility of identifying Cabernet Sauvignon wines of different origins of Mendoza that are associated with different plant material. The data presented is of interest for the wine industry because is the first time that Cabernet Sauvignon wines from different GIs of Argentina are chemically characterized. As well, to our knowledge this is the first comparison of the Clone 7 and Mount Eden plant material. The results contribute to characterize the terroir of the regions and to take oenological decisions by the selection of vegetative material, expanding also the current knowledge of Cabernet Sauvignon wines, and its geographical origin.
Data Availability Statement
The original contributions presented in the study are included in the article/Supplementary Material, further inquiries can be directed to the corresponding author/s.
Author Contributions
FM: conceptualization, methodology, investigation, formal analysis, data curation, writing—original draft, and writing—review and editing. RU: conceptualization, methodology, investigation, and writing—review and editing. FB: investigation and writing—review and editing. MR: investigation, methodology, investigation, formal analysis, and writing—review and editing. AF and FB: conceptualization, methodology, formal analysis, investigation, resources, data curation, visualization, supervision, project administration, funding acquisition, and writing—review and editing. All authors contributed to the article and approved the submitted version.
Funding
This work was supported by National Agency for Scientific and Technological Promotion (ANPCyT), Argentina (Grant Number: Banco Interamericano de Desarrollo (BID)-Proyectos de Investigación Científica y Tecnológica (PICT) N° 2016-0486), Secretaría de Investigación, Internacionales y Posgrado from Universidad Nacional de Cuyo (SIIP-UNCuyo, Grant Number: A688) and Catena Zapata Winery. FM is a scholar of CONICET. RU and FB are researchers at CIW. AF and FB are fellows of CONICET.
Conflict of Interest
The authors declare that the research was conducted in the absence of any commercial or financial relationships that could be construed as a potential conflict of interest.
Supplementary Material
The Supplementary Material for this article can be found online at: https://www.frontiersin.org/articles/10.3389/fsufs.2021.700642/full#supplementary-material
References
Aleixandre, J. L., Aleixandre-Tud,ó, J. L., Bolaños-Pizarro, M., and Aleixandre-Benavent, R. (2015). Global trends in scientific production in enology and viticulture in selected emerging economies (BRIC). Scientometrics 103, 649–668. doi: 10.1007/s11192-015-1543-4
Alonso, R., Berli, F. J., Fontana, A., Piccoli, P., and Bottini, R. (2016). Malbec grape (Vitis vinifera L.) responses to the environment: berry phenolics as influenced by solar UV-B, water deficit and sprayed abscisic acid. Plant Physiol. Biochem. 109, 84–90. doi: 10.1016/j.plaphy.2016.09.007
Anesi, A., Stocchero, M., Dal Santo, S., Commisso, M., Zenoni, S., Ceoldo, S., et al. (2015). Towards a scientific interpretation of the terroir concept: plasticity of the grape berry metabolome. BMC Plant Biol. 15, 1–17. doi: 10.1186/s12870-015-0584-4
Antoniolli, A., Fontana, A. R., Piccoli, P., and Bottini, R. (2015). Characterization of polyphenols and evaluation of antioxidant capacity in grape pomace of the cv. Malbec. Food Chem. 178, 172–178. doi: 10.1016/j.foodchem.2015.01.082
Azuma, A., Yakushiji, H., Koshita, Y., and Kobayashi, S. (2012). Flavonoid biosynthesis-related genes in grape skin are differentially regulated by temperature and light conditions. Planta 236, 1067–1080. doi: 10.1007/s00425-012-1650-x
Berli, F., D'Angelo, J., Cavagnaro, B., Bottini, R., Wuilloud, R., and Silva, M. F. (2008). Phenolic composition in grape (Vitis vinifera L. cv. Malbec) ripened with different solar UV-B radiation levels by capillary zone electrophoresis. J. Agric. Food Chem. 56, 2892–2898. doi: 10.1021/jf073421
Berli, F., Moreno, D., Piccoli, P., Hespanhol-Viana, L., Silva, M. F., Bressan-Smith, R., et al. (2010). Abscisic acid is involved in the response of grape (Vitis vinifera L.) cv. Malbec leaf tissues to ultraviolet-B radiation by enhancing ultraviolet-absorbing compounds, antioxidant enzymes and membrane sterols. Plant Cell Environ. 33, 1–10. doi: 10.1111/j.1365-3040.2009.02044.x
Berli, F. J., Fanzone, M., Piccoli, P., and Bottini, R. (2011). Solar UV-B and ABA are involved in phenol metabolism of Vitis vinifera L. increasing biosynthesis of berry skin polyphenols. J. Agric. Food Chem. 59, 4874–4884. doi: 10.1021/jf200040z
Caldwell, J. (2002). A Concise Guide to Grapevine Clones for Professionals, 2° Edition. Davis, CA: John Caldewell Viticultural Services.
Castellarin, S. D., Pfeiffer, A., Sivilotti, P., Degan, M., Peterlunger, E., and Di Gaspero, G. (2007). Transcriptional regulation of anthocyanin biosynthesis in ripening fruits of grapevine under seasonal water deficit. Plant Cell Environ. 30, 1381–1399. doi: 10.1111/j.1365-3040.2007.01716.x
Cross, R., Plantinga, A. J., and Stavins, R. N. (2011). What is the value of terroir? Am. Econ. Rev. 101, 152–156. doi: 10.1257/aer.101.3.152
Dal Santo, S., Fasoli, M., Negri, S., D'Inc,à, E., Vicenzi, N., Guzzo, F., et al. (2016). Plasticity of the berry ripening program in a white grape variety. Front. Plant Sci. 7:970. doi: 10.3389/fpls.2016.00970
Di Paola-Naranjo, R. D., Baroni, M. V., Podio, N. S., Rubinstein, H. R., Fabani, M. P., Badini, R. G., et al. (2011). Fingerprints for main varieties of Argentinean wines: terroir differentiation by inorganic, organic, and stable isotopic analyses coupled to chemometrics. J. Agric. Food Chem. 59, 7854–7865. doi: 10.1021/jf2007419
Drappier, J., Thibon, C., Rabot, A., and Geny-Denis, L. (2019). Relationship between wine composition and temperature: impact on Bordeaux wine typicity in the context of global warming. Crit. Rev. Food Sci. Nutr. 59, 14–30. doi: 10.1080/10408398.2017.1355776
Ferreyra, S., Torres-Palazzolo, C., Bottini, R., Camargo, A., and Fontana, A. (2021). Assessment of in-vitro bioaccessibility and antioxidant capacity of phenolic compounds extracts recovered from grapevine bunch stem and cane by-products. Food Chem. 348:129063. doi: 10.1016/j.foodchem.2021.129063
Foundation Plant Services Grape (2011). Cabernet Sauvignon/Mount Eden 2011. Available online at: https://fps.ucdavis.edu/fgrdetails.cfm?varietyid=356 [Consultado el 23 de abril de 2021].
Gianoli, E., and Valladares, F. (2012). Studying phenotypic plasticity: the advantages of a broad approach. Biol. J. Linn. Soc. 105, 1–7. doi: 10.1111/j.1095-8312.2011.01793.x
Gil, M., Bottini, R., Berli, F., Pontin, M., Silva, M. F., and Piccoli, P. (2013). Volatile organic compounds characterized from grapevine (Vitis vinifera L. cv. Malbec) berries increase at pre-harvest and in response to UV-B radiation. Phytochemistry 96, 148–157. doi: 10.1016/j.phytochem.2013.08.011
Gollop, R., Even, S., Colova-Tsolova, V., and Perl, A. (2002). Expression of the grape dihydroflavonol reductase gene and analysis of its promoter region. J. Exp. Bot. 53, 1397–1409. doi: 10.1093/jexbot/53.373.1397
Haselgrove, L., Botting, D., Van Heeswijck, R., Høj, P. B., Dry, P. R., Ford, C., et al. (2000). Canopy microclimate and berry composition: the effect of bunch exposure on the phenolic composition of Vitis vinifera L. cv. Shiraz grape berries. Aust. J. Grape Wine Res. 6, 141–149. doi: 10.1111/j.1755-0238.2000.tb00173.x
Instituto Nacional de Vitivinicultura (2019). Informe anual de superficie 2019. Available online at https://www.argentina.gob.ar/inv/vinos/estadisticas/superficie/anuarios [Consultado el 10 de febrero de 2021].
Jaffré, J., Valentin, D., Dacremont, C., and Peyron, D. (2009). Burgundy red wines: Representation of potential for aging. Food Qual. Pref. 20, 505–513. doi: 10.1016/j.foodqual.2009.05.001
Jiang, B., and Sun, Z. Y. (2019). Phenolic compounds, total antioxidant capacity and volatile components of Cabernet Sauvignon red wines from five different wine-producing regions in China. Food Sci. Technol. 39, 735–746. doi: 10.1590/fst.07818
Jin, X. D., Wu, X., and Liu, X. (2017). Phenolic characteristics and antioxidant activity of Merlot and Cabernet Sauvignon wines increase with vineyard altitude in a high-altitude region. South Afr. J. Enol. Viticult. 38, 132–143. doi: 10.21548/38-2-1068
Keller, M. (2010). Managing grapevines to optimise fruit development in a challenging environment: a climate change primer for viticulturists. Aust. J. Grape Wine Res. 16, 56–69. doi: 10.1111/j.1755-0238.2009.00077.x
Li, Z., Pan, Q., Jin, Z., Mu, L., and Duan, C. (2011). Comparison on phenolic compounds in Vitis vinifera cv. Cabernet Sauvignon wines from five wine-growing regions in China. Food Chem. 125, 77–83. doi: 10.1016/j.foodchem.2010.08.039
Marlowe, B., and Lee, S. (2018). Conceptualizing terroir wine tourism. Tour. Rev. Int. 22, 143–151. doi: 10.3727/154427218X15319286372298
Mori, K., Goto-Yamamoto, N., Kitayama, M., and Hashizume, K. (2007). Loss of anthocyanins in red-wine grape under high temperature. J. Exp. Bot. 58, 1935–1945. doi: 10.1093/jxb/erm055
Movahed, N., Pastore, C., Cellini, A., Allegro, G., Valentini, G., Zenoni, S., et al. (2016). The grapevine VviPrx31 peroxidase as a candidate gene involved in anthocyanin degradation in ripening berries under high temperature. J. Plant Res. 129, 513–526. doi: 10.1007/s10265-016-0786-3
Nicotra, A. B., and Davidson, A. (2010). Adaptive phenotypic plasticity and plant water use. Func. Plant Biol. 37, 117–127. doi: 10.1071/FP09139
Organización Internacional de la Viña y el Vino (2010). Definición de “Terroir” vitivinicola. Available online at: https://www.oiv.int/public/medias/380/viti-2010-1-es.pdf [Consultado el 28 de mayo de 2021].
Pajović, R., Wendelin, S., Forneck, A., and Eder, R. (2014). Varietal differentiation of grapes cv. ‘Vranac', ‘Kratošija' and ‘Cabernet Sauvignon' from Montenegro according to their polyphenolic composition. Mitt. Klosterneuburg 64, 9–19. Available online at: https://www.cabdirect.org/cabdirect/abstract/20143271766
Pigliucci, M. (2005). Evolution of phenotypic plasticity: where are we going now? Trends Ecol. Evol. 20, 481–486. doi: 10.1016/j.tree.2005.06.001
Price, S. F., Breen, P. J., Valladao, M., and Watson, B. T. (1995). Cluster sun exposure and quercetin in Pinot noir grapes and wine. Am. J. Enol. Vitic. 46, 187–194.
Ranaweera, R. K., Gilmore, A. M., Capone, D. L., Bastian, S. E., and Jeffery, D. W. (2021). Authentication of the geographical origin of Australian Cabernet Sauvignon wines using spectrofluorometric and multi-element analyses with multivariate statistical modelling. Food Chem. 335:127592. doi: 10.1016/j.foodchem.2020.127592
Schultz, H. (2000). Climate change and viticulture: a European perspective on climatology, carbon dioxide and UV-B effects. Aust. J. Grape Wine Res. 6, 2–12. doi: 10.1111/j.1755-0238.2000.tb00156.x
Schultz, H. R. (2003). Differences in hydraulic architecture account for near-isohydric and anisohydric behaviour of two field-grown Vitis vinifera L. cultivars during drought. Plant Cell Environ. 26, 1393–1405. doi: 10.1046/j.1365-3040.2003.01064.x
Senyard, J., Powell, E. E., Baker, T., Steffens, P., and Davidsson, P. (2011). “Stop whining and make the best of it: a cross-national comparison of response to regional disadvantage in the wine industry,” in Babson College Entrepreneurial Research Conference.
Tao, Y. S., Liu, Y. Q., and Li, H. (2009). Sensory characters of Cabernet Sauvignon dry red wine from Changli County (China). Food Chem. 114, 565–569. doi: 10.1016/j.foodchem.2008.09.087
Urvieta, R., Buscema, F., Bottini, R., Coste, B., and Fontana, A. (2018). Phenolic and sensory profiles discriminate geographical indications for Malbec wines from different regions of Mendoza, Argentina. Food Chem. 265, 120–127. doi: 10.1016/j.foodchem.2018.05.083
Urvieta, R., Jones, G., Buscema, F., Bottini, R., and Fontana, A. (2021). Terroir and vintage discrimination of Malbec wines based on phenolic composition across multiple sites in Mendoza, Argentina. Sci. Rep. 11, 1–13. doi: 10.1038/s41598-021-82306-0
Van Kleunen, M., and Fischer, M. (2005). Constraints on the evolution of adaptive phenotypic plasticity in plants. New Phytol. 166, 49–60. doi: 10.1111/j.1469-8137.2004.01296.x
Van Leeuwen, C., and Seguin, G. (2006). The concept of terroir in viticulture. J. Wine Res. 17, 1–10. doi: 10.1080/09571260600633135
Varela, A., Ibañez, V. N., Alonso, R., Zavallo, D., Asurmendi, S., Talquenca, S. G., et al. (2021). Vineyard environments influence Malbec grapevine phenotypic traits and DNA methylation patterns in a clone-dependent way. Plant Cell Rep. 40, 111–125. doi: 10.1007/s00299-020-02617-w
Versari, A., Laurie, V. F., Ricci, A., Laghi, L., and Parpinello, G. P. (2014). Progress in authentication, typification and traceability of grapes and wines by chemometric approaches. Food Res. Int. 60, 2–18. doi: 10.1016/j.foodres.2014.02.007
Wang, H., Cao, G., and Prior, R. L. (1997). Oxygen radical absorbing capacity of anthocyanins. J. Agric. Food Chem. 45, 304–309. doi: 10.1021/jf960421t
Weiner, J. (2004). Allocation, plasticity and allometry in plants. Perspect. Plant Ecol. Evol. Syst. 6, 207–215. doi: 10.1078/1433-8319-00083
Yamane, T., Jeong, S. T., Goto-Yamamoto, N., Koshita, Y., and Kobayashi, S. (2006). Effects of temperature on anthocyanin biosynthesis in grape berry skins. Am. J. Enol. Vitic. 57, 54–59. Available online at: http://www.ajevonline.org/content/57/1/54.abstract
Keywords: classification, geographical discrimination, grapevine, phenolic compounds, phenotypic plasticity, terroir
Citation: Muñoz F, Urvieta R, Buscema F, Rasse M, Fontana A and Berli F (2021) Phenolic Characterization of Cabernet Sauvignon Wines From Different Geographical Indications of Mendoza, Argentina: Effects of Plant Material and Environment. Front. Sustain. Food Syst. 5:700642. doi: 10.3389/fsufs.2021.700642
Received: 26 April 2021; Accepted: 23 June 2021;
Published: 20 July 2021.
Edited by:
Micha Horacek, HBLFA Francisco Josephinum, BLT Wieselburg, AustriaReviewed by:
Reinhard Eder, Höhere Bundeslehranstalt und Bundesamt für Wein- und Obstbau, AustriaMaria V. Baroni, Universidad Nacional de Córdoba, Argentina
Mariona H. Gil i Cortiella, Autonomous University of Chile, Chile
Copyright © 2021 Muñoz, Urvieta, Buscema, Rasse, Fontana and Berli. This is an open-access article distributed under the terms of the Creative Commons Attribution License (CC BY). The use, distribution or reproduction in other forums is permitted, provided the original author(s) and the copyright owner(s) are credited and that the original publication in this journal is cited, in accordance with accepted academic practice. No use, distribution or reproduction is permitted which does not comply with these terms.
*Correspondence: Ariel Fontana, YWZvbnRhbmFAbWVuZG96YS1jb25pY2V0LmdvYi5hcg==; Federico Berli, ZmJlcmxpQGZjYS51bmN1LmVkdS5hcg==
†These authors share senior authorship